- Department of Biological Sciences, University of Calgary, Calgary, AB, Canada
Variation in flower symmetry is a remarkable aspect of flowering plant diversity. Multiple evolutionary transitions from an ancestral form of radial symmetry to bilateral symmetry and reversals from bilateral to radial symmetry have occurred during angiosperm evolution. The high incident of symmetry transitions poses the question concerning the homology of underlying developmental processes. I conducted a comprehensive study of developmental expression of symmetry across angiosperms covering 72 families and 39 orders. A wide range of timing for the expression of symmetry was found from organ initiation, to enlargement, to late differentiation of organs. In many studied genera, the symmetry pattern was transient during development. Mapping the floral symmetry patterns on the phylogenetic tree indicated the scattered distribution of patterns across angiosperms. Mid to late onset of actinomorphy/zygomorphy was found in all major clades. The expression of symmetry was also versatile within families. No strong relationship was found between the degree of zygomorphy at mature stage and its time of expression. The developmental constraints exerted by the inflorescence, bracts, neighbor flowers or neighbor organs within a flower could provide a possible explanation for the observed variation of symmetry expression.
Introduction
One of the fascinating aspects of the floral organization is the balanced and harmonic arrangement of organs within a flower creating specific symmetry patterns. Actinomorphy (i.e., polysymmetry or radial symmetry with several symmetry planes) and zygomorphy (i.e., monosymmetry or bilateral symmetry with one symmetry plane) are the most common types of symmetry among flowering plants (Endress, 1999, 2010). Zygomorphy has diverse expressions in flowers, affecting organ categories in various combination (all organs, or only one organ category) and to various degrees (by differential shape, size, curvature, number) (Citerne et al., 2010; Endress, 2012). A weak degree of monosymmetry which is sometimes expressed due to the positional effect (positional monosymmetry, e.g., bending of stamens and styles due to gravity), may provide a precondition for the evolution of elaborate constitutional monosymmetry in polysymmetric groups (Endress, 1999). Except for Lamiales in which both corolla and androecium are remarkably affected, monosymmetry is commonly concentrated on or restricted to the perianth (Endress, 2012).
The reconstruction of the flower symmetry on the phylogenetic tree of angiosperms indicated actinomorphy as the ancestral state which is in agreement with its prevalence in flowers of basal angiosperms (Endress and Doyle, 2009). While relatively elaborate forms of zygomorphy are found in basal angiosperms (Aristolochiaceae of Piperales) and monocots (Orchidaceae of Asparagales, Commelinaceae of Commelinales), zygomorphy is mainly concentrated among rosids especially in Fabales and Malvales and among asterids especially in Lamiales, Asterales, and Dipsacales (Citerne et al., 2010; Endress, 2012). The analysis of symmetry evolution on the phylogenetic tree of angiosperms indicated frequent transitions from the ancestral actinomorphy to zygomorphy at least two times among the basal angiosperms, 29 times in monocots, and 99 times in the eudicots (Reyes et al., 2016). The high incident of zygomorphy and its homoplastic evolution poses the question concerning the homology of underlying developmental processes.
Floral symmetry is established through the activity of the floral meristem during organ initiation, enlargement, and subsequent differentiation. Several authors studied the manifestation of floral symmetry during ontogeny (Mair, 1977; Endress, 1999; Tucker, 1999). They reported a wide range of timing for the expression of symmetry, from organ initiation, to later enlargement of floral organs, to late differentiation of structures. In many studied genera, the symmetry pattern is transient during development. The symmetry established by the ordered initiation of floral organs at the beginning of development can be reduced, enhanced or changed later through differential growth of floral sectors. The developmental or evolutionary constraints underlying such labile expression of symmetry is poorly understood. It is suggested that the phylogenetic background of taxa and the degree of zygomorphy in mature flowers can influence the time of zygomorphy expression (Endress, 1999; Tucker, 1999). In many families with prevalent elaborate zygomorphy (e.g., Fabaceae), zygomorphy is manifested early at organs initiation and persists throughout development (Tucker, 2003). On the other hand, in zygomorphic taxa embedded in groups with predominantly actinomorphic flowers (e.g., Aconitum in Ranunculaceae), the flower is actinomorphic through most of its development and the manifestation of zygomorphy occurs toward the end of the ontogeny (Mair, 1977). However, the reverse condition also occurs. Some lamiaceous flowers with elaborate zygomorphy have shown a preceding actinomorphy in early development (Naghiloo et al., 2013a, b, 2014b). The weakly zygomorphic flowers of Capparis spinosa from the actinomorphic family Capparaceae express signs of zygomorphy at organ initiation (Naghiloo et al., 2015).
I conducted a comprehensive study of developmental expression of symmetry across angiosperms covering 72 families and 39 orders. Focusing on corolla symmetry as the most prominent manifestation of symmetry, I traced the evolution of developmental changes on a phylogenetic background. The objectives were (1) to determine patterns of symmetry expression within angiosperms, (2) to map the distribution of patterns on a phylogenetic tree, and (3) to infer evolutionary and developmental constraints underlying diversity of symmetry patterns.
Materials and Methods
Data Collection
The pattern of symmetry expression of 227 species belonging to 72 families and 39 orders of angiosperms was extracted from literature (for details of species and list of literature see Supplementary Appendix S1). The corolla symmetry in anthetic flowers was identified as zygomorphic (79 species) or actinomorphic (148 species) based on the available descriptions and images of the flower. Focusing on the corolla symmetry, I described tetramerous flowers of Brassicaceae and Oleaceae as actinomorphic while they are otherwise described as dissymmetric considering the whole flower.
I then used SEM micrographs from the literature to indicate the expression of symmetry in three developmental stages: (1) The early/initiation phase in which the first trace of organ primordia was detectable. (2) The mid/enlargement phase in which all primordia enlarged as clear bulges. (3) The late/differentiation phase during which the growth of primordia was accelerated and they started to cover the inner organs. The literatures in which the related stages could not be detected were excluded from the study.
The symmetry pattern in early phase was evaluated based on the order of primordia emergence; the simultaneous appearance was interpreted as actinomrphy, while the sequential appearance with a single dorsoventral axis was described as zygomrphy. The decision about organ initiation was based on clear separation from the floral meristem usually forming a furrow. In mid and late phases, the size difference was used as a criterion to indicate symmetry pattern; the size equality was described as actinomorphy while the size gradient along the dorsoventral axis was interpreted as zygomorphy.
Micrographs
Upon identifying the symmetry patterns, representative micrographs for each developmental pattern were extracted from the author’s previous publications and presented. For a description of the microscopic procedure, see the related publications (Dadpour et al., 2011a, b, 2012a; Naghiloo et al., 2012, 2013b, 2014a; Naghiloo and Classen-Bockhoff, 2016; Naghiloo and Claßen-Bockhoff, 2017).
Phylogenetic Framework and Character Optimization
I used the angiosperm phylogeny created by Soltis et al. (2011) to infer the relationships among the 72 families examined in the current study. This well-resolved tree was based on data from 17 DNA regions representing all three plant genomes, i.e., nucleus, plastid, and mitochondrion (Soltis et al., 2011). All branches were set equal with a value of 1.
To infer the evolutionary change in symmetry pattern, character optimization was carried out with the Maximum Parsimony method implemented in Mesquite 3.04 (Maddison and Maddison, 2015). All character changes were treated as an unordered and equally weighted.
