- 1Center for Education in Liberal Arts and Sciences (CELAS), Osaka University, Toyonaka, Japan
- 2Department of Biological Sciences, Graduate School of Science, Osaka University, Toyonaka, Japan
Floral organs are clade-specifically arranged to either spiral or whorled (concentric circles) phyllotaxis. The basic number (merosity) of perianth organs within a whorl is limited to three in most monocots and to four or five in most eudicots. Although the Fibonacci number (3, 5) of merosity is well-known to agree with that of the spirals in phyllotaxis, the evolutionary relationship between whorls and spiral phyllotaxis remains unclear. Focusing on aestivation (the relative positioning of margins of flower organs in the bud) to capture phyllotaxis including merosity of whorled flowers, trimerous-whorled flowers and spiral ones coexist within populations with intraspecific variation in organ numbers. In addition, a recent mathematical model showed that tetramerous and pentamerous whorls developed from spiral organ initiation by incorporating a post-meristematic organ displacement, depending on the interaction among organ primordia. Therefore, integrating the variation of aestivation with the spiral-to-whorl development may elucidate the underlying mechanism of the continuous spiral-whorl relationship with the merosity diversification. Here, we showed that the aestivation of perianth organs (tepals) of mature flowers was intra-specifically variable but constrained in wild populations of several Anemone and Eranthis species (Ranunculaceae); the spiral arrangements coexisted within a small population, with dimerous, trimerous, tetramerous, and pentamerous double-whorled arrangements, despite considerable possibilities in the geometry. We determined mathematically that most of these constrained aestivations of 5 to 11-tepaled flowers emerge upon the spiral phyllotaxis with a divergence angle between subsequent organs of 90–102 or 135–144° (known as the Lucas and Fibonacci angles, respectively). Incorporating the post-meristematic organ displacement into the model, double-whorled arrangements work as templates to form multiple whorls, the merosity of which is stabilized to trimery, tetramery, or pentamery depending on the divergence angle. These results demonstrate that spiral phyllotaxis promotes the constrained coexistence of whorl and spiral rather than their interspecific dichotomy. This polymorphic phyllotaxis provides an evolutionary scenario in which the floral bauplans of angiosperms could be differentiated into tri-, tetra- and penta-radial symmetries.
Introduction
Phyllotaxis is the regular arrangement of plant aerial organs around the plant stem (Adler et al., 1997). There are two major phyllotactic arrangement types, spiral and whorled. In spiral phyllotaxis, organs are arranged spirally around the stem while in whorled phyllotaxis organs are arranged in concentric circles (i.e., whorls) in a radially symmetric manner (Figure 1A). The perianth organs (tepals) of a flower are therefore arranged either in whorls or spirals, and this species-specific trait is thought to have emerged during the evolution of flowering plants (Endress, 2001a; Ronse de Craene et al., 2003; Endress and Doyle, 2009). However, it is a matter of debate whether the ancestral state is spiral or whorled (Sauquet et al., 2017; de-Paula et al., 2018; Sokoloff et al., 2018). On the other hand, during the floral development of many families within the angiosperm clade (e.g., Caryophyllaceae, Solanaceae, Rosaceae, Ranunculaceae, and Nymphaeaceae), organ primordia are initiated in a spiral arrangement but converge to whorls at maturity (Lyndon, 1978; Endress, 1987; Hill and Malmberg, 1996; Foster et al., 2003). Thus, the evolutionary relationship between spiral and whorled arrangements should be investigated further.
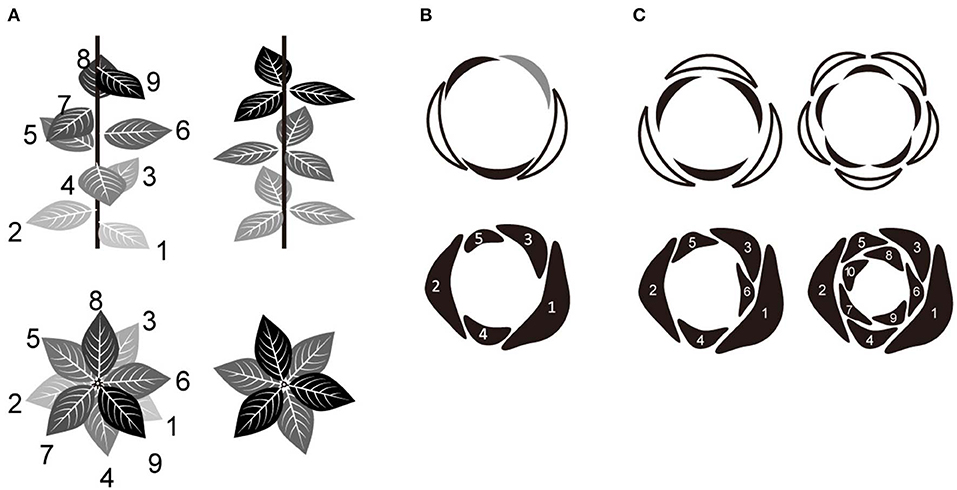
Figure 1. Relationship between spiral and whorl phyllotaxis. (A) Two major categories of phyllotaxis; spiral (left) and whorled (right). (B) Quincuncial aestivation of five tepals derived from the Fibonacci spiral (with a divergence angle of 137°). The arrangement of each organ in relation to neighboring ones was identified (top) as either external (white), internal (black), or alternating (gray). (C) Trimerous and pentamerous double-whorls (top) derived from the Fibonacci spiral (bottom). In some species, primordia initiate individually in a spiral order and then develop into whorled arrangements during floral development via a post-meristematic interaction between them. The numbers in (A–C) indicate the initiation order of each organ.
Merosity (Ronse de Craene and Smets, 1994; Ronse de Craene, 2016), defined as the number of floral organs within a whorl, is typically four or five in most eudicots and three in monocots and magnoliids (Figure 1C, upper panel). There is a tendency for all whorls to have the same organ number in the centrifugal development that is common in flowers (Smyth, 2018). It has been emphasized that basic trimerous and pentamerous forms represent Fibonacci numbers (Endress, 1987; Kubitzki, 1987), which define secondary spirals (parastichy) in spiral phyllotaxis. However, the detailed developmental mechanism for the transition from a spiral to a whorled arrangement is still largely unknown. A recent mathematical model of floral phyllotaxis was used to demonstrate that a pseudo-whorled arrangement (multiple organs arranged at nearly equal distance from the shoot apex; Kwiatkowska, 1999) can emerge from spiral initiation (Kitazawa and Fujimoto, 2015). According to this model, tetramerous and pentamerous whorls emerge from spiral initiation by organ displacement depending on post-meristematic interaction; the mechanism leading to trimerous whorls remains unclear.
