For many animal species, cognitive traits are important for fitness. Such traits may be especially important in rapidly changing environments, where innovation and learning about new challenges could mean the difference between persistence and extinction (Dukas, 2013; Mery, 2013). One factor that may affect cognitive performance is hybridization. Hybridization occurs when individuals from distinct species mate and produce offspring. It is widespread, with estimates suggesting 1–10% of all animal species hybridize (Mallet, 2005; Schwenk et al., 2008), and is expected to become increasingly common as species distributions shift due to climate change (Chunco, 2014). Yet, the extent to which hybridization affects cognition, and any resulting impacts on hybrid fitness, remain relatively unknown. Recently, Rice and McQuillan (2018) described several mechanisms by which hybridization could directly affect cognitive abilities and negatively influence hybrid fitness. Here, I also consider that hybridization's impacts on cognition could lead to positive fitness consequences, and indirectly affect the expression of cognitive traits. I further discuss how the trade-off between investment in cognition and other functions could have important implications for the ultimate evolutionary outcome of hybridization. Currently, little is known about hybridization's effects on the expression of and selection on cognitive traits, and I argue that this is an important area for future research.
What We Know About Hybrid Cognition
The effects of hybridization on cognition have been poorly studied, particularly in cases of natural hybridization. A recent study tested the relative spatial memory and problem-solving abilities of black-capped and Carolina chickadees and their naturally-occurring hybrids (McQuillan et al., 2018). Chickadees are scatter hoarders, caching food throughout the environment during the fall. They rely on spatial memory to retrieve this food in the winter, and individuals with better spatial memory are more likely to survive (Sonnenberg et al., 2019). Although black-capped and Carolina chickadees performed equally well on an associative learning spatial task and a novel problem-solving task, hybrids were less able to remember the location of a food item or to solve the problem (McQuillan et al., 2018). Further testing is needed to determine whether other aspects of cognition are similarly affected. In contrast to the results in hybrid chickadees, mules—hybrids produced by crossing domestic horses and donkeys—exhibit enhanced visual discrimination and problem solving compared to their parental species (Proops et al., 2009; Osthaus et al., 2013). Although mules are not subject to natural selection, being domesticated, these findings demonstrate that hybridization can also lead to enhanced cognitive abilities compared to parental species.
Together, these examples illustrate the potential for hybridization to both positively and negatively affect cognition; however, much remains unknown. How frequently does hybridization influence cognition in other taxa? By what mechanism(s)? For a given hybrid cross, does hybridization have similar or variable effects on the diverse aspects of cognition? What are the net fitness consequences in wild populations across a range of environments? And how does selection on hybrid cognition contribute to the evolutionary outcomes of hybridization?
Direct Effects of Hybridization on Cognition
Cognition can be shaped by natural (e.g., Roth et al., 2012) and artificial selection (e.g., Mery and Kawecki, 2002), and individual variation in cognitive performance is repeatable (Cauchoix et al., 2018), leading to the conclusion that it has a heritable genetic basis (Croston et al., 2015). Therefore, hybridization should be expected to directly affect cognitive traits by the same mechanisms as it affects other traits.
Hybridization frequently leads to negative fitness consequences. Cognitive traits may be subject to genetic incompatibilities in hybrids (Dobzhansky, 1936; Muller, 1942; Figure 1), leading either to negative fitness consequences, or to asymmetric fitness consequences depending on the cross direction (Orr, 1995; Turelli and Moyle, 2007) or the sex of hybrid individuals (“Haldane's Rule,” Schilthuizen et al., 2011). Likewise, when two parental species with cognitive abilities under divergent selection produce hybrids of intermediate ability, ecological selection may act against the hybrids (Hatfield and Schluter, 1999; McBride and Singer, 2010; Figure 1) following a classical “ecological speciation” scenario (Rundle and Nosil, 2005). Rice and McQuillan (2018) further elaborate on the direct mechanisms by which hybridization could affect cognitive traits, leading to negative fitness consequences and postzygotic reproductive isolation between parental species.
