- 1Zoolab, Department of Life Sciences and Systems Biology, Turin University, Turin, Italy
- 2Museum and Institute of Zoology, Polish Academy of Sciences, Warsaw, Poland
Ant nests and their surrounding territories represent a hoard of trophic resources, as well as of stable and protected environments for many arthropods involved in commensal, mutualistic, or parasitic associations. Among these organisms, called myrmecophiles, several are butterflies. Here, we explore the amazing diversity of strategies developed by myrmecophilous butterflies to “cheat” or manipulate ants and to elude the tough defenses of the colony. During oviposition, female butterflies use visual or plant volatile signals to identify the presence of ants, whereas chemical and vibroacoustic cues, either isolated or combined, are used by larvae and pupae to attract, deceive, or appease workers. Examples of mimicry and eavesdropping on both intraspecific and interspecific signals are discussed, primarily referring to the obligate-parasitic interactions involving Maculinea butterflies and Myrmica ants. Multimodal communication is crucial to maintaining the strong cohesion and social structure of ant societies, but its corruption is at the base of the evolution and persistence of interspecific associations, which can be beneficial or detrimental for the colony's fitness. In this framework, the remarkable complexity of signaling could have prompted the evolution of specialized life cycles enhancing the extraordinary butterfly diversity.
Introduction and Outlines
Peculiar attributes of ants such as their ecological dominance, wide distribution, and eusocial organization led to the evolution of a variety of associations sometimes with distantly-related organisms, called myrmecophiles (ant-loving) (Hölldobler and Wilson, 1990).
Myrmecophilous organisms tend to be exceptionally cryptic and spend variable amounts of their lives within insect societies. Therefore, the occurrence of myrmecophily across taxa (e.g., Hymenoptera, Diptera, Coleoptera, and Lepidoptera) could have been deeply underestimated. Nevertheless, based on Donisthorpe's work (Donisthorpe, 1927) and several other studies, Thomas et al. (2005) estimated that around 100,000 myrmecophilous species of invertebrates exist.
Among the others, the diversity, functional and evolutionary ecology of butterfly-ant interactions have been extensively reviewed by Hinton (1951), Malicky (1969), DeVries (1991b), Fiedler (1991, 1994), and Pierce et al. (2002). However, little attention has been paid to the signaling system necessary to foster and maintain myrmecophilous associations, even though interspecific communication is vital for these relationships.
By means of food secretion or furtiveness, the majority of myrmecophiles (commensals or mutualists) live undisturbed or actively protected within the territories of ants, but others are specialized to overcome the colony defenses and succeed in entering the ant nests (Hölldobler and Wilson, 1990; Thomas et al., 2005). Some myrmecophiles are obligately associated with ants and have evolved remarkable strategies “to break the communication code” of their symbionts (Hinton, 1951; Schönrogge et al., 2017). Being able to exploit the fiercely defended ant territory or the nest itself by “cheating” ants would provide the myrmecophilous organisms with several advantages [e.g., protection against predator and parasites, shelter, transport, and a potential depletion of pathogen contamination (New, 2017)] as well as a persistent source of food and protection (Wasmann, 1913; Barbero, 2016).
DeVries (1991c) suggested that butterfly-ant associations could have arisen as a consequence of earlier and long-lasting interactions between ants and angiosperms (Moreau et al., 2006). Ants can exploit plants or their litter as nesting resources (Wilson and Hölldobler, 2005) but can also prey on or benefit of the secretions elicited by plants or plant-associated herbivores (Davidson et al., 2003). To take advantages of ant protection against their natural enemies, plants have evolved amazing adaptations to attract them directly via extrafloral nectar exudations or indirectly by means of honey-dew secreting hemipterans (Rico-Gray and Oliveira, 2007). On the other hand, butterflies, that primarily need plants as food for their larval offspring, also started to appease patrolling ants to obtain protection against predators or parasitoids in the most vulnerable period of their life (i.e., larval development and pupation). This results in a faster growth rate and a higher survival or better reproductive success of attended individuals with respect to unattended ones (Cottrell, 1984; Pierce et al., 1987; Kaminski et al., 2013).
The larvae and pupae of myrmecophilous species lack a broad array of protections typical of other butterflies, such as defensive secretions, dense “hairiness,” or sequestration of toxic plant metabolites and associated aposematic colorations (Fiedler, 1991). However, in addition to food rewards by specialized organs, butterflies have developed an array of morpho-physiological, behavioral, chemical, or acoustic adaptations to attract, trick, alarm, or pacify their associated ants, which is discussed here.
Our review is narrowed to the exchange, emission, perception, manipulation, and eavesdropping of signals occurring in associations involving myrmecophilous butterflies because these systems (i) have been studied by experts of distinct disciplines providing insights on their function and biology; (ii) are textbook models of coevolutionary dynamics; (iii) often involve other trophic levels (e.g., plants and parasitoids) thereby providing the rare opportunity to explore the complexity of the “information web” interlaced with the “food web” (Dicke, 2000).
Given that myrmecophily is mostly present in two butterfly families (Lycaenidae and Riodinidae), which encompass about 30% of all butterfly species (Shields, 1989) most of the studies were performed on these taxa. Within these families, 75% of the species possess juvenile instars interacting with ants at various stages of the life cycle showing a variety of associations ranging from facultative to obligate and from mutualistic to parasitic (Pierce et al., 2002). For those lycaenid species whose life history is fully known, about 30% are closely associated with ants (obligate myrmecophiles), 45% are facultative myrmecophiles and about 25% show no association with ants (Pierce et al., 2002).
Depending on their degree of interaction, which summarizes time, space, kind, and specificity of the associations (Hinton, 1951), myrmecophilous adult butterflies can (i) use direct and indirect signals to detect the presence of ants in order to select ideal oviposition sites (see Signals Used During Butterfly Oviposition); while immature stages can (ii) secrete pacifying, rewarding or manipulating substances by dorsal nectary organs (DNOs) to gain protection against predators (see Chemical Signals); (iii) release allomones or alarming volatile compounds from tentacle organs (TOs) or other specialized organs to get access to their food sources (see Chemical Signals); (iv) subvert the nestmate recognition system based on cuticular hydrocarbons (CHCs) to enter and live in the ant nest (see Chemical Signals); and (v) produce mimetic vibroacoustic signals to attract the attention of ants (see Vibroacoustic Signals).
After introducing signal systems occurring in facultative and unspecialized interactions, we focus our review on obligate parasitic associations. In extreme cases, such as in the parasitic Maculinea butterflies, the interaction is so tight and close that requires the corruption of several host signals (both chemical and acoustical) for the butterfly juveniles to be accepted and treated like colony members by the host ants. These butterflies show a peculiar life cycle (Thomas, 1984) (Figure 1), which will be described throughout this review, highlighting the multimodal signaling which makes this parasitism successful. In brief, after egg-laying on species-specific food plants occurs, larvae spend a short phytophagous period gaining little body mass. Then caterpillars leave the flower buds and wait motionless before being discovered by a Myrmica ant. Following an adoption ritual of variable duration depending on the species, during which behavioral (Fiedler, 1990), chemical (Akino et al., 1999; Nash et al., 2008), and vibroacoustic (Sala et al., 2014) signals are used by the parasite in order to pretend to be a Myrmica larva, the “cheated” forager ant carries the parasite within its nest. Once inside the colony, Maculinea caterpillars integrate into the host colony by using chemical and vibroacoustic deceiving signals (Schönrogge et al., 2004; Barbero et al., 2009b). Some species directly prey on the ant brood (“predatory” species), while others (“cuckoo” species) are fed by food regurgitation by the nurse workers. In the nest, the final larval body mass is achieved and pupation occurs in the upper nest chambers. Thus, after 11–23 months -as 2-year developing larvae exist within the same population to cope with catastrophic events or habitat unpredictability (Thomas et al., 1998; Witek et al., 2006)- Maculinea butterflies leave the ant nest as adults.
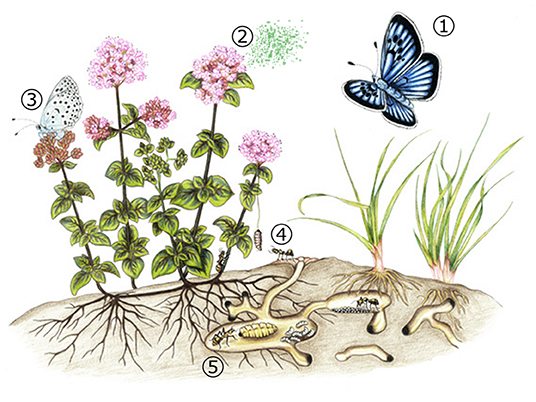
Figure 1. Life cycle of Maculinea arion and its interaction with Myrmica ants and Origanum vulgare following Patricelli et al. (2015). (1) Gravid female butterflies are visually attracted to the green-red buds of O. vulgare; when in their proximity, (2) by detecting the monoterpenoid volatile carvacrol emitted by plants co-occurring with ants of any Myrmica species (3) female lays eggs on phenologically suitable flower buds; (4) fourth-instar caterpillars leave the host plant and are “adopted” by Myrmica ants; (5) M. arion caterpillars spend 11 months within Myrmica colonies, feeding on ant brood and acquiring more than 98% of their final biomass. Artwork by Elisa Plazio.
Primarily using the case of Maculinea butterflies, we endeavor to highlight the importance of surveying the signaling beyond the pairwise level of organism interactions, by providing evidence that eavesdropping might occur when multiple connections (ant, butterfly, plant, parasitoids) are considered. Communication and its corruption are at the base of the evolution and maintenance of multiple interactions, and we believe that community context approaches are ideal to assess properly all the selective pressures shaping these complex systems. We discuss the hypothesis by several authors (Malicky, 1969; Atsatt, 1981; DeVries, 1991b,c; Fiedler, 1998; Pierce et al., 2002; Stadler et al., 2003; Pech et al., 2004; Pellissier et al., 2012; Schär et al., 2018) that the interactions with ants represented one of the most important factors in the butterfly (Lycaenidae) adaptive radiation.
Signals Used During Butterfly Oviposition
Female choice of an ideal egg-laying site is fundamental for offspring survival and consequently for the persistence of butterfly populations (Chew and Robbins, 1984; Renwick and Chew, 1994). The observed egg distribution on larval host plants (LHPs) is the outcome of several biotic and abiotic factors, such as the plant species, quality or distribution, larval intraspecific competition, microclimatic conditions, presence of mutualists or predators (Chew and Robbins, 1984; Renwick and Chew, 1994). Since early records of myrmecophilous behavior in butterflies, several authors have argued that selection may have favored the ability to locate both the LHPs and the host ants (Pierce and Elgar, 1985; Fiedler and Maschwitz, 1989a,b; Jordano et al., 1992; Wagner and Kurina, 1997; Patricelli et al., 2011). Laying eggs in the proximity of ant colonies would increase the probability of the juveniles to encounter the tending workers and this achievement is even more crucial in strictly obligate myrmecophilous species (Van Dyck et al., 2000). Hence it has been hypothesized that ants themselves, the release of their pheromones or trail compounds can serve as mating and oviposition cues.
The first empirical evidence of ant-mediated oviposition was gathered through field studies assessing lycaenid egg distribution on food plants with respect to ant presence/absence. Pierce and Elgar (1985) reported that females of the obligate mutualist species, Jalmenus evagoras, laid their eggs primarily on food plants colonized by honeydew-producing aphids to maximize chances of their larvae to be visited by the Iridomyrmex host ants. However, J. evagoras females did not perceive the workers directly, but followed indirect visual cues (i.e., the aphid occurrence) as proxies for the ant presence (Pierce and Elgar, 1985). In this species, males use the presence of ants and conspecific adults as mating signals also to spot newly-emerged females (Elgar and Pierce, 1988). Further field and laboratory experiments revealed that J. evagoras females are able to discriminate between species and to some extent also populations of attendant ants and lay their eggs close to their dominant and syntopic ant species (Fraser et al., 2002). Beyond suggesting that oviposition preferences could have a genetic basis, these results recall the possibility that “a behavioral imprinting process” where the ability to perceive chemical and visuals signals produced by the associated ants is established during butterfly development (Fraser et al., 2002). Yet, the direct perception of ant chemicals by gravid females remains to be formally tested.
