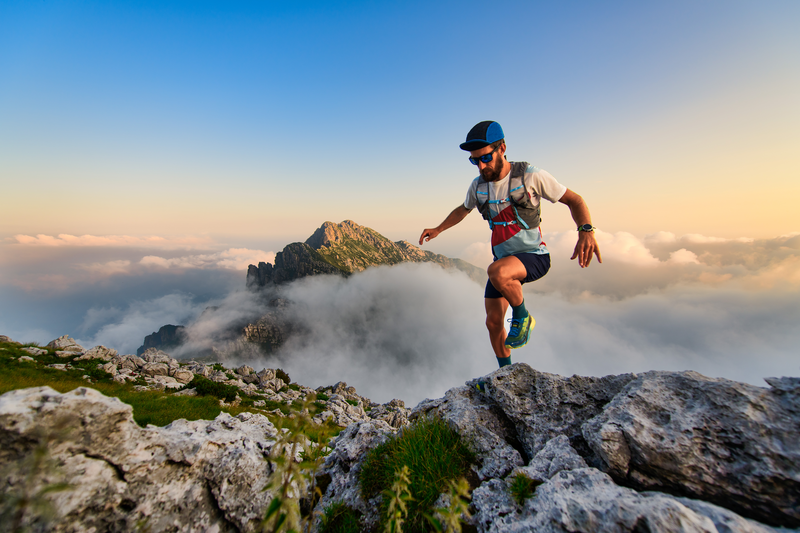
95% of researchers rate our articles as excellent or good
Learn more about the work of our research integrity team to safeguard the quality of each article we publish.
Find out more
BRIEF RESEARCH REPORT article
Front. Ecol. Evol. , 31 March 2020
Sec. Paleontology
Volume 8 - 2020 | https://doi.org/10.3389/fevo.2020.00059
The partial skeleton of a new extinct taxon, Linxiavis inaquosus, from the Liushu Formation (6–9 Ma) at the edge of the Tibetan Plateau in Gansu Province, China is the most substantial known fossil record of sandgrouse (Pteroclidae). While adding to the rapidly growing known Liushu avian fauna of vultures, falcons, pheasants, and ostrich, this new fossil is likely the oldest record of crown Pteroclidae (as a potential molecular clock calibration point), the oldest record of the group in Asia (from a probable African origin), and derives from a significant temporal gap in their Neogene history. The fossil specimen includes articulated and associated elements of the wings, shoulder girdle, vertebrae, and hind limb, exhibiting apomorphies of Columbiformes and Pteroclidae such as a notarium, and a short coracoid shaft. As part of the diverse Hipparion fauna, Linxiavis inaquosus reinforces the interpretation of the late Miocene Linxia Basin habitat as an arid savannah, associated with the uplift of the Tibetan Plateau. The holotype suggests that the arid high elevation Tibetan area habitats may have been continuously occupied since the late Miocene by sandgrouse carrying water in their modified breast feathers to their young.
Family Pteroclidae is a small clade of largely granivorous, dove-like, ground-dwelling birds commonly called sandgrouse, comprising 16 extant species placed in two genera (Pterocles and Syrrhaptes) spread across Africa and Eurasia, occurring in largely dry or arid treeless habitats such as deserts and savannahs, where they nest on the ground (Del Hoyo et al., 1997). All species of the family exhibit a suite of morphological and physiological adaptations for those hot arid habitats (Thomas, 1984), and sandgrouse are probably best known for their unique behavior of absorbing and carrying water in modified breast and abdominal feathers (Joubert and Maclean, 1973). The two species of Syrrhaptes are restricted to Asia, while the remaining 14 species in Pterocles occur across Eurasia and Africa. Syrrhaptes tibetanus is the only extant species distributed across the Tibetan Plateau and adjacent high elevation mountainous areas between 3,600 and 6,000 m (Del Hoyo et al., 1997; BirdLife International, 2016).
The phylogenetic position of Pteroclidae among extant birds has been long debated and Pteroclidae has been seen as relatives of Galliformes (Beddard, 1898; Shufeldt, 1901), Charadriiformes (Maclean, 1967), and Columbidae (Columbiformes) [see summaries in (Fjeldså, 1976; Johnsgard, 1987; De Juana, 1997; Mayr, 2011)]. While morphological data support a sister relationship between Pteroclidae and Columbidae in a clade termed Columbiformes (Mayr and Clarke, 2003; Livezey and Zusi, 2007), the latest molecular and genomic data support hypotheses that include Pteroclidae as the sister group either to the Madagascan Mesitornithidae with Columbidae as their sister clade (Jarvis et al., 2014; Prum et al., 2015), or with pteroclids as sister to Columbidae + Mesitornithidae (Hackett et al., 2008). Morphological characters that have been used to link Pteroclidae and Columbidae include the presence of a notarium, inflated pterygoids, the similar morphology of the stocky humerus, and the occurrence of a large index process on the proximal phalanx of the major manual digit (Mayr, 2011).
The phylogeny of the crown clade of Pteroclidae is not well-studied, and the most comprehensive analysis utilizing external morphological characters and molecular data (Cohen, 2011) supports the monophyly of Syrrhaptes and the paraphyly of Pterocles. The grouping of the species placed in Syrrhaptes has long been recognized by a suite of derived characters including dorsally feathered feet, loss of pedal digit I, and the occurrence of a form of syndactyly with the pedal digits “fused” along part of their length within a sheath of connective tissue forming a structure considered similar to a mammalian paw (Van Grouw, 2013).