Trimery and pentamery coexist in several genera and/or species of several clades scattered over angiosperms (Schoute, 1935; Endress, 1987; Kubitzki, 1987; Ronse de Craene et al., 2003; Damerval and Nadot, 2007; Ronse de Craene, 2016); e.g., Anemone (Ranunculaceae, eudicot; Schöffel, 1932; Ren et al., 2010; Kitazawa and Fujimoto, 2014), Eranthis (Ranunculaceae; Salisbury, 1919; Kitazawa and Fujimoto, 2016a), Aspidistra (Asparagaceae, monocot; Vislobokov et al., 2014), Nuphar (Nymphaeaceae, Nymphaeales; Endress, 2001b; Schneider et al., 2003; Padgett, 2007; Warner et al., 2008), and Cabomba (Cabombaceae, Nymphaeales; Rudall et al., 2009). One way to capture floral organization is aestivation, i.e., the relative positioning of floral organs in the bud (Figure 1B, upper panel; Schoute, 1935; Sattler, 1973; Ronse de Craene, 2010), which can explain phyllotaxis including merosity. In five-tepaled Anemone species, aestivations appear to be highly constrained: despite four geometrically possible aestivations (Cunnell, 1958), the quincuncial aestivation, which reflects spiral phyllotaxis appears to be dominant (Figure 1B; Kitazawa and Fujimoto, 2016b, 2018). An aestivation with trimerous double-whorls can emerge from the spiral phyllotaxis upon appearance of an excessive organ (Figure 1C, left bottom) and coexist with a quincuncial arrangement within a population (Kitazawa and Fujimoto, 2016b, 2018) with intraspecific variation of organ numbers, as observed in several Anemone species (Yule, 1902; Schöffel, 1932). Although the increase of organ numbers also increases the possible geometric arrangements of organs, only few arrangements, including that with double trimerous whorls, appear in natural populations of Anemone species (Kitazawa and Fujimoto, 2016b, 2018). Both the quincuncial and the double trimerous whorls are considered to also exist in Aspidistra (Figure 3 in; Vislobokov et al., 2014), Nuphar (Endress, 2001b; Schneider et al., 2003), and Cabomba (Rudall et al., 2009) species. Theoretically, further increase in organ numbers following the Fibonacci spiral will result in double pentamerous whorls (Figure 1C, right); a decrease to four organs will result in double dimerous whorls. The coexistence of whorled and spiral flowers could help clarifying a developmentally constrained pathway of floral organization evolution in angiosperms.
Based on aestivation variation and mathematical modeling approaches, the present report aimed to further investigate the constrained coexistence of whorled and spiral flowers with a wide range of tepal numbers in two Ranunculaceae genera, Anemone and Eranthis. We theoretically examined the relationship between the merosity and the divergence angle, a major parameter of spiral phyllotaxis, and confirmed that coexisting aestivations work as templates to form multiple whorls while keeping the merosity.
Materials and Methods
Positional Arrangement of Perianth Organs
The aestivation of perianth organs (i.e., tepals) was examined in mature flowers with four to 11 tepals only. Following previous studies (Morgan, 1874; Schoute, 1935; Cunnell, 1958; Kitazawa and Fujimoto, 2016b, 2018), we surmised aestivation by referring to the positional arrangements of tepals (Figures 1B,C). The aestivation of flowers with the same number of external and internal organs (represented in white and black, respectively; Figures 1B,C, upper panel) and no alternating organs (represented in gray; Figure 1B) are hereafter referred to as double-whorled. Except for the double-whorls, the aestivations derived from the spiral phyllotaxis that we mathematically confirmed (represented in orange, Figure 3), are referred to as spiral flowers. The external, internal, and alternating organs are referred to as E, I, and A, respectively. For simplicity, reflected and rotated arrangements were dismissed, and therefore the position of the flower with respect to the main axis and the direction of the spiral were ignored.
Plant Samples
We measured the positional arrangements of tepals in wild populations of Eranthis pinnatifida (Shiga, Hyogo, Okayama, and Hiroshima prefectures), Anemone nikoensis (Shiga, Osaka, Hyogo, and Okayama prefectures), Anemone flaccida (Hokkaido, Shiga, Osaka, Hyogo, and Okayama prefectures), Anemone hepatica (Shiga and Okayama prefectures), and two forms of Anemone × hybrida (Japanese anemone; designated as Anemone scabiosa in Kitazawa and Fujimoto, 2016a,b; Hokkaido, Tokyo, Mie, Shiga, Kyoto, Nara, Osaka, and Hyogo prefectures) in Japan. Perianth organs of these species are not differentiated into sepals and petals. Although there are several forms of A. × hybrida, we were unable to identify them at many of our observation sites. We used tepal color as the primary feature to distinguish between two of the forms: pale pink (PP) and white (W); the deep pink form was not examined. We did not count the flowers in cases where tepals were so narrow at their basal parts that their positional overlaps were lost as days elapsed after blooming. The absolute frequency of each arrangement was measured as the sum of the multiple populations within the species (Supplementary Table 1). The relative frequency was normalized to flower sample sizes and their respective tepal numbers, only when sample size was above 30 (Supplementary Table 1 and Figure 2). The modal tepal number in the sum of the observed populations was 5 for E. pinnatifida, A. flaccida, A. nikoensis, and A. × hybrida PP, 6 for A. hepatica, and 7 for A. × hybrida W (Supplementary Table 2).
