- 1Otago Palaeogenetics Laboratory, Department of Zoology, University of Otago, Dunedin, New Zealand
- 2Canterbury Museum, Christchurch, New Zealand
- 3National Museum of New Zealand Te Papa Tongarewa, Wellington, New Zealand
- 4Biodiversity Group, Department of Conservation, Christchurch, New Zealand
- 5Department of Zoology, University of Otago, Dunedin, New Zealand
Museum specimens provide a record of past species distribution and are an increasingly important resource for conservation genetic research. The scientific value of these specimens depends upon the veracity of their associated data and can be compromised by inaccurate details; including taxonomic identity, collection locality, and collector. New Zealand contains many endemic species that have been driven to extinction or reduced to relict distributions following the arrival of humans and mammalian predators, including the Acanthisittid wrens (of which only two of the eight described species presently persist). One of these is the New Zealand rock wren (Xenicus gilviventris), currently classified as an endangered species and experiencing ongoing population declines. Here we analyze ancient DNA retrieved from New Zealand rock wren museum skins to establish the veracity of their recorded collection localities—New Zealand rock wrens exhibit strong north-south genetic structuring along the Southern Alps of New Zealand's South Island. We include the only specimen reportedly collected from New Zealand's North Island, outside the known range of New Zealand rock wrens, specimens collected by Henry Hamersley Travers, a collector known for poor record keeping and potentially fraudulent specimen data, and type specimens of proposed Xenicus taxa. Multiple instances of inaccurate collection locality were detected, including that of the New Zealand rock wren reportedly collected from the North Island, which matches individuals from the southern South Island. Syntypes of X. haasti, and a syntype of X. gilviventris clustered with individuals belonging to the northern New Zealand rock wren lineage. Our results suggest that New Zealand rock wrens have not been historically extirpated from New Zealand's North Island, and that caution must be taken when utilizing museum specimens to inform conservation management decisions. Additionally, we describe the type locality of both X. gilviventris and X. haasti, with genetic and historical evidence suggesting that the specimens used to describe these taxa were collected from the headwaters of the Rakaia River. This study demonstrates that ancient DNA analysis can add value to museum specimens by revealing incorrect specimen data and inform the conservation management and taxonomy of endangered species.
Introduction
Museum specimens constitute a valuable resource for conservation focused research, providing a record of past species' distributions (Shaffer et al., 1998). This record can be used to inform present-day conservation management of biodiversity. However, the reliability of this record can be compromised by inaccurate (or non-existent) data (e.g., collection locality or taxonomic status) due to poor record keeping, or deliberate falsification (Boessenkool et al., 2009). Detection of unreliable data can often be difficult if the only information readily available are the collector's notes (Barbanera et al., 2016), with specimen labels prone to error (Winker, 2000). The majority of errors concern taxonomic identity or collection locality, and can lead to misconceptions about a species' distribution (Graham et al., 2004). Advances in DNA extraction techniques and sequencing technologies have facilitated the use of historical museum specimens in conservation genetic studies (Wandeler et al., 2007). Retrieval of ancient DNA (aDNA) from such specimens can be used to examine temporal changes in genetic diversity (Thomas et al., 1990), test for congruence between collection locality and genetic data (Boessenkool et al., 2009), determine the provenance of specimens for which locality data are lacking (Shepherd et al., 2013), and assign mislabeled specimens to the correct taxonomic unit (Rawlence et al., 2014a).
Ancient DNA analysis is also an important tool for the conservation of biodiversity, as knowledge of past biodiversity, geographic range expansions/contractions, and the factors that lead to population declines or extinctions is crucial for making informed management decisions (Leonard, 2008; Grealy et al., 2017). One potential tool available to conservation managers are species re-introductions, a form of species translocation whereby individuals of a particular species are intentionally translocated to an area of their former distribution in order to establish a new population and re-introduce the species to their former range (IUCN, 2013). To determine whether a species translocation constitutes a re-introduction, or a novel introduction (the introduction of a species to an area outside their known range) an accurate record of the past and present distribution of the species is key. Cooper et al. (1996) used aDNA to demonstrate that the Laysan duck (Anas laysanensis), historically known only from Laysan Island, Hawaii, was formerly widespread throughout the Hawaiian Islands and argued for the re-introduction of the anatid to its former range. Species re-introductions often form an integral part of conservation management plans for isolated island ecosystems like Hawaii and New Zealand, as many species have been driven to extinction or reduced to relict populations following anthropogenic impact (Olson and James, 1982; Holdaway, 1989).
The New Zealand rock wren (hereafter NZ rock wren; Xenicus gilviventris) is a small alpine passerine, and one of only two extant members of the endemic New Zealand wrens (Acanthisittidae), the sister taxa to all other passerines (Barker et al., 2004; Hackett et al., 2008; Jarvis et al., 2014). NZ rock wrens have decreased in both range and abundance over the past 100 years (Michelsen-Heath and Gaze, 2007), and are currently threatened with extinction, primarily due to predation by introduced stoats (Mustela erminea) (Little et al., 2017; O'Donnell et al., 2017; Weston et al., 2018). Presently, NZ rock wrens are only found within alpine habitat throughout mountainous regions of New Zealand's South Island (Gill et al., 2010). Subfossil Xenicus remains [attributable to either rock wren or the congeneric bush wrens (X. longipes subspp.)] are known from throughout the North and South Islands of New Zealand, but have not been identified to species level (Worthy and Holdaway, 2002). It is currently unknown whether NZ rock wrens once inhabited the North Island. A study of present-day NZ rock wren phylogeography (covering the known geographical range of the species) revealed strong phylogeographic structure in both mitochondrial and nuclear (microsatellite) DNA, with two highly divergent northern and southern lineages which diverged two million years ago with minimal gene flow; the contact zone occurring in the central region of the South Island's Southern Alps near Aoraki/Mount Cook (Weston and Robertson, 2015). Individuals from southern Fiordland (Lake Roe and Lake MacArthur) also formed a monophyletic clade within the southern lineage (Weston and Robertson, 2015). Intriguingly, NZ rock wrens from southern Fiordland have been described previously as a separate subspecies (X. gilviventris rineyi) on the basis of morphological (Falla, 1953) and behavioral (Riney, 1953) characteristics, although this subspecies status has never been widely accepted (Gill et al., 2010; Weston and Robertson, 2015). No genetic analysis of the type series specimens has been undertaken, and it is unknown whether the specimens used to describe this subspecies belong to the same genetic clade as contemporary individuals from the same locality.
The NZ rock wren was originally described as X. gilviventris in 1867 by August Pelzeln, and later described as X. haasti by Sir Walter Buller in 1869. Both taxa were described using specimens collected by Julius Haast in the 1860s (Buller, 1869; Naturhistorisches Museum Wien, unpublished archive), however, the collection localities of these specimens are either unknown (for X. gilviventris) or vague (listed as “alpine heights of the South Island” for X. haasti; Buller, 1869; see also Tennyson and Bartle, 2008; Gill et al., 2010). Historical evidence suggests these specimens may have been collected from the headwaters of the Rakaia River (Naturhistorisches Museum Wien, unpublished archive). Genetic analysis of these specimens could be used to lend supporting evidence to this theory as this area is suitably north of the hybrid zone that specimens collected from this area are likely to belong to the northern NZ rock wren lineage.
The Natural History Museum at Tring (NHMUK) holds a purported NZ rock wren skin labeled as collected from the “Rimutaka” [= Remutaka] Ranges in the lower North Island prior to the 1930s (NHMUK 1939.12.9.75), outside the current distribution of NZ rock wren. The precise collection date, and collector, are unknown. This specimen was once part of Lord Walter Rothschild's collections, a portion of which were donated to NHMUK upon his death in 1937. Much of Rothschild's collection, including other NZ rock wren specimens, was sold to the American Museum of Natural History (AMNH) in 1932. If the taxonomic identity and collection data for this specimen is correct, then NZ rock wrens once occurred in the North Island and have since been extirpated from the region. As such, a translocation of NZ rock wren to the Remutaka Ranges would constitute a species re-introduction to their former range. Alternatively, NHMUK 1939.12.9.75 may not be a genuine NZ rock wren specimen and could potentially be the morphologically similar NZ bush wren, perhaps the North Island subspecies (X. l. stokesii) which possibly persisted within the Wellington region until 1918 (Stidolph, 1926). These two species are thought to have diverged approximately 15 Myrs ago (Mitchell et al., 2016). We would expect to see significant genetic divergence between NHMUK 1939.12.9.75 and the South Island lineages of NZ rock wrens if the taxonomic identity and collection locality of NHMUK 1939.12.9.75 are correct, given the presence of multiple avian taxa endemic to either the North or South Island (e.g., Trewick, 1996; Miller and Lambert, 2006; Murphy et al., 2006; Grosser et al., 2017).