The fossil record of Pteroclidae is relatively poor, and none of the fossils attributed to Pteroclidae have been analyzed phylogenetically. The oldest fossils (Archaeoganga spp.) allocated to the clade are from the late Eocene to early Oligocene phosphatic fissure fill deposits in Quercy, France (Mourer-Chauviré, 1992, 1993; Mayr, 2016), and the youngest extinct pteroclid fossils (Leptoganga) are from the early Miocene (Aquitanian; MN1 and 2A; ~20–23.8 Ma) of France (Mourer-Chauviré, 1993). These early fossils do not appear to be members of the crown group based on characters present in the coracoid, including the presence of a long coracoid shaft which is shared (plesiomorphically) with Columbidae (Mourer-Chauviré, 1992, 1993). A number of Quaternary specimens referred to extant species of both crown genera are known from paleontological and archeological sites across Eurasia (Brodkorb, 1971; Hou, 1993; Gala and Tagliacozzo, 2014). Molecular clock estimates for the origin of the pteroclid lineage are in the Paleocene (Jarvis et al., 2014) or early Eocene (Prum et al., 2015; Soares et al., 2016), and the crown clade has been hypothesized to have originated in the late Oligocene (Soares et al., 2016) or early Miocene (Prum et al., 2015). The oldest fossil specimens of pteroclids have been interpreted as occurring in open and arid environments (Mourer-Chauviré, 1992), and many of the reported Quaternary pteroclid fossils derive from drier habitats with specimens from Beijing (Hou, 1993) and Inner Mongolia in China (Boule, 1928), and Iran (Gala and Tagliacozzo, 2014). Those data suggest that the biological adaptations and preferences present among extant sandgrouse likely have a deep evolutionary origin.
Adding significant data to our knowledge of the evolution of sandgrouse is a partial skeleton representing a new pteroclid taxon from the late Miocene of the Linxia Basin in Gansu Province, China, adjacent to the Tibetan Plateau (Figure 1). Furthermore, this specimen is the oldest associate skeleton of the group, and the first sandgrouse record from the late Miocene, occurring in a temporal gap in their fossil record between the early Miocene and Quaternary, when the crown clade likely radiated. Importantly, this fossil also was found at the edge of the Tibetan Plateau where the extant Tibetan sandgrouse lives.
Figure 1. Map of the holotype locality of Linxiavis inaquosus and other nearby fossil bird localities in central China adjacent to the Tibetan Plateau. The red box indicates the holotype locality for the new sandgrouse, and the green boxes mark previously published fossil bird sites (e.g., Hezheng, Guanghe).
The presence of an extinct sandgrouse species in the late Miocene deposits of the Linxia Basin (Figure 1) adds to the growing knowledge of its diverse avifauna including an ostrich (Hou et al., 2005), a stem falcon (Li et al., 2014), old world vultures (Zhang et al., 2010; Li et al., 2016), a pheasant with a hyperelongated trachea (Li et al., 2018), and an eogruid (Musser et al., 2019). The new fossil sandgrouse occupied dry, arid habitats alongside the well-known, diverse extinct mammalian fauna from the Linxia Basin. The collective vertebrate fossil record supports the past presence of a steppe community quite similar to that of the arid savannahs of sub-Saharan Africa today with its mixture of relatives of living rhinoceroses, elephants, horses, giraffes, hyenas, vultures, ostrich, and now sandgrouse (Deng et al., 2004; Deng, 2006). Furthermore, this new fossil adds evidence to the pattern of the increased aridification in the Linxia Basin at the edge of the Tibetan Plateau in the late Miocene.
Order Columbiformes Latham 1790.
Family Pteroclidae Bonaparte 1831.
Linxiavis inaquosus gen. et sp. nov.
The partial skeleton (Institute of Vertebrate Paleontology and Paleoanthropology, Beijing; IVPP V24116) preserves most of the pectoral girdle elements and forelimbs (i.e., the humerus, ulna, radius, radiale, ulnare, coracoid, scapula, furcula, and carpometacarpus with major manual phalanges), some thoracic vertebrae (including the notarium), and fragments of the hindlimb (tibiotarsus, fibula, and femur).
The holotype is from the late Miocene Liushu Formation, near the village of Baiwang in Gansu Province, China (Figure 1: red square). A recent magnetostratigraphic study constrains the age of the Hipparion fauna beds of the Liushu Formation to 6–9 Ma (Zhang et al., 2019).
The genus name refers to the Linxia Basin where the fossil was found, combined with “avis” in reference to its avian status. The specific epithet is Latin for arid, dry, or lacking water in reference to the pteroclid clade occurring in arid habitats. A new genus level taxon is erected for this fossil because it appears to be a member of the crown clade of Pteroclidae, it is not a member of Syrrhaptes, and the genus Pterocles is almost certainly not monophyletic (awaiting a revised taxonomy).
Species diagnosis same as for the genus by monotypy. Shares with Columbidae and Pteroclidae many features including a notarium, an undulating rim of the pneumotricipital fossa, and absence of a foramen n. supracoracoidei on the coracoid. Shares with Pteroclidae and not Columbidae, a craniocaudally elongated ellipsoid cross section (rather than subcircular) of the olecranon process of the ulna; and an indistinct olecranon fossa on the humerus. Shares with crown Pteroclidae and not non-crown pteroclid taxa, a short coracoid shaft. The holotype is smaller than the extinct stem taxa Leptoganga and most species of Archaeoganga (see Supplementary Data), and it has a shorter coracoid shaft than those extinct taxa. Larger than P. namaqua, P. senegallus, P. bicinctus, P. decoratus, P. burchelli, and S. paradoxus; smaller than P. gutterlis and P. orientalis, but similar in size to P. alchata (Supplementary Data). Shares relatively flat ventral margin of the radiale (with reduction of the tendinal groove for m. ulnometacarpalis ventralis and adjacent second tendinal groove—resulting in a straighter ventral margin) and a shortened ridge on the radiale (Supplementary Data) with P. orientalis, but not P. namaqua, P. senegallus, P. bicinctus, P. decoratus, P. burchelli, P. alchata, P. gutteralis, and S. paradoxus. Species uniquely displays a straight though obtuse angled dorsal margin of the ventral cotyle of the proximal ulna, and a dorsocaudally elongate omal end of the furcula with a pointed apex (rather than squared off or a broad rounded end). The new species also differs from other pteroclids in its coracoid morphology with respect to overhanging portion of the acrocoracoid above the m. supracoracoideus fossa; the humeral articular facet is rather narrow; and the base of the acrocoracoid omal to the humeral articular facet is rather slim.