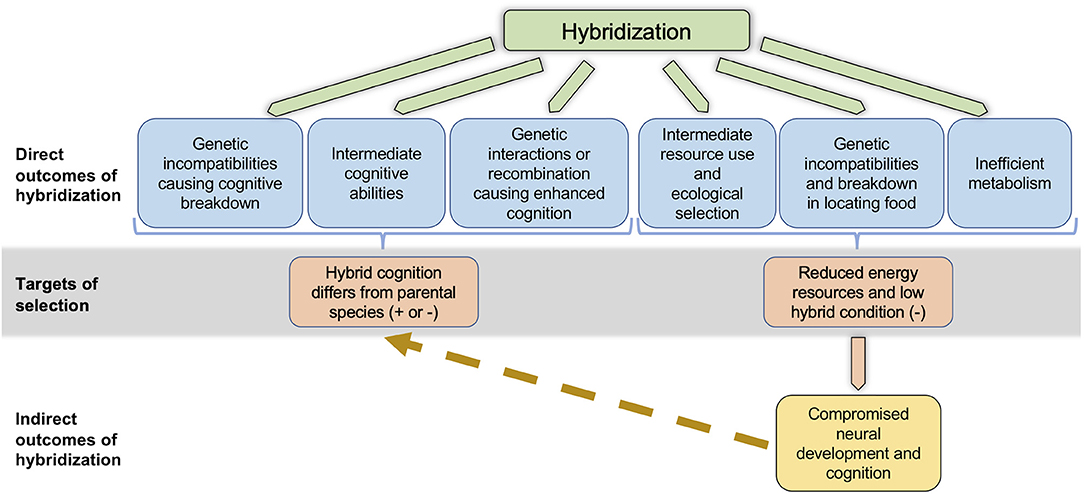
Figure 1. Non-mutually exclusive pathways by which hybridization can affect cognition. Hybridization can have direct effects on cognition through positive or negative genetic interactions, or through combinations of additive alleles. Reduced, intermediate, or enhanced cognition may result in hybrids, and the fitness consequences could be either positive or negative, depending on a variety of factors (see text). Alternatively, or even simultaneously, hybridization can indirectly affect cognition through its direct effects on resource use phenotypes, metabolic efficiency, or abilities to locate food. All of these direct outcomes are likely to lead to reduced energy and low condition in hybrids, likely causing selection against hybrids. Reduced energy and low condition can subsequently lead to compromised neural development and cognition in hybrids, which can in turn cause hybrid cognitive abilities to differ from those in the parental species.
Hybridization could also directly affect cognition in ways that lead to positive fitness consequences, and facilitate adaptation to novel environments. The mule example above shows that in some cases, cognitive abilities may be enhanced in hybrids relative to parental taxa (Proops et al., 2009; Osthaus et al., 2013). Enhanced cognitive abilities could result from positive genetic interactions between loci in the parental species (“heterosis”; Dagilis et al., 2019), or from the recombination of additive alleles in F1 and advanced generation hybrids (“transgressive segregation”; Rieseberg et al., 1999). Hybrids with enhanced abilities could experience high fitness (Figure 1), provided the fitness benefits of enhanced cognition in their specific environment outweigh any costs (Cole et al., 2012; Kotrschal et al., 2013). Such enhanced abilities, if caused by heterosis, may be transient, as it is less likely in advanced generation hybrids or back-crossed individuals (Barton, 2001). However, high fitness phenotypes in hybrids caused by transgressive segregation can persist across generations, as illustrated by the existence of stable hybrid species that are succeeding in novel or extreme environments, or are utilizing novel resources (e.g., Nolte et al., 2005; Gompert et al., 2006; Lamichhaney et al., 2018).
