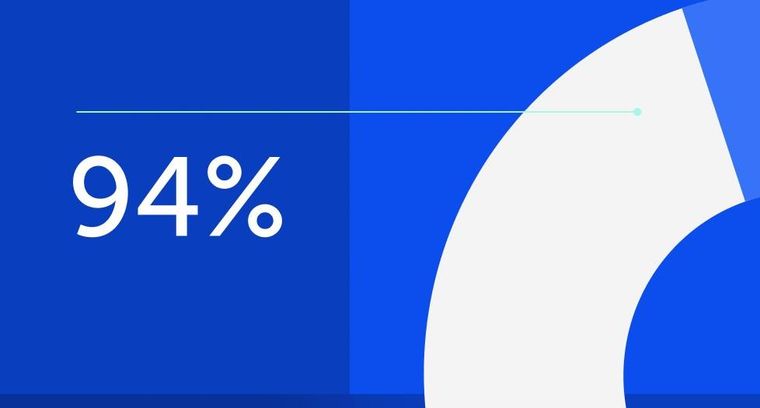
94% of researchers rate our articles as excellent or good
Learn more about the work of our research integrity team to safeguard the quality of each article we publish.
Find out more
ORIGINAL RESEARCH article
Front. Ecol. Evol., 22 January 2020
Sec. Behavioral and Evolutionary Ecology
Volume 7 - 2019 | https://doi.org/10.3389/fevo.2019.00520
Sexually selected signals reliably reflect individual phenotypic or genetic quality and, thus, survival prospects of holders. Telomere length is considered a good predictor of life expectancy and, consequently, exploring the links between telomere length and sexually selected traits is much needed to better understand the mechanisms that maintain the honesty of sexual signals. We manipulated the length of throat feathers in spotless starling (Sturnus unicolor) males (a sexually selected signal) before reproduction and explored its effects on telomere shortening and breeding performance in subsequent reproductive events. We did not detect an effect of the feathers clipping manipulation, but males with longer throat feathers before the experiment had shorter telomeres that also shortened more slowly than those of males with shorter throat feathers did. Moreover, length of throat feathers of males before manipulation was positively related to hatching success of second clutches. Thus, correlative but not experimental results support the expected associations between sexually selected signals, telomeres and reproduction. We discuss such results in scenarios of sexual selection where feather length reflects, but does not directly cause, telomere attrition, and enhanced reproductive success. Males with longer throat feathers might be older, more experienced males (i.e., with shorter telomeres), able to buffer telomere shortening between reproductive events. Because of the absence of experimental effects, differential incubation effort of females cannot explain the detected association with hatching success, but other sexually selected traits that covary with throat-feather length could be responsible. Exploring those physiological and/or morphological characteristics related to throat-feather length should therefore be the matter of future research.
The evolution of signals, defined as behavioral, morphological or physiological characteristics that function transferring information among organisms (Otte, 1974), have attracted the attention of evolutionary biologists for decades (Maynard-Smith and Harper, 2003). By definition, the use of signals must result in benefits for both the emitter and the receiver(s), which implies that the transmitted information should be reliable (Bradbury and Vehrencamp, 1998; Searcy and Nowicki, 2005). Extravagant secondary sexual characters of males are usually considered as examples of signals reflecting different components of their genetic and phenotypic quality (Andersson, 1994). Females, by preferring males with traits that correlate with their genetic and/or phenotypic quality, will gain direct (good parents) or indirect (good genes) beneficial fitness effects (Hamilton, 1990; Andersson, 1994; Møller, 1994; Møller and Jennions, 2001; Jones and Ratterman, 2009). On the other hand, by exaggerating attractive characters for females, males might gain breeding opportunities (Darwin, 1871), and increased investment in reproduction by females (Burley, 1988) that should result in higher reproductive success per reproductive event (Møller, 1994; Sheldon, 2000).
The above-described scenario explaining the evolution of sexually selected traits in males predicts a positive association between the character and the reproductive success of both males and females. Although it was suggested that male traits that are selected by females do not need to be related to phenotypic or genetic quality of holders (e.g., runaway sexual selection process, Fisher, 1930), it is now accepted that, mainly because of costs associated to intra sexual competition, these traits (i.e., sensory traps) would rapidly evolve reflecting the quality of males (Macías-García and Ramírez, 2005). Thus, sexually selected traits should reliably reflect phenotypic or genetic quality of the holder. Reliability of the signals is mainly warranted by associated costs that cheaters would not be able to afford (Zahavi, 1975, 1977; Maynard-Smith and Harper, 2003). These costs might refer to those of production (Hill, 2000; Pardal et al., 2018), maintenance (e.g., in time and energy Blount et al., 2003; Ruiz-Rodríguez et al., 2015), and showiness [social control Tibbetts and Dale, 2004; Webster et al., 2018 and detectability by parasites or predators (Stuart-Fox et al., 2003; Johnson and Candolin, 2017; but see Webster et al., 2018)]. Detecting such costs, and associations between the expression of the target trait and variables reflecting phenotypic or genetic quality of the holder, is essential to conclude in favor of the existence of reliable signals, and therefore to understand their evolution in a context of sexual selection.
Telomeres are regions of repeated non-coding DNA sequence (TTAGGG) that cap the end of eukaryotic chromosomes. Among other functions, telomeres protect wholeness of genetic information during cell division (Blackburn, 1991). Because replication failures frequently occur at the end of the chromosomes, telomeres continuously shorten as a consequence of cell division (Boonekamp et al., 2017). Thus, telomere length is a good predictor of senescence, both at cellular and organism levels (Suram et al., 2012; Campisi, 2013), and of survival prospects (Monaghan and Haussmann, 2006; Kotrschal et al., 2007; Soler et al., 2015). Moreover, telomere length and attrition apparently have significant genetic components (Dugdale and Richardson, 2018) and are related to antioxidant capabilities (Beaulieu et al., 2011; Badás et al., 2015; Kim and Velando, 2015), and to the level of stress suffered during development (Boonekamp et al., 2014; Herborn et al., 2014; Chatelain et al., 2019) including infection (Asghar et al., 2015, 2018; Eisenberg et al., 2017). Telomere length has even predicted reproductive success in wild birds (Boonekamp et al., 2014; Parolini et al., 2017; Bauer et al., 2018) and thus, detecting relationships between a sexually selected character and telomere length and/or attrition would suggest that the trait reflects (i.e., signals) phenotypic and/or genetic quality of the holder. Therefore, telomeres are likely candidates for maintaining reliability in sexually selected signals because their length is nowadays considered a good indicator of phenotypic quality (Bauch et al., 2013).