The Maximum Likelihood method (ML) was not employed here as this method does not accept polymorphisms which were numerous in my dataset.
Results
Patterns of Symmetry Expression
(A) Actinomorphic Species: The evaluation of symmetry expression in 148 actinomorphic species indicated the occurrence of four developmental patterns (Figures 1, 2):
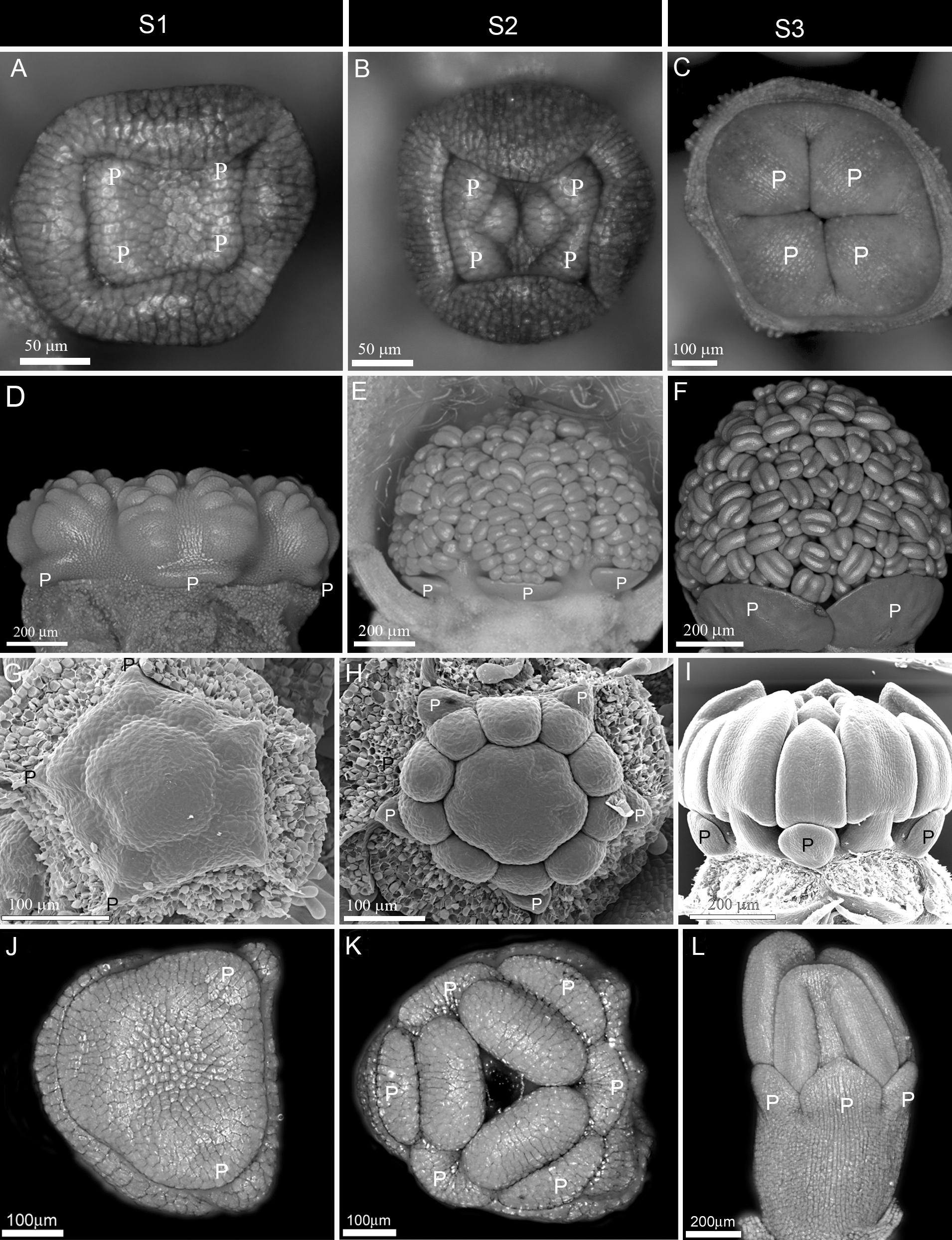
Figure 1. Early to mid-stage establishment of actinomorphy in corolla. (A–F) Simultaneous initiation followed by synchronous enlargement and differentiation in Syringa vulgaris from Oleaceae (A–C) and Alcea rosea from Malvaceae (D–F). (G–I) Non-simultaneous initiation followed by synchronous enlargement and differentiation in Zygophyllum fabago (Zygophyllaceae). (J–L) Non-simultaneous initiation of petals within each whorl followed by synchronous enlargement and differentiation in Crocus sativus (Iridaceae). P: petal, S1: Initiation phase, S2: Enlargement phase, S3: Differentiation phase.
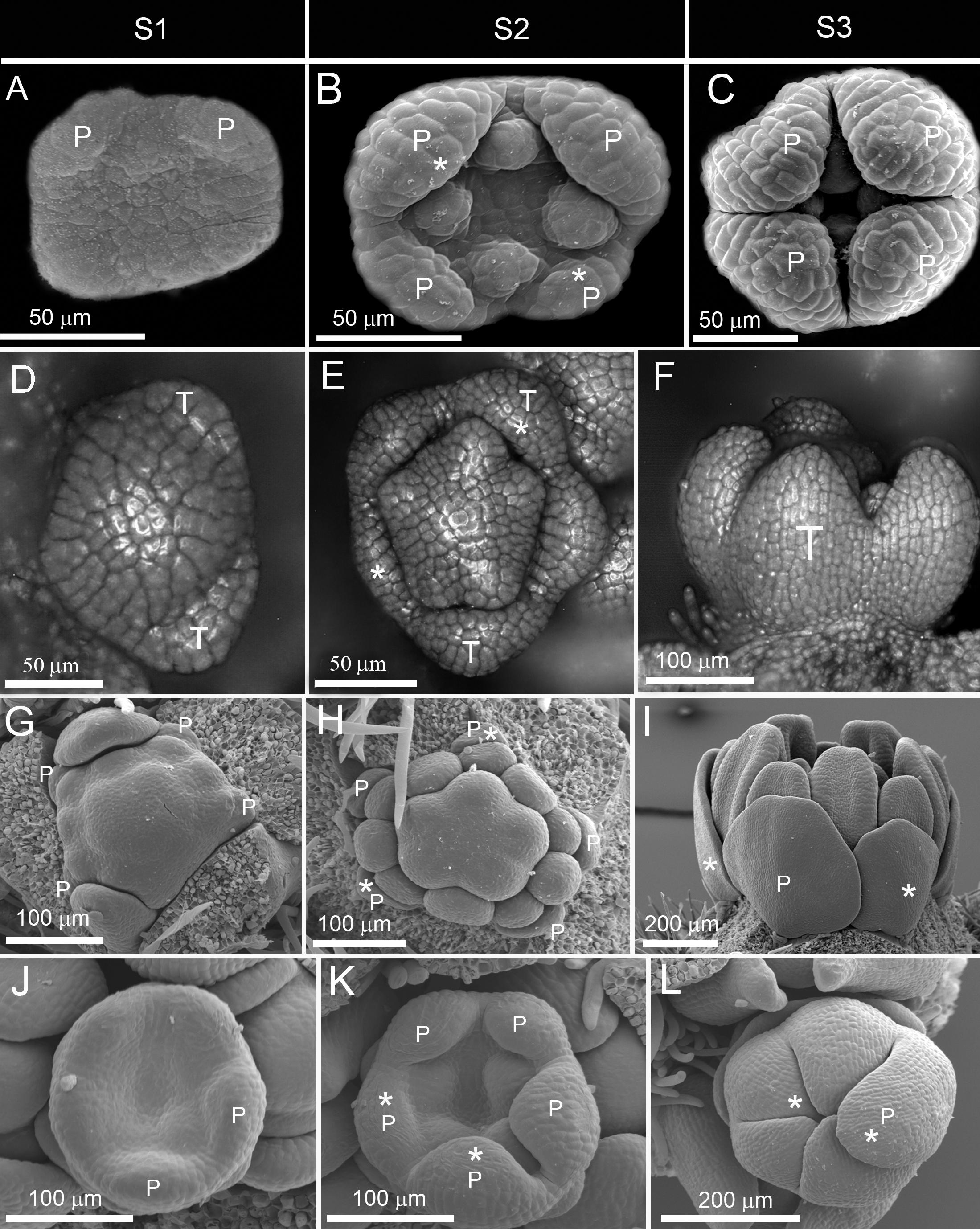
Figure 2. Late establishment of actinomorphy in corolla. (A–F) Non-simultaneous initiation followed by asynchronous enlargement and later synchronization of growth during differentiation in Rubia tinctorium from Rubiaceae (A–C) and Bougainvillea spectabilis from Nyctaginaceae (D–F). (G–L) Non-simultaneous initiation followed by asynchronous enlargement and differentiation in Larrea divaricata from Zygophyllaceae (G–I) and Patrinia gibbosa from Caprifoliaceae (J–L). P: petal, S1: initiation phase, S2: enlargement phase, S3: differentiation phase. Note the size difference between the largest and the smallest corolla members during asynchronous enlargement and differentiation (shown by stars).