Indirect Effects of Hybridization on Cognition
Hybrids often have lower success than parental species individuals in obtaining resources, or have less efficient metabolism, which could have indirect negative effects on cognition (Figure 1). Hybrids produced by parental species that have diverged in resource use may be intermediate in their resource use phenotypes, and poor competitors in either parental environment (Hatfield and Schluter, 1999; Pfennig and Rice, 2007). Hybridization may also interfere with an individual's ability to locate food (Linn et al., 2004; Turissini et al., 2017). Such hybrids are likely to obtain fewer resources, and thereby experience reduced energy availability during development. Further, laboratory studies have demonstrated that mismatches between mitochondrial and nuclear genomes can affect metabolic function (Tieleman et al., 2009; Arnqvist et al., 2010; Hoekstra et al., 2013). Consistent with these findings, naturally-occurring hybrids from two different avian hybrid zones were found to be less efficient at energy metabolism (Olson et al., 2010; McFarlane et al., 2016). The fitness consequences of inefficient metabolism are likely to be environment dependent (Hoekstra et al., 2013), and could be further exacerbated if hybrids also have difficulties obtaining resources. Cognitive abilities and neural development are frequently condition dependent (reviewed in Buchanan et al., 2013). For example, birds experiencing restricted diets during development exhibited poorer spatial learning (Pravosudov et al., 2005) and auditory memory (Bell et al., 2018) as adults. However, poor nutrition does not necessarily affect all aspects of cognition similarly (Pravosudov et al., 2005). Given that hybridization can affect condition and energy availability through multiple mechanisms, indirect effects on some aspects of hybrid cognition are likely to be widespread (Figure 1).
Trade-Offs, the Environment, and Selection on Cognition
If hybrids differ from their parental species in cognitive performance, whether due to direct or indirect effects of hybridization or both (Figure 1), it will be important to estimate the magnitude and direction of selection on these traits. This will determine the impact of cognitive performance on the evolutionary outcomes of hybridization, which can include weakening or strengthening of species barriers, adaptive introgression, and even the creation of new hybrid species (Abbott et al., 2013).
Decreased cognitive abilities in hybrids could actually provide a fitness benefit in some environments, while enhanced cognitive abilities could result in lower fitness. This is because the energy demands of maintaining the brain tissue underlying cognitive abilities are expected to be high (Mink et al., 1981; Bordone et al., 2019), leading to a trade-off between investment in cognition and in other energetically costly functions and traits. For example, lines of Drosophila selected for enhanced learning ability experienced a decline in average lifespan compared to control lines (Burger et al., 2008). A consistent result was found in lines of guppies selected for large brains; the large-brained lines exhibited enhanced cognition (Kotrschal et al., 2013) but also shorter lifespan (Kotrschal et al., 2019). A trade-off between learning ability and competitive ability was uncovered in Drosophila as well (Mery and Kawecki, 2003), and families of cabbage white butterflies showing enhanced learning produced fewer eggs (Snell-Rood et al., 2011). Thus, the net strength and direction of selection on cognitive abilities will incorporate the costs to other functions important to fitness, and may differ among resource-poor and resource-rich environments. If hybridization occurs across a range of such environments and affects cognition, hybrids in certain environments may be strongly selected against, leading to a strengthening of species boundaries and potentially narrow hybrid zones, while the opposite could be true in other environments.
It is worth noting for at least three reasons, however, that hybrids may be particularly likely to find themselves in environments favoring enhanced cognitive abilities, even in the face of trade-offs. First, species range overlap is often associated with environmental gradients and can shift with the climate (Chunco, 2014; Taylor et al., 2015), such that the opportunity for hybridization may be especially high in variable or novel environments. Second, hybridization may facilitate the colonization of novel environments, either through the adaptive introgression of genes underlying functionally important traits, or as a result of transgressive segregation (Pfennig et al., 2016). Third, areas of sympatry, such as hybrid zones, are likely to be complex social environments, as animals must navigate and process both intra- and interspecific interactions and signals (Pfennig and Pfennig, 2012). Variable, novel, and socially complex environments are expected to favor enhanced learning, memory, and problem-solving (Sol et al., 2002; Dukas, 2013; Mery, 2013; Ashton et al., 2018), so that hybrids with such abilities could experience high fitness.
