- 1Laboratory of Bio-inspired Chemistry and Ecological Innovations, ChimEco (UMR 5021), University of Montpellier, CNRS, Montpellier, France
- 2MIVEGEC, University of Montpellier, IRD, CNRS, Montpellier, France
Over the last decades, the geographical distribution of certain mosquito species carrying vector-borne diseases has considerably expanded. They represent a major health concern as they transmit pathogens causing alarming epidemics. In the absence of vaccines for most of the mosquito-borne diseases, the use of topical mosquito repellents is one of the main measures to prevent human-mosquito contacts. Synthetic repellents are the most used mosquito repellents. However, several concerns about their safety for consumers have led to a growing demand of natural alternatives. Here we present an overview of the most commonly used natural-based mosquito repellents: repellent activity, biological action, composition, stereochemical structure (when appropriate) and syntheses, paradoxically far from being eco-friendly. We discuss on future prospective that would combine sustainable chemical processes and synergistic effects promising for the development of natural, safe and efficient topical repellents.
Introduction
Mosquitoes represent a serious threat to public health as they are now considered as the deadliest animal in the world. They are powerful vectors spreading pathogens in human population. Hematophagous female mosquitoes belonging to the genera Aedes, Anopheles, and Culex can transmit disabling and deadly diseases. They infect humans with protozoan parasites causing malaria, oestridean parasites causing myasis, filarioidean parasites causing helminthiasis and viruses causing Dengue fever, Chikungunya, Japanese encephalitis, Sindbis fever, Rift Valley fever, West Nile fever, Yellow fever, and Zika (Amraoui et al., 2018). Mosquito-borne diseases outbreaks have soared over the last decades, exemplified by the 30 fold increase of Dengue fever over the last 30 years World Health Organization (WHO, 2015). The surge of mosquito-borne diseases outbreaks can be related to human behavior through climate change, deforestation, urbanization and globalization. The most striking example is the one of the Asian tiger mosquito – Aedes albopictus – the most invasive mosquito species in the world (Reinhold et al., 2018), which has spread from tropical forests in Southeast Asia to other tropical areas and more recently to temperate regions, as southern parts of Europe (Gasperi et al., 2012; ECDC, 2018), causing several reported autochthonous disease cases (Roiz et al., 2015; Venturi et al., 2017).
Unfortunately, no effective vaccine has been yet successfully developed against the above-mentioned mosquito-borne diseases except for Japanese encephalitis and Yellow fever (List of Vaccines | CDC, 2018). The most common control measure of vector natural populations is primarily based on the use of insecticides, but such a chemical control has some drawbacks, as for instance the increasing emergence of resistance to insecticides (Ranson et al., 2010) and the adverse effects on human health and environment. Physical protection with bed nets (Alonso et al., 1991) or long clothes is effective but their protection vastly depends on the mosquito species biting behavior (e.g., diurnal or nocturnal species) and obviously not always adjusted for outdoors activities. These reasons have brought the attention to the use of personal protection measures, as repellent formulations, as the most realistic and sensible approach to protect individuals. Spatial and contact repellents are often preferred and recommended by health authorities to prevent mosquitoes’ bites.
In the 1950’s, original plant-based repellents were replaced by synthetic repellents for their astonishing efficacy. The reference topical mosquito repellents currently commercialized in the European Union are the following synthetic molecules: N,N-diethyl-meta-toluamide (DEET) which is the gold standard of mosquito repellent, 3-(N-n-butyl-N-acetyl)-amino-propionic acid ethyl ester (IR3535), 3-phenoxybenzyl (1RS)-cis, trans-3-(2,2-dichlorovinyl)-2,2-dimethylcyclopropane carboxylate (permethrin), 2-(2-hydroxyethyl)-1-methylpropylstyrene 1-piperidine carboxylate (picaridin, also called KBR 3023, icaridin, or BayrepelTM) and para-menthane-3,8-diol (PMD).
Although being produced by chemical synthesis processes to be commercialized, IR3535, permethrin and PMD are bio-sourced while DEET and picaridin do not derive from natural compounds. The efficacy of DEET and picaridin is unbeatable – a 15% DEET solution provides 7–8 h of complete protection time against A. albopictus for example (Barnard and Xue, 2004). However, in the last decade several studies have brought to light the potential adverse effects on human health and environment. DEET inhibits the activity of acetylcholinesterase, a key enzyme of synaptic transmission, leading to neurotoxic effects in insects but also in humans (Corbel et al., 2009; Swale et al., 2014). DEET has also been reported to inhibit Na+ and K+ ion channels of mammalian cortical neurons and to behave as an allosteric modulator of M3 mAchR in humans inducing pro-angiogenic effects (Abd-Ella et al., 2015; Legeay et al., 2016). Moreover, all synthetic repellents can cause skin irritation; Picaridin has been reported to cause dermatitis in humans and hepatic toxicity in rats (Picaridin – A New Insect Repellent | The Medical Letter Inc., 2005). DEET and its derivatives also damage plastics and synthetic fibers (Diaz, 2016). Apart from presenting worrying signs of toxicity, clear hints of mosquito resistance to DEET have been evidenced lately. Genetic factors (a mutation leading to a non-response of the receptors involved in DEET recognition in insects) (Stanczyk et al., 2010) and non-genetic desensitization (Stanczyk et al., 2013) lead to a significant decrease in response to the repellent, which will undoubtedly cause dramatic consequences.
None of the non-bio-sourced repellents appears to be ideal for a long-term and safe use. In addition, the natural and environment-friendly criterions are of increasing interest due to a growing ecological awareness that redefines consumers’ expectations (Pohlit et al., 2011). We dedicated this review to the recent advances and developments of bio-sourced mosquito repellents using molecular tools of chemistry and bringing a new scale of understanding and analysis often restricted to biology or ecology.
In this review, we compare bio-sourced mosquito repellents that are allowed for commercialization within the European Union – IR3535, permethrin and PMD – and other natural active compounds not commercialized yet as such – methyl jasmonate, methyl anthranilate and fatty acids derived from glyceridic oils. We firstly expose their chemical composition, stereochemical structures, their mode of action, their repellent activity against mosquito and discuss about their potential impacts on human health and environment. Then, we present an overview of their synthesis routes and discuss about their controversial syntheses, which are not environment-friendly so far. Finally, we discuss about the potential of new formulations based on mixtures of different active compounds, which take into account the complex chemoreception system of mosquitoes.
IR3535 – 3-(N-N-Butyl-N-Acetyl)-Amino-Propionic Acid Ethyl Ester
IR3535 or Ethyl ButylAcetylAminoPropionate (EBAAP, EBAP, Merck 3535, OMS 3065) is an N-acyl β-aminoester. Its IUPAC designation is 3-(N-n-butyl-N-acetyl)-aminopropionic acid ethyl ester.
Repellent Activity of IR3535
IR3535 is an efficient topical repellent against reference mosquito species. It is as effective as DEET, the synthetic gold standard, in repelling Culex and Aedes species but may be less effective in repelling Anopheles species. A 20% formulation of IR3535 provides complete protection against Culex and Aedes mosquitoes for 7–10 h and for 3–4 h against Anopheles mosquitoes (Lupi et al., 2013).
Biological Mode of Action of IR3535
The molecular mode of action of IR3535, as well as most mosquito repellents, is getting more scientific interests but remains poorly documented (Sparks et al., 2018). Yet in 2011, Dickens and co-workers have identified two odorant receptors of Aedes aegypti, AaOR2 and AaOR8, involved in the recognition of attractive odorants (Bohbot et al., 2011). They tested the activity of IR3535 against these two receptors AaOR2 and AaOR8 in an AaOR/AaORco-expressing oocyte system. They found that IR3535 partially or totally inhibited the current response upon activation against AaOR2/ORco or AaOR8/ORco, respectively. In similar experiments, Leal and co-workers tested the activity of IR3535 against the receptor CquiOR136 of Culex quinquefasciatus in the CquiOR136/CquiORco-expressing oocyte system (Xu et al., 2014). They found that IR3535 activates the heterodimeric complex CquiOR136/CquiORco, in a dose-dependent manner and with the best activation constant among other repellents as DEET. Similarly CquiOR32 was found to be as well activated by IR3535 (Xu et al., 2018). These three studies suggest that IR3535 might target and modulate several odorant receptors within one species of mosquito, and according to the presence or absence of another odorant molecule. In another approach, focusing on gustatory receptor neurons of A. aegypti, Dickens and co-workers showed that gustatory receptors can be sensitive to IR3535 feeding, although the responding GRs remain to be identified (Sanford et al., 2013). Much systematic studies need to be performed to identify all the receptors targeted by IR3535 and other involved proteins in order to unveil its repellency mechanism.
Toxicity of IR3535
The toxicity of IR3535 to humans is still questionable since no extensive studies have been carried out since Klier and Kuhlow in 1976 (Nentwig, 2003). Oral and dermal toxicity were tested on laboratory animals and their values are only twice less harmful than DEET to rats. IR3535 causes serious eye irritation, permeates human skin after topical application (WHO, 2006; Specifications and Evaluations for Public Health Pesticides. Ethyl butylacetylaminopropionate.) and like DEET it may dissolve or damage synthetic materials (Doggett et al., 2018). However, a positive point is that this chemical might not be toxic to aquatic life and does not accumulate in environment as it is the case of other synthetic repellents (von Elert et al., 2016).
Syntheses of IR3535
The natural origin of IR3535 is controversial. It is not present in Nature but is classified as a natural repellent and considered as a biopesticide in the United States. The manufacturer Merck claims that IR3535 is inspired from nature (Discover the Insect Repellent IR3535® | Merck Global, 2019), while Islam et al. have classified IR3535 as a natural/synthetic repellent at the same time (Islam et al., 2017). Its chemical structure (Figure 1) is indeed inspired from β-alanine, an amino acid naturally occurring in two peptides carnosine and anserine, and in pantothenic acid (Patel et al., 2016). But this structural analogy with β-alanine causes confusion; the chemical synthesis of IR3535 brings clarity to the situation.
The synthesis of IR3535 is based on the one pot reaction sequence i) Michael addition of butylamine onto ethylacrylate, ii) acylation of secondary amine with acetyl chloride (Klier and Kuhlow, 1976; Jian et al., 2008; Scheme 1).
IR3535 does not derive from β-alanine but from ethylacrylate, butylamine and acetyl chloride, which are extremely hazardous, flammable or explosive, irritant, toxic and carcinogen (PubChem, 2019).
In conclusion, IR3535 presents a fragment of the natural β-alanine but does not derive from it, hence it should not be considered as a bio-sourced repellent and the developed chemical synthesis is far from reaching any eco-friendly criteria.
Permethrin – 3-Phenoxybenzyl (1RS)-cis, trans-3-(2,2-Dichlorovinyl)-2,2-Dimethylcyclopropanecarboxylate)
Permethrin is a synthetic pyrethroid insecticide that can also act as a contact repellent (Roberts et al., 1997). Its IUPAC designation is 3-Phenoxybenzyl (1RS)-cis,trans-3-(2,2-dichlorovinyl)-2,2-dimethylcyclopropane- carboxylate).
Always classified as a synthetic repellent, it is noteworthy that the chemical structure of permethrin is largely inspired from pyrethrins, which are naturally occurring in flowers of Chrysanthemum sp. (Figure 2).
