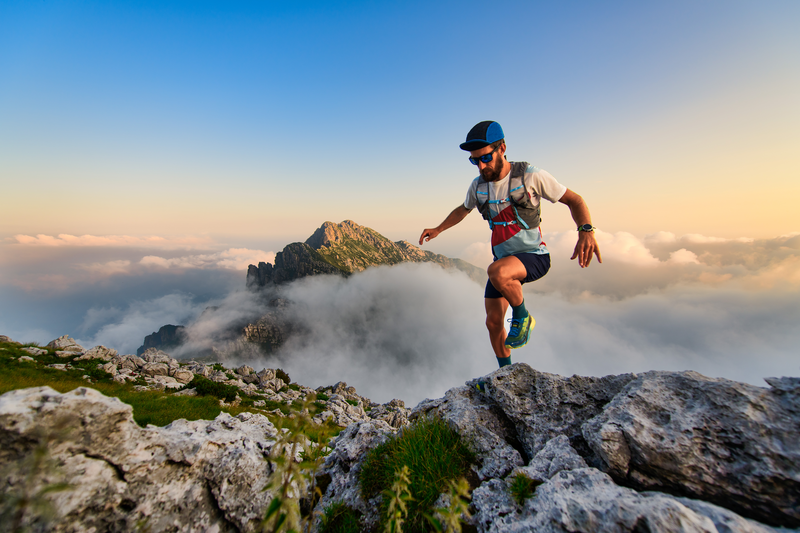
94% of researchers rate our articles as excellent or good
Learn more about the work of our research integrity team to safeguard the quality of each article we publish.
Find out more
ORIGINAL RESEARCH article
Front. Ecol. Evol. , 26 November 2019
Sec. Population, Community, and Ecosystem Dynamics
Volume 7 - 2019 | https://doi.org/10.3389/fevo.2019.00453
This article is part of the Research Topic The Genomics of Biological Invasion View all 8 articles
Clarifying the population structure and introduction history of non-native species is essential to preventing and mitigating effects of invasion, yet the invasion history of many long-established non-native species remain poorly understood. This study characterized the population structure of one of the most commonly encountered urban ants in North America using public participant-collected specimens and double-digest restriction-site associated DNA sequencing (ddRADseq). Based on this genomic approach we confirm the identity of populations ranging across the continent as Tetramorium immigrans Santschi, and report low genetic diversity across its range, suggesting that this entire population resulted from the establishment of one single, or a few closely related ant colonies, approximately 200 years ago. The absence of strong population structure suggests that this ant's spread across North America has been repeatedly aided by human-assisted dispersal. We find no evidence of inbreeding in T. immigrans; this contrasts with the inbreeding and unicoloniality of many other invasive ants. These results demonstrate how collections-based public participatory science and emerging high-throughput sequencing techniques each have the potential to vastly improve the amount of data available to researchers. Together, these techniques can be deployed to address ecological and evolutionary questions, including questions about invasion biology, in non-model organisms. We suggest that this model is particularly well-suited for research on common taxa that are ecologically important but poorly understood.
Studying the population genetics of non-model organisms, including non-native, urban and familiar “backyard” species, has historically been challenging because of the need for broad scale sampling and the limited availability of appropriate genetic markers. More recently, however, technological advances of the past decade—in both communications and genomics (Klaper and Thomas, 2004)—have made it feasible to carry out population-level research with nearly any taxon, including invasive species. The introduction of non-native species as an unintended result of global trade increasingly threatens the environment and public health, as invasive species can reduce biodiversity, displace native fauna, serve as vectors for pathogens, and disrupt ecosystem services (Hulme, 2009; Ascunce et al., 2011; Bertelsmeier et al., 2017). Preventing establishment of invasive species and mitigating impacts of established invasives requires understanding species' origins and introduction routes, yet origins and introduction histories of many non-native species remain unknown (Ascunce et al., 2011).
Population genomic approaches, in particular, restriction site-associated DNA sequencing (RADseq) (Miller et al., 2007; Baird et al., 2008; Peterson et al., 2012), offer a cost-effective solution for surveying genome-wide single nucleotide polymorphism (SNPs) in species without a sequenced genome. These techniques have proven useful to understanding species' invasion histories (Dupuis et al., 2018; Leydet et al., 2018; Bors et al., 2019; Cohen and Privman, 2019) and as a non-destructive method for identification and monitoring (reviewed in Taylor and Gemmell, 2016). More generally, emergence of RAD-based techniques have led to wide adoption of these methods in ecological and evolutionary studies of insects (Bagley et al., 2017; Storer et al., 2017; Lower et al., 2018; Zhang et al., 2018; De Jesús-Bonilla et al., 2019), including ants (Moreau and Wray, 2017; Boyle et al., 2018; Avril et al., 2019; Smith et al., 2019), which rank among the most damaging and difficult to control invasive species (Rabitsch, 2011).
If there is an exotic species characteristic of urban habitats in the USA, it is certainly the pavement ant Tetramorium immigrans Santschi, whose specific epithet “immigrans” speaks to its history of long-distance dispersal and whose common name, “pavement ant,” invokes the very essence of urban living: the paved environment. Like many other ants, this species forms large, subterranean colonies of many hundreds to thousands of workers (Figure 1). The generalist feeding habits of pavement ants make them exceptionally adaptable to new environments: they consume proteins, fats, and carbohydrates from plant and animal sources. Where food waste is common in cities, they are often the first animals to arrive at and break down discarded foodstuffs (Penick et al., 2015).
Figure 1. Pavement ants, Tetramorium immigrans, are often encountered in urban and suburban areas throughout temperate North America. Here, workers from two colonies battle over territorial boundaries on the margins of a reservoir (Photo: Alex Wild).