Selection on hybrid cognition is likely to be complex, and much additional research is required to assess how cognition contributes to the net fitness consequences of hybridization. Because hybrids may have reduced energy reserves compared to parental species individuals (see above), one interesting implication of the trade-off between investment in cognition and other functions is that the direction and magnitude of selection on cognition could vary between hybrids and parental species, even in similar environments. The optimal level of cognitive ability for a parental individual in a given environment might be higher than the optimal level for a hybrid—with reduced energy reserves—in the same environment. Expected patterns of selection are further complicated by the fact that individuals can vary in their performance across different aspects of cognition (DuBois et al., 2018; van Horik et al., 2018), and therefore hybridization may have variable effects on different aspects of cognition depending on which individuals are hybridizing.
Conclusion
Hybridization is likely to have important direct and indirect impacts on cognitive ability. Resulting cognitive performance in hybrids may lead to positive or negative fitness consequences. Further, the trade-off between investment in cognition and other important functions coupled with the potential for individual variation in performance across multiple aspects of cognition will complicate patterns of selection on hybrid cognition. The net strength and direction of this selection will determine how cognitive performance contributes to the ultimate evolutionary outcomes of hybridization. Currently, very little is known about hybridization's impact on cognition, and there is large scope for additional research. Important questions include: How frequently does hybrid cognition differ from parental species, and in what systems? Is it more common for hybridization to affect cognition through direct or indirect mechanisms, or both? What is the direction and magnitude of selection on hybrid cognition, and how does it vary across environments? Many study systems, across a broad range of taxonomic diversity, are well-suited for addressing these questions, particularly those with existing knowledge of traits affected by hybridization. Such research is needed if we are to evaluate the role of cognitive performance in the maintenance of species boundaries, or the links between hybridization and the expression of and selection on cognitive traits in the wild.
Author Contributions
AR wrote the manuscript.
Funding
AR was supported by National Science Foundation IOS-1754909, and Lehigh University.
Conflict of Interest
The author declares that the research was conducted in the absence of any commercial or financial relationships that could be construed as a potential conflict of interest.
Acknowledgments
Thank you to the organizers of the Research Topic on Links Between Cognition and Fitness: Mechanisms and Constraints in the Wild, and to three reviewers for helpful suggestions on the first version of this manuscript.
References
Abbott, R., Albach, D., Ansell, S., Arntzen, J. W., Baird, S. J. E., Bierne, N., et al. (2013). Hybridization and speciation. J. Evol. Biol. 26, 229–246. doi: 10.1111/j.1420-9101.2012.02599.x
Arnqvist, G., Dowling, D. K., Eady, P., Gay, L., Tregenza, T., Tuda, M., et al. (2010). Genetic architecture of metabolic rate: environment specific epistasis between mitochondrial and nuclear genes in an insect. Evolution 64, 3354–3363. doi: 10.1111/j.1558-5646.2010.01135.x
Ashton, B. J., Ridley, A. R., Edwards, E. K., and Thornton, A. (2018). Cognitive performance is linked to group size and affects fitness in Australian magpies. Nature 554, 364–367. doi: 10.1038/nature25503
