- 1Department of Ecology, Ecosystem Science and Plant Ecology, Technische Universität Berlin, Berlin, Germany
- 2Berlin-Brandenburg Institute of Advanced Biodiversity Research, Berlin, Germany
Understanding the contribution of cities to nature conservation is gaining increasing importance with a globally accelerating urbanization and requires insights into the mechanisms that underlie urban distribution patterns. While a considerable number of endangered plant species have been reported for cities, the spatial dependence of populations of these species on natural remnants versus anthropogenic ecosystems is critically understudied due to deficiencies in population distribution data. To which extent endangered species in anthropogenic ecosystems spatially rely on natural remnants is thus an open question. We used a unique dataset of 1,742 precisely mapped populations of 213 endangered plant species in the city of Berlin and related these point data to habitat patches that had been assigned to natural remnants, hybrid ecosystems and novel ecosystems according to the novel ecosystem approach. By applying point pattern analyses (Ripley’s K function, cross K function, cross pair correlation function) we unraveled the spatial dependence of the populations toward the different ecosystem types. Moreover, we tested how plant traits related to plant strategy and dispersal filter for species occurrence across ecosystems. Differentiating populations on anthropogenic sites revealed that populations in hybrid ecosystems spatially depended on natural remnants, but populations in novel ecosystems (i.e. more than a third of all populations) surprisingly didn’t. A conditional inference tree showed that endangered plant species in novel ecosystems are filtered for ruderal strategy type and wind dispersal syndrome, while competitive and stress-tolerant species were mainly confined to natural remnants. Our results highlight the importance of conserving natural remnants as habitats and seed sources of endangered plants. Yet novel urban ecosystems can support many populations of endangered plant species beyond the adjacency to natural remnants, with hybrid ecosystems likely acting as stepping stones. This indicates a specific contribution of urban ecosystems to biodiversity conservation. Since different filters modulate the species pools of different ecosystem types, novel urban ecosystems are not supposed to substitute fully the habitat functions of natural remnants. Our study thus highlights promising opportunities for involving the total range of urban ecosystem types into urban conservation approaches.
Introduction
Cities can harbor a high biological richness (Kühn et al., 2004; Aronson et al., 2014), also including a considerable richness of endangered plant species (Schwartz et al., 2002; Lawson et al., 2008; Lenzen et al., 2009; Shwartz et al., 2014; Ives et al., 2016; Kowarik and von der Lippe, 2018; Planchuelo et al., 2019). Urban areas in Australia, for example, host 30% of the country’s endangered plant species (Ives et al., 2016), with some of them occurring today exclusively in urban environments (Soanes and Lentini, 2019). While these studies indicate a high potential of cities to contribute to biodiversity conservation at a global scale (McKinney, 2008; Kowarik, 2011), survival in urban environments is a challenge for many species as demonstrated by establishment failures (Kowarik and von der Lippe, 2018), extinction debts (Hahs and McDonnell, 2014) and completed extinctions (Knapp et al., 2010; Duncan et al., 2011). Developing effective nature conservation strategies for cities thus requires insights into the mechanisms that underlie urban biodiversity patterns in general, and those of endangered species in particular.
Plants are not randomly distributed in cities, and biodiversity patterns were early identified in relation to urban structure, the level of human pressure, urbanization, or specific land use (Kowarik, 1990; McKinney, 2002, 2008; Shochat et al., 2006; Swan et al., 2011; Schmidt et al., 2014), thus resulting from an interaction of ecological and socio-economic parameters (Swan et al., 2011). Some major filters have been hypothesized to predict the composition of urban species assemblages by filtering for species with a specific suite of biological traits that support the colonization and persistence of species in cities (Williams et al., 2009; Aronson et al., 2016; Kowarik and von der Lippe, 2018). Yet, despite increasing insights into the mechanisms of urban species assembly (Knapp et al., 2009; Beninde et al., 2015; Williams et al., 2015; Aronson et al., 2016), the responses of endangered plant species to urban filters are critically understudied (Shwartz et al., 2014). This is an essential gap of knowledge because responses of large species sets to urbanization cannot be simply translated to endangered species, as indicated by contrasting species’ traits that have gone extinct or, alternatively, persist in cities (Knapp et al., 2010; Duncan et al., 2011; Williams et al., 2015).
Cities were often founded in landscapes of high geomorphological and biological richness (Kühn et al., 2004), leading to the inclusion of natural remnants into the urban matrix (e.g. Godefroid and Koedam, 2003; Rebelo et al., 2011; Schwartz et al., 2013). The importance of such remnants for endangered species is well established. Yet, an important part of the specific urban contribution to biodiversity conservation – beyond conserving natural remnants – depends on the role that anthropogenic urban ecosystems can play as habitats of endangered species. Such ecosystems are omnipresent in cities, resulting from a stepwise transformation of natural to novel urban ecosystems, and being modulated by different land-use types and associated legacies (Kowarik, 2011; Ramalho et al., 2014). Endangered species are filtered by their ability to colonize these usually highly fragmented anthropogenic sites through dispersal, and to negotiate with novel ecological features of urban sites.
Few recent studies have reported a considerable amount of endangered plant species also from habitats of specific urban origin (Kowarik and von der Lippe, 2018; Planchuelo et al., 2019; Soanes and Lentini, 2019), including two studies from Berlin, Germany. The first revealed that a similar share of endangered plant species was able to establish populations in natural remnants and anthropogenic ecosystems (Kowarik and von der Lippe, 2018). The second study showed that populations of a large set of plant species of highest conservation priority stretch over a broad range of natural and anthropogenic ecosystems; surprisingly with highest numbers of populations in novel ecosystems, but highest density of populations in natural remnants (Planchuelo et al., 2019).
These studies highlight conservation opportunities for novel anthropogenic ecosystems. Yet the role of the latter as habitats of endangered species might be compromised by a spatial dependence to remnant ecosystems. Species typical of ancient forests, for example, are critically dispersal-limited (Jacquemyn et al., 2001). Urban filters that determine the species’ assemblages of fragmented habitat patches (Williams et al., 2009; Aronson et al., 2016) can thus be expected to determine as well the colonization success of endangered species in novel urban habitats, with natural remnants as source habitats. Thus, one critical question for understanding the urban contribution to biodiversity conservation is to which extent endangered species are spatially confined to natural remnants and their vicinity (source-sink dynamic; Pulliam, 1988; Schreiber, 2010).
As dispersal is an ecological process that causes spatial autocorrelation in distribution patterns (Epperson, 2005; Bahn et al., 2008), spatial statistics can be used to assess whether or not populations located in anthropogenic urban ecosystems are still spatially dependent to populations in natural remnants. Point pattern analysis has already been widely applied in non-urban areas to assess the dependence of endangered plant species on specific habitat patches of other species (Wehenkel et al., 2015). Further examples involve work on the effects of animal-mediated dispersal of an endangered shrub (Rodríguez-Pérez et al., 2012), or on the minimum areas for the conservation of an endangered plant (Lim et al., 2008). Even if the importance of spatial patterning for biodiversity conservation in cities is increasingly acknowledged (Rastandeh et al., 2017), insights into related spatial patterns and processes are scarce because exact spatial information on the locations of endangered species in urban landscapes is often missing.
Many of the plants that pass dispersal filters in cities tend to be ruderal species in terms of the CSR scheme (Grime, 1977), as they can rapidly colonize new urban habitats while tolerating a wide range of urban disturbances (Williams et al., 2015). Other species might be pre-adapted or adapting to urban environments, e.g. in terms of advantageous dispersal traits (McDonnell and Hahs, 2015). For instance, some seed traits related to dispersal increase the probability of human-mediated long-distance dispersal in cities (von der Lippe and Kowarik, 2008). Correspondingly, species with human vectors as an additional dispersal pathway increased in abundance over time in densely urbanized neighborhoods (Johnson et al., 2017) and were less affected by urban dispersal limitations (Chytrý et al., 2012).
Here, we tested for the spatial dependence between populations of endangered plant species in anthropogenic habitats and natural remnants in the urban landscape of a European metropolis. We used a unique dataset with the exact geographical position of 1,742 populations of 213 endangered plant species in Berlin. These species had been identified as species of highest conservation priority due to their endangerment in Berlin or at larger spatial scales, and their populations had been subsequently precisely mapped by experts (Meißner and Seitz, 2010). While we have previously analyzed (Planchuelo et al., 2019) the affiliation of these populations to different types of biotopes and three types of ecosystems according to the novel ecosystem approach (i.e. natural remnants, hybrid ecosystems, novel ecosystems; Hobbs et al., 2009), we here focus on the spatial patterns among populations and the related biological features of the endangered species.
We hypothesized that populations of endangered plant species are not randomly distributed but spatially clustered in Berlin. We expected that populations in novel ecosystems occur independently from source populations in natural remnants since novel ecosystems filter for species with enhanced capacities of long-distance dispersal and for those that belong to a ruderal strategy type (Grime, 1977). Since hybrid ecosystems result from a less profound transformation of natural ecosystems compared to novel ecosystems, we assume that populations located in hybrid ecosystems still spatially relate to seed sources in natural remnants, with species pools filtered by different features compared to species that were able to colonize novel urban ecosystems.
In detail, we addressed the following research questions:
(1) Are populations (n = 1,742) of endangered plant species (n = 213) located within natural remnants, hybrid ecosystems and novel ecosystems in Berlin spatially clustered?