Even in their native range throughout Anatolia and the Caucasus, T. immigrans colonies commonly occupy human-modified habitats, nesting in pavement, rock walls, railways, and buildings as well as under stones along roadsides and in parks and gardens (Wagner et al., 2017). They favor warm, moist environments, especially during periods of high activity, although they easily tolerate cold and dry conditions during the winter phase of inactivity (Cordonnier et al., 2019). Pavement ants were likely first shipped unintentionally to the eastern USA in the early nineteenth century in soil ballast or in cargo (Steiner et al., 2008). The earliest confirmed records of this species in North America are from specimens collected in 1927 in the US North Atlantic states of Maryland, New Jersey, New York, Pennsylvania, and Washington D.C., and unconfirmed records exist from as early as 1748 (Wheeler, 1927; Brown, 1957). As of 2019, T. immigrans has been recorded in 39 states in the continental USA and three Canadian provinces (Guenard et al., 2017) (www.antmaps.org).
European pavement ants are not just widespread in North America, they are ubiquitous; multiple studies have found them to be the dominant ant species in urban environments (Pećarević et al., 2010; Buczkowski and Richmond, 2012; Lucky et al., 2014). Despite their prevalence in urban temperate North America, the identity and species limits of T. immigrans have long been uncertain (Wagner et al., 2017, 2018). Taxonomically, T. immigrans, along with several other morphologically similar species, belongs to the Tetramorium caespitum complex. A recent integrative taxonomic study of species boundaries in the complex which incorporated morphology, genes, and cuticular hydrocarbons (Wagner et al., 2017) paved the way for population-level study of T. immigrans and its close congeners. This research highlighted the possibility that populations of pavement ants across North America thought to be T. immigrans may represent multiple species. While one other species in the complex, the morphologically distinguishable Japanese Tetramorium tsushimae Emery, is present in a few midwestern and eastern states (Steiner et al., 2006), the possibility of other, cryptic European pavement ant species in North America remained.
Historically, restricted access to private property made biodiversity sampling in urban and suburban habitats challenging for researchers. However, public participants can collect in their own neighborhoods with ease, making a public participatory approach to sampling an easy solution (Dickinson et al., 2012; Ricciardi et al., 2017). Research partnerships with public participants offer one way to meet the extensive sampling needs of studies that assess population structure and draw inferences about widespread and common species (Dickinson et al., 2010). Common species, by definition, are relatively easy to find, even for non-specialists; nevertheless, they are often woefully under-represented in museum collections. Whereas, observational data have been used effectively for mapping phenotypic, phenological, and geographic traits, the collection and preservation of physical specimens are necessary for genetic research (Pimm et al., 2015; McKinley et al., 2017). To date, a limited number of studies have combined these two approaches (Lyons et al., 2012; Kerkvliet et al., 2017; Ryan et al., 2019), and the value of public participatory research for characterizing introduction histories of non-native, urban species remains to be widely demonstrated empirically.
The goals of this study were to combine citizen science and population genomics to characterize identity and population genetic structure of the pavement ant, T. immigrans, in its US exotic range, and to reconstruct introduction history. We first used citizen-collected samples as the basis to (1) determine species identity. We used DNA sequence data from the mitochondrial gene COI to clarify whether US populations of Tetramorium belong exclusively to the species T. immigrans or to additional, morphologically similar species within the T. caespitum complex (excluding T. tsushimae). We then (2) quantified genetic diversity among populations in the North American range using genome-wide SNPs generated with ddRADseq. We anticipated low genetic diversity of this population would reflect an introduction scenario involving establishment of one or few closely related colonies, limiting the genetic diversity to only a fraction of that present in the native range. By contrast, high genetic diversity would indicate that North American populations descended from multiple lineages of T. immigrans and support a wave-like introduction scenario involving multiple colonies becoming established, possibly over an extended time frame, bringing with them breadth of diversity from across the native range. Finally, we used genomic data to (3) consider alternate dispersal patterns within the North American range. If expansion from the original introduction site occurred via local dispersal through mating flights—without the assistance of human transportation—we expected genetic diversity to increase with distance. Signatures of this history would be detectable as in the genetic differentiation between major continental regions, particularly between east- and west-coast populations. Conversely, lack of regional genetic differentiation would indicate that human-assisted dispersal helped the pavement ant become established across the continent.
Nearly all of the ant specimens used in this study were collected by participants—most of them school children—in the School of Ants citizen science project (SOA, www.schoolofants.org), between 2011 and 2013 (Lucky et al., 2014). SOA was designed to facilitate public engagement in scientific research; one goal was to use participant-collected samples of widespread ants for population genetic studies. Other goals included increasing interest and trust in science through public participation in scientific research (Vitone et al., 2016), facilitating monitoring of ant distributions in human-modified environments (Lucky et al., 2014), and encouraging the study of urban biodiversity.
All specimens were collected following the standardized SOA protocol (Figure 2, Supplementary Material 1), in which participants used cookie crumbs to attract ants, then collected ants in zip-top bags, froze them overnight, and mailed them to the SOA team. Upon receipt, ant specimens were identified to genus and species (wherever possible) and vouchers were stored at −18°C in 95% EtOH.
Figure 2. (Top) Pavement ant specimens were collected by public participants, most of them children, through the public science project, the School of Ants (Photo: Jonas Stenstrom). (Bottom) Map of US Tetramorium immigrans specimen sampling localities; colors correspond to geographic regions.
From hundreds of samples containing Tetramorium workers, 90 samples from 26 states were included in genetic analyses, representing the broadest possible US geographic range (Figure 2, Supplementary Material 2). To minimize unintentional sampling of ants from the same colony, specimens were collected from localities at least 1 km from any other sample, far beyond the estimated territory size of 42 m2 in a single colony of T. immigrans (Brian and Elmes, 1974). DNA was extracted destructively using the Qiagen DNeasy blood and tissue kit (QIAGEN, Valencia, CA), according to standard protocol with the only change being the final elution volume of 75 μL DNA for each sample. A 658 bp fragment of the mitochondrial COI gene, commonly used as a “barcoding” gene, was amplified using primers LepF1 (5′-ATT CAA CCA ATC ATA AAG ATA TTG G-3′) and LepR1 (5′-TAA ACT TCT GGA TGT CCA AAA AAT CA-3′) using standard PCR conditions (Folmer et al., 1994).