However, even if the taxonomic identity of NHMUK 1939.12.9.75 is correct, its collection locality data may not be accurate. Erroneously-labeled yellow-eyed penguin (Megadyptes antipodes) specimens have been identified within the Lord Walter Rothschild collection held by the AMNH (Boessenkool et al., 2009). These specimens were reportedly collected from the sub-Antarctic Auckland and Campbell Islands by Henry Hammersley Travers (H. H. Travers) before being sold to Rothschild. Analysis of genetic microsatellites strongly suggest that these specimens originate from the South Island of New Zealand (Boessenkool et al., 2009). Additionally, H. H. Travers was involved with the mislabeling of South Island snipe (Coenocorypha iredalei) skins, including specimens within the AMNH Rothschild collection (Miskelly, 2012). As a result, Travers was probably falsely credited with the discovery of South Island snipe, as it is likely that he never visited the type locality of C. iredalei and that the type specimens were sourced from an unknown collector by H. H. Travers, before being sold to Lord Rothschild (Miskelly, 2012). Finally, it has also been suggested that Sir Walter Buller, who sold avian specimens to Rothschild (Bartle and Tennyson, 2009), mislabeled parts of his personal collection upon sale, with the misidentification of two Auckland Island shags (Leucocarbo colensoi) attributed to poor record keeping (Rawlence et al., 2014a). NHMUK 1939.12.9.75 could potentially have been supplied to Rothschild by a dealer such as Travers or Buller.
Here we use aDNA extracted from NZ rock wren specimens, and the strong phylogeographic structure present within NZ rock wrens, to infer their taxonomic identity and the accuracy of their recorded collection localities. We include the purported NZ rock wren recorded as collected from the Remutaka Ranges, to determine whether NZ rock wren historically inhabited the North Island of New Zealand. Type specimens of the putative NZ rock wren subspecies X. gilviventris rineyi were also examined to establish whether they belong to the same genetic clade as contemporary individuals from the same locality. Syntypes of both X. gilviventris and X. haasti were analyzed to ascertain the plausibility that they were collected from the headwaters of the Rakaia River. Finally, individuals collected throughout the late 1800s–early 1900s, many of which were associated with incomplete collection data, or obtained by potentially unreliable collectors, were examined to establish the veracity of their associated collection localities.
Methods
Ancient DNA Extraction, Amplification, and Sequencing
A total of 31 NZ rock wren museum skins were sampled from multiple institutions (Table 1, Figure 1). A clean scalpel blade was used to remove a single toepad from each specimen, with gloves and face-mask worn throughout sampling. A new scalpel blade and gloves were used for each specimen to minimize inter-sample contamination and contamination via exogenous DNA. All DNA extractions and PCR setup were performed in a dedicated, physically isolated, aDNA laboratory (Otago Palaeogenetics Laboratory) following strict aDNA guidelines (see Cooper and Poinar, 2000; Fulton and Shapiro, 2019). No Xenicus specimens had been analyzed within this laboratory prior to this study. Ancient DNA extractions followed the methodology of Thomas et al. (2017) whereby toepad samples were minced using a scalpel blade and incubated at 55°C overnight within 1 mL of the extraction buffer of Gilbert et al. (2007); followed by purification and elution of DNA according to Dabney et al. (2013). Negative DNA extraction controls (i.e., reagents only, no sample) were processed alongside museum specimens (~1 control per 7 specimens), and subjected to the same PCR conditions detailed below (as were negative PCR controls).
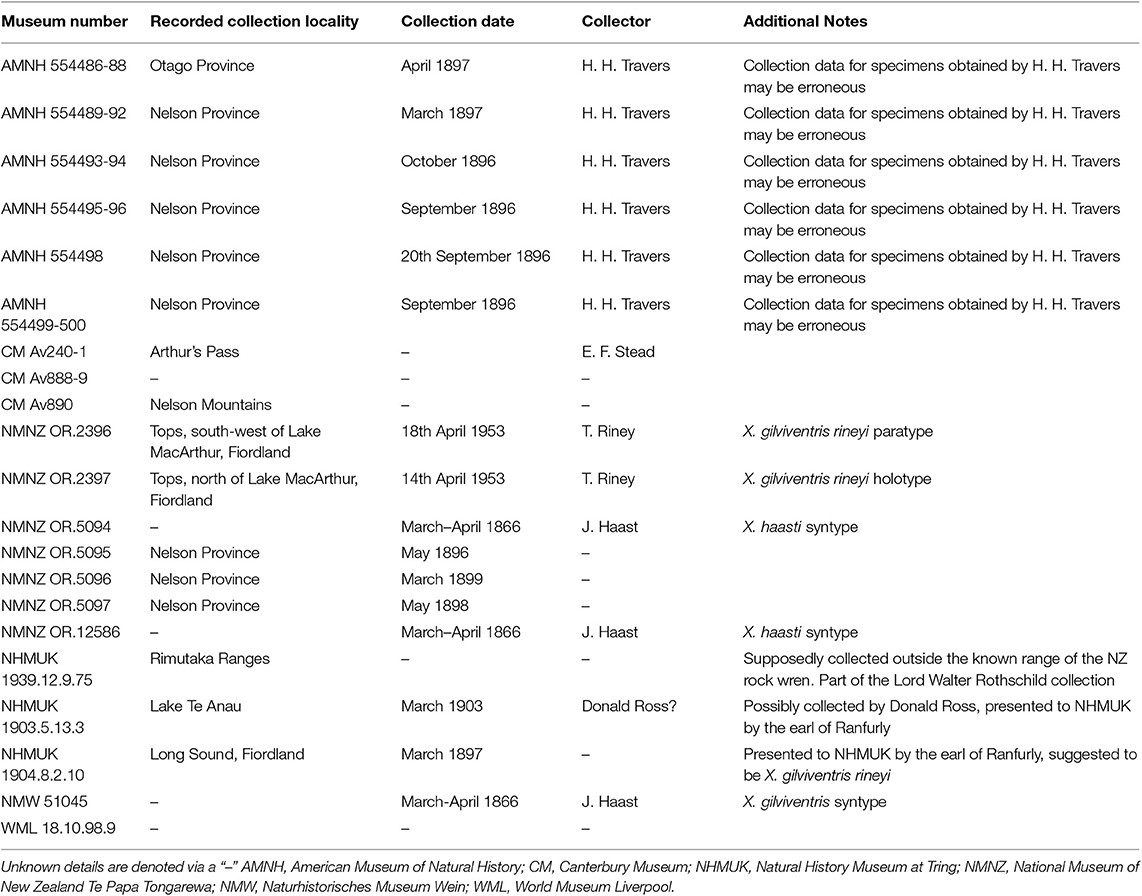
Table 1. Registration and collection information of the New Zealand rock wren (Xenicus gilviventris) museum specimens examined by this study.