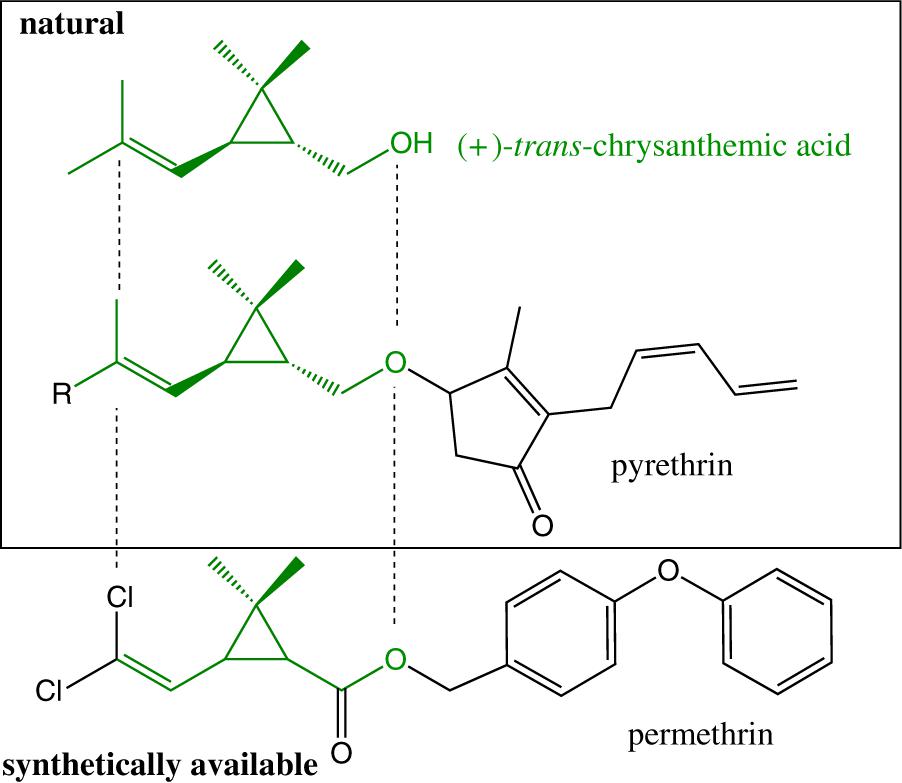
Figure 2. Structure similarity of permethrin, pyrethrins, and chrysanthemic acid (chrysantemic moiety colored in green).
Repellent Activity and Toxicity of Permethrin
Pyrethrins have been used for their insecticidal and insect-repellent properties for thousands of years. At low concentrations, they behave like repellents (Roberts et al., 1997); at higher doses, they act on the nervous system of insects (Narahashi, 2000); and at very high dose, they become toxic, even to humans by affecting the GABA neurotransmitter.
The chemical instability and the insufficient availability of pyrethrins have led to synthetic pyrethroids. The commercially used pyrethroids in mosquito control are permethrin, deltamethrin, resmethrin and sumithrin. Permethrin is the best-known synthetic pyrethroid. However, the use of permethrine as a topical repellent is limited due to a poor repellent efficacy: A. aegypti bites are delayed by 334 s for a 5% formulation of permethrin and by 7508 sec for a 50% formulation of DEET (Miot et al., 2008). Permethrin is mostly used as an insecticide instead. The insecticide action requires a direct contact with the mosquito, blocking the voltage-gated sodium channel in an activated state making the neuronal membranes persistently depolarized leading to mosquito paralysis and death (Raymond-Delpech et al., 2005; Field et al., 2017). As DEET, due to a lack of specificity to insect organisms, neurotoxic effects of permethrin were reported (Soderlund et al., 2002). Permethrin is more suited to be used on clothing and bed nets rather than being applied on the skin, although none cutaneous contact is difficult to be prevented (a 60 kg adult is exposed to 9.45 g of permethrin per day if sleeping under a bed net) (Ross et al., 2011; Duvallet and de Gentile, 2017). Apart from presenting clear signs of toxicity, evidence of mosquito resistance to permethrin, and other pyrethroids in general, has been reported for a decade (Moyes et al., 2017). More recently, it has been noticed that A. albopictus has evolved resistance to permethrin in territory invaded only 30 years ago (Pichler et al., 2018). In addition to being highly toxic to aquatic organisms and cold-blooded animals, permethrin is also known to be extremely toxic to bees (LD50 < 2 μg/bees) (Sanchez-Bayo and Goka, 2014; Piccolomini et al., 2018).
Syntheses of the Chrysanthemic Moiety
Rather different from the biosynthesis of the chrysanthemyl skeleton based on the coupling of two molecules of dimethylallyl pyrophosphate catalyzed by chrysanthemyl diphosphate synthase, two main strategies have been industrially developed (Scheme 2).
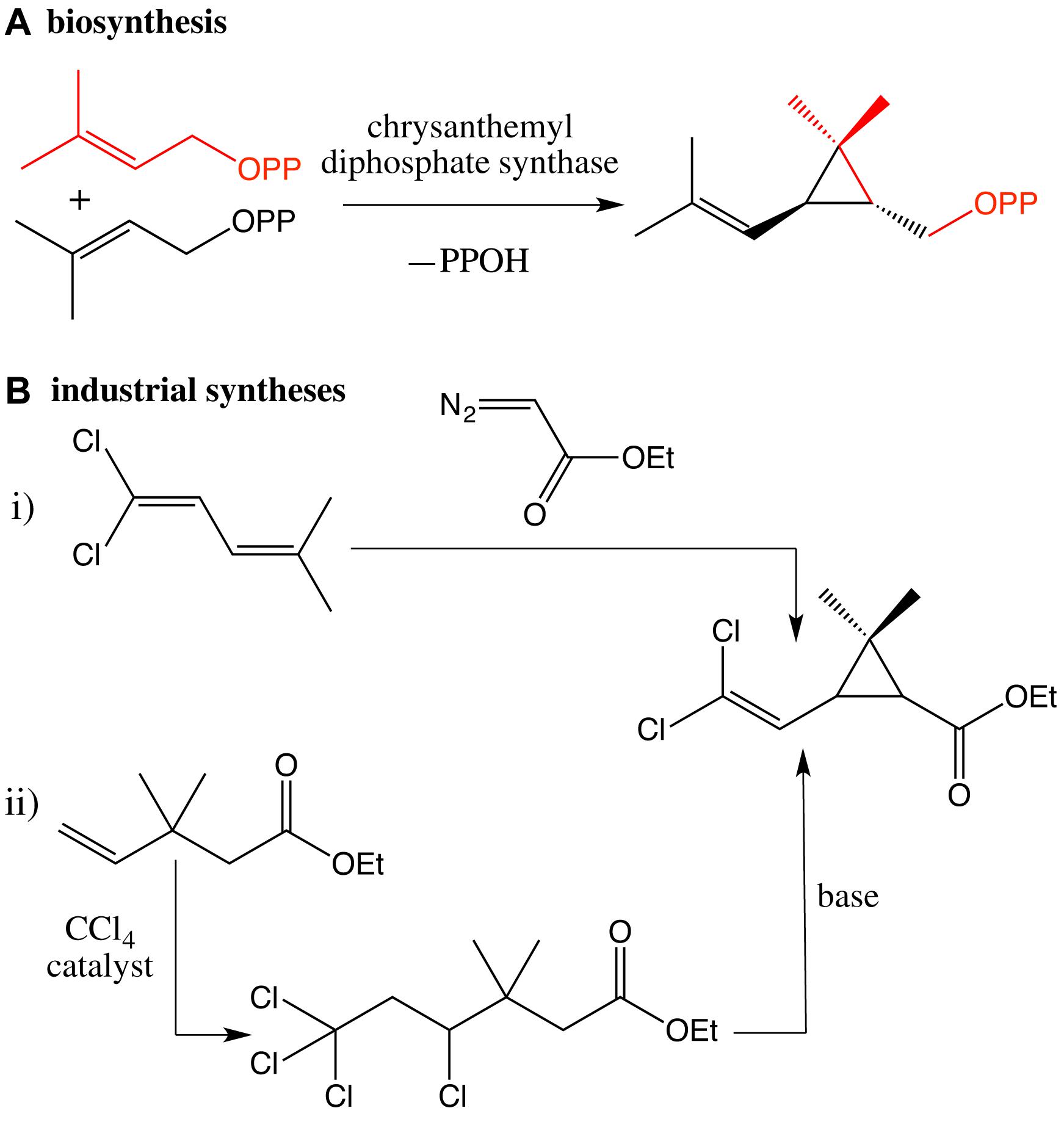
Scheme 2. (A) Key step of the biosynthetic pathway of chrysanthemic acid catalyzed by chrysanthemyl diphosphate synthase; (B) industrial syntheses (i,ii) of the chrysanthemic moiety.
The first one is based on the addition of an ethyldiazoacetate to 1,1-dichloro-4-methyl-1,3-pentadiene (Farkaš et al., 1959; Mizutani et al., 1975; Mori et al., 1978, 1980; O’Neil, 2013). The key reaction gives a mixture of cis and trans diastereomers of the chrysanthemic intermediate. The cis/trans ratio varies depending on the experimental conditions and can also vary with time due to differential rates of hydrolysis and photolysis. The cis- and trans-isomers can be separated from one another by selective crystallization. It is typically a mixture of (−) cis and (+) trans esters in a 40:60 ratio that is required for a protection against mosquito. Different ingenious improvements have been accomplished to induce the formation of the cis-isomer, which exhibits the best repulsive activity (Abdel-Magid and Bien, 2004).
The second strategy is based on the dehydrohalogenation of a 3,3-dimethyl-4,6,6,6-tetrachlorohexanoate via successive 1,2-elimination and 1,3-elimination in basic conditions (Itaya et al., 1977; Kondo et al., 1977). And the 3,3-dimethyl-4,4,6,6-tetrachlorohexanoate was prepared by the addition of tetrachloromethane onto ethyl 3,3-dimethyl-4-pentenoate, promoted by a radical initiator or by irradiation. The extension of this methodology to (2R)-1,1,1-trichloro-2-hydroxy-4-methyl-3-pentene and the subsequent formation of trichloromethyl bicyclic lactone allowed the synthesis of the key enantiomer (1R)-cis of the chrysanthemic intermediate (Kondo et al., 1980).
Although the chemical skeleton of permethrin is inspired from natural pyrethrins, the first presented strategies require the use of ethyl diazoacetate which is flammable, unstable, toxic and carcinogen. The second approach required carbon tetrachloride, which is hepatotoxic and can affect the central nervous system. A prolonged exposure could be fatal.
Methyl Jasmonate – Methyl {(3-oxo-2-[(2z)-2-penten-1-yl] Cyclopentyl}Acetate
Methyl jasmonate is a chiral cyclopentanone with two ring members: the first one is a methyl ester, the second carries a Z-pentenyl group. Its IUPAC designation is methyl {3-oxo-2-[(2Z)-2-penten-1-yl] cyclopentyl} acetate. Methyl jasmonate displays two chiral centers in positions and resulting in two pairs of enantiomers (1R,2S)/(1S,2R) and (1R/2R)/(1S,2S) (Figure 3).
Both cis-methyl epijasmonate (1R,2S) and trans-methyl jasmonate (1R,2R) are natural key hormones that play essential roles in plant developmental and defense pathways (Wasternack and Hause, 2013). Indeed a recent review stated the crucial roles of jasmonate, that shares common biosynthetic pathway with methyl-jasmonate, in plant defense against insects (Wang J. et al., 2019). Although trans-methyl jasmonate is more stable, the ratio cis/trans-methyl jasmonate varies greatly in plants, 20/80 in undamaged and 60/40 in damaged plant materials, suggesting non-redundant roles of both diastereoisomers (Tamogami et al., 2011).
Repellent Activity and Biological Mode of Action of Methyl Jasmonate
Among diverse applications [flavor and fragrance agent, promising anti-cancer drug (Cohen and Flescher, 2009)], methyl jasmonate appears to behave as a good repellent against nymphs of the tick species Ixodes ricinus (Garboui et al., 2007). The repellent efficacy of methyl jasmonate against mosquito species remains poorly documented. Yet Leal and co-workers found that methyl jasmonate targets the same OR (CquiOR136) as DEET and IR3535 in C. quinquefasciatus, and suggested that methyl jasmonate might be its natural ligand. As well, a similar study showed that methyl dihydrojasmonate, the saturated analog, demonstrated a strong repellency against C. quinquefasciatus and triggered a high odorant induced current response in the overexpressed CquiOR136/CquiORco Xenopus oocyte system (Zeng et al., 2018). However, no discrimination between the two natural diasteroisomers (1R,2S) and (1R,2R) versus mosquito repellency has been studied yet.