To contextualize North American T. immigrans among native range samples, we also took advantage of additional, although not fully overlapping (348 bp), COI sequences of European T. immigrans from previous study (Schlick-Steiner et al., 2006). We built a TCS haplotype network (Clement et al., 2000) using the combined European and our own COI data in the program POPART v1.7 (Leigh and Bryant, 2015).
Double digest Restriction-site Associated DNA sequencing (ddRADseq) library preparation followed (Peterson et al., 2012) using the 78 samples that successfully generated COI sequences. In addition, to compare inter- vs. intra-colonial levels of genetic diversity and test for inbreeding, we included 16 workers from a single colony in North Carolina. In brief, the rare EcoRI and common MseI restriction sites were used for restriction enzyme digestion. Illumina sequencing adaptors and custom barcodes between 8 and 14 bases in length, which varied by at least four bases (Parchman et al., 2012), were ligated onto the sticky ends and PCR-amplified using primers for Illumina sequencing adaptors. Amplified digestion-ligation products were pooled into equal volumes for sequencing. Gel-based size selection for 200–500 base pair fragments and purification of the sequencing library using Agencourt AMPure XP beads (Beckman Coulter) were performed at the UF ICBR core-sequencing laboratory (http://www.biotech.ufl.edu), and sequenced using Illumina NextSeq500 high-output flow cell for a shared 150-cycle sequencing run.
Illumina adaptor sequences were automatically trimmed from all reads by Illumina's BaseSpace software. All subsequent bioinformatics steps were conducted using Stacks v. 2.3e (Catchen et al., 2013). Raw Illumina reads were demultiplexed using process_radtags, quality controlled (-c -q -r) and trimmed to 100 base pairs (-t). The Stacks program denovo_map.pl was used for de novo assembly, locus discovery, and genotyping. A minimum of three mismatches was allowed between stacks during loci assembly (-M 3), and an allowance of two mismatches was permitted between stacks (-n 2). Finally, the Stacks populations program was used to identify loci violating Hardy-Weinberg equilibrium (-hwe), which were subsequently removed via the blacklist option (-B). The remaining loci were then filtered to include only loci present in all four populations (-p 4), and in 90% of the individuals (-r 0.9), for downstream analyses.
Basic population and summary statistics were calculated using Stacks populations sumstats.summary output, the R packages adegenet v2.1.1 (Jombart and Ahmed, 2011) and hierfstat v0.04-22 (Goudet, 2005). To infer population structure the Bayesian-based clustering analysis, STRUCTURE v2.3.4, was used (Pritchard et al., 2000). We tested the support for 1 through 5 genetic clusters (K = 1–5) and ran 10 replicates per K with 100,000 generations burn-in followed by 500,000 MCMC repetitions, using default settings. To infer the most likely number of unique genetic clusters we used the ΔK method (Evanno et al., 2005), implemented in STRUCTURE Harvester (Earl, 2012). The STRUCTURE plots were visualized using pophelper v2.2.9 (Francis, 2017). We also analyzed the SNPs data using the discriminant analysis of principle components (DAPC), which has fewer marked assumptions than STRUCTURE, using adegenet. The most probable number of clusters for DAPC was determined using sequential k-means clustering using the find.clusters function and the associated Bayesian Information Criterion (BIC). DAPC plots were made for individuals under the most probable cluster membership and labeled by U.S. geographic origins (Northeast, Mid-Atlantic, Midwest, and Western). Hierarchical analysis of molecular variance (AMOVA) was conducted to detect differences among populations using the R package poppr (Kamvar et al., 2014).
Out of the 90 specimens that were tested, 14 failed to amplify; this was presumed to be due to DNA degradation during shipping. We were able to verify that all specimens morphologically identified as T. immigrans in this study were indeed this species using DNA barcode authentication of samples. No other cryptic species in this complex were found among these samples and very little genetic difference was found within the US. Comparison of US and European populations using a short fragment of COI recovered all US haplotypes as identical to the most commonly collected haplotype from Europe (Figure 3).
Figure 3. TCS haplotype network of Tetramorium immigrans from the European and North American populations generated using a 348 bp COI fragment, with the following geographic designations: Anatolia/Caucasus (Armenia, Russia, Turkey), Eastern Europe (Bulgaria, Croatia, Czechia, Hungary, Romania), Southern Europe (Greece, Italy, Spain), Western Europe (Austria, France), and USA (Photo: Alex Wild).
The success rate of sequencing citizen-collected samples was high (98% success) with ddRADseq, on average, there were 970,000 ± 36,000 SE reads per individual. After stringent filtering 2,255 unlinked SNPs were retained for downstream analyses. The North American population of T. immigrans has little population structure: across the entire sampled range overall FST was low (FST = 0.0150, Table 1A). Within the one North Carolina colony for which 16 nestmates were sampled, we found near-identical genotypes (FST = 0.001, Table 1A). The inbreeding coefficient (FIS) for the North Carolina colony was −0.4706, while the continent-wide FIS was 0.0376 (Table 1A). Pairwise FST values between the four geographic regions were also low, ranging from 0.0056 to 0.0297 (Table 1B). The majority (94.82%) of genetic variation was found among individuals, rather than between regions as indicated by AMOVA (Table 2).
Table 1. (A) Summary statistics of the Tetramorium immigrans SNPs data (B) pairwise FST based on the four geographical regions (WE, Western; MA, Mid-Atlantic; MW, Midwest; NE, Northeast).
Table 2. Analysis of molecular variance (AMOVA) of the Tetramorium immigrans SNPs data using clustering between the four geographical regions.
The optimal number of populations supported by STRUCTURE analysis was K = 2 (Figure 4), with Midwest and Mid-Atlantic samples grouped together while Western and Northeast populations had greater similarity to each other. For K = 3, additional structure was detected with two Northeastern individuals from Vermont having shared ancestry assigned primarily to this third cluster (Figure 4). The optimal number of clusters detected using k-means clustering was also two, corresponding to the lowest BIC value (Figure 4). DAPC was subsequently carried out using the optimal two clusters and retained three discriminant eigenvalues, which separated Midwest population from the other three regions (Figure 5).
