- 1International Centre of Insect Physiology and Ecology (icipe), Nairobi, Kenya
- 2Department of Zoology and Entomology, University of Pretoria, Pretoria, South Africa
Oviposition site selection by gravid female insects is an important determinant in species distribution, abundance, and population dynamics. Females may assess the suitability of a potential oviposition substrate by using cues from conspecific or heterospecific individuals already present. Here, we assessed whether the presence of conspecific or heterospecific larvae and parasites influenced oviposition decisions by the stable fly, Stomoxys calcitrans (Linneaus). Using dual and multiple-choice oviposition bioassays, we found that gravid female S. calcitrans avoided substrates with conspecific larvae, the larvae of house flies, Musca domestica (Linneaus), and the mite Macrocheles muscaedomesticae (Scopoli). Avoidance of conspecific and heterospecific larvae persisted in the dark, suggesting that this behavior is mediated by chemical rather than visual cues. When we reared S. calcitrans in the presence of conspecific larvae and the larvae of house flies at different densities we found that this negatively affected emergence time, larval weight, larval survival, pupal weight, pupal survival, and adult weight. We also demonstrated that individuals of S. calcitrans developed in the presence of mites exhibited low egg hatchability, and poor larval and adult survival. Our study provides additional support for the “preference-performance” hypothesis in S. calcitrans, with gravid females preferring to lay eggs on a substrate that will enhance offspring fitness. We recommend that the chemical cues involved in avoidance by gravid female S. calcitrans of substrates with conspecific and heterospecific larvae should be elucidated. This could lead to the discovery of repellent chemicals important for S. calcitrans management.
Introduction
The fitness of holometabolic insects following metamorphosis is strongly influenced by the quality of the substrate in which they developed. Since the egg stage is immobile, gravid females need to lay eggs on a substrate that guarantees the fitness of their progeny. To select an appropriate breeding site, gravid females are guided by visual, mechanical, and chemical cues (Bentley and Day, 1989). In addition to these, intra- and inter-specific competition (Michaud and Jyoti, 2007) and parasitism (Sadek et al., 2010; Kacsoh et al., 2013) are also known to influence oviposition decisions by gravid female insects. This influence can be either facilitatory, neutral or inhibitory (Prokopy et al., 2001). Facilitation of oviposition occurs when the presence of conspecific or heterospecific individuals in a specific substrate is an indicator of high quality habitat (Köhncke, 2013). For example, Gonzalez et al. (2016) demonstrated that female Aedes aegyptii and Aedes albopictus (Linneaus) preferred to oviposit into water containing conspecific larvae. A neutral effect is evident when there is no observable cost of sharing the same habitat with other individuals. This has been shown in Pieris napi (Linneaus), where females laid an approximately equal number of eggs when exposed to substrates with and without conspecific cues (Raitanen et al., 2014).
Inhibition of oviposition results when females are deterred from laying eggs on a substrate by the presence of competitors. Gravid females may avoid competition for their offspring through direct, aggressive interactions with other females, the marking and detection of larval resources with oviposition pheromones, or avoidance of cues associated with eggs or larvae already present. For example, females of the olive fruit fly, Bactrocera oleae (Rossi), display aggressive behavior toward each other (wing waving, fast running toward the opponent, pouncing and boxing on the head and thorax of the foe) when they are close to oviposition sites (Benelli, 2014). In the apple maggot, Rhagoletis pomonella (Walsh), females avoid larval competition by marking their oviposition site with a pheromone that deters most females from laying eggs (Averill and Prokopy, 1987). In the convergent lady beetle, Hippodamia convergens (Guérin-Méneville), gravid females lay more eggs on cleaned sorghum plants compared to those bearing conspecific and heterospecific larvae of the wheat aphid, Schizaphis graminum (Rondani) (Michaud and Jyoti, 2007). The inhibitory effect is mainly explained by the avoidance by insect gravid females of larval competition, which can reduce offspring growth, negatively affect survival (Yoshioka et al., 2012), and sometimes increase predatory cannibalism (Vijendravarma et al., 2013; Ahmad et al., 2015) due to the over-exploitation of resources. Similarly, inhibition of insect oviposition behavior can also be triggered by the presence of parasites. By laying more eggs on a substrate devoid of parasites, gravid female insects protect their offspring. This is evident in Drosophila melanogaster (Meigen), where females prefer to oviposit on alcohol-laden food sources that protect hatched larvae from wasp parasitisation (Kacsoh et al., 2013).
Stable flies, Stomoxys calcitrans (Linneaus), are cosmopolitan blood-feeders that mechanically transmit viruses (e.g., West Nile fever virus, Rift Valley fever virus), bacteria (e.g., Bacillus anthracis, Pasteurella multocida), protozoans (e.g., Trypanosoma evansi, Besnoitia besnoit), and helminths (e.g., Habronema microstoma, Dirofilaria repens) to their hosts. Hosts include cattle, camels, horses, dogs, and humans (Baldacchino et al., 2013). During periods of high abundance, S. calcitrans can reduce weight gain in cattle by up to 19%, and lead to a 40–60% reduction in milk yields (Walker, 1990; Carn, 1996). In the USA, Taylor et al. (2012) estimated that S. calcitrans lead to economic losses of around $2.2 billion per year.
Gravid females S. calcitrans use vertebrate herbivore dung for oviposition (Baleba et al., 2019), as well as rotting plant material, such as silage, hay, grass clippings, and garden compost (Cook et al., 2018). Decaying plant-based material is also an important ecological niche for other invertebrate species (Sladecek et al., 2017) such as the house fly, Musca domestica (Linneaus) (Broce and Haas, 1999) and the mite Macrocheles muscaedomesticae (Scopoli) (Jalil and Rodriguez, 1970). Similar to S. calcitrans, gravid females of M. domestica seek manure, decaying vegetation and compost for egg deposition (Machtinger et al., 2014). Macrocheles muscaedomesticae is a free-living cosmopolitan mite inhabiting decaying organic matter, including manure (Gerson et al., 2003). This mite feeds on the eggs and early larval stages of flies (Axtell, 1963). When reared on sterilized cow manure and fed with M. domestica eggs, Farahi et al. (2018) demonstrated that the longevity of M. muscaedomesticae can vary from 38.63 to 37.48 days under bisexual and oedipal reproduction, respectively. These mites are also found attached to the abdomen of adult flies, which they infect and parasitise during adult fly emergence from the puparium. This interaction has long been considered phoretic, but it is suspected that mites can extract haemolymph from their hosts, thereby reducing their flight performance (Luong et al., 2015). Macrocheles mites harm their hosts in different ways. Polak and Markow (1995) showed that these mites influenced the copulatory success in the fruit fly Drosophila nigrospiracula (Patterson and Wheeler). They demonstrated that males infested with two mites copulated less frequently than uninfested individuals. But after removing mites, previously infested males copulated as many times as flies with no history of infestation. Also, under laboratory condition, Polak and Polak (1996) showed that Macrocheles mites negatively affected the survivorship of D. nigrospiracula. The authors also demonstrated that this mite infestation lengthened the pre-oviposition period and reduced the number of eggs laid by gravid female D. nigrospiracula.
In this study, we determined how competition and parasitism affected oviposition decisions by gravid female S. calcitrans. We hypothesized they would avoid substrates with conspecific or heterospecific larvae and parasites to protect their offspring from intra- or inter-specific competition and parasitism. To test this hypothesis, we first assessed whether the presence of S. calcitrans larvae, M. domestica larvae and M. muscaedomesticae adults in a breeding substrate could influence oviposition decisions by S. calcitrans. Next, we addressed the ultimate evolutionary question of the fitness costs incurred by S. calcitrans immature stages when developing on a substrate with high competition or parasitism. These results provide insights into how gravid females of S. calcitrans are likely to behave in the presence of conspecific and heterospecific larvae, or parasites on a substrate. Such information is important for the management of S. calcitrans because it could lead to the discovery of semiochemicals acting either as attractants or repellents.