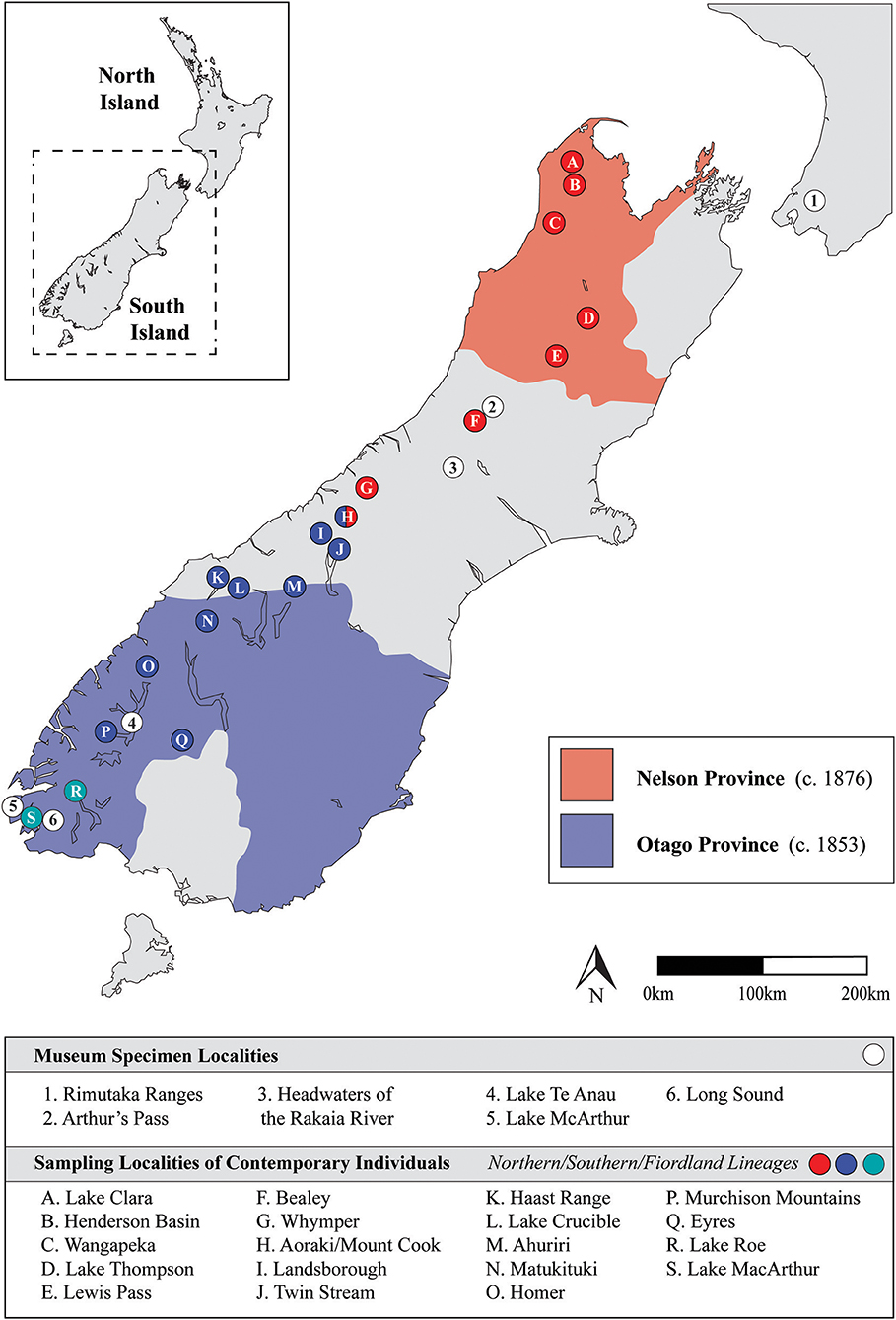
Figure 1. Map of New Zealand denoting relevant regions and localities. The historic Otago and Nelson province boundaries are illustrated, collection localities for museum specimens are indicated via numbered circles, while sampling localities for contemporary individuals (see Weston and Robertson, 2015) are indicated via lettered circles.
The mitochondrial cytochrome b (Cyt B) dataset of Weston and Robertson (2015), and the Cyt B sequences of partial NZ rock and bush wren mitochondrial genomes from Mitchell et al. (2016) (KX369035.1 and KX369033.1), were aligned and used to design PCR primer pairs that would amplify 172 bp of Cyt B, in two small overlapping fragments (overlap = 45 bp including primers, 5 bp sans primers), of NZ bush and rock wrens: Xenicus_cytb_Frag1F (5′-ATCCTAGTCCTCTTCCTCAG-3′) and Xenicus_cytb_Frag1R (5′-CTCCCGATTCATGTGAGGAT-3′) (128 bp including primers), and Xenicus_cytb_Frag2F (5′-TTGAATCCTAATCTCCAACC-3′) and Xenicus_cytb_Frag2R (5′-ATGGGGAATAGGATTAGGAG-3′) (129 bp including primers). The NZ rock wren control region (CR) dataset of Weston and Robertson (2015) was used to design primer pairs that would amplify a 280 bp portion of the NZ rock wren mitochondrial control region, in two small overlapping fragments (overlap = 62 bp including primers, 15 bp sans primers): RW_CR_Frag1F (5′-AAATTATGTCCACGCTTGC-3′) and RW_CR_Frag1R (5′-GGTGTATTTTGGTRGATCATTGG-3′) (205 bp including primers), and RW_CR_Frag2F (5′-CTGATTAYTATAACARTCCTACC-3′) with RW_CR_Frag2R (5′-GATGACAATATTTGTCCTGC-3′) (175 bp including primers). Within the 15 bp overlap between the two CR amplicons there are three fixed single nucleotide polymorphisms (SNPs) that distinguish the northern, southern, and Fiordland clades of NZ rock wren.
Each PCR (20 μL) contained 2 μL of undiluted or 1:10 diluted DNA, 0.25 μM of each primer, 0.63 mM dNTPs, 4.0 mM MgCl2, 1 M Betaine, 1 X PCR Buffer II (100 mM Tris-HCl, pH 8.3, 500 mM KCl), and 2 U AmpliTaq Gold (Life Technologies), made up to a total volume of 20 μL with UltraPure double-distilled water (ThermoFisher Scientific). PCR thermocycling conditions were 94°C for 5 min, 60 cycles of 94°C 30 s, 56°C 45 s, 72°C 60 s, and a final extension step of 72°C for 10 min. Unsuccessful PCRs were repeated using lower annealing temperatures (52–54°C). PCR amplification and downstream processes were carried out within a modern genetics laboratory. No modern Xencius specimens had been analyzed in this laboratory. Successful PCRs were identified via gel electrophoresis on a 2% agarose gel stained with SYBR safe (Thermo Fisher Scientific), visualized under blue light. Positive PCR products were purified using ExoSAP (1.5 U Exo1, 1 U SAP; GE Healthcare) following the manufacturer's instructions, then sequenced bidirectionally at the University of Otago using Big Dye terminator technology on an ABI 3730xl. When an inconsistency between sequences from an individual was observed (likely due to post-mortem DNA damage, i.e., G–A and C–T transitions; Hofreiter et al., 2001) additional PCRs and bidirectional sequencing were conducted, and a majority-rule consensus applied to the independent replicates (Brotherton et al., 2007; Winters et al., 2011). PCRs and DNA sequencing were also replicated for type specimens and specimens that exhibited discrepancies between collection locality and genetic sequence.
Phylogenetic Analysis
DNA sequences were checked by eye, and aligned against the Cyt B and CR datasets of Weston and Robertson (2015) and Mitchell et al. (2016), within Geneious v11.0.5 (Kearse et al., 2012) using the Geneious alignment algorithm with default parameters. The CR and Cyt B datasets were trimmed to 284 and 163 bp, respectively. The 163 bp alignment of Cyt B contains 14 variable sites, 7 of which are parsimony informative. The Cyt B dataset was primarily used to distinguish between NZ rock and bush wrens to determine the taxonomic identity of the museum specimens analyzed (particularly NHMUK 1939.12.9.75). While the 285 bp CR alignment contains 60 variable sites, with 33 of these being parsimony informative, to provide increased discriminatory power and assign museum specimens to one of the three major NZ rock wren clades previously identified by Weston and Robertson (2015). The Akaike Information Criterion implemented within jModelTest v2.1.10 (Darriba et al., 2012) was used to determine the most appropriate nucleotide substitution model for each dataset. These were HKY + G for the CR dataset, and HKY for the Cyt B dataset. The nested sampling package within BEAST v2.5 (Russel et al., 2018) was used to determine the most appropriate tree prior for Bayesian phylogenetics by converting marginal likelihood values into Bayes factors. The constant population size coalescent tree prior was determined to be the most appropriate prior for both datasets. Bayesian phylogenies were constructed using BEAST2 v2.5 (Bouckaert et al., 2014). The corresponding bush wren cytochrome b sequence (KX369035.1; Mitchell et al., 2016) was used as the outgroup for the Cyt B phylogeny, while the rifleman (Acanthisitta chloris) (AY325307.1; Harrison et al., 2004) was used for the CR phylogeny, as the partial bush wren mitogenome of Mitchell et al. (2016) does not contain the control region. The BEAST analysis used 10 million Markov Chain Mote Carlo (MCMC) generations, sampled every 1,000 iterations with the first 10% discarded as the burn-in, a constant population size coalescent tree prior, and the HKY (Cyt B) or HKY + G (CR) nucleotide substitution model. Tracer v1.7 (Rambaut et al., 2018) was used to check effective sample sizes (>200) and MCMC convergence. Bayesian phylogenetic trees were summarized using TreeAnnotator and visualized using FigTree v1.4.3 (https://github.com/rambaut/figtree/releases). Median-joining haplotype networks were constructed using PopART (Leigh and Bryant, 2015).
