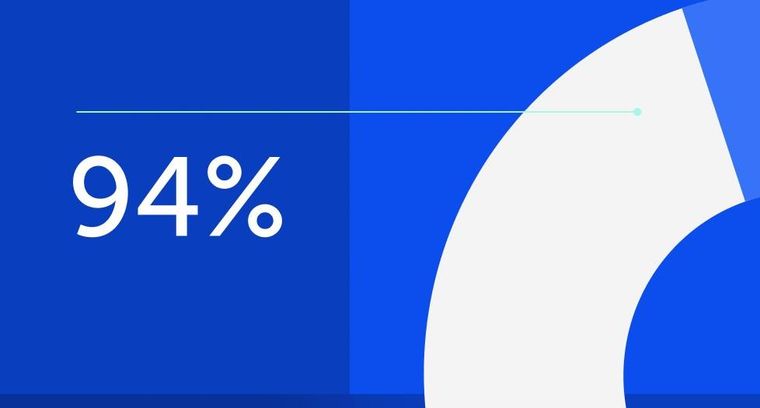
94% of researchers rate our articles as excellent or good
Learn more about the work of our research integrity team to safeguard the quality of each article we publish.
Find out more
ORIGINAL RESEARCH article
Front. Ecol. Evol., 27 March 2020
Sec. Conservation and Restoration Ecology
Volume 8 - 2020 | https://doi.org/10.3389/fevo.2020.00072
This article is part of the Research TopicUnderstanding the Impact and Invasion Success of Aquatic Non-native Species: How they Interact with Novel Environments and Native BiotaView all 11 articles
It is essential to incorporate mechanisms of biotic resistance into predictions of ecological impact conferred by invasive species. Trophically and functionally analogous native species have high potential to confer biotic resistance or to be susceptible to competitive exclusion by the invading species. In species with dominance hierarchies and high aggression the role of weapons, such as chelae, is emphasised and selected for. Differences in traits such as crushing capacity can indicate prey handling capabilities, while correlations between closing force and morphology can be used to understand the role of signaling in agonistic contests. Closing force strength can be used to infer the outcomes of both direct (predation) and indirect (competition) trophic interactions. Southern Africa has been invaded by two freshwater crayfish species (Cherax quadricarinatus and Procambarus clarkii). Biotic resistance of freshwater crabs toward crayfish invasions varies between geographic location and co-evolutionary history, thus comparing invasion histories without incorporating geographic context can produce unequivocal conclusions. We compared the closing force and chelae morphology of both crayfish species with a native trophically analogous freshwater crab, Potamonautes perlatus. Closing force increased significantly with mass for all species. There was significant interaction between sex and species on closing force. Potamonautes perlatus females showed significantly stronger maximum chela closing force than male P. perlatus, both sexes of P. clarkii and female C. quadricarinatus. Contrastingly, male C. quadricarinatus had significantly higher closing forces than both sexes of P. clarkii and female C. quadricarinatus, however, there was no difference between female P. perlatus. Native P. perlatus has the capacity to hold a competitive mechanical advantage over both invaders, but this varies with sex. Chelae length was not a significant predictor for closing strength in any of the species, which may be related to dishonest signaling in decapod species. This makes it imperative to assess whether factors such as closing force actually translate to resource holding potential in a contest scenario. We thus provide evidence that African freshwater crabs may exhibit biotic resistance toward invasion and the first measurements for C. quadricarinatus closing force strength.
Non-native species introductions and subsequent establishment continues to threaten global biodiversity (Seebens et al., 2017; Meyerson et al., 2019). The likelihood of a particular species successfully establishing and persisting in a novel environment is influenced by both abiotic and biotic factors (Byers, 2002; Pearson et al., 2018; Kinney et al., 2019). For example, evidence toward the environmental matching hypothesis suggests that when the climate of the incumbent ecosystem matches the climate of the native range, the invader has a higher potential to both persist and perform better than when the climate does not (Capinha et al., 2013; Iacarella et al., 2015). The community assemblage of the invaded system can also facilitate or impede establishment via biotic resistance (deRivera et al., 2005; Alofs and Jackson, 2014). Theoretically, areas with high species richness are more likely to have species that are strong competitors or, indeed, predators, which make the system less invadable than those with low species richness (Elton, 1958; Tilman, 1999). Although, in areas where species are functionally similar there is a likelihood that the analogous native species are outcompeted or niche excluded by the invader (Dick et al., 2017; Zeng et al., 2019).
Species interactions shape community dynamics through consumptive effects, but also in non-consumptive manners such as intraspecific and interspecific competition for resources such as food, shelter, and reproduction (Sih et al., 2010; Lopez et al., 2019; Mofu et al., 2019; Zeng et al., 2019). Biotic resistance can thus be exhibited in both consumptive and competitive manners, however, in freshwater systems biotic resistance is driven overwhelmingly by consumption (Alofs and Jackson, 2014). Aquatic environments exhibit a higher occurrence of generalist feeding and omnivory leading to a lack of intraspecific and interspecific competition (Alofs and Jackson, 2014). Moreover, dietary plasticity and frequency dependent predation (i.e., prey switching) are common traits of successful invaders as it allows persistence of species via trophic niche separation and capacity to consume new resources when one is over-exploited (Snyder and Evans, 2006; Olsson and Nyström, 2009; Havel et al., 2015). Often, successful invasive species also outcompete and competitively exclude native species for resources through agonistic interactions, though, aggressiveness does not always equate to resource holding potential (Camerlink et al., 2015; Lopez et al., 2019).
Freshwater crayfishes are highly successful invaders, having established widespread invasive populations. While the impacts, both positive and negative, have been reported for various crayfish species (see review by Lodge et al., 2012), the mechanisms that drive species persistence and increased impact are still somewhat unclear. The African continent is devoid of native crayfish species. This is potentially due to evolutionary competition with freshwater crabs (Ortmann, 1902; Lodge et al., 2012; Nunes et al., 2017a). Freshwater crabs of the genus Potamonautes are, however, present in almost all African freshwater habitats where they are trophically analogous to freshwater crayfish and provide essential nutrient cycling services (Hill and O'Keeffe, 1992; Dobson, 2004; Cumberlidge and Daniels, 2009; Peer et al., 2015). Potamonautid species typically exhibit high degrees of endemism and range restriction. These traits make them vulnerable to the impacts of habitat destruction and invasive species introductions (Cumberlidge and Daniels, 2009; Zeng and Yeo, 2018). Crabs and crayfish are polytrophic benthic omnivores that are both opportunistic scavengers and direct predators (Hill and O'Keeffe, 1992; Grey and Jackson, 2012). Due to the trophic similarities between crayfish and freshwater crabs it is likely that they will either provide an important component of biotic resistance or be competitively excluded by crayfish invasions (Lodge et al., 2012; Dick et al., 2017). Indeed, in Tanzania the red swamp crayfish, Procambarus clarkii (Girard, 1852) has replaced a native freshwater crab Potamonautes neumannii in many systems (Ogada, 2007), and has replaced Potamonautes loveni as the primary food source in African clawless otter diets in Lake Naivasha (Ogada et al., 2009). Similar trends are reported in Singapore where Australian redclaw crayfish Cherax quadricarinatus competitively excludes smaller native freshwater crabs from shelter resources (Zeng et al., 2019). In Cyprus the invasive P. clarkii shows shelter holding dominance over native freshwater crab Potamon potamios despite both species being equally aggressive (Savvides et al., 2015). Contrastingly, the European river crab Potamon fluviatile shows dominance in aggression and resource holding capacity toward the invasive P. clarkii but this may be driven by co-evolutionary history with the native Astropotamobius italicus crayfish (Cioni and Gherardi, 2004; Mazza et al., 2017). Again, there are similar reports that populations of native pseudothelphusid crabs persisting despite the C. quadricarinatus invasion in Mexico, likely due to the co-evolutionary history with native crayfish (Bortolini et al., 2007). Predicting the impacts of invasive crayfish species on native biota via consumptive and non-consumptive effects by way of comparing invasion histories can thus produce unequivocal conclusions due to geographical context.