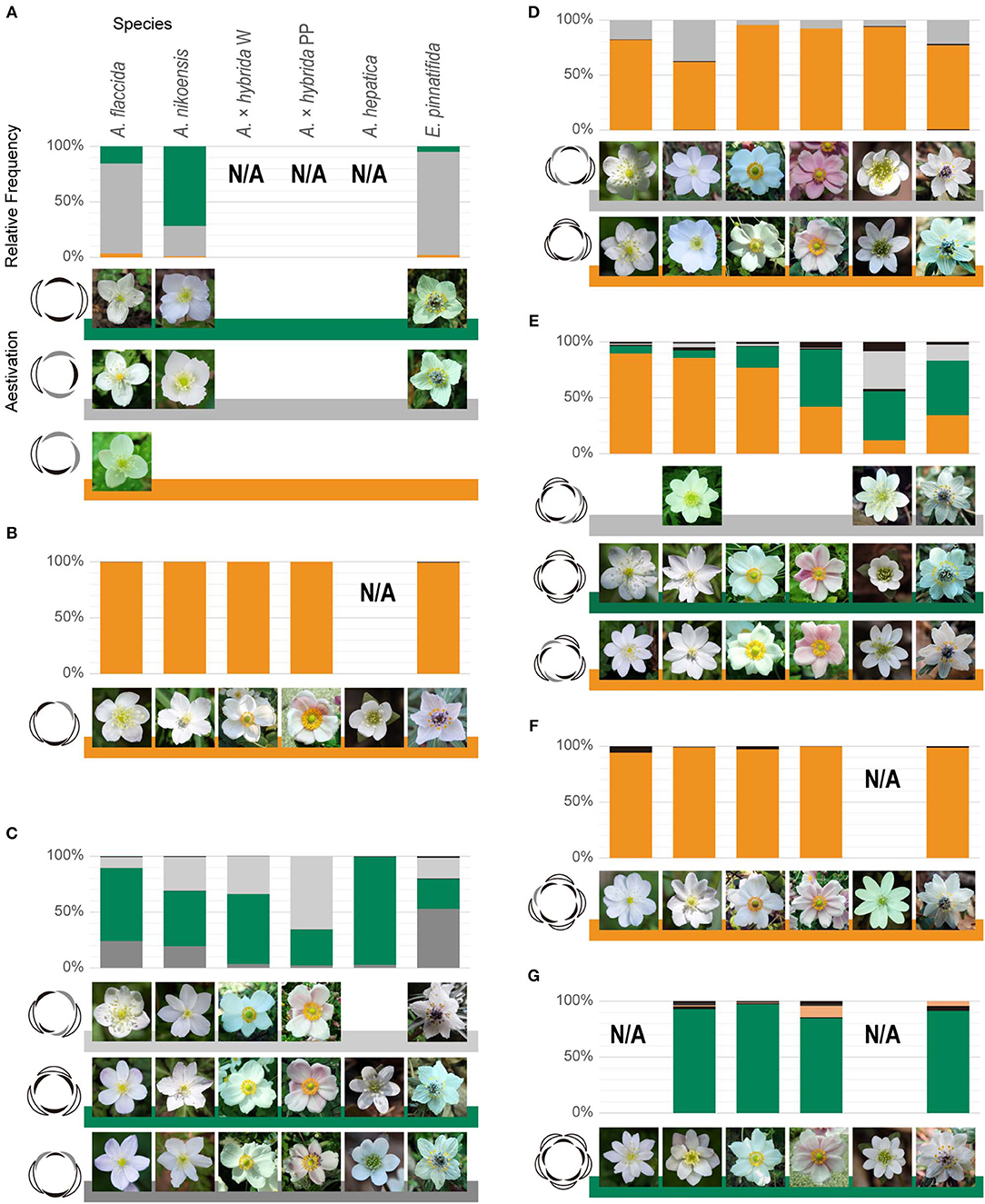
Figure 2. Constrained, intraspecific coexistence of whorled and spiral flowers. Relative frequency of aestivation in flowers with 4 (A), 5 (B), 6 (C), 7 (D), 8 (E), 9 (F), and 10 (G) tepals. Green and orange in bar charts denote double-whorled and spiral flowers, respectively, and dark and light gray denote other aestivations, consistently with coloring in Supplementary Table 1. 10-tepaled aestivation in orange denotes EAIEAIEIEI. The other aestivations with relative frequency below 10% are shown in black. Representative images of the major aestivations are shown for each species and form at the right side of the floral diagrams. N/A indicates the sample size was below 30. The rank in each tepal number indicated a negative correlation between tetramerous and trimerous double-whorls; trimerous and tetramerous double-whorls ranked first for 6-tepaled and second for 8-tepaled flowers, respectively, for A. flaccida, A. nikoensis, and A. × hybrida W; these ranks were the opposite for A. × hybrida PP and E. pinnatifida.
Mathematical Model
To theoretically infer the developmental mechanisms of the observed aestivations, we examined the relationship between divergence angle and merosity using a developmental model for phyllotaxis (Douady and Couder, 1996; Kitazawa and Fujimoto, 2015). In this model, a new primordium i emerges at a constant time interval τ and a constant divergence angle ϕ with the subsequent organ, at the edge of the meristem with radius R0 in the polar coordinate (R0, i ϕ mod 360°), where i ϕ mod 360° stands for the remainder of the division of i ϕ by 360°. We considered two types of models, namely the absence (Figure 3) and the presence (Figure 4) of a post-meristematic organ interaction. In the absence model, analytical calculation rigorously identified aestivation given the primordium number i and angle ϕ. In the presence model, after the initiation, the post-meristematic displacement of organs depending on their interaction was simulated by the Monte Carlo method (Kitazawa and Fujimoto, 2015) to examine which merosity was favored. Monte Carlo steps were repeated for τ multiplied by the number of existing primordia. In each step, a primordium k was selected randomly, and a new position () was assigned for it. The new coordinates and followed normal distributions and their means were rk and θk, respectively, with standard deviations σr and σθ, respectively. The potential energy Ug of each position was formulated as
where dkj denotes the distance between primordia k and j. The new position for k was selected when the energy difference between the original and the new position () was equal to or smaller than zero. When ΔU was positive, the new position was selected with probability exp(-βΔU) and the primordium stayed at the original position otherwise. Given and σθ = 2.0, the potential-dependent displacement occurs much more largely in the radial direction than in the angular direction.
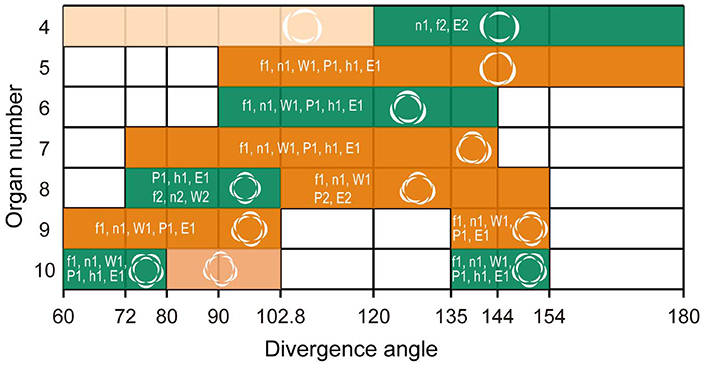
Figure 3. Double-whorls of trimery, tetramery, and pentamery can develop from spiral phyllotaxis at different divergence angles. Aestivation patterns derived from calculations using the spiral phyllotaxis model with a constant divergence angle (degree; horizontal axis) in relation to tepal number (vertical axis). Whorled and spiral flowers observed in Anemone and Eranthis species are shown in green and orange, respectively; their relative frequency is above 50% in some species or forms, except for the light-colored aestivations (EAAI, 4% maximum; EAIEAIEIEI, 11% maximum). Codes f1, n1, W1, P1, h1, and E1 indicate the most frequent aestivations in A. flaccida, A. nikoensis, A. × hybrida W, A. × hybrida PP, A. hepatica, and E. pinnatifida, respectively. For the 4-tepaled and 8-tepaled flowers, the second most frequent aestivations are also indicated as f2, n2, W2, P2, and E2, where letters indicate the same species as in the most frequent aestivations. Aestivations in white regions are hardly observed in nature (i.e., <3% of the relative frequency in all the species and forms we examined; see Supplementary Table 1).