Syntheses of Methyl Jasmonate
Methyl jasmonate is present in extremely low quantities in plants (Jasminum grandiflorum, Rosmarinus officinalis) and red algae (Galium latifolium). Given its importance, many efforts have been made to synthetize methyl jasmonates (epi, ent-epi, cis/trans, racemic, enantiopur) and analogs such as dihydrojasmonates (Scheme 3). A great variety of strategies have been studied for the construction of the cyclic moiety: preparation of a functionalized bicycle (Sisido et al., 1969), preparation of a chloroindenone by cycloaddition (Chapuis et al., 2006), functionalization of a cyclopentanone into 2 then 1 position (Buchi and Egger, 1971; Näf and Decorzant, 1978; Kataoka et al., 1987), functionalization of a cyclopentanone into 1 then 2 position (Luo and Negishi, 1985; McDougal and Schaus, 2003; Ito et al., 2005). In any cases, the Z olefin part was built by Wittig reaction, whereas the methyl ester group was introduced by homologation, opening of a lactone, oxidative cleavage of a C = C bond or Michael addition. In each case, syntheses of racemic or enantiopur methyl jasmonates correspond to complex total syntheses (from 4 to 16 steps), and remains a challenge especially to reach eco-friendly conditions (Deau, 2011).
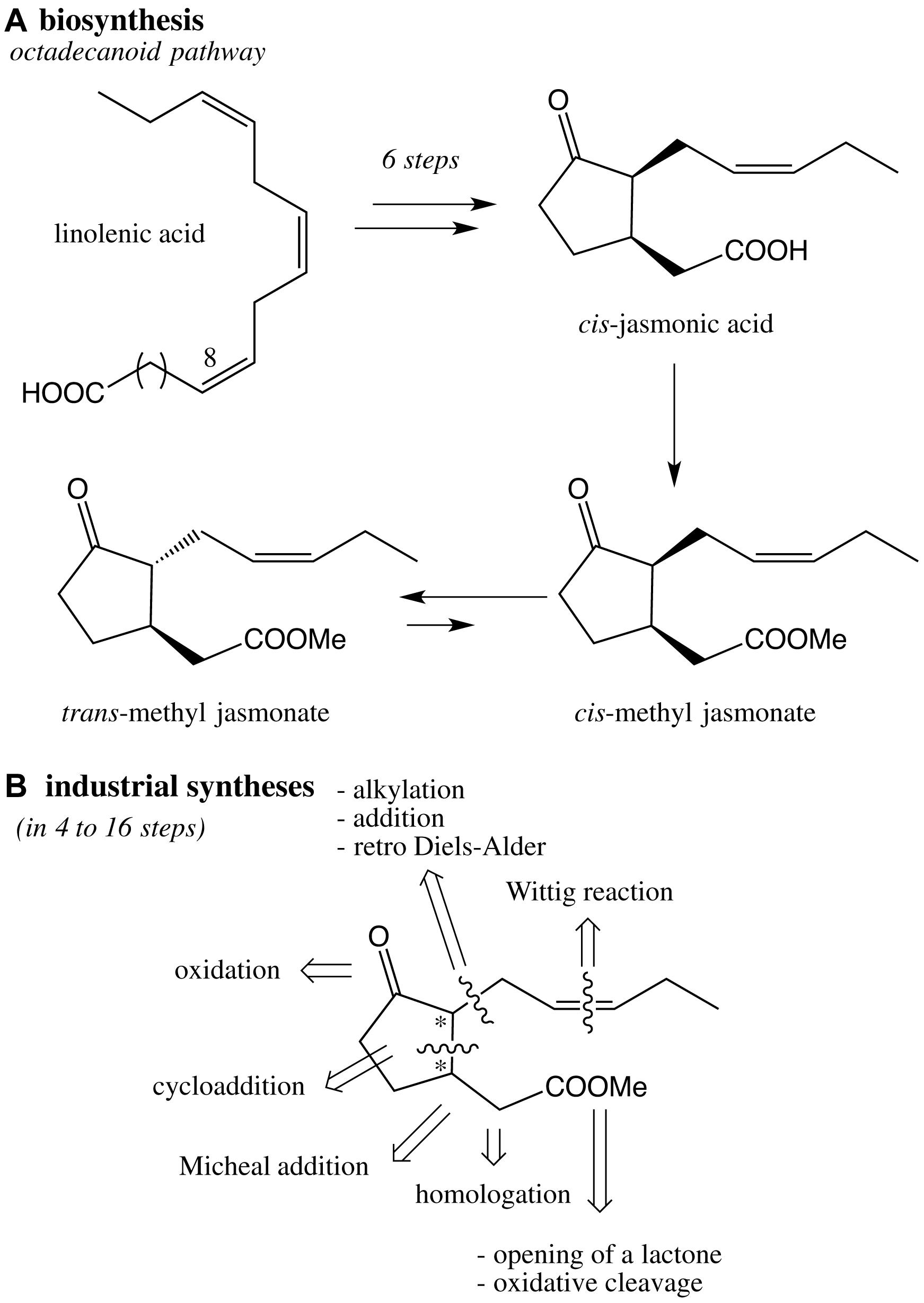
Scheme 3. (A) Key steps of the octadecanoid pathway of cis- and trans-methyl jasmonates in plants; (B) retrosynthesis based on industrial syntheses of methyl jasmonate.
Methyl Anthranilate – Methyl 2-Aminobenzoate
Methyl anthranilate is an amino ester comprising an aromatic backbone. Its IUPAC designation is methyl 2-aminobenzoate.
Methyl anthranilate is a natural product, present in small amounts in Washington concord grapes (Vitis labrusca) and in extracts of various plant species, such as neroli, bergamot, lemon, jasmine and mandarin. In plants, it might play a role in defense pathways since it was found to be formed in maize after herbivore damage (Köllner et al., 2010). It exhibits a pleasant fruit-like flavor and is used as a perfume, as a flavoring agent in drugs, candies, chewing gum, and soft drinks.
Repellent Activity of Methyl Anthranilate
Methyl anthranilate is extensively used as an agricultural bird repellent sprayed on crops, in landfills and airports. Recently, methyl anthranilate and analogs (ethyl anthranilate, butyl anthranilate, and methyl N,N-dimethyl anthranilate) were identified as potent repellents against Drosophila melanogaster in a computational structure-activity screen exhibiting similar skeleton as DEET (Figure 4; Kain et al., 2013). Methyl anthranilate, ethyl anthranilate and methyl N,N-dimethyl anthranilate have shown good repellent properties against A. aegypti. They presented similar activity as DEET in host-seeking experiments and a stronger oviposition deterrence than DEET (Afify et al., 2014). However, the biological action and targeted receptors of these repellents in mosquito are still unclear (Kain et al., 2013; Silbering et al., 2016).
Syntheses of Methyl Anthranilate
Commercial methyl anthranilate is produced by organic syntheses which largely differ from the biosynthesis pathway, which derives from shikimic acid and chorismic acid through the shikimate pathway (Wang and Luca, 2005; Scheme 4). Numerous synthetic pathways and manufacturing processes have been developed in which the challenge is the introduction of the amino group. The most common industrial processes are based on a Hoffmann-Type Rearrangement from phthalic anhydride (Gao, Lianjia, 2011), a key intermediate for the production of phthalate esters plasticizers, which are endocrine disruptors. Other classic strategies are based on the reaction sequence nitration/reduction (Min et al., 2016) and diazotation/reduction (Markiewicz et al., 2010), which requires sodium azide, a severe poison.
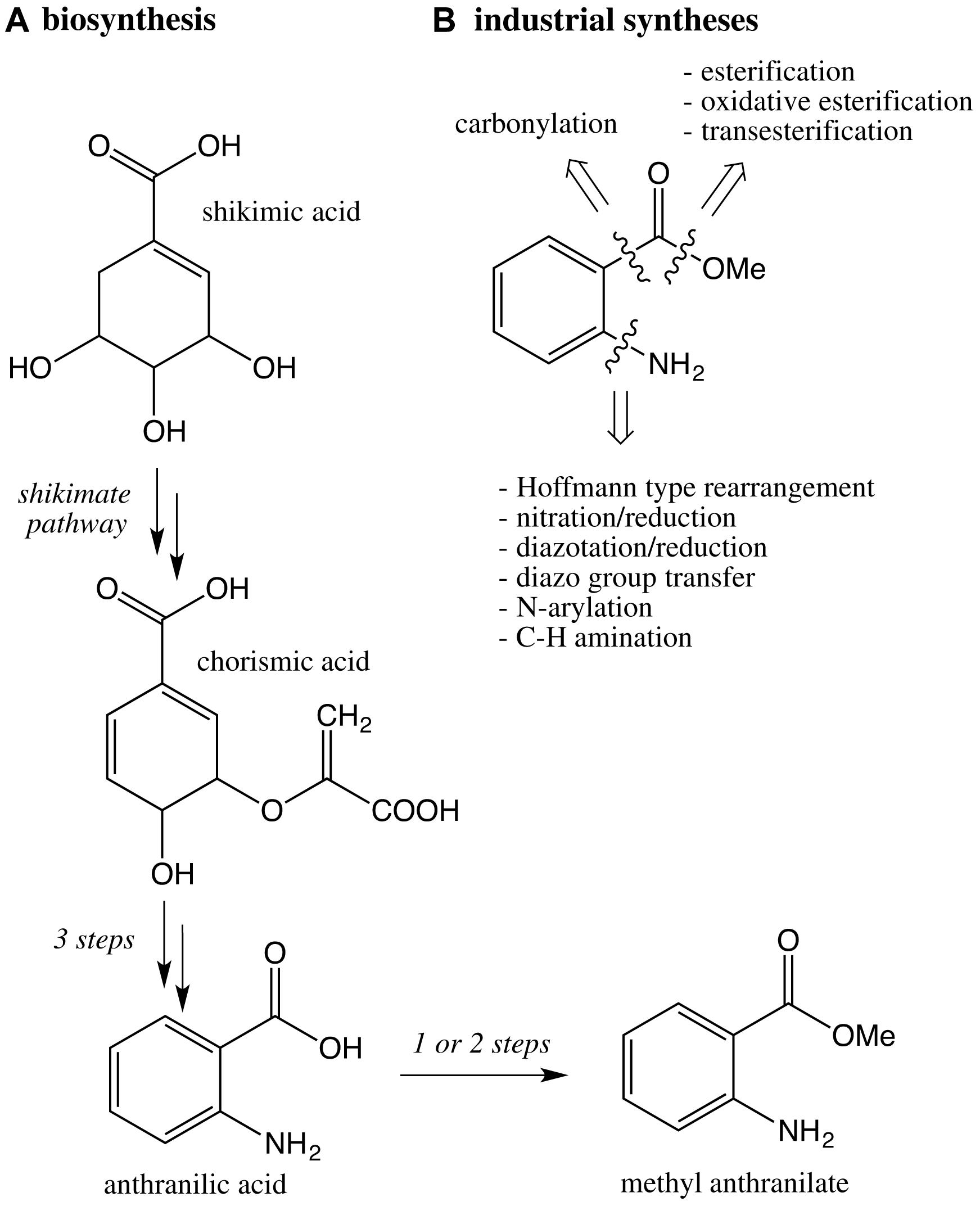
Scheme 4. (A) Key steps of the biosynthetic pathway of methyl anthranilate in plants; (B) retrosynthesis based on industrial syntheses of methyl anthranilate.
Various metal-catalyzed N-arylations have also been described (Xia and Taillefer, 2009; Siddegowda et al., 2012; Huang et al., 2017; Li et al., 2017). This strategy is elegant but combines an acute toxic solvent (DMF) and a hazardous catalyst [Cu(acac)2], which is suspected of damaging fertility and known to be toxic for aquatic ecosystem.