The right humerus is complete (Figure 2) with a humeral head that projects distinctly proximally. The deltopectoral crest is subtriangular in outline with a tubercular apex located just distal to the proximodistal level of the transverse ligamental groove (Figure 3). The pneumotricipital fossa is partially closed by a bony wall perforated by foramina. The ventral rim of the pneumotricipital fossa is expanded as in Syrrhaptes, but it appears that there are no pneumatic foramina on the expanded rim in the fossil (unlike Syrrhaptes). However, the surface is irregular with small pits that might be pneumatic in origin. The dorsal crus of the pneumotricipital fossa is short and oriented more dorsoventrally. There is a distinct m. scapulotriceps groove on the distal end. It appears that the flexor process and dorsal condyle extended approximately to the same distal level. However, the ventral condyle extends distal to both the dorsal condyle and flexor process. There is no distinct olecranon fossa on the specimen, with only a shallowly concave area. The dorsal supracondylar tubercle is proximodistally elongate (not a rounded tubercle) and located far proximally (proximal even to the level of the proximal end of the flexor process's base).
Figure 2. Photograph and colorized CT reconstruction of the major bones for the holotype of Linxiavis inaquosus (IVPP V24116). Anatomical abbreviations: bovi-sk–bovid skull, cor–coracoid, cm–carpometacarpus, fe–femur, fi–fibula, fur–furcula, hum–humerus, lcm–left carpometacarpus, lhu–left humerus, lra–left radius, lul–left ulna, mII–metacarpal II, mIII–metacarpal III, mdII–manual digit III phalanx 2, not–notarium, ra–radius, rad–radialae, rhu–right humerus, rra–right radius, rul–right ulna, sc–scapula, ti–tibia, tv–thoracic vertebrate centrum, ul–ulna, uln–ulnare. Scale bar equals 1 cm.
Figure 3. Extracted individual bone elements to show detailed features of the forelimb and shoulder girdle of Linxiavis inaquosus (IVPP V24116). (A,B) cranial and caudal view of the right humerus; (C,D) dorsal and ventral view of the proximal forelimb (left side) with a focus of the ulna radius and carpal bones; (E,F) dorsal and ventral view of the distal forelimb with a focus of the carpometacarpus and phalanges (left side); (G,H) lateral and dorsomedial view of the shoulder girdle. Anatomical abbreviations: ac–acrocoracoid process of coracoid, acr–acromial process of scapula, af–acromial process of furcula, ap–alular process, br–brachial fossa, cp–caudal process, dc–dorsal condyle, dr–deltopectoral crest, ds–dorsal supracondylar tubercle, ep–extensor process, hf–humeral articular facet of coracoid, hh–humeral head, ins–intermetacarpal space, mei–metacarpal incisura, pf–pneumotricipital fossa, rad–radiale, sf–sternal articular facet of coracoid, sy–symphysis of furcula, ts–tendinal sulcus, tu–tubercle, uln–ulnare, vc–ventral condyle. Scale bar equals 1 cm.
The articulated approximately distal two-thirds of the left radius and ulna lie above the proximal left humerus. A few small feather papillae are preserved on the left ulnar shaft, but not the right shaft. The distal end of the right ulna preserves a short blunt carpal tubercle lacking any notch. The right ulna preserves the proximal end of the bone with the olecranon process being elongate craniocaudally (not rounded in cross-section; Supplementary Figure 1). The outline of the ventral cotyle is roughly diamond shaped, but that is in part the result of the loss of bone on the ventral aspect of the cotyle. However, the straight obtuse angled dorsal margin of the ventral cotyle does not appear to be an artifact (Supplementary Figure 1). The dorsal margin of the cotyle is curved in Syrrhaptes with a subcircular outline of the cotyle in all species of Pterocles examined. The dorsal cotyle projects cranially extending cranial to the ventral cotyle with its apex at the cotyle's dorsal edge. Much of the proximal ventral surface of the right ulna is covered by another avian long bone that may be a portion of the shaft of the tibiotarsus or femur. The ulna is longer than the humerus (see Supplementary Table 1).
In distal view, the radiale is roughly trapezoidal in outline with the narrow side on the dorsal aspect (Supplementary Figure 1; Figure 3). The ridge cranially adjacent to the articular face for the radius is a continuous ridge that does not extend to the ventral end of the bone. The ventral margin of the bone is relatively straight and has a less distinct notch for the passage of the tendon of m. ulnometacarpalis ventralis than that present in most species of Pterocles (except P. orientalis and P. gutteralis), Syrrhaptes, and Columbidae (Mayr, 2014).