As far as we know, the expected association between a sexually selected trait and telomere length and/or dynamic, has only been explored and detected in two bird species, the barn swallow (Hirundo rustica) (Parolini et al., 2017) and the common yellowthroat (Geothlypis trichas) (Taff and Freeman-Gallant, 2017). In both studies, the focal sexually selected trait was plumage colouration. Telomere length of males and females correlated with sexually dimorphic colouration of swallows (Parolini et al., 2017). In the case of yellowthroats, plumage colouration was related to telomere length and rate of telomere loss between years, but only in males (Taff and Freeman-Gallant, 2017). More recently, it has been detected that colouration of spotless starlings (Sturnus unicolor, hereafter starlings) eggshells predicts telomere length of fledglings (Soler et al., 2018). Since eggshell colouration is a secondary sexual trait of females in this species (Soler et al., 2008), these results also suggest a link between telomeres and sexually selected traits. These examples are correlative results dealing with sexually selected characters mediated by pigments. We here go a step forward and test the expected association between telomere length and sexually selected traits in an experimental framework, focusing on a sexual trait for which expression is not related to pigments. In particular, we manipulated the length of the sexually dimorphic throat feathers of starling males (Hiraldo and Herrera, 1974; Ruiz-Rodríguez et al., 2015) and explored the effects on telomere dynamics, first egg-laying dates (date of reproduction) and reproductive success. The apical part of these feathers is quite flexible and males exhibit them very conspicuously during courtship (Ruiz-Rodríguez et al., 2015). In males, the apical part of throat feathers harbor more feather degrading keratinolytic bacteria and degrade more quickly than its basal part, suggesting that males with less degraded feathers are better able to control bacterial infections (Ruiz-Rodríguez et al., 2015). Moreover, length of the throat feathers is positively related with age (Hiraldo and Herrera, 1974), degree of individual homozygosity (Aparicio et al., 2001) and with the ability of counteracting the immunosuppressive effects of testosterone (Gil and Culver, 2011). Thus, there is reasonable evidence supporting the signaling nature of throat feathers of male starlings that females use in mate choice (Aparicio et al., 2001).
We manipulated the length of the throat feathers of males mainly just before egg laying (and in some cases after hatching eggs) and explored the effects on date of reproduction and success of subsequent reproductive events. Date of reproduction has been traditionally used as a proxy for mating success and thus for sexual selection component of fitness (Møller et al., 2006; Romano et al., 2017). We also explored the effects of experimental manipulation on telomere shortening by analyzing telomere length of males during subsequent reproductive seasons. Finally, we tested the relationship between length of throat feathers and telomere length and dynamics (as proxies of phenotypic and/or genetic quality) in males and females. Our expectation is that throat-feather length of starling males, but not that of females, should positively affect reproductive success, early pairing, and telomere length, reducing rate of telomere deterioration. Thus, we should find such associations in correlative frameworks, while the experimental reduction of throat feather length should result in lower reproductive success, later breeding dates and increased rates of telomere deterioration. In addition, if throat feathers function in social, non-sexual, communication of males, the experimental reduction could increase stress levels due to social interactions and thus, an effect on telomere deterioration should be detected. These interactions in starlings occur even at non-breeding period as seen in the European starling (Sturnus vulgaris) (Feare, 1984). During this period, dominance hierarchies are established and maintained through a series of pairwise interactions where throat feathers would play a role reducing probability of costly agonistic interaction (Creel, 2001; Wingfield and Silverin, 2002) between individuals greatly differing in throat feather length. By contrast, if throat feather length negatively affects mating success and, thus, reduces reproductive effort, telomere deterioration would be lower in males with experimentally shortened throat feathers.
The study was conducted during the years 2013, 2015, 2016, and 2017 in a south-eastern region of Spain (Hoya de Guadix, 37°15′N, 3°01′W) where nest-boxes attached to tree trunks or walls at 3–4 m above-ground are available for starlings to breed on (for further information on the study area see Soler et al., 2017).
The spotless starling is a medium-sized, hole-nesting and polygynous passerine (Cramp, 1998; Veiga and Polo, 2016). Starlings are sexually dimorphic (Hiraldo and Herrera, 1974). Apart from slight differences in beak and plumage coloration between males and females (Navarro et al., 2010; Veiga and Polo, 2016), the most apparent sexually dimorphic character are the throat feathers. This species has a post-breeding molt that finishes in October (Veiga and Polo, 2016). In the studied population, the reproduction (i.e., egg laying) starts in early April and most (about 80%) individuals lay a second clutch during May-June. The most common clutch size is 4–5 eggs. The incubation phase usually begins with the penultimate egg, and consequently, the last egg hatches later than the others (Veiga and Polo, 2016). Incubation is mostly carried out by females with sporadic help from males, and extends for around 14 days, while the nestling period lasts 21–22 days (Cramp, 1998). Both male and female parents contribute to feeding the offspring and remove nestling fecal sacs (Cramp, 1998). During the whole nesting process, adults bring feathers and aromatic plants to the nest, which have been shown to have antimicrobial-beneficial functions (Ruiz-Castellano et al., 2016; Soler et al., 2017). Reproductive success in the study area varies among years and breeding attempts, with second clutches usually showing lower reproductive success than first clutches (first clutches: mean ± SE = 2.9 ± 0.1 fledglings; second clutches: 2.6 ± 0.1 fledglings; F1, 402 = 3.97, p = 0.047).
In this population, courtship activity (e.g., singing, introducing fresh green plants, and feathers in nest boxes) starts in February, more than 1 month before egg laying (pers. obs.). During this period, some birds roost in nest-boxes and we take advantage of this fact for conducting yearly bird trapping sessions in the study area (twice a year between the end of February and the beginning of April; see Annex 1 for an overview of starling reproductive season with indication of trapping and manipulation performed). One hour before dawn, we closed the entrance of all nest boxes in the study area, and immediately after dawn, we captured by hand all individuals found roosting inside. We considered that they were paired if we found the male and female inside the same box, and the majority of them were already mated when captured (46 out of 51 males). Captured birds were individually kept in clean cotton bags hanging from a stick to keep birds quiet, and were released immediately after sampling. The maximum time that a captured starling was in the bag did never exceed 3 h. For additional details, see (Ruiz-Rodríguez et al., 2015). This procedure does not imply apparent negative effects on breeding performance of captured birds (Soler et al., 2008).
Every year, we also captured birds during the reproductive period (i.e., after egg laying), 4–5 days after the hatching date, by using nest-box traps for a maximum time of 1 h. All captured adults were ringed with a numbered metal ring (if not already ringed) and with a unique combination of three color rings. We also measured length of throat feathers of males (mean ± SE = 3.2 ± 0.1) and females (mean ± SE = 2.4 ± 0.1) with a ruler as shown in Figure 1B. Three measurements of the longest feather were taken to estimate its repeatability. In addition, we collected 0.5–0.6 ml of blood by puncturing the brachial vein and approximately 0.1 ml of this blood was conserved in ethanol for further molecular analyses. Finally, captured males were alternately assigned to control or experimental treatments. With the aid of scissors, we cut the distal half portion of throat feathers of experimental individuals, while we handled control males in the same way but without cutting throat feathers (Figure 1). Throat-feather length of males that were assigned to control or experimental treatment was similar (experimental group: means ± SE = 3.2 ± 0.1 cm; control group: means ± SE = 3.2 ± 0.1 cm; F1, 46 = 0.058, p = 0.811) but, after manipulation, that of experimental males was on average shortened by 1.7 ± 0.1 cm (between group comparisons, F1, 50 = 219.921, p < 0.0001; Figure 1). Males that were recaptured during different study years were assigned each time to the same experimental treatment, repeating the same procedure on them, with the exception of three males. Treatment of these three males changed from control in the first to experimental in the second capture, 1 year later. The effects of treatment in these males were considered as independent information in the analyses.