Figure 4. (Top) Molecular assignments of K = 2 of Tetramorium immigrans based on analysis of SNPs using STRUCTURE. (Middle) Alternative molecular assignment of K = 3. (Bottom) Delta K value showing the most likely number of clusters indicated by STRUCTURE HARVESTER.
Figure 5. (Top) Discriminant Analysis of Principle Components (DAPC) of Tetramorium immigrans SNPs data, grouped by geographic region. (Bottom) Optimal number of clusters based on k-means using find.clusters in adegenet.
The most common, widespread pavement ant in North America is unequivocally T. immigrans, as evidenced by COI and ddRADseq data sampled from colonies sampled across North America. The near absence of genetic diversity (~0.0%) revealed in our study is consistent with previous, small-scale studies that found 0.0–1.2% COI sequence divergence for T. immigrans in its native range (Schär et al., 2018). We found no evidence of other T. caespitum complex species beyond the known presence of T. tsushimae (Steiner et al., 2006), which was readily distinguishable from T. immigrans based on morphology and geographic distribution.
Despite having been established in North America since at least the 1800s, little genetic divergence exists among populations of T. immigrans within the non-native range. Whether this is a result of selection or reflects the limited diversity present in the population upon establishment is unclear. We recovered only a few genetic variants from across a vast geographic range, and thousands of sites across the genome. COI sequences, for example, were nearly identical across samples; among three haplotypes identified, each differed from the others by only a single SNP. When compared with other European samples, the USA specimens clustered with the most common haplotype found throughout different regions of Europe (Figure 3).
Genomic data also indicate that pavement ants comprise a single, largely undifferentiated population across North America, with close examination revealing differences in structure that varied depending upon the analytical method used. In both STRUCTURE and DAPC, SNP data provided support for the existence of two weakly differentiated T. immigrans clusters (Figures 4, 5). In the K = 2 scenario, most samples were assigned to one cluster, Cluster 1, that was commonly encountered in all four geographic regions, with Cluster 2 represented only in a small subset of samples from the Northeast and West. Additional, minor, genetic sub-clusters were identified when K = 3. By contrast, DAPC analyses identified the composition of Midwestern samples as distinct from the other three geographic regions (Figure 5). This overall lack of strong genetic structure was also confirmed by AMOVA, as genetic variation among individuals far surpassed variation between assigned geographical regions (Table 2). Surprisingly, this low diversity appears not to result from inbreeding, as indicated by a low inbreeding coefficient (FIS) among the 16 nestmates from a single North Carolina colony (Table 1A). While the patterns of nestmate variability could be underestimated by only examining a single colony, when combined with the lack of strong population structure across the US, they suggest that the entire North American population is likely descended from either a single introduction or multiple introductions from a single, genetically homogeneous lineage.
Our data do not allow us to distinguish between a single-colony and a multiple-colonies-from-a-single-lineage introduction scenario, but the presence of a rare social parasite, Tetramorium atratulum (Schenck) in the non-native range may provide an informative clue. This workerless ant is an obligate parasite of T. immigrans and is found at low densities in both the native and non-native range of its host (Dash and Sanchez, 2009). Tetramorium atratulum is fed and cared for exclusively by T. immigrans workers, which do not recognize this interloper as a threat. It seems unlikely that a viable population of this parasite could have become established in North America as a result of a single-colony host introduction. Instead, its presence suggests that a pulse of introduction and establishment of closely related pavement ant colonies may have been more likely. Future studies on the population genetics of T. atratulum could prove to be especially illuminating as to the introduction history of both this parasite and host species.
Despite the fact that the establishment of the European pavement ant in North America precedes that of other invasive ants by a century, the low genetic diversity in this species is nonetheless similar to that of other invasive ant species (Tsutsui and Suarez, 2003; Ascunce et al., 2011; Eyer et al., 2018). The combination of outbreeding and the genetic bottleneck experienced during founding resulted in a widespread population today that largely lacks genetic structure. The continent-wide range expansion from the initial site of establishment was likely facilitated by human-assisted dispersal, as we found no evidence of geographic isolation across the non-native range.
The genetic uniformity of T. immigrans across North America is surprising, considering its reproductive biology, which differs from that of other widespread and ecologically dominant non-native ant species. Low genetic diversity in this outbreeding species appears to be a result of the severely limited genetic diversity of the initial population upon establishment rather than inbreeding or clonal reproduction. Invasive ant success is often associated with unicoloniality, where colonies reach massive size and density as a result of multi-queen (polygynous) and multi-nest (polydomous) colonies that display no intercolonial aggression (Tsutsui and Suarez, 2003). Unicolonial populations often possess extremely low genetic diversity as a result of introduction bottlenecks and inbreeding (Tsutsui et al., 2003; Ascunce et al., 2011; Eyer et al., 2018) and in some cases clonal reproduction depresses diversity even further (Foucaud et al., 2009; Pearcy et al., 2011).
Tetramorium immigrans is widespread in paved habitats throughout most of temperate North America and maintains large and often competitively dominant colonies; however, each colony possesses only a single, outbreeding queen and has distinct territorial boundaries that workers aggressively defend against non-nestmates (Bruder and Gupta, 1972). By contrast, T. tsushimae, the other US introduced pavement ant in the T. caespitum complex, is polygynous. This difference in reproductive biology likely contributes to the competitive dominance of T. tsushimae where both species co-occur (Reuther, 2009; Trager, pers. comm.); whether this more recently introduced pavement ant will replace T. immigrans entirely remains to be seen.
Little is known about where, specifically, T. immigrans first became established in the US and precisely when this occurred. Brown (1957) suggests an introduction in or before the early 1800s. Given this timeline, it is surprising that stronger population structure does not exist in the non-native range. The yellow crazy ant, Anoplolepis gracilipes Smith, became structured and differentiated in its Australian exotic range within a matter of decades (Gruber et al., 2013). Reproductive biology may offer some insight into these differences. A. gracilipes colony foundation is dependent, with new colonies “budding” by fission from a parent colony; resulting in restricted gene flow and outbreeding distance. In contrast, a single mated T. immigrans queen independently establishes a new nest without attendant workers, a process known as independent colony foundation (Bruder and Gupta, 1972). Outbreeding and the mating flights of T. immigrans sets them apart from many other well-studied invasive ants and may explain why minimal population structure exists within the US.