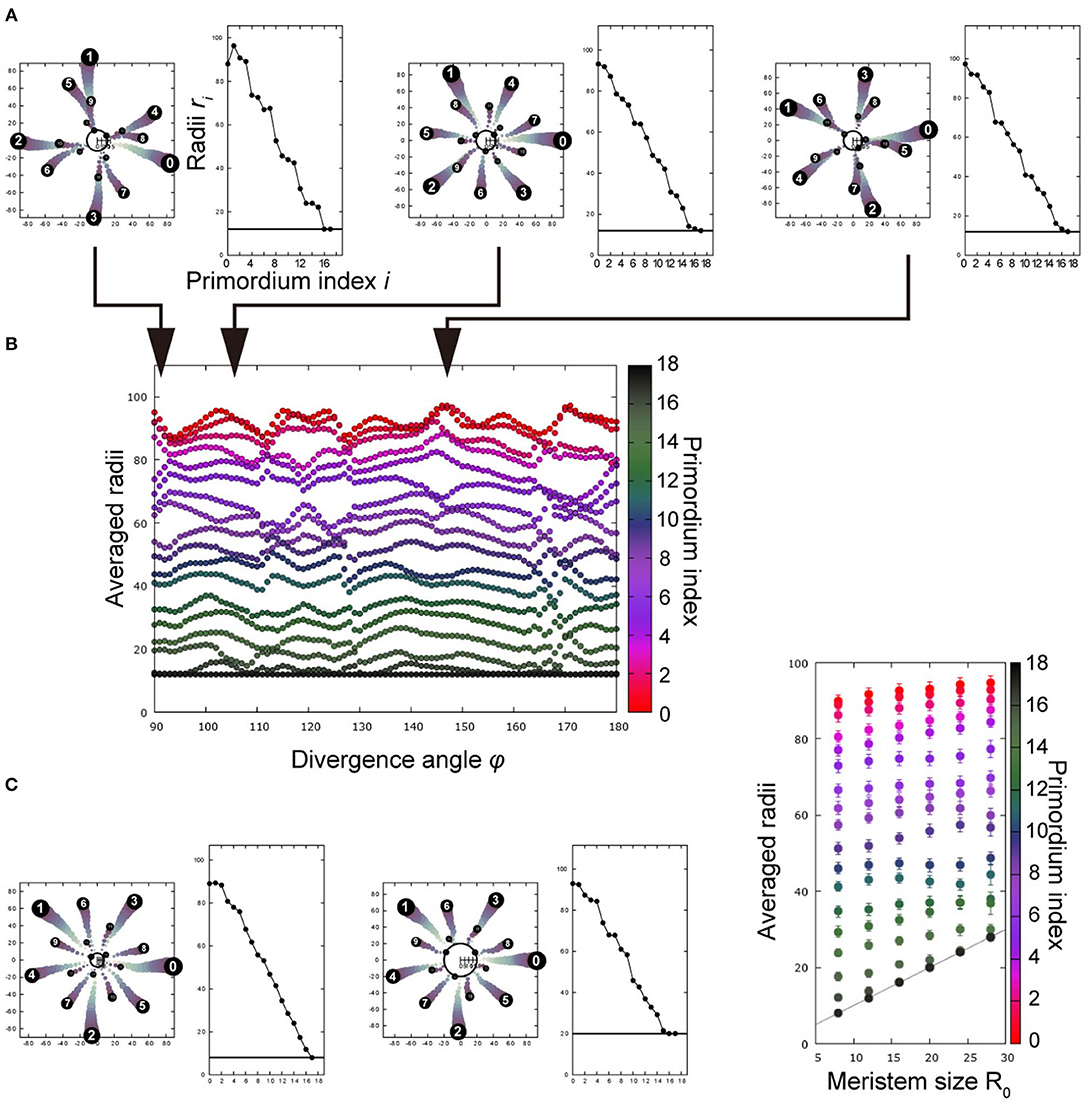
Figure 4. Post-meristematic organ displacement after spiral initiation promotes radial symmetries of trimerous, tetramerous, and pentamerous whorls depending on divergence angles. (A) A representative result of Monte Carlo simulation, showing five trimerous whorls. The left panel shows the arrangement in a 2D plane whereas the right panel shows radial positions as a function of primordium indices, evidencing the trimerous whorls separated by radial gaps. ϕ = 91° (left), 108° (middle), and 145° (right); R0 = 12.0. (B) Radii as a function of ϕ. Radii of primordia were averaged over 200 replicates at the timing of the 18th primordium initiation, and a file was obtained for each ϕ. The color scale indicates the primordium index. The vertical space corresponds to the radial gap between whorls, and the number of primordia separated by the spaces corresponds to the merosity. R0 = 12.0. (C) Dependence on the R0 at ϕ = 136°. The merosity of the outermost whorl changed from trimery (left, R0 = 8.0) to pentamery (middle, R0 = 20.0), as R0 increased. The averaged radii are shown in the right panel as in (B), where error bar denotes the standard deviation of 200 simulations.
Results
Constrained Coexistence of Whorled and Spiral Flowers in Populations as a Result of Intraspecific Variation of Tepal Numbers
In line with a previous report (Kitazawa and Fujimoto, 2018), we confirmed that the aestivations of 5- to 7-lobed flowers were constrained to several types including the trimerous double-whorl and the spirals, despite considerable intraspecific variation of tepal numbers in Anemone species (Supplementary Table 2) (Yule, 1902; Kitazawa and Fujimoto, 2014, 2016a), even when there was a two-fold increase in the sample size (Figures 2B–D, Supplementary Table 1). Therefore, we explored the constrained coexistence of whorled and spiral flowers in populations with more than 7 and <5 tepals, in four Anemone and one Eranthis species (sections Positional Arrangement of Perianth Organs and Plant Samples). We found that the aestivations of 8- to 10-lobed flowers were mostly constrained to five types, whereas the relative frequency of the remaining types appeared to be less than 10% in all the species and forms we examined (Figures 2E–G). Two of these five dominant types were the tetramerous and pentamerous double-whorled (represented in green, Figures 2E,G); two other dominant types were spiral (represented in orange, Figures 2E,F). The double pentamerous whorls appeared in more than 85% of the 10-tepaled flowers in each species (Figure 2G). One spiral was present in 94% of the 9-tepaled flowers in each species (Figure 2F). The tetramerous double-whorled and the other spiral arrangements were dominant in more than 90% of the 8-tepaled flowers, except in A. hepatica (57%) and E. pinnatifida (83%) where another aestivation (EAIEIEIA) was frequent instead (34% and 14% of flowers, respectively; represented in gray, Figure 2E). In addition, the spiral dominantly appeared in 98% of the 11-tepaled flowers in all species (n = 89), and the dimerous double-whorl appeared in 29% of the 4-tepaled flowers in all species (n = 87; Supplementary Table 1; represented in green, Figure 2A). These intraspecific coexistences appeared even in a single population of each species where the relative frequency of spirals and whorls showed considerable reproducibility among different years (Supplementary Table 3). Taken together, the aestivations of 5- to 11-tepaled flowers were mostly constrained to the double-whorls of trimery, tetramery, and pentamery as well as to spirals.