The ulnare has a long dorsal crus that is relatively squared off (or blocky in appearance), and slightly longer than the short crus (Supplementary Figure 1) that is very similar to the states in P. decoratus, P. burchelli, P. orientalis, and P. gutteralis (Figure 4). The metacarpal incisure is relatively shallow but wide, and the margin of the ulnare opposite the incisure is slightly concave (shared with P. namaqua). That concave margin is absent in P. senegallus where is it convex, and it is more deeply concave in P. bincintus and P. orientalis. The tubercle on the proximal end at the base of the long crus is broken away in the fossil.
Figure 4. Ulnare morphology in different species of Pteroclidae, (A) Pterocles bicinacitus (USNM 429081), (B) P. burchelli (USNM 430845), (C) P. gutteralis (USNM 488869), (D) P. decoratus (USNM 636741), (E) P. orientalis (USNM 344845), and (F) P. namaqua (USNM 64231). Scale bar equals 5 mm. USNM - the bird division of the National Museum of Natural History, Washington, DC, USA.
The preserved dorsal surface of the proximal end of the left carpometacarpus is concave. The preserved major metacarpals do not exhibit a small intermetacarpal process. The major metacarpal has a dorsally concave area for the passage of a tendon only at its distal end. Only parts of the minor metacarpals are preserved, making it appear that the intermetacarpal space widened distally, but the shape of the space differs slightly between the left and right sides. The concave proximal facet of the minor digit phalanx is preserved. The proximal phalanx of the left major digit has a small process on the cranial proximal base of the phalanx. There are two foramina near the center of the large phalanx of the major digit (Supplementary Figure 1). The flattened caudal portion of the phalanx is incomplete with its distal end worn away, and the flattened portion extends distal to the distal articular facet. The distal phalanx of the major digit has a concave face on its dorsocaudal proximal base. The distal phalanx has a small cranial process on the proximal end of its cranial face (in the ventral half of the phalanx).
There does not appear to have been a distinct hypocleideum or expansion near the synostosis of the furcular rami (Figures 2, 3). The sternal portion of the furcula and rami are flattened craniocaudally. The rami expand in their craniocaudal width dorsally. The articular surface of the furcula is craniocaudally elongate (offset from the dorsoventrally oriented ramus), and it comes to a narrowing, omally positioned, rounded point with a flattened medial surface (Figure 5). There does not appear to be a distinct mediolaterally expanded facet for coracoid articulation as there is in P. namaqua and P. alchata, and the apex of the furcular rami is squared off in P. gutteralis and P. senegallus.
Figure 5. Detailed articular anatomy of coracoid-scapula-furcula of Pterocles bicinctus (USNM 429081) (A) and Linxiavis inaquosus (B).
Medial portion of the sternal articulation of the coracoid has two distinct articulation surfaces at an angle to one another; a wider and rounder ventral articulation and a mediolaterally wide (but dorsoventrally narrow) facet on the dorsal aspect. The acrocoracoid is missing its ventral tip, but has a hooked facet for the furcula (as in Syrrhaptes and species of Pterocles). In the CT imagery, it appears that there is a pneumatic foramen (or at least a deep pit) in the m. supracoracoideus fossa on the caudal face of the acrocoracoid underneath the facet for the furcula. None of the extant species examined have a large pit or foramen, but several species (P. bincintus, P. burchelli, P. gutteralis, P. orientalis, and P. decoratus) exhibit a few small foramina under the facet for the furcula (Figure 6). There is no foramen for the n. supracoracoidei on the coracoid, and the procoracoid process appears short (but it may be broken or not rendering properly in the CT imagery).
Figure 6. Coracoid morphology of the new species Linxiavis inaquosus in comparison to extant species of Pterocles. (A,B) P. burchelli (USNM 430845); (C,D) P. namaqua (USNM 64231); (E,F) P. decoratus (USNM 636741); (G,H) P. orentalis (USNM 344845); (I,J) S. paradoxus (IVPP OV973); (K,L) Linxiavis inaquosus. Scale bar equals 1 cm.
The right scapula is preserved in articulation with the coracoid. The scapular blade lies alongside the notarium and on top of the bovid skull fragment (Figure 2). The distal blade is as wide or even wider than the proximal shaft, and was very long, extending caudally to near the caudal end of the most caudal thoracic vertebra, as in extant species. The acromion process is long and projects dorsally, and cranial end of the bone is quite similar to that of P. bicinctus (Figure 5).
The lateral condyle of the distal left femur has a rather short tibiofibularis crest, and the tubercle likely for the m. gastrocnemius lateralis positioned distally and not elevated relative to adjacent areas of the bone (Supplementary Figure 1), as in P. bicinctus, P. decoratus, P. burchelli, and P. gutteralis. The area is slightly elevated in P. namaqua, P. senegallus, and P. alchata. The two bone fragments adjacent to the medial edge of the femoral fragment likely are the proximal ends of the left tibiotarsus and fibula missing their proximal surfaces (Figure 2; Supplementary Figure 1). They only preserve a small proximal interosseus foramen between the bones.
A free thoracic vertebral centrum adjacent to the proximal tibiotarsus fragment has a rounded ventral face lacking any crest (Figure 2; Supplementary Figure 1). The notarium is present cranial to the free vertebrae, preserved as a set of fused and broken vertebrae with distinct thin ventral keels that extends for approximately the length of four vertebral centra (consistent with the notarium composed of four vertebrae in Pteroclidae and Columbidae). Shufeldt (1901) mentioned the presence of a notarium composed of three vertebrae in Syrrhaptes, and we can confirm a notarium of three vertebrae (in what appears to be an otherwise adult skeleton with all leg bone fusions and smooth bone surfaces) is present in Pterocles namaqua (USNM 642431) and P. senegallus (USNM 641935). The two free lumbar vertebrae in those specimens lack a thin ventral keel. In Pterocles orientalis (USNM 18850 and 344845), the fourth (more cranial) vertebra is incompletely fused to the other unit of three (more caudal) vertebrae. This likely ontogenetic character may suggest that the holotype individual is an older adult specimen with its completely fused notarium of four vertebrae. However, the distribution of the two free caudal thoracic vertebrae with a three vertebrae notarium in some species, and the complete fusion of the more caudal vertebra, but not the cranial vertebra in P. orientalis suggests either differing ontogenetic pathways among these species, or heterogeneity in the growth of the notarium across pteroclids generally (see Supplementary Data for longer description and a list of museum skeletons we examined).