Figure 1. (A) Mean ± CI 95% length of throat feathers for control (CTRL) and experimental spotless starling males [before (EXB) and after (EXA) feather cutting]. In addition, we show pictures of the ornamental throat feathers length estimation procedure in a male spotless starling (B), as well as the appearance of a control (C), and an experimental (D) individual.
Starling reproduction was monitored by checking nest-boxes every 3 days since the beginning of April, which allowed us to accurately detect the date of first-egg laying and clutch size (i.e., maximum number of eggs detected). Afterwards, nest boxes with known date of reproduction were visited weekly until 2 days before the expected hatching date (14 days after the first egg was laid), when we visited the nest every 2 days to estimate hatching success (percentage of laid eggs that hatched successfully). During the nestling phase, we visited the nest-boxes at an intermediate nestling age (~8 days after hatching), and few days before fledging (14 days after hatching). During this last visit, the number of nestlings was recorded, and fledging success was estimated as the percentage of hatchlings that survived until this day.
Experimental males were identified during breeding by capture at the nest boxes during the nesting phase (see above), or by visualizing the unique combinations of color rings in 1 h video recordings of nest-box entrances made during the second-third day after hatching. Males usually attend the same nest-box during the entire breeding season in our study area (e.g., in 63 of 65 nest boxes the male attending the first and the second clutch was the same, MAG, personal observation). Thus, for those males that were only recorded in one of the breeding attempts (either first or second), we reasonably assumed that they attended the same nest-box during the other. The two exceptions when we detected different males attending first and second breeding attempts in the same nest-box were excluded from the analyses. Finally, the experiment didn't affect the males changing partners (GLZ: = 1.93, p = 0.165, Nctrl = 28, Nexp = 23).
DNA was extracted from blood samples using a standard chloroform–isoamyl alcohol-based protocol [see (Ferraguti et al., 2013)]. DNA concentration was adjusted to 20 ng μL−1 using distilled water and conserved frozen until further analyses. Relative telomere length (hereafter telomere length) was estimated by q-PCR following the protocol and primers of Criscuolo et al. (2009). As control single-copy gene, we used the glyceraldehyde-3-phosphate dehydrogenase (GAPDH) to normalize the quantity of telomere sequence to the amount of DNA in the q-PCR. The final PCR volume was 20 μL containing 10 μL of LightCycler 480 SYBR Green I Master (Roche Diagnostics GmbH, Mannheim, Germany) and 1 μL of DNA at 20 ng μL−1 of DNA. The reactions for telomeres or GAPDH were done in different plates due to the differential PCR conditions. Telomere PCR conditions were 10 min at 95°C followed by 30 cycles of 1 min at 56°C and 1 min at 95°C. GAPDH PCR started with 10 min at 95°C followed by 40 cycles of 1 min at 60°C and 1 min at 95°C, both performed in a LightCycler 480 RT-PCR System (Roche). Each sample was run in duplicate, and samples with a coefficient of variation higher to 5% were removed from the analyses. Each 96-well plate included serial dilutions of DNA (40, 10, 2.5, 0.66 ng of DNA per well) from a reference pool (the internal control) run in triplicate, which were used to generate the standard curves, and a blank control with no DNA. Quantification cycle values (Ct) were transformed into normalized relative quantities (NRQs) following Hellemans et al. (2007) procedure, which controls for the amplifying efficiency of each qPCR. Amplification efficiency for telomere products ranged between 1.92 and 2.07 and for the GAPDH product between 1.94 and 2.02. The melting curves of the control gene cycles confirmed no evidence of primer dimer or non-specific amplification.
Telomere shortening was calculated by subtracting the NRQ value of samples from the last capture to those of the first capture. These differences were standardized by dividing telomere shortening for the length of telomere of first captures. Elapsed time between captures varied between 82 days (that included most of the breeding season) and two years. Telomere length was estimated only for individuals (males and females) that were caught at least twice, whose time between captures lasted more than 82 days and included at least one reproductive event. Unfortunately, due to blood conservation problems, samples from the 2013 breeding season could not be analyzed. For a small proportion of individuals telomere length estimated at second capture was longer than at first capture. This apparent telomere elongation may be due to the measurement error of the technique but several studies have confirmed that telomeres can elongate during the fledging period (Spurgin et al., 2018; Pérez-Rodríguez et al., 2019).
The three measurements of throat feathers in males and females were highly repeatable (r = 0.96, F145, 292 = 51.0, p < 0.0001) and, consequently, we used the mean value for further analyses. As approximations to the mating success of the males, we used date of laying of the first egg in the season. Hatching and fledging success were used as proxies of reproductive success in the analyses. Independently of the study year, the zero value of date of reproduction corresponded to the 1st of April.
To explore the associations between length of throat feather (dependent variable) and telomere length (independent variable) we used General Linear Models (hereafter GLMs) that also included study year as discrete fixed independent factor. In this analysis we only considered information on first captures of each individual (i.e., before experimental manipulation). In the case of individuals whose first capture was made in 2013, we used the first next capture. The association between throat-feather length and telomere shortening was also explored in GLMs that included telomere attrition as the dependent variable, throat-feather length at first capture and time elapsed between captures as continuous independent factors, and experimental treatment as fixed effect (in males). We did not manipulate throat feathers of females and thus, sex and experimental treatment could not be included in the same model. Thus, the association between telomere dynamic and throat feather length was separately explored for males and females. However, because we were also interested in detecting sexual differences in the expected association, we explored the interaction between sex and throat feather length in separate models that included sex instead of experimental treatment as independent factor.
The associations between experimental manipulation of throat-feather length (independent factor) and date of reproduction, hatching and fledgling success, and total number of fledglings (dependent variables) were also explored in GLMs, that included feather length as continuous predictor and capture event (first or second capture) and study year as independent categorical factors. For these analyses, the males that were manipulated after hatching of the first brood were considered as “controls” for first brood, but as experimental for the second brood. Individual identity did not explain a significant proportion of variance in reproductive variables (the variance within and between individuals was similar, P-values associated to individual identity as random factor are always larger than 0.2) and, thus, was not included in the models. Hatching, fledging success and number of fledglings were analyzed separately for first and second clutches. We did so because environmental conditions usually deteriorate during second clutches and, thus, the expected association with the sexually selected trait may be more easily detected in second breeding attempts. However, we explored the possibility that the expected associations between feather length and reproductive variables differ for first and second clutches by looking at the interaction between breeding attempt and feather length in separate models that also included main effects.