(2) Do populations located in the two types of anthropogenic ecosystems (i.e. hybrid ecosystems and novel ecosystems) spatially depend on natural remnants? If populations in novel ecosystems are not dependent on natural remnants, are they instead dependent on hybrid ecosystems?
(3) Are endangered plant species with populations in natural remnants, hybrid ecosystems and novel ecosystems filtered by (a) dispersal-related species traits and (b) different strategy types according to the CSR scheme (Grime, 1977)?
Materials and Methods
Study Area and Population Data
This study was carried out in the city of Berlin, the capital and most populous city of Germany with 3.6 million inhabitants as of 2017. Berlin has a total area of 891km2, from which 59% is covered by built-up areas and streets, while green and blue spaces occupy 41% of the area – including forests (17.7%), lakes and rivers (6.1%), parks (5.6%), allotment gardens (5.3%), fields (5%), and grasslands (1.3%) (SenStadtUm, 2016).
Since the 19th century, the flora of Berlin has been thoroughly studied (Sukopp, 1987). Information on populations dynamics has been collected and updated numerous times in Red Lists of endangered species (Seitz et al., 2018). Until today, 17% of Berlin’s flora has gone extinct since the mid-19th century and 29% is currently being endangered. In this study, we used a detailed dataset with the precise geographical location of 1,742 populations of 213 highly endangered plant species (Figure 1 and Supplementary Appendix S1) from Berlin’s Flora Protection Program (Berliner Florenschutzkonzept). These species have been selected for the Flora Protection Program according to their: (1) red list status in Berlin and/or at higher spatial scales, (2) long term population trends (50–150 years), (3) short term population trends (1–15 years), and (4) biogeographical responsibility, i.e. when the range of a species in Berlin constitutes an important part of the global range of this species (Seitz, 2007). The populations of these species are being monitored since 2009 by several experts (Meißner and Seitz, 2010).
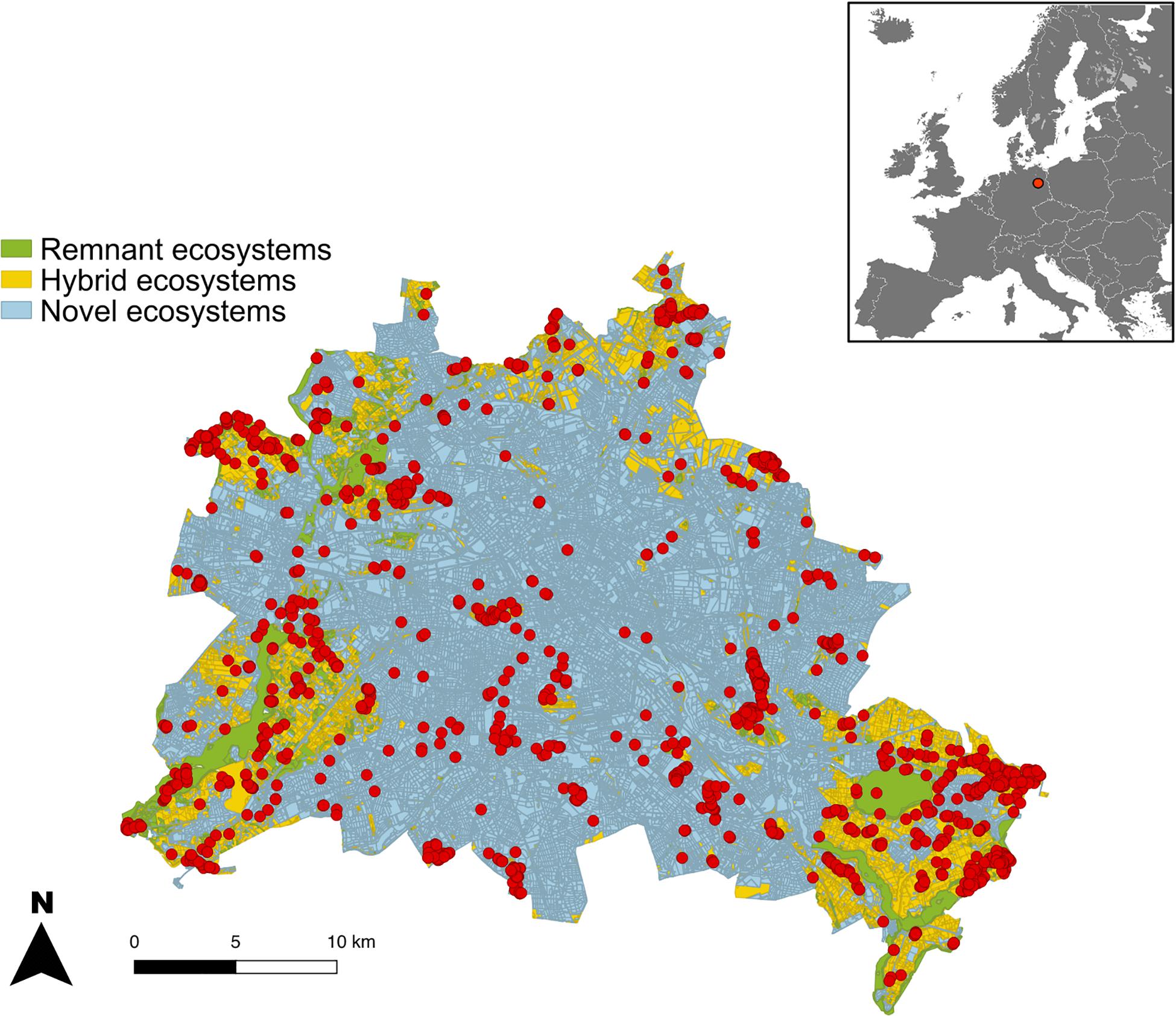
Figure 1. Location of populations of endangered plant species (213 species, 1742 populations, red dots) across remnant, hybrid and novel ecosystems inside the city limits of Berlin. The configuration of the urban matrix is overlaid in darker tones.
Biotope Types and Ecological Novelty
A methodological approach was developed in the 1980s to assign different biotope types to the whole area of Berlin by incorporating various combinations of land uses and environmental conditions (Sukopp and Weiler, 1988). The mapping was done as part of a combined work of 64 different projects between 2001 and 2014, where areas with high conservation value or low detectability in aerial photos such as forests were covered by field biotope mapping while built up areas and transportation corridors were primarily covered by data of the urban planning authorities (SenStadtUm, 2014). Today, the Berlin Environmental Atlas includes a very detailed area wide biotope mapping1 that is updated regularly (SenStadtUm, 2014). Currently, the city of Berlin is divided into 79,268 patches, with each of these patches being assigned to one of 12 biotope classes. These are forests, grasslands, ruderal sites, standing bodies of waters, built-up areas, bogs and marshes, hedges, green spaces, fields, flowing moving bodies of waters, heaths, and other types. These biotope classes are in turn further subdivided in 7,483 biotope types at several hierarchical levels.
To relate the geographical position of each of the 1,742 populations of endangered plant species to remnant, hybrid and novel ecosystems and assess their spatial correlation toward natural remnants, we used the ecological novelty map of Berlin (Planchuelo et al., 2019). Each of the 79,268 patches that had been discerned in the biotope map were assigned to either natural remnants, hybrid or novel ecosystems – see Planchuelo et al. (2019) for details:
• Natural remnant ecosystems are relicts of natural ecosystems that are often slightly affected by urban impacts but still remain within their historical range of modifications. These include biotopes from mires and wetlands to near-natural forests or moderately used dry or wet grasslands (Figure 1).
• Hybrid ecosystems are human mediated ecosystems that have been modified from their historical counterparts but still have the potential to return to historical conditions. Examples range from managed grasslands to urban green spaces, young tree plantations in forests and parks or intermediate succession stages following abandonment.
• Novel ecosystems are human shaped ecosystems that have potentially been irreversibly changed by large modifications to their abiotic conditions or biotic composition. They include built up areas, rooftops, vacant lots, abandoned industrial areas or intensively managed agricultural fields.
Spatial Analyses
To enable spatial point pattern analyses to assess the correlation between populations of endangered plants and patches of different novelty categories, the latter were represented by a point positioned at the centroid of each patch (Baddeley, 2008). Using the “polygon centroid” function in QGIS, we converted all 79,268 ecosystem patches that had been differentiated in Berlin’s biotope map into point data.
To respond to the first research question, we first tested whether populations within natural remnants, hybrid ecosystems and novel ecosystems were spatially clustered. This was done by means of Ripley’s L function (Dixon, 2002), which allows to verify if, and at which spatial scales, populations are clustered, regularly or randomly distributed. This test (function Linhom of the spatstat package in R) compares the spatial distribution of a point pattern against a generated set of points with a random distribution (which is named Poisson distribution, see Stoyan and Stoyan, 1994). If the curve of the L function of a point pattern lies above the Poisson line, the populations are clustered; if it lies below, the populations are regularly distributed; if it overlaps it, the populations are randomly distributed (Dixon, 2002). L plots of Ripley’s K functions were used (Dixon, 2002), as they stabilize the variance and are more powerful than simple plots of the K function (Baddeley et al., 2015).