Southern Africa is suffering from an over-invasion scenario by functionally similar crayfish species which have been introduced primarily through aquaculture ventures and the pet trade (Lodge et al., 2012; Russell et al., 2014; Nunes et al., 2017a,b; Weyl et al., 2020). Cherax quadricarinatus, the Australian redclaw crayfish and P. clarkii both have established invasive populations in South Africa (Nunes et al., 2017a,b). Cherax quadricarinatus is also present in Zambia (Nakayama et al., 2010; Nunes et al., 2016), Swaziland (Nunes et al., 2017a) and Zimbabwe (Marufu et al., 2018) while P. clarkii is invasive in Zambia, Uganda, Kenya, Egypt, Sudan, and Rwanda (Hobbs et al., 1989; Mikkola, 1996; Cumberlidge, 2009). Both species are likely to spread into ecologically and economically integral streams and wetlands, which will potentially threaten the stability of aquatic systems that provide refuge habitat for imperilled species such as Potamonautid crabs (Ahyong and Yeo, 2007; Belle et al., 2011; Nunes et al., 2017a,b; Zeng et al., 2019). To test whether there could be some degree of biotic resistance exhibited by African freshwater crabs, we measure and compare the maximum closing force of native Potamonautes perlatus to the invasive crayfish C. quadricarinatus and P. clarkii. Weapon performance can usually be assumed to be an honest signal and is correlated with aggression (Lappin and Husak, 2005; Wilson et al., 2007; Bywater and Wilson, 2012). We hypothesised that the invasive crayfish species would have a higher maximum closing force than P. perlatus. Closing force can indicate prey handling capacity (Meers, 2002; Miranda et al., 2016), but also, if closing force is related to morphology it can contribute to important signaling in agonistic contests (Wilson et al., 2007; Bywater et al., 2008; Bywater and Wilson, 2012). Thus, we also determined whether morphological and biological factors can be used to predict closing force.
Cherax quadricarinatus is native to Northern Australia and southeastern New Guinea. Populations have established in southern African freshwater systems where they were introduced and are spreading (Nunes et al., 2016, 2017a; Douthwaite et al., 2018). Cherax quadricarinatus tolerates a wide variety of habitats and environmental conditions (Masser and Rouse, 1997). One hundred and fifty live C. quadricarinatus samples (mean ± sd carapace length: 63.20 ± 4.93 mm, mass: 67.34 ± 11.26 g) were collected from sugarcane irrigation ponds in Nkomazi, Komatipoort (Mpumalanga Province) supplied by the Komati River (S 25.55°, E 31.90°). Komatipoort has been invaded by C. quadricarinatus since 2002 and represents an invasion core for the species in South Africa (de Moor, 2002; De Villiiers, 2015). A standard gear for trapping the redclaw crayfish was used, this consists of Promar collapsible traps baited with dry dog food. Traps were deployed at 1,600 h and retrieved at 800 h. Permits to transport and keep C. quadricarinatus were issued by the DEA (Permit Numbers: 50869181001115242, 50869181001120608). The crayfish caught were transported to a biosecure facility at the South African Institute for Aquatic Biodiversity (SAIAB) in insulated cooler boxes with source water from the dam and constantly aerated with battery pumps.
Procambarus clarkii is native to southern and south-eastern USA and northern Mexico. In Africa, P. clarkii was introduced to South Africa, Zambia, Uganda, Kenya, Egypt, Sudan, and Rwanda (Hobbs et al., 1989; Mikkola, 1996; Cumberlidge, 2009). Procambarus clarkii lives in a variety of freshwater habitats, including lakes, ponds, rivers, canals, streams, seasonally flooded swamps and marshes, and ditches with mud or sand substrata and plenty of organic debris (Huner and Barr, 1991). Fifty-six live P. clarkii crayfish samples (carapace length: 58.62 ± 6.86 mm, mass: 59.54 ± 7.05 g) were collected from Mimosa Dam (S 27.88°, E 26.69°) in Free State Province South Africa, where there has been a recent invasion ((DEA), 2018) using traps baited with fish heads and dry dog food. Traps were deployed at 1,600 h and retrieved at 800 h. Mimosa Dam represents an invasion core population of P. clarkii. The crayfish caught were transported from Mimosa Dam to the biosecure facility at SAIAB in insulated cooler boxes with source water from the dam and constantly aerated with battery pumps. Permits to transport and keep P. clarkii were issued by the Department of Environmental Affairs (DEA) (Permit Numbers: 50869181001113030, 50869181002121045).
The same gear was also used to trap P. perlatus samples from Eastern Cape Dams (S 33.32°, E 26.52°; S 33.32°, E 26.52°; S 33.29°, E 26.51°; S 33.41°, E 26.50°). Traps were deployed at 1,600 h and retrieved at 800 h. Permits to sample crabs were issued by the Eastern Cape Department of Economic Development, Environmental Affairs and Tourism (CRO 19/18CR and CRO 21/18CR). Larger crabs were selected to most closely match the mass of the crayfishes, however, there were few females caught in the traps. Twenty crabs (carapace length: 53.27 ± 4.55 mm, mass: 96.29 ± 22.15 g) were caught and placed in 60 L cooler boxes with fresh dam water with battery powered air pumps and transported to the biosecure facility at SAIAB.
Not all collected animals were used in this experiment as they were in use for other research purposes at the time, therefore the animals used were a random subsample of the total collected animals (Table 1). Animals were maintained in species specific and sex specific holding tanks (60 L) with constantly filtered and aerated aged tap water which was replaced twice a week to maintain good water quality. Water temperature was maintained at 23 ± 1°C by a computer controlled recirculating heating: cooling air conditioner unit. The laboratory was held under a 12:12 light:dark regime. All animals were maintained on cabbage leaves, broad leaved pondweed Potamogeton nodosus and fennel-leaved pond weed Stockenia pectinatus and cultured Eisenia sp. worms.
Table 1. Median and range of morphometric values and closing force (N) values for P. perlatus, C. quadricarinatus and P clarkii females and males.
Individual animals were selected from the holding tank haphazardly and patted dry before measurements were taken. Animals were weighed (to the nearest g), the chelae length (propodus) of both left and right chela, and cephalothorax length were measured with Vernier calipers (to the nearest mm), and sexed (Table 1). Animals with regenerated claws and females with eggs were excluded due to differences in energy conservation.