(1) Early expression: The actinomorphy first appeared during petal initiation (S1) due to the simultaneous emergence of primordia (Figures 1A,D). It was maintained during development due to synchronous enlargement (S2) and differentiation (S3) (Figures 1B,C,E,F). The flowers were, therefore, actinomorphic throughout development.
(2) Mid expression: The corolla started development with a zygomorphic, non-simultaneous initiation (Figures 1G,J). However, the initial zygomorphy soon disappeared due to the synchronous enlargement and growth of corolla members after initiation (Figures 1H,I,K,L).
(3) Late expression: The corolla development started with the zygomorphic, non-simultaneous initiation of primordia (Figures 2A,D). The zygomorphy was still evident during the enlargement of organ primordia (Figures 2B,E). The synchronization of petal growth only happened during organ differentiation (Figures 2C,F).
(4) Very late expression: The corolla development started with a zygomorphic, non-simultaneous initiation (Figures 2G,J). The zygomorphy maintained during enlargement and differentiation phases due to asynchronous growth (Figures 2H,I,K,L). The flowers were, therefore, zygomorphic throughout development and actinomorphy only appeared due to equalization of organ growth in pre-anthetic flowers.
(B) Zygomorphic Species: I could distinguish three developmental patterns among 79 zygomorphic species:
(1) Early expression: The zygomorphy first appeared at organ initiation due to the sequential emergence of petal primordia (Figures 3A,D). The first initiated primordium showed the highest growth rate, followed by the second and third initiated primordia (Figures 3B,E). The size gradient was evident during enlargement and differentiation phases (Figures 3C,F). The flowers were therefore zygomorphic throughout development.
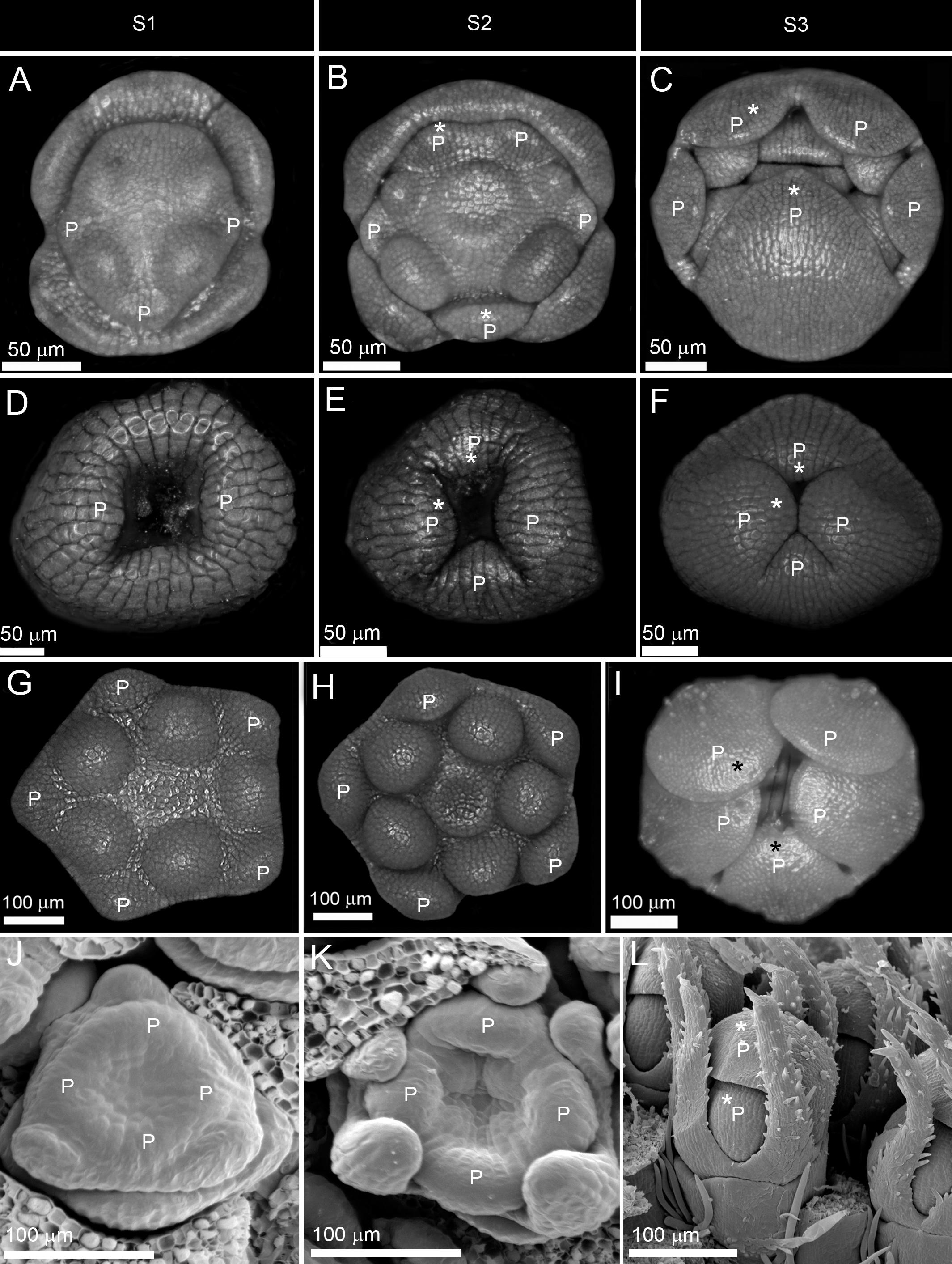
Figure 3. Early and late establishment of zygomorphy in corolla. (A–F) Non-simultaneous initiation followed by asynchronous enlargement and differentiation in Salvia nemorosa from Lamiaceae (A–C) and ray florets of Osteospermum ecklonis from Asteraceae (D–F). (G–L) Simultaneous initiation followed by synchronous enlargement and asynchronous differentiation in Verbascum thapsus from Scrophulariaceae (G–I) and Succisa pratensis from Caprifoliaceae (J–L). P: petal, S1: initiation phase, S2: enlargement phase, S3: differentiation phase. Note the size difference between the largest and the smallest corolla members during asynchronous enlargement and differentiation (shown by stars).