We also explored the possibility that the expected experimental effects varied between captures by including the interaction between capture event and experimental treatment in the above described models. Moreover, we also tested the possibility that the relationship between throat-feather length and variables reflecting early reproduction (i.e., mating success) and breeding success differ for experimental and control males by exploring the interaction between throat-feather length and treatment in the previously described models. In no case, these interactions reached statistical significance (p > 0.145) and, thus, were not considered further. In addition, we explored whether the experimental shortening of throat feathers had any effect on feather size in subsequent molts by mean of GLM where the dependent variable was the feather length in the second capture, with time elapsed in days between the second and first capture as a continuous variable and treatment as a discrete factor.
Annex 2 reports information collected from each captured individual for the different statistical tests. Sample sizes differ between analyses for several reasons. First, not all captured individuals were recaptured or identified during breeding. Second, a few genetic analyses failed or samples deteriorated before laboratory analyses. Finally, information from control and experimental individuals was useful for testing some but not other predictions.
Residuals of all statistical models were plotted and visually checked for normality. All analyses were performed with Statistica V13 (Dell-Inc. 2015).
The association between telomere length and throat feather length differs for males and females (GLM, interaction between sex and telomere length, F1, 56 = 15.60, p < 0.001). In males, telomere length and throat-feather length were negatively related [Beta (SE) = −0.58 (0.19), F1, 17 = 9.36, p = 0.007; Figure 2A), after controlling for the non-significant effect of study year (F2, 17 = 0.64, p = 0.538). In females, the association turned out to be positive [Beta (SE) = 0.33 (0.15), F1, 37 = 4.55, p = 0.039], even after controlling for the non-significant effect of study year (F2, 37 = 1.22, p = 0.308; Figure 2A). The association between telomere shortening and throat feather length also differed for males and females (GLM, interaction between sex and telomere shortening, F1, 42 = 7.00, p = 0.010). These variables were negatively related but only in males [Males: Beta(SE) = −0.55 (0.18), F1, 17 = 9.62, p = 0.006; Females: Beta(SE) = 0.14 (0.17), F1, 34 = 0.73, p = 0.399; Figure 2B]. Neither time elapsed between telomere length measurements (F1, 17 = 3.34, p = 0.08), nor experimental treatment [control (N = 10): mean (SE) = 0.21 (0.12), experimental (N = 11): mean (SE) = −0.06 (0.11), F1, 17 = 2.74, p = 0.116] explained additional proportion of variance in telomere shortening in males. Thus, males with longer throat feathers tended to have shorter telomeres but experienced lower rates of telomere shortening, while the manipulation of throat feathers did not affect rate of telomere shortening.
Figure 2. Relationship between telomere length and the average length of the throat feathers in males and females (A), and between telomere shortening and the average length of the throat feathers in control (Ctrl. N = 10) and experimental (Exp. N = 11) males, and in female spotless starlings (B). Lines are regression lines.
Length of throat feathers of males before manipulation was positively associated with hatching success of second breeding attempts after controlling for the non-significant effect of study year (Table 1, Figure 3). Accordingly, the interaction between breeding attempt and throat feather length explained a significant proportion of variance in hatching success (F1, 95 = 4.63, p = 0.034). The interactions between breeding attempt and fledging success (F1, 67 = 0.63, p = 0.429) or number of fledglings (F1, 112 = 0.023, p = 0.879) resulted far from statistical significance. No other variable, including experimental treatment, explained a significance proportion of variance for any of the considered reproductive variables (Table 1).
Table 1. Effects of the experimental manipulation of throat-feather length in spotless starling males (Control males: Ctrl; Experimental males: Exp.) on date of reproduction, hatching success, fledging success, and total number of fledglingxs.
Figure 3. Relationship between average length of the throat feathers of males and hatching success for experimental (Exp.) and control (Ctrl.) spotless starling males in first (1st) (NCtrl = 32; NExp = 29) and second (2nd) (NCtrl = 21; NExp = 22) breeding attempts.
The feather length in subsequent molts of control [(N = 10): mean ± SE = 3.20 ± 0.11] and experimental [(N = 9): mean ± SE = 3.08 ± 0.12] males did not differ significantly (F1, 16 = 0.53, p = 0.476).
Our main results are that spotless starling males with longer throat feathers had shorter telomeres that deteriorate more slowly than those of males with shorter throat feathers. Experimental shortening of throat feathers of males did not affect telomere deterioration rate, suggesting that the correlative association was not due to feather length but to correlated variables, or that time elapsed between captures was not enough for feather length to exert an effect on telomere deterioration. The association between length of throat feathers and telomere length also appeared in females, but in the opposite direction than in males. These results therefore suggest a link between throat-feathers and telomere length and dynamics that differ between sexes. Moreover, we found a positive association between length of throat feathers of males and hatching success of second clutches. Again, experimental manipulation of throat-feather length did not influence hatching success suggesting that the detected association were not directly mediated by feather length. The experiment did not affect the mating and fitness of males, which could be due to individuals having already mated before the experiment was performed. Below we discuss such results in scenarios of sexual selection where feather length reflects phenotypic quality of males in terms of telomere length and/or attrition rates.
Older spotless starling males usually possess longer throat feathers (Hiraldo and Herrera, 1974; Aparicio et al., 2001; Veiga and Polo, 2016). Moreover, telomeres often deteriorate with age (Haussmann and Vleck, 2002; Haussmann et al., 2003; Pauliny et al., 2006). Thus, the detected negative association between feather length and telomere length might be simply due to length of throat feathers reflecting male age-related telomere shortening. Unfortunately, the exact age of most individuals included in this study is unknown, thus we are not able to test for the potential effect of this factor. Another non-exclusive possibility explaining this negative association is related to physiological costs derived from developing exaggerated ornaments that would imply elevated telomere deterioration. Extensive evidence suggests that high testosterone levels are required to develop exaggerated ornaments (Gonzalez et al., 2001; Fusani, 2008). Elevated testosterone levels imply considerable costs in terms of, for instance, immunosuppression (Folstad and Karter, 1992; Gil and Culver, 2011) and increased oxidative stress (Blas et al., 2006; Alonso-Alvarez et al., 2007) and only the more efficients individuals counteracting its associated larger immune suppressive costs could showed a healthy look (long feathers; (Folstad and Karter, 1992)). Oxidative stress is one of the better-known causes of telomere deterioration (Von Zglinicki T., 2002; Richter and von Zglinicki, 2007; Chatelain et al., 2019, but see Boonekamp et al., 2017; Reichert and Stier, 2017) and, thus, sexual ornamentation and telomere length might be negatively associated (Monaghan, 2014), a prediction that our results fulfilled.