Materials and Methods
Study Animals and Substrates
Stomoxys calcitrans flies were obtained from a single culture established for approximately 1 year at the Duduville campus of the International Centre of Insect Physiology and Ecology (icipe) in Nairobi, Kenya (1° 13′ 12″ S, 36° 52′ 48″ E; ≈ 1,600 m above sea level). To establish this colony, we used wild individual of S. calcitrans captured on the icipe campus using Vavoua traps. Prior to the experiment, gravid females of S. calcitrans from the established colony were exposed to rabbit dung (fermented in a plastic bag for 1 week) placed in plastic containers (21.5 × 14.5 × 7.4 cm) for oviposition. Rabbit dung was collected from the icipe rabbit breeding unit. After exposure for 24 h we transferred these containers to another cage (75 × 60 × 45 cm), and we monitored the development of the larval and pupal stages until adult emergence. Emerged flies were fed twice per day (800 and 1600 h) on defibrinated bovine blood obtained from a local abattoir poured on moistened cotton. Larvae of M. domestica were from a culture established from wild individuals captured from Kapiti Plain in Machakos County, Kenya (1°37′60″S, 37°0′0″E), using Vavoua traps (Mihok et al., 1995). They were reared on rabbit dung as previously described, but adults were fed on rotten banana, orange and watermelon. Macrocheles muscaedomesticae (Figure 5Ai) were harvested directly from unsterilized rabbit dung collected at the icipe rabbit breeding unit. We identified this mite following the morphological characteristics described by Özbek et al. (2015) and Kamaruzaman et al. (2018). Insect rearing and experiments were done in a laboratory under buffered conditions of 25 ± 5°C and 65 ± 5% relative humidity. The photoperiod was 12L:12D unless otherwise stated.
Oviposition in the Presence of Conspecific and Heterospecific Larvae
We performed multiple-choice oviposition bioassays in cages (75 × 60 × 45 cm). In each cage, we allowed 20 gravid female S. calcitrans to oviposit on four types of substrate presented simultaneously: (1) rabbit dung, (2) rabbit dung with 10 S. calcitrans third instar larvae, (3) rabbit dung with 10 M. domestica third instar larvae, and (4) rabbit dung with 10 S. calcitrans and 10 M. domestica third instar larvae. For each replicate, we counted the number of eggs laid on each treatment 24 h after setting-up the bioassay. During all experiments performed in the laboratory, we used 50 g of rabbit dung, introduced in transparent 200 ml plastic cups. The high walls of these cups prevented escape of larvae from the dung. These bioassays were replicated 10 times. Here and in the following bioassays, flies in each replicate were used only once.
Oviposition in Response to Abundance of Conspecific and Heterospecific Larvae
In the previous bioassay, we found that gravid female S. calcitrans avoided laying eggs on a substrate containing conspecific larvae (Figure 1A). Here, we aimed to establish whether avoidance of conspecifics was dependent on the number of individuals present on a substrate. We performed a density-dependent response oviposition bioassay where we exposed 20 gravid female S. calcitrans to five rabbit dung substrates (as described previously) containing an increasing number (0, 10, 20, 30, and 40) of conspecific third instar larvae. We found that female S. calcitrans avoided all the substrates with conspecific larvae (Figure 1Bi). Afterwards, we assessed whether visual or chemical cues mediated conspecific avoidance. To do so, we followed the protocol developed by Yang and Shiao (2012) in two blow flies, Chrysomya megacephala (Fabricus) and Chrysomya rufifacies (Macquart). This involved two-choice bioassays in darkness (0L: 24D photocycle) performed in a different climate room to the one where the culture was held so that the diurnal rhythm of the culture was not affected. In a cage (34 × 34 × 34 cm) containing 10 gravid female S. calcitrans, we introduced two treatments: (1) rabbit dung and (2) rabbit dung+40 third instar S. calcitrans larvae. We found that gravid female S. calcitrans significantly avoided the substrate containing conspecific larvae (Figure 1Bii). We also assessed whether gravid female S. calcitrans could avoid a substrate already used by conspecific larvae. In a two-choice bioassay, we allowed gravid female S. calcitrans to choose between used (where 40 S. calcitrans eggs were reared until the pupae stage and removed) and unused substrates (1 week fermented rabbit dung). Each bioassay described above was performed for 24 h after which we counted the number of eggs laid on each substrate treatment. Each bioassay was replicated 10 times.
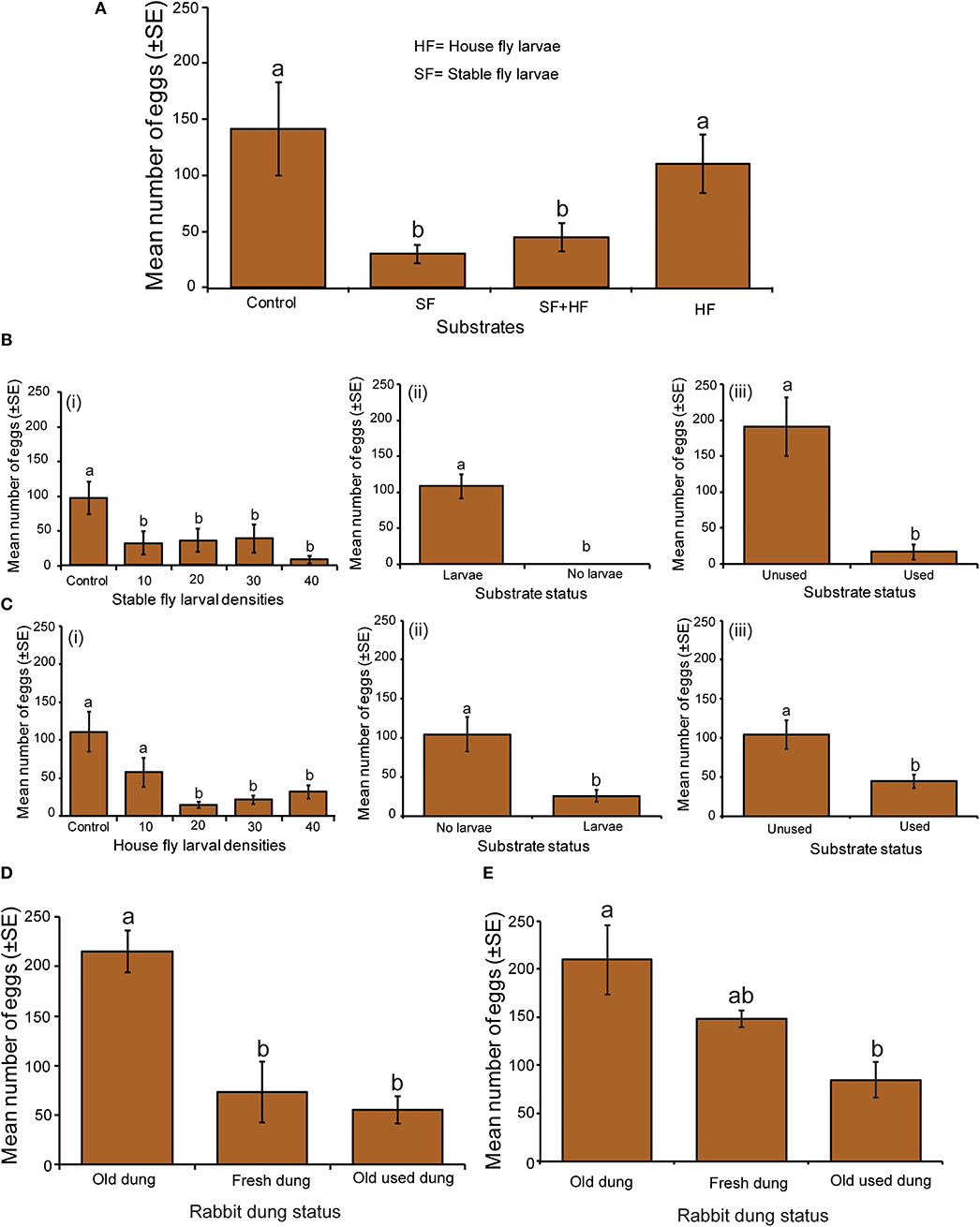
Figure 1. Influence of the presence of S. calcitrans and M. domestica larvae on oviposition decisions by S. calcitrans. (A) Bar chart showing the mean number of eggs laid by gravid female S. calcitrans in the presence of conspecific larvae and the larvae of the house fly (HF), M. domestica. (B) Bar charts illustrating the mean number of eggs laid by gravid female S. calcitrans in the presence of conspecific larvae following three experimental conditions: (i) different larval densities, (ii) in darkness, (iii) with unused and used substrates. (C) Bar charts depicting the mean number of eggs laid by gravid female S. calcitrans in the presence of M. domestica larvae following three experimental conditions: (i) different larval densities, (ii) in darkness, and (iii) with unused and used substrates. Error bars indicate the standard error of the mean. Treatments with different lowercase letters are significantly different from each other (Kruskal-Wallis test followed by Dunn's post-hoc tests or Wilcoxon–Mann–Whitney tests, P < 0.05, n = 10). (D) Bart chart showing the mean number of eggs oviposited by gravid female S. calcitrans when presented with fresh rabbit dung, old rabbit dung and old rabbit dung used by S. calcitrans larvae. (E) Bart chart depicting the mean number of eggs oviposited by gravid female S. calcitrans when presented with fresh rabbit dung, old rabbit dung, and old rabbit dung used by M. domesticae larvae.