Recently, an original process has been established from an elegant C-H amination by the pyridinium radical cation (Ham et al., 2019). This methodology is of academic interest, but is not commercially scalable at any stage of the process. Moreover, the experimental conditions (CH3CN, CH2Cl2) raise concerns in terms of toxicity or carcinogenic activity.
Another methodology is the introduction of the carboxyl group via the ortho-carbonylation of aniline derivatives (Houlden et al., 2009) or the amidocarbonylation of functionalized aryl halides (Fairlamb et al., 2007).
The preparation of the methyl ester can be achieved by classic esterification (Cai et al., 2018; Frost et al., 2019), transesterification (Wang Y.-W. et al., 2019) or oxidative esterification of 2-amino benzylalcohol (Powell and Stahl, 2013; Salam et al., 2014; Stahl et al., 2015; Hu and Li, 2017), and of 2-aminobenzaldehyde (Sarkar and Khan, 2015).
PMD – 2-(2-hydroxypropan-2-yl)-5-Methyl Cyclohexan-1-Ol
Para-Menthane-3,8-Diol is a 10-Carbon cyclic terpene diol whose structure is close to menthol andα-terpineol (Figure 5). Its IUPAC designation is 2-(2-hydroxypropan-2-yl)-5-methyl cyclohexan-1-ol.
Repellent Activity of PMD
Para-menthane-3,8-diol is considered as the natural and safe topical repellent alternative to DEET (Diaz, 2016). Several studies have demonstrated the high repellent activity and longevity of PMD against Aedes, Anopheles, and Culex genera (Barnard and Xue, 2004; Carroll and Loye, 2006), similar to DEET (Carroll and Loye, 2006; Goodyer et al., 2010; Drapeau et al., 2011), while having low toxicity – except eye irritation which is observed for all non-toxic natural active ingredients – and no adverse effect reported (Reifenrath et al., 2009). For example, a 26% PMD formulation provided complete protection for 7–8 h against A. albopictus and Ochlerotatus triseriatus and for 3 h against Culex nigripalpus, which is comparable to a 7% DEET formulation (Barnard and Xue, 2004). Moreover, recent studies have shown that the time of action of PMD-based formulation could be increased by the addition of vanillin or similar aromatic aldehydes (Davies and Moses, 2017). The addition of 15% of vanillin to a solution of 20% of PMD significantly changes the time of protection from 5 to 9 h of complete protection, while vanillin alone does not present any repellent activity. The explanation of such behavior is still hypothetic. Vanillin as an aromatic aldehyde could react with the diol moiety of PMD and form acetal derivatives, which could serve as a reservoir of PMD for prolonging the repulsive effect of the mixture. However, this hypothesis needs to be carefully considered as a pioneer study showed that the addition of vanillin to other repellents as DEET – that cannot covalently react with vanillin – increased systematically their time of action although not in the same proportion (Khan et al., 1975).
Biological Mode of Action of PMD
The olfactory receptors targeted by PMD have not been studied in details yet. However, Dickens and co-workers found that PMD partially inhibits, and clearly less than IR3535, the odorant-induced activation of AaOR2 and AaOR8 in A. aegypti (Bohbot et al., 2011); while Leal and co-workers found that PMD activates CquiOR136 and CquiOR32 in C. quinquefasciatus in the absence of an agonist (Xu et al., 2014, 2018). Apart from the sensory receptors, another class of proteins, the odorant binding proteins (OBPs), play an important role in the sensory system of mosquitoes (Leal and Leal, 2015). Among various functions, they transport hydrophobic odorant through the aqueous sensillar lymph to receptors embedded on dendritic membranes of olfactory receptor neurons (Sparks et al., 2018; Venthur and Zhou, 2018). They constitute the first recognition step for an odorant/gustative compound before reaching receptors, where the second recognition step takes place. Leal and co-workers have identified two OBP, CquiOBP2 and CquiOBP5, in C. quinquefasciatus that bind PMD with micromolar affinities (Yin et al., 2015). Interestingly, these two OBPs bind PMD with a 10 fold affinity difference, suggesting a potential selectivity toward PMD.
Stereoisomers of PMD
Para-menthane-3,8-diol presents three asymmetric centres and has theoretically eight stereoisomers. However, only mixture of the four stereoisomers, C1-C2-cis/C2-C5-trans-PMD and C1-C2-trans/C2-C5-trans-PMD, occur in nature (Figure 6). The repellent activity of each four natural stereoisomers was individually tested but gave inconsistent results. Whereas Barasa et al. (2002) have established that the four natural stereomers have similar repellent activity against Anopheles gambiae, Lett and Kraus (1992) have found that the cis isomer was more active than the trans isomer as an insect repellent.
Para-menthane-3,8-diol is naturally present in plants, which are rich in citronellal. PMD was found in essential oils (EO) of Citrus hystrix (Rutaceae), Corymbia citriodora (Myrtaceae), Cymbopogon winterianus (Poaceae), and Melissa officinalis (Lamiaceae). Contrary to citronellal, natural PMD is present in trace amounts in some EOs. The EO of C. citriodora leaves has the highest PMD content, about 0.7% [0.5% of (+)-PMD, 0.2% of (−)-PMD]. Interestingly, Degani et al. (2016) showed that the EO of C. citriodora leaves from plants grown under shade had higher levels of PMD, about 5% [4%(+)-PMD, 1%(−)-PMD].
It is clear that the hydrodistillation of leaves produces very low quantities of PMD; the development of natural PMD production cannot meet market demand. That is why the majority of the PMD used in repellents is derived from synthetic processes.
Syntheses of PMD
The biosynthesis of PMD has not been reported yet. However, one can assume that a hypothetical biosynthesis of PMD in leaves rich in citronellal consists of the ene-carbonyl reaction of citronellal followed by the addition of H2O onto the intermediate isopulegol (Scheme 5).
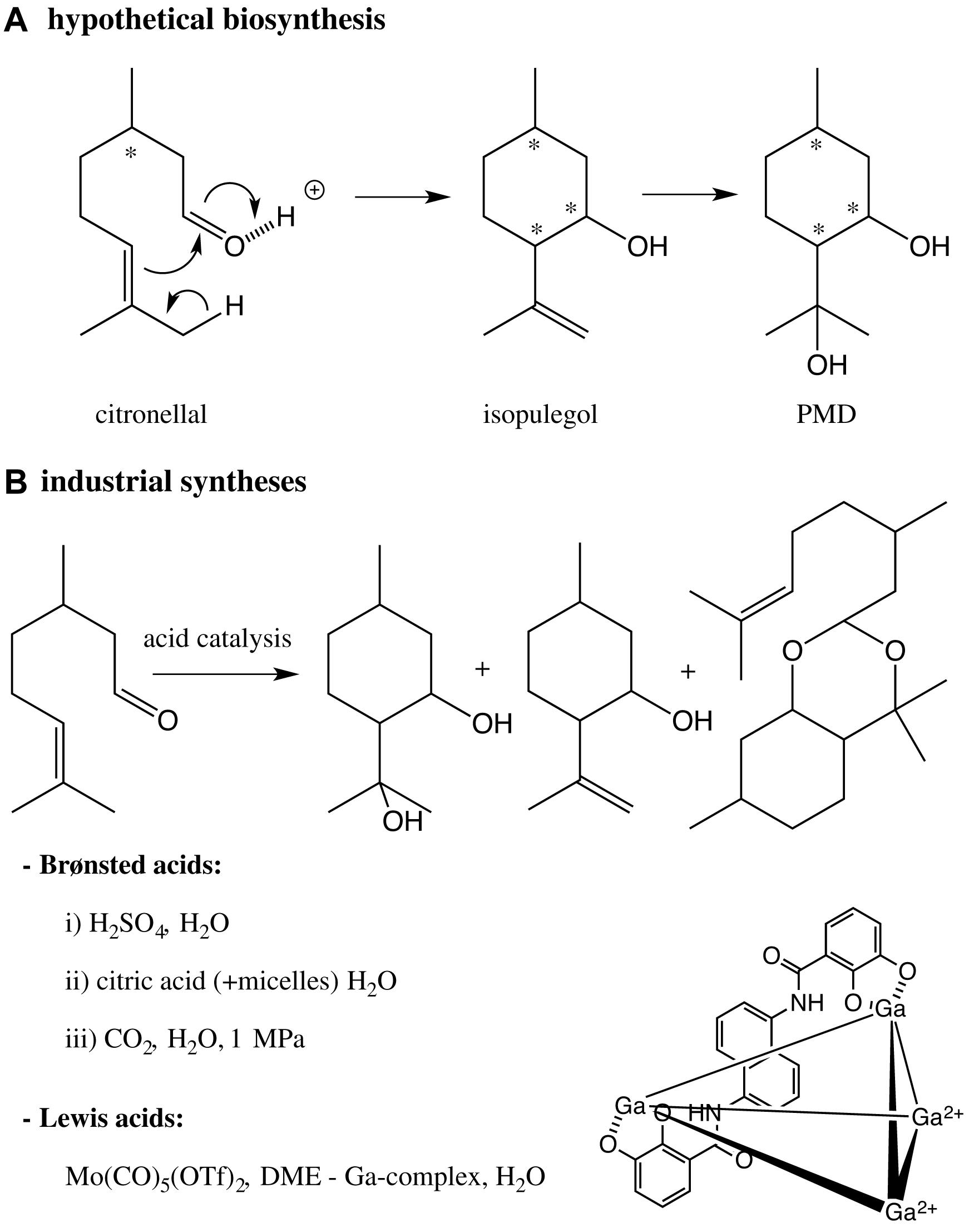
Scheme 5. (A) Hypothetical biosynthesis of PMD in plants enriched in citronellal; (B) bio-inspired industrial syntheses of PMD catalyzed by Brønsted or Lewis acids. * asymmetric centers.
Most of the industrial syntheses of PMD bio-mimic this reaction sequence and are catalyzed by Brønsted acids and more rarely Lewis acids. After the pioneering works of Zimmermann (Zimmerman and English, 1953), Takasago’s group (Kenmochi et al., 1999; Yuasa et al., 2000) has produced intense efforts to prepare a scalable preparation of PMD catalyzed by aqueous sulfuric acid. In the three studies, the syntheses led to a mixture of PMD, isopulegol, citronellal and an acetal (p-menthane diol citronellal acetal); and led to a mixture of stereosiomers of PMD. Following the work of Lett and Kraus (1992), the experimental conditions have been extensively optimized to favor the cis isomer [cristallisation at low temperatures (Shiroyama et al., 2001; Komatsuki et al., 2013), addition of surfactants (Clark et al., 1984; Dell, 2010, 2013) or phase transfert agents (Xuedong, 2013)].
Recently, greener processes have been described to synthesize PMD but not always at an industrial level. The reaction catalyzed by aqueous sulfuric acid was adapted to continuous flow and led to a modest yield (54%) despite high temperature (85°C–145°C) (Zeelie et al., 2009). Citric acid was used as an alternative natural catalyst (Schöftner, 2015), but led to modest cis/trans ratios (48/52-55/45). Micelles were also added to the citric acid catalyzed reaction and decreased its acidity (Clark et al., 1984). Although these methods using citric acid seem to be more environment-friendly, they require systematic work-up processes, neutralization and extraction, which generate unsatisfactory Sheldon factors (mass ratio of waste to desired product).
Another eco-friendly strategy consisted of generating an acidic solution, pH = 3.6, by the addition of CO2 into the aqueous reaction mixture. However, a pressure of 1 MPa was required (Cheng et al., 2009), which limits the large-scale development of this synthesis, as such high pressure represents an industrial risk.