A positive association between sexually selected characters and telomere length can also be predicted because males of higher phenotypic and/or genetic quality are better able to cope with costs associated with the expression of these traits (Andersson, 1994; Møller, 1994). In accordance with this last scenario, previous works exploring associations between sexually selected traits and telomere length in birds found positive rather than negative relationships (Parolini et al., 2017; Taff and Freeman-Gallant, 2017). In these cases, however, the considered secondary sexual trait did not change with age (Parolini et al., 2017; Taff and Freeman-Gallant, 2017), in contrast to length of throat feathers in starlings (Hiraldo and Herrera, 1974; Veiga and Polo, 2016). In addition, in accordance with sexually selected characteristics related to telomere length, Parolini et al. (2017) and Taff and Freeman-Gallant (2017) found that telomeres of males with more exaggerated sexual traits deteriorated at lower rates, a pattern that we also detected here. Telomere attrition conveys information on the capacity of individuals to maintain chromosome wholeness and, thus, it is a good predictor of survival and of maximum lifespan in birds (Tricola et al., 2018). Thus, since telomeres of starling males with longer throat feathers deteriorated at relatively lower rate, it is likely that the negative association between telomere and feather lengths was due to age being positively and negatively correlated with feather length and telomere length, respectively.
We experimentally reduced throat-feather length of male starlings, but found no effect in telomere deterioration. Throat feathers are not involved in flight activity but in social communication (Ruiz-Rodríguez et al., 2015). Thus, this result suggests that the association with telomere length and shortening should be mainly related to physiological pleiotropic effects rather than to social environment. Further studies should explore the role of physiological characteristics related to throat-feather length [e.g., immunocompetence (Gil and Culver, 2011)], which may predict telomere length and shortening. Different associations between sexually dimorphic characteristics and telomere dynamics of males and females have been reported (Parolini et al., 2017; Taff and Freeman-Gallant, 2017). Thus, it is likely that sexual hormones mediate the association between sexually selected traits and telomeres. Here, we found that length of throat feathers and telomere length of females were significantly related, but in the opposite direction that in males. Moreover, in females, telomere shortening was not predicted by throat-feather length. Throat feathers of females are 1 cm shorter than those of males, and they are not considered a sexually selected trait, at least to the same extent as in males (Ruiz-Rodríguez et al., 2015). It is therefore likely that development of these feathers in females does not depend on age or sexual hormones and, thus, its length would not be associated with testosterone provoking oxidative stress and being mirrored in their telomeres. In this case, throat-feather length might reflect phenotypic conditions of females at the molting time. Therefore, a positive rather than a negative association with telomere length could be expected as shown here.
Throat feathers of starling males are involved in sexual signaling (Aparicio et al., 2001; Gil and Culver, 2011; Ruiz-Rodríguez et al., 2015). Here, we found a positive association between throat-feather length and hatching success of second reproductive attempts, which support the expected reproductive values of the secondary sexual traits. This association was only detected for second clutches, which usually take place under poorer environmental conditions (as for example, an increase in temperature and a decrease in food availability) that might increase the probability of hatching failures (Salaberria et al., 2014). Four different non-exclusive scenarios might explain the detected association between ornamentation of males and hatching success. First, females might invest more in incubation duties when mated to attractive males (i.e., those with longer throat feathers) according to the differential allocation in relation to male ornamentation (Møller and Thornhill, 1998; Harris and Uller, 2009). However, the experimental reduction of throat-feather length did not cause a reduction of hatching success and, thus, feather length of males during the incubation period does not explain the detected association. The second possibility is that males with longer ornaments mated with females of higher genetic or phenotypic quality that improve hatching success. Previous studies in spotless starlings detected a positive association between hatching success and level of heterozygosity of females, but not of males (Cordero et al., 2004). Moreover, it is also known that males with longer throat feathers mate with highly heterozygous females (Aparicio et al., 2001). In our study, most experimental males were manipulated after mating with a female but before the female started the laying of the first eggs and thus, it is possible that heterozygosity of females already mated with experimental males explained the detected association between feather length and hatching success. A third possibility is that genetic characteristics of males rather than those of females, determine hatching success. These second and third explanations are however unlikely because the proposed genetic effects would have been detected not only in second but also in first breeding attempts. Finally, the fourth possibility explaining the detected association between ornamentation of males and hatching success is that highly ornamented males may be more helpful to incubate second clutches. Studies in European starlings have shown that monogamous males are more helpful in incubation than those with many females (Smith et al., 1995; Reid et al., 2002). Not all females in a population are able to lay a second clutch and, thus, opportunity of social polygyny of highly ornamented males is reduced during second reproductive attempts, which might result in clutches that are better incubated.
Summarizing, we found that the length of throat feathers, a sexually selected trait in male spotless starlings, reflects telomere length and possibly the age of individuals as well as their phenotypic quality in terms of preventing telomere deterioration. We also found a positive association between throat-feather length and hatching success, which might be related to particularities of females breeding with males with longer feathers, or to males of longer feathers investing differentially in the incubation of second clutches. Interestingly, the experimental reduction of throat-feather length did not produce a more pronounced telomere deterioration, nor a reduction in the fitness related variables considered. Therefore, the interesting detected associations might not be due to the physical appearance of throat feathers but, perhaps, to other physiological and/or non-manipulated morphological characteristics that covaried with them. Future studies should explore possible candidates that included antioxidant capability or related morphological signals.
Data used in this paper can be found in CSIC Institutional Repository, using the following link: http://dx.doi.org/10.20350/digitalCSIC/10526.
We performed the study following the relevant Spanish national (Decreto 142/2013, 1 de octubre) and regional guidelines. The ethics committee of the Spanish National Research Council (CSIC) approved and reviewed the animal study and the Consejería de Medio Ambiente de la Junta de Andalucía, Spain, provided all the necessary permits for nest and nestling manipulations (Ref: SGMN/GyB/JMIF). The protocols adhered to the ASAB/ABS Guidelines for the Use of Animals in Research. The authorization for carrying out the experimental work on animals in the field was granted by the Dirección General de Gestión del Medio Natural of the Consejería de Medio Ambiente of Junta de Andalucía.
JS, MR-R, GT, and MM-V: conceived and designed the experiments. MA-G with considerable assistance from JM and JF: performed molecular analyses. MA-G, JS, MR-R, GT, CR-C, and SD-L: fieldwork. TP-C: viewing and taking data from the videos. MA-G and JS: analyzed the data. Substantial contribution from all authors: contributed reagents, materials, analysis tools. MA-G wrote the first version with supervision of JS. All authors: substantially contributed to final version.
This work was supported by the currently named Ministerio de Ciencia, Innovación y Universidades and European (FEDER) funds (CGL2013-48193-C3-1-P, CGL2017-83103-P, CGL2017-89063-P, CGL2015-65055-P, and PGC2018-095704-B-100). MA-G was financed by a predoctoral contract (BES-2014-068661) from the Spanish Ministerio de Economía y Competitividad, and GT by the Ramón y Cajal Programme.
The authors declare that the research was conducted in the absence of any commercial or financial relationships that could be construed as a potential conflict of interest.
We thank Olga Corona Forero, Francisco Miranda, and Olaya García for technical assistance with DNA extraction from blood samples and amplification for telomere length estimation. We also thank Angela Martinez-Garcia, Natalia Juárez García-Pelayo, and Carmen Soler Zamora for the help in capturing birds and collecting data from the field.