Despite earlier insights (Pierce et al., 2002), recent works suggest the occurrence of ant-related oviposition choices also in facultative associations (Rodrigues et al., 2010; Bächtold et al., 2014). Most eggs and larvae of the facultative lycaenid, Allosmaitia strophius, were laid on stems of Peixotoa tomentosa an extrafloral nectaried shrub where Camponotus blandus and Ectatomma tuberculatum ants occur (Bächtold et al., 2014). Unfortunately, authors did not perform an in-depth survey of the signals used by gravid females to select plants visited by ants, which are therefore unknown. We cannot rule out that only visual cues are employed as they can be exceptionally precise, like it was demonstrated in the non-myrmecophilous Eunica bechina, a nymphalid butterfly which lays eggs on an ant-defended plant. In this case, however, females are able to discriminate the shape of more aggressive ants by sight thus preventing the oviposition on deadly ant-patrolled plants (Sendoya et al., 2009).
Although a strong ant-driven oviposition behavior is expected to maximize offspring survival in the obligate parasites of the genus Maculinea, which are locally hosted by one or few ant species and exploit rare and specific LHPs (Thomas et al., 1989), contrasting results are found for distinct populations. While field data consistently support the female ability to select specific LHP parts, characteristics, and bud blooming stages, some studies pointed out that females select LHPs primarily on the basis of the plant phenology rather than on the local host ant presence (Thomas and Elmes, 2001; Musche et al., 2006; Fürst and Nash, 2009; Czekes et al., 2014). Others revealed a role of the host ant distribution on the egg-laying pattern (Van Dyck et al., 2000; Wynhoff et al., 2008), but this outcome has been explained by Thomas and Elmes (2001) as the consequence of a food plant niche selection rather than a direct ant-driven oviposition choice. Wynhoff et al. (2015) found that the selection of the LHP by Maculinea alcon is not influenced by the host ant presence, but the number of eggs laid (egg load) increased on plants growing close to a Myrmica nest. In M. rebeli, a M. alcon close-related species, both the egg-laying probability and the egg loads on Gentiana asclepiadea are affected by the abundance of the host ant, M. scabrinodis (Carleial et al., 2018). The oviposition pattern observed is not a mere consequence of the positive effect of plant traits, such as flower numbers (also selected by the gravid females for egg laying), on Myrmica scabrinodis distribution. Authors highlighted that both the plant features and the host ant presence have direct, distinct, and positive effects on oviposition choices, which cannot be considered as a spurious outcome of niche selection (Carleial et al., 2018). The majority of earlier studies on oviposition choices did not account for the contribution of all Myrmica species occurring in the surrounding of LHPs but focused on the local host ant distribution. In contrast, Patricelli et al. (2011) found a strong correlation between Maculinea arion egg-laying preferences and the occurrence of any (not necessarily the local host) Myrmica species around the LHPs chosen. Approximately 80% of egg-laying events occurred on LHPs surrounded by Myrmica ants, suggesting the existence of a mechanisms by which females are able to identify plants growing in the proximity of a Myrmica colony.
An ant-mediated oviposition behavior is not so straightforward in all Maculinea species or populations, whereas plant features are crucial. Visual cues can be employed to spot the plant phenology and after landing females might avail themselves of the chemical cues related to the ant presence to decide how many eggs are worth laying. Although in other myrmecophilous butterflies the ability to directly detect the presence of ants by chemical or visual cues have been inferred or shown (respectively), this seems unlikely to occur in the Maculinea-Myrmica system (Thomas and Elmes, 2001) because: (i) Myrmica ants usually forage when female butterflies are less active in laying eggs, (ii) workers release highly volatile pheromone trails which fade quickly thus making it difficult for Maculinea females to follow them, and (iii) females do not frequently exhibit complex searching behavior (but see Van Dyck et al., 2000).
Females possess the full array of organs and receptors on antennae, tarsi, mouthparts or ovipositor to enable a precise detection of several chemical signals in order to select the right LHPs and to assess their quality. Therefore, some authors started to hypothesize that induced plant volatiles might work as indirect signals for the ant presence and provide hints for the egg-laying females (Van Dyck and Regniers, 2010; Wynhoff et al., 2015). Patricelli et al. (2015) provided the first evidence that Origanum vulgare plants, the LHP of M. arion, react to Myrmica workers by releasing a volatile organic compound (carvacrol), which in turn is used by gravid females to locate ideal food plants growing close to a Myrmica nest (Figure 1). Experimental O. vulgare plants grown with ants upregulate genes involved in the monoterpenes pathway thus releasing higher amounts of carvacrol than control plants (without ants). This monoterpene is perceived by butterfly antennae and attracts the gravid females in laboratory choice tests. Carvacrol has detrimental effects on ants, but by the upregulations of detoxifying genes these workers survive longer than other common ant species to environmental concentrations of this monoterpene compound. Therefore, authors initially suggested that Myrmica ants benefit from being resistant to carvacrol by occupying enemy-free spaces close to O. vulgare plants at the cost of enhancing their chances of being parasitized by M. arion. Very recently, it has been shown that this ant-plant interaction could be maintained through manipulation signals. Mixtures of carvacrol and thymol decrease Myrmica ant movements and increase their aggressive behaviors acting on the brain levels of biogenic amines (Mannino et al., 2018). Therefore, similarly to the scenario described by Hojo et al. (2015) (for further details see below), here oregano seems to manipulate Myrmica ants by volatile cues in order to increase partner fidelity and obtain protection against herbivores by patrolling workers.
Using a multidisciplinary approach, authors shed light on the indirect mechanism used by M. arion females to detect the presence of the Myrmica ants by means of a very tiny variation in the LHP volatilome (Patricelli et al., 2015) and on the way a certain degree of overlap between the two butterfly resources, LHPs and Myrmica ants, can be maintained (Mannino et al., 2018).
During the revision of papers dealing with oviposition behavior in myrmecophilous butterflies we did not find any robust evidence of the fact that females are able to directly detect the ant presence by perceiving their chemical cues. Indeed, this is only partially surprising because (i) butterflies coevolved primarily with their host plants, achieving remarkable abilities in sensing their signal variation as proxies for plant features and phenological states; and (ii) a chemical reaction is the most probable response of a plant which is interacting with insects, including ants. Therefore, it is likely that among the multifarious plant signals are those exploited by females to indirectly gain information about their LHP degree of association with ants.
Chemical Signals
The interaction between immature butterfly stages and ants is mediated above all by different mechanisms that involve the production of chemical substances.
Nectary Organs
In several species belonging to the subfamily Lycaenidae and Riodinidae one of the main mechanisms that allow larvae to manipulate ant behaviors by attracting and maintaining the attention of workers is the production of rewarding exudations. In lycaenids these secretions are released from the dorsal nectar organ (Figure 2B) (DNO; Newcomer, 1912; Fiedler and Maschwitz, 1989c; Leimar and Axén, 1993; Pierce et al., 2002) located on the seventh abdominal segment and in riodinids from paired tentacle nectary organs (TNOs) located on the eighth abdominal segment (DeVries, 1988).
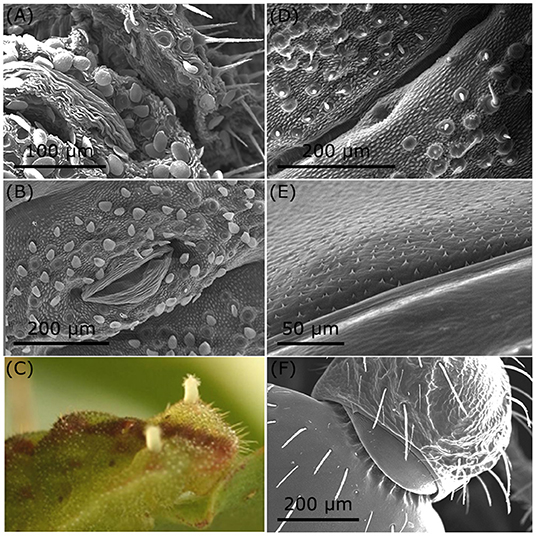
Figure 2. Morphology of organs producing chemicals and vibroacoustic signals in myrmecophiles and their host ants. (A) Pore cupola organs (PCOs) and (B) dorsal nectary organ (DNO) of fourth-instar Maculinea rebeli caterpillars; (C) tentacular organs of fourth-stage Scolitantides orion caterpillar; (D) the possible sound-producing organ of M. rebeli caterpillars; (E) stridulatory organ of M. rebeli pupa, formed by a stridulatory plate (pars stridens) located on the sixth abdominal segment and a file (plectrum) in the fifth abdominal segment. (F) Stridulatory organ of Myrmica sabuleti.
In Lycaenidae, many factors influence the production of rewarding secretions made available to mutualistic ants (Agrawal and Fordyce, 2000). When exposed to simulated threats, caterpillars of both Polyommatus icarus (Leimar and Axén, 1993) and Plebejus acmon (Agrawal and Fordyce, 2000) secrete more rewards and attract a higher number of attendant ants. However, beyond a threshold number of attendant ants, the benefit from producing metabolically expensive secretions may have scarce returns (Leimar and Axén, 1993; Axén et al., 1996). The rate at which larvae provide rewards may also depend on social context. Aggregated caterpillars of Jalmenus evagoras deliver less food rewards to ants than solitary larvae, and secretion rate decreases with increasing group size. Also, secretion rates are lower when IV stage caterpillars are paired with bigger V instar larva than when aggregate to a smaller III instar individual (Axén and Pierce, 1998).
In general, carbohydrates and amino acids represent the main components of these secretions, whose composition is unlikely to be explained by caterpillar diet (DeVries, 1988) or by the contents of the hemolymph but is more likely to be genetically determined and related to the degree of association with ants (Pierce et al., 1987; Daniels et al., 2005). In all species of Lycaenidae and Riodinidae analyzed so far, the secretions contain different combinations of sugars, one of them being usually more abundant. Sugars are principally sucrose and glucose (Daniels et al., 2005) in dilutions of around 5–10%, except for the Australian species Paralucia aurifera where glucose, the only carbohydrate, reaches average concentrations of 34% (Cushman et al., 1994). Secretions of the parasitic butterfly Niphanda fusca, contain three types of sugars of which trehalose shows the greatest concentrations (380 mmol 1-1) (Hojo et al., 2009), while in the obligate mutualist Jalmenus evagoras secretions consist of about 10% (dry weight) of sucrose and fructose (Pierce and Nash, 1999). The latter were the main components also in the droplets of the facultative mutualists Polyommatus hispanus (Maschwitz et al., 1975), Polyommatus coridon, P. icarus, and Zizeeria knysna (Daniels et al., 2005).
Also the amino acid content of the dorsal nectar, whose concentration is slightly higher than in aphid honeydew (Yao and Akimoto, 2002) and in floral and extrafloral nectars (Blüthgen et al., 2004), seems to be determined by the intimacy of associations with ant attendants, since a richer and more diversified mixture is likely to be produced by more strongly myrmecophilous species (Pierce et al., 1987; Daniels et al., 2005). For instance, the amino acid content of P. coridon secretions, one of the most strongly ant-associated, among facultative myrmecophilous species, reaches the highest concentrations (108 mmol l-1) among the caterpillars analyzed so far, with leucine being the predominant constituent (Daniels et al., 2005). In contrast, P. icarus and Z. knysna, which are weakly myrmecophilous, have low amino acid levels (respectively, 10 and 6 mmol l−1).
Several authors have demonstrated that the quantity and quality of nutritive rewards influence the persistence of guarding ants in attending caterpillars (Pierce et al., 2002). DNO secretions of the parasitic caterpillars of Niphanda fusca contain high concentrations of the amino acid glycine associated to several carbohydrates, i.e., mostly trehalose (Hojo et al., 2009) and glucose (Wada et al., 2001). Electrophysiological recordings of the taste receptors of the host ant species, C. japonicus, showed that the presence of even small amounts of glycine, combined with the main sugars, made attendant ants more attracted to these solutions and enhanced the electrophysiological response to sugar of sugar-receptor cells (Wada et al., 2001; Hojo et al., 2009).