Barton, N. H. (2001). The role of hybridization in evolution. Mol. Ecol. 10, 551–568. doi: 10.1046/j.1365-294x.2001.01216.x
Bell, B. A., Phan, M. L., Meillère, A., Evans, J. K., Leitner, S., Vicario, D. S., et al. (2018). Influence of early-life nutritional stress on songbird memory formation. Proc. R. Soc. B Biol. Sci. 285:20181270. doi: 10.1098/rspb.2018.1270
Bordone, M. P., Salman, M. M., Titus, H. E., Amini, E., Andersen, J. V., Chakraborti, B., et al. (2019). The energetic brain – A review from students to students. J. Neurochem. 151, 139–165. doi: 10.1111/jnc.14829
Buchanan, K. L., Grindstaff, J. L., and Pravosudov, V. V. (2013). Condition dependence, developmental plasticity, and cognition: implications for ecology and evolution. Trends Ecol. Evol. 28, 290–296. doi: 10.1016/j.tree.2013.02.004
Burger, J. M., Kolss, M., Pont, J., and Kawecki, T. J. (2008). Learning ability and longevity: a symmetrical evolutionary trade-off in Drosophila. Evolution 62, 1294–1304. doi: 10.1111/j.1558-5646.2008.00376.x
Cauchoix, M., Chow, P. K. Y., van Horik, J. O., Atance, C. M., Barbeau, E. J., Barragan-Jason, G., et al. (2018). The repeatability of cognitive performance: a meta-analysis. Philos. Trans. R. Soc. B Biol. Sci. 373:20170281. doi: 10.1098/rstb.2017.0281
Chunco, A. J. (2014). Hybridization in a warmer world. Ecol. Evol. 4, 2019–2031. doi: 10.1002/ece3.1052
Cole, E. F., Morand-Ferron, J., Hinks, A. E., and Quinn, J. L. (2012). Cognitive ability influences reproductive life history variation in the wild. Curr. Biol. 22, 1808–1812. doi: 10.1016/j.cub.2012.07.051
Croston, R., Branch, C. L., Kozlovsky, D. Y., Dukas, R., and Pravosudov, V. V (2015). Heritability and the evolution of cognitive traits. Behav. Ecol. 26, 1447–1459. doi: 10.1093/beheco/arv088
Dagilis, A. J., Kirkpatrick, M., and Bolnick, D. I. (2019). The evolution of hybrid fitness during speciation. PLoS Genet. 15:e1008125. doi: 10.1371/journal.pgen.1008125
Dobzhansky, T. (1936). Studies on hybrid sterility. II. Localization of sterility factors in Drosophila pseudoobscura hybrids. Genetics 21, 113–135.
DuBois, A. L., Nowicki, S., Peters, S., Rivera-Cáceres, K. D., and Searcy, W. A. (2018). Song is not a reliable signal of general cognitive ability in a songbird. Anim. Behav. 137, 205–213. doi: 10.1016/j.anbehav.2018.01.020
Dukas, R. (2013). Effects of learning on evolution: robustness, innovation and speciation. Anim. Behav. 85, 1023–1030. doi: 10.1016/j.anbehav.2012.12.030
Gompert, Z., Fordyce, J. A., Forister, M. L., Shapiro, A. M., and Nice, C. C. (2006). Homoploid hybrid speciation in an extreme habitat. Science 314, 1923–1925. doi: 10.1126/science.1135875
Hatfield, T., and Schluter, D. (1999). Ecological speciation in sticklebacks: environment-dependent hybrid fitness. Evolution 53, 866–873. doi: 10.1111/j.1558-5646.1999.tb05380.x
Hoekstra, L. A., Siddiq, M. A., and Montooth, K. L. (2013). Pleiotropic effects of a mitochondrial–nuclear incompatibility depend upon the accelerating effect of temperature in Drosophila. Genetics 195, 1129–1139. doi: 10.1534/genetics.113.154914
Kotrschal, A., Corral-Lopez, A., and Kolm, N. (2019). Large brains, short life: selection on brain size impacts intrinsic lifespan. Biol. Lett. 15:20190137. doi: 10.1098/rsbl.2019.0137
Kotrschal, A., Rogell, B., Bundsen, A., Svensson, B., Zajitschek, S., Brännström, I., et al. (2013). Artificial selection on relative brain size in the guppy reveals costs and benefits of evolving a larger brain. Curr. Biol. 23, 168–171. doi: 10.1016/j.cub.2012.11.058
Lamichhaney, S., Han, F., Webster, M. T., Andersson, L., Grant, B. R., and Grant, P. R. (2018). Rapid hybrid speciation in Darwin's finches. Science 359, 224–228. doi: 10.1126/science.aao4593