To respond to the second research question, we analyzed if populations in the two types of anthropogenic ecosystems (i.e. hybrid ecosystems with 632 populations and novel ecosystems with 687 populations) are spatially dependent on remnant ecosystem patches. Because one of our hypotheses was that populations in novel ecosystems are not related to natural remnants, we also tested if populations in novel ecosystems might instead be spatially dependent on hybrid ecosystem patches. Statistically significant spatial correlations between populations of endangered plant species and the patches of different novelty categories were assessed with global envelopes of cross L functions (function Lcross.inhom of the spatstat package in R). This spatial analysis compares the observed correlation between the two point patterns of the empirical data against a null model, which is generated by keeping the same location of the ecosystem patches while creating simulations with random locations for the populations (Baddeley et al., 2015). If the curve of the cross L function for the empirical point pattern transgresses outside of the global envelope of the null model at any moment, there is a statistically significant spatial correlation between the populations and the novelty category (Baddeley et al., 2014, 2015). Alternatively, if the curve does not transgress outside of the global envelope, we consider that there is a missing spatial correlation between the populations and the novelty category. Global envelopes were chosen because they avoid the problem of data snooping of pointwise envelopes and they allow to achieve an exact significance level: α = 1/(1 + n°simulations), see Baddeley et al., 2014. Thus, in order to achieve a 95% confidence interval, we generated global envelopes from 19 simulations of the null model. L plots of cross K functions were used (Dixon, 2002), as they stabilize the variance and are more powerful than the K plots (Baddeley et al., 2015).
In many cases, spatial correlations can be simply fruit of the spatial configuration of the urban matrix. Populations in hybrid ecosystems, for example, might be spatially dependent on remnant ecosystems simply because patches of hybrid ecosystems tend to be located in the proximity of patches of remnant ecosystems. We thus assessed with cross L functions if the patches of the different novelty categories were spatially correlated or not (see Supplementary Appendix S2). This was done only for patches where positive spatial relationships with populations of endangered plant species were previously shown.
The cross L function can reveal a spatial correlation between populations and novelty categories, but not if this correlation is positive or negative. We thus used a cross pair correlation function in a successive step (Penttinen et al., 1992; Baddeley, 2008; function pcfcross.inhom of the spatstat package in R) to assess the type of correlation at any given distance between the populations and the different novelty categories. There is a spatial dependence between the populations and a novelty category when the values of the cross pair correlation function lay over 1, with values below 1 indicating spatial repulsion (Penttinen et al., 1992; Baddeley et al., 2015). The higher or lower the values, the stronger the dependence or the repulsion.
All spatial statistics were performed in the R package spatstat (Baddeley et al., 2015). Ripley’s isotropic edge corrections (Ripley, 1991) were applied to all calculations. A prior Monte Carlo test for spatial homogeneity proved our point data to be inhomogeneous (the number of points per area is not constant throughout the whole space), so we used statistical functions in “spatstat” that were adapted for inhomogeneous data.
Dispersal Traits and CSR Strategy
To respond to the third research question on the importance of plant dispersal traits and CSR strategy types in explaining the spatial distribution of populations of endangered plant species across the different types of ecosystems, we first gathered information on plant dispersal traits and CSR strategy (Grime, 1977) from the BIOFLOR database (Klotz et al., 2002; Table 1). We used this database because it focuses on the German flora and it achieves a high level of detail by ranking the various dispersal syndromes of each species, allowing us to select the most important one in relation to our study area.
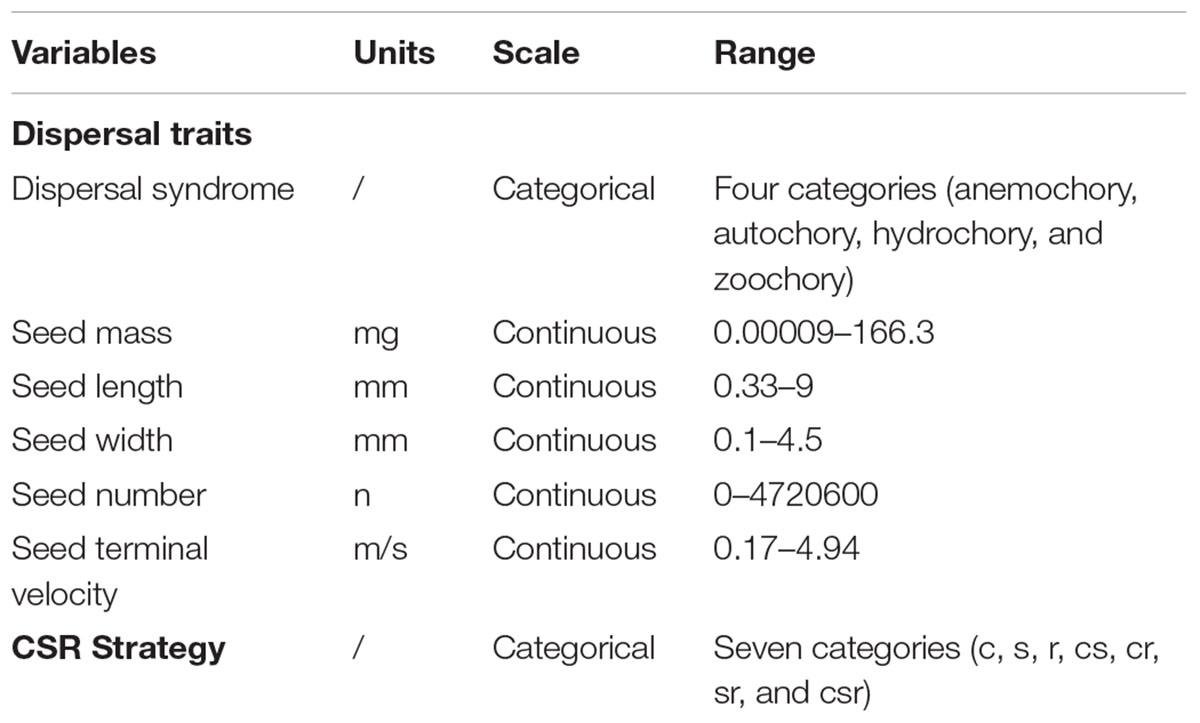
Table 1. Predictor variables (dispersal traits, CRS strategy) used in the conditional inference tree to predict occurrence of populations of endangered plant species across the different types of ecosystems.
In a second step, we performed a conditional inference tree analysis with the predictors shown in Table 1. A conditional inference tree is a non-parametric type of decision tree where the dataset is recursively split into dichotomous subsets which are discriminated by the most significant predictor (Hothorn et al., 2006). To analyze if populations attributed to different categories of ecological novelty in each terminal node contribute disproportionally to the different types of ecosystems, we performed a log-linear model using the R-function loglin and computed the standardized residuals to reveal any positive or negative deviations from the expected frequencies of population distribution across the different types of ecological novelty. These deviations were then included as symbols in the resulting nodes in the conditional inference tree, displaying over- or under- representation of populations compared to the expected frequencies. All analyses were performed with the statistical and programming software R version 3.5.2 (R Core Team, 2018).
Results
Spatial Clustering of Populations of Endangered Plant Species
The analysis of the distribution patterns of the endangered plant populations by means of Ripley’s L function revealed a spatial clustering of populations in all three novelty categories over the entire range of distances between the populations, as all curves of the Ripley’s L function exceed the dashed line that represents the null model of complete spatial randomness (Figure 2). Furthermore, populations that are located in natural remnant ecosystems have a higher level of clustering than those located in hybrid or novel ecosystems (Figure 2).
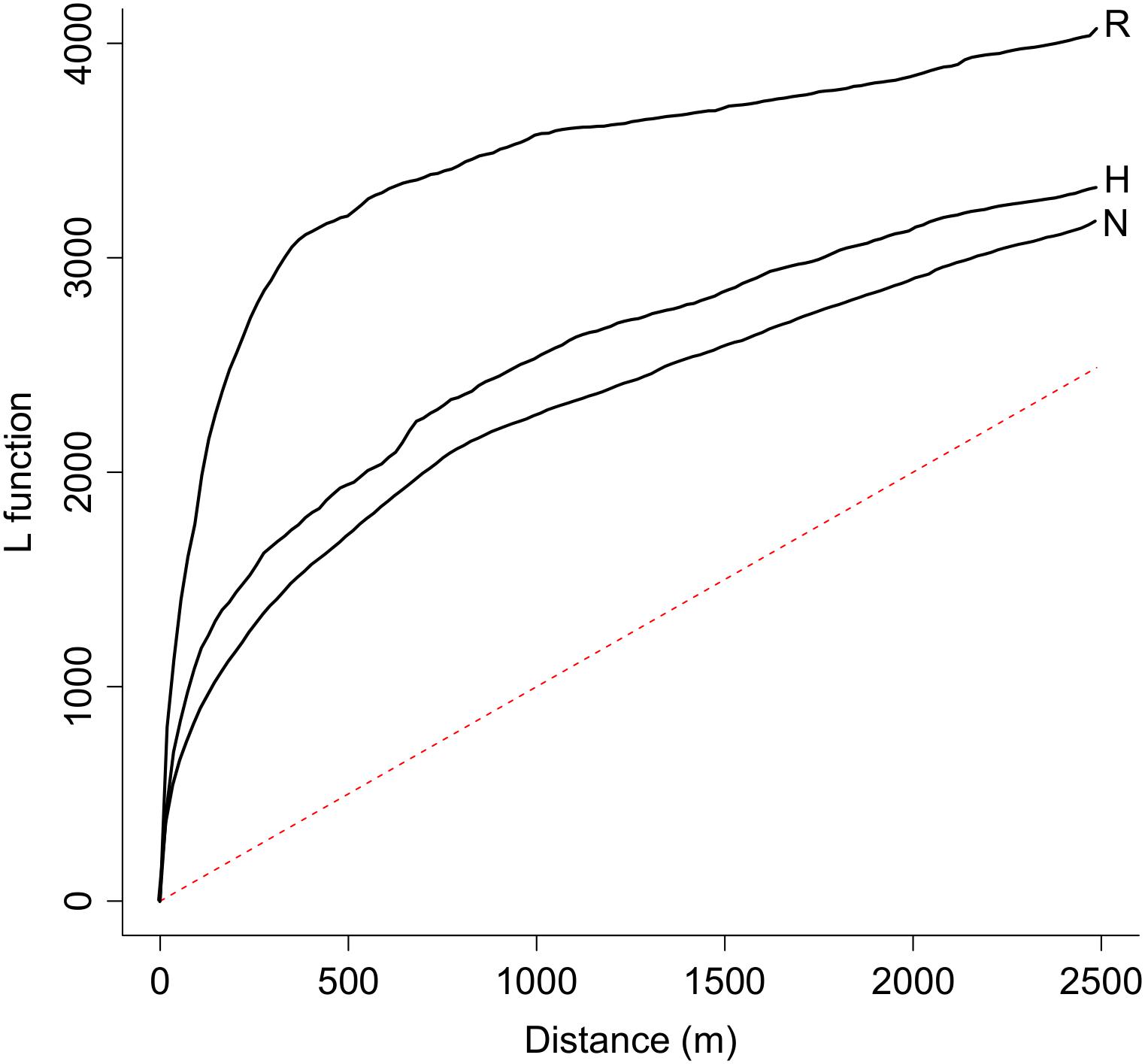
Figure 2. Populations of endangered plant species in Berlin are spatially clustered as indicated by the value of Ripley’s L-function over the entire range of distances between each two populations. The level of clustering is higher for populations located in natural remnant ecosystems (line R, n = 423) than for populations located in hybrid (line H, n = 632) or novel (line N, n = 687) ecosystems. A random spatial Poisson distribution is marked as a red dashed line.