The synthesis of PMD catalyzed by Lewis acid was less studied, because of less efficient and slow reactions. Kocovsky et al. used Mo(CO)5(OTf)2 as a Lewis catalyst in DME and obtained 80% of the cis stereoisomers after 48 h (Kočovský et al., 1999). Although this high stereoselectivity, the preparation of the catalytic complex was delicate, hazardous, expensive and the solvent toxic. A polyanionic supramolecular gallium-based assembly was used for driving protonation at the aldehyde oxygen of citronellal and a subsequent cyclization into PMD. Although this complex worked in water at moderate temperatures and at pH = 3.2, 10 mol% Ga and 28 h at 60°C were required to promote the reaction (Hart-Cooper et al., 2012), making the process difficult for an industrial development.
Although the syntheses PMD are bio-inspired, the indiscriminate “natural” classification assigned to PMD is debatable as it is produced by chemical syntheses, far from being environmental-friendly.
PMD in Essential Oils (EOs)
Syntheses of PMD Within EOs
In a context of attractiveness to natural products, the synthesis of PMD catalyzed by Brønsted acids can be performed using directly EOs enriched in citronellal. The EO of C. citriodora, containing a range of 73–80% of citronellal has been the most studied. Two strategies were described to promote the transformation of citronellal within the EO of C. citriodora into PDM (Figure 7). Xuedong added a mixture of 0.1–0.2% brominated polystyrene resin, tributylamine (acute toxicity) and sulfuric acid (0.3%) to the EO (Xuedong, 2013) while Drapeau et al. added citric acid to the EO (Drapeau et al., 2011). Although the second strategy is eco-friendlier, a subsequent neutralization step due to the solubility of citric acid in PMD was required and induced the formation of wastes (extraction solvent, inorganic salts) which presents a negative environmental impact (Wilson, 2007).
The current appellation of repellents containing PMD made from EO of C. citriodora can cause confusions (Citriodiol®, PMDRBO, “oil of lemon eucalyptus,” “Eucalyptus citriodora oil, hydrated, cyclized”). These products are considered and presented as “natural” but have gone through a chemical transformation. However, a substance is considered as “natural” when it is obtained from materials of plant, animal, microbiological or mineral origin by natural processes. This questions the natural status that could be attributed to modified or PMD enriched EO (Yuasa et al., 2000). Repellents containing PMD synthesized from C. citriodora EO do not meet the above-mentioned requirements and cannot be considered as “natural.” Besides, it is necessary to clearly distinguish PMD enriched EOs that offer a long-term protection from EOs with PMD naturally occurring in negligible amounts (<1%) whose repellent effect is much shorter.
Repellent Activity of PMD Within EOs
Comparison between the repellent activity of PMD by itself and mixed with an EO led to interesting results. Drapeau et al. (2011) showed that an EO from C. citriodora containing 13% of PMD has a repellent activity 1.5 times longer than a 13% solution of pure PMD produced by Takasago company and 10 times longer than the non-modified parent EO from C. citriodora. Three hypotheses can be raised here to explain the increased repellent activity of a solution of PMD within an EO versus a solution of pure PMD. The EO could modify the physicochemical properties of a solution of PMD and slower the evaporation rate of PMD. Thermogravimetric analyses were performed but could not support this hypothesis, as PMD within C. citriodora EO has the same vapor pressure as pure PMD (Drapeau et al., 2011). A second hypothesis is that PMD within C. citriodora EO could be partially trapped as the acetal described in Scheme 5 and slowly releases it upon time. However, this hypothesis is not verified, as the quantity of this acetal is negligible in comparison to the quantity of PMD within the EO (Drapeau et al., 2011). A third hypothesis is that mixing PMD with an EO could have a higher repellent activity than pure PMD. However, the experiment performed to assess the repellent activity was conducted by comparing the duration of repellency and not by evaluating the absolute repellency at a single time. It is therefore not possible to conclude accordingly, but such additive/synergistic effects should be specifically addressed.
The capacity to boost the repulsive activity against mosquitoes by mixing a combination of natural compounds deserves special attention.
The Synergistic Effect of Blends
Repellent Activity of EOs Linked to the Sensory System of Mosquitoes
Rare studies have focused on mixing active compounds of repellents but have clearly evaluated their subsequent repellent activity against mosquitoes (Pohlit et al., 2011; Islam et al., 2017). Odalo et al. (2005) studied the repellent activities against Anopheles gambiae of EOs from six plant species growing in Kenya, their major constituents and synthetic EOs made from mixing these major constituents. The major constituents of the EOs were tested individually and showed a relatively high repellent activity, but not as high as the parent EOs. Interestingly, the synthetic EOs showed either a comparable or higher repellent activity than the corresponding parent EOs. These results suggest additive or synergistic effects of the interaction of blended EO constituents (and/or also suppressive repellency diminishing effects of non-active components).
Taking into account the complexity of the sensory system and the vast repertoire of sensory receptor proteins of a mosquito species (Sparks et al., 2018), this observation is not surprising. An optimal repellent activity could be obtained with an adapted mixture of components. In insects, besides few exceptions, the same molecule can activate different sensory receptors, and the same receptor can be activated by different molecules (Andersson et al., 2015). Thus, increasing the number of active molecules present in a blend of repellent compounds could potentially lead to the activation of several receptors, triggering olfactory sensory neurons hosting these receptors to concurrently send multiple signals to higher brain centres of the insect and potentially synergizing the overall repellent effect. Besides, using a mixture of repellent molecules could as well slow down the appearance of resistance toward a given repellent formulation as loss-of-function mutations should take place on different sensory genes at the same time. Nonetheless, these two ideas remain to be tested.
Potential Toxicity of EOs
In this context, the chemical diversity of EOs composition should be an advantage (Pohlit et al., 2011). The repellent action against A. albopictus of 14 Lamiaceae-derived EOs and 24 of their terpenes was recently assessed (Giatropoulos et al., 2018). Origanum mantzuranum and Satureja thymbra (Lamiaceae) EOs had a high repellency against A. albopictus, due to their high content in carvacrol and thymol. These terpenes prevented A. albopictus landings on the skin to a greater extent than DEET. However, although their efficacy and their natural origin, the utilization of natural EOs as topical mosquito repellent should be carefully considered. Some of their active compounds, mainly terpenes, present adverse effects even at the low concentrations they are found in EOs (Llana-Ruiz-Cabello et al., 2014). Thymol can cause eye and skin damages, has led to skin irritation and sensitization, and is considered to be between moderately and very toxic (Gosselin et al., 1976; Salehi et al., 2018). Carvacrol provokes skin irritation, has been classified as very toxic to humans with an oral lethal dose of 50 mg/kg and showed moderate cytotoxic effects in HeLa cells (Gosselin et al., 1976; Opdyke, 1979; Sharifi-Rad et al., 2018). Apart from these two specific compounds, the major components of EOs used in mosquito repellents, that are citronellal, linalool, eugenol and its derivatives, also raised health concerns (Pohlit et al., 2011). Citronellol causes serious eye and skin irritation while linalool and eugenol are classified as allergenic substances (Restrictions and Allergens | Oxford Biosciences, 2003); eugenol and its derivatives are also classified as reasonably recognized to be human carcinogens (Strickman et al., 2009; National Toxicology Program, 2016 14th Report on Carcinogens).
Using EOs as a repellent, or adding active molecules to trigger a synergistic effect to it, is not without risk, since some EOs contain potentially hazardous molecules for health. Pinpointing the compounds detected by mosquitoes from a given formulation, and hence those that are not detected, would help to reduce the number of molecules to be used and get rid of the unwanted side effects of non-detected compounds. This knowledge would help to design mixtures of natural compounds with an optimized repellency and decreasing considerably their toxicity.
Repellent Activity of Fatty Acids Derived From Glyceridic Oil
Glyceridic oils, obtained by pressing fruits of plants, are widely used as carrier of EO components in mosquito repellent formulations. However, numerous glyceridic oils – castor, mustard, neem, margosa, olive, and soybean oils for example – present as stand-alone a repellent activity against mosquitoes (Pohlit et al., 2011). They have been tested as topical repellents and/or in burned sticks and provided mixed responses. An interesting example is the 2% soybean oil formulation, which exhibited repellency comparable to a 15% DEET solution against A. albopictus, C. Nigripalpus, and Aedes triseriatus (Barnard and Xue, 2004).
A few studies have focused on identifying the active compounds within glyceridic oils, which are constituted of a mixture of glycerides (Figure 8).
However, it has been known for some time that mosquitoes are sensitive to free long-chain fatty acids (Hwang et al., 1984). More recently, Zhu et al. (2018) studied the repellent activity of a glyceridic oil, the coconut oil and its hydrolyzed products mixed and separately, free fatty acids. While the coconut oil itself does not present any repellent activity, the mixture of free fatty acids and some of them individually present an impressive and long-lasting repellency, better than DEET, against blood-sucking insects. Interestingly, the authors noted that the mixture of free fatty acids has a better repellent activity than any of the free fatty acids alone, suggesting a synergistic effect. However, this effect was studied extensively only on stable flies and would be interesting to extend to mosquitoes, which already showed a higher sensitivity to the mixture of free fatty acids compared to DEET at similar concentrations.
Conclusion
Mosquito repellent formulations are facing new societal challenges that demand a high repellent activity of sustainable and natural products. Bio-sourced mosquito repellents represent an interesting and alternative tool of protection against rising vector-born diseases in the context of globalization. The bio-sourced repellents that were presented in this review, IR3535, pyrethrin and its derivatives, methyl jasmonate, methyl anthranilate and PMD, show a topical repellent activity similar to the best synthetic repellents as DEET and picaridine. However, the reagents and processes used in the synthetic routes leading to bio-sourced repellents are far from respecting a sustainable chemistry. Their industrial productions are clearly different from their biosynthesis pathways and are often based on reaction conditions that do not meet the REACH regulation. Their natural origin can be fully artificial as their carbon backbones present a natural moiety but do not synthetically derived from natural compounds. Much effort remains to be realized for developing mosquito repellents that can be certified as natural.
Although the toxicity of some components should be carefully addressed, the utilization of Essential Oils as mosquito repellents has shown renewed emphasis. The recent observation of additive/synergistic effects in EOs and in glyceridic oils, in which mixture of active compounds give a higher repellent activity than isolated active compounds, could become a potential solution. It would help the design of mixtures of natural, active and non-toxic compounds. PMD generated from citronellal within the EO of is the most striking example so far.
The recent discoveries on mosquito chemoreception should be considered to deepen this new approach of designing repellent mixtures. However, the current partial understanding of the complex chemoreception and the subsequent neural responses of mosquitoes restrict the design of new and efficient bio-sourced repellents. Multidisciplinary research combining insect neurobiology and green chemistry is crucial to develop highly efficacious repellents based on combination of nature-derived compounds.
Author Contributions
CG was the principal investigator of the review. DC was responsible of the biological part. FP and AM were responsible of the bibliography.
Funding
This work was supported by CNRS.
Conflict of Interest
The authors declare that the research was conducted in the absence of any commercial or financial relationships that could be construed as a potential conflict of interest.
Acknowledgments
The authors would like to thank the French National Center for Scientific Research (CNRS) for financial support. Sci-GuidEdit is acknowledged for writing assistance and proofreading the manuscript.
References
Abd-Ella, A., Stankiewicz, M., Mikulska, K., Nowak, W., Pennetier, C., Goulu, M., et al. (2015). The repellent DEET potentiates carbamate effects via insect muscarinic receptor interactions: an alternative strategy to control insect Vector-Borne diseases. PLoS One 10:e0126406. doi: 10.1371/journal.pone.0126406
Abdel-Magid, A. F., and Bien, J. (2004). Organometallics in Process Chemistry. Berlin: Springer Science & Business Media.
