- 1Facultad de Biología, Universidad Michoacana de San Nicolás de Hidalgo, Morelia, Mexico
- 2Instituto de Investigaciones sobre los Recursos Naturales, Universidad Michoacana de San Nicolás de Hidalgo, Morelia, Mexico
To recover the structure and functionality of a deforested ecosystem, two strategies of ecological restoration are considered: active restoration, which eliminates the disturbance agents and implements strategies to accelerate site recovery, and passive restoration, which eliminates disturbance agents, allowing natural regeneration to occur. Prior to choosing passive restoration, a field evaluation of the potential for natural regeneration is important. In this context, seedling and sapling density as well as patterns of recruitment and survival are appropriate indicators of restoration potential. In the present study, we deduced the potential of sacred fir (Abies religiosa) forest of the Monarch Butterfly Biosphere Reserve to recover by natural regeneration through seedling and sapling density and mortality, since A. religiosa is the dominant tree species in wintering sites of monarch butterfly. In 2015, we evaluated seedling density in 53 sites along an elevational gradient (3050–3550 m above sea level; m a.s.l.). There was a higher density of seedlings and saplings established in canopy gaps, compared to sites under dense forest canopy. Seedling recruitment was higher in sites at intermediate elevations (3050 to 3300 m a.s.l.) than in those at higher elevations. In a second survey, we studied A. religiosa seedling mortality over the dry season of 2016 to identify the environmental variables that cause the high seedling mortality and very low recruitment. Recently emerged seedling mortality was 49.2% at the end of the dry season (June 2016). The highest monthly mortality (14.3%) was recorded in April, a dry and warm month with the lowest values of moss thickness and soil moisture. We found no negative effects of moss layer on seedling mortality; indeed, moss appears to slow soil moisture reduction at the critical end of the warm and dry season. Soil and moss moisture values in April seem to be a critical factor for A. religiosa seedling recruitment, and we expect this condition will deteriorate under projected climatic change scenarios. Thus, the potential of MBBR A. religiosa forest to recover by passive restoration is highly constrained and will require management actions to achieve successful restoration outcomes.
Introduction
The sacred fir (Abies religiosa) is a conifer native to Mexico. It distributes in the mountainous areas (2100 to 3600 m a.s.l.) in central Mexico, presenting monospecific forests between 3000 and 3300 m a.s.l. (Rzedowski, 2006). These forests occur in locations that present very specific geographical, climatic and ecological conditions (Pineda-López et al., 2013), particularly on steep, humid and shaded slopes. One of the most emblematic Abies religiosa forests in Mexico is found in the Monarch Butterfly Biosphere Reserve (MBBR), which acts as refuge and habitat for the monarch butterfly (Danaus plexipus L.) that evades the winter conditions of Canada and the United States by annually migrating south to a few mountainsides in central Mexico (Honey-Rosés et al., 2018). The MBBR encompasses 56,259 ha and was designated as a UNESCO World Heritage Site.
Currently, the Reserve is threatened by various political, social and economic issues that lead to environmental degradation associated with logging activities, expansion of the agricultural frontier and overexploitation and inadequate management of natural resources. This is despite the fact that the Monarch Butterfly Reserve receives a considerable amount of financial resources from national and international organizations to carry out reforestation programs (Honey-Rosés et al., 2011). Between 2002 and 2010, the region received United States $9.2 million for reforestation programs (SEMARNAT, 2011).
To recover the structure and function of a deforested ecosystem, two strategies of ecological restoration are generally considered: active restoration, which eliminates the agents of disturbance and implements strategies to accelerate site recovery (e.g., tree planting and soil conservation practices), and passive restoration, which eliminates agents of disturbance in the area, relying on natural regeneration (Holl and Aide, 2011).
The process of natural regeneration is one of the most important issues in passive restoration, and can be seen as a continuous cycle of ecological processes, such as the development of seeds and their subsequent dispersal and predation, or the germination and establishment of seedlings, among others. The long-term success and dominance of tree species depends on these ecological processes (Pérez-López et al., 2013). Natural regeneration can be an appropriate option for passive restoration of forests (Pensado-Fernández et al., 2014); however, understanding the relationship between the structure and dynamics of canopy vegetation with seedling density, are crucial for predicting the likely effectiveness of passive restoration strategies (Grime and Hillier, 2000).
Natural regeneration rates are highly variable depending on the ecosystem, landscape context, land use history and passive restoration may not always be successful (Lara-González et al., 2009), taking longer to reach the goals established for the restoration of a site than an active restoration. Such delays in regeneration can sometimes be perceived as failures of passive restoration. Lands subject to passive restoration can be seen in developing countries as abandoned or unused land, which may encourage local people to use these areas for livestock or other activities. An advantage is that passive restoration is generally perceived as a low-cost alternative, although in general it has costs that are often not taken into account such as the purchase of material (fences or barriers) to isolate the ground from disturbance agents and payments for site surveillance (Zahawi et al., 2014). It has the potential to achieve similar levels of biodiversity and environmental services as an active restoration; however, it is only feasible in certain places where the disturbance was not so intense, natural communities are resilient and are far from human communities (Holl, 1999; Zahawi and Augspurger, 1999; Muñiz-Castro et al., 2006; Suding and Hobbs, 2009; Aide et al., 2010; Holl and Aide, 2011).
Due to the prevailing shade conditions throughout the understory, the rates of natural regeneration in temperate forests are reduced or even null in some cases. Temperate forests are renewed by the dynamic of gap formation (which can have both natural and artificial causes), where natural regeneration processes are increased considerably. Natural regeneration in situ, compared to traditional forest plantations, is an appropriate option for ecological rehabilitation on degraded land, especially if protected from livestock (Lara-González et al., 2009; Sánchez-Velásquez et al., 2016).
Populations of tree species differ genetically along elevational gradients, as a response to the selection pressure of temperature and precipitation gradients (Rehfeldt, 1991; Ortiz-Bibian et al., 2017). This makes it advisable to delineate elevational zonings to guide seed and seedling movement in reforestation programs. Castellanos-Acuña et al. (2014) reported a significant morphological differentiation among populations of A. religiosa along an elevational gradient: low-altitude populations have shorter needles and longer cones than high-altitude and these might have important consequences for seed production and seedling quality.
Scientific literature on A. religiosa shade tolerance is at times contradictory; according to Rzedowski (1978), the A. religiosa is a shade tolerant species and canopy gaps contribute to the regeneration of A. religiosa in the Cofre de Perote National Park, in Veracruz, Mexico, and seedling density is considerably greater in gaps than in the understory (Lara-González et al., 2009). However, some authors consider that the species can regenerate naturally in both clearings and understory (Narakawa and Yamamoto, 2001; Sugita and Tani, 2001; Mori and Takeda, 2002), while González et al. (1991) state that the species grows in open places in smaller proportions than in the understory.
Honey-Rosés et al. (2018) studied the drivers of forest cover both inside and outside the MBBR using a combination of remote sensing imagery and field-collected data. They found an increase in forest cover of 5,673 ha occurred between 1986 and 2012: 71% of this recovery was attributed to natural regeneration processes, while active restoration efforts only contributed 3.8%, raising questions about the effectiveness of active restoration. The rest (25%) was attributed to a combination of both techniques. The authors conclude that due to the high potential for natural regeneration in the reserve, management efforts should focus on passive restoration activities instead of investing in active restoration (Honey-Rosés et al., 2018).
While many forest managers may be attracted to the idea of supporting natural regrowth via passive restoration, various biophysical conditions may impede the successful recruitment of young seedlings in areas that are unhospitable to forest regrowth. For example, Manzanilla (1974) suggests a negative interaction of the moss layer thickness with A. religiosa seedling mortality; since thicker layers will generate a physical barrier that is responsible for the absence of natural regeneration. Local forest technicians and landowners of the MBBR support this assertion.