Spatial Relation Between Populations of Endangered Plant Species and Different Novelty Categories
Results from the cross L function show that from the populations located in anthropogenic ecosystems, only those in hybrid ecosystems are spatially correlated to natural remnant ecosystems (Figure 3A, green line), as the observed curve of the point process crosses outside of the boundaries of the global envelope of the null model (Baddeley et al., 2014). An additional cross pair correlation function indicates that this correlation is positive (dependence), especially at distances from 0 to 700 m (Figure 3B, green line), where values lie far above 1 (Penttinen et al., 1992; Baddeley et al., 2015).
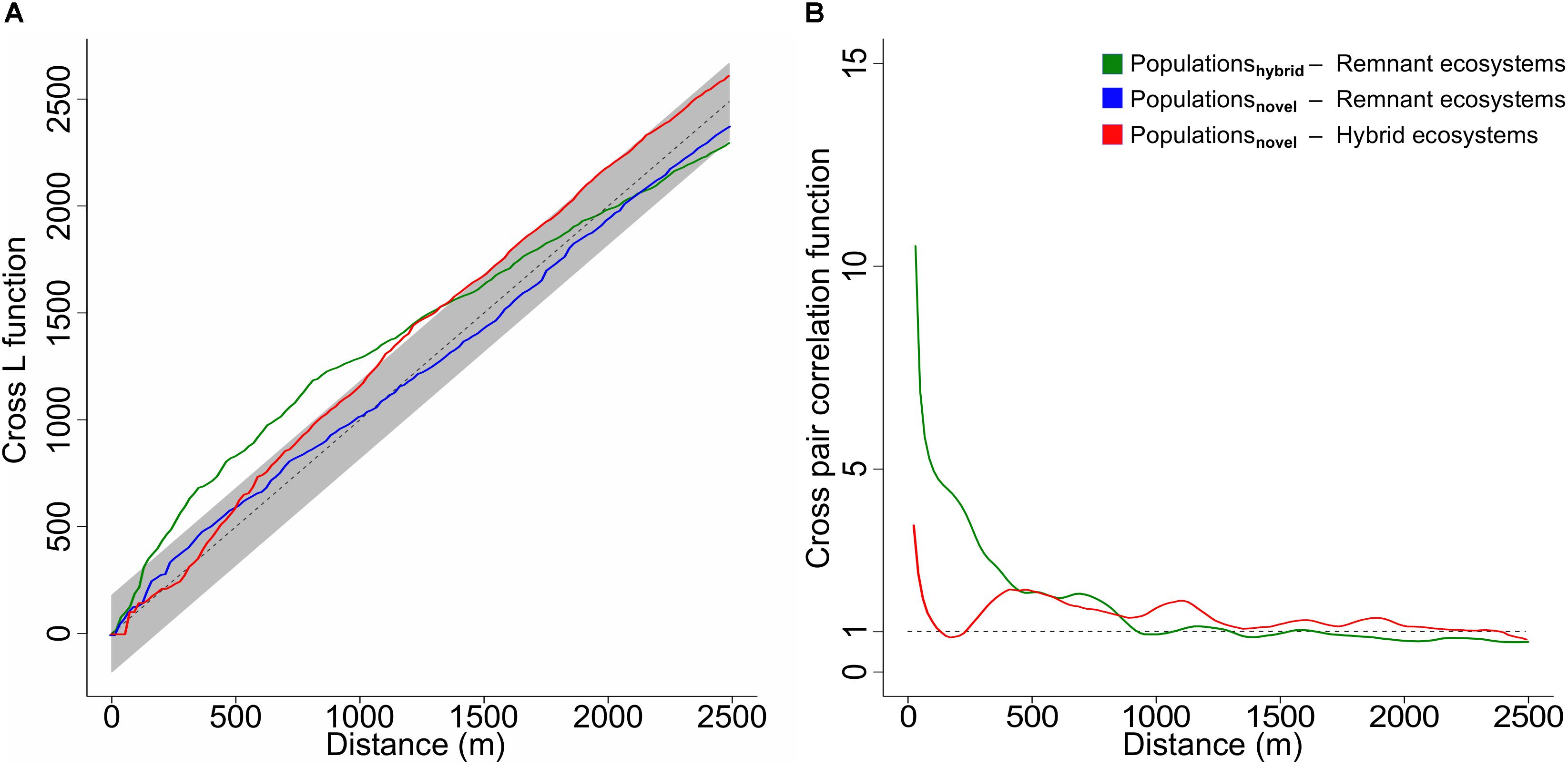
Figure 3. (A) Cross L function showing significant (p < 0.05) spatial correlations at increasing distances between populations in hybrid ecosystems (n = 632) and remnant ecosystem patches (n = 3426) (green line) and between populations in novel ecosystems (n = 687) and hybrid ecosystem patches (n = 15681) (red line), as in all these cases the line of the observed point process crosses at some point outside of the boundaries of the global envelope of the null model (dashed line with gray envelope generated from 19 simulations). No spatial correlation (independence) was found (95% confidence level) between populations in novel ecosystems (n = 687) and remnant ecosystem patches (n = 3426) (blue line), as the observed curve of the point process (blue line) does not cross outside of the boundaries of the global envelope of the null model. (B) Cross pair correlation function showing the type of correlation at increasing distances between populations in hybrid ecosystems (n = 632) and remnant ecosystem patches (n = 3426) (green line) and between populations in novel ecosystems (n = 687) and hybrid ecosystem patches (n = 15681) (red line). Values higher than 1 (dashed line) indicate an increasingly stronger positive correlation (dependence). Please note that the blue line is not represented as there is spatial independence between populations in novel ecosystems and remnant ecosystems (Figure 3A).
In contrast, populations located in novel ecosystems are spatially independent from remnant ecosystems (Figure 3A, blue line). Yet, they are spatially correlated to hybrid ecosystems (Figure 3A, red line). In the latter case, the cross pair correlation function indicates that this correlation is positive (dependence), with a lower intensity, especially at shorter distances from 0 to 400 m (Figure 3B, red line).
Dispersal Traits and CSR Strategy
From all the predictor variables, the conditional inference tree retained CSR strategy (p < 0.001) and dispersal syndrome (p = 0.001) as significant predictors of population occurrence across the different types of ecological novelty (Figure 4). Endangered species assigned to a ruderal strategy type (r, rc, rs, rcs) and with anemochory as main dispersal syndrome were exceedingly represented in novel ecosystems (Node 3, Figure 4). In contrast, competitive and stress-tolerant species (c, s, cs) were much more common in remnant ecosystems than elsewhere (Node 5, Figure 4). Finally, populations of species assigned to a ruderal strategy type in combination with other dispersal syndromes other than anemochory were represented equally amongst natural remnants, hybrid and novel ecosystems (Node 4, Figure 4).