Recent observations (Hojo et al., 2015) suggest that DNO droplets may be more than simply nutritious recompenses. In a supposed mutualistic lycaenid, Narathura japonica, DNO secretions, or CHCs (see below) alone do not elicit ant-caterpillar interaction, but together they act synergistically to promote ant allegiance (Hojo et al., 2014). DNO caterpillar secretions lower the locomotory activities of their attendant Pristomyrmex punctatus ants (Hojo et al., 2015) and increase the frequency of aggressive responses to tentacle organ eversion. Analysis of the neurogenic amines in the brains of ants that consumed caterpillar secretions showed a significant decrease in levels of dopamine, suggesting that DNO secretions of lycaenid larvae can manipulate attendant ant behavior by altering dopaminergic regulation and increasing partner fidelity (Hojo et al., 2015). Because a net nutritional benefit from the DNO droplets is doubtful, authors suggested this interaction which has been traditionally considered a mutualism could be indeed a parasitic association.
In parasitic lycaenid butterflies possessing in some cases large functional DNOs (Samson, 1989; Nomura, 1992; Sanetra and Fiedler, 1996), it is likely that ant manipulation is achieved solely by using other chemical (and vibroacoustic) strategies, e.g., chemical mimicry of CHCs (see below). For instance, final larval instars of Maculinea rebeli spend on average 18% of time actively secreting DNO droplets during the 11–23 months spent within Myrmica colonies (Elmes et al., 1991). Yet, they do not recycle sufficient sugar through their secretions to provide significant rewards to the adult ants, which in turn experience higher mortality and lower fitness when the colony is parasitized by M. rebeli (Wardlaw et al., 2000).
Tentacle Organs
The larvae of many myrmecophilous Lycaenidae and Riodinidae have a second pair of extensible organs to interact with ants. In lycaenids these organs, called “tentacle organs” -TO- (Figure 2C) (Cottrell, 1984; Kitching and Luke, 1985), are located on the eighth abdominal segment while a pair of “anterior tentacle organs” -ATO- (DeVries, 1988) has been detected on the third body segment of caterpillars of all myrmecophilous riodinids, except for the genus Eurybia (Harvey, 1987; DeVries, 1991c).
Although the function of tentacle organs has been debated over several decades, it has not been fully clarified yet. Some authors suggested that TOs may induce a response in ants by functioning as a tactile or visual cue (Murray, 1935; Malicky, 1969). This hypothesis may be partially corroborated by a recent work by Gnatzy et al. (2017) that investigated the internal fine structure of the TOs of P. coridon and P. icarus larvae and failed to find evident glandular structure or sign of secretory activity.
However, several authors have reported that the eversion of the TOs induce alertness or even alarm behavior in the attendant ants (Claassens and Dickson, 1977; Fiedler and Maschwitz, 1988, 1989c). Ants' response is usually observed only at a close range from the tentacle organs and not all ant species exhibit a reaction when a certain species of lycaenid extrudes its organs. The similarity of behavior of an attendant ant to ant reaction during a threatening situation, the specificity of the observed reaction, and the short range of activity led several authors (Henning, 1983; DeVries, 1984; Kitching and Luke, 1985) to suggest that TOs could produce volatile compounds mimicking ant alarm pheromones.
Support for this pheromone-mimic hypothesis initially came from a study by Henning (1983) who was able to obtain a dichloromethane extract of the TOs and the surrounding body area of the lycaenid Aloeides dentatis. In behavioral assays, alarming responses obtained from Acantholepis capensis attendant ants exposed to these extracts were similar to those of ants presented with dichloromethane extracts from conspecific mandibular glands (Henning, 1983).
A few years later, the secretions from the tentacle organ of another lycaenid species, Shirozua jonasi, were shown to contain dendrolasin, a C15 furan sequiterpenoid (Yamagushi and Shirozu, 1988), firstly isolated from the mandible glands of the ant Lasius fuliginosus where it may function as an alarm pheromone (Quilico et al., 1957). However, the chemicals released from the TOs and ATOs remain largely unknown (Pierce and Nash, 1999).
Pore Cupola Organs
On the cuticle of all lycaenid and riodinid immature stages, except for the myrmecophage Liphyra brassolis (Fiedler, 1991), a third set of minute epidermal glandular structures called pore cupola organs -PCOs- (Cottrell, 1984) are present (Figure 2A). Their morphology and distribution differ markedly between taxa (Kitching, 1987), but PCOs are generally present starting from the first instar and their density increases at every molt (Malicky, 1969). Also, in most lycaenid species PCOs concentrate around the spiracles and (if present) around the DNO (Fiedler, 1991).
Some caterpillars can be visited by several ants (Pierce, 1984) and caterpillars equipped with PCOs can elicit antennae behavior at different intensities. Some species arouse little attraction in ants, while others receive constant antennation (Malicky, 1969; Fiedler, 1991). During ant-caterpillar interactions, the densest PCO areas are frequently antennated and groomed by ants, suggesting that they may secrete ant-appeasing substances. In other cases, the signals have been considered as pacification cues (Maschwitz et al., 1985). PCOs can secrete amino acids in some species (Pierce, 1984). Malicky (1969) suggested that these chemical signals suppress ant aggression by mimicking ant chemical cues and specifically those emitted by the colony brood. More specifically, given that ant interest arises only after the first contact, it has been suggested that PCOs may be responsible for the production of CHCs, non-volatile compounds (Pierce, 1984) which are known for signaling colony membership, in ant societies.
However, whether PCOs are involved in the production of CHCs remains to be confirmed. Especially because, while PCOs are unique to lycaenid caterpillars, CHCs are universal among insects being primarily important in preventing desiccation (Gibbs, 1998) and facilitating osmoregulation (Nelson and Blomquist, 1995).
Cuticular Hydrocarbons
It is well-known that various myrmecophiles including butterflies exhibit CHCs that mimic those of their ant hosts to avoid ant attacks (for butterflies, Akino et al., 1999; Hojo et al., 2009; Barbero, 2016; Mizuno et al., 2018). In general, CHCs play a fundamental role in the nestmate recognition of social insects, and in particular of ants (van Zweden and d'Ettorre, 2010). Ants living in the same colony share a mixture of chemicals, which function as a “colony odor” and enables them to discriminate between nestmates and enemies (Lenoir et al., 2001).
Therefore, irrespective of their intensity of interaction, acquiring a composition of CHCs that are recognized and accepted by the attending ants is the most common, and almost essential, strategy for myrmecophilous butterfly caterpillars to exploit ant societies (Barbero, 2016 and references therein).
Hojo et al. (2014) demonstrated that CHCs are used by the mutualistic lycaenid N. japonica to be recognized by its tending ants P. punctatus. Indeed, glass beads coated with crude cuticular chemicals of N. japonica and CHC fractions extracted from caterpillars were significantly more tended by P. punctatus ants than control glass dummies or dummies coated with the non-hydrocarbon fraction. A comparison of caterpillar cuticle extracts of N. japonica and the myrmecoxenous Lycaena phlaeas revealed that the total amount of CHCs were not significantly different but differed in composition. While caterpillars of L. phlaeas have a simple set of hydrocarbons, mainly n-alkanes, N. japonica caterpillars have a complex mixture of n-alkanes, n-alkenes, and n-alkadienes, so that it is likely that the host ant would selectively recognize the unsaturated hydrocarbon fraction and use it for its nestmate recognition system (Hojo et al., 2014).
In another facultative myrmecophilous butterfly, Lycaeides argyrognomon, the CHC profiles of larvae and pupae are almost identical (a few methyl-branched alkanes lacking in the pupae), but differ from those of any host ant species (Omura et al., 2009). Unlike parasitic lycaenid larvae (see below), mutualistic lycaenids probably have not evolved CHC profiles mimicking ant CHCs of a particular species (Omura et al., 2009). The CHC profiles of L. argyrognomon larvae and pupae are dominated by n-alkanes, which may contribute to the lack of ant aggression (Dani et al., 2001; Omura et al., 2009). It is likely that in mutualistic species, associative learning of chemicals and DNO reward secretion can be accomplished with one or a few compounds (Guerrieri et al., 2009). Indeed, workers of several ant species can learn a specific blend of hydrocarbons when provided with nectar solutions (Bos et al., 2012; Hojo et al., 2014). In addition, in mutualistic butterflies other mechanism of ant manipulation may exist. Mizuno et al. (2018) found that cuticular lipids of pupae do not include only CHCs but also several long-chained aliphatic aldehydes, including 1-octacosanal and 1-triacontanal, which are absent from the larva and are responsible for suppressing ant aggression in certain attending ants during the pupal stage (Mizuno et al., 2018).
However, it is straightforward that the need to possess specific chemical adaptations to bypass the chemical recognition barriers is essential in obligate myrmecophiles, which enter the ant colonies to exploit their resources (Singer, 1998; Barbero, 2016). Obligate caterpillars imitate the CHC profile of their ant hosts to be adopted as nestmates and thereby integrate into the colonies of their hosts (Akino et al., 1999; Hojo et al., 2009). Henning (1983) was first to demonstrate that caterpillars of the inquiline species Lepidochrysops ignota possess cuticular compounds inducing carrying and brood-caring behavior in the specific host ant, Camponotus niveosetosus. Interestingly, post adoption caterpillars of the parasitic lycaenid butterfly Niphanda fusca exploit worker care by imitating the CHC profiles of host ant males rather than of the ant larvae of their host Camponotus japonicus (Hojo et al., 2009). Since the parasitic caterpillars are principally attended by workers and do not actively contact the host males, it is possible that N. fusca larvae are able to biosynthesize the mimetic CHCs that serve for integrating into the host colony (Hojo et al., 2009).
Probably the most intensively studied system from a chemical point of view is that of the parasitic species belonging to the genus Maculinea, whose strategies of chemical deception will be treated in detail.
Elmes et al. (1991) initially suggested that the system by which the butterflies of the genus Maculinea could enter the colonies of Myrmica ants was mediated by the imitation of host chemical signals. This hypothesis was confirmed by Akino et al. (1999) for the cuckoo species, Maculinea rebeli. In behavioral tests, workers of the host ant Myrmica schencki carried into their nest glass dummies covered with cuticular extracts of fourth-instar caterpillars.
The comparison between the CHCs of M. rebeli caterpillars and M. schencki workers confirmed that during the pre-adoption phase the parasitic caterpillars possess a CHC profile that weakly mimics that of its main host species (Akino et al., 1999; Elmes et al., 2002; Schönrogge et al., 2004). Compared to its host ants which usually have complex CHC profiles, the M. rebeli pre-adoption caterpillars have only a dozen of CHC compounds, most of which are linear alkanes, while methylated alkanes usually constitute no more than 5% of the total amount of linear compounds (Akino et al., 1999; Elmes et al., 2002; Schönrogge et al., 2004).
The CHC cuticular fraction seems to contain chemical cues which can be recognized by any Myrmica ant encountering a fourth-instar larva leaving its food plant. Nevertheless, the matching of host surface hydrocarbons can largely influence the adoption time and explain the differences in host use observed in Maculinea populations (Nash et al., 2008). For instance, caterpillars of the other cuckoo species, M. alcon, from Denmark are adopted an order of magnitude faster by colonies of M. rubra or M. ruginodis, which are suitable as host colonies, than by colonies of non-host M. scabrinodis (Als et al., 2001).
In a recent study where the chemical profiles of M. rebeli pre-adoption larvae were compared to those of Myrmica workers sampled in Italy at six locations, authors found a direct relationship between the chemical similarity of caterpillars and host workers, and the estimated survival rate of the social parasite within the ant colonies where more than one host species is used. The chemical similarity between pre-adoption M. rebeli larvae and Myrmica ants explained a significant proportion (around 30%) of the variation in the estimated survival of parasitic caterpillars (Casacci et al., 2019). Similar results were found by Thomas et al. (2013) who demonstrated that differences in the CHC profiles of two M. rebeli populations from Spain and Poland are strong enough to explain the differences in the local host ant use.
Additionally, it is likely for the adoption process to be mediated by few active compounds as shown by Solazzo et al. (2014). Indeed, through behavioral assays and chemical analyses, the authors proposed that tetracosane, a low volatility compound present on M. nausithous cuticle in the pre-adoption phase, plays a role in this process by enhancing the first interaction with M. rubra foraging workers.