The same series of experiments was then performed using M. domestica larvae. In the multiple-choice oviposition bioassay, we found that gravid females of S. calcitrans laid a similar number of eggs on the control substrate and the substrate with larvae of M. domestica (Figure 1A). We therefore sought to elucidate whether this behavior could change if the number of M. domestica larvae on the substrate increased, and to assess the role of visual or chemical cues in observed oviposition patterns using the same protocol as described for S. calcitrans.
In the previous experiments it may be that lower oviposition on substrates used by con- and heterospecfic larvae results from reduced nutrient content as the substrates aged. To test this, we conducted two additional oviposition bioassays. In the first bioassay, we provided 20 gravid female S. calcitrans with access to three substrate types: (1) fresh rabbit dung, (2) rabbit dung that S. calcitrans larvae had developed in, and (3) rabbit dung from the same batch as 2 that no S. calcitrans larvae had developed in. The second bioassay was similar to the first one except that we used larvae of M. domestica instead. In both bioassays, we used 50 g of rabbit dung where 10 larvae were reared up to the pupal stage. We counted the number of eggs on each substrate after 24 h and we replicated the procedure 10 times.
Oviposition in the Presence of Parasites
To evaluate how oviposition by S. calcitrans is affected by the presence of parasites in dung, we performed two-choice bioassays. Ten gravid female S. calcitrans were introduced to a cage (34 × 34 × 34 cm) containing a rabbit dung control and rabbit dung supplemented with 50 adults of the mite M. muscaedomesticae. We counted the number of eggs laid on each substrate after 24 h and we replicated the experiment 10 times. We found that S. calcitrans gravid females laid fewer eggs on the substrate with mites (Figure 2A). To find out if this behavior was driven by visual or olfactory cues, we repeated the two-choice bioassays in darkness as described previously. Ten replicates of these bioassays were performed.
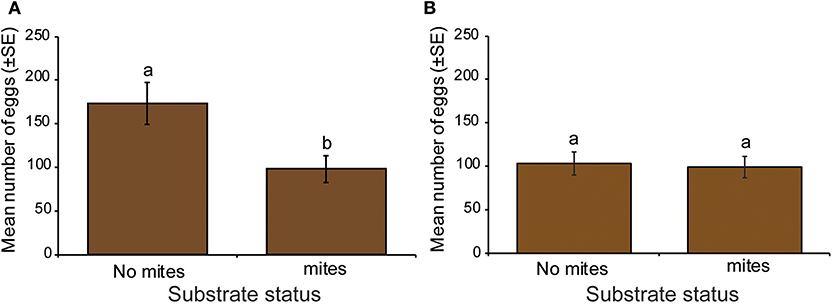
Figure 2. Influence of the presence of the mite Macrocheles muscaedomesticae on oviposition decisions by S. calcitrans. (A) Bar chart depicting the mean number of eggs laid by S. calcitrans on substrates with and without mites under light (Paired t-test, P < 0.05, n = 10). (B) Bar chart depicting the mean number of eggs laid by S. calcitrans on substrates with and without mites in darkness (Paired t-test, P > 0.05, n = 10). Error bars indicate the standard error of the mean. Treatments with different lowercase letters are significantly different from each other.
Cost of Developing on a Substrate With Conspecific and Heterospecific Larvae
Danchin and Wagner (1997) postulated that individuals developing in groups would pay a fitness cost due to increased competition for limited resources. Therefore, we hypothesized that S. calcitrans offspring developing with conspecific or heterospecific larvae at high density would exhibit poor development. To assess the effect of intraspecific competition on S. calcitrans, we reared first instar larvae in groups of 5, 15, and 25 in plastic cups filled with 50 g of rabbit dung. Daily, we monitored the development of these larvae until adult emergence by recording the following parameters: (1) emergence time (from first instar larvae to adult emergence), (2) larval weight (weighed at 4, 7, and 10 days after the beginning of the experiment), (3) pupation percentage (equivalent to larval mortality), (4) pupal weight, (5) emergence percentage (equivalent to pupal mortality), and (6) adult weight. We replicated each bioassay 10 times. For the weight parameter, we individually weighed all larvae, pupae and adults that emerged from each density treatment. We weighed S. calcitrans larvae and pupae using a Sartorius balance (0.0001 g precision) after gently cleaning them with wet paper towel. To record the adult weight, we used a cylindrical container (height: 4 cm; diameter: 3 cm) to avoid their escape. After taring the container, we introduced a single adult inside and recorded it's weight.
To test the fitness consequences for S. calcitrans developing in the presence of interspecific competition, we combined 10 first instar larvae of S. calcitrans with an incremental number of first instar M. domestica larvae as follows: 10:0, 10:10, 10:20, 10:30, 10:40. Each combination was introduced to 50 g of rabbit dung and replicated 10 times. Here, we also monitored the development of S. calcitrans larvae until adult emergence by recording the same six fitness parameters listed previously. Owing to our inability to differentiate S. calcitrans larvae from M. domestica larvae on day 4 and 7, we weighed S. calcitrans larvae only on day 10. On day 10, S. calcitrans larvae were smaller, with translucent bodies, in comparison with M. domestica larvae, which were larger and pale white to yellow in color.
Cost of Developing in a Substrate With Parasites
We hypothesized that if S. calcitrans eggs, larvae, or pupae were transferred to a substrate hosting the mite M. muscaedomesticae, egg hatchability, larval mortality, and adult survival in S. calcitrans would be affected. We introduced 10 eggs of S. calcitrans in transparent plastic cups of 200 ml prior containing 50 g of rabbit dung infested with 50 adult mites of M. muscaedomesticae. As a control, we transferred 10 eggs of S. calcitrans to rabbit dung without mites. Three days after, we determined the number of eggs that hatched by counting the number of larvae (L1 stage) found on each substrate treatment. To assess the effect of mite infestation on S. calcitrans larval survival, we introduced 10 L1 larvae of S. calcitrans to rabbit dung hosting mites as previously described. For the control treatment, we also reared 10 L1 larvae on substrates without mites. In both treatments, we counted the number of dead larvae and the number of pupae. To determine adult survival, we transferred S. calcitrans pupae on rabbit dung infested with mites to initiate parasitation during adult emergence. After emergence, we collected 10 individuals of S. calcitrans loaded with at least five mites on the abdomen, and we transferred them to another cage (20 × 15 × 14.5 cm). As a control, we used 10 new emerged healthy flies (without mites). For 8 days, we supplied flies of each treatment (infested and non-infested) with defibrinated bovine blood daily and counted the number of dead individuals. we replicated the bioassay assessing egg hatchability and the larvae survival 10 times, whereas we replicated the bioassay testing adult survival five times.
Data Analysis
All analyses were performed using the R environment for statisitcal computing (version 3.5.1) (R Core Team., 2018). The number of eggs laid in the presence of conspecific and heterospecific larvae, and with increasing abundance of S. calcitrans or M. domestica larvae, were not normally distributed (Shapiro-Wilk test: p < 0.05). For this reason, we used Kruskal-Wallis tests to compare oviposition among presented substrates, followed by Dunn's post-hoc tests to identify homogenous subsets. Eggs laid under dark conditions, and with used or unused substrates were also not normally distributed. To compare oviposition under these conditions, we used the paired Mann-Wilcoxon test. In the bioassay with substrates of different age, the number of eggs laid were normally distributed and their variance were homogeneous (Bartlett's test: p > 0.05), therefore we used the analysis of variance (ANOVA), followed by Student-Neuman-Keuls (SNK) post-hoc multiple comparison tests. The number of eggs laid by S. calcitrans in the presence or absence of the mite M. muscaedomesticae were also normally distributed and their variance was homogeneous, whether tested in the light or dark. To analyse these data, we used paired t-tests.