Chelae closing force measurements were completed in vivo in the laboratory using the Kistler system and the protocol outlined in Herrel et al. (1999), Singh et al. (2000), and Lailvaux et al. (2009). Animals were allowed to grip from the proximal region of the chela onto plates set at a gap of 6 mm, to allow comparison with Miranda et al. (2016). Closing force (N) was measured five times per individual, per chela, resulting in 10 measurements taken for each individual. Animals were given a rest period of 5 min between measurements. The laboratory, and holding tank water, were both held at (23 ± 1°C) throughout the entire acclimation and experimentation time.
Differences in mass and cephalothorax length between species was determined using one way non-parametric Kruskal-Wallis tests and Dunn test post-hoc, with p-values adjusted for multiple comparisons using Holm-Bonferroni corrections. Handedness was assessed separately for each species, by comparing left and right chela closing force measurements using Friedmans test to account for repeated measures.
Closing force data were log-transformed and linear mixed-effects models were fitted, based on maximum likelihood. Initially the models were fitted separately to each left and right chelae. It was assumed that chela length would affect closing force, and to account for some individuals having asymmetrical chelae lengths between the left and right claws, we first ran a model to account for this rather than taking data from only one chela. Fixed factors were “species,” “mass,” “sex” and “chela length,” while “individual identity” was used as a random factor nested within “species.” A full model was fitted, containing “species,” “mass,” “sex” and “chela length,” and their interactions. Chela length was not a significant predictor of closing force so was removed from the model (see results) and only the maximum closing force obtained per individual was selected, regardless of left or right chelae. Cephalothorax length was significantly correlated with mass and thus not included in the analysis (Figure 1). If either the fixed factors, or the interaction did not have a significant effect, in subsequent steps the model was re-run with non-significant terms removed, starting with the interaction term. The final model only contained significant terms to obtain the most parsimonious model. Differences were calculated post-hoc using χ2 to communicate effect size. All analyses were performed in an R environment (R Core Team, 2018), using the package nlme (Pinheiro et al., 2018).
Figure 1. Linear regressions and SE of Log10 mass (g) and Log10 Cephalothorax length (mm) of P. perlatus, C. quadricarinatus and P. clarkii.
There were significant differences in mass of each species (χ2 = 39.03, df = 2, p < 0.001; Table 1, Figure 1), where P. clarkii weighed less than both C. quadricarinatus (z = 5.83, p < 0.001; Table 1, Figure 1) and P. perlatus (z = 4.11, p < 0.001; Table 1, Figure 1), however there was no difference between P. perlatus and C. quadricarinatus mass (z = 0.08, p = 0.93; Table 1, Figure 1).
Potamonautes perlatus had significantly stronger right chela closing force than left (Friedmans χ2 = 15.05, df = 1, p < 0.0001; Supplementary Figures S1, S2). Contrastingly, both C. quadricarinatus and P. clarkii had significantly stronger left chela closing force (respectively: Friedmans χ2 = 5.68, df = 1, p < 0.05, Friedmans χ2 = 5.09, df = 1, p < 0.05; Supplementary Figures S1, S2). Female P. perlatus had a higher left and right chela closing force than males (Table 1, Figure 2) whereas, male C. quadricarinatus had a higher left and right chela closing force than females (Table 1, Figure 2). There were no sex differences in left or right chela closing force in P. clarkii (Table 1, Figure 2). Chelae length did not affect closing force in any species on either left or right chelae (left: χ2 = 0.19, df = 1, p = 0.65; right: χ2 = 0.83, df = 1, p = 0.36).
Figure 2. Median maximum closing force (N) of left and right chelae of female and male P. perlatus, C. quadricarinatus, and P. clarkii. Individual points represent raw data. Boxplots represent median value and 25–75% interquartile range.
There was a significant interaction between species and sex on maximum closing force (Table 2, Figure 3; Supplementary Figure S3). Species and sex both had significant main effects on closing fsorce (Table 2, Figure 3; Supplementary Figure S3). Closing force increased significantly with mass for all species (Table 2, Figure 3; Supplementary Figure S3). Opposite trends in maximum closing force were seen between male and female P. perlatus and C. quadricarinatus, however there was no difference between male and female closing force in P. clarkii (p = 0.46). Female P. perlatus had a significantly stronger closing force than male P. perlatus, female C. quadricarinatus, and both sexes of P. clarkii (all p < 0.01). There was no difference between female P. perlatus closing strength and male C. quadricarinatus or between male P. perlatus and female C. quadricarinatus (both p > 0.05). However, male C. quadricarinatus had significantly higher maximum closing forces than male P. perlatus (p < 0.01). Male P. perlatus had significantly stronger maximum closing forces than both sexes of P. clarkii (all p < 0.01). There was no difference between maximum closing force of P. clarkii females and C. quadricarinatus females (p = 0.58).
Table 2. Model terms for all factors from a linear mixed effects model used to determine differences in maximum closing force (N) with regards to fixed factors “species,” “sex,” and “mass,” using a Type 3 Anova and χ2 to report the effect size of a factor on the dependent variable.
Figure 3. Predicted maximum closing force correlation with mass (g) of female (left panel) and male (right panel) P. perlatus, C. quadricarinatus, and P. clarkii with 95% confidence intervals. Individual points indicate raw data points for each species.
Determining what makes systems resilient to biological invasion is a many faceted challenge (Holling, 1973; Havel et al., 2015). Nonetheless, by understanding basic differences in physical capacity it is possible to infer how performance can relate to species interaction outcomes within an invasion scenario (Griffen and Mosblack, 2011). We predicted that the invasive crayfish species would have a higher maximum closing force than native crabs, however, this proved to be unequivocal at least when considering animals of the same size. Given that there have been reports of invasive crayfish reducing abundance of trophically analogous decapods (Ogada, 2007; Ogada et al., 2009; Zeng et al., 2019), these results suggest that P. perlatus has the capacity to hold a competitive mechanical advantage over both invaders, but that this varies with sex. Unfortunately, our results are based on a small sample size of native P. perlatus which may confound results with regards to sex based differences. Nonetheless, there was a more even amount of males and females amongst smaller P. perlatus individuals (50–80 g) whereas the heavier individuals (>100 g) were predominantly female. Our results indicate that there are other mechanisms at play that may cause freshwater crabs to be competitively excluded by invasive crayfish species, rather than brute strength. Although, population size structure, growth rates, and maximum attainable mass of each species will affect competitive exclusion. For example, larger individuals will have a higher resource holding potential over smaller individuals of any species. Resultingly, it is possible to determine maximum chelae strength for these species through correlation with mass and sex but it is unclear as to whether chelae strength can actually be a predictor for resource holding potential or success in agonistic contests.