After 1 week within the host colony, the CHC profile of M. rebeli caterpillars change and larvae become chemically closer (more than 60% similarity) to their hosts (Schönrogge et al., 2004). Initially, it was hypothesized that the complete integration of the parasitic larvae depended on the passive absorption of colony odors through contact and exchange of secretions with the workers know as “chemical camouflage” (sensu Dettner and Liepert, 1994). Subsequently, however, it was highlighted that individuals that overcame the period of “initial integration” survived well with non-host species of Myrmica, only as long as the colony remained well-nourished and in favorable conditions. If, however, as often happens in nature, the colony was undergoing a lack of trophic resources or other types of stress, the larvae of M. rebeli continued to survive well with the local host Myrmica schencki, while caterpillars in Myrmica “non-host” colonies were killed and used as food for ant larvae (Elmes et al., 2004). The camouflage hypothesis could not, however, explain why survival was extremely low in “non-host” colonies under stress. If we admit that the larvae of M. rebeli passively acquire the smell of a colony, then they should parasitize with the same success any species of the genus Myrmica, having passed the initial period of integration (Elmes et al., 2002). Therefore, it became clear that the larvae of M. rebeli are able to actively synthesize (mimicry sensu Dettner and Liepert, 1994) additional hydrocarbon compounds that increase and amplify the overlapping (mimicry) with the (host) model (Elmes et al., 2002, 2004; Schönrogge et al., 2004).
While the mechanisms allowing the chemical integration of cuckoo species of Maculinea butterflies (M. rebeli and M. alcon) have been extensively explored, only a few studies have investigated those evolved by predatory species. Data on the CHC profiles have shown that the post-adoption larvae of predatory M. teleius do not achieve the same level of chemical integration as cuckoo caterpillars. In a study conducted on two Polish populations the level of chemical similarity of the parasite caterpillars varied between 32 and 60% depending on the host species considered (Witek et al., 2013). This is in line with the fact that M. teleius is the most generalist species of the genus and is usually less locally specific to single Myrmica host (Witek et al., 2014; Tartally et al., 2019). The highest degree of chemical similarity was found within the host colonies of M. rubra at both investigated sites, suggesting that an ancestral association with this Myrmica species may exist. Differently from cuckoo M. alcon, whose larvae acquire a limited number of CHCs (at maximum 28 peaks in the post-adoption phase), M. teleius caterpillars showed very complex profiles which ranged from 57 CHC peaks to more than 70, i.e., almost double than shown in the CHC profile of Myrmica workers. These results, even if derived from a limited number of samples, suggest that the predatory species may have evolved a different mechanism of integration, possibly based on the acquisition of compounds by contact with the host species or by feeding on the ant brood (Witek et al., 2013).
On the contrary, the less studied Maculinea species, M. nausithous, is known to parasitize primarily a single host (My rubra) throughout its distribution range, from Western Europe to Southern Siberia and Mongolia (Witek et al., 2014; Tartally et al., 2019). Nevertheless, M. nausithous caterpillars do not acquire the same degree of chemical similarity as M. alcon does within its host colonies. This level (43%) is also lower than that of the M. teleius larvae, sharing the same M. rubra colonies, but higher than M. teleius caterpillars exploiting other Myrmica ants (Witek et al., 2013). In addition, its CHC profile seems intermediate between those of cuckoo and predatory species, suggesting that it may have evolved an intermediate strategy based both on mimicry of the host CHC profile and on chemical camouflage (Patricelli et al., 2010). Although still untested, the latter hypothesis finds some support in large-scale ecological studies, as M. nausithous shows intermediate host-specify patterns, differing form predatory species in coevolutionary trajectories and from cuckoos in local adaptation strategies (Tartally et al., 2019).
Vibroacoustic Signals
Insect communication can also be achieved by generating mechanical signals that cause a perturbation of the conveying medium. Compared to chemicals, vibroacoustic cues allow sending rapid, directional and quickly adjustable signals at both short or long-range (Frings and Frings, 1958; Hunt and Richard, 2013).
In ants, vibroacoustic communication is currently known to play important roles in colony life, such as in defense and signaling of alarm, territory, mating, rescue, recruitment, and social or caste status (Hölldobler and Wilson, 1990; Hickling and Brown, 2000; Casacci et al., 2013; Schönrogge et al., 2017) and may have evolved independently several times (Golden and Hill, 2016).
For ants, the simplest way for producing vibrations is the substrate tapping with part of the exoskeleton (i.e., “drumming”). However, specialized stridulatory organs (Figure 2F) occur in five subfamilies: Ponerinae, Pseudomyrmecinae, Myrmicinae, Ectomminae, and Nothomyrmecinae (Hunt and Richard, 2013; Golden and Hill, 2016; Schönrogge et al., 2017). The signal (stridulation) is produced by rubbing two body parts together, a scraper (plectrum) on a series of ridges (pars stridens) (Hunt and Richard, 2013; Golden and Hill, 2016).
A similar mechanism involving slightly different stridulatory organs (Figure 2E) produces calls in butterfly pupae (Alvarez et al., 2014; Dolle et al., 2018), while the modalities of larval sounds emissions are more diversified and not yet fully unraveled (Figure 2D) (except for some riodinids which possess specialized structures called vibratory papillae (DeVries, 1991b; Schönrogge et al., 2017).
In several butterfly species the emission of sounds can act as generic scaring or deterrent signals against enemies (Downey, 1966). In the case of lycaenids, in contrast, larval and pupal sounds may have taken up a role comparable to that of chemical signals in fostering interactions with ants (Downey, 1966; DeVries, 1990; Travassos and Pierce, 2000).
To assess the function of sounds in ant-butterfly symbioses it is sometimes possible to artificially prevent caterpillars from producing vibroacoustic signals and to check for changes in ant behavior. The first experimental evidence that vibroacoustic signals are used by butterfly larvae to attract ants was provided for the riodinid Thisbe irenea. Calling larvae were indeed tended by a higher number of workers than muted caterpillars (DeVries, 1991a). Ten years later, Travassos and Pierce (Travassos and Pierce, 2000) demonstrated that also larvae and pupae of the obligate mutualist Jalmenus evagoras use acoustic signals to enhance care from the Iridomyrmex ants to which they are associated. Larvae of J. evagoras are able to produce grunts, hisses, and drumming. While drumming occurs in both tended and untended larvae, hisses are emitted only during the first minutes after encountering a worker, and grunts are produced during all the ant attendance period (Travassos and Pierce, 2000). The positive correlation between the number of calls produced and the weight of the emitting pupa is considered to be an honest signal for ants to invest in a good quality and healthy resource. J. evagoras juveniles produce a complex repertoire of calls, which can be modulated to attract higher or lesser numbers of guarding workers and varied according to context, suggesting the evolution of a fine-tuned system of communication with their host ants.
As for butterfly parasitic interactions, clear-cut evidence of the role of vibroacoustic signals is only documented in Maculinea species where acoustical mimicry is employed along with other chemical and behavioral adaptations to deceive host ants and exploit nest resources (Barbero et al., 2009a,b, 2012; Thomas et al., 2010; Settele et al., 2011; Sala et al., 2014; Barbero and Casacci, 2015). The function of vibroacoustic communication in this model system was explored using another approach with respect to previous works (artificially muted individuals). After recordings, acoustic parameters were compared between parasite juveniles and their host ants to asses if any degree of mimicry occurs. Afterwards, sound stimuli were played back to ants to evaluate the behavioral responses of workers (Barbero et al., 2009b). In 2009, the first case of vibroacoustic mimicry in a social parasite of ants was discovered (Barbero et al., 2009b). Authors showed that larvae and pupae of M. rebeli are able to emit stridulations much more similar to those produced by Myrmica schencki queens, than to those of worker ants. Although occurring (see Chemical Signals), chemical mimicry does not explain how these butterfly parasites achieve a high social level in the colony hierarchy to such an extent that they are fed or rescued in preference to the ant brood (Thomas and Elmes, 1998). Instead, playback experiments revealed that the emission of queen-like calls allow the butterfly parasite to obtain a “royal” status, enhancing in workers the same degree of attendance and attention as queens do. In response to M. rebeli and queen vibroacoustic stimuli, ant workers tapped with antennae, gathered together around the speaker and showed guarding behaviors more frequently than when control or worker signals were played.
The ability to mimic queen sounds was assessed also in other predatory or cuckoo species i.e., M. arion populations hosted by Myrmica sabuleti (Barbero et al., 2009a) as well as in M. alcon and M. teleius hosted by Myrmica scabrinodis (Sala et al., 2014). Playback bioassays were performed to compare the function of vibroacoustic signals between the two lifestyles (cuckoo vs. predatory) in distinct stages of the biological cycle (Sala et al., 2014). For both the species, vibroacoustic signals change along with larval development and cause different amounts and variety of ant responses. In predatory species, the queen-like calls produced by early, just-dropped, larvae elicited the strongest ant response, thus contributing to enhance their retrieval by ants. Vibroacoustic stimuli probably serve to improve the supposed (but this requires formal testing) weak chemical mimicry shown by predatory species, which leads to very long adoption rituals (Fiedler, 1990; Sala et al., 2014). In contrast, cuckoo species primarily use vibroacoustic signals in the post-adoption phase to strengthen their social status and become fully integrated in the colony to outcompete ant brood for the same resources (Elmes et al., 1991; Sala et al., 2014).
Research on the vibroacoustic behavior in the Maculinea-Myrmica system has provided the first evidence that, at least in Myrmica ants, vibroacoustic cues are more than generic alarm signals and also convey information on the social rank of the emitting individual. The parasite mimics the intraspecific communication signals of its host, either to become intimately integrated within the colony, like in the cuckoo species, or just to enhance other channels of communication that might not be fully developed, like in the case of pre-adoption predatory larvae.
Although vibroacoustic signals can function as very precise interspecific messages (Maculinea and Jalmenus butterflies), the ability of producing calls is not limited to ant-associated lycaenid larvae (Alvarez et al., 2014; Schönrogge et al., 2017), since it is also present in species not associated with ants (myrmecoxenous) (Alvarez et al., 2014; Riva et al., 2017). Fiedler (1992) has suggested that the ability to produce vibroacoustic calls could be widespread in lycaenid species, but while myrmecoxenous species produce simple calls as a response to disturbance, myrmecophilous species emit more frequent and complex calls.
Analyzing several species of lycaenids, Riva et al. (2017) found that sounds emitted are strictly species-specific, but overall those produced by species associated with ants are made of longer and less distant pulses than calls of ant-independent species. Nevertheless, they did not point out differences in sound complexity. Multivariate comparisons of the vibroacoustic parameters of these calls were used to test the hypothesis that the similarity in lycaenid sounds is better predicted by the degree of ant association than by their phylogenetic distance (Schönrogge et al., 2017). In several instances, congeneric species made sounds that were much more similar to calls of distantly related species which showed the same type of ant association [myrmecophilous category as described by Fiedler (1991)]. Although the inclusion of further call analysis is required, authors suggested that the ability to produce vibroacoustic signals is a preadaptation to myrmecophily (Schönrogge et al., 2017).
In this framework, myrmecoxenous species could have maintained the ability to emit vibroacoustic stimuli either to repel natural enemies (Bura et al., 2009, 2011), or conspecifics sharing the same host plant at high densities, as observed in some butterflies and moths (Yack et al., 2001; Riva et al., 2017).
Future Directions
Eavesdropping
A complex communication system coordinates a large number of individuals in the collective decision-making process and maintains the social organization ensuring the ant ecological dominance (Wilson, 1985). Since by definition communication involves an emitter and a receiver, information in signals can be detected and exploited by a third “unplanned” individual other than the primary target (Peake, 2005). Yet, the intraspecific exchange of chemical or vibroacoustic signals of ants can be eavesdropped by commensal, mutualistic, or parasitic organisms, thereby, respectively, generating benefits and costs. Among myrmecophilous lycaenids, caterpillars of two parasitic species, Euliphyra mirifica and E. leucyana, intercept and follow trail pheromones released by arboreal weaver ant, Oecophylla longinoda, to locate their host ant nests (Dejean and Beugnon, 1996). Similarly, it is suggested that the first instar larvae of a close related species, Liphyra brassolis, detect the ant nests of their host, O. smaragdina, following pheromone trails of ants after hatching in the vicinity of the nests. L. brassolis larvae should also use the same trails to move between nests after depleting the brood of a nest or after host nest translocation (Common and Waterhouse, 1981).