The objective of this study was to study factors that may affect natural regeneration of A. religiosa seedlings at MBBR, an essential consideration for implementing passive ecological restoration. We studied regeneration capacity through seedling density in response to elevation, canopy closure, and other abiotic factors such moss layer thickness and soil moisture. This research is intended to guide decision-making regarding the implementation of adequate restoration and conservation strategies at the Monarch butterfly overwintering sites in the MBBR.
Materials and Methods
Study Site
This study was carried out at Ejido de La Mesa, in the municipality of San José Del Rincon, Estado de México (19° 34′, 35.7″ N and 100° 14′, 30.2″ W), in the central-western part of the Mexican Transvolcanic-Belt.
In September and October of 2015, natural regeneration of A. religiosa seedlings was monitored along an elevational gradient (3050 to 3550 m a.s.l) in the MBBR. The transect range was classified into two different elevational bands: intermediate (3050–3300 m a.s.l.) and upper (3301–3550 m a.s.l.), according to the elevational zoning of Castellanos-Acuña et al. (2014). It was not possible to measure seedling density at the lower elevational band (2800–3050 m a.s.l.), since this is an area with a long history of impact by human settlement and agricultural and livestock activities, and the original A. religiosa trees at this low elevation remain only in small forest fragments.
At both elevational bands (intermediate and upper), we selected 53 sites with and without canopy gaps (canopy type): 25 sites in the intermediate band (11 under forest cover and 14 in gaps) and 28 sites in the upper band (9 under forest cover and 19 in gaps). The area for each gap was different (<400m2): the diameter not less than 15 × 15, nor more than 23 × 23 m (resembling the size of an adult tree canopy). In closed canopy sites a 15 m diameter circle was used (Supplementary Figure S1).
The selected sites presented slopes of less than 22° (on steeper slopes the effect of the gap decreases due to inclination of the crowns of adjacent trees). Abundance, height and diameter of seedlings (0–2 mm root collar diameter) and saplings (<5 cm DBH) were measured throughout each site with canopy gaps and within the 15 m circle for sites without canopy gaps. Additional parameters measured in each site included: canopy cover, slope, elevation and gap diameter. Percent cover of rocks, shrubs, herbaceous plants, mosses and bare soil was recorded in three 2 × 2 m square quadrats per site.
Seedling Mortality Measurements
We conducted an additional survey in the same area, evaluating the mortality of naturally regenerated A. religiosa seedlings throughout the 2016 dry season (from February to early June). Thirty quadrats of 4 m2 (2 × 2 m) were delimited and distributed in the same elevational bands (15 quadrats each in the intermediate and upper bands). The quadrats were always located beneath forest canopy (>60% of tree cover) with a minimum distance of 50 m apart to avoid spatial autocorrelation. In each quadrat, all of the recently emerged seedlings that appeared to be less than 1-year-old (older seedlings show a lignified stem) were individually labeled. Each month, we recorded alive seedlings and carefully collected dead seedlings for dry weight measurement.
In quadrats with presence of moss, the moss layer thickness was measured monthly in three adjacent sites per quadrat (to avoid disturbing seedlings and moss layer inside the quadrat). We collected small samples of moss and soil adjacent from each quadrat, weighed in situ, and packed fresh in zip sealed plastic bags for subsequent drying in the laboratory to estimate the relationship of volumetric moisture content.
Circular plots of 0.1 ha (17.8 m radius) were established to count adult trees above each quadrat and we measured height and diameter at breast height (DBH) of each tree recorded and grouped in three categories (<25 cm, 25–45 cm, >45 cm) according to Pineda-López et al. (2013) and Manzanilla (1974). Canopy cover was estimated from hemispheric photographs taken with Winscanopy (Regent Instruments Inc.) (Guay, 2014).
The samples of moss and soil were dried in an oven at 70°C for 5 days and weighed. Dead collected seedlings were divided into their root and aerial parts, which were dried in an oven for measurement of dry weight. The biomass allocation estimates were done to asses if dead seedlings fail to reach the soil beneath the moss layer. When dead seedlings were in moss, we recorded if the roots penetrated the moss and made it into the soil below since local forest technicians have claimed this is the main cause of seedling mortality.
Data Analysis
Seedling and sapling density was analyzed using a generalized linear model with a Poisson distribution. The independent variables were elevational band (intermediate or upper), canopy (gap or forest cover) and the interactions among these factors. Linear regression or Spearman rank correlation tests were applied to assess the relationship between the various environmental variables and seedling density.
To determine temporal variation of A. religiosa seedling mortality during 2016 dry season, we performed a repeated measures ANOVA, with a post hoc Tukey paired test (the dependent variable was the number of surviving seedlings per month while elevational band was the independent variable). The temporal comparison was conducted with paired Wilcoxon and Kruskal-Wallis tests.
Moisture content of moss and soil was estimated through the formula of gravimetric moisture: , where Ma is the weight of water lost following drying and Ms is the fresh weight of soil or moss (Universidad Nacional de Córdoba, 1993), representing the percentage or weight of water in 1 g of soil or moss.
The canopy photographs taken were analyzed with the Winscanopy (Regent Instruments Inc.), (Guay, 2014) and percentage of canopy cover was estimated.
In addition, linear and quadratic regressions were performed between seedling mortality and moss layer thickness, moss gravimetric moisture content and soil gravimetric moisture content, to identify a threshold that promoted major seedling mortality.
Finally, we applied a Cox proportional hazards model to analyze the influence of environmental and forest structural variables on seedling survival time. The independent variables were soil organic matter content, moss cover, maximum moisture content of moss, tree density, maximum and minimum thickness of moss layer, minimum moisture content of moss and minimum soil moisture content. A few seedlings disappeared from quadrats during the study. These may have been eaten by herbivores instead of dying but we included these individuals in the analyses. All statistical analyses were performed with the packages R 3.1.3 and JMP 8.0 SAS Institute Inc.
Results
Seedling and Sapling Density
There was a higher density of seedlings at the intermediate elevational band compared to upper band. Most of the individuals recorded in the intermediate band beneath the forest were seedlings (68%) while canopy gaps harbored a higher proportion of saplings than sites without canopy gaps (Figure 1). In the upper zone, in addition to the lower overall density, only 3% of individuals were seedlings, with similar proportions in each canopy type. However, there was a higher density of saplings in gaps. The results of the generalized linear model were significant for both parameters: elevational band (x2 = 352.7, df = 10, p < 0.001) and canopy type (x2 = 198.4, df = 10, p < 0.001). Saplings also showed significant differences: elevational band (x2 = 483, df = 10, p < 0.001) and canopy type (x2 = 243, df = 10, p < 0.001). However, there was no significant effect of interactions between these independent variables.