Closing force relates to ability of an individual to pinch down onto a subject. Whether this is for direct predation, during agonistic contests, reproductive purposes, or even to withstand abiotic disturbances such as high flow rates (Gherardi, 2002; Ion et al., 2019). It can be assumed that the larger the closing force the more damage may be conferred to the recipient despite high force conferring a high energy cost to the individual (Herrel et al., 1999; Wilson et al., 2007). Our results of chela closing force fall well within the range reported for P. perlatus (18–598 N) (Miranda et al., 2016), and for P. clarkii (males: 1.35 ± 0.41 N/g; females: 2.22 ± 0.89 N/g; see Supplementary Figure 3 for N/g results) (Claussen et al., 2007; Malavé et al., 2018). Similar to our results (Claussen et al., 2007) and Malavé et al. (2018) found sexual dimorphism in chela length where male P. clarkii had longer chela than females but this did not relate to closing force. This is likely related to reproductive activities and the cost of signaling during male-male contests (Stein, 1976). Contrastingly, P. perlatus females were stronger than males indicating a difference in resource holding potential between sexes and species. These differences may also be exacerbated in a natural setting when individuals are in or out of reproductive status. For example, female P. clarkii are more aggressive and more successful when they are maternal compared to non-maternal females and males (Peeke et al., 1995; Figler et al., 2005). Another native African crab species (Potamonautes sidneyi) had a significantly weaker closing force than P. perlatus (8–43 N) (Miranda et al., 2016). There is no prior published data on the closing force of C. quadricarinatus, but for comparative purposes the closing force of the largest terrestrial arthropod, the coconut crab (Birgus latro), is 29.4–1765.2 N (Oka et al., 2016), of which the lower ranges all overlap with the three species in the present study. In a first attempt to incorporate C. quadricarinatus into impact assessments, Zeng et al. (2019), show that larger bodied C. quadricarinatus have a competitive advantage over a native freshwater crab (Parathelphusa maculata) when competing for shelter space.
The presence of heterochely or “cutter vs. crusher” is well-established in marine decapods but it is less evident in freshwater crayfish species (Govind, 1989; Schenk and Wainwright, 2001; Lele and Pârvulescu, 2019). In essence it describes potential handedness between left and right chelae. Potamonautes perlatus had stronger right chela, whereas C. quadricarinatus and P. clarkii had stronger left chela. As claw length was not an effective predictor of strength it suggests a degree of ambidexterity between left and right chelae in all of these species as a response to likelihood of losing chelae during agonistic bouts (Kouba et al., 2011; Lele and Pârvulescu, 2019). The lack of strong morphometric predictors reinforces the concept of dishonest signaling in crayfish species (Wilson et al., 2007; Malavé et al., 2018). This combination of potential dishonest signaling and opposite trends in dominant chelae could be a factor in competition between crab and crayfish species despite P. perlatus females dominating mechanically. Potential for biotic resistance, either consumptive or competitive, is likely to be species specific and still further regulated by other biotic and abiotic parameters (deRivera et al., 2005). In this case, size mis-matches, differential spatial ecology, abundance, and type of resources present in a system are all possible factors that could be driving the likelihood of biotic resistance.
Freshwater crabs and crayfish are polytrophic keystone consumers that occupy an unusually large dietary niche breadth as a result of generalist feeding strategies (Jackson et al., 2014, 2016). Chelae are regularly used in decapod feeding to assist in subduing, capturing, holding, and manipulating resource items (Loya-Javellana et al., 1993; Mariappan et al., 2000). This is particularly important when considering durophagous feeding, as crushing predators are thus limited by their strength to process prey items such as snails, but also limited by the shell resistance and predator induced phenotype changes (DeWitt et al., 2000; Evers et al., 2011; Miranda et al., 2016). There is limited information on crush resistance of African gastropod species, but the invasive snail Tarebia granifera has a resistance of 100 ± 6 (mean ± se) N, while native Melanoides tuberculata has a resistance of 31 ± 4 N (Miranda et al., 2016). However, in its invasive range in Lake Malawi M. tuberculata has a crush resistance range of 18.63–94.73 N (Evers et al., 2011). Bulinus globosus and Bulinus nyassanus are native gastropods of Lake Malawi, which have crush resistance ranges of 2.29–4.79 N and 8.33–117.82 N, respectively (Evers et al., 2011). All three of the species represented in this study, besides female P. clarkii, have the capacity to handle all of these gastropod species. The relatively high crush resistance of the invaders suggests that this may facilitate their persistence in a system, however it should also be considered that if the southern African crayfish invasion persists there could be an invasion meltdown scenario where predation is concentrated on the native gastropods and facilitates population expansion of the invasive gastropods (Ricciardi, 2001; Simberloff, 2006). An invasion meltdown scenario may also be facilitated in the wild by Potamonautes sp. via differences in biotic resistance. Although, the cost-benefit of undertaking crushing activities for food, rather than reproductive efforts, should be investigated as it is likely that predators select for forage with low handling demands (Murdoch, 1969; Behrens Yamada and Boulding, 1998). The present study focuses on the relative differences in closing force with respect to resource utilisation and non-consumptive competition but direct predation by either crabs or crayfish upon heterospecific juveniles is also likely. Therefore, addressing actual contest outcomes and consumption rates between different sized individuals of each species would further our understanding of competitive interactions.
Handling vegetation and crushing prey items require differences in closing force and dentition patterns (Sibbing, 1991; Herrel et al., 1999). Thus, differences in closing force could also relate to niche separation between the species when they occur in sympatry, which could in turn facilitate species persistence of both natives and invaders. Procambarus clarkii exhibit this pattern of niche breadth reduction when found in sympatry with P. loveni, where they affect leaf litter breakdown due to direct consumption (Jackson et al., 2016; Nishijima et al., 2017), possibly related to differences in chela morphology (Sibbing, 1991). Further, P. clarkii is capable of exerting predatory pressure on planktonic prey items which do not need strong crushing capacity to handle (South et al., 2019). Little work has been completed on the diet and trophic niche of C. quadricarinatus in either its invasive or native range. Nonetheless, Marufu et al. (2018) found the main diet components of the Lake Kariba C. quadricarinatus population to be predominantly macrophytes, detritus and macroinvertebrates. In Lake Kariba the trophic niche of crayfish differed with size class, wherein macroinvertebrate consumption increased with size, which could potentially be due to the positive relationship between mass and closing force (Marufu et al., 2018). Comparative functional morphology of decapod chelae and feeding apparatus should thus be incorporated into invasion risk assessments as ecomorphology can help to predict impact (Nagelkerke et al., 2018).
Due to the complex nature of trophic interactions and food web structuring, particularly within a stochastic aquatic environment, it is difficult to determine mechanisms of biotic resistance in situ (Havel et al., 2015). Comparing species traits is a first step in assessing whether native species will exhibit some degree of either competitive or consumptive resistance toward invaders (Funk et al., 2008; Kumschick and Richardson, 2013; Zeng et al., 2015). The results presented here indicate that chelae closing force can be predicted by body mass and sex of the individual for all three decapod species but in order to correctly predict biotic resistance these must be validated further by assessing actual resource holding potential. Consequently, when trait based analysis should be complemented with other predictive assessments such as the comparative functional response and relative impact potential metrics (Dick et al., 2017; Dickey et al., 2018; South et al., 2019; but see Vonesh et al., 2017), but also with contest based experiments (Lopez et al., 2019; Zeng et al., 2019). Unfortunately, there is a severe paucity of data on the ecological impact of C. quadricarinatus but also on the basic ecology of Potamonautid crabs in southern Africa. Further, the specific dynamics of invasion and the recipient system can mediate trait expression in populations across the invasion gradient, whereupon crayfish at the invasion front can be more aggressive (Pintor et al., 2009), or they have smaller and less heavy chela as a response to reduced competition (Messager and Olden, 2019). Therefore, a considerable amount of baseline assessment (i.e., abundance, size structure, fecundity, distribution, and diet) is needed to be completed in order to effectively assess the risk that both invasive crayfish species pose toward functionally similar and ecologically important species such as freshwater crabs. Further, assessing actual interaction frequency and habitat or resource use overlap between different sized native and invasive decapod species would determine the potential degree of biotic resistance in the environment.