As extensively reviewed above, ants may also be used as oviposition cues by adult females of myrmecophilous butterflies, but only indirect behavioral evidences are available. Further studies should investigate what signals, either chemical, vibroacoustic, or visual (Mota and Oliveira, 2016) are eavesdropped by females searching for the optimal LHP and the functioning of sensory structures possibly located on antennae and ovipositors which are used to perceive host plants colonized by ants.
Eavesdroppers can also intercept signals exchanged between ants and other organisms, but true instances of interspecific eavesdropping are rarely identified in multitrophic associations involving myrmecophilous butterflies.
Mathew et al. (2008) envisaged that the calls emitted by Feniseca tarquinius, which represents the first reported case of a calling Miletinae butterfly, might use vibroacoustic mimicry to avoid predation by ants. Authors argued that these larvae mimic the vibroacoustic signals produced by the ant-associated wooly aphids upon which they feed (Mathew et al., 2008). Beyond using these vibroacoustic signals to appease ants, would it be possible that caterpillars may use the putative vibroacoustic signals emitted by aphids to locate them? Unfortunately, no further studies were published, and data reported in this paper are not sufficient to support these speculations.
Although based on correlative data, field and laboratory experiments concur to suggest that orb-web spiders and braconid parasitoids, two natural enemies of the lycaenid Jalmenus evagoras, pinpoint their butterfly “preys” and increase their own success by exploiting the same ant volatiles that are supposedly used by the butterfly to detect the presence of their host ant, Iridomyrmex mayri (Elgar et al., 2016).
A well-documented case of interspecific eavesdropping is described in the second paragraph of this review. To locate the presence of the host ants and select the ideal oviposition site, Maculinea arion females follow signals emitted by their food plants responding and interacting with ants (Patricelli et al., 2015).
The paucity of robust evidence for interspecific eavesdropping is probably due to the lack of a community context approach in the study of multitrophic associations. Several studies focused on pairwise relations and do not consider other signals involved in levels not necessarily linked by trophic relations. For instance, it is expected that parasitoids of Maculinea butterflies exploit some form of interspecific eavesdropping to identify the presence of the host larvae inside the ant nest or within the flower buds. By releasing chemical compounds which elicit aggressive behavior and combats in workers, Ichneumon wasps create a safe route to brood chambers where they attack Maculinea cuckoo larvae (Thomas et al., 2002). They locate Myrmica colonies by ant-odor cues, but only enter those nests which host Maculinea larvae (Thomas and Elmes, 1993). How do they detect the butterfly larva inside the chamber in such a “chemical fuzziness?” Could it be possible that these parasitoids are able to perceive some components of Maculinea calls which is similar, but not entirely identical to the ant stridulations?
Maculinea predatory species, instead, are targeted by Neotypus parasitoids when they are still on their food plants (Tartally, 2005). Are these parasitoids able to perceive some plant response to egg-laying of Maculinea butterflies and use these signals to locate their own oviposition site?
Research considering the inclusive variation of costs and benefits in each combination of distinct trophic and information networks would be timely and would allow getting crucial insights on all the possible outcomes as well as on co-evolutionary dynamics in myrmecophilous systems.
Multimodal Communication
Another issue concerns the context, meaning, and function of multimodal signaling. It has been suggested that ants can use several signals (visual, tactile, and chemical) in peculiar intra-colony contexts to cause a prompt reaction in nestmates (Hölldobler and Wilson, 1990). Nonetheless, we possess little knowledge about the possibility that myrmecophilous organisms might manipulate ant behavior using multiple cues. Exploring the use of CHCs and vibrations in Maculinea species, authors collected increasing evidence that both types of signals are important and play distinct functions in different phases of the life cycle (Figure 3). Outside the colony, mimetic cuticular profiles, although simple, promote the adoption of Maculinea cuckoo larvae which usually occurs in few seconds after the first contact with the foraging ants; in predatory species, there are lines of evidence that this process could be fostered by vibroacoustic emissions (Sala et al., 2014). Since the adoption process can last for hours, predatory larvae may possess less mimetic cuticular profiles than cuckoo caterpillars and may have evolved more efficient vibroacoustic signals to compensate the chemical deficiency. Inside the nest, the CHC profile of parasites increase their resemblance with the host profile (Witek et al., 2013), but this achievement may not be sufficient to sneak undisturbed into the larval chamber to feed on the colony brood. The vibroacoustic signals, whose resemblance to the host stridulations arises inside the colony, could act together with chemical signals increasing the ant response and therefore the parasite acceptance.
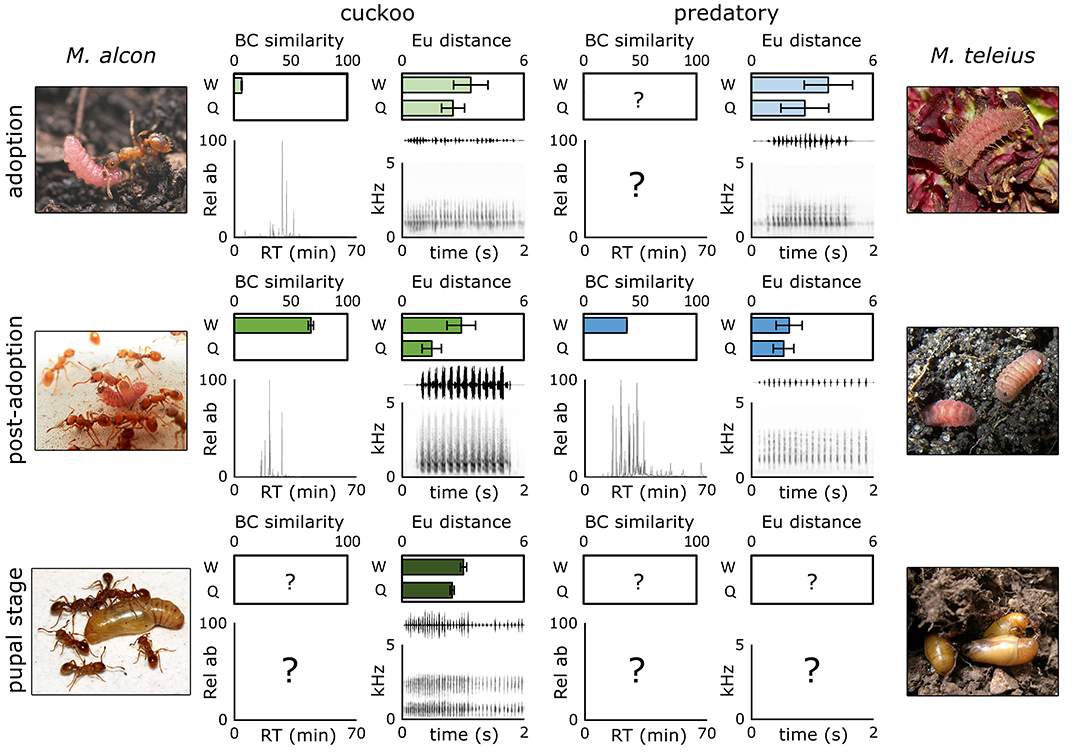
Figure 3. Comparison of chemicals and vibroacoustic patterns used by cuckoo and predatory caterpillars of Maculinea butterflies during pre- and post-adoption and the pupal phase to deceive their Myrmica host ants. Bray-Curtis (BC) similarities calculated on relative abundances (Rel ab) of cuticular hydrocarbons of parasitic caterpillars and host workers in pre-adoption (Schönrogge et al., 2004) and during the integration phase within host colonies (Witek et al., 2013) are reported as well as Euclidean (Eu) distances between the vibroacoustic patterns of the host queens (Q) and workers (W) and the parasite in pre- and post-adoption (Sala et al., 2014) and during the pupal stage (Barbero et al., 2009b). Pre-adoption chemical data and vibroacoustic patterns for pupal stage refer to M. rebeli and its host ant M. schencki. Chemical and vibroacoustic patterns showed for the post-adoption phase refer to M. alcon and M. teleius exploiting M. scabrinodis.
It is likely that the chemical and vibroacoustic signals have evolved independently and in the myrmecophilous parasite species most intimately linked to their host they have begun to operate in a multimodal way to allow the parasite to reach the highest level of integration within the colony. In other myrmecophilous organisms, commensal or mutualistic species, it is possible that the interaction with the host species is primarily reinforced by chemical signals and rewarding substances, while only slightly modulated by vibroacoustic cues as calling signals.
There are still many aspects to be completely discerned and it is clear that future research should try to better categorize signals collecting data on ant responses both to the multimodal composite signal and to each unimodal element. Testing the response to both chemical and vibroacoustic emissions separately would be necessary to determine whether the components produce the same or different outcomes (Partan and Marler, 2005). While the response of a colony to “isolated” vibroacoustic stimuli has been assessed in playback experiments, bioassays testing the role of CHC employing dummies are almost lacking. In addition, investigating ant behavioral responses to CHC-painted dummies supplemented with vibroacoustic signals could allow to gather robust insights on how distinct channels of communication, such as the chemical and the vibroacoustic one, may interact.
Concluding Remarks
The ant-association patterns are considered “a template” for the evolutionary radiation of lycaenid butterflies (Pierce et al., 2002). On the other hand, communication, which can be considered as an extended phenotype (sensu Dawkins, 1982) of the colony, may have played a pivotal role in the evolution of social insects. In our review, we tried to point out that the amazing complexity of signaling between myrmecophiles and their attendant ants could have boosted the evolutionary onsets of specialized life cycles thereby acting as a source of increasing diversity within butterflies.
Author Contributions
FB led the writing of the manuscript to which all authors contributed and gave final approval for publication.
Funding
LC was supported by the National Science Center, Poland, under grant Polonez UMO-2016/23/P/NZ8/04254. This project had received funding from the European Union's Horizon 2020 research and innovation program under the Marie Skłodowska Curie grant agreement No. 665778.
Conflict of Interest
The authors declare that the research was conducted in the absence of any commercial or financial relationships that could be construed as a potential conflict of interest.
Acknowledgments
Authors wish to thank Elisa Plazio for artworks.