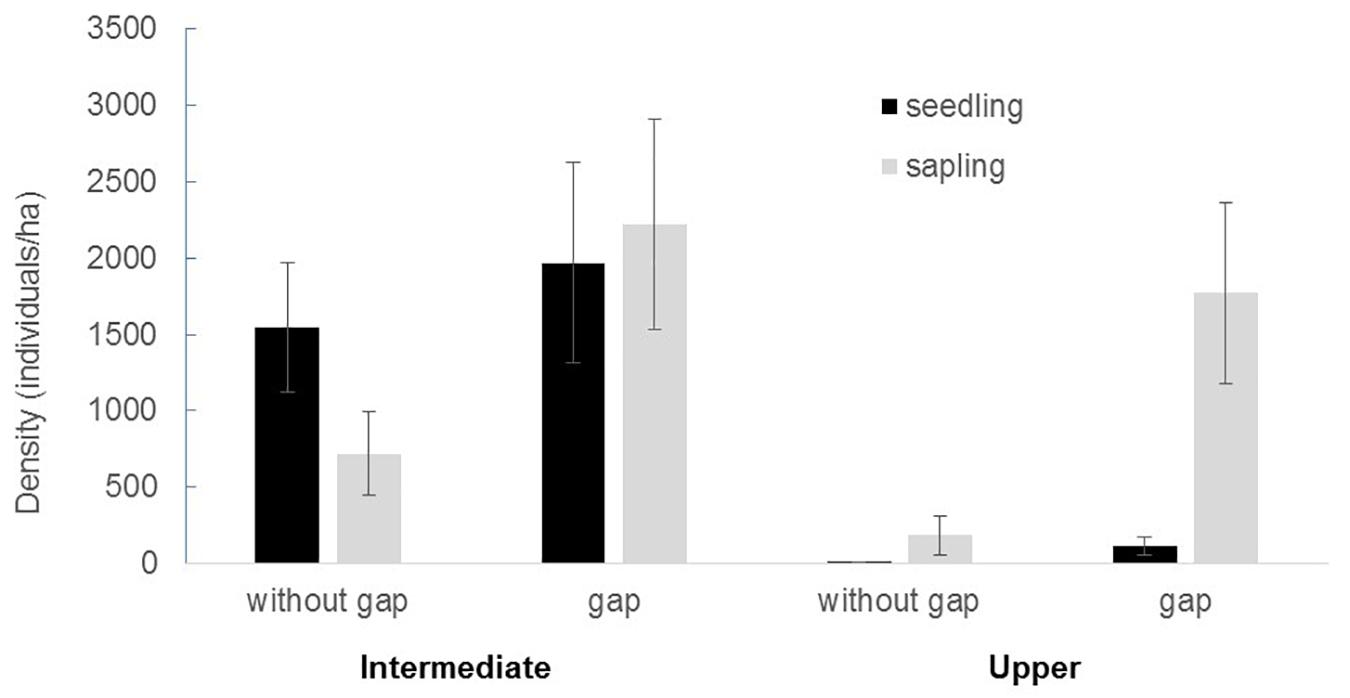
Figure 1. Seedling and sapling density by elevational band (intermediate 3050 to 3300 m a.s.l., upper band 3301 to 3550 m a.s.l.) and canopy type (with and without canopy gap).
Understory Conditions
The most common companion species at the sites were Acaena elongata (a shrub of 0.3 to 1 m in height), Alchemilla procumbens (a creeping grass of up to 30 cm in height), and Roldana angulifolia (a shrub of 1 to 2.5 m in height).
Despite the small difference in tree coverage between the gap and forest canopy types (Table 1), statistically significant differences were found. The results suggest that A. religiosa seedlings experience suitable conditions in the gaps (intermediate levels of light) for initiation of the natural regeneration process.
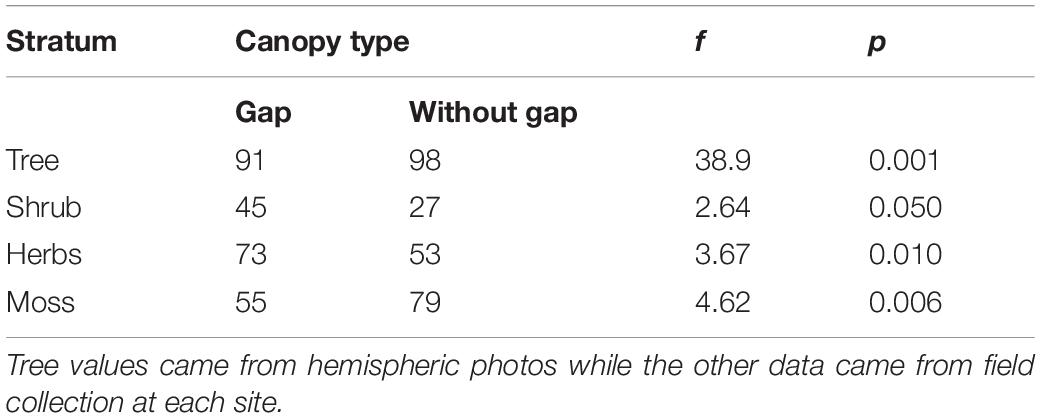
Table 1. Mean coverage (%) of different forest strata per canopy type (with and without gap), and significance of the difference between canopy types in each case.
The gaps presented a higher shrub and herb coverage than sites without gap, while there was higher coverage of moss beneath the forest canopy. In all four strata, significant differences were present between sites with and without gaps (Table 1). No differences in rock and bare soil coverage were observed between gaps and without gaps sites.
A non-parametric correlation of Spearman ranks was conducted between tree canopy openness and seedling density, revealing a weak relationship (p = 0.009, rs = 0.351, n = 53).
No significant differences were found when correlating moss cover with seedling density using the Spearman rank coefficient. However, moss cover was negatively related to other understory components (rocks, bare soil, and shrubs), and positively related to herbs (Table 2).
Seedling Mortality Survey
Six-hundred sixty-one A. religiosa seedlings were marked and monitored in 30 quadrats. In the upper elevational band, 15 quadrats were established and 378 seedlings monitored. In the intermediate elevational band, 15 quadrats were established and 283 seedlings monitored. We found that mortality increased during dry season reaching 48% (in either elevational band) when the rainy season began. In either elevational band the highest mortality occurred in April and the lowest in June (Figure 2).
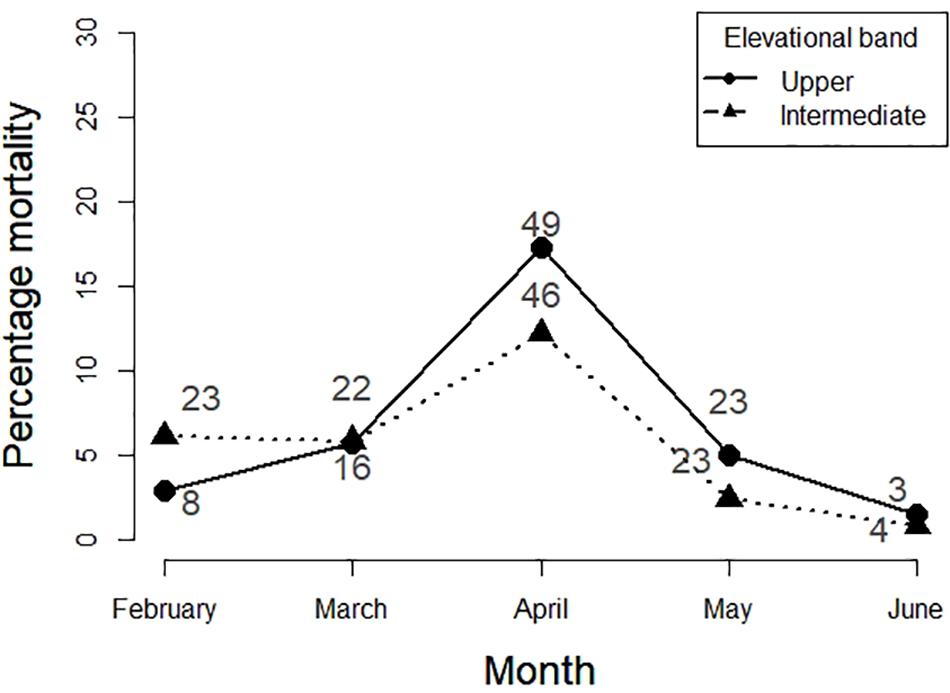
Figure 2. Abies religiosa mortality during the dry season between elevational bands, (numbers show seedling mortality in each sampling month). In March, 22 seedlings died in the upper band and 16 in the intermediate one.
Factors Associated With Seedling Mortality
No significant differences were found between the two elevational bands (Figure 2). A low proportion of the seedlings disappeared in the month of April and May and these individuals apparently had been consumed by herbivores or had decomposed during the interval between the two monitoring periods (Figure 3).