The Supplementary Material for this article can be found online at: https://www.frontiersin.org/articles/10.3389/fevo.2019.00520/full#supplementary-material
Alonso-Alvarez, C., Bertrand, S., Faivre, B., Chastel, O., and Sorci, G. (2007). Testosterone and oxidative stress: the oxidation handicap hypothesis. Proc. R. Soc. Lond. B. Biol. Sci. 274, 819–825. doi: 10.1098/rspb.2006.3764
Aparicio, J. M., Cordero, P. J., and Veiga, J. P. (2001). A test of the hypothesis of mate choice based on heterozygosity in the spotless starling. Anim. Behav. 62, 1001–1006. doi: 10.1006/anbe.2001.1840
Asghar, M., Hasselquist, D., Hansson, B., Zehtindjiev, P., Westerdahl, H., and Bensch, S. (2015). Hidden costs of infection: chronic malaria accelerates telomere degradation and senescence in wild birds. Science 347, 436–438. doi: 10.1126/science.1261121
Asghar, M., Yman, V., Homann, M. V., Sonden, K., Hammar, U., Hasselquist, D., and Farnert, A. (2018). Cellular aging dynamics after acute malaria infection: A 12-month longitudinal study. Aging Cell 17:8. doi: 10.1111/acel.12702
Badás, E. P., Martínez, J., Rivero de Aguilar Cachafeiro, J., Miranda, F., Figuerola, J., and Merino, S. (2015). Ageing and reproduction: antioxidant supplementation alleviates telomere loss in wild birds. J. Evol. Biol. 28, 896–905. doi: 10.1111/jeb.12615
Bauch, C., Becker, P. H., and Verhulst, S. (2013). Telomere length reflects phenotypic quality and costs of reproduction in a long-lived seabird. Proc. R. Soc. Lond. B. Biol. Sci. 280:20122540. doi: 10.1098/rspb.2012.2540
Bauer, C. M., Graham, J. L., Abolins-Abols, M., Heidinger, B. J., Ketterson, E. D., and Greives, T. J. (2018). Chronological and biological age predict seasonal reproductive timing: an investigation of clutch initiation and telomeres in birds of known age. Am. Nat. 191, 777–782. doi: 10.1086/697224
Beaulieu, M., Reichert, S., Le Maho, Y., Ancel, A., and Criscuolo, F. (2011). Oxidative status and telomere length in a long-lived bird facing a costly reproductive event. Funct. Ecol. 25, 577–585. doi: 10.1111/j.1365-2435.2010.01825.x
Blackburn, E. H. (1991). Structure and function of telomeres. Nature 350, 569–573. doi: 10.1038/350569a0
Blas, J., Perez-Rodriguez, L., Bortolotti, G. R., Vinuela, J., and Marchant, T. A. (2006). Testosterone increases bioavailability of carotenoids: Insights into the honesty of sexual signaling. Proc. Natl. Acad. Sci. U.S.A. 103, 18633–18637. doi: 10.1073/pnas.0609189103
Blount, J. D., Metcalfe, N. B., Birkhead, T. R., and Surai, P. F. (2003). Carotenoid modulation of immune function and sexual attractiveness in zebra finches. Science 300, 125–127. doi: 10.1126/science.1082142
Boonekamp, J. J., Bauch, C., Mulder, E., and Verhulst, S. (2017). Does oxidative stress shorten telomeres? Biol. Lett. 13:20170164. doi: 10.1098/rsbl.2017.0164
Boonekamp, J. J., Mulder, G. A., Salomons, H. M., Dijkstra, C., and Verhulst, S. (2014). Nestling telomere shortening, but not telomere length, reflects developmental stress and predicts survival in wild birds. Proc. R. Soc. Lond. B. Biol. Sci. 281:20133287. doi: 10.1098/rspb.2013.3287
Bradbury, J. W., and Vehrencamp, S. L. (1998). Principles of Animal Communication. Sunderland, MA: Sinauer Associates, Inc.
Burley, N. (1988). The differential-allocation hypothesis: an experimental test. Am. Nat. 132, 611–628. doi: 10.1086/284877
Campisi, J. (2013). Aging, cellular senescence, and cancer. Annu. Rev. Physiol. 75, 685–705. doi: 10.1146/annurev-physiol-030212-183653
Chatelain, M., Drobniak, S. M., and Szulkin, M. (2019). The association between stressors and telomeres in non-human vertebrates: a meta-analysis. Ecol. Lett. 23, 381–398. doi: 10.1111/ele.13426
Cordero, P. J., Aparicio, J. M., and Veiga, J. P. (2004). Parental genetic characteristics and hatching success in the spotless starling, Sturnus unicolor. Anim. Behav. 67, 637–642. doi: 10.1016/j.anbehav.2003.06.005
Creel, S. (2001). Social dominance and stress hormones. Trends Ecol. Evol. 16, 491–497. doi: 10.1016/S0169-5347(01)02227-3
Criscuolo, F., Bize, P., Nasir, L., Metcalfe, N. B., Foote, C. G., Griffiths, K., Gault, E. A., and Monaghan, P. (2009). Real-time quantitative PCR assay for measurement of avian telomeres. J. Avian Biol. 40, 342–347. doi: 10.1111/j.1600-048X.2008.04623.x
Dugdale, H. L., and Richardson, D. S. (2018). Heritability of telomere variation: it is all about the environment! Philos. Trans. R. Soc. Lond. B Biol. Sci. 373:14. doi: 10.1098/rstb.2016.0450
Eisenberg, D. T. A., Borja, J. B., Hayes, M. G., and Kuzawa, C. W. (2017). Early life infection, but not breastfeeding, predicts adult blood telomere lengths in the Philippines. Am. J. Human Biol. 29:11. doi: 10.1002/ajhb.22962
Ferraguti, M., Martinez-de la Puente, J., Ruiz, S., Soriguer, R., and Figuerola, J. (2013). On the study of the transmission networks of blood parasites from SW Spain: diversity of avian haemosporidians in the biting midge Culicoides circumscriptus and wild birds. Parasites Vectors 6:208. doi: 10.1186/1756-3305-6-208
Folstad, I., and Karter, A. J. (1992). Parasites, bright males, and the immunocompetence handicap. Am. Na. 139, 603–622. doi: 10.1086/285346
Fusani, L. (2008). Testosterone control of male courtship in birds. Horm. Behav. 54, 227–233. doi: 10.1016/j.yhbeh.2008.04.004
Gil, D., and Culver, R. (2011). Male ornament size in a passerine predicts the inhibitory effect of testosterone on macrophage phagocytosis. Funct. Ecol. 25, 1278–1283. doi: 10.1111/j.1365-2435.2011.01878.x
Gonzalez, G., Sorci, G., Smith, L. C., and de Lope, F. (2001). Testosterone and sexual signalling in male house sparrows (Passer domesticus). Behav. Ecol. Sociobiol. 50, 557–562. doi: 10.1007/s002650100399
Harris, W. E., and Uller, T. (2009). Reproductive investment when mate quality varies: differential allocation versus reproductive compensation. Philos. Trans. R. Soc. Lond. B Biol. Sci. 364, 1039–1048. doi: 10.1098/rstb.2008.0299
Haussmann, M. F., and Vleck, C. M. (2002). Telomere length provides a new technique for aging animals. Oecologia 130, 325–328. doi: 10.1007/s00442-001-0827-y
Haussmann, M. F., Winkler, D. W., O'Reilly, K. M., Huntington, C. E., Nisbet, I. C. T., and Vleck, C. M. (2003). Telomeres shorten more slowly in long-lived birds and mammals than in short-lived ones. Proc. R. Soc. Lond. B. Biol. Sci. 270, 1387–1392. doi: 10.1098/rspb.2003.2385
Hellemans, J., Mortier, G., De Paepe, A., Speleman, F., and Vandesompele, J. (2007). qBase relative quantification framework and software for management and automated analysis of real-time quantitative PCR data. Genome Biol. 8, 1–14. doi: 10.1186/gb-2007-8-2-r19
Herborn, K. A., Heidinger, B. J., Boner, W., Noguera, J. C., Adam, A., Daunt, F., and Monaghan, P. (2014). Stress exposure in early post-natal life reduces telomere length: an experimental demonstration in a long-lived seabird. Proc. R. Soc. Lond. B. Biol. Sci. 281:3151. doi: 10.1098/rspb.2013.3151
Hill, G. E. (2000). Energetic constraints on expression of carotenoid-based plumage coloration. J. Avian Biol. 31, 559–566. doi: 10.1034/j.1600-048X.2000.310415.x
Hiraldo, F., and Herrera, C. M. (1974). Dimorfismo sexual y diferenciación de edades en Sturnus unicolor Temm. Doñana. Acta Vert. 1, 149–170.