Afify, A., Horlacher, B., Roller, J., and Galizia, C. G. (2014). Different repellents for Aedes aegypti against Blood-Feeding and Oviposition. PLoS One 9:e103765. doi: 10.1371/journal.pone.0103765
Alonso, P. L., Lindsay, S. W., Armstrong, J. R. M., de Francisco, A., Shenton, F. C., Greenwood, B. M., et al. (1991). The effect of insecticide-treated bed nets on mortality of Gambian children. Lancet 337, 1499–1502. doi: 10.1016/0140-6736(91)93194-E
Amraoui, F., Pain, A., Piorkowski, G., Vazeille, M., Couto-Lima, D., de Lamballerie, X., et al. (2018). Experimental adaptation of the yellow fever virus to the mosquito aedes albopictus and potential risk of urban epidemics in Brazil. South America. Sci. Rep. 8:14337. doi: 10.1038/s41598-018-32198-32194
Andersson, M. N., Löfstedt, C., and Newcomb, R. D. (2015). Insect olfaction and the evolution of receptor tuning. Front. Ecol. Evol. 3:53. doi: 10.3389/fevo.2015.00053
Barasa, S. S., Ndiege, I. O., Lwande, W., and Hassanali, A. (2002). Repellent activities of stereoisomers of p -menthane-3,8-diols against Anopheles gambiae (diptera: culicidae). J. Med. Entomol. 39, 736–741. doi: 10.1603/0022-2585-39.5.736
Barnard, D. R., and Xue, R.-D. (2004). Laboratory evaluation of mosquito repellents against Aedes albopictus, Culex nigripalpus, and Ochierotatus triseriatus (Diptera: Culicidae). J. Med. Entomol. 41, 726–730. doi: 10.1603/0022-2585-41.4.726
Bohbot, J. D., Fu, L., Le, T. C., Chauhan, K. R., Cantrell, C. L., and Dickens, J. C. (2011). Multiple activities of insect repellents on odorant receptors in mosquitoes. Med. Vet. Entomol. 25, 436–444. doi: 10.1111/j.1365-2915.2011.00949.x
Buchi, G., and Egger, B. (1971). New synthesis of cyclopentenones. Methyl jasmonate and jasmone. J. Org. Chem. 36, 2021–2023. doi: 10.1021/jo00813a045
Cai, Z., Li, S., Gao, Y., Fu, L., and Li, G. (2018). Weak, bidentate chelating group assisted cross-coupling of C(sp3)–H bonds in aliphatic acid derivatives with aryltrifluoroborates. Chem. Commun. 54, 12766–12769. doi: 10.1039/C8CC07481J
Carroll, S. P., and Loye, J. (2006). PMD, a registered botanical mosquito repellent with deet-like efficacy. J. Am. Mosq. Control Assoc. 22, 507–514. doi: 10.2987/8756-971x(2006)22%5B507:parbmr%5D2.0.co;2
CDC, (2018). List of Vaccines. Available at: https://www.cdc.gov/vaccines/vpd/vaccines-list.html (accessed August, 2019).
Chapuis, C., Cantatore, C., and de Saint Laumer, J.-Y. (2006). An expeditious synthesis of methyl jasmonate. Helvetica Chim. Acta 89, 1258–1264. doi: 10.1002/hlca.200690124
Cheng, H., Meng, X., Liu, R., Hao, Y., Yu, Y., Cai, S., et al. (2009). Cyclization of citronellal to p-menthane-3,8-diols in water and carbon dioxide. Green Chem. 11, 1227–1231. doi: 10.1039/B823297K
Clark, B. C., Chamblee, T. S., and Iacobucci, G. A. (1984). Acid-catalyzed cyclization of terpenoids in a micellar system. Selectivity and rate enhancement in the cyclization of citronellal. J. Org. Chem. 49, 4557–4559. doi: 10.1021/jo00197a054
Cohen, S., and Flescher, E. (2009). Methyl jasmonate: a plant stress hormone as an anti-cancer drug. Phytochemistry 70, 1600–1609. doi: 10.1016/j.phytochem.2009.06.007
Corbel, V., Stankiewicz, M., Pennetier, C., Fournier, D., Stojan, J., Girard, E., et al. (2009). Evidence for inhibition of cholinesterases in insect and mammalian nervous systems by the insect repellent deet. BMC Biol 7:47. doi: 10.1186/1741-7007-7-47
Davies, J. H., and Moses, J. (2017). Insect Repellent Composition and Method of Use. Available at: https://patents.google.com/patent/WO2017081445A1/en (accessed December 10, 2018).
Deau, E. (2011). Synthèse Asymétrique de l’épi-Jasmonate de Méthyle et de son Énantiomère (ent-épi-Jasmonate de Méthyle) par voie Chimique et Enzymatique. Available at: https://tel.archives-ouvertes.fr/tel-00760982/document (accessed April 29, 2019).
Degani, A. V., Dudai, N., Bechar, A., and Vaknin, Y. (2016). Shade effects on leaf production and essential oil content and composition of the novel herb Eucalyptus citriodora hook. J. Essential Oil Bear. Plants 19, 410–420. doi: 10.1080/0972060X.2014.890080
Dell, I. T. (2010). Composition Containing p-Menthane-3, 8-Diol and Its Use as Insect Repellant. Available at: https://patents.google.com/patent/US20100278755A1/en?oq=US20100278755A1 (accessed April 30, 2019).
Dell, I. T. (2013). Composition Contenant du p-Menthane-3, 8-Diol et Son Utilisation Comme Répulsif à Insectes. Available at: https://patents.google.com/patent/EP2638802A1/fr (accessed April 30, 2019).
Diaz, J. H. (2016). Chemical and plant-based insect repellents: efficacy, safety, and toxicity. Wilderness Environ. Med. 27, 153–163. doi: 10.1016/j.wem.2015.11.007
Doggett, S. L., Miller, D. M., and Lee, C. (2018). Advances in the Biology and Management of Modern Bed Bugs. Hoboken, NJ: John Wiley & Sons Ltd, doi: 10.1002/9781119171539
Drapeau, J., Rossano, M., Touraud, D., Obermayr, U., Geier, M., Rose, A., et al. (2011). Green synthesis of para-Menthane-3,8-diol from Eucalyptus citriodora: application for repellent products. Comptes Rendus Chimie 14, 629–635. doi: 10.1016/j.crci.2011.02.008
Duvallet, G., and de Gentile, L. eds (2017). Protection Personnelle Antivectorielle. Marseille: IRD Éditions.
ECDC (2018). Aedes Albopictus. Current Known Distribution: June 2018 European Centre for Disease Prevention and Control. Available at: http://ecdc.europa.eu/en/publications-data/aedes-albopictus-current-known-distribution-june-2018 (accessed September 22, 2018).
Fairlamb, I. J. S., Grant, S., McCormack, P., and Whittall, J. (2007). Alkoxy- and amidocarbonylation of functionalised aryl and heteroaryl halides catalysed by a Bedford palladacycle and dppf: a comparison with the primary Pd(II) precursors (PhCN)2PdCl2 and Pd(OAc)2. Dalton Trans. 0, 859–865. doi: 10.1039/B615874A
Farkaš, J., Kouøím, P., and Šorm, F. (1959). Relation between chemical structure and insecticidal activity in pyrethroid compounds. I. An analogue of chrysanthemic acid containing chlorine in the side chain. Collect. Czech. Chem. Commun. 24, 2230–2236. doi: 10.1135/cccc19592230
Field, L. M., Emyr Davies, T. G., O’Reilly, A. O., Williamson, M. S., and Wallace, B. A. (2017). Voltage-gated sodium channels as targets for pyrethroid insecticides. Eur. Biophys. J. 46, 675–679. doi: 10.1007/s00249-016-1195-1191
Frost, G. B., Mittelstaedt, M. N., and Douglas, C. J. (2019). Chemoselectivity for alkene cleavage by palladium-catalyzed intramolecular diazo group transfer from Azide to Alkene. Chem. A Eur. J. 25, 1727–1732. doi: 10.1002/chem.201805904
Garboui, S. S., Jaenson, T. G. T., Borg-Karlson, A.-K., and Pålsson, K. (2007). Repellency of methyl jasmonate to Ixodes ricinus nymphs (Acari: Ixodidae). Exp. Appl. Acarol. 42, 209–215. doi: 10.1007/s10493-007-9066-9061
Gasperi, G., Bellini, R., Malacrida, A. R., Crisanti, A., Dottori, M., and Aksoy, S. (2012). A new threat looming over the mediterranean basin: emergence of viral diseases transmitted by aedes Albopictus mosquitoes. PLoS Neglect. Trop. Dis. 6:e1836. doi: 10.1371/journal.pntd.0001836
Giatropoulos, A., Kimbaris, A., Michaelakis, A, Papachristos, D. P., Polissiou, M. G., and Emmanouel, N. (2018). Chemical composition and assessment of larvicidal and repellent capacity of 14 Lamiaceae essential oils against Aedes albopictus. Parasitol. Res. 117, 1953–1964. doi: 10.1007/s00436-018-5892-5899
Goodyer, L. I., Croft, A. M., Frances, S. P., Hill, N., Moore, S. J., Onyango, S. P., et al. (2010). Expert review of the evidence base for arthropod bite avoidance. J. Travel. Med. 17, 182–192. doi: 10.1111/j.1708-8305.2010.00402.x
Gosselin, R. E., Smith, R. P., Hodge, H. C., and Gleason, M. N. (1976). “Clinical toxicology of commercial products 4th ed. Baltimore,” in, II–126.
Ham, W. S., Hillenbrand, J., Jacq, J., Genicot, C., and Ritter, T. (2019). Divergent late-stage (Hetero)aryl C-H amination by the pyridinium radical cation. Angewandte Chem. Int. Edn. 58, 532–536. doi: 10.1002/anie.201810262
Hart-Cooper, W. M., Clary, K. N., Toste, F. D., Bergman, R. G., and Raymond, K. N. (2012). Selective monoterpene-like cyclization reactions achieved by water exclusion from reactive intermediates in a supramolecular catalyst. J. Am. Chem. Soc. 134, 17873–17876. doi: 10.1021/ja308254k
Houlden, C. E., Hutchby, M., Bailey, C. D., Ford, J. G., Tyler, S. N. G., Gagné, M. R., et al. (2009). Room-temperature palladium-catalyzed C-H activation: ortho-carbonylation of aniline derivatives. Angewandte Chem. Int. Edn. 48, 1830–1833. doi: 10.1002/anie.200805842
Hu, Y., and Li, B. (2017). Efficient and selective palladium-catalyzed direct oxidative esterification of benzylic alcohols under aerobic conditions. Tetrahedron 73, 7301–7307. doi: 10.1016/j.tet.2017.11.025
Huang, X., Xiao, R., You, C., Yan, T., and Cai, M. (2017). A highly efficient heterogeneous copper-catalysed cascade reaction of aryl iodides with acetamidine hydrochloride leading to primary arylamines. J. Chem. Res. 41, 315–320. doi: 10.3184/174751917X14931195075580
Hwang, Y.-S., Schultz, G. W., and Mulla, M. S. (1984). Structure-activity relationship of unsaturated fatty acids as mosquito ovipositional repellents. J. Chem. Ecol. 10, 145–151. doi: 10.1007/BF00987651
Islam, J., Zaman, K., Duarah, S., Raju, P. S., and Chattopadhyay, P. (2017). Mosquito repellents: an insight into the chronological perspectives and novel discoveries. Acta Trop. 167, 216–230. doi: 10.1016/j.actatropica.2016.12.031
Itaya, N., Matsuo, T., Ohno, N., Mizutani, T., Fujita, F., and Yoshioka, H. (1977). “Recent progress in syntheses of the new and most potent pyrethroids,” in Proceedings of the Synthetic Pyrethroids ACS Symposium Series, (Washington, DC: American Chemical Society), 45–54. doi: 10.1021/bk-1977-0042.ch004
Ito, H., Takenaka, Y., Fukunishi, S., and Iguchi, K. (2005). A practical preparation of 2-Hydroxymethyl-2-cyclopenten-1-one by Morita-Baylis-Hillman reaction. Synthesis 2005, 3035–3038. doi: 10.1055/s-2005-916030
Jian, Z., Chao, C., and Tian-tao, W. (2008). Synthesis and Efficacy Test of Repellent IR3535. hinese Journal of Hygienic Insecticides & Equipments. Available at: http://en.cnki.com.cn/Article_en/CJFDTOTAL-WSSC200804013.htm (accessed August, 2019).