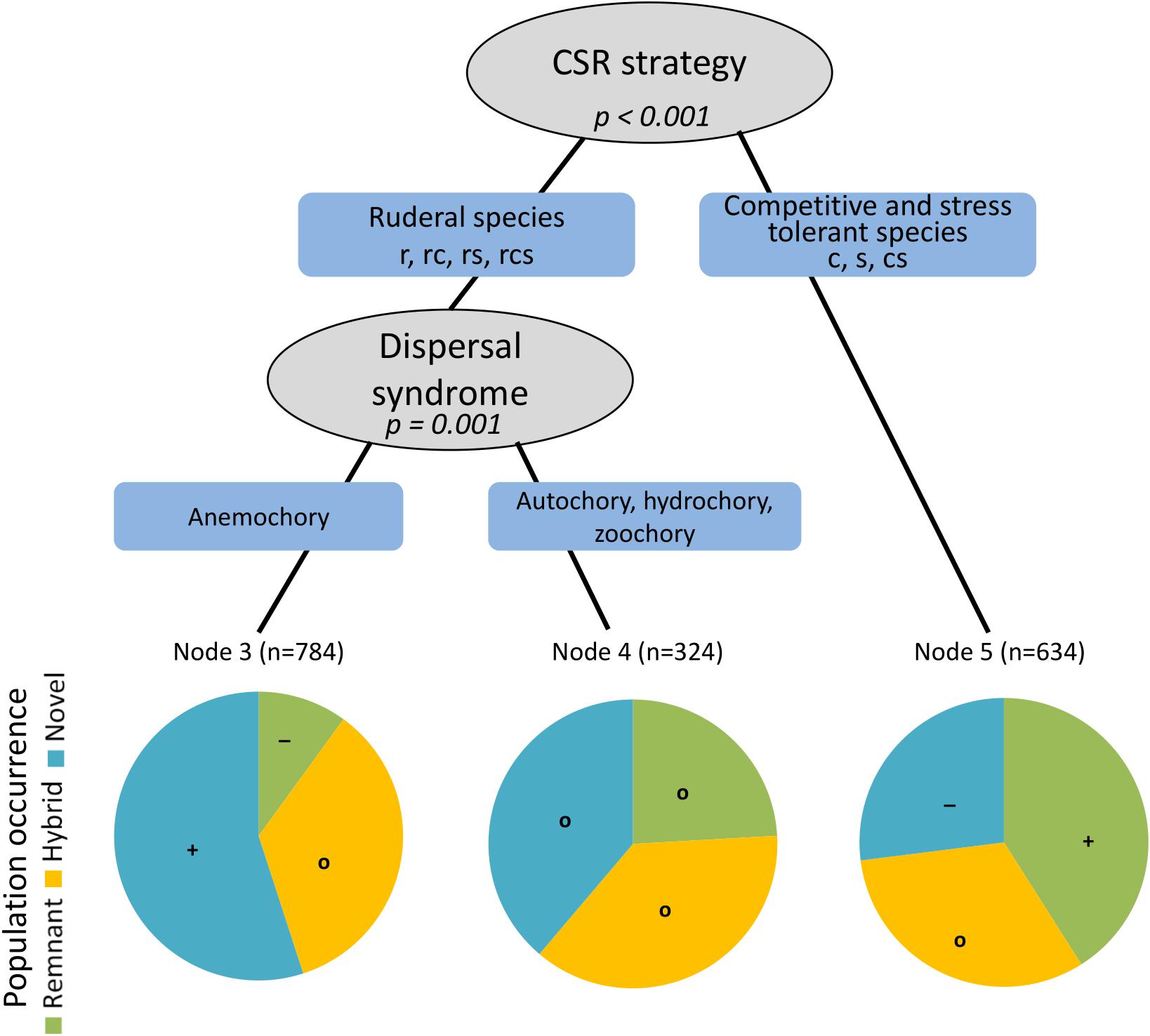
Figure 4. Conditional inference tree showing the partitioning effects of plant dispersal syndrome and CSR strategy on the occurrence of populations of endangered plant species across natural remnant (green), hybrid (yellow) and novel (blue) ecosystems in Berlin. Positive and negative deviations from the expected frequencies of population from the three novelty types are displayed by symbols in the pie charts (+, more than four standardized residuals showing an overrepresentation of populations of the respective category; –, less than four standardized residuals showing an underrepresentation of populations; o, –2 to 2 standardized residuals, showing a similar representation of populations to the expected frequencies); n indicates the number of populations in each branch.
Discussion
It is well established that plants in cities are not randomly distributed in space, but follow specific spatial patterns, driven by feedbacks between ecological and socio-economic factors (Swan et al., 2011). While many studies have highlighted the importance of natural remnants in hosting species of conservation concern in urban regions (e.g. Godefroid and Koedam, 2003; Celesti-Grapow et al., 2013), recent studies also revealed a high relative importance of anthropogenic habitats for endangered plant species in cities (Kowarik and von der Lippe, 2018; Planchuelo et al., 2019; Soanes et al., 2019). Yet to which extent populations of endangered plant species in anthropogenic habitats rely on the adjacency to natural remnants as seed sources is a largely open question, since point data on the exact location of endangered plant species are mostly missing for cities.
This study makes a step forward by linking the location of each of 1,742 populations of 213 plant species of highest conservation concern in Berlin to one of three ecosystem types that represent different transformation stages of natural to urban landscapes, i.e. natural remnants, hybrid ecosystems and novel ecosystems, following the novel ecosystem approach (Hobbs et al., 2009). Our methodological approach advances the understanding of spatial patterns of endangered species at the city scale, because the scarce studies on this topic are often based on species inventories of grid cells (e.g. Kühn et al., 2004; Schwartz et al., 2013; Schmidt et al., 2014). While spatial relationships between anthropogenic habitats and natural remnants can be masked by the environmental heterogeneity of grid cells, our study relies on point data for populations and on spatially explicit data from about 80,000 biotope patches covering the entire area of Berlin, which in a previous study were classified as natural remnants, hybrid or novel ecosystems (Planchuelo et al., 2019). This enabled us to test for the habitat function of different transformation stages of natural ecosystems in cities for endangered plant species.
Spatial Relationship Between Natural Remnants and Populations of Endangered Plant Species
A first insight of this study is that the total populations of endangered plant species are not randomly distributed throughout Berlin but are overall clustered inside their respective habitats, as commonly happens in highly dynamic landscapes (Johst et al., 2002). Spatial clustering of endangered plant populations was most pronounced in natural remnants. It is likely that clustering of populations in this habitat type merely reflects the rarity and scattered spatial distribution of natural remnants in Berlin. Natural remnants only occupy 7% of the surface area within the city borders but host 24% of all populations of endangered plant species (Planchuelo et al., 2019).
As a major and unexpected result of our study we found contrasting spatial dependences of endangered plant populations among the two anthropogenic types of ecosystems and natural remnants (Figure 3A). Populations in hybrid ecosystems spatially depended on natural remnants – while populations in novel ecosystems did not. As dispersal is the ecological phenomenon that most often causes spatial dependence (Epperson, 2005; Bahn et al., 2008), our results suggest an exchange of species between natural remnants and hybrid ecosystems. This could happen through a source-sink dynamic (Pulliam, 1988; Schreiber, 2010), in which remnant ecosystems are acting as seed sources for adjacent hybrid ecosystems. The importance of natural remnants as relict habitats of endangered plant species is well known (Rebelo et al., 2011; Kowarik and von der Lippe, 2018). Correspondingly, Jarošík et al. (2011) demonstrated that the number of endangered species decreased with distance to natural areas. In Christchurch, New Zealand, a forest remnant within the city functioned as seed source for the colonization of adjacent gardens by native bush species, yet mostly within only 250 m of the forest margin (Doody et al., 2010). Our results corroborate these findings, as the strength of the spatial interaction between natural remnants and populations in hybrid ecosystems was highest in a spatial range between 0 and approximately 400 m (Figure 3B), suggesting that dispersal capabilities prevent many species from directly dispersing further than that (Jacquemyn et al., 2003).
As an alternative but not mutually exclusive explanation, some populations in hybrid ecosystems might be relicts of former remnants that have persisted despite land use change or increasing levels of urbanization. Grasslands, for example, are a largely anthropogenic type of vegetation in Berlin (Sukopp, 1990), but some endangered grassland species stem from habitats in open forests, clearings or forest edges (e.g. Festuca psammophila or Botrychium lunaria) and have survived the transformation of forests to grassland as hybrid ecosystems. To untangle the relative role of dispersal-related versus persistence-related mechanisms underlying the presence of endangered plants in hybrid ecosystems is a future research direction which requires spatial information about land-use legacies.
Since novel urban ecosystems have experienced much deeper changes to the abiotic conditions and species assemblages (Hobbs et al., 2009; Kowarik, 2011), relict populations or seed banks of endangered plant species likely do not persist in these systems. However, novel ecosystems can offer habitat analogs for a range of native species (Lundholm and Richardson, 2010), i.e. new environmental conditions to which some species may already have been pre-adapted in advance (McDonnell and Hahs, 2015). In Berlin, a considerable amount of 687 populations (40% of the total) encompassing 142 highly endangered species occur in novel ecosystems. One important insight of this study is that in Berlin these populations are spatially independent from natural remnant ecosystems, likely due to dispersal barriers associated with higher levels of urbanization in novel ecosystems. In this context, hybrid ecosystems could partly act as stepping stones between natural remnants and novel ecosystems, as populations in novel ecosystems were slightly dependent on hybrid ecosystems – while at the same time populations in hybrid ecosystems were spatially dependent on remnant ecosystems. However, species exchange between hybrid ecosystems and novel ecosystems alone cannot explain the vast number of populations of endangered plant species in the latter, as populations in Berlin’s novel ecosystems are only weakly correlated to hybrid ecosystems, and only so in distances over 400 m. Thus, the high number of populations here could be explained by additional natural or human mediated long-distance dispersal from far away sources and a subsequent establishment of meta-communities within ecologically interconnected novel ecosystems (Loreau et al., 2003). In our dataset, for instance, some species occur across the urban matrix in novel ecosystems that are very distant from each other (e.g. Cystopteris fragils or Dianthus carthusianorum). Conducting genetic analyses to assess whether these populations originate from the same source would be an interesting path for future research.