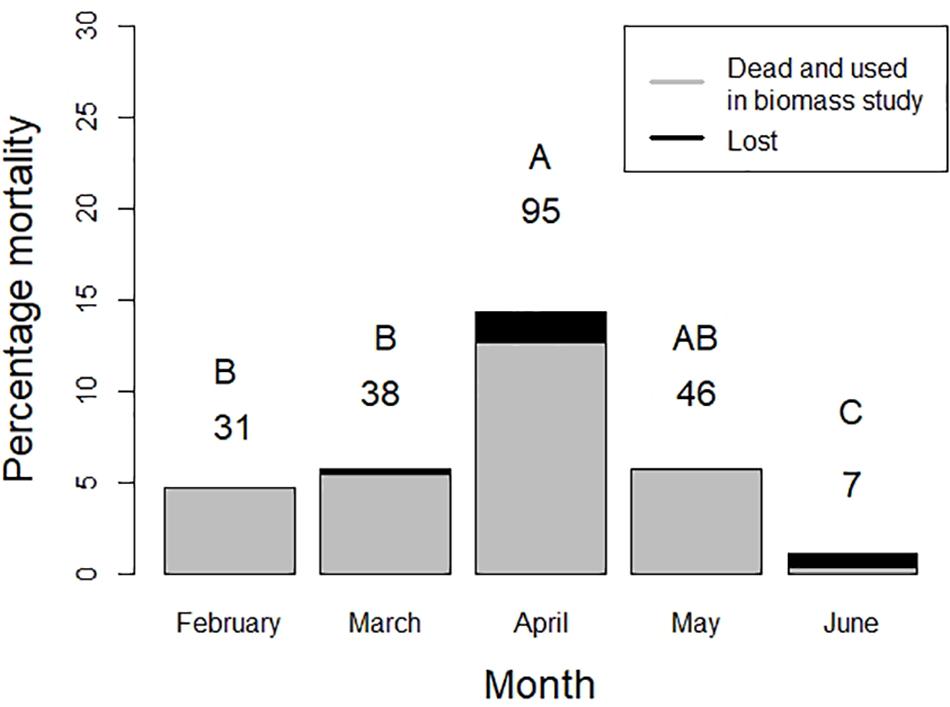
Figure 3. Causes of seedling mortality: this includes individuals with no obvious cause of death and were used in biomass allocation estimate, and “lost” refers to individuals where the entire plant was gone; letters show significant differences as result of repeated measures ANOVA.
Biomass Allocation Estimate
The dead seedlings presented greater average aboveground biomass compared to belowground biomass allocation, 65 and 35% in the upper band, and 68 vs. 32% at the intermediate band. The ANOVA shows significant differences between this biomass allocation, but no differences were found in this respect between elevational bands. We also analyzed the correlation between average aerial biomass and canopy cover of each site but there is not a significant relation between these variables.
Moss Layer Thickness and Moisture Content
The average initial thickness of the moss layer was 3.2 cm, and this decreased to a minimum value in April (a warm and dry month), at an average of 2.1 cm, before recovering quickly as a consequence of the early rains in June. April was the only statistically different month revealed in the repeated measures ANOVA. February and June showed the highest average values of thickness, with 3.1 and 2.9 cm, respectively (Figure 4). There were no statistically significant differences in moss layer thickness between elevational bands for any month.
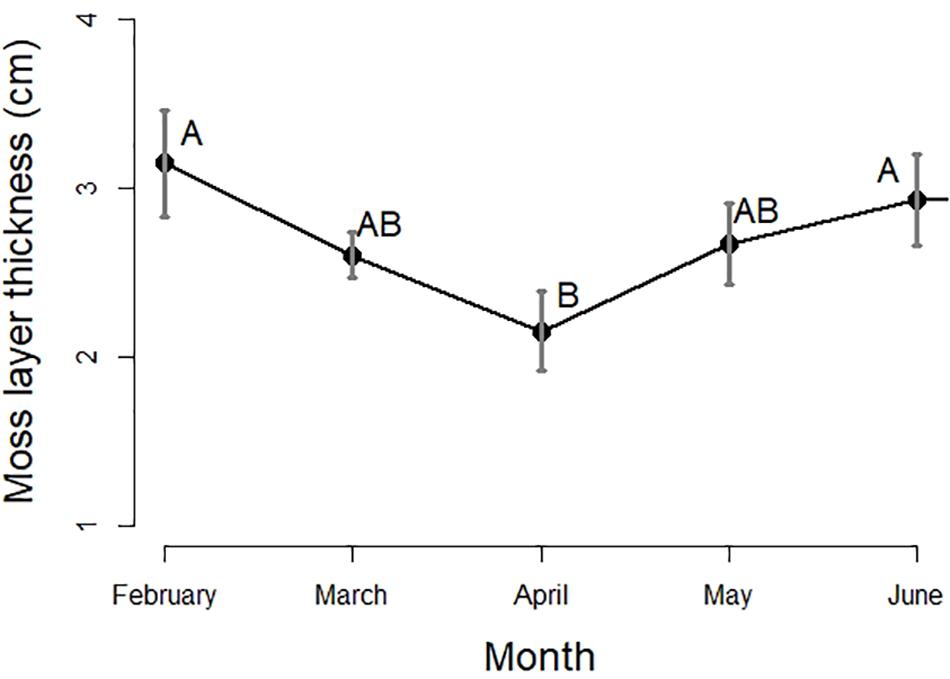
Figure 4. Moss layer thickness variation throughout the dry season. Different letters indicate significant (p < 0.05) differences among months.
Gravimetric Moisture Content of Moss and Soil
The moss layer showed a higher water retention capacity, containing up to 2.6 g of water/g of moss, as well as rapid dehydration and rehydration with precipitation. For both substrates (moss and soil), the lowest water content was observed in April, with statistically significant differences observed compared to the other months. The lowest thickness of the moss layer and the highest seedling mortality was also in April. In contrast, the highest moisture content was observed in June for both substrates because that month had the highest rainfall (Figure 5).
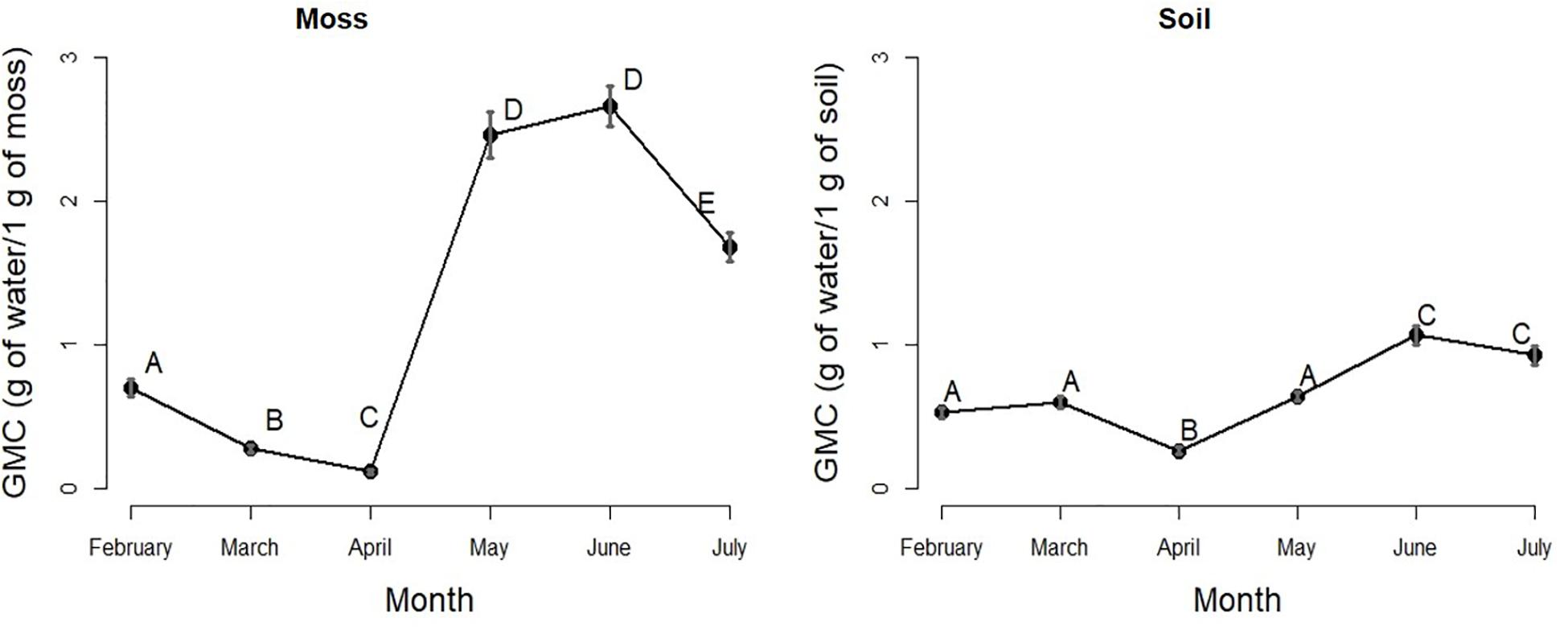
Figure 5. Gravimetric moisture content (GMC) of soil (right) and moss (left) over time; (different letters indicate statistical differences among the months as revealed by the repeated measures ANOVA).