References
Agrawal, A. A., and Fordyce, J. A. (2000). Induced indirect defence in a lycaenid-ant association, the regulation of a resource in a mutualism. Proc. R. Soc. Lond. B 267, 1857–1861. doi: 10.1098/rspb.2000.1221
Akino, T., Knapp, J. J., Thomas, J. A., and Elmes, G. W. (1999). Chemical mimicry and host specificity in the butterfly Maculinea rebeli, a social parasite of Myrmica ant colonies. Proc. R. Soc. Lond. B 266, 1419–1426. doi: 10.1098/rspb.1999.0796
Als, T. D., Nash, D. R., and Boomsma, J. J. (2001). Adoption of parasitic Maculinea alcon caterpillars (Lepidoptera: Lycaenidae). by three Myrmica ant species. Anim. Behav. 62, 99–106. doi: 10.1006/anbe.2001.1716
Alvarez, M., Munguira, M. L., and Martínez-Ibáñez, M. D. (2014). Comparative study of the morphology of stridulatory organs of the Iberian lycaenid butterfly pupae (Lepidoptera). J. Morph. 275, 414–430. doi: 10.1002/jmor.20224
Atsatt, P. R. (1981). Lycaenid butterflies and ants: selection for enemy-free space. Am. Nat. 118, 638–654. doi: 10.1086/283859
Axén, A. H., Leimar, O., and Hoffman, V. (1996). Signalling in a mutualistic interaction. Anim. Behav. 52, 321–333. doi: 10.1006/anbe.1996.0178
Axén, A. H., and Pierce, N. E. (1998). Aggregation as a cost-reducing strategy for lycaenid larvae. Behav. Ecol. 9, 109–115. doi: 10.1093/beheco/9.2.109
Bächtold, A., Alves-Silva, E., Kaminski, L. A., and Del-Claro, K. (2014). The role of tending ants in host plant selection and egg parasitism of two facultative myrmecophilous butterflies. Naturwissenschaften 101, 913–919. doi: 10.1007/s00114-014-1232-9
Barbero, F. (2016). Cuticular lipids as a cross-talk among ants, plants and butterflies. Int. J. Mol. Sci. 17:1966. doi: 10.3390/ijms17121966
Barbero, F., Bonelli, S., Thomas, J. A., Balletto, E., and Schönrogge, K. (2009a). Acoustical mimicry in a predatory social parasite of ants. J. Exp. Biol. 212, 4084–4090. doi: 10.1242/jeb.032912
Barbero, F., and Casacci, L. P. (2015). Butterflies that trick ants with sound. Phys. Today 68, 64–65. doi: 10.1063/PT.3.2757
Barbero, F., Patricelli, D., Witek, M., Balletto, E., Casacci, L. P., Sala, M., et al. (2012). Myrmica ants and their butterfly parasites with special focus on the acoustic communication. Psyche 2012:725237. doi: 10.1155/2012/725237
Barbero, F., Thomas, J. A., Bonelli, S., Balletto, E., and Schönrogge, K. (2009b). Queen ants make distinctive sounds that are mimicked by a butterfly social parasite. Science 323, 782–785. doi: 10.1126/science.1163583
Blüthgen, N. E., Stork, N., and Fiedler, K. (2004). Bottom-up control and co-occurrence in complex communities: honeydew and nectar determine a rainforest ant mosaic. Oikos 106, 344–358. doi: 10.1111/j.0030-1299.2004.12687.x
Bos, N., Lefevre, T., Jensen, A., and D'ettorre, P. (2012). Sick ants become unsociable. J. Evol. Biol. 25, 342–351. doi: 10.1111/j.1420-9101.2011.02425.x
Bura, V. L., Fleming, A. J., and Yack, J. E. (2009). What's the buzz? Ultrasonic and sonic warning signals in caterpillars of the great peacock moth (Saturnia pyri). Naturwissenschaften 96, 713–718. doi: 10.1007/s00114-009-0527-8
Bura, V. L., Rohwer, V. G., Martin, P. R., and Yack, J. E. (2011). Whistling in caterpillars (Amorpha juglandis, Bombycoidea): sound-producing mechanism and function. J. Exp. Biol. 214, 30–37. doi: 10.1242/jeb.046805
Carleial, S., Maurel, N., van Kleunen, M., and Stift, M. (2018). Oviposition by the mountain alcon blue butterfly increases with host plant flower number and host ant abundance. Basic Appl. Ecol. 28, 87–96. doi: 10.1016/j.baae.2018.02.008
Casacci, L. P., Schönrogge, K., Thomas, J. A., Balletto, E., Bonelli, S., and Barbero, F. (2019). Host specificity pattern and chemical deception in a social parasite of ants. Sci. Rep. 9:1619. doi: 10.1038/s41598-018-38172-4
Casacci, L. P., Thomas, J. A., Sala, M., Treanor, D., Bonelli, S., Balletto, E., et al. (2013). Ant pupae employ acoustics to communicate social status in their colony's hierarchy. Curr.Biol. 23, 323–327. doi: 10.1016/j.cub.2013.01.010
Chew, F. S., and Robbins, R. K. (1984). “Egg-laying in butterflies,” in The Biology of Butterflies, eds R. I. Vane-Wright, and P. R. Ackery (London: Academic, 65–79.
Claassens, A., and Dickson, C. (1977). A study of the myrmecophilous behaviour of the immature stages of Aloeides thryra (L.) (Lep.: Lycaenidae) with special reference to the function of the retractile tubercles and with additional notes of the general biology of the species. Entomol. Rec. J. Var. 89, 253–258.
Common, I. F. B., and Waterhouse, D. F. (1981). Butterflies of Australia. Revised Edition (Sydney: Angus & Robertson).
Cottrell, C. B. (1984). Aphytophagy in butterflies - its relationship to myrmecophily. Zool. J. Linn. Soc. 80, 1–57. doi: 10.1111/j.1096-3642.1984.tb02318.x
Cushman, J. H., Rashbrook, V. K., and Beattie, A. J. (1994). Assessing benefits to both participants in a lycaenid-ant association. Ecology 75, 1031–1041. doi: 10.2307/1939427
Czekes, Z., Marko, B., Nash, D. R., Ferencz, M., Lazar, B., and Rakosy, L. (2014). Differences in oviposition strategies between two ecotypes of the endangered myrmecophilous butterfly Maculinea alcon (Lepidoptera: Lycaenidae) under unique syntopic conditions. Insect Conserv. Divers. 7, 122–131. doi: 10.1111/icad.12041
Dani, F. R., Jones, G. R., Destri, S., Spencer, S. H., and Turillazzi, S. (2001). Deciphering the recognition signature within the cuticular chemical profile of paper wasps. Anim. Behav. 62, 165–171. doi: 10.1006/anbe.2001.1714
Daniels, H., Gottsberger, G., and Fiedler, K. (2005). Nutrient composition of larval nectar secretions from three species of myrmecophilous butterflies. J. Chem. Ecol. 31, 2805–2821. doi: 10.1007/s10886-005-8395-y
Davidson, D. W., Cook, S. C., Snelling, R. R., and Chua, T. H. (2003). Explaining the abundance of ants in lowland tropical rainforest canopies. Science 300, 969–972. doi: 10.1126/science.1082074
Dejean, A., and Beugnon, G. (1996). Host-ant trail following by myrmecophilous larvae of Liphyrinae (Lepidoptera, Lycaenidae). Oecologia 106, 57–62. doi: 10.1007/BF00334407
Dettner, K., and Liepert, C. (1994). Chemical mimicry and camouflage. Annu. Rev. Entomol. 39, 129–154. doi: 10.1146/annurev.en.39.010194.001021
DeVries, P.J. (1988). The larval ant-organs of Thisbe irenea (Lepidoptera: Riodinidae). and their effects upon attending ants. Zool. J. Linn. Soc. 94, 379–393. doi: 10.1111/j.1096-3642.1988.tb01201.x
DeVries, P. J. (1984). Of crazy-ants and curetinae: are curetis butterflies tended by ants? Zool. J. Linn. Soc. 80, 59–66. doi: 10.1111/j.1096-3642.1984.tb02319.x
DeVries, P. J. (1990). Enhancement of symbioses between butterfly caterpillars and ants by vibrational communication. Science 248, 1104–1106. doi: 10.1126/science.248.4959.1104
DeVries, P. J. (1991a). Mutualism between thisbe-irenea butterflies and ants, and the role of ant ecology in the evolution of larval-ant associations. Biol. J. Linn. Soc. Lond. 43, 179–195. doi: 10.1111/j.1095-8312.1991.tb00592.x
DeVries, P. J. (1991b). Call production by myrmecophilous riodinid and lycaenid butterfly caterpillars (Lepidoptera).: morphological, acoustical, functional, and evolutionary patterns. Am. Mus. Novit. 3025, 1–23.
DeVries, P. J. (1991c). “Evolutionary and ecological patterns in myrmecophilous-riodinid butterflies,” in Ant - Plant Interactions, eds C.R. Huxley and D.F. Cutler (Oxford: Oxford University Press, 143–156.
Dicke, M. (2000). Chemical ecology of host-plant selection by herbivorous arthropods: a multitrophic perspective. Biochem. System. Ecol. 28, 601–617. doi: 10.1016/S0305-1978(99)00106-4
Dolle, P., Klein, P., Fischer, O. W., Schnitzler, H.-U., Gilbert, L. E., and Boppré, M. (2018). Twittering pupae of papilionid and nymphalid butterflies (Lepidoptera): novel structures and sounds. Ann. Entomol. Soc. Amer. 111, 341–354. doi: 10.1093/aesa/say029
Elgar, M. A., Nash, D. R., and Pierce, N. E. (2016). Eavesdropping on cooperative communication within an ant-butterfly mutualism. Sci. Nat. 103:84. doi: 10.1007/s00114-016-1409-5
Elgar, M. A., and Pierce, N. E. (1988). “Mating success and fecundity in an ant-tended lycaenid butterfly” in Reproductive Success: Studies of Selection and Adaptation in Contrasting Breeding Systems ed T. H. Clutton-Brock (Chicago, IL: Chicago University Press, 59–75.
Elmes, G., Akino, T., Thomas, J., Clarke, R., and Knapp, J. (2002). Interspecific differences in cuticular hydrocarbon profiles of Myrmica ants are sufficiently consistent to explain host specificity by Maculinea (large blue) butterflies. Oecologia 130, 525–535. doi: 10.1007/s00442-001-0857-5
Elmes, G., Wardlaw, J., and Thomas, J. (1991). Larvae of Maculinea rebeli, a large-blue butterfly and their Myrmica host ants: patterns of caterpillar growth and survival. J. Zool. 224, 79–92. doi: 10.1111/j.1469-7998.1991.tb04789.x
Elmes, G. W., Wardlaw, J. C., Schönrogge, K., Thomas, J. A., and Clarke, R. T. (2004). Food stress causes differential survival of socially parasitic caterpillars of Maculinea rebeli integrated in colonies of host and non-host Myrmica ant species. Entomol. Exp. Appl. 110, 53–63. doi: 10.1111/j.0013-8703.2004.00121.x
Fiedler, K. (1990). New information on the biology of Maculinea nausithous and M. teleius (Lepidoptera: Lycaenidae). Nota Lepid. 12, 246–256.
Fiedler, K. (1991). Systematic, evolutionary, and ecological implications of myrmecophily within the Lycaenidae (Insecta: Lepidoptera: Papilionoidea). Bonn. Zool. Monogr. 31, 1–210.
Fiedler, K. (1992). Recent contributions to the behavioural ecology and evolution of lycaenid-ant associations (Lepidoptera, Lycaenidae). Nota Lepid. 4, 11–13.
Fiedler, K. (1994). Lycaenid butterflies and plants: is myrmecophily associated with amplified hostplant diversity? Ecol. Entomol. 19, 79–82. doi: 10.1111/j.1365-2311.1994.tb00393.x
Fiedler, K. (1998). Geographical patterns in life-history traits of Lycaenidae butterflies: ecological and evolutionary implications. Zoology 100, 336–347.
Fiedler, K., and Maschwitz, U. (1988). Functional analysis of the myrmecophilous relationships between ants (Hymenoptera: Formicidae). and lycaenids (Lepidoptera: Lycaenidae). Oecologia 75, 204–206. doi: 10.1007/BF00378598
Fiedler, K., and Maschwitz, U. (1989a). Adult myrmecophily in butterflies: the role of the ant Anoplolepis longipes in the feeding and oviposition behaviour of Allotinus unicolor (Lepidoptera, Lycaenidae) Lepid. Sci. 40, 241–251.
Fiedler, K., and Maschwitz, U. (1989b). The symbiosis between the weaver ant, Oecophylla smaragdina, and Anthene emolus, an obligate myrmecophilous lycaenid butterfly. J. Nat. Hist. 23, 833–846. doi: 10.1080/00222938900770441
Fiedler, K., and Maschwitz, U. (1989c). Functional analysis of the myrmecophilous relationships between ants (Hymenoptera: Formicidae). and Lycaenids (Lepidoptera: Lycaenidae). I. Release of food recruitment in ants by lycaenid larvae and pupae. Ethology 80, 71–80. doi: 10.1111/j.1439-0310.1989.tb00730.x
Fraser, A., Tregenza, T., Wedell, N., Elgar, M., and Pierce, N. (2002). Oviposition tests of ant preference in a myrmecophilous butterfly. J. Evol. Biol. 15, 861–870. doi: 10.1046/j.1420-9101.2002.00434.x
Frings, H., and Frings, M. (1958). Uses of sounds by insects. Annu. Rev. Entomol. 3, 87–106. doi: 10.1146/annurev.en.03.010158.000511
Fürst, M. A., and Nash, D. R. (2009). Host ant independent oviposition in the parasitic butterfly Maculinea alcon. Biol. Lett. 6, 174–176. doi: 10.1098/rsbl.2009.0730
Gibbs, A. G. (1998). Water-proofing properties of cuticular lipids. Amer. Zool. 38, 471–482. doi: 10.1093/icb/38.3.471
Gnatzy, W., Jatho, M., Kleinteich, T., Gorb, S., and Hustert, R. (2017). The eversible tentacle organs of Polyommatus caterpillars (Lepidoptera, Lycaenidae): morphology, fine structure, sensory supply and functional aspects. Arthropod. Struct. Develop. 46, 788–804. doi: 10.1016/j.asd.2017.10.003
Golden, T., and Hill, P. (2016). The evolution of stridulatory communication in ants, revisited. Ins. Soc. 63, 309–319. doi: 10.1007/s00040-016-0470-6
Guerrieri, F. J., Nehring, V., Jørgensen, C. G., Nielsen, J., Galizia, C. G., and d'Ettorre, P. (2009). Ants recognize foes and not friends. Proc. R. Soc. Lond. B 276, 2461–2468. doi: 10.1098/rspb.2008.1860
Harvey, D. (1987). The higher classification of the Riodinidae (PhD dissertation). University of Texas, Austin, TX, United States.