(2) Late expression: The corolla development started with an actinomorphic, simultaneous initiation (Figures 3G,J). The petals developed synchronously during enlargement and early differentiation phases (Figures 3H,I,K,L). The zygomorphy only appeared at the late differentiation phase prior to anthesis.
(3) The double shift of symmetry: The corolla development started with a zygomorphic, non-simultaneous initiation of primordia (Figures 4A,D,G). However, the initial zygomorphy was masked due to the equalization of primordia during enlargement phase (Figures 4B,E,H). After this period of actinomorphy, zygomorphy appeared again during the differentiation phase due to asynchronous organ growth (Figures 4C,F,I).
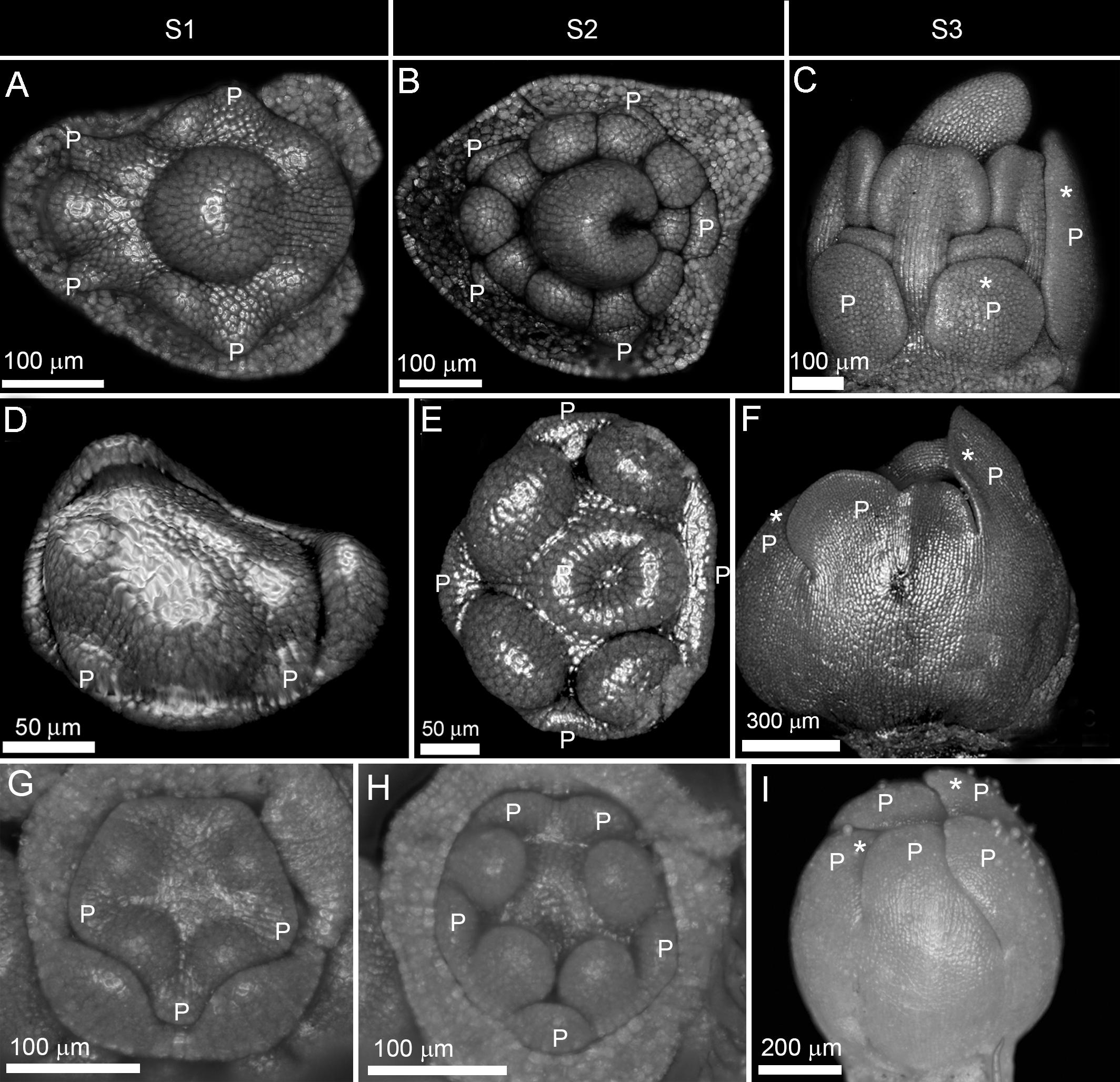
Figure 4. Double change of symmetry in zygomorphic corollas. (A–I) Non-simultaneous initiation followed by synchronous enlargement and asynchronous differentiation causing a double shift in symmetry in Astragalus compactus from Fabaceae (A–C), Rhyncocorys elephas from Orobanchaceae (D–F), and Ziziphora tenuior from Lamiaceae (G–I). P: petal, S1: initiation phase, S2: enlargement phase, S3: differentiation phase. Note the size difference between the largest and the smallest corolla members during asynchronous differentiation (shown by stars).
Evolution of Symmetry Patterns
Tracing the evolution of symmetry indicated that the ancestral state for angiosperms has been an actinomorphic flower which manifested actinomorphy at mid to late stage of development (Figure 5 and Supplementary Appendixes S2–S4). All major angiosperm clades (rosids, asterids, and monocots) were also characterized by an ancestral actinomorphic flower with a preceding early zygomorphy. The transition to early actinomorphy occurred several times in rosids (Fabaceae, Euphorbiaceae, Malvaceae- Neuradaceae clade, Cleomaceae, Rutaceae, Geraniaceae-Myrtaceae clade, and Vitaceae), asterids (Oleaceae, Rubiaceae, Solanaceae, Apiaceae, and Caprifoliaceae), eudicots (Caryophyllaceae), and basal angiosperms (Annonaceae). On the other hands, the transition to late actinomorphy also happened frequently by a shift to zygomorphy at the enlargement or differentiation phase. Such a transition was found in several families among rosids (Elaeagnaceae, Passifloraceae, and Zygophyllaceae), asterids (Plantaginaceae, Rubiaceae, Apiaceae, Caprifoliaceae, and Hydrangeaceae), eudicots (Portulacaceae, Nyctaginaceae, Phytolaccaceae, Berberidaceae, Loranthaceae, Olacaceae, and Ranunculaceae) monocots (Heliconiaceae, Arecaceae) and basal angiosperms (Lauraceae, Nymphaceae).