The fossil shares many characters with Columbidae and Pteroclidae (Columbiformes) including the cranially projecting triangular shape of the deltopectoral crest, the rim of the pneumotricipital fossa that undulates with a ventral (proximally directed) concavity to its margin, a humeral dorsal tubercle flush with the shaft, the procoracoid process lacking a foramen (for n. supracoracoidei), large sternal articulation facets on the coracoid, a large inflated acrocoracoid with a medial hook, and a proximally positioned dorsal supracondylar tubercle on the humerus (Worthy et al., 2009; Worthy, 2012). The fossil has a concave scapular cotyle, a relatively short coracoid shaft, and the pneumatic pocket within the impression of m. sterncoracoidei is on the lateral aspect (rather than medial), and those features are shared with extant Pteroclidae, not Columbidae. Other features of the skeleton including the elongate dorsal supracondylar process of the humerus, absence of a distinct olecranon fossa on the humerus, and an elongate contact surface on the furcula for the acrocoracoid further help to differentiate the fossil and Pteroclidae from Columbidae. Within Pteroclidae, the holotype displays a mosaic of features shared with different groups of extant species (see above) indicating homoplasy in details of character evolution among pteroclids. The gross similarities appear to be with P. orientalis in having very similar radiale and ulnare morphologies, but not with its close relative Syrrhaptes (Figure 4). Features also are shared more frequently among the species examined, with P. decoratus and P. burchelli. In other words, the skeleton of Linxiavis inaquosus fits easily within the variation present among the extant species of Pteroclidae.
Extant sandgrouse occur across a variety of habitat types, but are specialists of some of the driest parts of world, such as North Africa and Central Asia (including the high elevation Tibetan Plateau) (Del Hoyo et al., 1997). The unique ability and behavior of male individuals to absorb water in their breast and abdominal feathers and carry it to supply their young (Cad and Maclean, 1967), in addition to other arid associated adaptations (Thomas, 1984), provide the essential tools for surviving where other birds typically do not venture to breed and reside. Their exceptional adaptations can be seen in the Tibetan sandgrouse that occurs at extreme elevations up to 6,000 m (BirdLife International, 2016). Most likely Linxiavis foraged and nested on the ground as in extant sandgrouse and the primitive state for crown columbids (Lapiedra et al., 2013). Given that most extant sandgrouse are granivores (Del Hoyo et al., 1997), this extinct species is the only likely granivore of the Hipparion fauna in Liushu Formation, extending the ecological diversity among the fossil vertebrates.
Previously, the oldest fossils of the pteroclid crown group were from the Quaternary, with some stem group fossils extending back into the late Eocene (Mourer-Chauviré, 1992, 1993). The youngest known stem fossils are from the early Miocene (Mourer-Chauviré, 1993) and are within the age range estimated by molecular clocks for the origin of the crown group [late Oligocene to early Miocene (Prum et al., 2015; Soares et al., 2016)]. No fossils of sandgrouse are known from the Pliocene, and the description of Linxiavis begins to fill a nearly 20 million-year long gap in their paleontological history during which the sandgrouse crown clade radiated, left Africa, and reached the rising Tibetan Plateau in Central Asia. Importantly, Linxiavis appears to be a member of the crown clade, and it is the oldest record of Pteroclidae in Asia. Those facts may provide data anchors for future molecular clock analyses focused on determining the dates of origin and rates of evolution of the crown clade and its component lineages. While the exact stratigraphic position of the holotype locality is not known, the minimum age of the Liushu Formation is 6.0 Ma, providing a minimum date for the crown group, the dispersal of sandgrouse out of Africa and into Central Asia, and possibly for the Central Asian sandgrouse group of species.
Based on the molecular phylogeny of (Cohen, 2011) it appears likely that the crown clade of Pteroclidae evolved in Africa with the basal species of the paraphyletic Pterocles having an African geographic distribution. Thus, the occurrence of pteroclids in Asia would be the result of a geographic range expansion through immigration. With this Chinese fossil as the oldest record of the group in Asia, it places a minimum age (~6.0 Ma) on the Africa to Asia dispersal of the crown group. Furthermore, this fossil demonstrates that the occurrence of sandgrouse in Asia occurred millions of years before Quaternary climate change and during the uplift of the Tibetan Plateau with its associated aridification. The dispersal of mammals between Africa and Asia also is well-known in the late Miocene (Bibi, 2011), and Linxiavis adds a bird to the list of Africa to Asia immigrants during this time.