The gravimetric moisture content of the moss showed a positive relationship with canopy cover only in the wettest month (June). The relationship of seedling mortality with monthly moisture content (of moss or soil) is statistically significant and shows clearly that lower humidity values are associated with higher monthly mortality rates (Figure 6). A general trend is evident: a mortality rate greater than 4% occurs when a critical threshold of 1 g of water/g of moss, or 0.7 g of water/g of soil, is reached during the dry season.
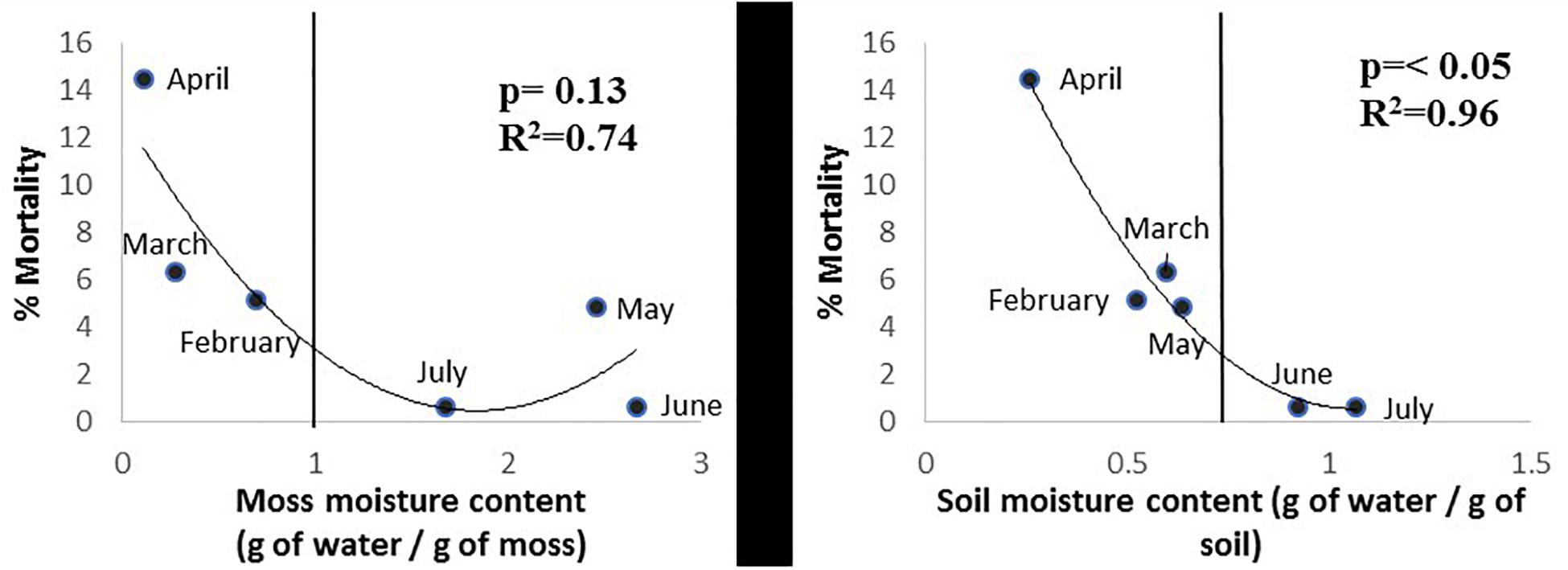
Figure 6. Quadratic regression of seedling mortality against: (left) moss moisture content, (right) soil moisture content. (Vertical black line indicates what appears to be a critical humidity threshold in relation to seedling mortality).
Proportional Risk Analysis
The Cox regression or proportional hazard analysis shows that three parameters had an effect on A. religiosa seedling mortality: soil organic matter content increases 29% the risk of seedling mortality, and tree density surrounding the sites (1%) and moss cover has a significant but weak effect in seedling mortality (Table 3).
Forest Structure
There were many differences in adult trees surrounding 4 m2 quadrats between the two elevational bands. Sites in the intermediate band showed an average tree density of 904/ha vs. 529 trees/ha in the upper band (f = 11.1, df = 1, p < 0.002). In the upper band, average tree height was 30.2 vs. 18.2 m in the intermediate band (f = 26.0, df = 1, p < 0.01). DBH was significantly higher in the upper band (f = 13.0, df = 1, p < 0.01), while no significant differences were recorded in the canopy cover (79.5% in intermediate vs. 76.8% in the upper band).
The tree diameter distribution showed that the average density of trees with dbh less than 25 cm is clearly higher in the intermediate band compared to the upper band, (f = 17.2, df = 1, p < 0.001). In the following two categories of DBH (25–45 cm and >45.1 cm), the density decreased and was significantly higher in the upper band (f = 9.1, df = 1, p < 0.001) and (f = 12.4, df = 1, p < 0.001), (Figure 7).
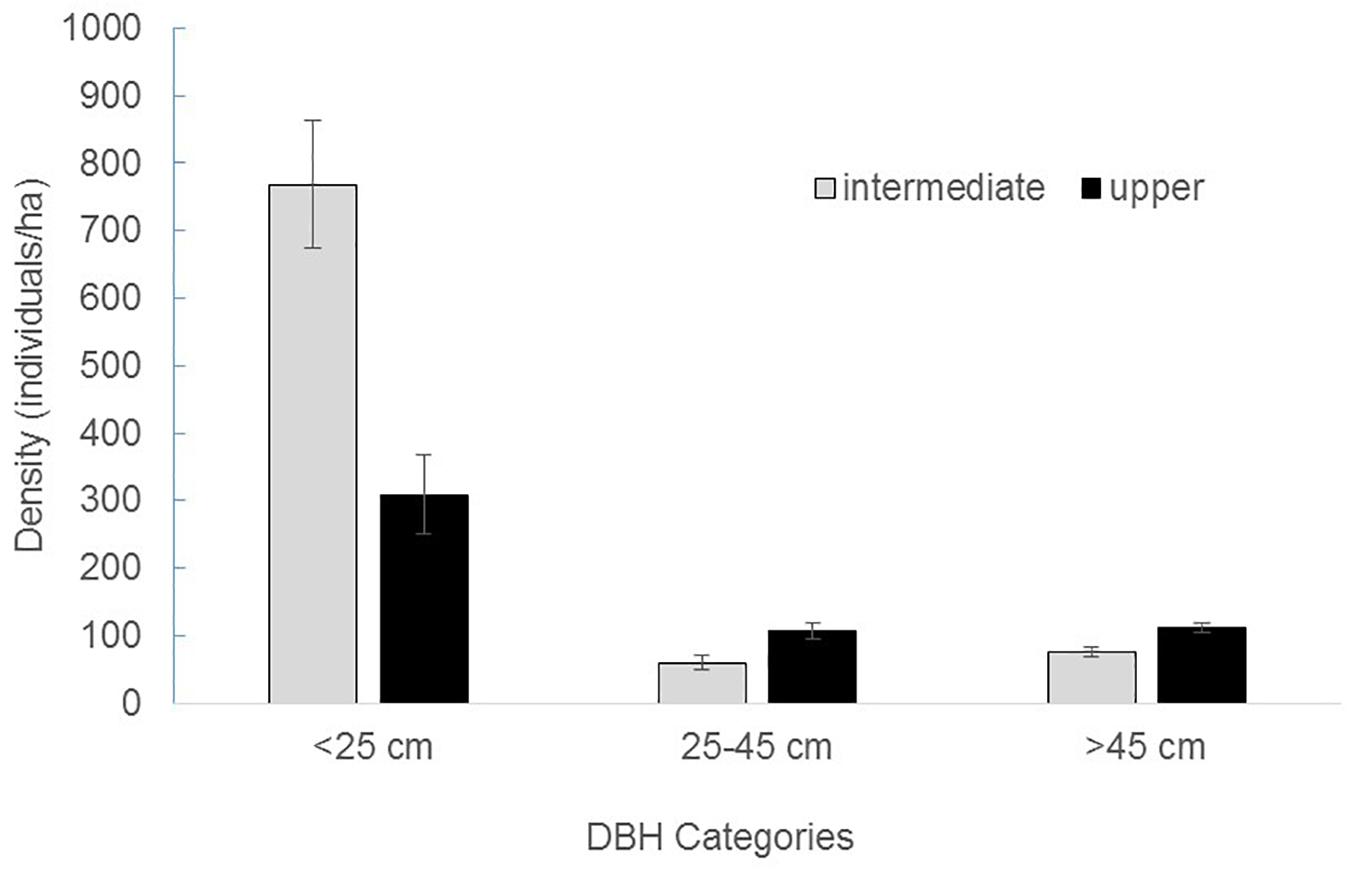
Figure 7. Average density (individuals/ha) of adult trees in each diameter category per elevational band.