In our experiment assessing the effects of intraspecific competition on fitness parameters of S. calcitrans, emergence time and pupal weight data were not normally distributed (Shapiro-Wilk test: p < 0.05). Due to this, we used Kruskal-Wallis tests followed by Dunn's post-hoc tests to see how emergence time and pupal weight varied across the tested larval densities. For the larval weight, except weight recorded at day 7, which was not normally distributed (we used the Kruskal-Wallis test followed by Dunn's post-hoc test for analysis), data from larval weight recorded on days 3 and 10 followed a normal distribution (Shapiro-Wilk test: p > 0.05). Consequently, we ran analyses of variance (ANOVA) followed by Dunnett-Tukey-Kramer (DTK) post-hoc multiple comparisons tests (due to the inequality of the samples size) using the R library “DTK” (Lau, 2013). We also used ANOVA followed by DTK post-hoc tests to compare adult weight across the different larval densities owing to their normal distribution. Due to the binary nature of pupation (pupated vs. non-pupated) and adult emergence (emerged vs. non-emerged), we used generalized linear models (GLM) with binomial distribution to test whether these variables were affected by larval density (Warton and Hui, 2011). We established the significance of the model using analysis of deviance (with chi-squared test). Using the “emmeans” R package (Lenth, 2018), we ran Tukey's multiple comparisons tests to identify homogenous subsets in pupation and adult emergence among the larval densities.
In the bioassay testing the effect of interspecific competition on S. calcitrans fitness parameters, emergence time, and pupal weight data were not normally distributed (Shapiro-Wilk test: p < 0.05), so we ran Kruskal-Wallis tests followed by Dunn's post-hoc tests to see which M. domestica larval densities differed from each other. Larval and pupal weight data were normally distributed (Shapiro-Wilk test: p > 0.05), so we ran ANOVA tests followed by DTK post-hoc tests. We used a generalized linear model (GLM) with binomial distribution and the analysis of deviance test (with Chi-square test) followed by the Tukey's multiple comparisons tests to determine how pupation and the percentage emergence varied across the different larval densities.
For the bioassay aiming to assess the effect of mite infestation on egg hatchability and larval survival of S. calcitrans data were binary (hatched vs. unhatched). For this reason, we used a generalized linear model (GLM) with binomial distribution and an analysis of deviance (with chi-squared test). To compare survivorship of adult flies with mites and without mites, we performed Kaplan–Meier survival analysis with the Mantel-Cox log-rank chi-squared test using the R package “survival” (Therneau, 2015).
Results
Oviposition in the Presence of Conspecific and Heterospecific Larvae
Female S. calcitrans deposited significantly more eggs on rabbit dung without conspecific larvae than on the substrates with conspecific larvae (Figure 1A; H = 11.45, df = 3, P = 0.009). This avoidance behavior was not noted when the substrate was already colonized by the larvae of M. domestica. The number of eggs laid by female S. calcitrans on substrates with different conspecific larval density did not differ but was significantly lower than on uncolonized rabbit dung (Figure 1Bi, H = 14.13, df = 4, P = 0.007). Under dark conditions, females completely avoided the substrate with conspecific larvae (Figure 1Bii: U = 0, P < 0.001). They also preferred the unused substrate over the substrate used by conspecific larvae (Figure 1Biii: U = 55, P = 0.002).
An incremental increase in the number of heterospecific larvae colonizing rabbit dung led to fewer eggs laid by female S. calcitrans. The number of eggs laid by female S. calcitrans was significantly lower on substrates with 20–40 M. domestica larvae than on a control or dung with 10 heterospecific larvae (Figure 1Ci: H = 13.61, df = 4, P = 0.008). Under dark conditions, female S. calcitrans laid significantly fewer eggs on a substrate with 40 M. domestica larvae in comparison with the control (Figure 1Cii: U = 55, P = 0.002). Similarly, significantly fewer eggs were laid on the substrate used for M. domestica development than the control (Figure 1Ciii: U = 42, P = 0.019).
When investigating the role of dung age on avoidance of used substrates, the number of eggs oviposited by gravid female S. calcitrans varied significantly across the different rabbit dung treatments [Figure 1D: F(2, 27) = 14.62, P < 0.0001, Figure 1E: F(2, 27) = 6.73, P = 0.004]. Female S. calcitrans laid significantly more eggs on old, unused rabbit dung than its counterpart used by S. calcitrans larvae. They also avoided fresh rabbit dung (Figure 1D). Likewise, these females laid fewer eggs on rabbit dung used by M. domestica larvae than on their unused counterpart (Figure 1E).
Oviposition in the Presence of Parasites
Deposition of eggs by S. calcitrans on rabbit dung without or with mites differed. In the experiment performed under light, gravid female S. calcitrans laid significantly more eggs on the substrate devoid of mites than the substrate with mites (Figure 2A: t = −2.63, df = 9, P = 0.018). Under dark conditions, the number of eggs laid by females did not differ between the two treatments (Figure 2B: t = −0.14, df = 9, P = 0.82).
Costs of Developing on a Substrate With Conspecific and Heterospecific Larvae
Effect of Intraspecific Competition on S. Calcitrans Fitness Parameters
Larvae reared in a group of 5 and 15 reached the adult stage significantly faster than those reared in a group of 25 (Figure 3A; H = 7.73, df = 2, P = 0.02). Also, larval density significantly influenced larval weight [Day 4: F(2−63) = 3.5, P = 0.035; Day 7: H = 15.60, df = 2, P < 0.001; Day 10: F(2−50) = 20.91, P < 0.001]. Larvae reared in a group of 5, followed by those reared in a group of 15 were heavier in comparison with those reared in a group of 25 (Figure 3B). Despite differences in the body weight of larvae, the percentage of larvae reaching the pupal stage did not differ statistically (Figure 3C: GLM, χ2 = 2.27, d.f = 2, P = 0.32). Pupal weight differed significantly across the larval density treatments (Figure 3D, H = 98.4, df = 2, P < 0.0001), with pupal weight declining significantly with each incremental increase in larval density. The percentage of adults emerging from pupae reared in a group of 5 was significantly higher than at the higher larval densities (Figure 3E; GLM, χ2 = 17.06, d.f = 2, P < 0.001). Larval density also significantly affected adult weight [F(2−75) = 4.50, P < 0.05]. Adults from larvae reared in a group of 5 and 15 weighed more than those reared in a group of 25 (Figure 3F).
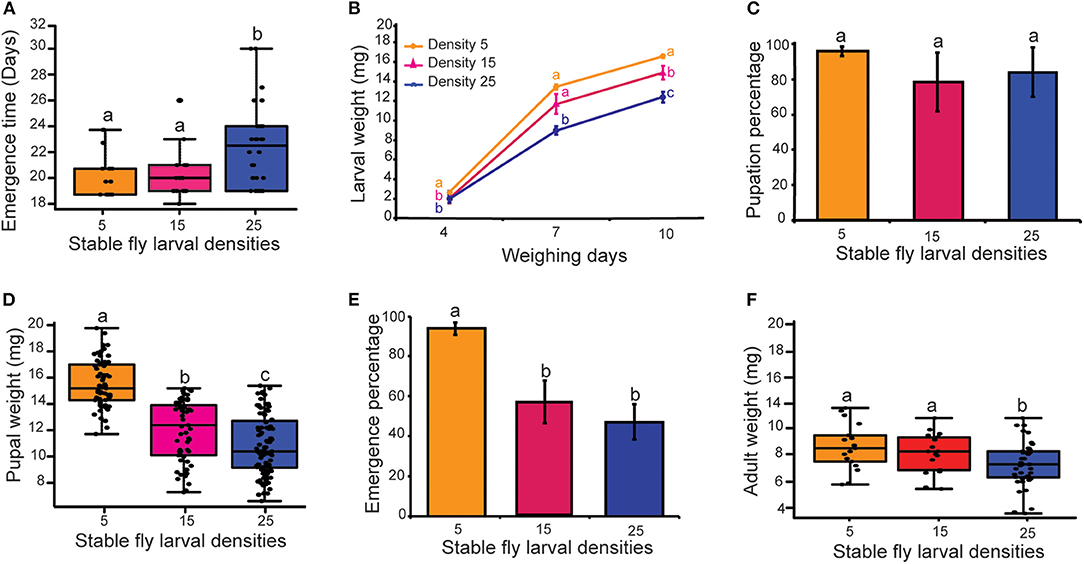
Figure 3. Effect of conspecific larvae density on fitness traits of S. calcitrans. (A) Boxplot showing S. calcitrans emergence time across the different larval densities (Kruskal-Wallis test followed by Dunn's post-hoc tests, P < 0.05). (B) Line graph illustrating the change of S. calcitrans larvae weight across the different larval densities after 4 days (ANOVA test followed by SNK's post-hoc test, P < 0.05), 7 days (Kruskal-Wallis test followed by Dunn's post-hoc test, P < 0.05) and 10 days (ANOVA test followed by SNK's post-hoc test, P < 0.05) of eclosion. (C) Bar charts depicting the mean percentage of S. calcitrans larvae reaching the pupal stage across the different larval densities (GLM with binomial distribution followed by Tukey post-hoc mean separation test, P < 0.05, n = 10). (D) Boxplot showing S. calcitrans pupal weight across the different larval densities (Kruskal-Wallis test followed by Dunn's post-hoc test, P < 0.05). (E) Bar chart illustrating the mean percentage of S. calcitrans emerging as adults from the pupal stage across the different larval densities (GLM with binomial distribution followed by Tukey post-hoc mean separation test, P < 0.05, n = 10). (F) Boxplot depicting S. calcitrans adult weight across the different larval densities (ANOVA test followed by DTK's post-hoc test, P < 0.05). On each boxplot, the minimum and maximum values of all the data are represented by the ends of boxplot whiskers. On the line graph and bar charts, error bars indicate the standard error of the mean. Treatments with different lowercase letters are significantly different from each other.