The raw data generated and used in the analysis are publicly available at https://doi.org/10.6084/m9.figshare.11993961.
This research was given ethics clearance by the Animal Ethics Subcommittee, Rhodes University (Ethics No. DIFS2718) and SAIAB Ethics Committee (#25/4/1/7/5_2018_06).
JS, TM, JM, and OW conceived the study. JM provided the equipment. OW provided the funding. JS, NT, and TM conducted the experiments. JS analysed the data and wrote the first draft. JS, TM, NT, JM, and OW all contributed toward manuscript editing and final manuscript.
This study was funded by the National Research Foundation (NRF)—South African Research Chairs Initiative of the Department of Science and Innovation (DSI) (Inland Fisheries and Freshwater Ecology, Grant No. 110507).
The authors declare that the research was conducted in the absence of any commercial or financial relationships that could be construed as a potential conflict of interest.
We acknowledge use of infrastructure and equipment provided by the NRF-SAIAB Research Platform and the funding channelled through the NRF-SAIAB Institutional Support system. We are grateful to Andre Hoffman from Mpumalanga Parks and Tourism Agency (MTPA) and Dr. Leon Barkhuizen from Free State Department of Economic, Small Business Development, Tourism, and Environmental Affairs (FS DESTEA) for assisting the collection of redclaw and red swamp crayfish, respectively. Any opinion, finding, and conclusion or recommendation expressed in this material is that of the authors. The NRF of South Africa does not accept any liability in this regard.
The Supplementary Material for this article can be found online at: https://www.frontiersin.org/articles/10.3389/fevo.2020.00072/full#supplementary-material
Supplementary Figure 1. Median maximum closing force (N) per mm of chelae right chelae for P. perlatus, C. quadricarinatus, and P. clarkii. Individual points represent raw data. Boxplots represent median value and 25–75% interquartile range.
Supplementary Figure 2. Median maximum closing force (N) per mm of chelae left chelae for P. perlatus, C. quadricarinatus, and P. clarkii. Individual points represent raw data. Boxplots represent median value and 25–75% interquartile range.
Supplementary Figure 3. Median maximum closing force (N) per g of animal for P. perlatus, C. quadricarinatus, and P. clarkii. Individual points represent raw data. Boxplots represent median value and 25–75% interquartile range.
Ahyong, S. T., and Yeo, D. C. J. (2007). Feral populations of the Australian red-claw crayfish (Cherax quadricarinatus von Martens) in water supply catchments of Singapore. Biol. Invasions. 9, 943–946. doi: 10.1007/s10530-007-9094-0
Alofs, K. M., and Jackson, D. A. (2014). Meta-analysis suggests biotic resistance in freshwater environments is driven by consumption rather than competition. Ecology 95, 3259–3270. doi: 10.1890/14-0060.1
Behrens Yamada, S., and Boulding, B. E. G. (1998). Claw morphology, prey size selection and foraging efficiency in generalist and specialist shell-breaking crabs. J. Exp. Mar. Biol. Ecol. 220, 191–211. doi: 10.1016/S0022-0981(97)00122-6
Belle, C. C., Wong, J. Q. H., Yeo, D. C. J., Tan, S. H., Tan, H. H., Clews, E., et al. (2011). Ornamental trade as a pathway for Australian -redclaw crayfish introduction and establishment. Aquat. Biol. 12, 69–79. doi: 10.3354/ab00324
Bortolini, J. L., Alvarez, F., and Rodríguez-Almaraz, G. (2007). On the presence of the Australian redclaw crayfish, Cherax quadricarinatus, in Mexico. Biol. Invasions. 9, 615–620. doi: 10.1007/s10530-006-9054-0
Byers, J. E. (2002). Physical habitat attribute mediates biotic resistance to non-indigenous species invasion. Oecologia 130, 146–156. doi: 10.1007/s004420100777
Bywater, C., Angilletta, M., and Wilson, R. (2008). Weapon size is a reliable indicator of strength and social dominance in female slender crayfish (Cherax dispar). Funct. Ecol. 22, 311–316. doi: 10.1111/j.1365-2435.2008.01379.x
Bywater, C. L., and Wilson, R. S. (2012). Is honesty the best policy? Testing signal reliability in fiddler crabs when receiver-dependent costs are high. Funct. Ecol. 26, 804–811. doi: 10.1111/j.1365-2435.2012.02002.x
Camerlink, I., Turner, S. P., Farish, M., and Arnott, G. (2015). Aggressiveness as a component of fighting ability in pigs using a game-theoretical framework. Anim. Behav. 108, 183–191. doi: 10.1016/j.anbehav.2015.07.032
Capinha, C., Brotons, L., and Anastácio, P. (2013). Geographical variability in propagule pressure and climatic suitability explain the European distribution of two highly invasive crayfish. J. Biogeogr. 40, 548–558. doi: 10.1111/jbi.12025
Cioni, A., and Gherardi, F. (2004). Agonism and interference competition in freshwater decapods. Behaviour 141:1297–1324. doi: 10.1163/1568539042729702
Claussen, D. L., Gerald, G. W., Kotcher, J. E., and Miskell, C. A. (2007). Pinching forces in crayfish and fiddler crabs, and comparisons with the closing forces of other animals. J. Comp. Physiol. B. 178, 333–342. doi: 10.1007/s00360-007-0226-8
Cumberlidge, N. (2009). “Freshwater crabs and shrimps (crustacea: decapoda) of the Nile Basin,” in The Nile. Origin, Environments, Limnology and Human Use, Monographiae Biologicae, Vol. 89, ed H. J. Dumont (New York, NY: Springer), 547–561.
Cumberlidge, N., and Daniels, S. R. (2009). A conservation assessment of the freshwater crabs of southern Africa (Brachyura: Potamonautidae). Afr. J. Ecol. 46, 74–79. doi: 10.1111/j.1365-2028.2007.00815.x
de Moor, I. (2002). Potential impacts of alien freshwater crayfish in South Africa. Afr. J. Aquat. Sci. 27, 125–139. doi: 10.2989/16085914.2002.9626584
De Villiiers, M. (2015). Freshwater Crayfish Found in Komati River. Lowvelder (accessed July 08, 2015).