It does not appear that this extinct species is a close relative of Syrrhaptes despite its location adjacent to the Tibetan Plateau where only S. tibetanus occurs today, south of the geographic range of S. paradoxus, and far from the nearest member of Pterocles (i.e., P. orientalis) where it is partially sympatric with S. paradoxus in northwestern China (Mackinnon et al., 2000). It is possible given the current molecular clock estimates for the crown clade origin (early Miocene or earlier, see above), the morphological similarities shared between Linxiavis and P. orientalis, and the geographic location of this fossil within the extant geographic range of Syrrhaptes, that Linxiavis may be a late Miocene member of the Central Asian clade comprising Syrrhaptes and P. orientalis (Cohen, 2011). The earlier diversification occurred within the Central Asian, different from later occupation of the region by P. orientalis and Syrrhaptes (Cohen, 2011). If correct, the occurrence of this fossil at the edge of the Tibetan Plateau within the geographic range of extant Syrrhaptes, also may relate to the origin of the lineage leading to the high elevation specialist S. tibetanus found across the Tibetan Plateau today. However, Linxiavis also could be a member of a different Asian clade, possibly related to P. indicus or other species of Pterocles not examined (Cohen, 2011). Increased sampling of modern skeletal morphology and wider phylogenetic analysis of the extant and extinct molecular and morphological material should provide greater insight into this question.
The elevation of the Linxia Basin today varies from 1,800–2,600 m with adjacent mountains extending from 3,000 to 4,000 m (Zhang et al., 2019). Accelerated uplift was inferred for the Linxia region since the middle Miocene due to the crustal shortening and thickening across northeastern Tibet (Lease, 2014). Even at present day elevations of the basin, Linxiavis occurred at higher elevations than most extant sandgrouse species, and it likely lived even higher on the adjacent rising plateau.
The known Liushu Formation vertebrate (Hipparion) fauna is composed of relatives of living rhinoceroses, elephants, hyenas, bovids, pigs, horses, falcons, pheasants, vultures, and ostriches (Deng et al., 2013). That extinct fauna indicates the past presence of a steppe/savannah set of habitats in the late Miocene of the Linxia Basin. Fossil mammalian stable isotope data support prior identified climatic variation through the millions of years of the Liushu Formation with both wetter and drier periods of time (Biasatti et al., 2010). The Liushu Formation and its fauna has been interpreted as having been deposited in warmer than today conditions of a sub or semiarid steppe/grassland environment impacted by a stronger than present day East Asian monsoon (Zhang et al., 2019). The ecological shift in the basin from forested environment to dry and open habitats began around 8 million years ago, consistent with the late Miocene age and our ecological interpretation of this sandgrouse fossil (Fan et al., 2006, 2007). Asian monsoonal storms associated with the uplift of the Tibetan Plateau are thought to have begun by 20–25 million years ago (Guo et al., 2002; Qiang et al., 2011), and were present and stronger even during the increasing aridity in the Miocene. The permanent water bodies indicated by fluvial sedimentary deposits in the Liushu Formation (Zhang et al., 2019) along with the monsoonal rains may have provided an ample water supply for male sandgrouse transporting water to their young. This hypothesis also is supported by the recently found distribution and diversification pattern of a new species of Eogruidae from the region (Musser et al., 2019).
CT data is deposited at IVPP Digital data repository ADMorph.org.
ZL, TS, TD, and ZZ: Contributed to the design of the study, interpretation of results, and manuscript preparation. ZL and TS: Performed the data analyses. All authors contributed to the writing of the manuscript.
This work was supported by: one hundred talents project from the Chinese Academy of Sciences (KC 217113) to ZL; the National Natural Science Foundation of China (NSFC 41772013) to ZL and TS; and the Strategic Priority Research Program of the Chinese Academy of Sciences (Grant No. XDA 9050102, XDA 20070203, and XDB26000000).
The authors declare that the research was conducted in the absence of any commercial or financial relationships that could be construed as a potential conflict of interest.
We thank Miao Song, Gao Wei, and Liu Xinzheng for technical assistance. Helen James and Christopher Milensky provided access to comparative material at the USNM. We thank the reviewers and editor for helpful comments on an earlier draft of the manuscript.
The Supplementary Material for this article can be found online at: https://www.frontiersin.org/articles/10.3389/fevo.2020.00059/full#supplementary-material
Beddard, F. E. (1898). The Structure and Classification of Birds. London; New York, NY; Bombay: Longmans, Green, and Company.
Biasatti, D., Wang, Y., and Deng, T. (2010). Strengthening of the East Asian summer monsoon revealed by a shift in seasonal patterns in diet and climate after 2–3 Ma in northwest China. Palaeogeogr. Palaeoclimatol. Palaeoecol. 297, 12–25. doi: 10.1016/j.palaeo.2010.07.005
Bibi, F. (2011). Mio-Pliocene faunal exchanges and African biogeography: the record of fossil bovids. PLoS ONE 6:e16688. doi: 10.1371/journal.pone.0016688
BirdLife International (2016). Syrrhaptes tibetanus. BirdLife International 2016. The IUCN Red List of Threatened Species. Available online at: http://www.birdlife.org
Boule, M. (1928). Le Paléolithique de la Chine, Vol. 4. Paris: Archives de l'Institut de paleontologie humanie, 136.
Brodkorb, P. (1971). Catalogue of Fossil Birds: Part 4 (Columbiformes Through Piciformes). Gainesville: University of Florida.
Cad, T. J., and Maclean, G. L. (1967). Transport of water by adult sandgrouse to their young. Condor Ornithol. Appl. 69, 323–343. doi: 10.2307/1366197
Cohen, C. (2011). The Phylogenetics, Taxonomy and Biogeography of African Arid Zone Terrestrial Birds: The Bustards (Otididae), Sandgrouse (Pteroclidae), Coursers (Glareolidae) and Stone Partridge (Ptilopachus). Doctoral Dissertation, University of Cape Town.
De Juana, E. (1997). “Family Pteroclidae (Sandgrouse),” in Handbook of the Birds of the World, Vol. 4 (Barcelona: Lynx Edicions), 30–57.
Del Hoyo, J., Elliott, A., Sargatal, J., and Cabot, J. (1997). Sandgrouse to Cuckoos. Barcelona: Lynx Edicions.