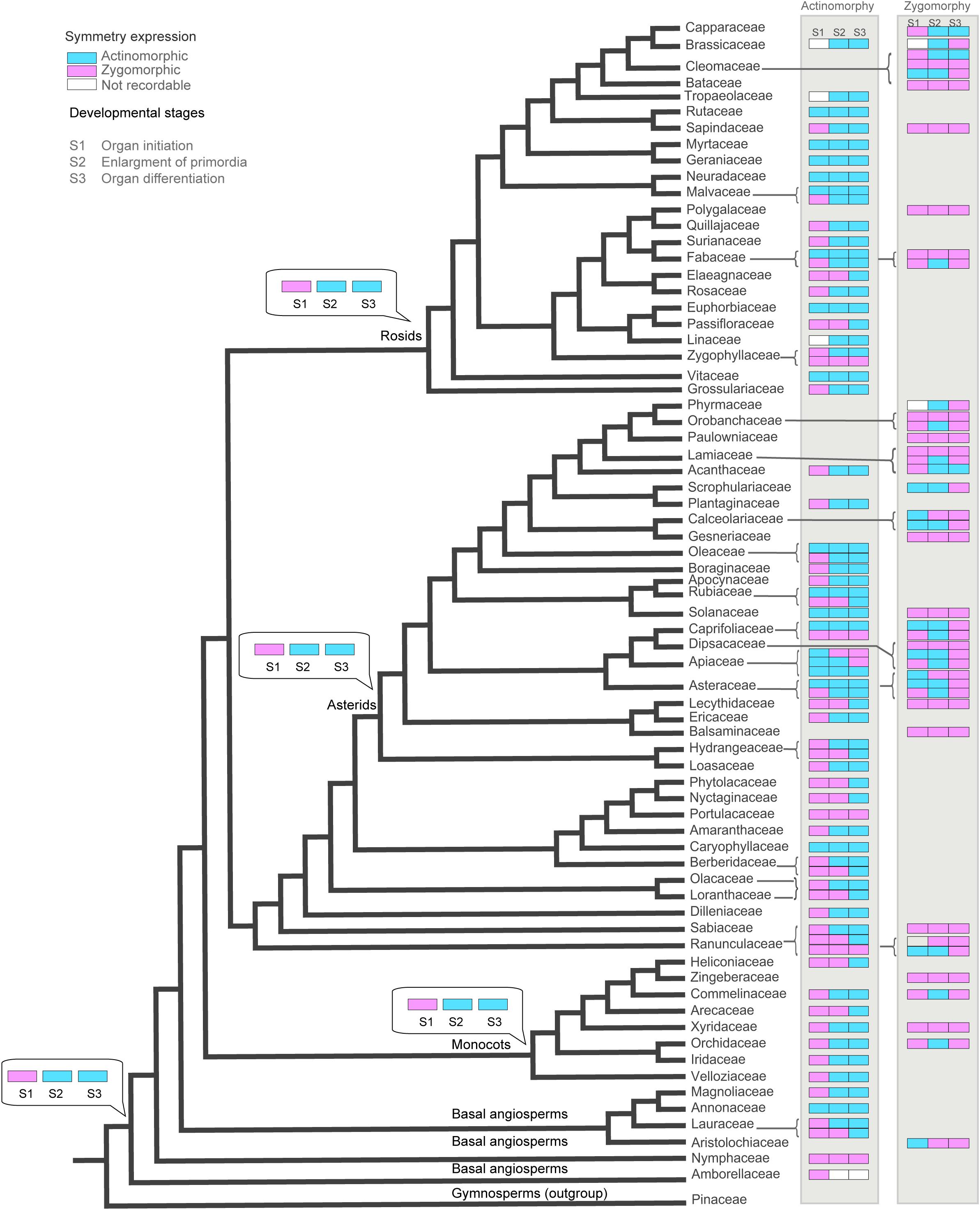
Figure 5. The evolution of corolla symmetry reconstructed on the phylogenetic tree of angiosperms (extracted from Soltis et al., 2011). The ancestral character stats (obtained under Maximum Parsimony method implemented in Mesquite) are shown for the nodes of interest.
The majority of zygomorphic flowers were derived from the ancestral state by the transition to zygomorphy during both enlargement and differentiation phases. Examples of such early zygomorphic flowers were found among rosids (Fabaceae, Bataceae, Cleomaceae, and Sapindaceae), asterids (Orobanchaceae, Paulowniaceae, Gesneriaceae, Solanaceae, Caprifoliaceae, Lecythidaceae, and Balsaminaceae), eudicots (Dilleniaceae, Sabiaceae), monocots (Xyridaceae, Zingiberaceae). However, transition to mid-late zygomorphy also happened several times among rosids (Fabaceae, Brassicaceae, and Cleomaceae), asterids (Orobanchaceae, Phrymaceae, Lamiaceae, Scrophulariaceae, Calceolariaceae, Asteraceae, and Caprifoliaceae), monocots (Commelinaceae, Orchidaceae), and basal angiosperms (Aristolochiaceae).
Discussion
Developmental Flexibility and Variation of Symmetry Patterns
Tracing developmental expression of symmetry indicated that it does not follow a fixed pattern and that the symmetry expression at each stage could be independent of the previous or the following stages. The symmetry in mature flowers is often independent of the early expression of symmetry. Regardless of being actinomorphic or zygomorphic, the majority of flowers were characterized by zygomorphic initiation which was supported as the ancestral state for the angiosperms. The results, therefore, suggest the existence of three independent steps for the establishment of symmetry in the course of ontogeny: organ initiation, enlargement, and differentiation. Hypothetically, if we consider both possible symmetry expressions (zygomorphy or actinomorphy) at each step, the combination of steps will yield eight developmental patterns (Figure 6). In agreement with this hypothesis, examples of all predicted symmetry patterns were found among angiosperms. It seems that flexibility in the developmental regulation of floral symmetry provides a broad scope for the evolution of symmetry pattern.