Strategy Type and Dispersal Syndrome Filter Species Pools of Different Habitat Types
Shifts of biological traits in urban floras compared to rural floras are well established (Knapp et al., 2008a, 2010; Williams et al., 2015). Our study revealed that biological features (i.e. strategy type, dispersal syndrome) filtered endangered plant species differently for natural remnants, hybrid and novel ecosystems.
As expected, the conditional inference tree revealed that species assigned to a ruderal strategy (component) according to Grime (1977) were more present in novel urban ecosystems than anticipated by chance, while species related to competitive or stress strategies were more confined to remnant ecosystems (Figure 4). This goes along with the review by Williams et al. (2015) who found that many studies report increased numbers of ruderal species in urban floras – despite diverging results existing as well. Our study helps understand ambiguous previous results since different strategy types matter for different types of ecosystems. Thus, the transformation from natural ecosystems to novel urban ecosystems filters for species adhering to the ruderal strategy types, while no specifications were detected for hybrid ecosystems. Natural remnants, in contrast, are characterized by a higher presence of competitive and stress-tolerant species, most probably because of their limited dispersal as a consequence of a trade-off between competition and dispersal (Pellissier, 2015) and the low reproductive effort of stress-tolerant species (Chapin et al., 1993).
As spatial dependence in distribution patterns of plants is closely related to dispersal processes (Epperson, 2005; Bahn et al., 2008), we expected that dispersal-related plant traits filtered the suite of endangered plant species across the different types of ecosystems. A major insight of this study is that dispersal syndrome filtered for species composition of natural remnants versus novel ecosystems. Anemochory (i.e. wind dispersal) was significantly related to the latter, but only for species with a ruderal strategy component (Figure 4). The revealed relevance of wind dispersal for endangered species in novel ecosystems seems to contradict results of Williams et al. (2015) who found that most of the few studies on species extinction in cities showed that wind-dispersed species were more likely to disappear than species dispersed by other vectors (e.g. Williams et al., 2005; Knapp et al., 2010); yet studies on the total urban flora did not find consistent patterns in dispersal modes (Williams et al., 2015). These ambiguous results can be explained by the context dependence of relevant species traits in relation to different urban ecosystems as illustrated by Figure 4, and contribute to the understanding of the spatial independence of populations in novel ecosystems from natural remnants, since wind dispersal often translates to long-distance dispersal (Cain et al., 2000).
While our study revealed the importance of long-distance dispersal by wind for filtering species pools of endangered species throughout different urban ecosystem types, the role of human-mediated long-distance dispersal might be underestimated in our analyses. Indeed, dispersal by humans is considered one of the most important mechanisms of long-distance dispersal (Nathan, 2006), and species with human vectors as an additional dispersal pathway are increasingly found over time in highly urbanized neighborhoods (Johnson et al., 2017). Correspondingly, urban roads function as dispersal highways for a broad range of native species (von der Lippe and Kowarik, 2008), and likely more for anthropogenic than for remnant ecosystems as the former are usually more exposed to transportation corridors. Given that vehicles can move native species over long-distances along urban-rural gradients (von der Lippe and Kowarik, 2008), human-mediated dispersal might thus link novel with other ecosystem types. Furthermore, high seed production, which is a feature of many ruderal species (Grime, 1977), increases the chance of dispersal by vehicles, especially for seeds with a low terminal velocity like those of wind dispersed species (von der Lippe and Kowarik, 2012). In consequence, seeds of wind dispersed species might be introduced from distant sources (i.e. hybrid ecosystems) into novel ecosystems through the road network, while the same mechanism could support meta-communities of species already established in novel ecosystems. Work on pollen dispersal correspondingly indicates a high level of exchange between different types of urban ecosystems (Van Rossum, 2010; Van Rossum and Triest, 2012).
Future Perspectives
The present study suggests that meta-communities of plants might exist in novel ecosystems within cities. A future direction is to examine the spatial structure and dynamics of populations in novel urban environments (and probably related sources) also with genetic analyses.
On a different note, our research shows that differences in interspecific plant traits are related to the distribution of endangered plants across different kinds of urban ecosystems. Previous research has shown rapid changes and evolutionary adaptations in some urban plants (McDonnell and Hahs, 2015; Dubois and Cheptou, 2017), and that trait frequencies can shift as a consequence of different levels of urbanization (Knapp et al., 2008b) or urban stressors (Williams et al., 2015). Thus, it would be most interesting to assess patterns of intraspecific trait adaptations throughout populations of the same species located in ecosystems with varying levels of ecological novelty.
Finally, though Berlin shares similar land use legacies (Ramalho and Hobbs, 2012) and configuration of urban elements (Louf and Barthelemy, 2014) with other historical European cities, results of this study cannot be generalized for all urban contexts. The classification of the urban ecosystems according to their ecological novelty is a first step in providing a general scheme to compare species distributions between cities of different history, and comparable studies in other cities could be used to verify to which extent biodiversity patterns vary amongst them.
Conclusion
The urban contribution to biodiversity conservation is widely debated (Shwartz et al., 2014; Soanes et al., 2019), with one important question being the role of urban ecosystems of anthropogenic origin for endangered plant species. While previous studies have revealed an important relative contribution of human-shaped hybrid and novel ecosystems as habitats of endangered plant species (Kowarik and von der Lippe, 2018; Planchuelo et al., 2019) this study adds further insights into the underlying mechanisms, based on fine-grained georeferenced data for a high number of populations of endangered plant species and urban biotope patches.
The spatial analyses allow three major conclusions to be drawn. First, the spatial dependence of populations in hybrid ecosystems on natural remnants shows the latter are important habitats and seed sources for endangered plant species and highlights the importance of conserving these remains of natural landscapes in urban regions. Second, the missing spatial dependence of populations in novel ecosystems demonstrates that novel urban ecosystems can support populations of a range of endangered species beyond the adjacency to natural remnants. Third, our results suggest that hybrid ecosystems may act as essential stepping stones by mediating the spread of endangered plant species into novel urban ecosystems.
The existence of natural remnants in cities can partly be explained by the fact that urban agglomerations have preferably been founded in naturally rich landscapes (Kühn et al., 2004). Yet at least in Berlin, these legacies do not ultimately determine the spatial patterns of endangered plant species in profoundly changed novel urban ecosystems. This is a strong argument for including the total range of natural and anthropogenic ecosystems in urban conservation strategies.
Discerning different biological features (i.e. strategy type, dispersal syndrome) related to the presence of endangered plants in natural remnants, hybrid ecosystems and novel ecosystems demonstrates that different filters modulate the presence of endangered plants across the trajectory of urban habitat transformation. Identifying traits of species that successfully colonize novel urban ecosystems demonstrates environmental barriers for other endangered species. This has important implications for conservation efforts, e.g. for conserving and managing those species with highest priority in natural remnants that are likely unable to colonize novel urban ecosystems; or by strictly selecting dispersal-limited species for introduction to novel ecosystems in restoration measures.
Understanding the spatial relationships between populations of endangered plant species in different urban ecosystems thus supports conservation approaches that cover the total range of urban ecosystems and associated opportunities for biodiversity conservation in cities.
Data Availability Statement
The datasets generated for this study are available on request to the corresponding author.
Author Contributions
All persons entitled to authorship have been so named. All authors conceived the ideas, designed methodology, and contributed to writing. GP processed the data. GP and ML analyzed the data.
Funding
GP was funded by the Hans Böckler Foundation and the TU Berlin STIBET – DAAD Grant. Parts of this study were funded by the German Federal Ministry of Education and Research (BMBF) within the Collaborative Project “Bridging in Biodiversity Science-BIBS” (funding number 01LC1501A-H). We acknowledge support by the German Research Foundation and the Open Access Publication Fund of TU Berlin.
Conflict of Interest
The authors declare that the research was conducted in the absence of any commercial or financial relationships that could be construed as a potential conflict of interest.
Acknowledgments
We thank Justus Meißner and Stiftung Naturschutz Berlin for sharing the population data, and Berlin’s Senate Department for the Environment, Transport and Climate Protection for providing GIS layers of biotope and land use maps. We thank likewise Jonas Tumbrink for his comments on the manuscript. We also thank reviewers for their comments. This manuscript includes content from the doctoral dissertation of GP (Planchuelo, 2019).
Supplementary Material
The Supplementary Material for this article can be found online at: https://www.frontiersin.org/articles/10.3389/fevo.2020.00018/full#supplementary-material
Footnotes
References
Aronson, M. F., La Sorte, F. A., Nilon, C. H., Katti, M., Goddard, M. A., Lepczyk, C. A., et al. (2014). A global analysis of the impacts of urbanization on bird and plant diversity reveals key anthropogenic drivers. Proc. R. Soc. B Biol. Sci. 281:20133330. doi: 10.1098/rspb.2013.3330
Aronson, M. F., Nilon, C. H., Lepczyk, C. A., Parker, T. S., Warren, P. S., Cilliers, S. S., et al. (2016). Hierarchical filters determine community assembly of urban species pools. Ecology 97, 2952–2963. doi: 10.1002/ecy.1535
Baddeley, A. (2008). Analysing Spatial Point Patterns in R. Workshop Notes. CSIRO Online Technical Publication. Available at: https://darrylmcleod.com/wp-content/uploads/2016/06/Analysing-spatial-point-patterns-in-R.pdf
Baddeley, A., Diggle, P. J., Hardegen, A., Lawrence, T., Milne, R. K., and Nair, G. (2014). On tests of spatial pattern based on simulation envelopes. Ecol. Monogr. 84, 477–489. doi: 10.1890/13-2042.1
Baddeley, A., Rubak, E., and Turner, R. (2015). Spatial Point Patterns: Methodology and Applications with R. London: Chapman and Hall/CRC.