Discussion
In the present study, several variables were considered to have affected the density of seedlings and saplings of A. religiosa, one of which is elevation. Ortiz-Bibian et al. (2019) found that populations of this species in the central part of their elevational distribution (intermediate zone) exhibit a higher number of viable seeds and greater germination capacity. This pattern could explain the larger number of seedlings and juveniles we recorded in the intermediate band.
The observed higher seedling and sapling density in gaps compared to forest is similar to the results of Lara-González et al. (2009), who found that regeneration of A. religiosa is favored in sites with greater canopy openness. Manzanilla (1974) reports that the regeneration of A. religiosa occurs clumped in sites with high availability of sunlight.
Regarding the size of the seedlings and saplings, diameter showed a similar pattern (most individuals belonging to smaller categories). However, there were notable differences between elevational bands. At the intermediate band most of the individuals recorded were seedlings (68% in forest and 45% in gaps); while for the upper band, only 3% were seedlings and 97% saplings. This suggests recruitment of seedlings to saplings is limited in the intermediate elevation band. On the other hand, seed limitation, either from a lack of seed production, germination, or early post-germination survival might be occurring at higher elevations. Likewise, in gaps at the intermediate band, a vigorous germination process could be underway, which would ensure that suitable plants are established for the regeneration of the forest.
In relation to canopy type, individuals in gaps had greater size and gaps had the highest percentage of shrub and herbaceous plant coverage. This vegetation could therefore play a “nurse plant” role, which might act to favor the establishment and growth of A. religiosa. Sánchez-Velázquez et al. (2011), and also Blanco-García et al. (2011) measured the effect of nurse plants such as Baccharis conferta and Lupinus elegans in an A. religiosa reforestation trial and documented lower mortality and higher growth of Abies when growing under the canopy of these shrubs.
Bautista (2013) and Lara-González et al. (2009) reported that morphological variables (number and length of lateral buds) and natural regeneration (seedling density) of A. religiosa are favored with increased canopy openness, as confirmed by the present study. Even when canopy cover between gaps and forests was slightly different (92 vs. 99%, respectively) these differences could determine the suitability of the light conditions for Abies religiosa.
The Abies religiosa forests of Mexico are relatively dense because of their closed canopies; the light that comes to the ground is low and the understory is scarce, so the existence of gaps is not common even though its contribution to forest regeneration is very important. It has been observed that in open areas the regeneration is more successful under the canopy of some shrubs that act as nurse plants facilitating fir regeneration (Lara-González et al., 2009).
Seedling Mortality Survey
The seedling mortality recorded in our survey was lower compared to results reported for Abies pinsapo (Arista, 1993). In the latter species, the possible factors contributing to seedling mortality were high light intensity, low humidity and competition with herbaceous plants. It was noted that seedlings died quickly as soon as spring and summer began, possibly as a result of water stress since they were exposed to full sunlight. The following year, the same author (Arista, 1994) reported contrasting data for another population of the same species, where seedlings less than 1-year-old presented 45% survival in understory and 82% in an open field, while older seedlings presented survival of 75% in the forest and 83% in an open field. That study indicates low humidity, light and extreme temperatures as the main factors that contribute to high mortality. Moreover, where humidity was not a limiting factor, mortality was attributed to a possible fungal attack or lack of mycorrhizae.
Ángeles-Cervantes and López Mata (2009) investigated mortality in a cohort of A. religiosa seedlings in patches affected and unaffected by fires, and found that an important factor increasing Abies seedling mortality is desiccation. This is attributable to the layer of moss and accumulated litter, which prevents the root from reaching and penetrating the mineral soil beneath. This could be one of the factors by which sites with denser canopies (which have the highest percentage of moss) present less natural regeneration, since even though moss may constitute a suitable microsite for the germination of the A. religiosa seed, thicker layers of moss actually behave as a barrier for the longer-term persistence of the seedling. This concurs with comments made by nursery managers located within the MBBR, as well as forest technicians, who report that the presence of the moss strongly causes mortality of A. religiosa seedlings and that its partial removal might increase A. religiosa seedling survival.
Biomass Allocation Estimate
The difference in recorded biomass allocation may have an effect on seedling mortality, since the failure of the root system to supply water to the plant or to regenerate new roots will lead to a vicious circle of water stress and depletion of carbohydrates, which will cause a delay or a reduction of regrowth, or even the death of the plant, since desiccation of the roots is considered to have the most damaging effect on plant vitality (Brønnum, 2005).
Effect of Moss Layer
Our results suggest that the moss layer is not a primary limiting factor for A. religiosa seedling survival. Manzanilla (1974) found that in the A. religiosa forests, the thick layer of moss is responsible for the low natural regeneration, since it acts as a mechanical barrier reaching up to 30 cm in thickness in an understory with abundant vegetation that prevents the seedling root from reaching and anchoring to the mineral soil. Our study did not find moss layers as thick as those reported in Manzanilla (1974) and only 3.8% of dead seedlings roots failed to penetrate the moss layer in our quadrats. Manzanilla (1974) suggests the hypothesis that the negative interaction of the moss with the seedlings will generate a physical barrier that is responsible for the absence of natural regeneration. Local forest technicians and land owners also support this assertion.
Similarly, there have been reports of positive and negative effects on germination and recruitment generated by the organic matter layer, since this layer usually reduces soil temperature and water evaporation, increasing moisture in the soil and promoting better conditions for germination. Nevertheless, it can generate an allelopathic inhibition, reduce the incidence of light or form a physical barrier to the penetration of the seedling roots (Dechoum et al., 2015).
In contrast to the potential negative effects of moss on seedling establishment described above, in our study sites, the moss seems to provide a suitable environment for seed germination and seedling establishment, which is very important for the dispersion capability and establishment of woody species (Dechoum et al., 2015). However, with the onset of the dry season of the year, the moss loses its moisture very quickly, causing thinning of the moss layer and a subsequent loss of soil moisture, leaving the seedlings more exposed to other agents that can potentially cause mortality, such as temperature, solar radiation, lack of environmental humidity. This effect of the humidity has been described by Chen et al. (2015) as influencing the richness and abundance of moss species during the transition from dry to wet periods, and its variation due to the differential tolerance of some species to this abiotic factor.