Results
Amplifiable DNA was successfully extracted from 30 of the 31 NZ rock wren museum skins analyzed. The two Cyt B and CR fragments were successfully amplified from 30 and 28 specimens, respectively. No amplicons were produced by our negative DNA extraction or PCR controls. The singular specimen (CM Av889) that did not produce amplifiable DNA did not have locality data, and no discrepancies between collection locality and Cyt B sequence were detected for the further two specimens (CM Av888 and NMNZ OR.5097) that failed to produce CR amplicons. Both the CR and Cyt B phylogenies (Figures 2, 3) showed strong support (posterior probability: 0.88–1) for the northern and southern NZ rock wren lineages (and the southern Fiordland clade) previously identified by Weston and Robertson (2015). The NZ rock wren specimen allegedly collected from the southern North Island (NHMUK 1939.12.9.75; Remutaka Ranges) does not cluster with the bush wren Cyt B sequence but sits within the southern lineage of NZ rock wren, specifically, the southern Fiordland clade (Figure 2; an uncollapsed version of this tree can be found within the Supplementary Material as Figure S1). This phylogenetic position is further supported by the CR data (Figure 3). Further discrepancies between collection data and genetic sequence were detected in additional specimens. Three specimens (AMNH 554486, AMNH 554487, and AMNH 554488) collected by Henry H. Travers labeled “Otago Province” in the southern South Island, clustered within the northern NZ rock wren lineage (Figures 2, 3) as opposed to the southern lineage, indicating their associated collection locality data is incorrect. Conversely, a single specimen from “Nelson Province” in the northern South Island (NMNZ OR.5096) consistently grouped with individuals from southern Fiordland (Figures 2, 3). The holotype and a paratype of X. g. rineyi (NMNZ OR.2396 and NMNZ OR.2397) also clustered within this southern Fiordland clade, alongside contemporary individuals collected from the same region.
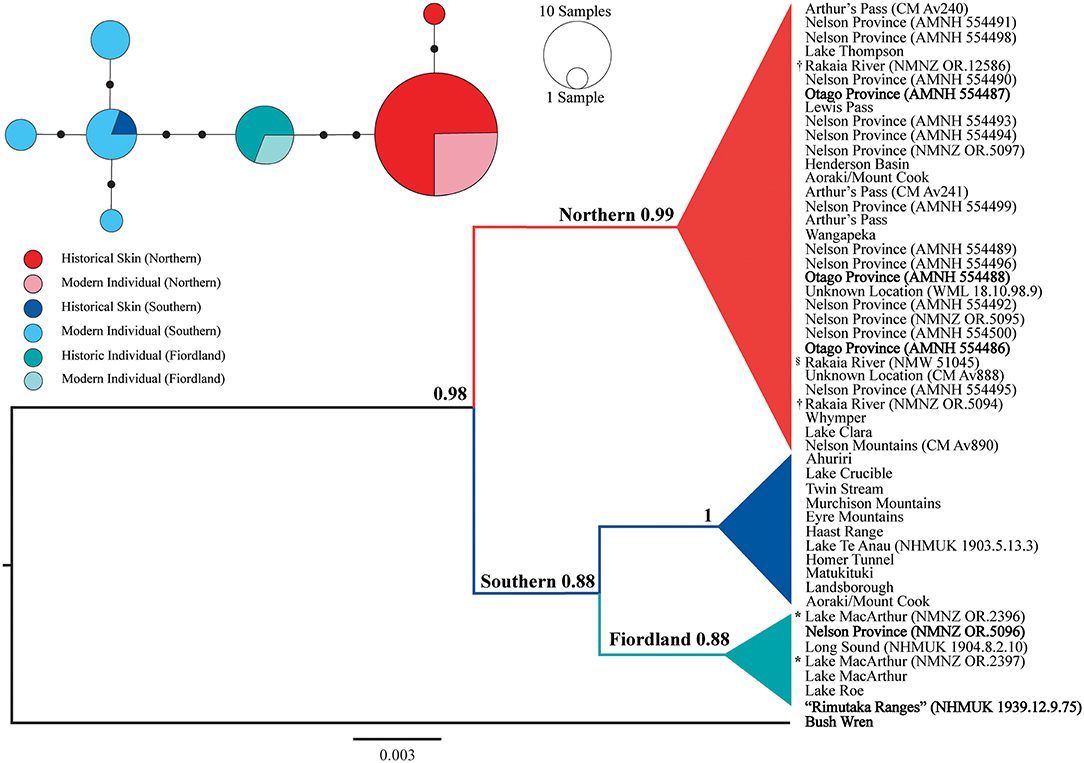
Figure 2. Collapsed Bayesian phylogeny and median-joining haplotype network of NZ rock wren (Xenicus gilviventris) mitochondrial cytochrome b sequences (163 bp). Major clades mentioned within the text are identified and posterior probabilities are displayed next to main nodes. Bold labels denote museum specimens for which the recorded collection locality and genetic sequence do not match. *X. gilviventris rineyi type series specimen, †X. haasti syntype, §X. gilviventris syntype. The scale bar depicts the distance corresponding to 0.003 nucleotide substitutions per site. Within the haplotype network, mutational steps are illustrated via small black circles, and haplotypes are colored according to genetic lineage and specimen type (contemporary individual or museum skin).
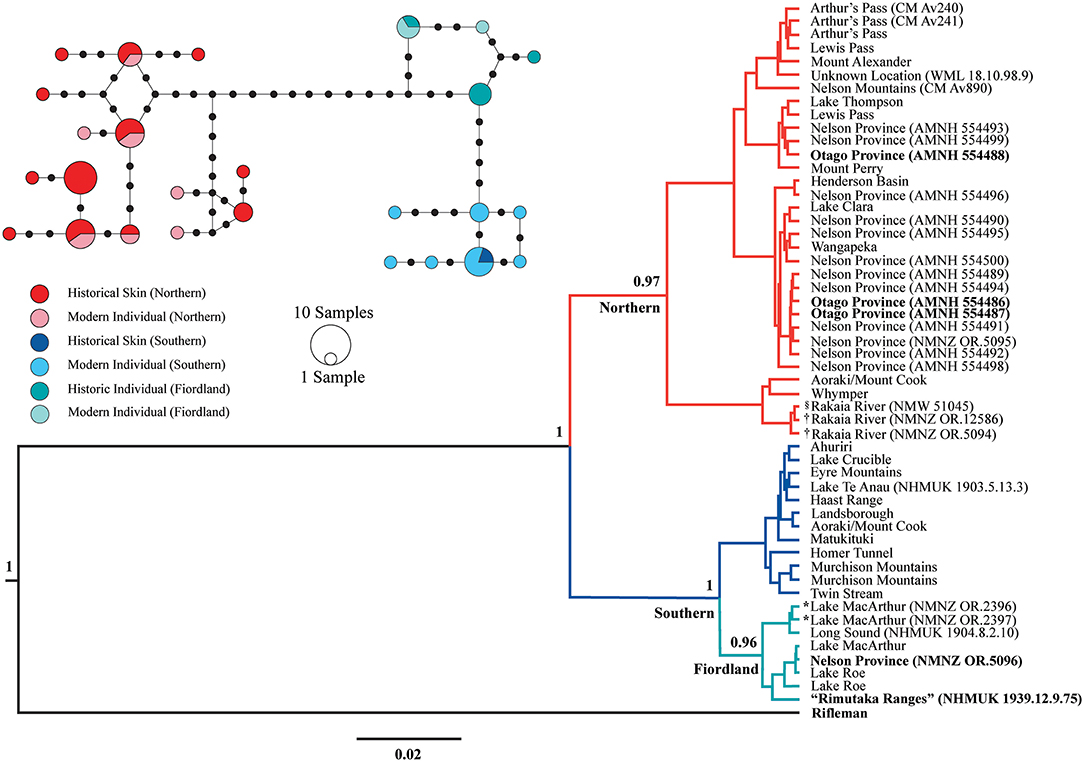
Figure 3. Bayesian phylogeny and median-joining haplotype network of NZ rock wren (Xenicus gilviventris) mitochondrial control region sequences (284 bp). Major clades mentioned within the text are identified and posterior probabilities are displayed next to main nodes. Bold labels denote museum specimens for which the recorded collection locality and genetic sequence do not match. *X. gilviventris rineyi type series specimen, †X. haasti syntype, §X. gilviventris syntype. The scale bar depicts the distance corresponding to 0.02 nucleotide substitutions per site. Within the haplotype network, mutational steps are illustrated via small black circles, and haplotypes are colored according to genetic lineage and specimen type (contemporary individual or museum skin).