Species- and Population-Specificity of the Constrained Aestivation
The relative frequencies of the double-whorled and spiral flowers varied across species. The double tetramerous whorls and spiral ranked first and second, respectively, in 8-tepaled flowers for A. × hybrida PP, A. hepatica, and E. pinnatifida, whereas these ranks in 8-tepaled flowers were the opposite for A. flaccida, A. nikoensis, and A. × hybrida W (Figure 2E). The relative frequency distribution of tetramerous double-whorls was more than 40% for A. × hybrida PP, A. hepatica, and E. pinnatifida, 19% for A. × hybrida W, and 7% for A. flaccida and A. nikoensis (Supplementary Table 2). The trimerous double-whorl ranked first in 6-tepaled flowers for A. flaccida, A. nikoensis, A. × hybrida W, and A. hepatica, and second for A. × hybrida PP and E. pinnatifida (EAIEIA and EAIAEI ranked first, respectively, for the last two species; Figure 2C), in line with the results of a previous report (Kitazawa and Fujimoto, 2018). In addition, in several populations, the 6-tepaled aestivation exhibited a reproducible difference from the above-mentioned average of total populations. The trimerous double-whorl was first-ranked with more than 73% of the relative frequency in two populations of E. pinnatifida (HSB1 and HSB2 in Supplementary Table 3) and 27% on average in E. pinnatifida (Supplementary Table 2). Conversely, it ranked third with <3% of the frequency in a population of A. nikoensis (HSY1 in Supplementary Table 3), corresponding to an average 50% for this species.
A Spiral Phyllotaxis Model With a Constant Divergence Angle Reproduced the Trimerous, Tetramerous, and Pentamerous Whorls
The spiral phyllotaxis model with a constant divergence angle ϕ (section Mathematical Model) showed that the double trimerous whorls and the spirals observed for the 5-, 7-, 9-, and 11-tepaled flowers simultaneously occur at 90° ≤ ϕ ≤ 102.8° and 135° ≤ ϕ ≤ 144° angle ranges (Figure 3). The aestivations for the 4-, 8-, and 10-tepaled arrangements in nature were reproduced depending on these angular regions in the model; the dimerous double-whorl, spiral (EAIEAIEI), and pentamerous double-whorl appeared at the 135° ≤ ϕ ≤ 144° angle range in the model, whereas the spiral (EAAI), tetramerous double-whorl, and spiral (EAIEAIEIEI) appeared at the 90° ≤ ϕ ≤ 102.8° angle range (Figure 3 and Supplementary Table 1). The aestivations of 8-tepaled flowers at the 135° ≤ ϕ ≤ 144° angle range (i.e., spiral) ranked first in A. nikoensis, A. flaccida, and A. × hybrida W, whereas the double tetramerous whorls at the 90° ≤ ϕ ≤ 102.8° angle range appeared most frequently in A. × hybrida PP, A. hepatica, and E. pinnatifida. The aestivations of 10-tepaled flowers at the 135° ≤ ϕ ≤ 144° and 90° ≤ ϕ ≤ 102.8° angle ranges were first- and second-ranked in all the species and forms we observed (Figure 2G and Supplementary Table 1). Therefore, the spiral phyllotaxis model with constant divergence angles at the 90° ≤ ϕ ≤ 102.8° and 135° ≤ ϕ ≤ 144° ranges accounted for most aestivations observed in nature (9 of 14 aestivations with relative frequency above 10% in at least one species and form; Supplementary Table 1), whereas the aestivations that appeared in the other angular regions of the model are hardly observed in nature (3% at most, highlighted in cyan in Supplementary Table 1).
The Spiral Model With Post-meristematic Organ Displacement Promotes Multiple Whorls
We theoretically examined whether spiral initiation with a fixed divergence angle ϕ results in an arrangement with multiple whorls, incorporating the post-meristematic interaction among floral organ primordia (Kitazawa and Fujimoto, 2015; section Mathematical Model). Post-meristematic interaction caused radial displacement of organ primordia, separating outer and inner whorls, where the radial distance of each primordium from the meristem center primordia became almost equal within each whorl. The subsequent primordia were arranged following the whorled phyllotaxis, thereby resulting in more than two whorls (Figure 4A). The merosity of whorls was tetramerous near ϕ = 90° and pentamerous near ϕ = 144° upon initiation (Figure 4A left and right; Figure 4B), consistent with the above model without post-meristematic interaction. The trimerous whorls stably appeared near ϕ = 120° (Figure 4A, middle). Merosity can also be affected by another parameter, meristem diameter R0. For example, when ϕ was 136°, the merosity changed from three to five as R0 increased (Figure 4C, left and middle); when ϕ = 144°, the merosity of the outermost whorl remained five while R0 remained in the same range. Therefore, the main parameter of merosity is the divergence angle as in the above model without post-meristematic interaction; around the marginal angles of the arrangements (e.g., ϕ = 136° for pentamery, Figure 4C), the merosity became unstable and changed depending on R0.