Henning, S. F. (1983). Chemical communication between Iycaenid larvae (Lepidoptera: Lycaenidae) and ants (Hymenoptera: Formicidae). J Entomol. Soc. South. Afr. 46, 341–366.
Hickling, R., and Brown, R. L. (2000). Analysis of acoustic communication by ants. J Acoust. Soc. Am. 108, 1920–1929. doi: 10.1121/1.1290515
Hinton, H. E. (1951). Myrmecophilous Lycaenidae and other Lepidoptera - a summary. Proc. Trans. South Lond. Entomol. Nat. Hist. Soc. 1949–50, 111–175.
Hojo, M. K., Pierce, N. E., and Tsuji, K. (2015). Lycaenid caterpillar secretions manipulate attendant ant behavior. Curr. Biol. 25, 2260–2264. doi: 10.1016/j.cub.2015.07.016
Hojo, M. K., Wada-Katsumata, A., Akino, T., Yamaguchi, S., Ozaki, M., and Yamaoka, R. (2009). Chemical disguise as particular caste of host ants in the ant inquiline parasite Niphanda fusca (Lepidoptera: Lycaenidae). Proc. R. Soc. Lond. B 276, 551–558. doi: 10.1098/rspb.2008.1064
Hojo, M. K., Yamamoto, A., Akino, T., Tsuji, K., and Yamaoka, R. (2014). Ants use partner specific odors to learn to recognize a mutualistic partner. PLoS ONE 9:e86054. doi: 10.1371/journal.pone.0086054
Hölldobler, B., and Wilson, E. O. (1990). The Ants. Berlin; Heidelberg: Springer Verlag. doi: 10.1007/978-3-662-10306-7
Hunt, J., and Richard, F.-J. (2013). Intracolony vibroacoustic communication in social insects. Ins. Soc. 60, 403–417. doi: 10.1007/s00040-013-0311-9
Jordano, D., Rodriguez, J., Thomas, C., and Haeger, J. F. (1992). The distribution and density of a lycaenid butterfly in relation to Lasius ants. Oecologia 91, 439–446. doi: 10.1007/BF00317635
Kaminski, L. A., Mota, L. L., Freitas, A. V. L., and Moreira, G. R. P. (2013). Two ways to be a myrmecophilous butterfly: natural history and comparative immature-stage morphology of two species of Theope (Lepidoptera: Riodinidae). Biol. J. Linn. Soc. Lond. 108, 844–870. doi: 10.1111/bij.12014
Kitching, R. (1987). Aspects of the natural history of the lycaenid butterfly Allotinus major in Sulawesi. J. Nat. Hist. 21, 535–544. doi: 10.1080/00222938700770311
Kitching, R., and Luke, B. (1985). The myrmecophilous organs of the larvae of some British Lycaenidae (Lepidoptera): a comparative study. J. Nat. Hist. 19, 259–276. doi: 10.1080/00222938500770211
Leimar, O., and Axén, A. H. (1993). Strategic behaviour in an interspecific mutualism: interactions between lycaenid larvae and ants. Anim. Behav. 46, 1177–1182. doi: 10.1006/anbe.1993.1307
Lenoir, A., D'Ettorre, P., Errard, C., and Hefetz, A. (2001). Chemical ecology and social parasitism in ants. Annu. Rev. Entomol. 46, 573–599. doi: 10.1146/annurev.ento.46.1.573
Malicky, H. (1969). Versuch einer Analyse der ökologischen Beziehungen zwischen Lycaeniden (Lepidoptera). und Formiciden (Hymenoptera). Tijdschrift voor Entomologie 112, 213–298.
Mannino, G., Abdi, G., Maffei, M. E., and Barbero, F. (2018). Origanum vulgare terpenoids modulate Myrmica scabrinodis brain biogenic amines and ant behaviour. PLoS ONE 13:e0209047. doi: 10.1371/journal.pone.0209047
Maschwitz, U., Schroth, M., Hanel, H., and Tho, Y. (1985). Aspects of the larval biology of myrmecophilous lycaenids from West Malaysia (Lepidoptera). Nachr. ent. Ver. Apollo. Frankfurt. 6, 181–200.
Maschwitz, U., Wüst, M., and Schurian, K. (1975). Bläulingsraupen als Zuckerlieferanten für Ameisen. Oecologia 18, 17–21. doi: 10.1007/BF00350631
Mathew, J., Travassos, M., Canfield, M., Murawski, D., Kitching, R., and Pierce, N. (2008). The singing reaper: diet, morphology and vibrational signaling in the nearctic species Feniseca tarquinius (Lepidoptera: Lycaenidae, Miletinae). Trop. Lepid. Res. 18, 24–29.
Mizuno, T., Hagiwara, Y., and Akino, T. (2018). Chemical tactic of facultative myrmecophilous lycaenid pupa to suppress ant aggression. Chemoecology 28, 173–182. doi: 10.1007/s00049-018-0270-8
Moreau, C. S., Bell, C. D., Vila, R., Archibald, S. B., and Pierce, N. E. (2006). Phylogeny of the ants: diversification in the age of angiosperms. Science 312, 101–104. doi: 10.1126/science.1124891
Mota, L. L., and Oliveira, P. S. (2016). Myrmecophilous butterflies utilise ant–treehopper associations as visual cues for oviposition. Ecol. Entomol. 41, 338–343. doi: 10.1111/een.12302
Murray, D. P. (1935). South African Butterflies: A Monograph of the Family Lycaenidae. London: Staples Press.
Musche, M., Anton, C., Worgan, A., and Settele, J. (2006). No experimental evidence for host ant related oviposition in a parasitic butterfly. J. Ins. Behav. 19, 631–643. doi: 10.1007/s10905-006-9053-0
Nash, D. R., Als, T. D., Maile, R., Jones, G. R., and Boomsma, J. J. (2008). A mosaic of chemical coevolution in a large blue butterfly. Science 319, 88–90. doi: 10.1126/science.1149180
Nelson, D., and Blomquist, G. (1995). “Insect waxes,” in Waxes: Chemistry, Molecular Biology and Functions, ed. R. J. Hamilton (Dundee: The Oily Press, 1–90.
New, T. R. (2017). Mutualisms and Insect Conservation. Berlin: Springer. doi: 10.1007/978-3-319-58292-4
Newcomer, E. J. (1912). Some observations on the relations of ants and lycaenid caterpillars, and a description of the relational organs of the latter. J. N. Y. Entomol. Soc. 20, 31–36.
Nomura, K. (1992). Problems in the symbiosis between the lycaenid butterfly larva, Niphanda fusca and Camponotus japonicus (1). J. Lepid. Soc. Jpn. 43, 138–143.
Omura, H., Watanabe, M., and Honda, K. (2009). Cuticular hydrocarbons of larva and pupa of Reverdin's blue, Lycaeides argyrognomon (Lycaenidae). and its tending ants. Lepid. Sci. 60, 203–210.
Partan, S. R., and Marler, P. (2005). Issues in the classification of multimodal communication signals. Am. Nat. 166, 231–245. doi: 10.1086/431246
Patricelli, D., Barbero, F., La Morgia, V., Casacci, L. P., Witek, M., Balletto, E., et al. (2011). To lay or not to lay: oviposition of Maculinea arion in relation to Myrmica ant presence and host plant phenology. Anim. Behav. 82, 791–799. doi: 10.1016/j.anbehav.2011.07.011
Patricelli, D., Barbero, F., Occhipinti, A., Bertea, C. M., Bonelli, S., Casacci, L. P., et al. (2015). Plant defences against ants provide a pathway to social parasitism in butterflies. Proc. R. Soc. Lond. B 282:20151111. doi: 10.1098/rspb.2015.1111
Patricelli, D., Witek, M., Barbero, F., Casacci, L. P., Bonelli, S., and Balletto, E. (2010). Evidence of high larval host ant (Hymenoptera: Formicidae). specificity in the first post-adoption phase for the myrmecophilous butterfly Phengaris (Maculinea). nausithous (Lepidoptera: Lycaenidae). Sociobiology 55, 861–870.
Peake, T. M. (2005). “Eavesdropping in communication networks,” in Animal Communication Networks, ed. P. K. McGregor (Cambridge: Cambridge University Press), 13–37. doi: 10.1017/CBO9780511610363.004
Pech, P., Fric, Z., Konvička, M., and Zrzavý, J. (2004). Phylogeny of Maculinea blues (Lepidoptera: Lycaenidae). based on morphological and ecological characters: evolution of parasitic myrmecophily. Cladistics 20, 362–375. doi: 10.1111/j.1096-0031.2004.00031.x
Pellissier, L., Litsios, G., Guisan, A., and Alvarez, N. (2012). Molecular substitution rate increases in myrmecophilous lycaenid butterflies (Lepidoptera). Zool. Scripta. 41, 651–658. doi: 10.1111/j.1463-6409.2012.00556.x
Pierce, N., Kitching, R., Buckley, R., Taylor, M., and Benbow, K. (1987). The costs and benefits of cooperation between the Australian lycaenid butterfly, Jalmenus evagoras, and its attendant ants. Behav. Ecol. Sociobiol. 21, 237–248. doi: 10.1007/BF00292505
Pierce, N. E. (1984). “Amplified species diversity: a case study of an Australian lycaenid butterfly and its attendant ants,” in The Biology of Butterflies, eds R. I. Vane-Wright and P. R. Ackery (London: Academic, 197–200.
Pierce, N. E., Braby, M. F., Heath, A., Lohman, D. J., Mathew, J., Rand, D. B., et al. (2002). The ecology and evolution of ant association in the Lycaenidae (Lepidoptera). Annu. Rev. Entomol. 47, 733–771. doi: 10.1146/annurev.ento.47.091201.145257
Pierce, N. E., and Elgar, M. A. (1985). The influence of ants on host plant selection by Jalmenus evagoras, a myrmecophilous lycaenid butterfly. Behav. Ecol. Sociobiol. 16, 209–222. doi: 10.1007/BF00310983
Pierce, N. E., and Nash, D. R. (1999). The imperial blue, Jalmenus evagoras (Lycaenidae). Monogr. Austr. Lepid. 6, 279–315.