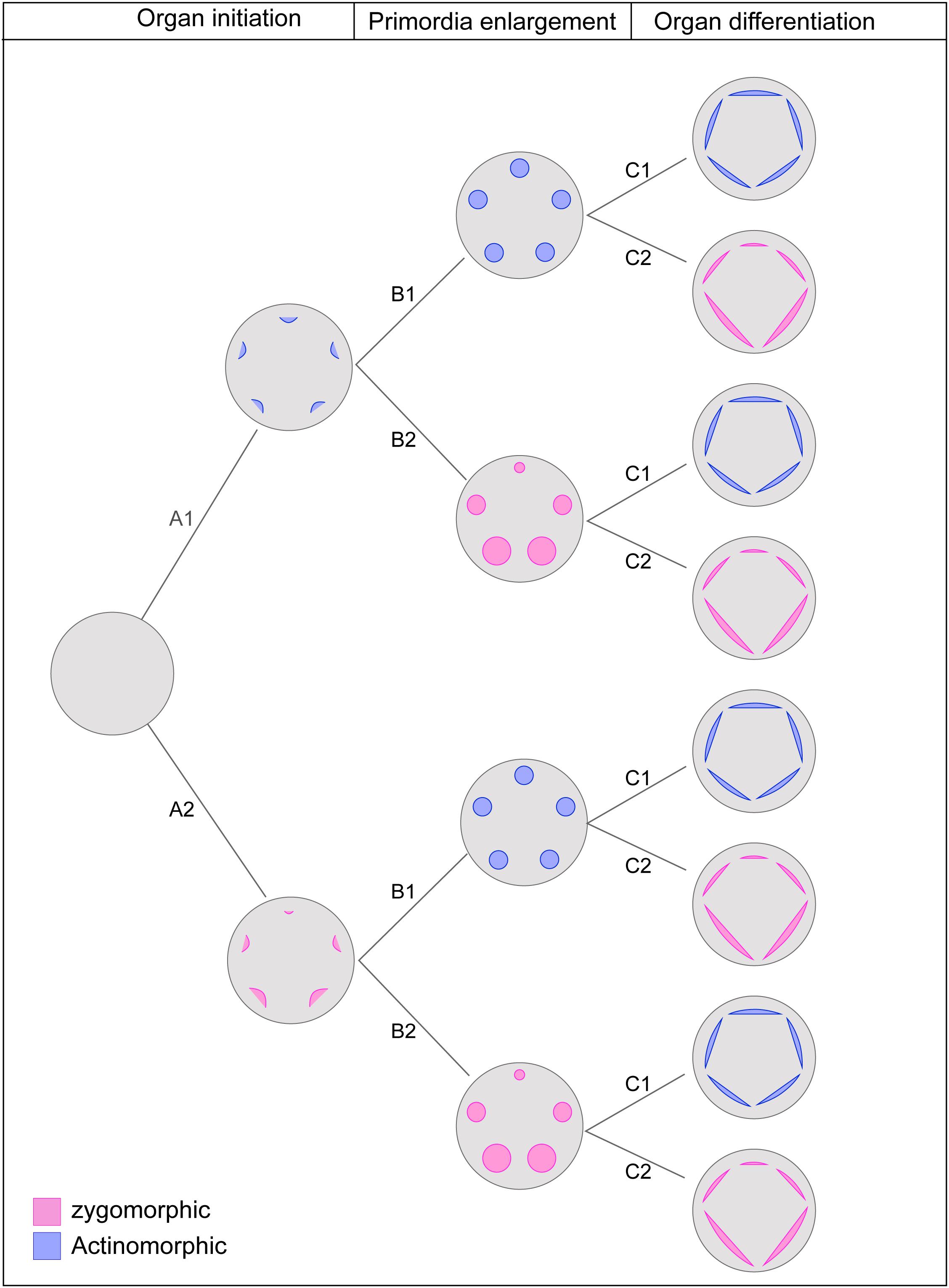
Figure 6. The theoretical pathway of symmetry establishment considering three independent developmental stages with two possible expression of actinomorphy or zygomorphy at each stage. The combination of possible changes results in eight pattern of symmetry expression: Early expression of actinomorphy: A1-B1-C1; Late expression of zygomorphy: A1-B1-C2; Late expression of actinomorphy with a shift at mid stage: A1-B2-C1; Mid-stage expression of zygomorphy: A1-B2-C2; Mid-stage expression of actinomorphy: A2-B1-C1; Late expression of zygomorphy with a shift at mid stage: A2-B2-C1; Late expression of actinomorphy: A2-B2-C1; Early expression of Zygomorphy: A2-B2-C2.
The first insights into the genetic mechanism underlying symmetry expression came from the study of model species A. majus. Two recently duplicated TCP family transcription factors, CYCLOIDEA (CYC) and DICHOTOMA (DICH), specify the dorsal floral identity (Luo et al., 1996, 1999). The expression of CYC and DICH is restricted to the dorsal region of the floral meristem, beginning from organ initiation and maintaining throughout enlargement and differentiation (Luo et al., 1996, 1999; Almeida et al., 1997). The function of both genes has been shown to be preserved in zygomorphic flowers across different clades of angiosperms (Hileman, 2014). The expression of CYC homologes has been studied in relation to the evolutionary transition from zygomorphic to actinomorphic symmetry. In Plantago, one CYC-like paralog is expressed across the dorsoventral flower axis, while the other paralog has been lost (Reardon et al., 2009; Preston et al., 2011). The combination of expanded CYC expression and loss of CYC expression, therefore, mediates the transition to a derived actinomorphy. In Cadia and Tengia, a similar expansion of one CYC paralog across the dorsoventral axis in parallel with the downregulation and loss of expression of the other paralog is suggested to be responsible for the evolution of actinomorphic flowers from zygomorphic ancestors (Citerne et al., 2006; Pang et al., 2010). In contrast, the reversal to radial symmetry in Psychopterys and Sphedamnocarpus involves the entire loss of both CYC-like paralogs (Zhang et al., 2013). An specific pattern of CYC-like paralogs is reported in derived actinomorphic flowers of Bournea (Zhou et al., 2008). Despite expanded expression of one CYC-like paralog, the other paralog is neither lost nor downregulated, but retains dorsal-specific expression at early stage (Zhou et al., 2008). Such transient dorsal-specific expression can explain the initial zygomorphic pattern in Bournea. Considering all mentioned examples, I would suggest that changes in the timing, duration, and localization of CYC-like gene expression are implicated in the developmental and evolutionary transition of floral symmetry.
Evolution of Symmetry Patterns and Developmental Constraints
Mapping the floral symmetry patterns on the phylogenetic tree indicated the scattered distribution of patterns across angiosperms. Mid-late onset of actinomorphy were found in all major clades including basal angiosperms (Lauraceae), monocots (Arecaceae and Heliconiaceae), eudicots (Ranunculaceae, Olacaceae, Loranthaceae, Berberidaceae, Phytolaccaceae, Nyctaginaceae, and Portulacaceae), asterids (Hydrangeaceae, Lecythidaceae, Caprifoliaceae, Apiaceae, Rubiaceae, and Plantaginaceae), and rosids (Zygophyllaceae, Passifloraceae, and Elaeagnaceae). The same was true for the mid-late onset of zygomorphy with representative examples in eudicots (Ranunculaceae), asterids (Caprifoliaceae, Dipsacaceae, Asteraceae, Calceolariaceae, and Scrophulariaceae), and rosids (Cleomaceae). The expression of symmetry was also versatile within families. The onset of actinomorphy in families such as Ranunculaceae, Oleaceae, Apiaceae, and Zygophyllaceae ranged from early to mid or late development depending on the taxa (see Supplementary Appendix S1). A similar variation was found within zygomorphic families. In Lamiaceae, some species like Marrubium parviflorum, Stachys byzantine, and Salvia nemorosa were zygomorphic from petal inception till anthesis, while in Phlomis armeniana a double change in symmetry pattern was found (i.e., from early zygomorphy at initiation time to actinomorphy at enlargement and back to zygomorphy at late development) (Naghiloo et al., 2013b, 2014b). Fabaceae represented another zygomorphic family in which both early expression (Amherstia nobilis, Duparquetia orchidacea) as well as the double change in symmetry pattern (Astragalus compactus, Wisteria sinensis) were found (Tucker, 2000; Prenner and Klitgaard, 2008; Naghiloo and Dadpour, 2010; Naghiloo et al., 2012). In zygomorphic flowers of Caprifoliaceae, examples of early zygomorphy (Dipsacus follunum, Knautia arvensis, and Morina persica), late zygomorphy (Succisa pratensis, Lonicera periclymenum) and shift in symmetry pattern (e.g., Lomelosia palaestina, Succisella inflexa, Cephalaria transsylvanica) were found (Naghiloo and Claßen-Bockhoff, 2017). The time of zygomorphy expression in ray flowers of Asteraceae depended on the taxa, ranging from early (e.g., Osteospermum ecklonis, Xeranthemum squarrosum) to mid or late stages of development (Senecio glabellus, Mutisia coccinea) (Harris, 1995; Dadpour et al., 2011a, 2012b).