The results of this study show a higher genetic diversity among colonies than within a single colony (Table 1A), confirming that, at least in the region of this single-colony sample (North Carolina), T. immigrans does not form supercolonies in the exotic range. If not unicoloniality, what, then, can account for the success of T. immigrans? It seems likely that the combination of habitat suitability and the almost complete lack of competition from other North American ant species in the human-built environment (that is, open, gravelly, and well-drained sites), has aided in the expansion of T. immigrans (Cordonnier et al., 2019). The development of roads and railways, as well as increasing urbanization, have effectively rolled out the red carpet for this species. The generalist feeding habit of T. immigrans, combined with a preference for habitats sharing the qualities of paved environments in temperate climates, created a suitable and largely competitor-free niche for T. immigrans after its introduction into early 1800s North America. Urban T. immigrans have been found to have carbon-13 levels similar to those in human foods, to a greater extent than co-occurring ant species, suggesting that T. immigrans is exquisitely capable of taking advantage of human-produced (and discarded) food resources (Penick et al., 2015).
Although T. immigrans is most often associated with urban areas and paved surfaces, populations of this species have also become established in undisturbed, unpaved habitats in the western US, in California, Nevada, Utah, and Idaho, where the incursion of this species into intact ecosystems is associated with reduction of local ant species richness (Ward, pers. comm.). As the pavement ant is not strictly limited to the built environment, research on this species' ability to compete with native ants would be of interest. Further studies comparing the intraspecific genetic variations and behavior of this species in both its native and non-native ranges would shed more light on the mechanisms leading to the success of pavement ants, and indeed other synanthropic species, in the Anthropocene.
In this study we have shown that samples of ants collected by public participants can be incorporated into population genomic studies to answer otherwise intractable questions. Our results confirm that the commonly encountered pavement ant in North America is largely limited to a single species, T. immigrans, which ranges across the USA and southern Canada. Weak population structure among geographically distant populations suggests that this species' origins can be attributed to the establishment of one or a few genetically similar colonies that successfully proliferated and dispersed over two centuries. By synergistically combining citizen-collected specimens and molecular evolutionary studies, we offer an example of a feasible way to take citizen science into the genomic era. Partnerships between these two disciplines can both engage the public in the tools and methods of modern evolutionary biology and, simultaneously, permit evolutionary biologists to address questions on a scale that would be difficult to impossible without the assistance of public participants. Relatively inexpensive genomics and informatics tools have the potential to powerfully enhance the research and educational impacts associated with the rich diversity of questions, subjects, and modes of participation that citizen science projects address (Pocock et al., 2017; Ryan et al., 2019). We hope the results presented here encourage collaboration among scientists and educators as this proven framework for rapid and cost-effective population genomics can be used to characterize other non-model species.
All COI sequences are deposited on Genbank accession numbers KT339872—KT339947, demultiplexed ddRADseq data are stored at Short Read Archive (SRA) under BioProject ID PRJNA539993.
AL, TV, and RD conceived and designed the study and collected the data. TV, YZ, AP, and CS analyzed the data. JH and SM contributed materials and analysis tools. AL, TV, and YZ wrote the paper with contributions from all authors.
This project was partially funded by U.S. National Science Foundation grant DEB-1541005 awarded to SM, while the citizen science component was funded by DRL-1319293 awarded to RD. CS was supported by the UF/IFAS SFRC.
The authors declare that the research was conducted in the absence of any commercial or financial relationships that could be construed as a potential conflict of interest.
We thank the many, many School of Ants participants, including individuals, teachers, programs, and institutions that contributed to this project and without whom this study would not have been possible. A very special thanks to the many SOA team members at North Carolina State University and the University of Florida for their dedication to specimen processing and identification as well as public education and outreach. We gratefully acknowledge A. Wild, B. Boudinot, J. Trager, L. Nichols, R. Johnson, L. Davis, and P. S. Ward for providing ant identifications and insight into the biology of Tetramorium. M. Gaynor helpfully consulted on population genetic analyses. We also acknowledge the University of Florida Research Computing for providing computational resources and support that have contributed to the research results reported in this publication. We are grateful for insightful comments from two reviewers that significant improved this manuscript.