Using the phylogenetic framework of Weston and Robertson (2015), we were able to assign individual specimens with no formal collection locality data (CM Av888, NMNZ OR.12586, NMNZ 5094, NMW 51045, and WML 18.10.98.9) to a broad geographical region based upon their genetic lineage. All specimens with unknown locality data, including a syntype of X. gilviventris (NMW 51045), and the syntypes of X. haasti (NMNZ OR.12586, NMNZ OR.5094), cluster with the northern lineage (Figures 2, 3), suggesting they were collected from the northern South Island, potentially as far south as Aoraki/Mount Cook. In addition, historical evidence (discussed below) strongly suggests that the type specimens of X. gilviventris and X. haasti were collected from the headwaters of the Rakaia River, allowing us to formally identify the type locality of both X. gilviventris and X. haasti.
Median joining haplotype networks (Figures 2, 3) also revealed strong north-south phylogeographic structuring in the CR and Cyt b datasets as previously reported by Weston and Robertson (2015), and the phylogenetic analyses presented here. There were fewer segregating sites within the Cyt B dataset. The northern lineage is dominated by one haplotype, with a singular museum specimen (CM Av890), exhibiting a single base pair difference from this common haplotype (Figure 2). All specimens representing the southern Fiordland clade have the same haplotype, while another four haplotypes form a haplogroup representing the remainder of the southern lineage. The CR dataset contained higher levels of genetic diversity with distinct northern and southern haplogroups separated by 12 mutational steps (Figure 3).
Discussion
The scientific value of museum specimens is dependent on their associated data, including collection locality and taxonomic identity (Shepherd et al., 2013). Inaccurate data can distort inferences of historical population connectivity, identification of conservation management units, and formulation of conservation management plans (Boessenkool et al., 2009). Here we detect five discrepancies between recorded collection locality and genetic sequence by analyzing NZ rock wren specimens held within museum collections worldwide, and combine historical records with our genetic data to describe the type locality of both X. gilviventris and X. haasti.
A discrepancy with important conservation implications is the mislabeling of a specimen likely collected from southern Fiordland (based on DNA), as “Rimutaka [= Remutaka] Ranges” in the southern North Island. This label data suggested that the past distribution of NZ rock wrens was previously much more extensive than the present-day, and that NZ rock wrens have been historically extirpated from the North Island of New Zealand; however, this position is not supported by genetic evidence and hence the data for this specimen is erroneous. Our results strongly suggest that (1) the taxonomic identity of NHMUK 1939.12.9.75 is indeed a NZ rock wren, not a bush wren, and (2) that the specimen has been mislabeled and was not collected in the southern North Island but was instead collected in the southern South Island. Therefore, there is currently no definitive record of NZ rock wrens inhabiting the North Island of New Zealand.
Additional erroneously-labeled specimens were also discovered, including three specimens from the AMNH Rothschild collection reportedly collected from the Otago Province in April 1897 by H. H. Travers. Mitochondrial DNA sequence analysis suggests that these specimens were collected north of Aoraki/Mount Cook in the central South Island, well outside the historical boundary of the Otago Province. Each specimen has a Rothschild Museum label, as well as a label from H. H. Travers. These labels state “Otago” (i.e., Otago Province) and “H. H. Travers,” alongside an approximate collection date (“4/97” i.e., April 1897) and the sex of each specimen (Thomas Trombone, personal communication). Whether this mislabeling constitutes deliberate fraud or poor record keeping is unknown. Furthermore, little is known about the discrepant specimen held within the National Museum of New Zealand (NMNZ OR.5096). Collected in 1899, it is likely that H. H. Travers also obtained this individual, as he was one of the primary collectors and sellers of birds to the National Museum of New Zealand during the late 1800s and early 1900s, and is associated with 52 other bird specimens labeled “Nelson” or “Nelson Province” in the National Museum of New Zealand collections (AJDT, unpublished data). If this hypothesis is correct, then a total of four NZ rock wren specimens collected by H. H. Travers have erroneous collection data, in addition to other Travers' bird specimens previously identified (see Boessenkool et al., 2009; Miskelly, 2012). Our results cast further doubt on the label accuracy of specimens obtained from H. H. Travers.
While we acknowledge the considerable debate over the reliability of single gene trees with reference to incomplete lineage sorting (Ballard and Whitlock, 2004; Rubinoff and Holland, 2005; Galla and Johnson, 2015), and changing tree topology due to historically high levels of genetic diversity (Rawlence et al., 2014b, 2015), the strong north-south phylogeographic structure within NZ rock wrens (3.68 ± 0.5% inter-lineage genetic divergence within Cyt B, and 13.3 ± 4.9% inter-lineage genetic divergence within the CR) is well-supported by both mitochondrial and nuclear microsatellite DNA (Weston and Robertson, 2015). While the mitochondrial DNA split between southern Fiordland individuals and the remainder of the southern lineage is shallower, with 1.2% genetic divergence within Cyt B (Weston and Robertson, 2015). Multiple substitutions, consistent between contemporary and historical NZ rock wrens, govern the placement of individuals into one of the three major clades. We believe that this strong phylogeographic structure and consistency across time periods is sufficient to rule out the impacts of incomplete lineage sorting and historical genetic diversity on tree topology.
Were New Zealand Rock Wrens Ever Present Within the North Island?
Our genetic results demonstrate that there is currently no definitive record of NZ rock wren having inhabited the North Island and suggests that the NZ rock wren was not extirpated from the North Island following the arrival of Europeans. However, it is possible that NZ rock wrens were present within the North Island post-European arrival and were never sighted or collected. Only two specimens of laughing owl (Ninox albifaces) were ever collected from the North Island, and have since been lost (Worthy, 1997), while no museum skins of kakapo (Strigops habroptilus) are known from the North Island, although there are sightings of the species from there into the early twentieth century (Williams, 1956). Additionally, it is still unknown whether NZ rock wrens were present within the North Island prior to European arrival. Polynesian colonization of New Zealand (~280 A.D.; Wilmshurst et al., 2008) also marked the arrival of kiore, the pacific rat (Rattus exulans), which devastated the invertebrate and small vertebrate faunas of New Zealand (Holdaway, 1989; Towns and Daugherty, 1994; Gibbs, 2009). The current distribution of NZ rock wrens may reflect where populations survived human-mediated extinction pressures. Within the New Zealand archipelago, the North Island is known to have suffered the greatest loss of avifauna (Holdaway et al., 2001; Rawlence et al., 2019). The kea (Nestor notabilis), another endemic avian species for which alpine areas constitute an important habitat, was extirpated from the North Island subsequent to the arrival of Polynesians, likely due to predation by humans and kiore (Tennyson et al., 2014). Ancient DNA analysis, or a reappraisal of the morphology and osteology of subfossil Xenicus remains is necessary to confirm whether NZ rock wrens occurred within the North Island prior to human arrival.
Species Translocations
Most conservation translocations of New Zealand species involve the movement of individuals between areas within their historical or prehistoric range, to ensure the persistence of a species Miskelly and Powlesland, 2013. However, some species have been introduced to areas outside their range for a variety of reasons, including the establishment of insurance populations during pest eradication schemes [e.g., Codfish Island fernbird (Bowdleria punctata wilsoni) (McClelland, 2002)] or the replacement of extinct relatives (e.g., replacement of the South Island snipe (C. iredalei) by the Snares Island snipe (C. huegeli) (Miskelly et al., 2012) (reviewed by Miskelly and Powlesland, 2013). According to our genetic results, any translocation of NZ rock wrens to the North Island may constitute a novel introduction of the species to a new area, rather than a species re-introduction or a species restoration. Ongoing management of NZ rock wrens includes translocation to predator-free sites (Willans and Weston, 2005; Weston, 2006), including a successful translocation to Secretary Island in southern Fiordland (Reid and Edge Hill, 2017). Presently, translocation of NZ rock wrens to the North Island does not satisfy the objective of securing a population in a predator-free site to ensure species persistence.