Department of Environmental Affairs (DEA) (2018). Discovery of Alien and Highly Invasive Freshwater Crayfish in the Free State Goldfields. Available online at: https://www.environment.gov.za/mediarelease/freestategoldfields_freshawater_invasivecrayfish (accessed October 10, 2018).
deRivera, C. E., Ruiz, G. M., Hines, A. H., and Jivoff, P. (2005). Biotic resistance to invasion: native predator limits abundance and distribution of an introduced crab. Ecology 86, 3364–3376. doi: 10.1890/05-0479
DeWitt, T. J., Robinson, B. W., and Wilson, D. S. (2000). Functional diversity among predators of a freshwater snail imposes an adaptive trade-off for shell morphology. Evol. Ecol. Res. 2, 129–148. Available online at: https://www.researchgate.net/publication/228495044_Functional_diversity_among_predators_of_a_freshwater_snail_imposes_an_adaptive_trade-off_for_shell_morphology
Dick, J. T. A., Alexander, M. E., Ricciardi, A., Laverty, C., Downey, P. O., Xu, M., et al. (2017). Functional responses can unify invasion ecology. Biol. Invasions. 19, 1667–1672. doi: 10.1007/s10530-016-1355-3
Dickey, J. W. E., Cuthbert, R. N., Rea, M., Laverty, C., Crane, K., South, J., et al. (2018). Assessing the relative potential ecological impacts and invasion risks of emerging and future invasive alien species. NeoBiota 40, 1–24. doi: 10.3897/neobiota.40.28519
Dobson, M. (2004). Freshwater crabs in Africa. Freshwater Forum 21, 3–26. Available online at: http://aquaticcommons.org/id/eprint/4685
Douthwaite, R. J., Jones, E. W., Tyser, A. B., and Vrdoljak, S. M. (2018). The introduction, spread and ecology of redclaw crayfish Cherax quadricarinatus in the Zambezi catchment. Afr. J. Aquat. Sci. 43, 353–366. doi: 10.2989/16085914.2018.1517080
Evers, B. N., Madsen, H., and Stauffer, J. R. Jr. (2011). Crush-resistance of soft-sediment gastropods of Lake Malawi: implications for prey selection by Molluscivorous fishes. J. Freshw. Ecol. 26, 85–90. doi: 10.1080/02705060.2011.553921
Figler, M. H., Blank, G. S., and Peeke, H. V. S. (2005). Shelter competition between resident male red swamp crayfish Procambarus clarkii (Girard) and conspecific intruders varying by sex and reproductive status. Mar. Freshw. Behav. Physiol. 38, 237–248. doi: 10.1080/10236240500376477
Funk, J. L., Cleland, E. E., Suding, K. N., and Zavaleta, E. S. (2008). Restoration through reassembly: plant traits and invasion resistance. Trends Ecol. Evol. 23, 695–703. doi: 10.1016/j.tree.2008.07.013
Gherardi, F. (2002). “Behaviour,” in Biology of Freshwater Crayfish, ed D. M. Holdich (Oxford: Blackwell Science), 258–290.
Grey, J., and Jackson, M. C. (2012). ‘Leaves and Eats Shoots’: direct terrestrial feeding can supplement invasive red swamp crayfish in times of need. PLoS ONE 7:e42575. doi: 10.1371/journal.pone.0042575
Griffen, B. D., and Mosblack, H. (2011). Predicting diet and consumption rate differences between and within species using gut ecomorphology. J. Anim. Ecol. 80, 854–863. doi: 10.1111/j.1365-2656.2011.01832.x
Havel, J. E., Kovalenko, K. E., Thomaz, S. M., Amalfitano, S., and Kats, L. B. (2015). Aquatic invasive species: challenges for the future. Hydrobiologia 750, 147–170. doi: 10.1007/s10750-014-2166-0
Herrel, A., Spithoven, L., Van Damme, R., and De Vree, F. (1999). Sexual dimorphism of head size in Gallotia galloti: testing the niche divergence hypothesis by functional analyses. Funct. Ecol. 13, 289–297. doi: 10.1046/j.1365-2435.1999.00305.x
Hill, M. P., and O'Keeffe, J. H. (1992). Some aspects of the ecology of the freshwater crab (Potamonautes perlatus Milne Edwards) in the upper reaches of the Buffalo River, Eastern Cape province South Africa. Southern Afr. J. Aquat. Sci. 18, 42–50. doi: 10.1080/10183469.1992.9631323
Hobbs, H. H., Jass, J. P., and Huner, J. V. (1989). A review of global crayfish introductions with particular emphasis on two North American species. Crustaceana 56, 299–316. doi: 10.1163/156854089X00275
Holling, C. S. (1973). Resilience and stability of ecological systems. Ann. Rev. Ecol. Syst. 4, 1–23. doi: 10.1146/annurev.es.04.110173.000245
Huner, J. V., and Barr, J. E. (1991). Red Swamp Crayfish, Biology and Exploitation, 3rd Edn. Louisiana: Louisiana State University, Baton Rouge.
Iacarella, J. C., Dick, J. T. A., Alexander, M. E., and Ricciardi, A. (2015). Ecological impacts of invasive alien species along temperature gradients: testing the role of environmental matching. Ecol. Appl. 25, 706–716. doi: 10.1890/14-0545.1
Ion, M. C., Puha, A. E., Suciu, T., and Pârvulescu, L. (2019). Get a grip: unusual disturbances drive crayfish to improvise. Behaviour 1, 1–20. doi: 10.1163/1568539X-00003583
Jackson, M. C., Grey, J., Miller, K., Britton, J. R., and Donohue, I. (2016). Dietary niche constriction when invaders meet natives: evidence from freshwater decapods. J. Anim. Ecol. 85, 1098–1107. doi: 10.1111/1365-2656.12533
Jackson, M. C., Jones, T., Milligan, M., Sheath, D., Taylor, J., Ellis, A., et al. (2014). Niche differentiation among invasive crayfish and their impacts on ecosystem structure and functioning. Freshw. Biol. 59, 1123–1135. doi: 10.1111/fwb.12333
Kinney, K. A., Pintor, L. M., and Byers, J. E. (2019). Does predator-driven, biotic resistance limit the northward spread of the non-native green porcelain crab, Petrolisthes armatus? Biol. Invasions. 21, 245–260. doi: 10.1007/s10530-018-1821-1
Kouba, A., Burič, M., Policar, T., and Kozák, P. (2011). Evaluation of body appendage injuries to juvenile signal crayfish (Pacifastacus leniusculus): relationships and consequences. Knowl. Manag. Aquat. Ecosyst. 401:05. doi: 10.1051/kmae/2011012
Kumschick, S., and Richardson, D. M. (2013). Species-based risk assessments for biological invasions: advances and challenges. Divers. Distrib. 19, 1095–1105 doi: 10.1111/ddi.12110
Lailvaux, S. P., Reaney, L. T., and Backwell, P. R. Y. (2009). Dishonest signalling of fighting ability and multiple performance traits in the fiddler crab Uca mjoebergi. Funct. Ecol. 23, 359–366. doi: 10.1111/j.1365-2435.2008.01501.x
Lappin, A. K., and Husak, J. F. (2005). Weapon performance, not size, determines mating success and potential reproductive output in the collared lizard (Crotaphytus collaris). Am. Nat. 166, 426–436. doi: 10.1086/432564
Lele, S., and Pârvulescu, L. (2019). Crayfish chelae usage suggests predominantly ambidextrous habitude, Crustaceana 92, 257–267. doi: 10.1163/15685403-00003886
Lodge, D. M., Deines, A., Gherardi, F., Yeo, D. C. J., Arcella, T., Baldridge, A. K., et al. (2012). Global introductions of crayfishes: evaluating the impact of species invasions on ecosystem services. Annu. Rev. Ecol. Evol. Syst. 43, 449–472. doi: 10.1146/annurev-ecolsys-111511-103919
Lopez, L. K., Hendry, K., Wong, M. Y. L., and Davis, A. R. (2019). Insight into invasion: interactions between a critically endangered and invasive crayfish. Austral. Ecol. 44, 78–85. doi: 10.1111/aec.12654
Loya-Javellana, G. N., Fielder, D. R., and Thorne, M. J. (1993). Food choice by free-living stages of the tropical freshwater crayfish, Cherax quadricarinatus (Parastacidae: Decapoda). Aquaculture 118, 299–308. doi: 10.1016/0044-8486(93)90464-A
Malavé, B. M., Styga, J. M., and Clotfelter, E. D. (2018). Size, shape, and sex-dependent variation in force production by crayfish chelae. J. Morphol. 279, 312–318. doi: 10.1002/jmor.20773
Mariappan, P., Balasundaram, C., and Schmitz, B. (2000). Decapod crustacean chelipeds: an overview. J. Biosci. 25, 301–313. doi: 10.1007/BF02703939
Marufu, L., Dalu, T., Crispen, P., Barson, M., Simango, R., Utete, B., et al. (2018). The diet of an invasive crayfish, Cherax quadricarinatus (Von Martens, 1868), in Lake Kariba, inferred using stomach content and stable isotope analyses. BIR 7, 121–132. doi: 10.3391/bir.2018.7.2.03
Masser, M. P., and Rouse, D. B. (1997). Australian Red Claw Crayfish. Southern Regional Aquaculture Center. SRAC Publication No. 244.