In our study quadrats, soil moisture seems to be the abiotic factor that most affects the mortality of A. religiosa seedlings both directly and indirectly. For the genus Abies, availability of water is very important at the seedling stage, since several species are extremely sensitive to a moisture deficit in the substrate. Indeed, it is considered the most important factor in the mortality of coniferous seedlings within the first 5 years of growth (Van der Salm et al., 2007; Rodríguez-Laguna et al., 2015). A clear example is the high sensitivity to stress due to desiccation reported for Abies prosera in a study conducted under controlled conditions (Brønnum, 2005). In addition, it is essential to consider the possible impact on ecosystems as a consequence of climate change (Ledo et al., 2015), which is modifying the patterns and frequency of the dry period and will likely have severe effects on the recruitment of seedlings in the forests.
Finally, it is possible that critical environmental thresholds (such as mortality greater than 4% per month with a reduction of 1 g of water/g of moss or 0.7 g of water/g of soil) would be lowered given projected climate change scenarios (Sáenz-Romero et al., 2012). Higher temperatures and lower precipitation could prevent the successful establishment of some tree species or may limit their establishment to favorable years only, ultimately changing the structure and functioning of the forest ecosystem (Von Arx et al., 2013).
Conclusion
Abies religiosa seedlings are more abundant at intermediate sites (3050 to 3300 m a.s.l.) than at upper (3301 to 3550 m a.s.l.) elevations, where poor establishment and recruitment of seedlings over the last 20 years have been observed. Additionally, canopy gaps play a positive and very important role in seedling recruitment, but a high proportion of seedling failure occurs at intermediate elevations, and the consequent lack of recruitment is an important issue that requires further research.
We found no evidence that the moss layer is responsible for seedling mortality; indeed, it constitutes an excellent moist microsite for seed establishment and germination, as well as protecting the bare soil from excessive moisture loss through evapotranspiration.
The most important factor increasing seedling mortality is soil moisture in the critical warm and dry month of April. This condition is likely to worsen under future scenarios of climatic change, affecting the regeneration of the Abies religiosa forest.
The upper elevational range of the MBBR is experiencing serious changes and active restoration might be needed to maintain forest cover and the ecosystem services it provides for inhabitants of the region, including the overwintering Monarch butterfly colonies.
Passive restoration practice in MBBR is favored by a high seed production and germination but constrained by a low seedling density (especially beneath closed canopies in upper elevation sites) and low recruitment (especially in intermediate elevation sites). Forest management activities might be needed to promote gap formation and improve seedling recruitment to sapling stage.
Author Contributions
AB-G and CS-R conceived the research project. GG-A, AC-N, and AB-G carried out the field measurements and conducted the statistical analysis. LL-T and YH-D provided helpful comments during the development of the project and proposed and conducted specific statistical analyses. All of the co-authors revised and contributed to the manuscript. AB-G led the writing of the manuscript.
Funding
Financial support was provided to AB-G by the UMSNH Coordinación de la Investigación Científica; the Basic Research CONACYT Fund (Ciencia Básica-2014-242985) and the UNAM Laboratorio Nacional de Análisis y Síntesis Ecológica (LANASE 2018-293701).
Conflict of Interest
The authors declare that the research was conducted in the absence of any commercial or financial relationships that could be construed as a potential conflict of interest.
The handling Editor and reviewer JD, declared their involvement as co-editors in the Research Topic, and confirm the absence of any other ongoing collaboration.
Acknowledgments
We thank Sr. Francisco Ramírez Cruz and Sra. Doña Petra Cruz-Cruz, for the facilities for site selection and seedling measurement at Ejido La Mesa. Without their help, this study would not have been possible. The MBBR staff helped to select sites for fieldwork and granted the license required to conduct the research. Thanks to Marcos Sandoval-Soto, Beatriz Guerrero-Carmona, Nancy Farías-Rivero, Miriam Linares, Jorge Herrera-Franco, Francisco Loera-Padilla, and other UMSNH students for assistance with taking measurements. We also thank two reviewers and an English proofreader for improving the quality of this manuscript.
Supplementary Material
The Supplementary Material for this article can be found online at: https://www.frontiersin.org/articles/10.3389/fevo.2020.00115/full#supplementary-material
FIGURE S1 | Two different canopy types: on the left, a gap; on the right a site without gap (beneath the forest).
References
Aide, T. M., Ruiz-Jaén, M. C., and Grau, H. R. (2010). “What is the state of tropical montane cloud forest restoration?,” in Tropical Montane Cloud Forests Science for Conservation and Management, eds L. A. Bruijnzeel, F. N. Scatena, and L. S. Hamilton (Cambridge: Cambridge University Press), 101–110.
Ángeles-Cervantes, E., and López Mata, L. Y. (2009). Supervivencia de una cohorte de plántulas de Abies religiosa bajo diferentes condiciones postincendio. Boletín Soc. Bot. México 84, 25–33.
Arista, M. (1993). Germinación de las Semillas y supervivencia de las plántulas de abies pinsapo boiss. Acta Bot. Malacit. 18, 173–177.
Arista, M. (1994). Supervivencia de las plántulas de abies pinsapo boiss. en su habitat natural. Anales Jardín Botánico de Madrid 2, 193–198.
Bautista, S. C. (2013). Estructura del bosque y arquitectura de brinzales de Abies religiosa en Tlaxco, Tlaxcala. Ph. D thesis, Institución de Enseñanza e Investigación en Ciencias Agrícolas, Mexico.
Blanco-García, A., Sáenz-Romero, C., Martorell, C., Alvarado-Sosa, P., and Lindig-Cisneros, R. (2011). Nurse-plant and mulching effects on three conifer species in a Mexican temperate forest. Ecol. Eng. 37, 994–998.
Brønnum, P. (2005). preplanting indicators of survival and growth of desiccated abies procera bareroot planting stock. Scand. J. Forest Res. 1, 36–46.
Castellanos-Acuña, D., Sáenz, C., Lindig-Cisneros, R. A., and Silva-Farías, M. A. (2014). Zonificación altitudinal provisional de Abies religiosa en una área cercana a la reserva de la biosfera mariposa monarca, Michoacán. Revist. Chapingo Ser. Cienc. Forest. Ambient. 20, 215–225. doi: 10.5154/r.rchscfa.2013.11.041
Chen, S., Silk, J., Gao, J., Mao, L. F., and Shen, M. W. (2015). Latitudinal diversity gradients in bryophytes and woody plants: roles of temperature and water availability. J. Syst. Evol. 6, 535–545.
Dechoum, M. S., Zenni, R. D., Castellani, T. T., Zalba, S. M., and Rejmánek, M. (2015). Invasions across secondary forest successional stages: effects of local plant community, soil, litter, and herbivory on Hovenia dulcis seed germination and seedling establishment. Plant Ecol. 216, 823–833.
González, G., Musalem, M., Zarate, A., Lara, G., and Velásquez, M. A. (1991). Estudios de germinación en Oyamel (Abies religiosa [H.B.K.] Schl et Cham) bajo ambientes naturales en Zoquiapan, México. Rev. Chapingo 15, 59–66.
Grime, J. P., and Hillier, S. H. (2000). “The contribution of seedling regeneration to the structure and dynamics of plant communities, ecosystems and larger units in the landscape,” in Seeds: The Ecology of Regeneration in Plant Communities, 2nd Edn, ed. M. Fenner (Wallingford: CAB International), 361–374.
Holl, K. D. (1999). Factors limiting tropical rain forest regeneration in abandoned pasture: seed rain, seed germination, microclimate, and soil. Biotropica 31, 229–242.
Holl, K. D., and Aide, T. M. (2011). When and where to actively restore ecosystems? Forest Ecol. Manag. 261, 1558–1563.