In zygomorphic species of the otherwise radially symmetric family Ranunculaceae, the zygomorphy either expressed at an early stage through precocious initiation of some petal members (e.g., Delphinium, Naghiloo Unpublished Data) or at a late stage of development through the formation of a petal spur (Aconitum, Mair, 1977; Jabbour et al., 2009). Other examples of zygomorphy in families with predominantly actinomorphic flowers are Schizanthus and Schwenckia from Solanaceae, and Iberis amara from Brassicaceae. In the two first species, zygomorphy is apparent from the onset of organ initiation (Ampornan and Armstrong, 1988, 1989), while in Iberis amara zygomorphy establish through heterogeneous growth of petals at the late stage of development (Busch and Zachgo, 2007).
Given the parallel occurrence of symmetry patterns (early, mid or late expression) in different angiosperm clades and their variation among closely related species, the phylogenetic signal (although not tested here) seems unlikely to be the main determinant of the symmetry pattern. I would rather suggest that developmental constraints exerted by the entire inflorescence, bracts, neighbor flowers or neighbor organs within a flower could provide a possible explanation for the observed variation of symmetry expression. As an example, I refer to the correlation found between cymose monochasial inflorescences and late expression of actinomorphy in some taxa (Centranthus, Valerianaceae, Qualea, and Vochysiaceae) (Eichler, 1878). In Patrinia, which expresses actinomorphy at a very late stage of development, the flowers are arranged in dichasia with monochasial peripheral braches. In early floral development, the two petals and stamens on the side toward the weaker subsequent ramification of the dichasium appear earlier than their counterparts (Hofmann and Gottmann, 1990). Beside the ramification pattern of inflorescence, the mechanical forces generated by subtending leaves or bracts can also influence the order of organ initiation (Naghiloo et al., 2012). An association between order of initiation and the presence of bracteoles has been suggested for some members of Papilionoideae (Prenner, 2004).
Another example of developmental constraints which can influence the pattern of symmetry expression is found in species with a double change of floral symmetry (i.e., from zygomorphy to actinomorphy and back to zygomorphy). The size gradient in mature zygomorphic flowers often follows the initiation sequence; organs that are initiated first are usually the largest at maturity and the pattern of early symmetry is maintained throughout development. However, in some taxa (e.g., Papilionoideae, Lamiaceae, Orobanchaceae, Dipsacaceae) the size gradient in mature petals is opposite to the direction of their initiation; the last initiated petal is the largest one at maturity. In such cases, a period of synchronization is required before the new size gradient establishes through differential growth. The mismatch between the direction of zygomorphy in early and late stages could, therefore, explain double changes of symmetry during development.
Data Availability Statement
The datasets generated for this study is available as Supplementary Material online.
Author Contributions
SN performed all aspects of this research.
Conflict of Interest
The author declares that the research was conducted in the absence of any commercial or financial relationships that could be construed as a potential conflict of interest.
Supplementary Material
The Supplementary Material for this article can be found online at: https://www.frontiersin.org/articles/10.3389/fevo.2020.00104/full#supplementary-material
References
Almeida, J., Rocheta, M., and Galego, L. (1997). Genetic control of flower shape in Antirrhinum majus. Development 124, 1387–1392.
Ampornan, L., and Armstrong, J. (1988). The floral ontogeny of Schizanthus, a zygomorphic member of the Solanaceae. Am. J. Bot. 75:54.
Ampornan, L., and Armstrong, J. (1989). The floral ontogeny of Salpiglossis, a zygomorphic member of the Solanaceae. Am. J. Bot. 76:64.
Busch, A., and Zachgo, S. (2007). Control of corolla monosymmetry in the Brassicaceae Iberis amara. Proc. Natl. Acad. Sci. U.S.A. 104, 16714–16719. doi: 10.1073/pnas.0705338104
Citerne, H., Jabbour, F., Nadot, S., and Damerval, C. (2010). “The evolution of floral symmetry,” in Advances in Botanical Research, eds J.-C. Kader and M. Delseny (Cambridge, MA: Academic Press), 85–137. doi: 10.1016/s0065-2296(10)54003-5
Citerne, H., Toby Pennington, R., and Cronk, Q. (2006). An apparent reversal in floral symmetry in the legume Cadia is a homeotic transformation. Proc. Natl. Acad. Sci. U.S.A. 103, 12017–12020. doi: 10.1073/pnas.0600986103
Dadpour, M. R, Naghiloo, S., Gohari, G., and Aliakbari, M. (2011a). Inflorescence and floral ontogeny in Osteospermum ecklonis L. (Asteraceae). Botany 89, 605–614. doi: 10.1139/b11-052
Dadpour, M. R., Naghiloo, S., Peighambardoust, S. H., Panahirad, S., Aliakbari, M., and Movafeghi, A. (2011b). Comparison of floral ontogeny in wild-type and double-flowered phenotypes of Syringa vulgaris L. (Oleaceae). Sci. Horticult. 127, 535–541. doi: 10.1016/j.scienta.2010.12.004
Dadpour, M. R., Naghiloo, S., and Gohari, G. (2012a). Inflorescence and floral ontogeny in Crocus sativus L. (Iridaceae). Flora 27, 257–263. doi: 10.1016/j.flora.2012.02.001
Dadpour, M. R., Naghiloo, S., and Neycharan, S. F. (2012b). The development of pistillate and perfect florets in Xeranthemum squarrosum (Asteraceae). Plant Biol. 14, 234–243. doi: 10.1111/j.1438-8677.2011.00469.x
Endress, P. (2010). Evolution of floral symmetry. Curr. Opin. Plant Biol. 4, 86–91. doi: 10.1016/s1369-5266(00)00140-0
Endress, P. K. (1999). Symmetry in flowers: diversity and evolution. Int. J. Plant Sci. 160, S3–S23.
Endress, P. K. (2012). The immense diversity of floral monosymmetry and asymmetry across angiosperms. Bot. Rev. 78, 345–397. doi: 10.1007/s12229-012-9106-3
Endress, P. K., and Doyle, J. A. (2009). Reconstructing the ancestral angiosperm flower and its initial specializations. Am. J. Bot. 96, 22–66. doi: 10.3732/ajb.0800047
Harris, E. M. (1995). Inflorescence and floral ontogeny in Asteraceae: A synthesis of historical and current concepts. Bot. Rev. 61:93. doi: 10.1007/bf02887192
Hileman, L. C. (2014). Trends in flower symmetry evolution revealed through phylogenetic and developmental genetic advances. Philos. Trans. R. Soc. Lond. B Biol. Sci. 369:20130348. doi: 10.1098/rstb.2013.0348
Hofmann, U., and Gottmann, J. (1990). Morina L. und Triplostegia Wall. ex DC. im Vergleich mit Valerianaceae und Dipsacaceae. Bot. Jahrb. Syst. 111, 499–553.