New Zealand Rock Wren Taxonomy
The northern and southern NZ rock wren (X. gilviventris Pelzeln, 1867) lineages are currently considered separate evolutionarily significant units (Robertson et al., 2016). A subspecies, X. gilviventris rineyi Falla, 1953, was described from southern Fiordland (see also Riney, 1953), but never widely accepted (e.g., Kinsky, 1970). It is currently treated as a junior synonym (Gill et al., 2010). Falla (1953) compared southern Fiordland NZ rock wren individuals to museum specimens from the northern South Island and considered those from southern Fiordland to be slightly smaller- and to have brighter plumage than their northern counterparts. Riney (1953) suggested that southern Fiordland individuals lacked the bobbing behavior prevalent in other NZ rock wren populations. However, Weston (2014) observed no significant behavioral or morphological differences between contemporary southern Fiordland individuals and other NZ rock wren populations but this was before the exact demarcation of the southern sub-populations was defined using genetics. Further morphological analysis may expose physical differences between genetically distinct populations. Genetic sequences from the holotype and a paratype of X. gilviventris rineyi (NMNZ OR.2396 and NMNZ OR.2397), collected in 1953, closely matched (Figures 2, 3) recently sampled individuals from the type locality (Weston and Robertson, 2015).
Genetic analyses of X. haasti (NMNZ OR.5094 and NMNZ OR.12586), and X. gilviventris (NMW 51045) syntypes determined that all three specimens belong to the northern NZ rock wren lineage (Figures 2, 3), adding support to the hypothesis that these specimens were collected from the headwaters of the Rakaia River. The exact type locality of X. gilviventris Pelzeln, 1867 and Xenicus haasti Buller, 1869 has until now never been determined. The name X. gilviventris Pelzeln, 1867 was based on a single specimen included with 72 birds sent by Julius Haast to Georg Frauenfeld in Vienna (Naturhistorisches Museum Wien, unpublished archive). Frauenfeld was chief zoologist aboard the Frigate Novara and Haast had probably met him when he first arrived in New Zealand. August Pelzeln was also aboard the Novara and was tasked by Frauenfeld to describe Haast's specimens. In March and early April of 1866, Julius Haast and Frederick Fuller, his taxidermist, visited the headwaters of the Rakaia River. Over a period of 40 days they collected “160 skins of birds … several of them either new to science or at least very rare, and desirable objects for the completion of our own collection” (Haast, 1866). Haast describes the habits of a wren that are presumably the NZ rock wren from the vicinity of Mein's Knob:
“Another very interesting inhabitant of this district is a large greenish-brown Wren with a drab-colored breast (Certhiparus?), which lives exclusively amongst the large talusses of debris high on the mountain sides. This bird, instead of flying away when frightened or when thrown at with stones, or even when shot at, hides itself among the angular debris of which these huge talusses are composed. We tried several times, in vain, by removing some of the blocks and surrounding it to catch one of them alive. It reminded me strongly of the habits and movements of the lizards which live in the same region in similar localities.”
Pelzeln's collection of specimens received from Haast contained 39 species all of which were either mentioned in Haast's account of his trip to the Rakaia (Haast, 1866) or are typical of the area. Pelzeln described two new species, X. gilviventris Pelzeln, 1867 and a putative taxon of alpine bellbird Anthornis ruficeps (Pelzeln, 1867) [= Anthornis melanura melanura (Pelzeln, 1867)], from Haast's collections. It appears that Walter Buller also received NZ rock wren specimens from this same collection at approximately the same time, i.e., “collected in the Southern Alps in the 1860s by J.F. Haast” (Bartle and Tennyson, 2009). Buller's collection that contains the types of his Xenicus haasti Buller, 1869, also included a syntype of his ‘new’ parrot Platycercus alpinus Buller, 1869 [= Cyanoramphus malherbi Souancé, 1857] (Bartle and Tennyson, 2009). Buller “received several specimens” of this parrot from Haast “from the forests of the Southern Alps” (Buller, 1869), so these were probably also part of the same collection.
The type localities and dates of collection for all four taxa can thus be restricted to “Headwaters of the Rakaia River, March or April 1866. Collected by J. Haast and F. Fuller.”
Concluding Remarks
The genetic data presented within our study illustrates the applied use of aDNA to enhance the utility of museum collections and inform both taxonomy and conservation management; yet highlights the need to determine the veracity of specimen data. Museum specimens should be treated with caution when they are used to formulate conservation management plans that depend upon the accuracy of their associated data. Steps should be taken to discern the accuracy of such details, especially for specimens reportedly obtained by collectors known for poor record keeping or potential data falsification. Preferably, these steps should incorporate a multidisciplinary approach that includes the use of museum records and collectors' notes, alongside biological information (e.g., DNA sequence data). This approach helps to ensure that museum specimens remain a valuable resource for future scientific research. Additionally, future genetic research on museum specimens should also utilize the power of high throughput DNA sequencing, depending upon the nature of the research question(s) addressed. This technology allows for greater detection of post-mortem DNA damage and exogenous contamination, aiding in the authentication of aDNA sequence data. Authentication of aDNA sequencing data continues to form an essential aspect of the aDNA field, and helps ensure that the results of aDNA studies are robust and reliable.
Data Availability Statement
The datasets generated for this study can be found within GenBank (https://www.ncbi.nlm.nih.gov/genbank/). Accession Numbers: MN402619–MN402676.
Ethics Statement
Ethical review and approval was not required for this study because no living animals were collected or examined. DNA samples were taken solely from museum specimens.
Author Contributions
BR, KW, and NR conceived the study. AV and LS performed the laboratory work. AV analyzed the data and wrote the manuscript with contributions from all authors. LS created the figures. AT and RS examined archival material and provided comment on historical avian collectors and NZ rock wren taxonomy. All authors reviewed the manuscript.
Funding
Funding for the presented research was provided to BR by the New Zealand Department of Conservation, and to NR by the Royal Society of New Zealand Marsden Fund (16-UOO-096).
Conflict of Interest
The authors declare that the research was conducted in the absence of any commercial or financial relationships that could be construed as a potential conflict of interest.
Acknowledgments
We are grateful to Hein van Grouw and Alex Bond (Natural History Museum at Tring), Tony Parker (World Museum Liverpool), Hans-Martin Berg (Naturhistorisches Museum Wien), and Thomas Trombone and Paul Sweet (American Museum of Natural History) for access to museum collections and supporting information/comments on the NZ rock wren specimens held by their respective institutions. Thank you to Rosa Wilkie, Andrea van Turnhout, and Nikita-Maree Norris for their assistance with laboratory work as part of the GENE312 course at the University of Otago.
Supplementary Material
The Supplementary Material for this article can be found online at: https://www.frontiersin.org/articles/10.3389/fevo.2019.00496/full#supplementary-material
References
Ballard, J. W. O., and Whitlock, M. C. (2004). The incomplete natural history of mitochondria. Mol. Ecol. 13, 729–744. doi: 10.1046/j.1365-294X.2003.02063.x
Barbanera, F., Moretti, B., Guerrini, M., Al-Sheikhly, O. F., and Forcina, G. (2016). Investigation of ancient DNA to enhance natural history museum collections: misidentification of smooth-coated otter (Lutrogale perspicillata) specimens across multiple museums. Belg. J. Zool. 146, 101–112.
Barker, F. K., Cibois, A., Schikler, P., Feinstein, J., and Cracraft, J. (2004). Phylogeny and diversification of the largest avian radiation. Proc. Natl. Acad. Sci. U.S.A. 101, 11040–11045. doi: 10.1073/pnas.0401892101
Bartle, J. A., and Tennyson, A. J. D. (2009). History of Walter Buller's collections of New Zealand birds. Tuhinga Rec. Mus. N. Z. Te Papa Tongarewa 20, 81–136.