Johnson, S., and Candolin, U. (2017). Predation cost of a sexual signal in the threespine stickleback. Behav. Ecol. 28, 1160–1165. doi: 10.1093/beheco/arx080
Jones, A. G., and Ratterman, N. L. (2009). Mate choice and sexual selection: what have we learned since Darwin? Proc. Natl. Acad. Sci. U.S.A. 106:10001–10008. doi: 10.1073/pnas.0901129106
Kim, S. Y., and Velando, A. (2015). Antioxidants safeguard telomeres in bold chicks. Biol. Lett. 11:211. doi: 10.1098/rsbl.2015.0211
Kotrschal, A., Ilmonen, P., and Penn, D. J. (2007). Stress impacts telomere dynamics. Biol. Lett. 3, 128–130. doi: 10.1098/rsbl.2006.0594
Macías-García, C., and Ramírez, E. (2005). Evidence that sensory traps can evolve into honest signals. Nature 434, 501–505. doi: 10.1038/nature03363
Møller, A. P., Chabi, Y., Cuervo, J. J., Lope, F., Kilpimaa, J., Kose, M., et al. (2006). An analysis of continent-wide patterns of sexual selection in a Passerine bird. Evolution 60, 856–868. doi: 10.1111/j.0014-3820.2006.tb01162.x
Møller, A. P., and Jennions, M. D. (2001). How important are direct fitness benefits of sexual selection? Naturwissenschaften 88, 401–415. doi: 10.1007/s001140100255
Møller, A. P., and Thornhill, R. (1998). Male parental care, differential parental investment by females and sexual selection. Anim. Behav. 55, 1507–1515. doi: 10.1006/anbe.1998.0731
Monaghan, P. (2014). Organismal stress, telomeres and life histories. J. Exp. Biol. 217, 57–66. doi: 10.1242/jeb.090043
Monaghan, P., and Haussmann, M. F. (2006). Do telomere dynamics link lifestyle and lifespan? Trends Ecol. Evol. 21, 47–53. doi: 10.1016/j.tree.2005.11.007
Navarro, C., Pérez-Contreras, T., Avilés, J. M., McGraw, K. J., and Soler, J. J. (2010). Beak colour reflects circulating carotenoid and vitamin A levels in spotless starlings (Sturnus unicolor). Behav. Ecol. Sociobiol. 64, 1057–1067. doi: 10.1007/s00265-010-0920-5
Otte, D. (1974). Effects and functions in the evolution of signaling systems. Ann. Rev. Ecol. Syst. 5, 385–417. doi: 10.1146/annurev.es.05.110174.002125
Pardal, S., Alves, J. A., Mota, P. G., and Ramos, J. A. (2018). Dressed to impress: breeding plumage as a reliable signal of innate immunity. J. Avian Biol. 49:13. doi: 10.1111/jav.01579
Parolini, M., Romano, A., Costanzo, A., Khoriauli, L., Santagostino, M., Nergadze, S. G., et al. (2017). Telomere length is reflected by plumage coloration and predicts seasonal reproductive success in the barn swallow. Mol. Ecol. 26, 6100–6109. doi: 10.1111/mec.14340
Pauliny, A., Wagner, R. H., Augustin, J., Szép, T., and Blomqvist, D. (2006). Age-independent telomere length predicts fitness in two bird Species. Mol. Ecol. 15, 1681–1687. doi: 10.1111/j.1365-294X.2006.02862.x
Pérez-Rodríguez, L., Redondo, T., Ruiz-Mata, R., Camacho, C., Moreno-Rueda, G., and Potti, J. (2019). Vitamin E supplementation–but not induced oxidative stress-influences telomere dynamics during early development in wild passerines. Front. Ecol. Evol. 7:173. doi: 10.3389/fevo.2019.00173
Reichert, S., and Stier, A. (2017). Does oxidative stress shorten telomeres in vivo? a review. Biol. Lett. 13:7. doi: 10.1098/rsbl.2017.0463
Reid, J. M., Monaghan, P., and Ruxton, G. D. (2002). Males matter: the occurrence and consequences of male incubation in starlings (Sturnus vulgaris). Behav. Ecol. Sociobiol. 51, 255–261. doi: 10.1007/s00265-001-0435-1
Richter, T., and von Zglinicki, T. (2007). A continuous correlation between oxidative stress and telomere shortening in fibroblasts. Exp. Gerontol. 42, 1039–1042. doi: 10.1016/j.exger.2007.08.005
Romano, A., Costanzo, A., Rubolini, D., Saino, N., and Moller, A. P. (2017). Geographical and seasonal variation in the intensity of sexual selection in the barn swallow Hirundo rustica: a meta-analysis. Biol. Rev. 92, 1582–1600. doi: 10.1111/brv.12297
Ruiz-Castellano, C., Tomás, G., Ruiz-Rodríguez, M., Martín-Galvez, D., and Soler, J. J. (2016). Nest material shapes eggs bacterial environment. PLoS ONE 11:e0148894. doi: 10.1371/journal.pone.0148894
Ruiz-Rodríguez, M., Tomás, G., Martín-Gálvez, D., Ruiz-Castellano, C., and Soler, J. J. (2015). Bacteria and the evolution of honest signals. The case of ornamental throat feathers in spotless starlings. Funct. Ecol. 29, 701–709. doi: 10.1111/1365-2435.12376
Salaberria, C., Celis, P., Lopez-Rull, I., and Gil, D. (2014). Effects of temperature and nest heat exposure on nestling growth, dehydration and survival in a Mediterranean hole-nesting passerine. Ibis 156, 265–275. doi: 10.1111/ibi.12121
Searcy, W. A., and Nowicki, S. (2005). The Evolution of Animal Communication. Reliability and Deception in Signaling Systems. Princeton, NJ: Princeton University Press.