Jabbour, F., Ronse De Craene, L. P., Nadot, S., and Damerval, C. (2009). Establishment of zygomorphy on an ontogenic spiral and evolution of perianth in the tribe Delphinieae (Ranunculaceae). Ann. Bot. 104, 809–822. doi: 10.1093/aob/mcp162
Luo, D., Carpenter, R., Copsey, L., Vincent, C., Clark, J., and Coen, E. (1999). Control of organ asymmetry in flowers of Antirrhinum. Cell 99, 367–376. doi: 10.1016/s0092-8674(00)81523-8
Luo, D., Carpenter, R., Vincent, C., Copsey, L., and Coen, E. (1996). Origin of floral asymmetry in Antirrhinum. Nature 383, 794–799. doi: 10.1038/383794a0
Maddison, W. P., and Maddison, D. R. (2015). Mesquite: A Modular System for Evolutionary Analysis. Available online at: Version 3.04. http://mesquiteproject.org (accessed July, 2019).
Mair, O. (1977). Zur Entwicklungsgeschichte monosymmetrischer Dicotylen-Blüten. Diss. Bot. 38, 260–274.
Naghiloo, S., and Classen-Bockhoff, R. (2016). Developmental analysis of merosity and sexual morphs in Rubiaceae: A case study in Rubia and Cruciata. Flora 222, 52–59. doi: 10.1016/j.flora.2016.03.010
Naghiloo, S., and Claßen-Bockhoff, R. (2017). Developmental changes in time and space promote evolutionary diversification of flowers: A case study in Dipsacoideae. Front. Plant Sci. 8:1665–1665. doi: 10.3389/fpls.2017.01665
Naghiloo, S., and Dadpour, M. (2010). Floral ontogeny in Wisteria sinensis (Fabaceae: Faboideae: Millettieae) and its systematic implications. Aust. Syst. Bot. 23, 393–400. doi: 10.1071/SB10027
Naghiloo, S., Dadpour, M. R., and Movafeghi, A. (2012). Floral ontogeny in Astragalus compactus (Leguminosae: Papilionoideae: Galegeae): variable occurrence of bracteoles and variable patterns of sepal initiation. Planta 235, 793–805. doi: 10.1007/s00425-011-1538-1
Naghiloo, S., Esmaillou, Z., and Dadpour, M. R. (2014a). Comparative floral ontogeny of single-flowered and double-flowered phenotypes of Alcea rosea (Malvaceae). Aust. J. Bot. 62, 217–228. doi: 10.1071/BT14070
Naghiloo, S., Khodaverdi, M., Nikzat Siahkolaee, S., and Dadpour, M. R. (2014b). Comparative floral development in Lamioideae (Lamiaceae): Marrubium, Phlomis, and Stachys. Plant Syst. Evol. 300, 1269–1283. doi: 10.1007/s00606-013-0960-1
Naghiloo, S., Esmaillou, Z., Gohari, G., and Dadpour, M. (2013a). Comparative inflorescence and floral ontogeny in the genus Mentha (Mentheae: Nepetoideae: Lamiaceae): Variable sequences of organ appearance and random petal aestivation. Plant Syst. Evol. 300, 329–345. doi: 10.1007/s00606-013-0885-8
Naghiloo, S., Khodaverdi, M., Esmaillou, Z., Dadpour, M., and Rudall, P. (2013b). Comparative floral development in the tribe Mentheae (Nepetoideae: Lamiaceae) and its bearing on the evolution of floral patterns in asterids. J. Syst. Evol. 52, 195–214. doi: 10.1111/jse.12072
Naghiloo, S., Reza Fathollahi, M., and Claßen-Bockhoff, R. (2015). Flower ontogeny in Capparis spinosa (Capparaceae) with special emphasis on symmetry expression. Nordic J. Bot. 33, 754–760. doi: 10.1111/njb.00768
Pang, H., Sun, Q. W., He, S. Z., and Wang, Y.-Z. (2010). Expression pattern of CYC-like genes relating to a dorsalized actinomorphic flower in Tengia (Gesneriaceae). J. Syst. Evol. 48, 309–317. doi: 10.1111/j.1759-6831.2010.00091.x
Prenner, G. (2004). New aspects in floral development of Papilionoideae: initiated but suppressed bracteoles and variable initiation of sepals. Ann. Bot. 93, 537–545. doi: 10.1093/aob/mch076
Prenner, G., and Klitgaard, B. (2008). Towards unlocking the deep nodes of Leguminosae: Floral development and morphology of the enigmatic Duparquetia orchidacea (Leguminosae, Caesalpinioideae). Am. J. Bot. 95, 1349–1365. doi: 10.3732/ajb.0800199
Preston, J. C., Martinez, C. C., and Hileman, L. C. (2011). Gradual disintegration of the floral symmetry gene network is implicated in the evolution of a wind-pollination syndrome. Proc. Natl. Acad. Sci. U.S.A. 108, 2343–2348. doi: 10.1073/pnas.1011361108
Reardon, W., Fitzpatrick, D. A., Fares, M. A., and Nugent, J. M. (2009). Evolution of flower shape in Plantago lanceolata. Plant Mol. Biol. 71, 241–250. doi: 10.1007/s11103-009-9520-z
Reyes, E., Sauquet, H., and Nadot, S. (2016). Perianth symmetry changed at least 199 times in angiosperm evolution. Taxon 65, 945–964. doi: 10.12705/655.1
Soltis, D. E., Smith, S. A., Cellinese, N., Wurdack, K. J., Tank, D. C., Brockington, S. F., et al. (2011). Angiosperm phylogeny: 17 genes, 640 taxa. Am. J. Bot. 98, 704–730. doi: 10.3732/ajb.1000404
Tucker, S. C. (1999). Evolutionary lability of symmetry in early floral development. Int. J. Plant Sci. 160, S25–S39.
Tucker, S. C. (2000). Floral development in Tribe Detarieae (Leguminosae: Caesalpinioideae): Amherstia, Brownea, and Tamarindus. Am. J. Bot. 87, 1385–1407. doi: 10.2307/2656867
Tucker, S. C. (2003). Floral development in legumes. Plant Physiol. 131, 911–926. doi: 10.1104/pp.102.017459
Zhang, W., Steinmann, V. W., Nikolov, L., Kramer, E. M., and Davis, C. C. (2013). Divergent genetic mechanisms underlie reversals to radial floral symmetry from diverse zygomorphic flowered ancestors. Front. Plant. Sci. 4:302. doi: 10.3389/fpls.2013.00302
Keywords: symmetry expression, angiosperms, developmental constraints, actinomorphy, zygomorphy
Citation: Naghiloo S (2020) Patterns of Symmetry Expression in Angiosperms: Developmental and Evolutionary Lability. Front. Ecol. Evol. 8:104. doi: 10.3389/fevo.2020.00104
Received: 25 September 2019; Accepted: 30 March 2020;
Published: 28 April 2020.
Edited by:
Louis Philippe Ronse De Craene, Royal Botanic Garden Edinburgh, United KingdomReviewed by:
Sophie Nadot, Université Paris-Sud, FranceErik Smets, Naturalis Biodiversity Center, Netherlands
Copyright © 2020 Naghiloo. This is an open-access article distributed under the terms of the Creative Commons Attribution License (CC BY). The use, distribution or reproduction in other forums is permitted, provided the original author(s) and the copyright owner(s) are credited and that the original publication in this journal is cited, in accordance with accepted academic practice. No use, distribution or reproduction is permitted which does not comply with these terms.
*Correspondence: Somayeh Naghiloo, c29tYXllaF9uYWdoaWxvb0B5YWhvby5jb20=