Quilico, A., Piozzi, F., and Pavan, M. (1957). The structure of dendrolasin. Tetrahedron 1, 177–185. doi: 10.1016/0040-4020(57)88037-5
Renwick, J., and Chew, F. (1994). Oviposition behavior in Lepidoptera. Annu. Rev. Entomol. 39, 377–400. doi: 10.1146/annurev.en.39.010194.002113
Rico-Gray, V., and Oliveira, P. S. (2007). The Ecology and Evolution of Ant-Plant Interactions. Chicago, IL: University of Chicago Press. doi: 10.7208/chicago/9780226713540.001.0001
Riva, F., Barbero, F., Bonelli, S., Balletto, E., and Casacci, L. P. (2017). The acoustic repertoire of lycaenid butterfly larvae. Bioacoustics 26, 77–90. doi: 10.1080/09524622.2016.1197151
Rodrigues, D., Kaminski, L. A., Freitas, A. V., and Oliveira, P. S. (2010). Trade-offs underlying polyphagy in a facultative ant-tended florivorous butterfly: the role of host plant quality and enemy-free space. Oecologia 163, 719–728. doi: 10.1007/s00442-010-1626-0
Sala, M., Casacci, L. P., Balletto, E., Bonelli, S., and Barbero, F. (2014). Variation in butterfly larval acoustics as a strategy to infiltrate and exploit host ant colony resources. PLoS ONE 9:e94341. doi: 10.1371/journal.pone.0094341
Samson, P. (1989). Morphology and biology of Acrodipsas illidgei (waterhouse and lyell), a myrmecophagous lycaenid (lepidoptera: lycaenidae: theclinae). Aust. J. Entomol. 28, 161–168. doi: 10.1111/j.1440-6055.1989.tb00874.x
Sanetra, M., and Fiedler, K. (1996). Behaviour and morphology of an aphytophagous lycaenid caterpillar: Cigaritis (Apharitis) acamas Klug, 1834 (Lepidoptera: Lycaenidae). Nota Lepid. 18, 57–76.
Schär, S., Eastwood, R., Arnaldi, K. G., Talavera, G., Kaliszewska, Z. A., Boyle, J. H., et al. (2018). Ecological specialization is associated with genetic structure in the ant-associated butterfly family Lycaenidae. Proc. R. Soc. Lond. B 285:20181158. doi: 10.1098/rspb.2018.1158
Schönrogge, K., Barbero, F., Casacci, L. P., Settele, J., and Thomas, J. A. (2017). Acoustic communication within ant societies and its mimicry by mutualistic and socially parasitic myrmecophiles. Anim. Behav. 134, 249–256. doi: 10.1016/j.anbehav.2016.10.031
Schönrogge, K., Wardlaw, J., Peters, A., Everett, S., Thomas, J., and Elmes, G. (2004). Changes in chemical signature and host specificity from larval retrieval to full social integration in the myrmecophilous butterfly Maculinea rebeli. J Chem. Ecol. 30, 91–107. doi: 10.1023/B:JOEC.0000013184.18176.a9
Sendoya, S. F., Freitas, A. V., and Oliveira, P. S. (2009). Egg-laying butterflies distinguish predaceous ants by sight. Am. Nat. 174, 134–140. doi: 10.1086/599302
Settele, J., Barbero, F., Musche, M., Thomas, J. A., and Schönrogge, K. (2011). Singing the blues: from experimental biology to conservation application. J. Exp. Biol. 214, 1407–1410. doi: 10.1242/jeb.035329
Singer, T. L. (1998). Roles of hydrocarbons in the recognition systems of insects. Am. Zool. 38, 394–405. doi: 10.1093/icb/38.2.394
Solazzo, G., Seidelmann, K., Moritz, R. F. A., and Settele, J. (2014). Tetracosane on the cuticle of the parasitic butterfly Phengaris (Maculinea) nausithous triggers the first contact in the adoption process by Myrmica rubra foragers. Physiol. Entomol. 60, 57–64. doi: 10.1007/s00040-012-0265-3
Stadler, B., Kindlmann, P., Šmilauer, P., and Fiedler, K. (2003). A comparative analysis of morphological and ecological characters of European aphids and lycaenids in relation to ant attendance. Oecologia 135, 422–430. doi: 10.1007/s00442-003-1193-8
Tartally, A. (2005). Neotypus melanocephalus (Hymenoptera: Ichneumonidae): the first record of a parasitoid wasp attacking Maculinea teleius (Lycaenidae). Nota Lepid. 28, 65–57.
Tartally, A., Thomas, J. A., Anton, C., Balletto, E., Barbero, F., Bonelli, S., et al. (2019). Patterns of host use by brood parasitic Maculinea butterflies across Europe. Philos. Trans. R. Soc. B 374:20180202. doi: 10.1098/rstb.2018.0202
Thomas, J. (1984). The behaviour and habitat requirements of Maculinea nausithous (the dusky large blue butterfly) and M. teleius (the scarce large blue) in France. Biol. Conserv. 28, 325–347. doi: 10.1016/0006-3207(84)90040-5
Thomas, J., and Elmes, G. (1993). Specialized searching and the hostile use of allomones by a parasitoid whose host, the butterfly Maculinea rebeli, inhabits ant nests. Anim. Behav. 45, 593–602. doi: 10.1006/anbe.1993.1069
Thomas, J., Elmes, G., and Wardlaw, J. (1998). Polymorphic growth in larvae of the butterfly Maculinea rebeli, a social parasite of Myrmica ant colonies. Proc. R. Soc. Lond. B 265, 1895–1901. doi: 10.1098/rspb.1998.0517
Thomas, J., Knapp, J., Akino, T., Gerty, S., Wakamura, S., Simcox, D., et al. (2002). Insect communication: parasitoid secretions provoke ant warfare. Nature 417:505. doi: 10.1038/417505a
Thomas, J. A., and Elmes, G. W. (1998). Higher productivity at the cost of increased host-specificity when Maculinea butterfly larvae exploit ant colonies through trophallaxis rather than by predation. Ecol. Entomol. 23, 457–464. doi: 10.1046/j.1365-2311.1998.00153.x
Thomas, J. A., and Elmes, G. W. (2001). Food-plant niche selection rather than the presence of ant nests explains oviposition patterns in the myrmecophilous butterfly genus Maculinea. Proc. R. Soc. Lond. B 268, 471–477. doi: 10.1098/rspb.2000.1398
Thomas, J. A., Elmes, G. W., Sielezniew, M., Stankiewicz-Fiedurek, A., Simcox, D. J., Settele, J, et al. (2013). Mimetic host shifts in an endangered social parasite of ants. Proc. R. Soc. Lond. B 280:20122336. doi: 10.1098/rspb.2012.2336
Thomas, J. A., Elmes, G. W., Wardlaw, J. C., and Woyciechowski, M. (1989). Host specificity among Maculinea butterflies in Myrmica ant nests. Oecologia 79, 452–457. doi: 10.1007/BF00378660
Thomas, J. A., Schönrogge, K., Bonelli, S., Barbero, F., and Balletto, E. (2010). Corruption of ant acoustical signals by mimetic social parasites: Maculinea butterflies achieve elevated status in host societies by mimicking the acoustics of queen ants. Commun. Integr. Biol. 3, 169–171. doi: 10.4161/cib.3.2.10603
Thomas, J. A., Schönrogge, K., and Elmes, G. W. (2005). “Specializations and Host Associations of Social Parasites of Ants,” in Insect Evolutionary Ecology, eds M. D. E. Fellowes, G. J Holloway, and J. Rolff (London: Royal Entomological Society), 475–514. doi: 10.1079/9780851998121.0479
Travassos, M. A., and Pierce, N. E. (2000). Acoustics, context and function of vibrational signalling in a lycaenid butterfly–ant mutualism. Anim. Behav. 60, 13–26. doi: 10.1006/anbe.1999.1364
Van Dyck, H., Oostermeijer, J. G. B., Talloen, W., Feenstra, V., van der Hidde, A., and Wynhoff, I. (2000). Does the presence of ant nests matter for oviposition to a specialized myrmecophilous Maculinea butterfly? Proc. R. Soc. Lond. B 267, 861–866. doi: 10.1098/rspb.2000.1082
Van Dyck, H., and Regniers, S. (2010). Egg spreading in the ant-parasitic butterfly, Maculinea alcon: from individual behaviour to egg distribution pattern. Anim. Behav. 80, 621–627. doi: 10.1016/j.anbehav.2010.06.021
van Zweden, J. S., and d'Ettorre, P. (2010). “Nestmate recognition in social insects and the role of hydrocarbons,” in Insect Hydrocarbons: Biology, Biochemistry, and Chemical Ecology, eds G. J. Blomquist and A. G. Bagneres (New York, NY: Cambridge University Press), 222–243. doi: 10.1017/CBO9780511711909.012
Wada, A., Isobe, Y., Yamaguchi, S., Yamaoka, R., and Ozaki, M. (2001). Taste-enhancing effects of glycine on the sweetness of glucose: a gustatory aspect of symbiosis between the ant, Camponotus japonicus, and the larvae of the lycaenid butterfly, Niphanda fusca. Chem. Senses. 26, 983–992. doi: 10.1093/chemse/26.8.983
Wagner, D., and Kurina, L. (1997). The influence of ants and water availability on oviposition behaviour and survivorship of a facultatively ant-tended herbivore. Ecol. Entomol. 22, 352–360. doi: 10.1046/j.1365-2311.1997.00077.x
Wardlaw, J., Thomas, J., and Elmes, G. (2000). Do Maculinea rebeli caterpillars provide vestigial mutualistic benefits to ants when living as social parasites inside Myrmica ant nests? Entomol. Exp. Appl. 95, 97–103. doi: 10.1046/j.1570-7458.2000.00646.x
Wilson, E. O. (1985). The sociogenesis of insect colonies. Science 228, 1489–1495. doi: 10.1126/science.228.4707.1489
Wilson, E. O., and Hölldobler, B. (2005). The rise of the ants: a phylogenetic and ecological explanation. Proc. Nat. Acad. Sci. U.S.A. 102, 7411–7414. doi: 10.1073/pnas.0502264102
Witek, M., Barbero, F., and Markó, B. (2014). Myrmica ants host highly diverse parasitic communities: from social parasites to microbes. Ins. Soc. 61, 307–323. doi: 10.1007/s00040-014-0362-6
Witek, M., Casacci, L. P., Barbero, F., Patricelli, D., Sala, M., Bossi, S., et al. (2013). Interspecific relationships in co-occurring populations of social parasites and their host ants. Biol. J. Linn. Soc. 109, 699–709. doi: 10.1111/bij.12074
Witek, M., Sliwinska, E. B., Skorka, P., Nowicki, P., Settele, J., and Woyciechowski, M. (2006). Polymorphic growth in larvae of Maculinea butterflies, as an example of biennialism in myrmecophilous insects. Oecologia 148, 729–733. doi: 10.1007/s00442-006-0404-5
Wynhoff, I., Bakker, R. B., Oteman, B., Arnaldo, P. S., and van Langevelde, F. (2015). Phengaris (Maculinea) alcon butterflies deposit their eggs on tall plants with many large buds in the vicinity of Myrmica ants. Insect Conserv. Divers. 8, 177–188. doi: 10.1111/icad.12100
Wynhoff, I., Grutters, M., and van Langevelde, F. (2008). Looking for the ants: selection of oviposition sites by two myrmecophilous butterfly species. Anim. Biol. 58, 371–388. doi: 10.1163/157075608X383683
Yack, J., Smith, M., and Weatherhead, P. (2001). Caterpillar talk: acoustically mediated territoriality in larval Lepidoptera. Proc. Nat. Acad. Sci. U.S.A. 98, 11371–11375. doi: 10.1073/pnas.191378898
Yamagushi, S., and Shirozu, T. (1988). The Life Histories of Five Myrmecophilous Lycaenid Butterflies of Japan. Tokyo: Kodansha.
Keywords: ants, chemical cues, eavesdropping, manipulation, mimicry, oviposition, vibroacoustic signals
Citation: Casacci LP, Bonelli S, Balletto E and Barbero F (2019) Multimodal Signaling in Myrmecophilous Butterflies. Front. Ecol. Evol. 7:454. doi: 10.3389/fevo.2019.00454
Received: 16 April 2019; Accepted: 11 November 2019;
Published: 29 November 2019.
Edited by:
Astrid T. Groot, University of Amsterdam, NetherlandsReviewed by:
Emily Burdfield-Steel, University of Jyväskylä, FinlandJosef Settele, Helmholtz Centre for Environmental Research (UFZ), Germany
Copyright © 2019 Casacci, Bonelli, Balletto and Barbero. This is an open-access article distributed under the terms of the Creative Commons Attribution License (CC BY). The use, distribution or reproduction in other forums is permitted, provided the original author(s) and the copyright owner(s) are credited and that the original publication in this journal is cited, in accordance with accepted academic practice. No use, distribution or reproduction is permitted which does not comply with these terms.
*Correspondence: Francesca Barbero, francesca.barbero@unito.it