Discussion
Perianth Phyllotaxis Polymorphism Provides an Evolutionary Scenario for Merosity Differentiation
Our field observations showed that, although Anemone and Eranthis tepal numbers and arrangements were highly variable, they were not random; rather, they were limited to double-whorled and spiral flowers (Figure 2). The spiral model with a constant divergence angle accounted for the observed coexistence of whorled and spiral arrangements, depending mainly on the divergence angle (Figure 3). The spiral organ initiation model incorporating the post-meristematic organ displacement further ensured that this double-whorled aestivation works as the template to subsequently form multiple whorls with the same merosity (i.e., trimery, tetramery, or pentamery; Figure 4). The debate on the evolution of ancestral flowers has treated whorled and spiral phyllotaxis as opposite hypotheses (Sauquet et al., 2017). The polymorphism of spirals and whorls within populations presented here provides an alternative scenario in which angiosperm floral bauplans evolutionarily diversified to trimerous, tetramerous, and pentamerous whorls. This scenario is further supported by the intraspecific coexistence of spiral and whorled phyllotaxis observed in several clades, including the stamens and carpels of Anemone (Schöffel, 1932; Ren et al., 2010) and Magnolia (Magnoliaceae; Zagórska-Marek, 1994) species. In addition, some of the tepal arrangement polymorphisms observed in double-whorls of dimery, trimery, and tetramery as well as in spirals (e.g., quincuncial, EAIEIEI; Figure 2) are commonly observed in Aspidistra (Figure 3 in Vislobokov et al., 2014) and Cabomba (Ørgaard, 1991; Rudall et al., 2009) species. To date, the coexistence of spiral and whorled tepal phyllotaxis have only been known among species in neighboring clades; e.g., spiral in M. stellata with a divergence angle of nearly 137° and trimerous-whorled in M. denudata and Liriodendron tulipifera (Magnoliaceae) within the 120–137° divergence angle range (Kubitzki, 1987). The appearance of trimery at this angle range is consistent with the model with post-meristematic organ displacement (Figure 4). Additionally, in Nymphaeales, tepals appear to be tetramerous whorled as well (e.g., Nymphaea species; Schneider et al., 2003). Confirming the intraspecific coexistence of spirals and whorls in these clades as well as in Nuphar would indicate that such polymorphism is a possible ancestral state of angiosperm flowers.
Possible Developmental Origins of Organ Number and Aestivation Variations
To discuss the frequency of spirals and whorls that differs among species (Figure 2), we need to clarify the underlying developmental mechanism of organ number variation. The plausible mechanism of a stochastic increase in tepals is homeosis, where some stamen primordia transform into tepals (Kitazawa and Fujimoto, 2014) due to the fading border of fate determinants (Buzgo et al., 2005; Soltis et al., 2006; Wang et al., 2015). In the mathematical model with post-meristematic modification, the radius of a newer primordium can reach or exceed that of older primordia (Figure 4); thus, the newer primordium can assume the outer organ fate, even in an ideal model of concentric fate determination (Coen and Meyerowitz, 1991).
Differences in aestivations among species as well as populations (Figure 2 and Supporting Table 3) suggest that underlying mechanisms exist genetically and/or geographically. They were mainly observed in the 6- or 8-tepaled flowers. Our present and previous theoretical studies provided two possible scenarios to explain those differences. The present scenario is that floral phyllotaxis is species-specifically biased. Most of the observed aestivations were produced from spiral phyllotaxis with constant divergence angle ranges of 135–144° and 90–102.8° (Figure 3). The representative angles of 137° and 99° have been reported in the floral phyllotaxis of a single species, e.g., A. nemorosa and A. hepatica (Schöffel, 1932) or in the magnollid Drimys winteri (Doust, 2001). Theoretically, angles of 137° and 99° are defined as the limits of the Fibonacci sequence (xn+1= xn + xn−1, x1= 1, x2= 2; limn → ∞ 360° xn/xn+1 = 137°) and the Lucas sequence (xn+1= xn + xn−1, x1= 1, x2= 3; limn → ∞ 360° xn/xn+1 = 99°), respectively (Bravais and Bravais, 1837a,b). In addition, these two divergence angles coexisted at a value of the fundamental parameter of spiral phyllotaxis, given by the logarithm of the plastochron ratio ~0.1 (Douady and Couder, 1996). This value is further consistent with the floral meristem of Anemone species (e.g., Figures 7, 10 in Ren et al., 2010). Therefore, even in identical genetic and geographic backgrounds, both Fibonacci and Lucas spiral phyllotaxis can stably coexist showing the polyphenism, while different backgrounds are likely to occur in the Anemone spp. and E. pinnatifida tepal arrangement. The consistencies between model and nature patterns (sections A Spiral Phyllotaxis Model With a Constant Divergence Angle Reproduced the Trimerous, Tetramerous, and Pentamerous Whorls and The Spiral Model With Post-Meristematic Organ Displacement Promotes Multiple Whorls) suggest that the Fibonacci angle (137°) resulting pentamery is more frequent than the Lucas angle (99°) resulting tetramery in A. nikoensis, A. flaccida, and A. × hybrida W; the opposite is true for A. × hybrida PP, A. hepatica, and E. pinnatifida.
On the other hand, this constant divergence angle model cannot account for two aestivations in the 6-tepaled and one of 7-tepaled flowers (represented in light and dark gray, Figures 2C,D), which were explained by the other scenario. In the previous model, the divergence angle was variable among organs depending on an inhibitory field potential from preexisting primordia (Kitazawa and Fujimoto, 2016b, 2018); this is probably due to genetic, environmental, or developmental factors that affect phyllotactic parameters such as organ growth rate, as seen in Arabidopsis thaliana inflorescence phyllotaxis (Mirabet et al., 2012; Besnard et al., 2014; Refahi et al., 2016). The variable angle model included the constant angle model whereas the latter simply accounted for the coexisting whorls and spiral. Further analyses of the aestivations and angles in the above-mentioned clades would clarify which model is better to summarize the coexistence.
Future Implications of Our Models
The several observed aestivations that have not yet been explained by the constant angle model, were all bilateral (i.e., EAIA, EAIAEI, EAIEIA, and EAIEIEIA; represented in gray, Figure 2A). The first arrangement is also widely observed in members of Nymphaeaceae (Schneider et al., 2003; Hu et al., 2009) whereas the others, to our best knowledge, have only been reported in Anemone and Eranthis species. One possible cause for such bilaterally symmetric arrangements is the presence of certain structures around the flower, such as bracts (Endress, 1999; Ronse de Craene, 2007, 2018; Nakagawa and Fujimoto, 2020). In Anemone and Eranthis species, three to six bracts are arranged bilaterally. In addition, A. hepatica typically has three bracts and trimerous flowers; however, flowers with four bracts tend to have tetramerous double-whorled arrangements. It would also be interesting to study whether such outer structures bias to the 99° and 137° angles resulting in different merosities. Investigating its effect on the polymorphism of spiral and whorls may therefore elucidate the underlying mechanism of merosity diversification in angiosperm flowers.
Data Availability Statement
All datasets generated for this study are included in the article/Supplementary Material.
Author Contributions
KF contributed conception and design of the study and performed the field work. MK and KF performed the mathematical modeling, wrote the manuscript, read, and approved the submitted version.
Funding
This work was supported by Grants-in-Aid for Scientific Research from Ministry of Education, Culture, Sports, Science, and Technology of Japan to KF (17H06386, and 16H06378).