Honey-Rosés, J., Baylis, K., and Ramírez, M. (2011). A spatially explicit estimate of avoided forest loss. Conserv. Biol. 25, 1032–1043. doi: 10.1111/j.1523-1739.2011.01729.x
Honey-Rosés, J., Maurer, M., Ramirez, M. I, and Corbera, E. (2018). Quantifying active and passive restoration in Central Mexico from 1986–2012: assessing the evidence of a forest transition. Restor. Ecol. 26, 1180–1189.
Lara-González, R., Sánchez-Velásquez, L. R., and Corral-Aguirre, J. (2009). Regeneración de Abies religiosa en claros del dosel versus sotobosque, parque nacional cofre de perote, México. Agrociencia 43, 739–747.
Ledo, A., Cayuela, L., Manso, R., and Condes, S. (2015). Recruitment patterns and potential mechanisms of community assembly in an Andean cloud forest. J. Veget. Sci. 26, 876–888.
Manzanilla, H. (1974). Investigaciones Epidométricas Y Silvícolas En Bosques Mexicanos De Abies Religiosa. Ciudad de México: Secretaría de Agricultura y Ganadería.
Mori, A., and Takeda, H. (2002). Light-related competitive effects of overstory trees on the understory conifer saplings in a subalpine forest. J. For. Res. 8, 163–168.
Muñiz-Castro, M. A., Williams-Linera, G., and Benayas, J. M. (2006). Distance effect from cloud forest fragments on plant community structure in abandoned pastures in Veracruz, Mexico. J. Tropical Ecol. 22, 431–440.
Narakawa, Y., and Yamamoto, I. S. (2001). Gap formation, microsite variation and the conifer seedling occurrence in a subalpine old-growth forest, Central Japan. Ecol. Res. 16, 617–625.
Ortiz-Bibian, M. A., Blanco-García, A., Lindig-Cisneros, R. A., Gómez-Romero, M., Castellanos-Acuña, D., and Herrerías-Diego, et al. (2017). Genetic variation in Abies religiosa for quantitative traits and delineation of elevational and climatic zoning for maintaining monarch butterfly overwintering sites in Mexico, considering climatic change. Silvae Genet. 66, 14–23.
Ortiz-Bibian, M. A., Castellanos-Acuña, D., Gómez-Romero, M., Lindig-Cisneros, R. A., Silva-Farías, M. A., and Sáenz-Romero, C. (2019). Variation among Abies religiosa (H.B.K.) schl. et cham populations along an elevational gradient of seed germination capacity. Revist. Fitotecn. Mexican. 42, 301–308.
Pensado-Fernández, J. A., Sánchez-Velásquez, L. R., Pineda-López, M., del, R., and Díaz-Fleischer, F. (2014). Plantaciones forestales vs. regeneración natural in situ: el caso de los pinos y la rehabilitación en el parque nacional cofre de perote. Bot. Sci. 92, 617–622.
Pérez-López, P., López-Barrera, F., García-Oliva, F., Cuevas-Reyes, P., and González-Rodríguez, A. (2013). Procesos de regeneración natural en bosques de encinos: factores facilitadores y limitantes. Biol. Public. Espec. 1, 18–24.
Pineda-López, M. R., Ortega-Solís, R., Sánchez-Velásquez, L. R., Ortiz-Ceballos, G., and Vázquez-Domínguez, G. (2013). Estructura poblacional de Abies religiosa (Kunth) Schltdl. et Cham., en el ejido el conejo del parque nacional cofre de perote, Veracruz, Mexico. Revist. Chapingo Ser. Cienc. Forest. Ambient. 3, 375–385.
Rehfeldt, G. E. (1991). “Gene resource management: using models of genetic variation in silviculture,” in Proceedings of the Genetics/Silviculture Workshop, Wenatchee.
Rodríguez-Laguna, R., Razo-Zárate, R., Fonseca-González, J., Capulín-Grande, J., and Goche-Telles, R. (2015). Regeneración natural post-incendio de Abies religiosa (H B K) Schl. et Cham, En El Parque Nacional ‘El chico’ Hidalgo. Revist. Iberoameric. Cienc. 2, 11–22.
Rzedowski, J. (2006). Vegetación de México, 1st Edn, México: Comisión Nacional para el Conocimiento y Uso de la Biodiversidad.
Sáenz-Romero, C., Rehfeldt, G. E., Duval, P., and Lindig-Cisneros, R. A. (2012). Abies religiosa habitat prediction in climatic change scenarios and implications for monarch butterfly conservation in Mexico. Forest Ecol. Manag. 275, 98–106.
Sánchez-Velásquez, L. R., Pineda-López, M., Avendaño-Yáñez, L., Lara-González, R., Pensado-Fernández, J. A., Ortega-Solís, R., et al. (2016). “Ecología, restauración y regeneración en la región del Cofre de Perote,” in El Cofre de Perote Situación, perspectivas e importancia. eds H. V. Narave-Flores, L. Garibay-Pardo, M. Chamorro-Zárate, L. R. Álvarez-Oseguera, and Y. De la Cruz-Elizondo (México: Universidad Veracruzana), 147–152.
Sánchez-Velázquez, L. R., Dominguez-Hernández, D., Pineda-Lopez, M., del, R., and Lara-González, R. (2011). Does Baccharis conferta shrub Act as a nurse plant to the Abies religiosa seedling? Open Forest Sci. J. 4, 67–70. doi: 10.2174/1874398601104010067
Suding, K. N., and Hobbs, R. J. (2009). Threshold models in restoration and conservation: a developing framework. Trends Ecol. Evol. 24, 271–279. doi: 10.1016/j.tree.2008.11.012
Sugita, H., and Tani, M. (2001). Differences in microhabitat related regeneration patterns between two subalpine conifers, Tsuga diversifolia and Abies mariesii, on Mount Hayachine, northern Honshu, Japan. Ecol. Res. 16, 423–433.
Universidad Nacional de Córdoba (1993). Determinación del Contenido Hídrico del Suelo. Technical Report, NO. 8.
Van der Salm, C., de Vries, W., Reinds, G. J., and Dise, N. B. (2007). N Leaching across European forests: derivation and validation of empirical relationships using data from intensive monitoring quadrats. Forest Ecol. Manag. 238, 81–91.
Von Arx, G., Graf, E. P., Thimonier, A., and Rebetez, M. (2013). Microclimate in forests with varying leaf area index and soil moisture: potential implications for seedling establishment in a changing climate. J. Ecol. 5, 1201–1213.
Zahawi, R. A., and Augspurger, C. K. (1999). Early plant succession in abandoned pastures in Ecuador. Biotropica 31, 540–552.
Keywords: Abies religiosa, soil moisture, natural regeneration, seedlings, elevational gradient
Citation: Guzmán-Aguilar G, Carbajal-Navarro A, Sáenz-Romero C, Herrerías-Diego Y, López-Toledo L and Blanco-García A (2020) Abies religiosa Seedling Limitations for Passive Restoration Practices at the Monarch Butterfly Biosphere Reserve in Mexico. Front. Ecol. Evol. 8:115. doi: 10.3389/fevo.2020.00115
Received: 31 December 2018; Accepted: 09 April 2020;
Published: 13 May 2020.
Edited by:
Ryan G. Drum, United States Fish and Wildlife Service (USFWS), United StatesReviewed by:
Jordi Honey-Roses, The University of British Columbia, CanadaJay E. Diffendorfer, United States Geological Survey (USGS), United States
Copyright © 2020 Guzmán-Aguilar, Carbajal-Navarro, Sáenz-Romero, Herrerías-Diego, López-Toledo and Blanco-García. This is an open-access article distributed under the terms of the Creative Commons Attribution License (CC BY). The use, distribution or reproduction in other forums is permitted, provided the original author(s) and the copyright owner(s) are credited and that the original publication in this journal is cited, in accordance with accepted academic practice. No use, distribution or reproduction is permitted which does not comply with these terms.
*Correspondence: Arnulfo Blanco-García, YXJudWxmb2JsYW5jb0B5YWhvby5jb20ubXg=