Linn, C. E., Dambroski, H. R., Feder, J. L., Berlocher, S. H., Nojima, S., and Roelofs, W. L. (2004). Postzygotic isolating factor in sympatric speciation in Rhagoletis flies: reduced response of hybrids to parental host-fruit odors. Proc. Natl. Acad. Sci. U.S.A. 101, 17753–17758. doi: 10.1073/pnas.0408255101
Mallet, J. (2005). Hybridization as an invasion of the genome. Trends Ecol. Evol. 20, 229–237. doi: 10.1016/j.tree.2005.02.010
McBride, C. S., and Singer, M. C. (2010). Field studies reveal strong postmating isolation between ecologically divergent butterfly populations. PLoS Biol. 8:e1000529. doi: 10.1371/journal.pbio.1000529
McFarlane, S. E., Sirkiä, P. M., Ålund, M., and Qvarnström, A. (2016). Hybrid dysfunction expressed as elevated metabolic rate in male Ficedula flycatchers. PLoS ONE 11:e0161547. doi: 10.1371/journal.pone.0161547
McQuillan, M. A., Roth, T. C., Huynh, A. V., and Rice, A. M. (2018). Hybrid chickadees are deficient in learning and memory. Evolution 72, 1155–1164. doi: 10.1111/evo.13470
Mery, F. (2013). Natural variation in learning and memory. Curr. Opin. Neurobiol. 23, 52–56. doi: 10.1016/j.conb.2012.09.001
Mery, F., and Kawecki, T. J. (2002). Experimental evolution of learning ability in fruit flies. Proc. Natl. Acad. Sci. U.S.A. 99, 14274–14279. doi: 10.1073/pnas.222371199
Mery, F., and Kawecki, T. J. (2003). A fitness cost of learning ability in Drosophila melanogaster. Proc. R. Soc. B Biol. Sci. 270, 2465–2469. doi: 10.1098/rspb.2003.2548
Mink, J. W., Blumenschine, R. J., and Adams, D. B. (1981). Ratio of central nervous system to body metabolism in vertebrates: its constancy and functional basis. Am. J. Physiol. Integr. Comp. Physiol. 241, R203–R212. doi: 10.1152/ajpregu.1981.241.3.R203
Muller, H. J. (1942). “Isolating mechanisms, evolution and temperature,” in Biological Symposia, ed. T. Dobzhansky (Lancaster, PA: The Jaques Cattell Press), 71–125.
Nolte, A. W., Freyhof, J., Stemshorn, K. C., and Tautz, D. (2005). An invasive lineage of sculpins, Cottus sp. (Pisces, Teleostei) in the Rhine with new habitat adaptations has originated from hybridization between old phylogeographic groups. Proc. R. Soc. B Biol. Sci. 272, 2379–2387. doi: 10.1098/rspb.2005.3231
Olson, J. R., Cooper, S. J., Swanson, D. L., Braun, M. J., and Williams, J. B. (2010). The relationship of metabolic performance and distribution in black-capped and Carolina chickadees. Physiol. Biochem. Zool. 83, 263–275. doi: 10.1086/648395
Orr, H. A. (1995). The population genetics of speciation: the evolution of hybrid incompatibilities. Genetics 139, 1805–1813.
Osthaus, B., Proops, L., Hocking, I., and Burden, F. (2013). Spatial cognition and perseveration by horses, donkeys and mules in a simple A-not-B detour task. Anim. Cogn. 16, 301–305. doi: 10.1007/s10071-012-0589-4
Pfennig, D. W., and Pfennig, K. S. (2012). Evolution's Wedge. Berkeley, CA: University of California Press. doi: 10.1525/california/9780520274181.001.0001
Pfennig, D. W., and Rice, A. M. (2007). An experimental test of character displacement's role in promoting postmating isolation between conspecific populations in contrasting competitive environments. Evolution 61, 2433–2443. doi: 10.1111/j.1558-5646.2007.00190.x
Pfennig, K. S., Kelly, A. L., and Pierce, A. A. (2016). Hybridization as a facilitator of species range expansion. Proc. R. Soc. B Biol. Sci. 283:20161329. doi: 10.1098/rspb.2016.1329
Pravosudov, V. V., Lavenex, P., and Omanska, A. (2005). Nutritional deficits during early development affect hippocampal structure and spatial memory later in life. Behav. Neurosci. 119, 1368–1374. doi: 10.1037/0735-7044.119.5.1368