Bahn, V., Krohn, W. B., and O’Connor, R. J. (2008). Dispersal leads to spatial autocorrelation in species distributions: a simulation model. Ecol. Modell. 213, 285–292. doi: 10.1016/j.ecolmodel.2007.12.005
Beninde, J., Veith, M., and Hochkirch, A. (2015). Biodiversity in cities needs space: a meta-analysis of factors determining intra-urban biodiversity variation. Ecol. Lett. 18, 581–592. doi: 10.1111/ele.12427
Cain, M. L., Milligan, B. G., and Strand, A. E. (2000). Long-distance seed dispersal in plant populations. Am. J. Bot. 87, 1217–1227. doi: 10.2307/2656714
Celesti-Grapow, L., Capotorti, G., Del Vico, E., Lattanzi, E., Tilia, A., and Blasi, C. (2013). The vascular flora of Rome. Plant Biosyst. 147, 1059–1087.
Chapin, F. S. III, Autumn, K., and Pugnaire, F. (1993). Evolution of suites of traits in response to environmental stress. Am. Nat. 142, 78–92.
Chytrý, M., Lososová, Z., Horsák, M., Uher, B., Èejka, T., Danihelka, J., et al. (2012). Dispersal limitation is stronger in communities of microorganisms than macroorganisms across Central European cities. J. Biogeogr. 39, 1101–1111. doi: 10.1111/j.1365-2699.2011.02664.x
Dixon, P. M. (2002). “Ripley’s K function,” in The Encyclopedia of Environmetrics, eds A.H. El-Shaaraui. and W.W. Piergorsch. (New York, NY: Wiley), 1796–1803.
Doody, B. J., Sullivan, J. J., Meurk, C. D., Stewart, G. H., and Perkins, H. C. (2010). Urban realities: the contribution of residential gardens to the conservation of urban forest remnants. Biodivers. Conserv. 19, 1385–1400. doi: 10.1007/s10531-009-9768-2
Dubois, J., and Cheptou, P. O. (2017). Effects of fragmentation on plant adaptation to urban environments. Phil. Trans. R. Soc. Lond. B Biol. Sci. 372:20160038. doi: 10.1098/rstb.2016.0038
Duncan, R. P., Clemants, S. E., Corlett, R. T., Hahs, A. K., McCarthy, M. A., McDonnell, M. J., et al. (2011). Plant traits and extinction in urban areas: a meta–analysis of 11 cities. Glob. Ecol. Biogeogr. 20, 509–519. doi: 10.1111/j.1466-8238.2010.00633.x
Epperson, B. K. (2005). Estimating dispersal from short distance spatial autocorrelation. Heredity 95, 7–15. doi: 10.1038/sj.hdy.6800680
Godefroid, S., and Koedam, N. (2003). How important are large vs. small forest remnants for the conservation of the Woodland Flora in an urban context? Glob. Ecol. Biogeogr. 12, 287–298. doi: 10.1046/j.1466-822x.2003.00035.x
Grime, J. P. (1977). Evidence for the existence of three primary strategies in plants and its relevance to ecological and evolutionary theory. Am. Nat. 111, 1169–1194. doi: 10.1086/283244
Hahs, A. K., and McDonnell, M. J. (2014). Extinction debt of cities and ways to minimise their realisation: a focus on Melbourne. Ecol. Manag. Restor. 15, 102–110. doi: 10.1111/emr.12112
Hobbs, R. J., Higgs, E., and Harris, J. A. (2009). Novel ecosystems: implications for conservation and restoration. Trends Ecol. Evol. 24, 599–605. doi: 10.1016/j.tree.2009.05.012
Hothorn, T., Hornik, K., and Zeileis, A. (2006). Unbiased recursive partitioning: a conditional inference framework. J. Comput. Graph. Stat. 15, 651–674. doi: 10.1198/106186006x133933
Ives, C. D., Lentini, P. E., Threlfall, C. G., Ikin, K., Shanahan, D. F., Garrard, G. E., et al. (2016). Cities are hotspots for threatened species. Glob. Ecol. Biogeogr. 25, 117–126. doi: 10.1111/geb.12404
Jacquemyn, H., Butaye, J., and Hermy, M. (2001). Forest plant species richness in small, fragmented mixed deciduous forest patches: the role of area, time and dispersal limitation. J. Biogeogr. 28, 801–812. doi: 10.1046/j.1365-2699.2001.00590.x
Jacquemyn, H., Butaye, J., and Hermy, M. (2003). Influence of environmental and spatial variables on regional distribution of forest plant species in a fragmented and changing landscape. Ecography 26, 768–776. doi: 10.1111/j.0906-7590.2003.03620.x
Jarošík, V., Konvièka, M., Pyšek, P., Kadlec, T., and Beneš, J. (2011). Conservation in a city: do the same principles apply to different taxa? Biol. Conserv. 144, 490–499. doi: 10.1016/j.biocon.2010.10.002
Johnson, A. L., Borowy, D., and Swan, C. M. (2017). Land use history and seed dispersal drive divergent plant community assembly patterns in urban vacant lots. J. Appl. Ecol. 55, 451–460. doi: 10.1111/1365-2664.12958
Johst, K., Brandl, R., and Eber, S. (2002). Metapopulation persistence in dynamic landscapes: the role of dispersal distance. Oikos 98, 263–270. doi: 10.1034/j.1600-0706.2002.980208.x
Klotz, S., Kühn, I., Durka, W., and Briemle, G. (2002). BIOLFLOR: Eine Datenbank mit Biologisch-Ökologischen Merkmalen zur Flora von Deutschland, Vol. 38. Bonn: Bundesamt für Naturschutz.
Knapp, S., Kühn, I., Bakker, J. P., Kleyer, M., Klotz, S., Ozinga, W. A., et al. (2009). How species traits and affinity to urban land use control large-scale species frequency. Divers. Distrib. 15, 533–546. doi: 10.1111/j.1472-4642.2009.00561.x
Knapp, S., Kühn, I., Mosbrugger, V., and Klotz, S. (2008a). Do protected areas in urban and rural landscapes differ in species diversity? Biodivers. Conserv. 17, 1595–1612. doi: 10.1007/s10531-008-9369-5
Knapp, S., Kühn, I., Stolle, J., and Klotz, S. (2010). Changes in the functional composition of a Central European urban flora over three centuries. Perspect. Plant Ecol. Evol. Syst. 12, 235–244. doi: 10.1016/j.ppees.2009.11.001
Knapp, S., Kuehn, I., Wittig, R., Ozinga, W. A., Poschlod, P., and Klotz, S. (2008b). Urbanization causes shifts of species’ trait state frequencies. Preslia 80, 375–388.
Kowarik, I. (1990). “Some responses of flora and vegetation to urbanization in Central Europe,” in Plants and Plant Communities in the Urban Environment, eds H. Sukopp, S. Hejny, and I. Kowarik, (The Hague: SPB Academic Publishing), 45–74.
Kowarik, I. (2011). Novel urban ecosystems, biodiversity, and conservation. Environ. Pollut. 159, 1974–1983. doi: 10.1016/j.envpol.2011.02.022
Kowarik, I., and von der Lippe, M. (2018). Plant population success across urban ecosystems: a framework to inform biodiversity conservation in cities. J. Appl. Ecol. 55, 2354–2361. doi: 10.1111/1365-2664.13144
Kühn, I., Brandl, R., and Klotz, S. (2004). The flora of German cities is naturally species rich. Evol. Ecol. Res. 6, 749–764.
Lawson, D. M., Lamar, C. K., and Schwartz, M. W. (2008). Quantifying plant population persistence in human-dominated landscapes. Conserv. Biol. 22, 922–928. doi: 10.1111/j.1523-1739.2008.00936.x
Lenzen, M., Lane, A., Widmer-Cooper, A., and Williams, M. (2009). Effects of land use on threatened species. Conserv. Biol. 23, 294–306. doi: 10.1111/j.1523-1739.2008.01126.x
Lim, Y., Na, S.-T., Lee, S.-J., Cho, K. H., and Shin, H. (2008). Spatial distribution patterns and implications for conservation of Scrophularia takesimensis (Scrophulariaceae), an endangered endemic species on Ulleung Island, Korea. J. Plant Biol. 51:213. doi: 10.1007/bf03030701
Loreau, M., Mouquet, N., and Holt, R. D. (2003). Meta-ecosystems: a theoretical framework for a spatial ecosystem ecology. Ecol. Lett. 6, 673–679. doi: 10.1046/j.1461-0248.2003.00483.x
Louf, R., and Barthelemy, M. (2014). A typology of street patterns. J. R. Soc. Interface 11:20140924. doi: 10.1098/rsif.2014.0924
Lundholm, J. T., and Richardson, P. J. (2010). Habitat analogues for reconciliation ecology in urban and industrial environments. J. Appl. Ecol. 47, 966–975. doi: 10.1111/j.1365-2664.2010.01857.x
McDonnell, M. J., and Hahs, A. K. (2015). Adaptation and adaptedness of organisms to urban environments. Ann. Rev. Ecol. Evol. Syst. 46, 261–280. doi: 10.1146/annurev-ecolsys-112414-054258
McKinney, M. L. (2008). Effects of urbanization on species richness: a review of plants and animals. Urban Ecosyst. 11, 161–176. doi: 10.1007/s11252-007-0045-4
Meißner, J. S., and Seitz, B. (2010). Das florenschutzkonzept im land Berlin und seine umsetzung. Verh. Bot. Ver. Berlin Brandenburg 143, 111–120.