Sheldon, B. C. (2000). Differential allocation: tests, mechanisms and implications. Trends Ecol. Evol. 15, 397–402. doi: 10.1016/S0169-5347(00)01953-4
Smith, H. G., Sandell, M. I., and Bruun, M. (1995). Paternal care in the European starling, Sturnus vulgaris: incubation. Anim. Behav. 50, 323–331. doi: 10.1006/anbe.1995.0248
Soler, J. J., Navarro, C., Pérez-Contreras, T., Avilés, J. M., and Cuervo, J. J. (2008). Sexually selected egg coloration in spotless starlings. Am. Na. 171, 183–194. doi: 10.1086/524958
Soler, J. J., Ruiz Castellano, C., Martínez-de la Puente, J., Tomás, G., Ruiz-Rodríguez, M., and Figuerola, J. (2015). Telomere dynamics in parasitic great spotted cuckoos and their magpie hosts. J. Evol. Biol. 28, 1610–1617. doi: 10.1111/jeb.12680
Soler, J. J., Ruiz-Castellano, C., Figuerola, J., Martinez-de la Puente, J., Ruiz-Rodriguez, M., and Tomas, G. (2018). Egg colouration predicts brood size, telomere length and body condition of spotless starling fledglings. J. Avian Biol. 49:12. doi: 10.1111/jav.01686
Soler, J. J., Ruiz-Castellano, C., Figuerola, J., Martin-Vivaldi, M., Martinez-de la Puente, J., Ruiz-Rodriguez, M., et al. (2017). Telomere length and dynamics of spotless starling nestlings depend on nest-building materials used by parents. Anim. Behav. 126, 89–100. doi: 10.1016/j.anbehav.2017.01.018
Spurgin, L. G., Bebbington, K., Fairfield, E. A., Hammers, M., Komdeur, J., Burke, T., et al. (2018). Spatio-temporal variation in lifelong telomere dynamics in a long-term ecological study. J. Anim. Ecol. 87, 187–198. doi: 10.1111/1365-2656.12741
Stuart-Fox, D. M., Moussalli, A., Marshall, N. J., and Owens, I. P. F. (2003). Conspicuous males suffer higher predation risk: visual modelling and experimental evidence from lizards. Anim. Behav. 66, 541–550. doi: 10.1006/anbe.2003.2235
Suram, A., Kaplunov, J., Patel, P. L., Ruan, H. H., Cerutti, A., Boccardi, V., et al. (2012). Oncogene-induced telomere dysfunction enforces cellular senescence in human cancer precursor lesions. EMBO J. 31, 2839–2851. doi: 10.1038/emboj.2012.132
Taff, C. C., and Freeman-Gallant, C. R. (2017). Sexual signals reflect telomere dynamics in a wild bird. Ecol. Evol. 7, 3436–3442. doi: 10.1002/ece3.2948
Tibbetts, E. A., and Dale, J. (2004). A socially enforced signal of quality in a paper wasp. Nature 432, 218–222. doi: 10.1038/nature02949
Tricola, G. M., Simons, M. J. P., Atema, E., Boughton, R. K., Brown, J. L., Dearborn, D. C., et al. (2018). The rate of telomere loss is related to maximum lifespan in birds. Philos. Trans. R. Soc. Lond. B Biol. Sci. 373:11. doi: 10.1098/rstb.2016.0445
Veiga, J., and Polo, P. (2016). “Estornino negro–Sturnus unicolor,” in: Enciclopedia Virtual de los Vertebrados Españoles, eds A. Salvador, and M. B. Morales (Madrid: Museo Nacional de Ciencias Naturales).
Von Zglinicki, T. (2002). Oxidative stress shortens telomeres. Trends Biochem. Sci. 27, 339–344. doi: 10.1016/S0968-0004(02)02110-2
Webster, M. S., Ligon, R. A., and Leighton, G. M. (2018). Social costs are an underappreciated force for honest signalling in animal aggregations. Anim. Behav. 143, 167–176. doi: 10.1016/j.anbehav.2017.12.006
Wingfield, J. C., and Silverin, B. (2002). “Ecophysiological studies of hormone-behavior relations in birds,” in Hormones, Brain and Behavior, Vol. 2, eds D. W. Pfaff, A. P. Arnold, A. M. Etgen, S. E. Fahrbach, and R. T. Rubin (Amsterdam: Elsevier Science), 587–647. doi: 10.1016/B978-012532104-4/50033-0
Zahavi, A. (1975). Mate selection–a selection for a handicap. J. Theor. Biol. 53, 205–214. doi: 10.1016/0022-5193(75)90111-3
Keywords: hatching success, sexual selection, sexual signaling, Sturnus unicolor, telomere dynamics, throat-feathers length
Citation: Azcárate-García M, Ruiz-Rodríguez M, Díaz-Lora S, Ruiz-Castellano C, Martín-Vivaldi M, Figuerola J, Martínez-de la Puente J, Tomás G, Pérez-Contreras T and Soler JJ (2020) Ornamental Throat Feathers Predict Telomere Dynamic and Hatching Success in Spotless Starling (Sturnus unicolor) Males. Front. Ecol. Evol. 7:520. doi: 10.3389/fevo.2019.00520
Received: 23 September 2019; Accepted: 20 December 2019;
Published: 22 January 2020.
Edited by:
François Criscuolo, Centre National de la Recherche Scientifique (CNRS), FranceReviewed by:
Allison Cornell, Cedar Crest College, United StatesCopyright © 2020 Azcárate-García, Ruiz-Rodríguez, Díaz-Lora, Ruiz-Castellano, Martín-Vivaldi, Figuerola, Martínez-de la Puente, Tomás, Pérez-Contreras and Soler. This is an open-access article distributed under the terms of the Creative Commons Attribution License (CC BY). The use, distribution or reproduction in other forums is permitted, provided the original author(s) and the copyright owner(s) are credited and that the original publication in this journal is cited, in accordance with accepted academic practice. No use, distribution or reproduction is permitted which does not comply with these terms.
*Correspondence: Manuel Azcárate-García, bWF6Y2FyYXRlZ2FyY2lhQGdtYWlsLmVz
Disclaimer: All claims expressed in this article are solely those of the authors and do not necessarily represent those of their affiliated organizations, or those of the publisher, the editors and the reviewers. Any product that may be evaluated in this article or claim that may be made by its manufacturer is not guaranteed or endorsed by the publisher.
Research integrity at Frontiers
Learn more about the work of our research integrity team to safeguard the quality of each article we publish.