Conflict of Interest
The authors declare that the research was conducted in the absence of any commercial or financial relationships that could be construed as a potential conflict of interest.
Acknowledgments
We thank the Kuwagata family, S. Maeda and K. Horibe for their support in field work.
Supplementary Material
The Supplementary Material for this article can be found online at: https://www.frontiersin.org/articles/10.3389/fevo.2020.00070/full#supplementary-material
References
Adler, I., Barabé, D., and Jean, R. V. (1997). A history of the study of phyllotaxis. Ann. Bot. 80, 231–244. doi: 10.1006/anbo.1997.0422
Besnard, F., Refahi, Y., Morin, V., Marteaux, B., Brunoud, G., Chambrier, P., et al. (2014). Cytokinin signalling inhibitory fields provide robustness to phyllotaxis. Nature 505, 417–421. doi: 10.1038/nature12791
Bravais, L., and Bravais, A. (1837a). Essai sur la disposition des feuilles curviseriees. Ann. Sci. Nat. Sec. Ser. 7, 42–110.
Bravais, L., and Bravais, A. (1837b). Essai sur la disposition symmetrique des inflorescences. Ann. Sci. Nat. Sec. Ser. 8, 11–42.
Buzgo, M., Soltis, P., Kim, S., and Soltis, D. E. (2005). The making of the flower. Biologist 52, 149–154.
Coen, E. S., and Meyerowitz, E. M. (1991). The war of the whorls: genetic interactions controlling flower development. Nature 353, 31–37. doi: 10.1038/353031a0
Cunnell, G. J. (1958). Aestivation in Ranunculus repens L. New Phytol. 57, 340–352. doi: 10.1111/j.1469-8137.1958.tb05323.x
Damerval, C., and Nadot, S. (2007). Evolution of perianth and stamen characteristics with respect to floral symmetry in Ranunculales. Ann. Bot. 100, 631–640. doi: 10.1093/aob/mcm041
de-Paula, O. C., Assis, L. C., and de Craene, L. P. R. (2018). Unbuttoning the ancestral flower of angiosperms. Trend. Plant Sci. 23, 551–554. doi: 10.1016/j.tplants.2018.05.006
Douady, S., and Couder, Y., (1996). Phyllotaxis as a dynamical self organizing process part I: The spiral modes resulting from time-periodic iterations. J. Theor. Biol.178, 255–273. doi: 10.1006/jtbi.1996.0024
Doust, A. N. (2001). The developmental basis of floral variation in Drimys winteri (Winteraceae). Int. J. Plant Sci. 162, 697–717. doi: 10.1086/320790
Endress, P. K. (1999). Symmetry in flowers: diversity and evolution. Int. J. Plant Sci. 160, S3–S23. doi: 10.1086/314211
Endress, P. K. (2001b). The flowers in extant basal angiosperms and inferences on ancestral flowers. Int. J. Plant Sci. 162 1111–1140. doi: 10.1086/321919
Endress, P. K., (2001a). “Origins of flower morphology,” in The Character Concept in Evolutionary Biology, ed G. P. Wagner (Academic Press), 493–510. Available online at: https://www.elsevier.com/books/the-character-concept-in-evolutionary-biology/wagner/978-0-12-730055-9
Endress, P. K., and Doyle, J. A. (2009). Reconstructing the ancestral angiosperm flower and its initial specializations. Am. J. Bot. 96, 22–66. doi: 10.3732/ajb.0800047
Foster, T., Johnston, R., and Seleznyova, A. (2003). A morphological and quantitative characterization of early floral development in apple (Malus × domestica Borkh.). Ann. Bot. 92, 199–206. doi: 10.1093/aob/mcg120
Hill, J. P., and Malmberg, R. L. (1996). Timing of morphological and histological development in premeiotic anthers of Nicotiana Tabacum cv. Xanthi (Solanaceae). Am. J. Bot. 83, 285–295. doi: 10.1002/j.1537-2197.1996.tb12709.x
Hu, G.-W., Lei, L.-G., Liu, K.-M., and Long, C.-L. (2009). Floral development in Nymphaea tetragona (Nymphaeaceae). Bot. J. Linn. Soc. 159, 211–221. doi: 10.1111/j.1095-8339.2008.00905.x
Kitazawa, M. S., and Fujimoto, K. (2014). A developmental basis for stochasticity in floral organ numbers. Front. Plant Sci. 5:545. doi: 10.3389/fpls.2014.00545
Kitazawa, M. S., and Fujimoto, K. (2015). A dynamical phyllotaxis model to determine floral organ number. PLoS Comput. Biol. 11:e1004145. doi: 10.1371/journal.pcbi.1004145
Kitazawa, M. S., and Fujimoto, K. (2016a). Relationship between the species-representative phenotype and intraspecific variation in Ranunculaceae floral organ and Asteraceae flower numbers. Ann. Bot. 117, 925–935. doi: 10.1093/aob/mcw034
Kitazawa, M. S., and Fujimoto, K. (2016b). Stochastic occurrence of trimery from pentamery in floral phyllotaxis of Anemone (Ranunculaceae). Acta Soc. Bot. Pol. 85, 1–10. doi: 10.5586/asbp.3530
Kitazawa, M. S., and Fujimoto, K. (2018). Spiral phyllotaxis underlies constrained variation in Anemone (Ranunculaceae) tepal arrangement. J. Plant Res. 131, 459–468. doi: 10.1007/s10265-018-1025-x
Kubitzki, K. (1987). Origin and significance of trimerous flowers. Taxon 36, 21–28. doi: 10.1002/j.1996-8175.1987.tb03919.x
Kwiatkowska, D. (1999). Formation of pseudowhorls in Peperomia verticillata (L.) A. Dietr. shoots exhibiting various phyllotactic patterns. Ann. Bot. 83, 675–685. doi: 10.1006/anbo.1999.0875
Lyndon, R. F. (1978). Phyllotaxis and the initiation of primordia during flower development in Silene. Ann. Bot. 42, 1349–1360. doi: 10.1093/oxfordjournals.aob.a085581
Mirabet, V., Besnard, F., Vernoux, T., and Boudaoud, A. (2012). Noise and robustness in phyllotaxis. PLoS Comput. Biol. 8:e1002389. doi: 10.1371/journal.pcbi.1002389
Nakagawa, A., Kitazawa, M. S., and Fujimoto, K., (2020). A design principle for floral organ number and arrangement in flowers with bilateral symmetry. Development 147:dev182907. doi: 10.1242/dev.182907
Ørgaard, M. (1991). The genus Cabomba (Cabombaceae) - a taxonomic study. Nord. J. Bot. 11, 179–203. doi: 10.1111/j.1756-1051.1991.tb01819.x
Padgett, D. (2007). A monograph of Nuphar (Nymphaeaceae). Rhodora 109, 1–95. doi: 10.3119/0035-4902(2007)109[1:AMONN]2.0.CO;2
Refahi, Y., Brunoud, G., Farcot, E., Jean-Marie, A., Pulkkinen, M., Vernoux, T., et al. (2016). A stochastic multicellular model identifies biological watermarks from disorders in self-organized patterns of phyllotaxis. Elife 5:e14093. doi: 10.7554/eLife.14093
Ren, Y. I., Chang, H.-L., and Endress, P. K. (2010). Floral development in anemoneae (Ranunculaceae). Bot. J. Linn. Soc. 162, 77–100. doi: 10.1111/j.1095-8339.2009.01017.x
Ronse de Craene, L. P. (2007). Are petals sterile stamens or bracts? The origin and evolution of petals in the core eudicots. Ann. Bot. 100, 621–630. doi: 10.1093/aob/mcm076
Ronse de Craene, L. P. (2010). Floral Diagrams: An Aid to Understanding Flower Morphology and Evolution. Cambridge: Cambridge University Press.