Mazza, G., Tricarico, E., Cianferoni, F., Stasolla, G., Inghilesi, A. F., Zoccola, A., et al. (2017). Native crab and crayfish co-occurrence: first evidence in Europe. Biologia 72, 790–795. doi: 10.1515/biolog-2017-0086
Meers, M. B. (2002). Maximum bite force and prey size of Tyrannosaurus rex and their relationships to the inference of feeding behavior. Hist. Biol. 16, 1–12. doi: 10.1080/0891296021000050755
Messager, M. L., and Olden, J. D. (2019). Phenotypic variability of rusty crayfish (Faxonius rusticus) at the leading edge of its riverine invasion. Freshw. Biol. 64, 1196–1209. doi: 10.1111/fwb.13295
Meyerson, L. A., Carlton, J. T., Simberloff, D., and Lodge, D. M. (2019). The growing peril of biological invasions. Front. Ecol. Environ. 17, 191–191. doi: 10.1002/fee.2036
Mikkola, H. (1996). Alien freshwater crustacean and indigenous mollusc species with aquaculture potential in Eastern and Southern Africa. Southern Afr. J. Aquat. Sci. 22, 90–99. doi: 10.1080/10183469.1996.9631375
Miranda, N. A. F., Measey, G. J., Peer, N., Raw, J. L., Perissinotto, R., and Appleton, C. C. (2016). Shell crushing resistance of alien and native thiarid gastropods to predatory crabs in South Africa. Aquat. Invasions 11, 303–311. doi: 10.3391/ai.2016.11.3.08
Mofu, L., South, J., Wasserman, R. J., Woodford, D. J., Dick, J. T. A., and Weyl, O. L. F. (2019). Inter-specific differences in invader and native fish functional responses illustrate neutral effects on prey but superior invader competitive ability. Freshw. Biol. 64, 1655–1663. doi: 10.1111/fwb.13361
Murdoch, W. W. (1969). Switching in general predators: experiments on predator specificity and stability of prey populations. Ecol. Monogr. 39, 335–354. doi: 10.2307/1942352
Nagelkerke, L. A. J., Onselen, E., van Kessel, N., and van Leuven, R. S. E. W. (2018). Functional feeding traits as predictors of invasive success of alien freshwater fish species using a food-fish model. PLoS ONE. 13:e0197636. doi: 10.1371/journal.pone.0197636
Nakayama, S. M. M., Ikenaka, Y., Muzandu, K., Choongo, K., Oroszlany, B., Teraoka, H., et al. (2010). Heavy metal accumulation in lake sediments, fish (Oreochromis niloticus and Serranochromis thumbergi), and crayfish (Cherax quadricarinatus) in Lake Itezhi-tezhi and Lake Kariba, Zambia. Arch. Environ. Contam. Toxicol. 59, 291–300. doi: 10.1007/s00244-010-9483-8
Nishijima, S., Nishikawa, C., and Miyashita, T. (2017). Habitat modification by invasive crayfish can facilitate its growth through enhanced food accessibility. BMC Ecol. 17:37. doi: 10.1186/s12898-017-0147-7
Nunes, A. L., Douthwaite, R. J., Tyser, B., Measey, G. J., and Weyl, O. L. F. (2016). Invasive crayfish threaten Okavango Delta. Front. Ecol. Environ. 14, 237–238. doi: 10.1002/fee.1287
Nunes, A. L., Hoffman, A. C., Zengeya, T. A., Measey, G. J., and Weyl, O. L. F. (2017b). Red swamp crayfish, Procambarus clarkii, found in South Africa 22 years after attempted eradication: red swamp crayfish in South Africa. Aquat. Conserv. Mar Freshw Ecosyst. 27, 1334–1340. doi: 10.1002/aqc.2741
Nunes, A. L., Zengeya, T. A., Measey, G. J., and Weyl, O. L. F. (2017a). Freshwater crayfish invasions in South Africa: past, present and potential future. Afr. J. Aquat. Sci. 42, 309–323. doi: 10.2989/16085914.2017.1405788
Ogada, M. O., Aloo, P. A., and Muruthi, P. M. (2009). The African clawless otter Aonyx capensis (Schinz, 1821) and its diet as an indicator of crayfish invasion dynamics in aquatic systems. Afr. J. Ecol. 47, 119–120 doi: 10.1111/j.1365-2028.2007.00910.x
Oka, S.-i., Tomita, T., and Miyamoto, K. (2016). A mighty claw: pinching force of the coconut crab, the largest terrestrial crustacean. PLoS ONE 11:e0166108. doi: 10.1371/journal.pone.0166108
Olsson, K., and Nyström, P. (2009). Non-interactive effects of habitat complexity and adult crayfish on survival and growth of juvenile crayfish (Pacifastacus leniusculus). Freshw. Biol. 54, 35–46. doi: 10.1111/j.1365-2427.2008.02089.x
Ortmann, A. E. (1902). The geographical distribution of freshwater decapods and its bearing upon ancient geography. Proc. Am. Philos. Soc. 41, 267–400 doi: 10.5962/bhl.title.16020
Pearson, D. E., Ortega, Y. K., Eren, Ö., and Hierro, J. L. (2018). Community assembly theory as a framework for biological invasions. Trends Ecol. Evol. 33, 313–325. doi: 10.1016/j.tree.2018.03.002
Peeke, H. V. S., Twum, M., Finkelstein, J. E., and Figler, M. H. (1995). Maternal aggression in red swamp crayfish (Procambarus Clarkii, Girard): the relation between reproductive status and outcome of aggressive encounters with male and female conspecifics. Behaviour 132, 107–125. doi: 10.1163/156853995X00324
Peer, N., Perissinotto, R., Miranda, N. A. F., and Raw, J. L. (2015). A stable isotopic study of the diet of potamonautes sidneyi (Brachyura: Potamonautidae) in two coastal lakes of the iSimangaliso Wetland Park, South Africa. Water SA 41, 549–558. doi: 10.4314/wsa.v41i4.15
Pinheiro, J., Bates, D., DebRoy, S., Sarkar, D., R Core Team (2018). nlme: Linear and Nonlinear Mixed Effects Models. R package version 3.1-145. Available online at: https://CRAN.R-project.org/package=nlme.