Deng, T. (2006). Paleoecological comparison between late Miocene localities of China and greece based on Hipparion faunas. Geodiversitas 28, 499–516.
Deng, T., Qiu, Z., Wang, B., Wang, X., and Hou, S. (2013). “Late Cenozoic biostratigraphy of the Linxia Basin, Northwestern China,” in Fossil Mammals of Asia: Neogene Biostratigraphy and Chronology, eds X. M. Wang, L. J. Flynn, M. Fortelius (New York, NY: Columbia University Press), 243–273.
Deng, T., Wang, X., Ni, X., and Liu, L. (2004). Sequence of the cenozoic mammalian faunas of the Linxia Basin in Gansu, China. Acta Geol. Sin-Engl. Ed. 78, 8–14. doi: 10.1111/j.1755-6724.2004.tb00669.x
Fan, M., Dettman, D. L., Song, C., Fang, X., and Garzione, C. N. (2007). Climatic variation in the Linxia basin, NE Tibetan Plateau, from 13.1 to 4.3 Ma: the stable isotope record. Palaeogeogr. Palaeoclimatol. Palaeoecol. 247, 313–328. doi: 10.1016/j.palaeo.2006.11.001
Fan, M., Song, C., Dettman, D. L., Fang, X., and Xu, X. (2006). Intensification of the Asian winter monsoon after 7.4 Ma: grain-size evidence from the Linxia Basin, northeastern Tibetan Plateau, 13.1 Ma to 4.3 Ma. Earth Planet. Sci. Lett. 248, 186–197. doi: 10.1016/j.epsl.2006.05.025
Fjeldså, J. (1976). The systematic affinities of sandgrouses, pteroclididae. Vidensk. Meddr Dansk Naturh. Foren. 139, 179–243.
Gala, M., and Tagliacozzo, A. (2014). The role of birds in the settlement of Shahr-i Sokhta (Sistan, Iran) during the 3rd Millennium BC. Int. J. Osteoarchaeol. 24, 319–330. doi: 10.1002/oa.2389
Guo, Z. T., Ruddiman, W. F., Hao, Q. Z., Wu, H. B., Qiao, Y. S., Zhu, R. X., et al. (2002). Onset of Asian desertification by 22 Myr ago inferred from Loess deposits in China. Natute 416, 159–163. doi: 10.1038/416159a
Hackett, S. J., Kimball, R. T., Reddy, S., Bowie, R. C. K., Braun, E. L., Braun, M. J., et al. (2008). A phylogenomic study of birds reveals their evolutionary history. Science 320, 1763–1768. doi: 10.1126/science.1157704
Hou, L. (1993). Avian fossils of Pleistocene from Zhoukoudian. Mem. Inst. Vert. Palaeont. Palaeoanthr. Acad. Sinica 19, 165–297.
Hou, L., Zhou, Z., Zhang, F., and Wang, Z. (2005). A Miocene ostrich fossil from Gansu Province, Northwest China. Chinese Sci. Bull. 50, 1808–1810. doi: 10.1360/982005-575
Jarvis, E. D., Mirarab, S., Aberer, A. J., Li, B., Houde, P., Li, C., et al. (2014). Whole-genome analyses resolve early branches in the tree of life of modern birds. Science 346, 1320–1331. doi: 10.1126/science.1253451
Johnsgard, P. A. (1987). “Diving birds of North America: 4 comparative diets and foraging ecologies,” in Diving Birds of North America, by Paul Johnsgard, ed J. P. Lincoln (Lincoln: University of Nebraska Press), 37–53.
Joubert, C. S. W., and Maclean, G. L. (1973). The structure of the water-holding feathers of the Namaqua Sandgrouse. Zool. Afr. 8, 141–152. doi: 10.1080/00445096.1973.11448511
Lapiedra, O., Sol, D., Carranza, S., and Beaulieu Jeremy, M. (2013). Behavioural changes and the adaptive diversification of pigeons and doves. Proc. Biol. Sci. 280:20122893. doi: 10.1098/rspb.2012.2893
Lease, R. O. (2014). Cenozoic mountain building on the northeastern Tibetan Plateau. Geol. Soc. Am. Spec. Pap. 507, 115–127. doi: 10.1130/2014.2507(06)
Li, Z., Clarke, J. A., Eliason, C. M., Stidham, T. A., Deng, T., and Zhou, Z. (2018). Vocal specialization through tracheal elongation in an extinct Miocene pheasant from China. Sci. Rep. 8:8099. doi: 10.1038/s41598-018-26178-x
Li, Z., Clarke, J. A., Zhou, Z., and Deng, T. (2016). A new old world vulture from the late miocene of China sheds light on Neogene shifts in the past diversity and distribution of the Gypaetinae. Auk Ornithol. Adv. 133, 615–625. doi: 10.1642/AUK-15-240.1
Li, Z., Zhou, Z., Deng, T., Li, Q., and Clarke, J. A. (2014). A falconid from the late miocene of northwestern China yields further evidence of transition in late neogene steppe communities. Auk 131, 335–350. doi: 10.1642/AUK-13-245.1
Livezey, B. C., and Zusi, R. L. (2007). Higher-order phylogeny of modern birds (theropoda, aves: Neornithes) based on comparative anatomy. II. Analysis and discussion. Zool. J. Linn. Soc. 149, 1–95. doi: 10.1111/j.1096-3642.2006.00293.x
Mackinnon, J. R., Mackinnon, J., Phillipps, K., and He, F. Q. (2000). A Field Guide to The Birds of China. New York, NY: Oxford University Press.