Effect of Interspecific Competition on S. Calcitrans Fitness Parameters
Density of M. domestica larvae significantly affected the emergence time (H = 13.88, df = 4, P = 0.007), larval weight [F(4−104) = 4.45, P = 0.002], pupation percentage (GLM, χ2 = 44.51, d.f = 4, P < 0.001), pupal weight (H = 35.54, df = 4, P < 0.001), emergence percentage (GLM, χ2 = 24.38, d.f = 4, P < 0.001) and adult weight [F(4−57) = 7.95, P < 0.001] of the ten S. calcitrans individuals present in each substrate treatment. In comparison with S. calcitrans larvae reared in the presence of 0, 10, 20, and 30 M. domestica larvae, S. calcitrans larvae reared in the presence of 40 house fly larvae took longer to reach the adult stage (Figure 4A). When S. calcitrans were reared with 30 or 40 M. domestica larvae their larval weight was less than in other treatments (Figure 4B). The percentage of S. calcitrans larvae that reached the pupal stage was higher on the substrate devoid of house fly larvae (Figure 4C). Individuals from the substrate without larvae or with 10 M. domestica larvae had greater pupal weight (Figure 4D) and emergence percentage (Figure 4E) than those from other treatments. Adults from the substrate without house fly larvae were heavier than those reared from other treatments (Figure 4F).
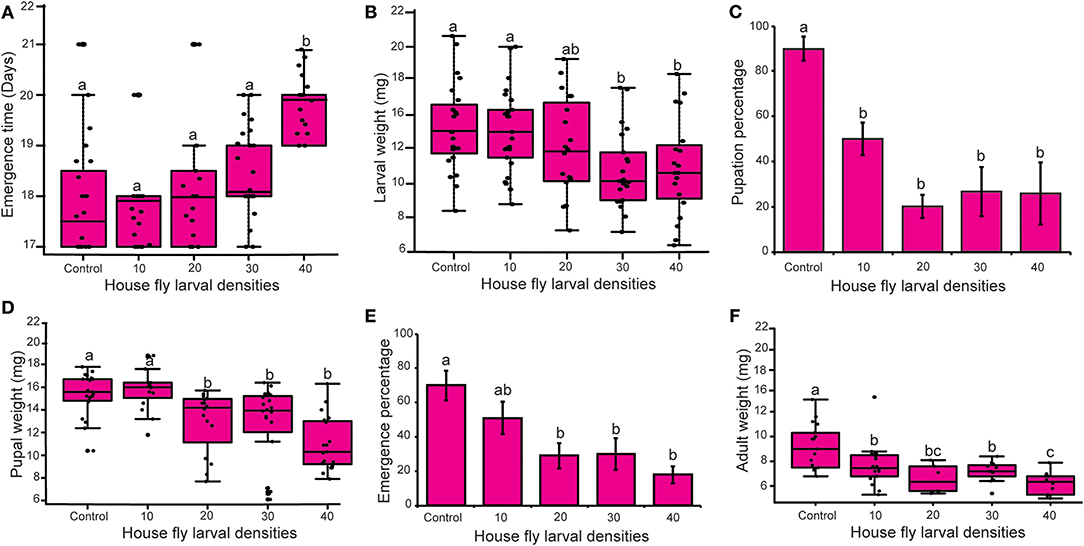
Figure 4. Effect of M. domestica larval density on fitness parameters of S. calcitrans. (A) Boxplot showing the variation of S. calcitrans emergence time across the different larval densities (Kruskal-Wallis test followed by Dunn's post-hoc test, P < 0.05). (B) Boxplot illustrating the change of S. calcitrans larvae weight across the different larval densities (ANOVA test followed by DTK's post-hoc test, P < 0.05). (C) Histograms depicting the pupation percentages of S. calcitrans across the different larval densities (GLM with binomial distribution followed by Tukey post-hoc mean separation test, P < 0.05, n = 10), (D) Bar chart showing the variation of S. calcitrans pupae weight across the different larval densities (Kruskal-Wallis test followed by Dunn's post-hoc test, P < 0.05). (E) Bar chart illustrating the variation of S. calcitrans emergence percentage across the different larval densities (GLM with binomial distribution followed by Tukey post-hoc mean separation test, P < 0.05, n = 10). (F) Boxplot depicting the variation of S. calcitrans adult weight across the different larval densities (ANOVA test followed by DTK's post-hoc test, P < 0.05). On each boxplot, the minimum and maximum values of all the data are represented by the ends of boxplot whiskers. On the bar charts, the error bars indicate the standard error of the mean (SEM). Treatments with different lowercase letters are significantly different from each other.
Cost of Developing in a Substrate With Parasites
The percentage of eggs that hatched was lower when placed on substrates hosting mites in comparison with substrates without mites (Figure 5B: GLM, χ2 = 59.55, df = 1, P < 0.001). Survival of larvae transferred to a substrate with mites was lower than those transferred to a substrate devoid of mites (Figure 5C: GLM, χ2 = 171.03, df = 1, P < 0.001). Mite infestation of the substrate negatively affected adult survival (Figure 5D; log-rank test, χ2 = 6.4, P = 0.011).
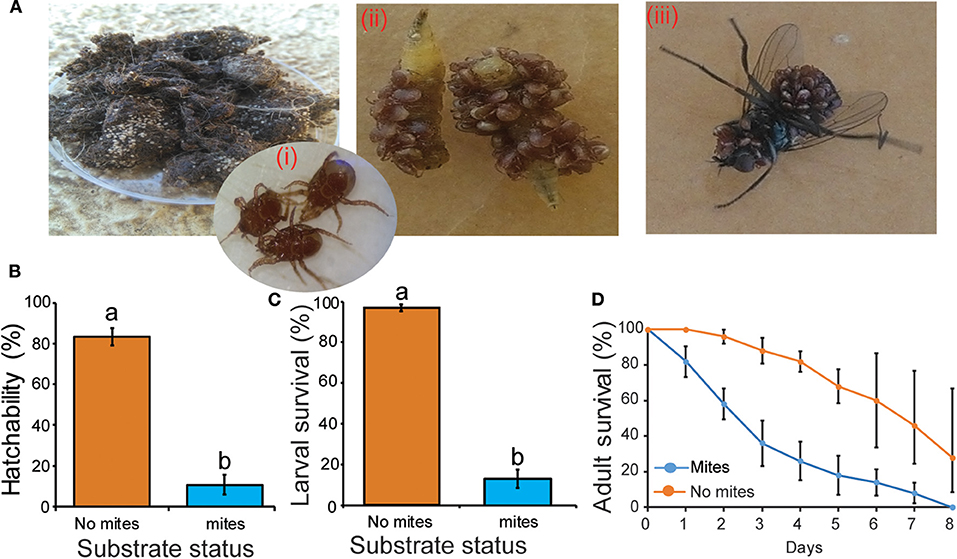
Figure 5. Effect of the mite M. muscaedomesticae on S. calcitrans survival. (A) Adult M. muscaedomesticae (i) aggregated on dead S. calcitrans larvae as well as (ii) dead and (iii) live adult S. calcitrans. (B,C) Bar charts showing hatchability of S. calcitrans eggs (B) and larval survival (C) on substrates with and without mites (GLM with binomial distribution followed by the analysis of deviance test, P < 0.05, n = 10). (D) Kaplan-Meier curve showing survivorship over time in adult of S. calcitrans with or without mite infestation (Mantel-Cox log-rank χ2 test, P < 0.05, n = 5). Error bars indicate the standard error of the mean. Treatments with different lowercase letters are significantly different from each other.