Boessenkool, S., Star, B., Scofield, R. P., Seddon, P. J., and Waters, J. M. (2009). Lost in translation or deliberate falsification? Genetic analyses reveal erroneous museum data for historic penguin specimens. Proc. R. Soc. B Biol. Sci. 277, 1057–1064. doi: 10.1098/rspb.2009.1837
Bouckaert, R., Heled, J., Kühnert, D., Vaughan, T., Wu, C.-H., Xie, D., et al. (2014). BEAST 2: a software platform for Bayesian evolutionary analysis. PLoS Comput. Biol. 10:e1003537. doi: 10.1371/journal.pcbi.1003537
Brotherton, P., Endicott, P., Sanchez, J. J., Beaumont, M., Barnett, R., Austin, J., et al. (2007). Novel high-resolution characterization of ancient DNA reveals C > U-type base modification events as the sole cause of post mortem miscoding lesions. Nucleic Acids Res. 35, 5717–5728. doi: 10.1093/nar/gkm588
Buller, W. (1869). On some new species of New-Zealand birds. Ibis 5, 37–43. doi: 10.1111/j.1474-919X.1869.tb07092.x
Cooper, A., and Poinar, H. N. (2000). Ancient DNA: do it right or not at all. Science 289, 1139–1139. doi: 10.1126/science.289.5482.1139b
Cooper, A., Rhymer, J., James, H. F., Olson, S. L., McIntosh, C. E., Sorenson, M. D., et al. (1996). Ancient DNA and island endemics. Nature 381:484. doi: 10.1038/381484a0
Dabney, J., Knapp, M., Glocke, I., Gansauge, M.-T., Weihmann, A., Nickel, B., et al. (2013). Complete mitochondrial genome sequence of a Middle Pleistocene cave bear reconstructed from ultrashort DNA fragments. Proc. Natl. Acad. Sci. U.S.A. 110, 15758–15763. doi: 10.1073/pnas.1314445110
Darriba, D., Taboada, G. L., Doallo, R., and Posada, D. (2012). jModelTest 2: more models, new heuristics and parallel computing. Nat. Methods 9:772. doi: 10.1038/nmeth.2109
Fulton, T. L., and Shapiro, B. (2019). Setting up an ancient DNA laboratory. Methods Mol Biol. 1963, 1–13. doi: 10.1007/978-1-4939-9176-1_1
Galla, S. J., and Johnson, J. A. (2015). Differential introgression and effective size of marker type influence phylogenetic inference of a recently divergent avian group (Phasianidae: Tympanuchus). Mol. Phylogenet. Evol. 84, 1–13. doi: 10.1016/j.ympev.2014.12.012
Gibbs, G. W. (2009). The end of an 80-million year experiment: a review of evidence describing the impact of introduced rodents on New Zealand's ‘mammal-free’invertebrate fauna. Biol. Invasions 11, 1587–1593. doi: 10.1007/s10530-008-9408-x
Gilbert, M. T. P., Tomsho, L. P., Rendulic, S., Packard, M., Drautz, D. I., Sher, A., et al. (2007). Whole-genome shotgun sequencing of Mitochondria from ancient hair shafts. Science 317, 1927–1930. doi: 10.1126/science.1146971
Gill, B. J., Bell, B. D., Chambers, G. K., Medway, D. G., Palma, R. L., Scofield, R. P., et al. (2010). Checklist of the birds of New Zealand, Norfolk and Macquarie Islands, and the Ross Dependency, Antarctica. Wellington: Te Papa Press.
Graham, C. H., Ferrier, S., Huettman, F., Moritz, C., and Peterson, A. T. (2004). New developments in museum-based informatics and applications in biodiversity analysis. Trends Ecol. Evol. 19, 497–503. doi: 10.1016/j.tree.2004.07.006
Grealy, A., Rawlence, N., and Bunce, M. (2017). Time to spread your wings: a review of the avian ancient DNA field. Genes 8:184. doi: 10.3390/genes8070184
Grosser, S., Abdelkrim, J., Wing, J., Robertson, B. C., and Gemmell, N. J. (2017). Strong isolation by distance argues for separate population management of endangered blue duck (Hymenolaimus malacorhynchos). Conserv. Genet. 18, 327–341. doi: 10.1007/s10592-016-0908-4
Hackett, S. J., Kimball, R. T., Reddy, S., Bowie, R. C. K., Braun, E. L., Braun, M. J., et al. (2008). A phylogenomic study of birds reveals their evolutionary history. Science 320, 1763–1768. doi: 10.1126/science.1157704
Harrison, G., McLenachan, P., Phillips, M., Slack, K. E., Cooper, A., and Penny, D. (2004). Four new avian mitochondrial genomes help get to basic evolutionary questions in the late Cretaceous. Mol. Biol. Evol. 21, 974–983. doi: 10.1093/molbev/msh065
Hofreiter, M., Jaenicke, V., Serre, D., Haeseler, A. V., and Pääbo, S. (2001). DNA sequences from multiple amplifications reveal artifacts induced by cytosine deamination in ancient DNA. Nucleic Acids Res. 29, 4793–4799. doi: 10.1093/nar/29.23.4793
Holdaway, R. N. (1989). New Zealand's pre-human avifauna and its vulnerability. N. Z. J. Ecol. 12, 11–25.
Holdaway, R. N., Worthy, T. H., and Tennyson, A. J. D. (2001). A working list of breeding bird species of the New Zealand region at first human contact. NZ. J. Zool. 28, 119–187. doi: 10.1080/03014223.2001.9518262
IUCN (2013). Guidelines for Reintroductions and Other Conservation Translocations. Version 1.0. Gland: IUCN Species Survival Commission.
Jarvis, E. D., Mirarab, S., Aberer, A. J., Li, B., Houde, P., Li, C., et al. (2014). Whole-genome analyses resolve early branches in the tree of life of modern birds. Science 346, 1320–1331. doi: 10.1126/science.1253451
Kearse, M., Moir, R., Wilson, A., Stones-Havas, S., Cheung, M., Sturrock, S., et al. (2012). Geneious basic: an integrated and extendable desktop software platform for the organization and analysis of sequence data. Bioinformatics 28, 1647–1649. doi: 10.1093/bioinformatics/bts199
Kinsky, F. C. (1970). Annotated Checklist of the Birds of New Zealand. Wellington: A.H. and A.W. Reed.
Leigh, J. W., and Bryant, D. (2015). popart: full-feature software for haplotype network construction. Methods Ecol. Evol. 6, 1110–1116. doi: 10.1111/2041-210X.12410
Leonard, J. A. (2008). Ancient DNA applications for wildlife conservation. Mol. Ecol. 17, 4186–4196. doi: 10.1111/j.1365-294X.2008.03891.x
Little, L., King, C. M., and O'Donnell, C. F. J. (2017). Behaviour of stoats (Mustela erminea) raiding the nests of rock wrens (Xenicus gilviventris) in alpine New Zealand. Notornis 64, 124–135.
McClelland, P. J. (2002). “Eradication of Pacific rats (Rattus exulans) from Whenua Hou Nature Reserve (Codfish Island), Putauhinu and Rarotoka Islands, New Zealand,” in Turning the Tide: The Eradication of Invasive Species, eds C. R. Veitch and M. N. Clout (Gland; Cambridge: IUCN SSC Invasive Specialist Group), 173–181.
Michelsen-Heath, S., and Gaze, P. (2007). Changes in abundance and distribution of the rock wren (Xenicus gilviventris) in the South Island, New Zealand. Notornis 54, 71–78.
Miller, H. C., and Lambert, D. M. (2006). A molecular phylogeny of New Zealand's Petroica (Aves: Petroicidae) species based on mitochondrial DNA sequences. Mol. Phylogenet. Evol. 40, 844–855. doi: 10.1016/j.ympev.2006.04.012
Miskelly, C. M. (2012). Discovery and extinction of the South Island Snipe (Coenocorypha iredalei) on islands around Stewart Island. Notornis 59, 15–31.
Miskelly, C. M., Charteris, M. R., and Fraser, J. R. (2012). Successful translocation of Snares Island snipe (Coenocorypha huegeli) to replace the extinct South Island snipe (C. iredalei). Notornis 59, 32–38.
Miskelly, C. M., and Powlesland, R. G. (2013). Conservation translocations of New Zealand birds, 1863–2012. Notornis 60, 3–28.
Mitchell, K. J., Wood, J. R., Llamas, B., McLenachan, P. A., Kardailsky, O., Scofield, R. P., et al. (2016). Ancient mitochondrial genomes clarify the evolutionary history of New Zealand's enigmatic acanthisittid wrens. Mol. Phylogenet. Evol. 102, 295–304. doi: 10.1016/j.ympev.2016.05.038
Murphy, S. A., Flux, I. A., and Double, M. C. (2006). Recent evolutionary history of New Zealand's North and South Island Kokako (Callaeas cinerea) inferred from mitochondrial DNA sequences. Emu-Austral Ornithol. 106, 41–48. doi: 10.1071/MU05007
O'Donnell, C. F. J., Weston, K. A., and Monks, J. M. (2017). Impacts of introduced mammalian predators on New Zealands alpine fauna. N. Z. J. Ecol. 41, 1–22. doi: 10.20417/nzjecol.41.18
Olson, S. L., and James, H. F. (1982). Fossil Birds from the Hawaiian Islands: evidence for wholesale extinction by man before western contact. Science 217, 633–635. doi: 10.1126/science.217.4560.633
Pelzeln, A. v. (1867). Ueber eine von Herrn Julius Haast erhaltene Sendung von Vogelbälgen aus Neu Seeland. Verhandlungen der Zoologisch-Botanischen Gesellschaft in Wien 17, 315–318.