The Supplementary Material for this article can be found online at: https://www.frontiersin.org/articles/10.3389/fevo.2019.00453/full#supplementary-material
Ascunce, M. S., Yang, C.-C., Oakey, J., Calcaterra, L., Wu, W.-J., Shih, C.-J., et al. (2011). Global invasion history of the fire ant Solenopsis invicta. Science 331, 1066–1068. doi: 10.1126/science.1198734
Avril, A., Purcell, J., Brelsford, A., and Chapuisat, M. (2019). Asymmetric assortative mating and queen polyandry are linked to a supergene controlling ant social organization. Mol. Ecol. 28, 1428–1438. doi: 10.1111/mec.14793
Bagley, R. K., Sousa, V. C., Niemiller, M. L., and Linnen, C. R. (2017). History, geography and host use shape genomewide patterns of genetic variation in the redheaded pine sawfly (Neodiprion lecontei). Mol. Ecol. 26, 1022–1044. doi: 10.1111/mec.13972
Baird, N. A., Etter, P. D., Atwood, T. S., Currey, M. C., Shiver, A. L., Lewis, Z. A., et al. (2008). Rapid SNP discovery and genetic mapping using sequenced RAD markers. PLoS ONE 3:e3376. doi: 10.1371/journal.pone.0003376
Bertelsmeier, C., Ollier, S., Liebhold, A., and Keller, L. (2017). Recent human history governs global ant invasion dynamics. Nat. Ecol. Evol. 1:0184. doi: 10.1038/s41559-017-0184
Bors, E. K., Herrera, S., Morris, J. A., and Shank, T. M. (2019). Population genomics of rapidly invading lionfish in the Caribbean reveals signals of range expansion in the absence of spatial population structure. Ecol. Evol. 9, 3306–3320. doi: 10.1002/ece3.4952
Boyle, J. H., Martins, D. J., Pelaez, J., Musili, P. M., Kibet, S., Ndung'u, S. K., et al. (2018). Polygyny does not explain the superior competitive ability of dominant ant associates in the African ant-plant, Acacia (Vachellia) drepanolobium. Ecol. Evol. 8, 1441–1450. doi: 10.1002/ece3.3752
Brian, M., and Elmes, G. (1974). Production by the ant Tetramorium caespitum in a Southern English heath. J. Anim. Ecol. 43, 889–903. doi: 10.2307/3542
Bruder, K., and Gupta, A. (1972). Biology of the pavement ant, Tetramorium caespitum (Hymenoptera: Formicidae). Ann. Entomol. Soc. Am. 65, 358–367. doi: 10.1093/aesa/65.2.358
Buczkowski, G., and Richmond, D. S. (2012). The effect of urbanization on ant abundance and diversity: a temporal examination of factors affecting biodiversity. PLoS ONE 7:e41729. doi: 10.1371/journal.pone.0041729
Catchen, J., Hohenlohe, P. A., Bassham, S., Amores, A., and Cresko, W. A. (2013). Stacks: an analysis tool set for population genomics. Mol. Ecol. 22, 3124–3140. doi: 10.1111/mec.12354
Clement, M., Posada, D., and Crandall, K. A. (2000). TCS: a computer program to estimate gene genealogies. Mol. Ecol. 9, 1657–1659. doi: 10.1046/j.1365-294x.2000.01020.x
Cohen, P., and Privman, E. (2019). Speciation and hybridization in invasive fire ants. BMC Evol. Biol. 19:111. doi: 10.1186/s12862-019-1437-9
Cordonnier, M., Bellec, A., Dumet, A., Escarguel, G., Kaufmann, B., Hassall, C., et al. (2019). Range limits in sympatric cryptic species: a case study in Tetramorium pavement ants (Hymenoptera: Formicidae) across a biogeographical boundary. Insect Conserv. Divers. 12, 109–120. doi: 10.1111/icad.12316
Dash, S. T., and Sanchez, L. (2009). New distribution record for the social parasitic ant Anergates atratulus (Schenck, 1852)(Hymenoptera: Formicidae): an IUCN red-listed species. West. N. Am. Nat. 69, 140–142. doi: 10.3398/064.069.0109
De Jesús-Bonilla, V. S., Meza-Lázaro, R. N., and Zaldívar-Riverón, A. (2019). 3RAD-based systematics of the transitional Nearctic-Neotropical lubber grasshopper genus Taeniopoda (Orthoptera: Romaleidae). Mol. Phylogenet. Evol. 137, 64–75. doi: 10.1016/j.ympev.2019.04.019
Dickinson, J. L., Shirk, J., Bonter, D., Bonney, R., Crain, R. L., Martin, J., et al. (2012). The current state of citizen science as a tool for ecological research and public engagement. Front. Ecol. Environ. 10, 291–297. doi: 10.1890/110236
Dickinson, J. L., Zuckerberg, B., and Bonter, D. N. (2010). Citizen science as an ecological research tool: challenges and benefits. Annu. Rev. Ecol. Evol. Syst. 41, 149–172. doi: 10.1146/annurev-ecolsys-102209-144636
Dupuis, J. R., Sim, S. B., San Jose, M., Leblanc, L., Hoassain, M. A., Rubinoff, D., et al. (2018). Population genomics and comparisons of selective signatures in two invasions of melon fly, Bactrocera cucurbitae (Diptera: Tephritidae). Biol. Invasions 20, 1211–1228. doi: 10.1007/s10530-017-1621-z
Earl, D. A. (2012). STRUCTURE HARVESTER: a website and program for visualizing STRUCTURE output and implementing the Evanno method. Conserv. Genet. Resour. 4, 359–361. doi: 10.1007/s12686-011-9548-7
Evanno, G., Regnaut, S., and Goudet, J. (2005). Detecting the number of clusters of individuals using the software STRUCTURE: a simulation study. Mol. Ecol. 14, 2611–2620. doi: 10.1111/j.1365-294X.2005.02553.x
Eyer, P. A., McDowell, B., Johnson, L. N. L., Calcaterra, L. A., Fernandez, M. B., Shoemaker, D., et al. (2018). Supercolonial structure of invasive populations of the tawny crazy ant Nylanderia fulva in the US. BMC Evol. Biol. 18:209. doi: 10.1186/s12862-018-1336-5
Folmer, O., Black, M., Hoeh, W., Lutz, R., and Vrijenhoek, R. (1994). DNA primers for amplification of mitochondrial cytochrome c oxidase subunit I from diverse metazoan invertebrates. Mol. Mar. Biol. Biotechnol. 3, 294–299.