Kain, P., Boyle, S. M., Tharadra, S. K., Guda, T., Pham, C., Dahanukar, A., et al. (2013). Odour receptors and neurons for DEET and new insect repellents. Nature 502, 507–512. doi: 10.1038/nature12594
Kataoka, H., Yamada, T., Goto, K., and Tsuji, J. (1987). An efficiemt synthetic method of methyl (±)-jasmonate. Tetrahedron 43, 4107–4112. doi: 10.1016/S0040-4020(01)83449-83449
Kenmochi, H., Akiyama, T., Yuasa, Y., Kobayashi, T., and Tachikawa, A. (1999). Method for Producing Para-Menthane-3,8-Diol. Available at: https://patents.google.com/patent/US5959161A/en (accessed August, 2019).
Khan, A. A., Maibach, H. I., and Skidmore, D. L. (1975). Addition of vanillin to mosquito repellents to increase protection time. Mosquito News 35, 223–225.
Klier, M., and Kuhlow, F. (1976). Neue insektenabwehrmittel - am stickstoff disubstituierte ß-Alaninderivate. J. Soc. Cosmetic Chem. 27, 141–153.
Kočovský, P., Ahmed, G., Šrogl, J., Malkov, A. V., and Steele, J. (1999). New Lewis-Acidic Molybdenum(II) and Tungsten(II) catalysts for intramolecular carbonyl ene and prins Reactions. Reversal of the stereoselectivity of cyclization of citronellal. J. Org. Chem. 64, 2765–2775. doi: 10.1021/jo9821675
Köllner, T. G., Lenk, C., Zhao, N., Seidl-Adams, I., Gershenzon, J., Chen, F., et al. (2010). Herbivore-induced SABATH Methyltransferases of maize that methylate anthranilic acid using S-Adenosyl-l-Methionine. Plant Physiol. 153, 1795–1807. doi: 10.1104/pp.110.158360
Komatsuki, Y., Ishida, K., and Shiroyama, K. (2013). P-Menthane-3,8-diol Isomer Mixture, Composition Comprising the Same, and Products Comprising the Mixture or Composition. Available at: https://patents.google.com/patent/US20130136704A1/en (accessed August, 2019).
Kondo, K., Matsui, K., and Negishi, A. (1977). “New synthesis of the acid moiety of pyrethroids,” in Proceedings of the Synthetic Pyrethroids ACS Symposium Series, (Washingdon, DC: American Chemical Society), 128–136. doi: 10.1021/bk-1977-0042.ch012
Kondo, K., Takashima, T., Negishi, A., Matsui, K., Fujimoto, T., Sugimoto, K., et al. (1980). Stereochemical and chiral aspects in the synthesis of 3-(2,2- dihalovinyl)-2,2-dimethylcyclopropanecarboxylic acids. Pest. Sci. 11, 180–187. doi: 10.1002/ps.2780110212
Leal, G. M., and Leal, W. S. (2015). Binding of a fluorescence reporter and a ligand to an odorant-binding protein of the yellow fever mosquito. Aedes aegypti. F1000Res 3:305. doi: 10.12688/f1000research.5879.2
Legeay, S., Clere, N., Hilairet, G., Do, Q.-T., Bernard, P., Quignard, J.-F., et al. (2016). The insect repellent N,N-diethyl-m-toluamide (DEET) induces angiogenesis via allosteric modulation of the M3 muscarinic receptor in endothelial cells. Sci. Rep. 6:28546. doi: 10.1038/srep28546
Lett, B. D., and Kraus, H. S. (1992). Insect Repellent. Available at: https://patents.google.com/patent/WO1992002136A1/en?oq=Lett%2c+B.+D.;+Kraus%2c+H.+S.+PCT+Int.+Appl.+WO9202136%2c+1992;+Chem.+Abstr.1992%2c+117%2c+2827 (accessed August, 2019).
Li, Z., Yu, H., and Bolm, C. (2017). Dibenzothiophene sulfoximine as an NH3 surrogate in the synthesis of primary amines by copper-catalyzed C-X and C-H bond amination. Angewandte Chem. Int. Edn. 56, 9532–9535. doi: 10.1002/anie.201705025
Lianjia, G. (2011). Preparation Method of Methyl Anthranilate. Available at: https://patents.google.com/patent/CN101948400A/en (accessed August, 2019).
Llana-Ruiz-Cabello, M., Gutiérrez-Praena, D., Pichardo, S., Moreno, F. J., Bermúdez, J. M., Aucejo, S., et al. (2014). Cytotoxicity and morphological effects induced by carvacrol and thymol on the human cell line Caco-2. Food Chem. Toxicol. 64, 281–290. doi: 10.1016/j.fct.2013.12.005
Luo, F.-T., and Negishi, E. (1985). Palladium-catalyzed allylation of lithium 3-alkenyl-1-cyclopentenolates-triethylborane and its application to a selective synthesis of methyl (z)-jasmonate1. Tetrahedron Lett. 26, 2177–2180. doi: 10.1016/S0040-4039(00)98955-X
Lupi, E., Hatz, C., and Schlagenhauf, P. (2013). The efficacy of repellents against Aedes, Anopheles, Culex and Ixodes spp. – A literature review. Travel Med. Infect. Dis. 11, 374–411. doi: 10.1016/j.tmaid.2013.10.005
Markiewicz, J. T., Wiest, O., and Helquist, P. (2010). Synthesis of primary Aryl Amines through a copper-assisted aromatic substitution reaction with sodium Azide. J. Org. Chem. 75, 4887–4890. doi: 10.1021/jo101002p
McDougal, N. T., and Schaus, S. E. (2003). Asymmetric Morita-Baylis-Hillman reactions catalyzed by Chiral Brønsted acids. J. Am. Chem. Soc. 125, 12094–12095. doi: 10.1021/ja037705w
Merck Global, (2019). Discover the Insect Repellent IR3535®. Available at: http://www.merckgroup.com/en/expertise/cosmetics/care-solutions/insect-repellent/about-IR3535.html (accessed August, 2019).
Min, J., Guo, K., Suryadevara, P. K., Zhu, F., Holbrook, G., Chen, Y., et al. (2016). Optimization of a novel series of Ataxia-Telangiectasia mutated kinase inhibitors as potential radiosensitizing agents. J. Med. Chem. 59, 559–577. doi: 10.1021/acs.jmedchem.5b01092
Miot, H. A., Ferreira, D. P., Mendes, F. G., Carrenho, F. R. H., Amui, I., de, O., et al. (2008). Efficacy of Topical Permethrin as Repellent Against’s bites. Dermatology Online Journal 14. Available at: https://escholarship.org/uc/item/8pr6v8zp (accessed August, 2019).
Mizutani, T., Ohno, N., Ume, Y., Toyonaka, O., and Matsuo, T. (1975). A process for Preparing Esters of 3-phenoxybenzyl Alcohol. Available at: https://patents.google.com/patent/DE2437882A1/en (accessed August, 2019).
Mori, F., Omura, Y., Nishida, T., and Itoi, K. (1978). Process for Preparation of Substituted Cyclopropane Carboxylic Acids and Esters Thereof and Intermediates of Said Acids and Esters. Available at: https://patents.google.com/patent/US4113968A/en (accessed August, 2019).
Mori, F., Omura, Y., Nishida, T., and Itoi, K. (1980). Preparation of 1,1,1-Trihalogeno-4-Methyl-3-Penten-2-ol. Available at: https://patents.google.com/patent/US4190730/un (accessed August, 2019).
Moyes, C. L., Vontas, J., Martins, A. J., Ng, L. C., Koou, S. Y., Dusfour, I., et al. (2017). Contemporary status of insecticide resistance in the major Aedes vectors of arboviruses infecting humans. PLoS Neglect. Trop. Dis. 11:e0005625. doi: 10.1371/journal.pntd.0005625
Näf, F., and Decorzant, R. (1978). An efficient synthesis of Methyl (±)-Jasmonate and (Z)-Jasmone. Helvetica Chim. Acta 61, 2524–2529. doi: 10.1002/hlca.19780610723
Narahashi, T. (2000). Neuroreceptors and ion channels as the basis for drug action: past, present, and future. J. Pharmacol. Exp. Ther. 294, 1–26.
National Toxicology Program (2016). 14th Report on Carcinogens National Toxicology Program (NTP). Available at: https://ntp.niehs.nih.gov/go/roc14 (accessed August, 2019).
Nentwig, G. (2003). Use of repellents as prophylactic agents. Parasitol. Res. 90, S40–S48. doi: 10.1007/s00436-002-0755-758
Odalo, J. O., Omolo, M. O., Malebo, H., Angira, J., Njeru, P. M., Ndiege, I. O., et al. (2005). Repellency of essential oils of some plants from the Kenyan coast against Anopheles gambiae. Acta Trop. 95, 210–218. doi: 10.1016/j.actatropica.2005.06.007
O’Neil, M. J. (2013). The Merck Index: an Encyclopedia of Chemicals, Drugs, and Biologicals. Cambridge, MA: RSC Publishing.
Oxford Biosciences (2003). Restrictions and Allergens. Available at: http://oxfordbiosciences.com/restrictions-and-allergens/ (accessed August, 2019).
Patel, R. V., Shaeer, K. M., Patel, P., Garmaza, A., Wiangkham, K., Franks, R. B., et al. (2016). EPA-registered repellents for mosquitoes transmitting emerging viral disease. Pharmacotherapy 36, 1272–1280. doi: 10.1002/phar.1854
Piccolomini, A. M., Whiten, S. R., Flenniken, M. L., O’Neill, K. M., and Peterson, R. K. D. (2018). Acute toxicity of Permethrin, Deltamethrin, and Etofenprox to the Alfalfa Leafcutting Bee. J. Econ. Entomol. 111, 1001–1005. doi: 10.1093/jee/toy014
Pichler, V., Bellini, R., Veronesi, R., Arnoldi, D., Rizzoli, A., Lia, R. P., et al. (2018). First evidence of resistance to pyrethroid insecticides in Italian Aedes albopictus populations 26?years after invasion. Pest Manag. Sci. 74, 1319–1327. doi: 10.1002/ps.4840
Pohlit, A. M., Lopes, N. P., Gama, R. A., Tadei, W. P., Neto, V. F., and de, A. (2011). Patent literature on mosquito repellent inventions which contain plant essential oils–a review. Planta Med. 77, 598–617. doi: 10.1055/s-0030-1270723
Powell, A. B., and Stahl, S. S. (2013). Aerobic oxidation of diverse primary alcohols to methyl esters with a readily accessible Heterogeneous Pd/Bi/Te catalyst. Org. Lett. 15, 5072–5075. doi: 10.1021/ol402428e
PubChem (2019). Ethyl Acrylate. Available at: https://pubchem.ncbi.nlm.nih.gov/compound/8821 (accessed August, 2019).
Ranson, H., Burhani, J., Lumjuan, N., and Black, W. C. IV (2010). Insecticide resistance in dengue vectors. TropIKA.net [online] 1.
Raymond-Delpech, V., Matsuda, K., Sattelle, B. M., Rauh, J. J., and Sattelle, D. B. (2005). Ion channels: molecular targets of neuroactive insecticides. Invert. Neurosci. 5, 119–133. doi: 10.1007/s10158-005-0004-9
Reifenrath, W. G., Olson, J. J., Vedula, U., and Osimitz, T. G. (2009). Percutaneous absorption of an insect repellent p-Menthane-3,8-DIOL: a model for human dermal absorption. J. Toxicol. Environ. Health Part A 72, 796–806. doi: 10.1080/15287390902800371
Reinhold, J. M., Lazzari, C. R., and Lahondère, C. (2018). Effects of the environmental temperature on aedes aegypti and Aedes albopictus mosquitoes: a review. Insects 9:158. doi: 10.3390/insects9040158
Roberts, D. R., Chareonviriyaphap, T., Harlan, H. H., and Hshieh, P. (1997). Methods of testing and analyzing excito-repellency responses of malaria vectors to insecticides. J. Am. Mosq. Control Assoc. 13, 13–17.