Discussion
The results obtained in this work showed that oviposition decisions by gravid female S. calcitrans are influenced by the presence of conspecific, heterospecific larvae, and parasites on a substrate. Gravid female S. calcitrans laid significantly fewer eggs on substrates containing conspecific and heterospecific larvae. We demonstrated that 10 conspecific larvae were enough to trigger this avoidance, whereas in the presence of M. domestica, avoidance began when there were more than 10 larvae. This suggests that gravid female S. calcitrans consider not only the density but identity of competitors when selecting oviposition sites. Similarly, Wachira et al. (2010) demonstrated that substrate augmentation of Culex quinquefasciatus (Say) larval density significantly reduced the oviposition activity index of gravid female Anopheles gambiae.
In darkness, S. calcitrans laid significantly fewer eggs in substrates with conspecific and M. domestica larvae, suggesting that instead of visual cues this avoidance is guided by chemical cues. Further, avoidance of oviposition substrates continued after larvae had been removed from them. The oviposition-deterrent effect of conspecific- and heterospecific-larvae found in this work has been shown in several species of Diptera. In Culiseta longiareolata (Macquart), 88% of gravid females laid eggs in pools with low conspecific larval density in comparison with pools with a high larval density (Kiflawi et al., 2003). In Culex restuans (Theobald), Reiskind and Wilson (2004) observed that gravid females avoided laying in containers with conspecific larvae. In Chrysomya megacephala, when liver with and without larvae of Chrysomya rufifacies were provided to gravid females, they oviposited on liver without larvae (Yang and Shiao, 2012). We suggest that S. calcitrans and M. domestica larvae may produce one or several chemical cues that strongly deter the oviposition behavior of gravid female S. calcitrans. Several chemical cues from conspecific- and heterospecific-larvae acting as repellents have already been identified in various studies. For instance, the alkane n-heneicosane found in the cuticle of A. aegypti larvae deters oviposition of this species at 100 and 1,000 mg/l (Seenivasagan et al., 2009). Gravid female Aedes albopictus are also repelled by n-heneicosane (secreted by Ae. aegypti larvae) at 30, 50, 100 and 200 ppm (Mendki et al., 2000). Several esters extracted from A. aegypti eggs (methyl dodecanoate, methyl tetradecanoate, methyl (Z)-9-hexadecenoate, methyl hexadecanoate (Z)-9-hexadecenoic acid, methyl (Z)-9-octadecenoate, and methyl octadecanoate (Z)-9-octadecenoic acid) were found to repel Ae. aegypti gravid females (Seenivasagan et al., 2009). Frass produced by developing larvae may also represent a source of chemical cues used by S. calcitrans to avoid substrates. Frass of conspecific- and hetero-specific larvae has been found to repel a range of insects, including Cydalima perspectalis (Walker) (Molnár et al., 2017), Monochamus alternatus (Hope) (Li and Zhang, 2006), Delia radicum (Linneaus) (Jones and Finch, 1987) and Ostrinia furnacalis (Guenée), Ostrinia scapulalis (Walker), and Ostrinia latipennis (Warren) (Li and Ishikawa, 2004). Our observations offer the potential for discovery of repellent compounds that may be used to manage S. calcitrans and reduce the spread of diseases that they transmit.
We found that intra- or inter-specific competition negatively affected the fitness of S. calcitrans offspring. This provides a functional basis for avoidance of substrates with conspecific or heterospecific larvae by gravid female S. calcitrans. The impact of larval density on fitness parameters has also been found in other Diptera including Hermetia illucens (Barragan-Fonseca et al., 2018), B. oleae (Burrack et al., 2009), D. melanogaster (Durisko and Dukas, 2013), Ae. albopictus (Yoshioka et al., 2012) and Ae. aegypti (Reiskind and Lounibos, 2009). When resources are limited, larval crowding induces exploitative competition that lengthens larval developmental time, increases larval mortality, and reduces larval, pupal and adult weight (Sugiura et al., 2007; Legros et al., 2009). This effect is mainly attributed to a reduction in the share of nutrients available in the breeding substrate for individuals. For illustration, Dutra et al. (2016) observed that glycogen concentration in Ae. aegypti larvae decreased by almost 50% as larval density increased. In H. illucens, crude fat concentration is higher in larvae reared at low density than those reared at high density (Barragan-Fonseca et al., 2018). Other studies suggest that high larval mortality observed with increased larval density results from interference competition. In crowded situations, D. melanogaster larvae exhibit cannibalism by killing and consuming other larvae (Vijendravarma et al., 2013; Ahmad et al., 2015).
Our study revealed that gravid female S. calcitrans were able to distinguish between substrates hosting parasites from substrates without them. In the light, gravid female S. calcitrans avoided the substrate containing the mite M. muscaedomesticae. Avoidance of parasitized substrates for oviposition is also found in other insect groups. Sadek et al. (2010) demonstrated that the moth Spodoptera littoralis (Biosduval) preferred to oviposit on alfafa compared to cotton where their eggs are more likely to be parasitized by the wasp, Chelonus inanitus (Linneaus). In darkness, we observed that gravid female S. calcitrans deposited the same number of eggs on substrates with and without mites. This indicates that rather than chemical cues, S. calcitrans may use visual cues to detect mites on the substrate. In D. melanogaster, Kacsoh et al. (2013) showed that oviposition in an alcohol-laden food source that protects hatched larvae from wasps was mediated by visual cues. As in the case of substrate colonization by con- and heterospecific larvae, avoidance of M. muscaedomesticae also benefited the fitness of S. calcitrans offspring. We found that egg hatchability, and larval and adult survival were higher in substrates without the mite M. muscaedomesticae. Axtell (1986) reported that this mite could kill three to four S. calcitrans eggs per day. As well as hemolymph consumption when attached to larvae or adults, it is also suspected that on the host, the mite also induces melanisation which is an immune response involving the enzyme phenoloxidase. González-Santoyo and Córdoba-Aguilar (2012) indicated that the production of this enzyme has a fitness cost in the host. We suggest that these two factors contributed to higher mortality of S. calcitrans larvae and adults in the presence of mites. As such, M. muscaedomesticae may represent a viable biological control agent for S. calcitrans.
In summary, our results indicate that gravid female S. calcitrans avoid substrates colonized by conspecific larvae, M. domestica larvae and the mite M. muscaedomesticae. This is likely due to the high fitness costs of competition and parasitisation for their offspring. We believe that the avoidance of substrates with conspecific and heterospecific larvae in S. calcitrans is mediated by chemical cues. Therefore, we recommend that additional studies be conducted to identify the chemical cues implicated in this avoidance behavior and their source. This will aid in development of improved control strategies against S. calcitrans.
Data Availability Statement
All data collected during this study are archived in Dryad data repository (https://datadryad.org/stash/share/D1eo6OeGBa91y7SB_izKUH7WQ1ihnmsJxOQM5oy34Tg).
Author Contributions
SB conceived the research idea, designed the experiment, collected and analyzed the data, and wrote the manuscript. DM and BT supervised and proofread the manuscript. MG and CW designed the experiment, supervised, and assisted with manuscript preparation. All authors have read and approved the manuscript.
Conflict of Interest
The authors declare that the research was conducted in the absence of any commercial or financial relationships that could be construed as a potential conflict of interest.