Rambaut, A., Drummond, A. J., Xie, D., Baele, G., and Suchard, M. A. (2018). Posterior summarisation in Bayesian phylogenetics using Tracer 1.7. Syst. Biol. 67, 901–904. doi: 10.1093/sysbio/syy032
Rawlence, N. J., Kennedy, M., Anderson, C. N. K., Prost, S., Till, C. E., Smith, I. W. G., et al. (2015). Geographically contrasting biodiversity reductions in a widespread New Zealand seabird. Mol. Ecol. 24, 4605–4616. doi: 10.1111/mec.13338
Rawlence, N. J., Kennedy, M., Waters, J. M., and Scofield, R. P. (2014a). Morphological and ancient DNA analyses reveal inaccurate labels on two of Buller's bird specimens. J. R. Soc. N. Z. 44, 163–169. doi: 10.1080/03036758.2014.972962
Rawlence, N. J., Tennyson, A. J. D., Cole, T. L., Verry, A. J. F., and Scofield, R. P. (2019). Evidence for breeding of Megadyptes penguins in the North Island at the time of human arrival. NZ. J. Zool. 46, 165–173. doi: 10.1080/03014223.2018.1523202
Rawlence, N. J., Till, C. E., Scofield, R. P., Tennyson, A. J. D., Collins, C. J., Lalas, C., et al. (2014b). Strong phylogeographic structure in a sedentary seabird, the Stewart Island Shag (Leucocarbo chalconotus). PLoS ONE 9:e90769. doi: 10.1371/journal.pone.0090769
Reid, R., and Edge Hill, K. A. (2017). Conserving Fiordland's Biodiversity 1987-2015. The Challenges, the Achievements, the Knowledge. Department of Conservation.
Riney, T. (1953). Notes on habitat and behaviour of the rock wren subspecies Xenicus gilviventris rineyi Falla. Notornis 5, 186–188.
Robertson, H. A., Baird, K., Dowding, J. E., Elliott, G. P., Hitchmough, R. A., Miskelly, C. M., et al. (2016). “Conservation status of New Zealand birds, 2016,” in New Zealand Threat Classification Series (Wellington: Department of Conservation), 1–27.
Rubinoff, D., and Holland, B. S. (2005). Between two extremes: mitochondrial DNA is neither the panacea nor the nemesis of phylogenetic and taxonomic inference. Syst. Biol. 54, 952–961. doi: 10.1080/10635150500234674
Russel, P. M., Brewer, B. J., Klaere, S., and Bouckaert, R. R. (2018). Model selection and parameter inference in phylogenetics using Nested Sampling. Syst. Biol. 68, 219–233. doi: 10.1093/sysbio/syy050
Shaffer, H. B., Fisher, R. N., and Davidson, C. (1998). The role of natural history collections in documenting species declines. Trends Ecol. Evol. 13, 27–30. doi: 10.1016/S0169-5347(97)01177-4
Shepherd, L. D., Tennyson, A. J. D., and Lambert, D. M. (2013). Using ancient DNA to enhance museum collections: a case study of rare kiwi (Apteryx spp.) specimens. J. R. Soc. N. Z. 43, 119–127. doi: 10.1080/03036758.2012.732585
Stidolph, R. H. D. (1926). Bird-life around Wellington, N.Z. Emu - Austral Ornithol. 25, 204–207. doi: 10.1071/MU925204
Tennyson, A. J., and Bartle, J. S. (2008). Catalogue of type specimens of birds in the Museum of New Zealand Te Papa Tongarewa. Tuhinga Rec. Mus. N. Z. Te Papa Tongarewa 19, 185–207.
Tennyson, A. J. D., Easton, L. J., and Wood, J. R. (2014). Kea (Nestor notabilis)–another North Island human-caused extinction. Notornis 61, 174–176.
Thomas, J. E., Carvalho, G. R., Haile, J., Martin, M. D., Castruita, J. A. S., Niemann, J., et al. (2017). An ‘Aukward’ tale: a genetic approach to discover the whereabouts of the Last Great Auks. Genes 8:164. doi: 10.3390/genes8060164
Thomas, W. K., Pääbo, S., Villablanca, F. X., and Wilson, A. C. (1990). Spatial and temporal continuity of kangaroo rat populations shown by sequencing mitochondrial DNA from museum specimens. J. Mol. Evol. 31, 101–112. doi: 10.1007/BF02109479
Towns, D. R., and Daugherty, C. H. (1994). Patterns of range contractions and extinctions in the New Zealand herpetofauna following human colonisation. N. Z. J. Zool. 21, 325–339. doi: 10.1080/03014223.1994.9518003
Trewick, S. A. (1996). Morphology and evolution of two takahe: flightless rails of New Zealand. J. Zool. 238, 221–237. doi: 10.1111/j.1469-7998.1996.tb05391.x
Wandeler, P., Hoeck, P. E. A., and Keller, L. F. (2007). Back to the future: museum specimens in population genetics. Trends Ecol. Evol. 22, 634–642. doi: 10.1016/j.tree.2007.08.017
Weston, K. A. (2006). Post-translocation Monitoring of Rock Wren Xenicus gilviventris on Anchor Island. Internal Report, Department of Conservation, Te Anau.
Weston, K. A. (2014). Conservation genetics of alpine rock wren (Xenicus gilviventris) (Ph.D. Thesis). University of Otago, Dunedin, New Zealand.
Weston, K. A., O'Donnell, C. F. J., van dam-Bates, P., and Monks, J. M. (2018). Control of invasive predators improves breeding success of an endangered alpine passerine. Ibis 160, 892–899. doi: 10.1111/ibi.12617
Weston, K. A., and Robertson, B. C. (2015). Population structure within an alpine archipelago: strong signature of past climate change in the New Zealand rock wren (Xenicus gilviventris). Mol. Ecol. 24, 4778–4794. doi: 10.1111/mec.13349
Willans, M., and Weston, K. A. (2005). Translocation of Rock Wren Xenicus gilviventris From Murchison Mountains to Anchor Island, Dusky Sound, Fiordland. Te Anau: Department of Conservation Internal Report.
Williams, G. R. (1956). The Kakapo (Strigops Habrotilus, Gray): a review and reappraisal of a near-extinct species. Notornis 7, 29–56.
Wilmshurst, J. M., Anderson, A. J., Higham, T. F. G., and Worthy, T. H. (2008). Dating the late prehistoric dispersal of Polynesians to New Zealand using the commensal Pacific rat. Proc. Natl. Acad. Sci. U.S.A. 105, 7676–7680. doi: 10.1073/pnas.0801507105
Winker, K. (2000). Obtaining, preserving, and preparing bird specimens. J. Field Ornithol. 71, 250–297. doi: 10.1648/0273-8570-71.2.250
Winters, M., Barta, J. L., Monroe, C., and Kemp, B. M. (2011). To clone or not to clone: method analysis for retrieving consensus sequences in ancient DNA samples. PLoS ONE 6:e21247. doi: 10.1371/journal.pone.0021247
Worthy, T. (1997). A survey of historical laughing owl (Sceloglaux albifacies) specimens in museum collections. Notornis 44, 241–252.
Keywords: conservation, translocation, re-introduction, taxonomy, museum skin
Citation: Verry AJF, Scarsbrook L, Scofield RP, Tennyson AJD, Weston KA, Robertson BC and Rawlence NJ (2019) Who, Where, What, Wren? Using Ancient DNA to Examine the Veracity of Museum Specimen Data: A Case Study of the New Zealand Rock Wren (Xenicus gilviventris). Front. Ecol. Evol. 7:496. doi: 10.3389/fevo.2019.00496
Received: 01 July 2019; Accepted: 04 December 2019;
Published: 19 December 2019.
Edited by:
Laura Parducci, Uppsala University, SwedenReviewed by:
Cristiano Vernesi, Fondazione Edmund Mach, ItalyMichael Hofreiter, University of Potsdam, Germany
Copyright © 2019 Verry, Scarsbrook, Scofield, Tennyson, Weston, Robertson and Rawlence. This is an open-access article distributed under the terms of the Creative Commons Attribution License (CC BY). The use, distribution or reproduction in other forums is permitted, provided the original author(s) and the copyright owner(s) are credited and that the original publication in this journal is cited, in accordance with accepted academic practice. No use, distribution or reproduction is permitted which does not comply with these terms.
*Correspondence: Alexander J. F. Verry, dmVycnlhbGV4OTRAZ21haWwuY29t