Foucaud, J., Orivel, J., Fournier, D., Delabie, J. H., Loiseau, A., Le Breton, J., et al. (2009). Reproductive system, social organization, human disturbance and ecological dominance in native populations of the little fire ant, Wasmannia auropunctata. Mol. Ecol. 18, 5059–5073. doi: 10.1111/j.1365-294X.2009.04440.x
Francis, R. M. (2017). pophelper: an R package and web app to analyse and visualize population structure. Mol. Ecol. Resour. 17, 27–32. doi: 10.1111/1755-0998.12509
Goudet, J. (2005). Hierfstat, a package for R to compute and test hierarchical F-statistics. Mol. Ecol. Notes 5, 184–186. doi: 10.1111/j.1471-8286.2004.00828.x
Gruber, M., Hoffmann, B., Ritchie, P., and Lester, P. (2013). The conundrum of the yellow crazy ant (Anoplolepis gracilipes) reproductive mode: no evidence for dependent lineage genetic caste determination. Insectes Soc. 60, 135–145. doi: 10.1007/s00040-012-0277-z
Guenard, B., Weiser, M. D., Gomez, K., Narula, N., and Economo, E. P. (2017). The Global Ant Biodiversity Informatics (GABI) database: synthesizing data on the geographic distribution of ant species (Hymenoptera: Formicidae). Myrmecol. News 24, 83–89. doi: 10.25849/myrmecol.news_024:083
Hulme, P. E. (2009). Trade, transport and trouble: managing invasive species pathways in an era of globalization. J. Appl. Ecol. 46, 10–18. doi: 10.1111/j.1365-2664.2008.01600.x
Jombart, T., and Ahmed, I. (2011). adegenet 1.3-1: new tools for the analysis of genome-wide SNP data. Bioinformatics 27, 3070–3071. doi: 10.1093/bioinformatics/btr521
Kamvar, Z. N., Tabima, J. F., and Grünwald, N. J. (2014). Poppr: an R package for genetic analysis of populations with clonal, partially clonal, and/or sexual reproduction. PeerJ 2:e281. doi: 10.7717/peerj.281
Kerkvliet, J., de Boer, T., Schilthuizen, M., and Kraaijeveld, K. (2017). Candidate genes for shell colour polymorphism in Cepaea nemoralis. PeerJ 5:e3715. doi: 10.7717/peerj.3715
Klaper, R., and Thomas, M. A. (2004). At the crossroads of genomics and ecology: the promise of a canary on a chip. Bioscience 54, 403–412. doi: 10.1641/0006-3568(2004)054[0403:ATCOGA]2.0.CO;2
Leigh, J. W., and Bryant, D. (2015). popart: full-feature software for haplotype network construction. Methods Ecol. Evol. 6, 1110–1116. doi: 10.1111/2041-210X.12410
Leydet, K. P., Grupstra, C. G., Coma, R., Ribes, M., and Hellberg, M. E. (2018). Host-targeted RAD-Seq reveals genetic changes in the coral Oculina patagonica associated with range expansion along the Spanish Mediterranean coast. Mol. Ecol. 27, 2529–2543. doi: 10.1111/mec.14702
Lower, S. E., Stanger-Hall, K. F., and Hall, D. W. (2018). Molecular variation across populations of a widespread North American firefly, Photinus pyralis, reveals that coding changes do not underlie flash color variation or associated visual sensitivity. BMC Evol. Biol. 18:129. doi: 10.1186/s12862-018-1251-9
Lucky, A., Savage, A. M., Nichols, L. M., Castracani, C., Shell, L., Grasso, D. A., et al. (2014). Ecologists, educators, and writers collaborate with the public to assess backyard diversity in the school of ants project. Ecosphere 5, 1–23. doi: 10.1890/ES13-00364.1
Lyons, J. I., Pierce, A. A., Barribeau, S. M., Sternberg, E. D., Mongue, A. J., and De Roode, J. C. (2012). Lack of genetic differentiation between monarch butterflies with divergent migration destinations. Mol. Ecol. 21, 3433–3444. doi: 10.1111/j.1365-294X.2012.05613.x
McKinley, D. C., Miller-Rushing, A. J., Ballard, H. L., Bonney, R., Brown, H., Cook-Patton, S. C., et al. (2017). Citizen science can improve conservation science, natural resource management, and environmental protection. Biol. Conserv. 208, 15–28. doi: 10.1016/j.biocon.2016.05.015
Miller, M. R., Dunham, J. P., Amores, A., Cresko, W. A., and Johnson, E. A. (2007). Rapid and cost-effective polymorphism identification and genotyping using restriction site associated DNA (RAD) markers. Genome Res. 17, 240–248. doi: 10.1101/gr.5681207
Moreau, C. S., and Wray, B. D. (2017). An empirical test of reduced-representation genomics to infer species-level phylogenies for two ant groups. Insect Syst. Divers. 1:ixx009. doi: 10.1093/isd/ixx009
Parchman, T. L., Gompert, Z., Mudge, J., Schilkey, F. D., Benkman, C. W., and Buerkle, C. A. (2012). Genome-wide association genetics of an adaptive trait in lodgepole pine. Mol. Ecol. 21, 2991–3005. doi: 10.1111/j.1365-294X.2012.05513.x
Pearcy, M., Goodisman, M. A., and Keller, L. (2011). Sib mating without inbreeding in the longhorn crazy ant. Proc. Biol. Sci. 278, 2677–2681. doi: 10.1098/rspb.2010.2562
Pećarević, M., Danoff-Burg, J., and Dunn, R. R. (2010). Biodiversity on broadway-enigmatic diversity of the societies of ants (Formicidae) on the streets of New York City. PLoS ONE 5:e13222. doi: 10.1371/journal.pone.0013222
Penick, C. A., Savage, A. M., and Dunn, R. R. (2015). Stable isotopes reveal links between human food inputs and urban ant diets. Proc. Biol. Sci. 282:20142608. doi: 10.1098/rspb.2014.2608
Peterson, B. K., Weber, J. N., Kay, E. H., Fisher, H. S., and Hoekstra, H. E. (2012). Double digest RADseq: an inexpensive method for de novo SNP discovery and genotyping in model and non-model species. PLoS ONE 7:e37135. doi: 10.1371/journal.pone.0037135
Pimm, S. L., Alibhai, S., Bergl, R., Dehgan, A., Giri, C., Jewell, Z., et al. (2015). Emerging technologies to conserve biodiversity. Trends Ecol. Evol. 30, 685–696. doi: 10.1016/j.tree.2015.08.008
Pocock, M. J., Tweddle, J. C., Savage, J., Robinson, L. D., and Roy, H. E. (2017). The diversity and evolution of ecological and environmental citizen science. PLoS ONE 12:e0172579. doi: 10.1371/journal.pone.0172579
Pritchard, J. K., Stephens, M., and Donnelly, P. (2000). Inference of population structure using multilocus genotype data. Genetics 155, 945–959.
Rabitsch, W. (2011). The hitchhiker's guide to alien ant invasions. BioControl 56, 551–572. doi: 10.1007/s10526-011-9370-x
Reuther, K. D. (2009). Early Invasion Dynamics of the Japanese Pavement Ant, Tetramorium Sushimae, in the Saint Louis, Missouri Metropolitan Area (Ph. D.). St. Louis, MO: Saint Louis University.