Acknowledgments
We thank Deutscher Akademischer Austauschdienst (DAAD) for providing SB with a doctoral scholarship through the ARPPIS-DAAD scholarship programme. This work was supported by the IBCARP camel, grant no. DCI-FOOD/2014/346-739 - mainly by the European Union and Max Planck Institute for Chemical Ecology - icipe partner group to MG. We also gratefully acknowledge the financial support for this research by the following organizations and agencies: Swedish International Development Cooperation Agency (Sida); UK Department for International Development (DFID); the Swiss Agency for Development and Cooperation (SDC); and the Kenyan Government. The views expressed herein do not necessarily reflect the official the opinion of the donors.
References
Ahmad, M., Chaudhary, S. U., Afzal, A. J., and Tariq, M. (2015). Starvation-induced dietary behaviour in Drosophila melanogaster larvae and adults. Sci. Rep. 5:14285. doi: 10.1038/srep14285
Averill, A. L., and Prokopy, R. J. (1987). Intraspecific competition in the Tephritid fruit fly Rhagoletis Pomonella. Ecology 68, 878–886. doi: 10.2307/1938359
Axtell, R. C. (1963). Acarina occurring in domestic animal manure. Ann. Entomol. Soc. Am. 56, 628–633. doi: 10.1093/aesa/56.5.628
Axtell, R. C. (1986). Fly Control in Confined Livestock and Poultry Production. Technical Monograph. Greensboro, NC: Ciba-Geigy Corporation. 1–59
Baldacchino, F., Muenworn, V., Desquesnes, M., Desoli, F., Charoenviriyaphap, T., and Duvallet, G. (2013). Transmission of pathogens by Stomoxys flies (Diptera, Muscidae): a review. Parasite 20:26. doi: 10.1051/parasite/2013026
Baleba, S. B. S., Torto, B., Masiga, D., Weldon, C. W., and Getahun, M. N. (2019). Egg-laying decisions based on olfactory cues enhance offspring fitness in Stomoxys calcitrans L. (Diptera: Muscidae). Sci. Rep. 9:3850. doi: 10.1038/s41598-019-40479-9
Barragan-Fonseca, K. B., Dicke, M., and van Loon, J. J. A. (2018). Influence of larval density and dietary nutrient concentration on performance, body protein, and fat contents of black soldier fly larvae (Hermetia illucens). Entomol. Exp. Appl. 166, 761–770. doi: 10.1111/eea.12716
Benelli, G. (2014). Aggressive behavior and territoriality in the olive fruit fly, Bactrocera oleae (Rossi) (Diptera: Tephritidae): role of residence and time of day. J. Insect Behav. 27, 145–161. doi: 10.1007/s10905-013-9411-7
Bentley, M. D., and Day, J. F. (1989). Chemical ecology and behavioral aspects of mosquito oviposition. Annu. Rev. Entomol. 34, 401–421 doi: 10.1146/annurev.en.34.010189.002153
Broce, A. B., and Haas, M. S. (1999). Relation of cattle manure age to colonization by stable fly and house fly (Diptera: Muscidae). J. Kansas Entomol. Soc. 72, 60–72.
Burrack, H. J., Fornell, A. M., Connell, J. H., O'Connell, N. V., Phillips, P. A., Vossen, P. M., et al. (2009). Intraspecific larval competition in the olive fruit fly (Diptera: Tephritidae). Environ. Entomol. 38, 1400–1410. doi: 10.1603/022.038.0508
Carn, V. M. (1996). The role of dipterous insects in the mechanical transmission of animal viruses. Br. Vet. J. 152, 377–393. doi: 10.1016/S0007-1935(96)80033-9
Cook, D. F., Telfer, D. V., Lindsey, J. B., and Deyl, R. A. (2018). Substrates across horticultural and livestock industries that support the development of stable fly, Stomoxys calcitrans (Diptera: Muscidae): Substrates that support stable fly. Austral Entomol. 57:344–8. doi: 10.1111/aen.12282
Danchin, E., and Wagner, R. H. (1997). The evolution of coloniality: the emergence of new perspectives. Trends Ecol. Evol. 12, 342–347. doi: 10.1016/S0169-5347(97)01124-5
Durisko, Z., and Dukas, R. (2013). Attraction to and learning from social cues in fruitfly larvae. Proc. R. Soc. Lond. 280:20131398. doi: 10.1098/rspb.2013.1398
Dutra, H. L. C., Lopes da Silva, V., da Rocha Fernandes, M., Logullo, C., Maciel-de-Freitas, R., and Moreira, L. A. (2016). The influence of larval competition on Brazilian Wolbachia-infected Aedes aegypti mosquitoes. Parasit Vectors 9:282. doi: 10.1186/s13071-016-1559-5
Farahi, S., Shishehbor, P., and Nemati, A. (2018). Bisexual and oedipal reproduction of Macrocheles muscaedomesticae (Acari, Macrochelidae) feeding on Musca domestica (Diptera, Muscidae) eggs. Acarologia 58, 430–441. doi: 10.24349/acarologia/20184251
Gerson, U., Smiley, R. L., Ochoa, R., and Gerson, U. (2003). Mites (acari) for Pest Control. 2nd Edn. Oxford; Malden, MA: Blackwell Science. doi: 10.1002/9780470750995
Gonzalez, P. V., González Audino, P. A., and Masuh, H. M. (2016). Oviposition behavior in Aedes aegypti and Aedes albopictus (Diptera: Culicidae) in response to the presence of heterospecific and conspecific larvae. J. Med. Entomol. 53, 268–272. doi: 10.1093/jme/tjv189
González-Santoyo, I., and Córdoba-Aguilar, A. (2012). Phenoloxidase: a key component of the insect immune system. Entomol. Exp. App. 142, 1–16. doi: 10.1111/j.1570-7458.2011.01187.x
Jalil, M., and Rodriguez, J. G. (1970). Studies of behaviour of Macrocheles muscaedomesticae (Acarina: Macrochelidae) with emphasis on its attraction to the house fly. Ann. Entomol. Soc. Am. 63, 738–744. doi: 10.1093/aesa/63.3.738
Jones, T., and Finch, S. (1987). The effect of a chemical deterrent, released from the frass of caterpillars of the garden pebble moth, on cabbage root fly oviposition. Entomol. Exp. App. 45, 283–288. doi: 10.1111/j.1570-7458.1987.tb01096.x
Kacsoh, B. Z., Lynch, Z. R., Mortimer, N. T., and Schlenke, T. A. (2013). Fruit flies medicate offspring after seeing parasites. Science 339, 947–950. doi: 10.1126/science.1229625
Kamaruzaman, N. A. C., Mašán, P., Velásquez, Y., González-Medina, A., Lindström, A., Braig, H. R., et al. (2018). Macrocheles species (Acari: Macrochelidae) associated with human corpses in Europe. Exp. Appl. Acarol. 76, 453–471. doi: 10.1007/s10493-018-0321-4
Kiflawi, M., Blaustein, L., and Mangel, M. (2003). Oviposition habitat selection by the mosquito Culiseta longiareolata in response to risk of predation and conspecific larval density. Ecol. Entomol. 28, 168–173. doi: 10.1046/j.1365-2311.2003.00505.x
Köhncke, A. (2013). When and Where to Lay Your Eggs? Berlin: Humboldt-Universität zu Berlin, Mathematisch-Naturwissenschaftliche Fakultät I.
Lau, M. K. (2013). DTK: Dunnett-Tukey-Kramer Pairwise Multiple Comparison Test Adjusted for Unequal Variances and Unequal Sample Sizes. R Package Version 3.5. Available online at: https://CRAN.R-project.org/package=DTK
Legros, M., Lloyd, A. L., Huang, Y., and Gould, F. (2009). Density-dependent intraspecific competition in the larval stage of Aedes aegypti (Diptera: Culicidae): revisiting the current paradigm. J. Med. Entomol. 46, 409–419. doi: 10.1603/033.046.0301
Lenth, R. (2018). Emmeans: Estimated Marginal Means, Aka Least-Squares Means. R Package Version 1.2.2. Available online at: https://CRAN.R-project.org/package=emmeans
Li, G., and Ishikawa, Y. (2004). Oviposition deterrents in larval frass of four Ostrinia species fed on an artificial diet. J. Chem. Ecol. 30, 1445–1456. doi: 10.1023/B:JOEC.0000037750.64844.4b
Li, S.-Q., and Zhang, Z.-N. (2006). Influence of larval frass extracts on the oviposition behaviour of Monochamus alternatus (Col., Cerambycidae). J. Appl. Entomol. 130, 177–182. doi: 10.1111/j.1439-0418.2006.01039.x
Luong, L. T., Penoni, L. R., Horn, C. J., and Polak, M. (2015). Physical and physiological costs of ectoparasitic mites on host flight endurance. Ecol. Entomol. 40, 518–524. doi: 10.1111/een.12218
Machtinger, E. T., Geden, C. J., Hogsette, J. A., and Leppla, N. C. (2014). Development and oviposition preference of house flies and stable flies (Diptera: Muscidae) in six substrates from Florida equine facilities. J. Med. Entomol. 51, 1144–1150. doi: 10.1603/ME14040
Mendki, M. J., Ganesan, K., Prakash, S., Suryanarayana, M. V. S., Malhotra, R. C., Rao, K. M., et al. (2000). Heneicosane: an oviposition-attractant pheromone of larval origin in Aedes aegypti mosquito. Curr. Sci. 78, 1295–1296.