Roiz, D., Boussès, P., Simard, F., Paupy, C., and Fontenille, D. (2015). Autochthonous chikungunya transmission and extreme climate events in Southern France. PLoS Negl. Trop. Dis. 9:e0003854. doi: 10.1371/journal.pntd.0003854
Ross, J. H., Reifenrath, W. G., and Driver, J. H. (2011). Estimation of the percutaneous absorption of permethrin in humans using the parallelogram Method. J. Toxicol. Environ. Health Part A 74, 351–363. doi: 10.1080/15287394.2011.534425
Salam, N., Banerjee, B., Roy, A. S., Mondal, P., Roy, S., Bhaumik, A., et al. (2014). Silver nanoparticles embedded over mesoporous organic polymer as highly efficient and reusable nanocatalyst for the reduction of nitroarenes and aerobic oxidative esterification of alcohols. Appl. Catalysis A 477, 184–194. doi: 10.1016/j.apcata.2014.03.014
Salehi, B., Mishra, A. P., Shukla, I., Sharifi-Rad, M., Contreras, M. D. M., Segura-Carretero, A., et al. (2018). Thymol, thyme, and other plant sources: health and potential uses. Phytother. Res. 32, 1688–1706. doi: 10.1002/ptr.6109
Sanchez-Bayo, F., and Goka, K. (2014). Pesticide residues and Bees – a risk assessment. PLoS One 9:e94482. doi: 10.1371/journal.pone.0094482
Sanford, J. L., Shields, V. D. C., and Dickens, J. C. (2013). Gustatory receptor neuron responds to DEET and other insect repellents in the yellow-fever mosquito. Aedes aegypti. Naturwissenschaften 100, 269–273. doi: 10.1007/s00114-013-1021-x
Sarkar, S., and Khan, A. T. (2015). Beyond conventional routes, an unprecedented metal-free chemoselective synthesis of anthranilate esters via a multicomponent reaction (MCR) strategy. Chem. Commun. 51, 12673–12676. doi: 10.1039/C5CC03369A
Schöftner, R. (2015). Insektenabwehrmittel. Available at: https://patents.google.com/patent/EP2862442A1/en?oq=EP2862442 (accessed August, 2019).
Sharifi-Rad, M., Varoni, E. M., Iriti, M., Martorell, M., Setzer, W. N., and Del Mar, et al. (2018). Carvacrol and human health: a comprehensive review. Phytother. Res. 32, 1675–1687. doi: 10.1002/ptr.6103
Shiroyama, K., Sawano, K., and Ohta, H. (2001). Cool Feeling Composition. Available at: https://patents.google.com/patent/US6328982B1/en?oq=US6328982B1 (accessed August, 2019).
Siddegowda, M. S., Yathirajan, H. S., and Ramakrishna, R. A. (2012). A ligand-free and base-free copper catalyzed reaction: arylation of ammonia and primary amines as their acetate salts. Tetrahedron Lett. 53, 5219–5222. doi: 10.1016/j.tetlet.2012.07.048
Silbering, A. F., Bell, R., Münch, D., Cruchet, S., Gomez-Diaz, C., Laudes, T., et al. (2016). Ir40a neurons are not DEET detectors. Nature 534, E5–E7. doi: 10.1038/nature18321
Sisido, K., Kurozumi, S., and Utimoto, K. (1969). Synthesis of methyl dl-jasmonate. J. Org. Chem. 34, 2661–2664. doi: 10.1021/jo01261a038
Soderlund, D. M., Clark, J. M., Sheets, L. P., Mullin, L. S., Piccirillo, V. J., Sargent, D., et al. (2002). Mechanisms of pyrethroid neurotoxicity: implications for cumulative risk assessment. Toxicology 171, 3–59. doi: 10.1016/S0300-483X(01)00569-568
Sparks, J. T., Botsko, G., Swale, D. R., Boland, L. M., Patel, S. S., and Dickens, J. C. (2018). Membrane proteins mediating reception and transduction in chemosensory neurons in mosquitoes. Front. Physiol. 9:1309. doi: 10.3389/fphys.2018.01309
Stahl, S. S., Powell, A. B., Root, T. W., Mannel, D. S., and Ahmed, M. S. (2015). Conversion Of Alcohols To Alkyl Esters And Carboxylic Acids Using Heterogeneous Palladium-Based Catalysts. Available at: https://patents.google.com/patent/US20150314272A1/en (accessed August, 2019).
Stanczyk, N. M., Brookfield, J. F. Y., Field, L. M., and Logan, J. G. (2013). Aedes aegypti mosquitoes exhibit decreased repellency by DEET following previous exposure. PLoS One 8:e54438. doi: 10.1371/journal.pone.0054438
Stanczyk, N. M., Brookfield, J. F. Y., Ignell, R., Logan, J. G., and Field, L. M. (2010). Behavioral insensitivity to DEET in Aedes aegypti is a genetically determined trait residing in changes in sensillum function. PNAS 107, 8575–8580. doi: 10.1073/pnas.1001313107
Strickman, D., Frances, S. P., and Debboun, M. (2009). Prevention of Bug Bites, Stings, and Disease, 1 Edn. New York: Oxford University Press.
Swale, D. R., Sun, B., Tong, F., and Bloomquist, J. R. (2014). Neurotoxicity and mode of action of N. N-Diethyl-Meta-Toluamide (DEET). PLoS One 9:e103713. doi: 10.1371/journal.pone.0103713
Tamogami, S., Agrawal, G. K., and Rakwal, R. (2011). “Chapter 6 - jasmonates to jasmolites in plants: past, present, and future,” in Advances in Botanical Research, eds J.-C. Kader, and M. Delseny, (Academic Press), 309–348. doi: 10.1016/B978-0-12-385851-1.00006-8
The Medical Letter Inc. (2005). Picaridin - A New Insect Repellent. Available at: https://secure.medicalletter.org/w1210b (accessed August, 2019).
Venthur, H., and Zhou, J.-J. (2018). Odorant receptors and odorant-binding proteins as insect pest control targets: a comparative analysis. Front. Physiol. 9:1163. doi: 10.3389/fphys.2018.01163
Venturi, G., Luca, M. D., Fortuna, C., Remoli, M. E., Riccardo, F., Severini, F., et al. (2017). Detection of a chikungunya outbreak in Central Italy, August to September 2017. Eurosurveillance 22, 17–00646. doi: 10.2807/1560-7917.ES.2017.22.39.17-00646
von Elert, E., Preuss, K., and Fink, P. (2016). Infodisruption of inducible anti-predator defenses through commercial insect repellents? Environ. Pollut. 210, 18–26. doi: 10.1016/j.envpol.2015.11.032
Wang, J., and Luca, V. D. (2005). The biosynthesis and regulation of biosynthesis of Concord grape fruit esters, including ‘foxy’ methylanthranilate. Plant J. 44, 606–619. doi: 10.1111/j.1365-313X.2005.02552.x
Wang, J., Wu, D., Wang, Y., and Xie, D. (2019). Jasmonate action in plant defense against insects. J. Exp. Bot. 70, 3391–3400. doi: 10.1093/jxb/erz174
Wang, Y.-W., Zheng, L., Jia, F.-C., Chen, Y.-F., and Wu, A.-X. (2019). Oxidative ring-opening of isatins for the synthesis of 2-aminobenzamides and 2-aminobenzoates. Tetrahedron 75, 1497–1503. doi: 10.1016/j.tet.2019.01.067
Wasternack, C., and Hause, B. (2013). Jasmonates: biosynthesis, perception, signal transduction and action in plant stress response, growth and development. An update to the 2007 review in Annals of Botany. Ann. Bot. 111, 1021–1058. doi: 10.1093/aob/mct067
WHO (2006). Specifications and Evaluations for Public Health Pesticides. Ethyl Butylacetylaminopropionate. Available at: http://www.who.int/whopes/quality/en/IR3535_eval_april_2006.pdf (accessed August, 2019).
WHO (2015). World Health Organization. Available at: https://www.who.int/neglected_diseases/vector_ecology/mosquito-borne-diseases/en/ (accessed August, 2019).
Wilson, K. (2007). R. A. Sheldon, I. Arends and U. Hanefeld. Green chemistry and catalysis. Wiley-VCH, 2007, 448 pp; ISBN 978-3-527-30715-9 (Hardcover). Appl. Organ. Chem. 21, 1002–1002. doi: 10.1002/aoc.1306
Xia, N., and Taillefer, M. (2009). A very simple copper-catalyzed synthesis of anilines by employing Aqueous ammonia. Angewandte Chem. Int. Edition 48, 337–339. doi: 10.1002/anie.200802569
Xu, P., Choo, Y.-M., Chen, Z., Zeng, F., Tan, K., Chen, T.-Y., et al. (2018). Peripheral, intrareceptor inhibition in mosquito olfaction. bioRxiv[Preprint] doi: 10.1101/243162
Xu, P., Choo, Y.-M., De La Rosa, A., and Leal, W. S. (2014). Mosquito odorant receptor for DEET and methyl jasmonate. Proc. Natl. Acad. Sci. U.S.A. 111, 16592–16597. doi: 10.1073/pnas.1417244111
Xuedong, P. (2013). Preparation of cis-p-Menthyl-3,8-diol. Available at: https://patents.google.com/patent/CN103193598A/en (accessed August, 2019).
Yin, J., Choo, Y.-M., Duan, H., and Leal, W. S. (2015). Selectivity of odorant-binding proteins from the southern house mosquito tested against physiologically relevant ligands. Front. Physiol. 6:56. doi: 10.3389/fphys.2015.00056
Yuasa, Y., Tsuruta, H., and Yuasa, Y. (2000). A Practical and Efficient Synthesis of p-Menthane-3,8-diols. Org. Process Res. Dev. 4, 159–161. doi: 10.1021/op9901036
Zeelie, B., Rust, N., Gouws, S., Dugmore, G. M., and Mpuhlu, B. (2009). A Method of Producing Cyclic Diols. Available at: https://patents.google.com/patent/WO2009135229A1/en (accessed August, 2019).
Zeng, F., Xu, P., Tan, K., Zarbin, P. H. G., and Leal, W. S. (2018). Methyl dihydrojasmonate and lilial are the constituents with an “off-label” insect repellence in perfumes. PLoS One 13:e0199386. doi: 10.1371/journal.pone.0199386
Zhu, J. J., Cermak, S. C., Kenar, J. A., Brewer, G., Haynes, K. F., Boxler, D., et al. (2018). Better than DEET repellent compounds derived from coconut oil. Sci Rep 8, 14053. doi: 10.1038/s41598-018-32373-32377
Keywords: mosquito, bio-sourced repellent, essential oil, synergy, chemosensation
Citation: Grison C, Carrasco D, Pelissier F and Moderc A (2020) Reflexion on Bio-Sourced Mosquito Repellents: Nature, Activity, and Preparation. Front. Ecol. Evol. 8:8. doi: 10.3389/fevo.2020.00008
Received: 19 September 2019; Accepted: 15 January 2020;
Published: 07 February 2020.
Edited by:
Juergen Gross, Julius Kühn-Institut, GermanyReviewed by:
Paul Walter Charles Green, Syngenta (United Kingdom), United KingdomJunwei Jerry Zhu, Agricultural Research Service (USDA), United States
Copyright © 2020 Grison, Carrasco, Pelissier and Moderc. This is an open-access article distributed under the terms of the Creative Commons Attribution License (CC BY). The use, distribution or reproduction in other forums is permitted, provided the original author(s) and the copyright owner(s) are credited and that the original publication in this journal is cited, in accordance with accepted academic practice. No use, distribution or reproduction is permitted which does not comply with these terms.
*Correspondence: Claude Grison, Y2xhdWRlLmdyaXNvbkBjbnJzLmZy