Pintor, L. M., Sih, A., and Kerby, J. L. (2009). Behavioral correlations provide a mechanism for explaining high invader densities and increased impacts on native prey. Ecology 90, 581–587. doi: 10.1890/08-0552.1
R Core Team (2018). R: A Language and Environment for Statistical Computing. R Foundation for Statistical Computing, Vienna, Austria. URL https://www.R-project.org/.
Ricciardi, A. (2001). Facilitative interactions among aquatic invaders: is an “invasional meltdown” occurring in the Great Lakes? Can. J. Fish. Aquat. Sci. 58, 2513–2525. doi: 10.1139/f01-178
Russell, J. C., Sataruddin, N. S., and Heard, A. D. (2014). Over-invasion by functionally equivalent invasive species. Ecology95, 2268–2276. doi: 10.1890/13-1672.1
Savvides, P., Vasilis, L., and Sfenthourakis, S. (2015). Competition for shelter occupancy between a native freshwater crab and an invasive crayfish. Aquatic Ecol. 49, 273–278. doi: 10.1007/s10452-015-9522-7
Schenk, S. C., and Wainwright, P. C. (2001). Dimorphism and the functional basis of claw strength in six brachyuran crabs. J. Zool. 255, 105–119. doi: 10.1017/S0952836901001157
Seebens, H., Blackburn, T. M., Dyer, E. E., Genovesi, P., Hulme, P. E., Jeschke, J. M., et al. (2017). No saturation in the accumulation of alien species worldwide. Nat. Commun. 8:14435. doi: 10.1038/ncomms14435
Sibbing, F. A. (1991). “Food capture and oral processing,” in Cyprinid Fishes. Fish & Fisheries Series, Vol. 3, eds I. J. Winfield, and J. S. Nelson (Dordrecht: Springer)
Sih, A., Bolnick, D. I., Luttbeg, B., Orrock, J. L., Peacor, S. D., Pintor, L. M., et al. (2010). Predator–prey naïveté, antipredator behavior, and the ecology of predator invasions. Oikos 119, 610–621. doi: 10.1111/j.1600-0706.2009.18039.x
Simberloff, D. (2006). Invasional meltdown 6 years later: important phenomenon, unfortunate metaphor, or both? Ecol. Lett. 9, 912–919. doi: 10.1111/j.1461-0248.2006.00939.x
Singh, G., Block, J. D., and Rebach, S. (2000). Measurement of the crushing force of the crab claw. Crustaceana 73, 633–637, doi: 10.1163/156854000504606
Snyder, W. E., and Evans, E. W. (2006). Ecological effects of invasive arthropod generalist predators. Annu. Rev. Ecol. Evol. Syst. 37, 95–122. doi: 10.1146/annurev.ecolsys.37.091305.110107
South, J., McCard, M., Khosa, D., Mofu, L., Madzivanzira, T. C., Dick, J. T. A., et al. (2019). The effect of prey identity and substrate type on the functional response of a globally invasive crayfish. NeoBiota 52, 9–24. doi: 10.3897/neobiota.52.39245
Stein, R. A. (1976). Sexual dimorphism in crayfish chelae: functional significance linked to reproductive activities. Can. J. Zool. 54, 220–227. doi: 10.1139/z76-024
Tilman, D. (1999). The ecological consequences of changes in biodiversity: a search for general principles. Ecology 80, 1455–1474. doi: 10.2307/176540
Vonesh, J., McCoy, M., Altwegg, R., Landi, P., and Measey, J. (2017). Functional responses can't unify invasion ecology. Biol. Invasions 19, 1673–1676. doi: 10.1007/s10530-016-1356-2
Weyl, O. L. F., Ellender, B. R., Wassermann, R. J., Truter, M., Dalu, T., Zengeya, T. A., et al. (2020). “Alien freshwater fauna in South Africa,” in Biological Invasions in South Africa. Invading Nature - Springer Series in Invasion Ecology, Vol. 14, eds B. van Wilgen, J. Measey, D. Richardson, J. Wilson, and T. Zengeya (Cham: Springer), 153–183. doi: 10.1007/978-3-030-32394-3_6
Wilson, R. S., Angilletta, M. J. Jr., James, R. S., Navas, C., and Seebacher, F. (2007). Dishonest signals of strength in male slender crayfish (Cherax dispar) during agonistic encounters. Am. Nat. 170, 284–291. doi: 10.1086/519399
Zeng, Y., Chong, K. Y., Grey, E. K., Lodge, D. M., Yeo, D. C. J. (2015). Disregarding human pre-introduction selection can confound invasive crayfish risk assessments. Biol. Invas. 17, 2373–2385. doi: 10.1007/s10530-015-0881-8
Zeng, Y., Shakir, K. K., and Yeo, D. C. J. (2019). Competition between a native freshwater crab and an invasive crayfish in tropical Southeast Asia. Biol. Invasions. 21, 2653–2663. doi: 10.1007/s10530-019-02009-6
Keywords: Procambarus clarkii, Cherax quadricarinatus, Potamonautes perlatus, invasive species, competition, strength
Citation: South J, Madzivanzira TC, Tshali N, Measey J and Weyl OLF (2020) In a Pinch: Mechanisms Behind Potential Biotic Resistance Toward Two Invasive Crayfish by Native African Freshwater Crabs. Front. Ecol. Evol. 8:72. doi: 10.3389/fevo.2020.00072
Received: 02 December 2019; Accepted: 04 March 2020;
Published: 27 March 2020.
Edited by:
Elena Tricarico, University of Florence, ItalyReviewed by:
Antonín Kouba, University of South Bohemia, CzechiaCopyright © 2020 South, Madzivanzira, Tshali, Measey and Weyl. This is an open-access article distributed under the terms of the Creative Commons Attribution License (CC BY). The use, distribution or reproduction in other forums is permitted, provided the original author(s) and the copyright owner(s) are credited and that the original publication in this journal is cited, in accordance with accepted academic practice. No use, distribution or reproduction is permitted which does not comply with these terms.
*Correspondence: Josie South, am9zaWVzb3V0aDkzQGdtYWlsLmNvbQ==
†ORCID: Josie South orcid.org/0000-0002-6339-4225
John Measey orcid.org/0000-0001-9939-7615
Olaf LF Weyl orcid.org/0000-0002-8935-3296
Disclaimer: All claims expressed in this article are solely those of the authors and do not necessarily represent those of their affiliated organizations, or those of the publisher, the editors and the reviewers. Any product that may be evaluated in this article or claim that may be made by its manufacturer is not guaranteed or endorsed by the publisher.
Research integrity at Frontiers
Learn more about the work of our research integrity team to safeguard the quality of each article we publish.