Ronse de Craene, L. P. (2016). Meristic changes in flowering plants: how flowers play with numbers. Flora 221, 22–37. doi: 10.1016/j.flora.2015.08.005
Ronse de Craene, L. P. (2018). Understanding the role of floral development in the evolution of angiosperm flowers: clarifications from a historical and physico-dynamic perspective. J. Plant Res. 131, 367–393. doi: 10.1007/s10265-018-1021-1
Ronse de Craene, L. P., Soltis, P. S., and Soltis, D. E. (2003). Evolution of floral structures in basal angiosperms. Int. J. Plant Sci. 164, S329–S363. doi: 10.1086/377063
Ronse de Craene, L. P. R., and Smets, E. F. (1994). Merosity in flowers: definitions, origin and taxonomic significance. Plant Syst. Evol. 191, 83–104. doi: 10.1007/BF00985344
Rudall, P. J., Remizowa, M. V., Prenner, G., Prychid, C. J., Tuckett, R. E., and Sokoloff, D. D. (2009). Nonflowers near the base of extant angiosperms? Spatiotemporal arrangement of organs in reproductive units of Hydatellaceae and its bearing on the origin of the flower. Am. J. Bot. 96, 67–82. doi: 10.3732/ajb.0800027
Salisbury, E. J. (1919). Variation in Eranthis hyemalis, Ficaria verna, and other members of the Ranunculaceae, with special reference to trimery and the origin of the perianth. Ann. Bot. 33, 47–79. doi: 10.1093/oxfordjournals.aob.a089702
Sattler, R. (1973). Organogenesis of Flowers: A Photographic Text Atlas. Toronto, ON: University of Toronto Press.
Sauquet, H., von Balthazar, M., Magallón, S., Doyle, J. A., Endress, P. K., Bailes, E. J., et al. (2017). The ancestral flower of angiosperms and its early diversification. Nat. Commun. 8:16047. doi: 10.1038/ncomms16047
Schneider, E. L., Tucker, S. C., and Williamson, P. S. (2003). Floral development in the Nymphaeales. Int. J. Plant Sci. 164, S279–S292. doi: 10.1086/376883
Schöffel, K. (1932). Untersuchungen über den Blütenbau der Ranunculaceen. Planta 17, 315–371. doi: 10.1007/BF01909279
Schoute, J. C. (1935). On corolla aestivation and phyllotaxis of floral phyllomes. Verh kon akad Wet Amsterdam Afd Natuurk 34, 1–77.
Smyth, D. R. (2018). Evolution and genetic control of the floral ground plan. New Phytol. 220, 70–86. doi: 10.1111/nph.15282
Sokoloff, D. D., Remizowa, M. V., Bateman, R. M., and Rudall, P. J. (2018). Was the ancestral angiosperm flower whorled throughout? Am. J. Bot. 105, 5–15. doi: 10.1002/ajb2.1003
Soltis, P. S., Soltis, D. E., Kim, S., Chanderbali, A., and Buzgo, M. (2006). “Expression of floral regulators in basal angiosperms and the origin and evolution of ABC-function” in Advances in Botanical Research 44: Developmental Genetics of the Flower, eds D. E. Soltis, J. H. Leebens-Mack, and P. S. Soltis. (San Diego, CA: Elsevier), 483–506.
Vislobokov, N. A., Sokoloff, D. D., Degtjareva, G. V., Valiejo-Roman, C. M., Kuznetsov, A. N., and Nuraliev, M. S. (2014). Aspidistra paucitepala (Asparagaceae), a new species with occurrence of the lowest tepal number in flowers of Asparagales. Phytotaxa 161, 270–282. doi: 10.11646/phytotaxa.161.4.2
Wang, P., Liao, H., Zhang, W., Yu, X., Zhang, R., Shan, H., et al., (2015). Flexibility in the structure of spiral flowers and its underlying mechanisms. Nat. Plants 2:15188. doi: 10.1038/nplants.2015.188
Warner, K. A., Rudall, P. J., and Frohlich, M. W. (2008). Differentiation of perianth organs in Nymphaeales. Taxon 57, 1096–1109. doi: 10.1002/tax.574006
Yule, G. U., (1902). Variation of the number of sepals in Anemone nemorosa. Biometrika 1, 307–308. doi: 10.2307/2331542
Keywords: phyllotaxis, whorl, spiral, Ranunculaceae, floral development, floral evolution, phenotypic polymorphism, Fibonacci number
Citation: Kitazawa MS and Fujimoto K (2020) Perianth Phyllotaxis Is Polymorphic in the Basal Eudicot Anemone and Eranthis Species. Front. Ecol. Evol. 8:70. doi: 10.3389/fevo.2020.00070
Received: 06 December 2019; Accepted: 04 March 2020;
Published: 27 March 2020.
Edited by:
Louis Philippe Ronse De Craene, Royal Botanic Garden Edinburgh, United KingdomReviewed by:
Florian Jabbour, Muséum National d'Histoire Naturelle, FranceDmitry D. Sokoloff, Lomonosov Moscow State University, Russia
Copyright © 2020 Kitazawa and Fujimoto. This is an open-access article distributed under the terms of the Creative Commons Attribution License (CC BY). The use, distribution or reproduction in other forums is permitted, provided the original author(s) and the copyright owner(s) are credited and that the original publication in this journal is cited, in accordance with accepted academic practice. No use, distribution or reproduction is permitted which does not comply with these terms.
*Correspondence: Koichi Fujimoto, fujimoto@bio.sci.osaka-u.ac.jp