Ricciardi, A., Blackburn, T. M., Carlton, J. T., Dick, J. T., Hulme, P. E., Iacarella, J. C., et al. (2017). Invasion science: a horizon scan of emerging challenges and opportunities. Trends Ecol. Evol. 32, 464–474. doi: 10.1016/j.tree.2017.03.007
Ryan, S. F., Lombaert, E., Espeset, A., Vila, R., Talavera, G., Dinca, V., et al. (2019). Global invasion history of the agricultural pest butterfly Pieris rapae revealed with genomics and citizen science. Proc. Natl. Acad. Sci. U.S.A. 116, 20015–20024. doi: 10.1073/pnas.1907492116
Schär, S., Talavera, G., Espadaler, X., Rana, J. D., Andersen Andersen, A., Cover, S. P., et al. (2018). Do Holarctic ant species exist? Trans-Beringian dispersal and homoplasy in the Formicidae. J. Biogeogr. 45, 1917–1928. doi: 10.1111/jbi.13380
Schlick-Steiner, B. C., Steiner, F. M., Moder, K., Seifert, B., Sanetra, M., Dyreson, E., et al. (2006). A multidisciplinary approach reveals cryptic diversity in Western Palearctic Tetramorium ants (Hymenoptera: Formicidae). Mol. Phylogenet. Evol. 40, 259–273. doi: 10.1016/j.ympev.2006.03.005
Smith, C. C., Weber, J. N., Mikheyev, A. S., Roces, F., Bollazzi, M., Kellner, K., et al. (2019). Landscape genomics of an obligate mutualism: concordant and discordant population structures between the leafcutter ant Atta texana and its two main fungal symbiont types. Mol. Ecol. 28, 2831–2845. doi: 10.1111/mec.15111
Steiner, F. M., Schlick-Steiner, B. C., Trager, J. C., Moder, K., Sanetra, M., Christian, E., et al. (2006). Tetramorium tsushimae, a new invasive ant in North America. Biol. Invasions 8, 117–123. doi: 10.1007/s10530-004-1249-7
Steiner, F. M., Schlick-Steiner, B. C., VanDerWal, J., Reuther, K. D., Christian, E., Stauffer, C., et al. (2008). Combined modelling of distribution and niche in invasion biology: a case study of two invasive Tetramorium ant species. Divers. Distrib. 14, 538–545. doi: 10.1111/j.1472-4642.2008.00472.x
Storer, C., Payton, A., McDaniel, S., Jordal, B., and Hulcr, J. (2017). Cryptic genetic variation in an inbreeding and cosmopolitan pest, Xylosandrus crassiusculus, revealed using ddRADseq. Ecol. Evol. 7, 10974–10986. doi: 10.1002/ece3.3625
Taylor, H. R., and Gemmell, N. J. (2016). Emerging technologies to conserve biodiversity: further opportunities via genomics. Response to Pimm et al. Trends Ecol. Evol. 31, 171–172. doi: 10.1016/j.tree.2016.01.002
Tsutsui, N. D., and Suarez, A. V. (2003). The colony structure and population biology of invasive ants. Conserv. Biol. 17, 48–58. doi: 10.1046/j.1523-1739.2003.02018.x
Tsutsui, N. D., Suarez, A. V., and Grosberg, R. K. (2003). Genetic diversity, asymmetrical aggression, and recognition in a widespread invasive species. Proc. Natl. Acad. Sci. U.S.A. 100, 1078–1083. doi: 10.1073/pnas.0234412100
Vitone, T., Stofer, K., Steininger, M. S., Hulcr, J., Dunn, R., and Lucky, A. (2016). School of ants goes to college: integrating citizen science into the general education classroom increases engagement with science. J. Sci. Commun. 15:A03. doi: 10.22323/2.15010203
Wagner, H. C., Arthofer, W., Seifert, B., Muster, C., Steiner, F. M., and Schlick-Steiner, B. C. (2017). Light at the end of the tunnel: Integrative taxonomy delimits cryptic species in the Tetramorium caespitum complex (Hymenoptera: Formicidae). Myrmecol. News 25, 95–129. doi: 10.25849/myrmecol.news_025:095
Wagner, H. C., Gamisch, A., Arthofer, W., Moder, K., Steiner, F. M., and Schlick-Steiner, B. C. (2018). Evolution of morphological crypsis in the Tetramorium caespitum ant species complex (Hymenoptera: Formicidae). Sci. Rep. 8:12547. doi: 10.1038/s41598-018-30890-z
Wheeler, W. M. (1927). The occurrence of the pavement ant (Tetramorium caespitum L.) in Boston. Psyche: J. Entomol. 34, 164–165. doi: 10.1155/1927/13652
Keywords: COI, high-throughput sequencing, invasive species, population genetics, social insects, Tetramorium immigrans, SNPs
Citation: Zhang YM, Vitone TR, Storer CG, Payton AC, Dunn RR, Hulcr J, McDaniel SF and Lucky A (2019) From Pavement to Population Genomics: Characterizing a Long-Established Non-native Ant in North America Through Citizen Science and ddRADseq. Front. Ecol. Evol. 7:453. doi: 10.3389/fevo.2019.00453
Received: 30 August 2019; Accepted: 11 November 2019;
Published: 26 November 2019.
Edited by:
Emiliano Mori, University of Siena, ItalyReviewed by:
Enrico Schifani, University of Palermo, ItalyCopyright © 2019 Zhang, Vitone, Storer, Payton, Dunn, Hulcr, McDaniel and Lucky. This is an open-access article distributed under the terms of the Creative Commons Attribution License (CC BY). The use, distribution or reproduction in other forums is permitted, provided the original author(s) and the copyright owner(s) are credited and that the original publication in this journal is cited, in accordance with accepted academic practice. No use, distribution or reproduction is permitted which does not comply with these terms.
*Correspondence: Andrea Lucky, YWx1Y2t5QHVmbC5lZHU=
Disclaimer: All claims expressed in this article are solely those of the authors and do not necessarily represent those of their affiliated organizations, or those of the publisher, the editors and the reviewers. Any product that may be evaluated in this article or claim that may be made by its manufacturer is not guaranteed or endorsed by the publisher.
Research integrity at Frontiers
Learn more about the work of our research integrity team to safeguard the quality of each article we publish.