Nathan, R. (2006). Long-distance dispersal of plants. Science 313, 786–788. doi: 10.1126/science.1124975
Pellissier, L. (2015). Stability and the competition-dispersal trade-off as drivers of speciation and biodiversity gradients. Front. Ecol. Evol. 3:52.
Penttinen, A., Stoyan, D., and Henttonen, H. M. (1992). Marked point processes in forest statistics. Forest Sci. 38, 806–824.
Planchuelo, G. (2019). Urbanisation Impacts on Rare and Endangered Plant Species. Dr. Dissertation, Technische Universität Berlin, Berlin.
Planchuelo, G., von der Lippe, M., and Kowarik, I. (2019). Untangling the role of urban ecosystems as habitats for endangered plant species. Landsc. Urban Plan. 189, 320–334. doi: 10.1016/j.landurbplan.2019.05.007
Pulliam, H. R. (1988). Sources, sinks, and population regulation. Am. Nat. 132, 652–661. doi: 10.1086/284880
R Core Team (2018). R: A Language and Environment for Statistical Computing. Vienna: R Foundation for Statistical Computing.
Ramalho, C. E., and Hobbs, R. J. (2012). Time for a change: dynamic urban ecology. Trends Ecol. Evol. 27, 179–188. doi: 10.1016/j.tree.2011.10.008
Ramalho, C. E., Laliberte, E., Poot, P., and Hobbs, R. J. (2014). Complex effects of fragmentation on remnant woodland plant communities of a rapidly urbanizing biodiversity hotspot. Ecology 95, 2466–2478. doi: 10.1890/13-1239.1
Rastandeh, A., Brown, D. K., and Pedersen Zari, M, (2017). “Biodiversity conservation in urban environments: a review on the importance of spatial patterning of landscapes,” in Proceeding of the Ecocity World Summit, Melbourne, VIC, 12–14.
Rebelo, A. G., Holmes, P. M., Dorse, C., and Wood, J. (2011). Impacts of urbanization in a biodiversity hotspot: conservation challenges in Metropolitan Cape Town. S. Afr. J. Bot. 77, 20–35. doi: 10.1016/j.sajb.2010.04.006
Ripley, B. D. (1991). Statistical Inference for Spatial Processes. Cambridge: Cambridge university press.
Rodríguez-Pérez, J., Wiegand, T., and Traveset, A. (2012). Adult proximity and frugivore’s activity structure the spatial pattern in an endangered plant. Funct. Ecol. 26, 1221–1229. doi: 10.1111/j.1365-2435.2012.02044.x
Schmidt, K. J., Poppendieck, H. H., and Jensen, K. (2014). Effects of urban structure on plant species richness in a large European city. Urban Ecosyst. 17, 427–444. doi: 10.1007/s11252-013-0319-y
Schreiber, S. J. (2010). Interactive effects of temporal correlations, spatial heterogeneity and dispersal on population persistence. Proc. R. Soc. B Biol. Sci. 277, 1907–1914. doi: 10.1098/rspb.2009.2006
Schwartz, M. W., Jurjavcic, N. L., and O’Brien, J. M. (2002). Conservation’s disenfranchised urban poor. Bioscience 52, 601–606.
Schwartz, M. W., Smith, L. M., and Steel, Z. L. (2013). Conservation investment for rare plants in urban environments. PLoS One 8:e83809. doi: 10.1371/journal.pone.0083809
Seitz, B. (2007). Konzeption zum Florenschutz im Land Berlin. Berlin: Gutachten im Auftrag des Landesbeauftragten für Naturschutz und Landschaftspflege Berlin.
Seitz, B., Ristow, M., Meißner, J., Machatzi, B., and Sukopp, H. (2018). Rote Liste und Gesamtartenliste der etablierten Farn-und Blütenpflanzen von Berlin. Berlin: Universitätsverlag der TU Berlin.
SenStadtUm (2014). Berlin Environmental Atlas, Biotope Types. Berlin: Senate Department for Urban Development and Housing.
SenStadtUm (2016). Berlin Environmental Atlas, Map Actual Use and Vegetation. Berlin: Senate Department for Urban Development and Housing.
Shochat, E., Warren, P. S., Faeth, S. H., McIntyre, N. E., and Hope, D. (2006). From patterns to emerging processes in mechanistic urban ecology. Trends Ecol. Evol. 21, 186–191. doi: 10.1016/j.tree.2005.11.019
Shwartz, A., Turbe, A., Julliard, R., Simon, L., and Prevot, A. C. (2014). Outstanding challenges for urban conservation research and action. Glob. Environ. Change 28, 39–49. doi: 10.1016/j.gloenvcha.2014.06.002
Soanes, K., and Lentini, P. E. (2019). When cities are the last chance for saving species. Front. Ecol. Environ. 17, 225–231. doi: 10.1002/fee.2032
Soanes, K., Sievers, M., Chee, Y. E., Williams, N. S., Bhardwaj, M., Marshall, A. J., et al. (2019). Correcting common misconceptions to inspire conservation action in urban environments. Conserv. Biol. 33, 300–306. doi: 10.1111/cobi.13193
Stoyan, D., and Stoyan, H. (1994). Fractals, random shapes and point fields: methods of geometrical statistics. Z. Angew. Math. Mech. 75, 614–614. doi: 10.1002/zamm.19950750815
Sukopp, H. (1987). On the history of plant geography and plant ecology in Berlin. Englera 7, 85–103.
Sukopp, H., and Weiler, S. (1988). Biotope mapping and nature conservation strategies in Urban areas of the Federal-Republic-of-Germany. Lands. Urban Plan. 15, 39–58. doi: 10.1016/0169-2046(88)90015-1
Swan, C. M., Pickett, S. T., Szlavecz, K., Warren, P., and Willey, K. T. (2011). “Biodiversity and community composition in urban ecosystems: coupled human, spatial, and metacommunity processes,” in Handbook of urban ecology, (New York, NY: Oxford University Press), 179–186. doi: 10.1093/acprof:oso/9780199563562.003.0021
Van Rossum, F. (2010). Reproductive success and pollen dispersal in urban populations of an insect-pollinated hay-meadow herb. Perspect. Plant Ecol. Evol. Syst. 12, 21–29. doi: 10.1016/j.ppees.2009.08.002
Van Rossum, F., and Triest, L. (2012). Stepping-stone populations in linear landscape elements increase pollen dispersal between urban forest fragments. Plant Ecol. Evol. 145, 332–340. doi: 10.5091/plecevo.2012.737
von der Lippe, M., and Kowarik, I. (2008). Do cities export biodiversity? Traffic as dispersal vector across urban-rural gradients. Divers. Distrib. 14, 18–25. doi: 10.1016/j.scitotenv.2019.02.228
von der Lippe, M., and Kowarik, I. (2012). Interactions between propagule pressure and seed traits shape human-mediated seed dispersal along roads. Perspect. Plant Ecol. Evol. Syst. 14, 123–130. doi: 10.1016/j.ppees.2011.09.006
Wehenkel, C., Brazão-Protázio, J. M., Carrillo-Parra, A., Martínez-Guerrero, J. H., and Crecente-Campo, F. (2015). Spatial distribution patterns in the very rare and species-rich Picea chihuahuana tree community (Mexico). PLoS One 10:e0140442. doi: 10.1371/journal.pone.0140442
Williams, N. S., Morgan, J. W., Mcdonnell, M. J., and Mccarthy, M. A. (2005). Plant traits and local extinctions in natural grasslands along an urban–rural gradient. J. Ecol. 93, 1203–1213. doi: 10.1111/j.1365-2745.2005.01039.x
Williams, N. S. G., Hahs, A. K., and Vesk, P. A. (2015). Urbanisation, plant traits and the composition of urban floras. Perspect. Plant Ecol. Evol. Syst. 17, 78–86. doi: 10.1016/j.ppees.2014.10.002
Keywords: endangered species, natural remnants, point-pattern analysis, population distribution, spatial distribution, species traits, urban ecosystems, dispersal syndrome
Citation: Planchuelo G, Kowarik I and von der Lippe M (2020) Endangered Plants in Novel Urban Ecosystems Are Filtered by Strategy Type and Dispersal Syndrome, Not by Spatial Dependence on Natural Remnants. Front. Ecol. Evol. 8:18. doi: 10.3389/fevo.2020.00018
Received: 10 October 2019; Accepted: 23 January 2020;
Published: 14 February 2020.
Edited by:
Christopher Swan, University of Maryland, Baltimore County, United StatesReviewed by:
Boris Schröder, Technische Universität Braunschweig, GermanyMarco Moretti, Swiss Federal Institute for Forest, Snow and Landscape Research (WSL), Switzerland
Copyright © 2020 Planchuelo, Kowarik and von der Lippe. This is an open-access article distributed under the terms of the Creative Commons Attribution License (CC BY). The use, distribution or reproduction in other forums is permitted, provided the original author(s) and the copyright owner(s) are credited and that the original publication in this journal is cited, in accordance with accepted academic practice. No use, distribution or reproduction is permitted which does not comply with these terms.
*Correspondence: Greg Planchuelo, Z3JlZy5wbGFuY2h1ZWxvQGFsdW1uaS50dS1iZXJsaW4uZGU=