Michaud, J. P., and Jyoti, J. L. (2007). Repellency of conspecific and heterospecific larval residues to Hippodamia convergens (Coleoptera: Coccinellidae) ovipositing on sorghum plants. Eur. J. Entomol. 104, 399–405. doi: 10.14411/eje.2007.059
Mihok, S., Kang'ethe, E. K., and Kamau, G. K. (1995). Trials of traps and attractants for Stomoxys spp. (Diptera: Muscidae). J. Med. Entomol. 32, 283–289. doi: 10.1093/jmedent/32.3.283
Molnár, B. P., Tóth, Z., and Kárpáti, Z. (2017). Synthetic blend of larval frass volatiles repel oviposition in the invasive box tree moth, Cydalima perspectalis. J. Pest Sci. 90, 873–885. doi: 10.1007/s10340-017-0837-0
Özbek, H. H., Bal, D. A., and Dogan, S. (2015). The genus Macrocheles Latreille (Acari: Mesostigmata: Macrochelidae) from Kelkit Valley (Turkey), with three newly recorded mite species. Turk. J. Zool. 39, 768–780. doi: 10.3906/zoo-1409-14
Polak, M., and Markow, T. A. (1995). Effect of ectoparasitic mites on sexual selection in a Sonoran desert fruit fly. Evolution 49, 660–669. doi: 10.1111/j.1558-5646.1995.tb02302.x
Polak, M., and Polak, M. (1996). Ectoparasitic effects on host survival and reproduction: the Drosophila- Macrocheles association. Ecology 77, 1379–1389. doi: 10.2307/2265535
Prokopy, R. J., Roitberg, B. D., Prokopy, R. J., and Roitberg, B. D. (2001). Joining and avoidance behavior in nonsocial insects. Annu. Rev. Entomol. 46, 631–665. doi: 10.1146/annurev.ento.46.1.631
R Core Team. (2018). R: A language and Environment for Statistical Computing R Foundation for Statistical Computing. Vienna. Available online at: https://www.R-project.org/
Raitanen, J., Forsman, J. T., Kivelä, S. M., Mäenpää, M. I., and Välimäki, P. (2014). Attraction to conspecific eggs may guide oviposition site selection in a solitary insect. Behav. Ecol. 25, 110–116. doi: 10.1093/beheco/art092
Reiskind, M. H., and Lounibos, L. P. (2009). Effects of intraspecific larval competition on adult longevity in the mosquitoes Aedes aegypti and Aedes albopictus. Med. Vet. Entomol. 23, 62–68. doi: 10.1111/j.1365-2915.2008.00782.x
Reiskind, M. H., and Wilson, M. L. (2004). Culex restuans (Diptera: Culicidae) oviposition behavior determined by larval habitat quality and quantity in south-eastern Michigan. J. Med. Entomol. 41, 179–186. doi: 10.1603/0022-2585-41.2.179
Sadek, M. M., Hansson, B. S., and Anderson, P. (2010). Does risk of egg parasitism affect choice of oviposition sites by a moth? A field and laboratory study. Basic Appl. Ecol. 11, 135–143. doi: 10.1016/j.baae.2009.09.003
Seenivasagan, T., Sharma, K. R., Sekhar, K., Ganesan, K., Prakash, S., and Vijayaraghavan, R. (2009). Electroantennogram, flight orientation, and oviposition responses of Aedes aegypti to the oviposition pheromone n-heneicosane. Parasitol. Res. 104, 827–833. doi: 10.1007/s00436-008-1263-2
Sladecek, F. X. J., Segar, S. T., Lee, C., Wall, R., and Konvicka, M. (2017). Temporal segregation between dung-inhabiting beetle and fly species. PLoS ONE 12:e0170426. doi: 10.1371/journal.pone.0170426
Sugiura, S., Yamazaki, K., and Yamaura, Y. (2007). Intraspecific competition as a selective pressure on the choice of oviposition site in a phytophagous insect: intraspecific competition of a leaf miner. Biol. J. Linnean Soc. 92, 641–650. doi: 10.1111/j.1095-8312.2007.00857.x
Taylor, D. B., Moon, R. D., and Mark, D. R. (2012). Economic impact of stable flies (Diptera: Muscidae) on dairy and beef cattle production. J. Med. Entomol. 49, 198–209. doi: 10.1603/ME10050
Therneau, T. (2015). A Package for Survival Analysis in S. Version 2.38. Available online at: https://CRAN.R-project.org/package=survival
Vijendravarma, R. K., Narasimha, S., and Kawecki, T. J. (2013). Predatory cannibalism in Drosophila melanogaster larvae. Nat. Commun. 4:2744. doi: 10.1038/ncomms2744
Wachira, S. W., Ndung'u, M., Njagi, P. G. N., and Hassanali, A. (2010). Comparative responses of ovipositing Anopheles gambiae and Culex quinquefasciatus females to the presence of Culex egg rafts and larvae. Med. Vet. Entomol. 24, 369–374. doi: 10.1111/j.1365-2915.2010.00913.x
Walker, A. R. (1990). “Disease caused by arthropods,” in Handbook on Animal Diseases in the Tropics (4th Edn), eds M. M. H. Sewell, and D. W. Brocklesby (London: Bailliere Tindall), 44.
Warton, D. I., and Hui, F. K. (2011). The arcsine is asinine: the analysis of proportions in ecology. Ecology 92, 3–10. doi: 10.1890/10-0340.1
Yang, S.-T., and Shiao, S.-F. (2012). Oviposition preferences of two forensically important blow fly species, Chrysomya megacephala and C. rufifacies (Diptera: Calliphoridae), and implications for postmortem interval estimation. J. Med. Entomol. 49, 424–435. doi: 10.1603/ME11133
Yoshioka, M., Couret, J., Kim, F., McMillan, J., Burkot, T. R., Dotson, E. M., et al. (2012). Diet and density dependent competition affect larval performance and oviposition site selection in the mosquito species Aedes albopictus (Diptera: Culicidae). Parasite Vector. 5:225. doi: 10.1186/1756-3305-5-225
Keywords: Stomoxys calcitrans, oviposition, competitors, parasites, offspring fitness
Citation: Baleba SBS, Torto B, Masiga D, Getahun MN and Weldon CW (2020) Stable Flies, Stomoxys calcitrans L. (Diptera: Muscidae), Improve Offspring Fitness by Avoiding Oviposition Substrates With Competitors or Parasites. Front. Ecol. Evol. 8:5. doi: 10.3389/fevo.2020.00005
Received: 04 September 2019; Accepted: 08 January 2020;
Published: 28 January 2020.
Edited by:
Elise Huchard, UMR5554 Institut des Sciences de l'Evolution de Montpellier (ISEM), FranceReviewed by:
Vivek Kempraj, Macquarie University, AustraliaFrancisco Garcia-Gonzalez, Estación Biológica de Doñana (EBD), Spain
Copyright © 2020 Baleba, Torto, Masiga, Getahun and Weldon. This is an open-access article distributed under the terms of the Creative Commons Attribution License (CC BY). The use, distribution or reproduction in other forums is permitted, provided the original author(s) and the copyright owner(s) are credited and that the original publication in this journal is cited, in accordance with accepted academic practice. No use, distribution or reproduction is permitted which does not comply with these terms.
*Correspondence: Steve B. S. Baleba, c2Jlcm5hcmRzdGV2ZSYjeDAwMDQwO2dtYWlsLmNvbQ==; YmJhbGViYSYjeDAwMDQwO2ljaXBlLm9yZw==