Maclean, G. L. (1967). Die systematische stellung der flughühner(pteroclididae). J. Für Ornithol. 108, 203–217. doi: 10.1007/BF01671410
Mayr, G. (2011). Metaves, mirandornithes, strisores and other novelties – a critical review of the higher-level phylogeny of neornithine birds. J. Zool. Syst. Evol. Res. 49, 58–76. doi: 10.1111/j.1439-0469.2010.00586.x
Mayr, G. (2014). Comparative morphology of the radial carpal bone of neornithine birds and the phylogenetic significance of character variation. Zoomorphology 133, 425–434. doi: 10.1007/s00435-014-0236-5
Mayr, G. (2016). Avian Evolution: The Fossil Record of Birds and its Paleobiological Significance. Oxford: John Wiley & Sons. doi: 10.1002/9781119020677
Mayr, G., and Clarke, J. (2003). The deep divergences of neornithine birds: a phylogenetic analysis of morphological characters. Cladistics 19, 527–553. doi: 10.1111/j.1096-0031.2003.tb00387.x
Mourer-Chauviré, C. (1992). Un ganga primitif (aves, columbiformes, pteroclidae) de très grande taille dans le paléogène des phosphorites du quercy (France). Comptes rendus de l'Académie des sciences. Série 2, Mécanique, Physique, Chimie, Sciences de l'univers, Sciences de la Terre. 314, 229–235.
Mourer-Chauviré, C. (1993). Les Gangas (aves, columbiformes, pteroclidae) du paleogene et du miocene inferieur de France. Palaeovertebrata 22, 73–98.
Musser, G., Li, Z., and Clarke, J. A. (2019). A new species of Eogruidae (Aves: Gruiformes) from the miocene of the Linxia Basin, Gansu, China: evolutionary and climatic implications. Auk 137, 1–13. doi: 10.1093/auk/ukz067
Prum, R. O., Berv, J. S., Dornburg, A., Field, D. J., Townsend, J. P., Lemmon, E. M., et al. (2015). A comprehensive phylogeny of birds (Aves) using targeted next-generation DNA sequencing. Nature 526, 569–573. doi: 10.1038/nature15697
Qiang, X., An, Z., Song, Y., Chang, H., Sun, Y., Liu, W., et al. (2011). New eolian red clay sequence on the western Chinese Loess Plateau linked to onset of Asian desertification about 25 ma ago. Sci. China Earth Sci. 54, 136–144. doi: 10.1007/s11430-010-4126-5
Shufeldt, R. W. (1901). On the systematic position of the Sand Grouse (pterocles; syrrhaptes). Am. Nat. 35, 11–16. doi: 10.1086/277842
Soares, A. E., Novak, B. J., Haile, J., Heupink, T. H., Fjeldså, J., Gilbert, M. T. P., et al. (2016). Complete mitochondrial genomes of living and extinct pigeons revise the timing of the columbiform radiation. BMC Evol. Biol. 16:230. doi: 10.1186/s12862-016-0800-3
Thomas, D. H. (1984). Sandgrouse as models of avian adaptations to deserts. S. Afr. J. Zool. 19, 113–120. doi: 10.1080/02541858.1984.11447868
Van Grouw, K. (2013). The Unfeathered Bird. Princeton, NJ; Oxford: Princeton University Press. doi: 10.1515/9781400844890
Worthy, T., Hand, S. P., Worthy, J., Tennyson, A., and Scofield, R. (2009). A Large Fruit Pigeon (Columbidae) from the early miocene of New Zealand. Auk 126, 649–656. doi: 10.1525/auk.2009.08244
Worthy, T. H. (2012). A phabine pigeon (aves: columbidae) from oligo-miocene Australia. Emu-Austral Ornithol. 112, 23–31. doi: 10.1071/MU11061
Zhang, W., Appel, E., Jiuyi, W., Xiaomin, F., Jinbo, Z., Yibo, Y., et al. (2019). New paleomagnetic constraints for Platybelodon and Hipparion faunas in the Linxia Basin and their ecological environmental implications. Glob. Planet. Change 176, 71–83. doi: 10.1016/j.gloplacha.2019.03.002
Keywords: sandgrouse, late Miocene, aridification, Linxia Basin, Tibetan Plateau
Citation: Li Z, Stidham TA, Deng T and Zhou Z (2020) Evidence of Late Miocene Peri-Tibetan Aridification From the Oldest Asian Species of Sandgrouse (Aves: Pteroclidae). Front. Ecol. Evol. 8:59. doi: 10.3389/fevo.2020.00059
Received: 17 September 2019; Accepted: 26 February 2020;
Published: 31 March 2020.
Edited by:
Mary Teresa Silcox, University of Toronto Scarborough, CanadaReviewed by:
Gerald Mayr, Senckenberg Museum, GermanyCopyright © 2020 Li, Stidham, Deng and Zhou. This is an open-access article distributed under the terms of the Creative Commons Attribution License (CC BY). The use, distribution or reproduction in other forums is permitted, provided the original author(s) and the copyright owner(s) are credited and that the original publication in this journal is cited, in accordance with accepted academic practice. No use, distribution or reproduction is permitted which does not comply with these terms.
*Correspondence: Zhiheng Li, bGl6aGloZW5nQGl2cHAuYWMuY24=; Thomas A. Stidham, cHJlc2J5b3JuaXNAZ21haWwuY29t
Disclaimer: All claims expressed in this article are solely those of the authors and do not necessarily represent those of their affiliated organizations, or those of the publisher, the editors and the reviewers. Any product that may be evaluated in this article or claim that may be made by its manufacturer is not guaranteed or endorsed by the publisher.
Research integrity at Frontiers
Learn more about the work of our research integrity team to safeguard the quality of each article we publish.