Proops, L., Burden, F., and Osthaus, B. (2009). Mule cognition: a case of hybrid vigour? Anim. Cogn. 12, 75–84. doi: 10.1007/s10071-008-0172-1
Rice, A. M., and McQuillan, M. A. (2018). Maladaptive learning and memory in hybrids as a reproductive isolating barrier. Proc. R. Soc. B Biol. Sci. 285:20180542. doi: 10.1098/rspb.2018.0542
Rieseberg, L. H., Archer, M. A., and Wayne, R. K. (1999). Transgressive segregation, adaptation and speciation. Heredity 83, 363–372. doi: 10.1038/sj.hdy.6886170
Roth, T. C., LaDage, L. D., Freas, C. A., and Pravosudov, V. V. (2012). Variation in memory and the hippocampus across populations from different climates: a common garden approach. Proc. R. Soc. B Biol. Sci. 279, 402–410. doi: 10.1098/rspb.2011.1020
Rundle, H. D., and Nosil, P. (2005). Ecological speciation. Ecol. Lett. 8, 336–352. doi: 10.1111/j.1461-0248.2004.00715.x
Schilthuizen, M., Giesbers, M. C., and Beukeboom, L. W. (2011). Haldane's rule in the 21st century. Heredity 107, 95–102. doi: 10.1038/hdy.2010.170
Schwenk, K., Brede, N., and Streit, B. (2008). Introduction. Extent, processes and evolutionary impact of interspecific hybridization in animals. Philos. Trans. R. Soc. B Biol. Sci. 363, 2805–2811. doi: 10.1098/rstb.2008.0055
Snell-Rood, E. C., Davidowitz, G., and Papaj, D. R. (2011). Reproductive tradeoffs of learning in a butterfly. Behav. Ecol. 22, 291–302. doi: 10.1093/beheco/arq169
Sol, D., Timmermans, S., and Lefebvre, L. (2002). Behavioural flexibility and invasion success in birds. Anim. Behav. 63, 495–502. doi: 10.1006/anbe.2001.1953
Sonnenberg, B. R., Branch, C. L., Pitera, A. M., Bridge, E., and Pravosudov, V. V. (2019). Natural selection and spatial cognition in wild food-caching mountain chickadees. Curr. Biol. 29, 670–676.e3. doi: 10.1016/j.cub.2019.01.006
Taylor, S. A., Larson, E. L., and Harrison, R. G. (2015). Hybrid zones: windows on climate change. Trends Ecol. Evol. 30, 398–406. doi: 10.1016/j.tree.2015.04.010
Tieleman, B. I., Versteegh, M. A., Fries, A., Helm, B., Dingemanse, N. J., Gibbs, H. L., et al. (2009). Genetic modulation of energy metabolism in birds through mitochondrial function. Proc. R. Soc. B Biol. Sci. 276, 1685–1693. doi: 10.1098/rspb.2008.1946
Turelli, M., and Moyle, L. C. (2007). Asymmetric postmating isolation: Darwin's corollary to Haldane's Rule. Genetics 176, 1059–1088. doi: 10.1534/genetics.106.065979
Turissini, D. A., Comeault, A. A., Liu, G., Lee, Y. C., and Matute, D. R. (2017). The ability of Drosophila hybrids to locate food declines with parental divergence. Evolution 71, 960–973. doi: 10.1111/evo.13180
Keywords: hybridization, cognition, selection, trade-off, environmentally dependent selection
Citation: Rice AM (2020) The Overlooked Influence of Hybridization on Cognition. Front. Ecol. Evol. 8:39. doi: 10.3389/fevo.2020.00039
Received: 15 October 2019; Accepted: 10 February 2020;
Published: 25 February 2020.
Edited by:
Blandine Françoise Doligez, Centre National de la Recherche Scientifique (CNRS), FranceReviewed by:
Norman A. Johnson, University of Massachusetts Amherst, United StatesThore Bergman, University of Michigan, United States
Carrie Branch, Cornell University, United States
Copyright © 2020 Rice. This is an open-access article distributed under the terms of the Creative Commons Attribution License (CC BY). The use, distribution or reproduction in other forums is permitted, provided the original author(s) and the copyright owner(s) are credited and that the original publication in this journal is cited, in accordance with accepted academic practice. No use, distribution or reproduction is permitted which does not comply with these terms.
*Correspondence: Amber M. Rice, amr511@lehigh.edu
†ORCID: Amber M. Rice orcid.org/0000-0002-5475-8226