- 1Department of Biology, Wesleyan University, Middletown, CT, United States
- 2Department of Ecology and Evolutionary Biology, University of Connecticut, Storrs, CT, United States
Ecological interactions increasingly occur in the context of anthropogenic landscape alteration, such as landscape fragmentation, which engenders numerous changes to abiotic and biotic processes. Theory and empirical evidence suggest that species that are ecologically specialized or positioned at higher trophic levels are most sensitive to the effects of landscape fragmentation, yet the mechanisms underlying this sensitivity remain hypothetical. Here we present an initial test of the hypothesis that landscape fragmentation affects tri-trophic interactions more severely for dietary specialist than for generalist insect herbivores. We specifically tested a bottom-up hypothesis, that fragmentation reduces hostplant food quality to herbivores, with the most pronounced effects for dietary specialists. We also tested a top-down hypothesis, that fragmentation reduces parasitism of caterpillars, with the most pronounced effects on dietary specialists. We studied interactions among trees, caterpillars, and parasitoids in forest fragments (3–1013 ha) in Connecticut, U.S.A. in 2017 and 2018. To address the bottom-up hypothesis, we measured the growth performance of multiple dietary specialist and generalist caterpillar species fed leaves from red maple and witch hazel trees sampled from large or small forest patches. We assessed the top-down hypothesis by quantifying mortality from insect parasitoids of many of the same caterpillar species on these tree species sampled from a range of forest patch sizes. Fragmentation did not reduce herbivore growth performance for either dietary specialist or generalist caterpillars even though it accelerated desiccation rates of leaves of both tree species over the growing season. However, dietary specialization increased herbivore sensitivity to yearly variation in food quality, as the growth efficiency of specialist caterpillars varied between 2017 and 2018, while that of generalist caterpillars did not. As predicted, parasitism rates of dietary specialist caterpillars declined as forest fragment size declined, whereas parasitism of generalists was independent of fragment size. Therefore, interactions involving dietary specialist herbivores were most sensitive to top-down environmental variation. Assuming that dietary specialist caterpillars are primarily attacked by host-specific parasitoids, this top-down effect is consistent with the trophic theory of island biogeography, which predicts the sharpest declines of ecologically specialized predators and parasites in small habitat patches.
Introduction
The anthropogenic fragmentation of landscapes has the potential to dramatically alter species interactions (Aizen et al., 2012; Martinson and Fagan, 2014; Morante-Filho et al., 2016). The mechanisms by which species interactions may be altered by landscape fragmentation are numerous (Bagchi et al., 2018), complex (Murphy et al., 2016), and often context-dependent (Martinson and Fagan, 2014; Pfeifer et al., 2017). Community responses to landscape fragmentation are variable (Fáveri et al., 2008) and may be associated with differences in species traits such as dispersal ability (Roland and Taylor, 1997; Öckinger et al., 2010), physiological tolerance to extreme or variable abiotic conditions (Fourcade et al., 2017), or the ability to consume multiple resource types when resources are limited or absent (i.e., broad diet breadth) (Öckinger et al., 2010). That is, landscape fragmentation may differentially affect species and their interactions, and the community differences may be attributed to specific traits of the interactors.
Two theoretical frameworks seek to explain how landscape fragmentation changes communities. The trophic theory of island biogeography offers the most general and clear predictions. This framework proposes that area and isolation effects will exclude species that are ecologically specialized in some dimension relative to their generalist counterparts in the same community (Holt, 2010; Gravel et al., 2011). Additionally, species that are positioned at higher trophic levels, or have large resource requirements may be especially sensitive to the effects of landscape fragmentation (Kruess and Tscharntke, 1994; Holt et al., 1999; Gravel et al., 2011). Although there are grounds for both theoretical predictions, empirical evidence is still sparse.
The edge resource model is an alternative theoretical perspective that focuses on species responses to resource availability along habitat edges rather than area and isolation effects (Ries and Sisk, 2004; Ries et al., 2004). This model suggests that because of altered or variable abiotic and biotic conditions at habitat edges, resources will be spatially patchy or reduced in quality. One general prediction from this hypothesis is that habitat specialist species will tend to have negative responses to edges, whereas habitat generalists will have neutral or positive responses (Ries et al., 2004; Murphy et al., 2016). Although there is some empirical evidence for this prediction, this model has received limited testing (Ries et al., 2004; Wimp et al., 2011; Murphy et al., 2016).
As a major component of many fragmented terrestrial ecosystems, insect herbivores provide an opportunity to test alternative hypotheses that predict how species with contrasting levels of ecological specialization respond to landscape fragmentation. As insect herbivore population sizes are shaped by both their resources and the abundance and composition of predator communities (Vidal and Murphy, 2018), it is necessary to consider how landscape fragmentation may alter both the bottom-up (resource driven) and top-down (predator-driven) mechanisms (Murphy et al., 2016; Bagchi et al., 2018).
Bottom-Up Mechanisms
Landscape fragmentation may alter the availability of hostplant species through neutral sampling processes (Connor and McCoy, 1979), or by island biogeographic processes (MacArthur and Wilson, 1967; Hanski, 1999). As the ability for herbivores to locate specific hosts decreases in fragmented landscapes (Brown and Crone, 2016), limited hostplant availability may force insect herbivores to use alternative or suboptimal hosts, including hosts of the same or different species (Fox and Morrow, 1981), which may alter herbivore performance (Gripenberg et al., 2010).
Even when host plants are not limiting, landscape fragmentation may directly alter plant-herbivore interactions by acting at the plant phenotypic level. However, the extent to which landscape fragmentation may modify intraspecific plant phenotypic variation in anti-herbivore resistance traits or overall palatability is poorly understood (Bagchi et al., 2018). One hypothesis is that landscape fragmentation may alter the abiotic and biotic drivers of phenotypic variation in plant traits that determine food quality for herbivores. In small habitat patches, plant inbreeding may occur more frequently as population sizes diminish (Honnay et al., 2005; Leimu et al., 2010). Inbred plants may have altered nutritional quality and defensive responses (Ridley et al., 2011; Kittelson et al., 2015). Therefore, plant inbreeding has the potential to alter herbivore performance (Hull-Sanders and Eubanks, 2005).
The edge effects associated with landscape fragmentation may directly alter abiotic conditions that mediate intraspecific hostplant quality. Landscape fragmentation often adds new edges and increases the proportion of edge-to-interior habitat, intensifying edge effects in small, heavily fragmented landscapes (Ries and Sisk, 2004; Briant et al., 2010; Tuff et al., 2016). Fragment edges are often warmer, drier, more climatically variable, and have higher photosynthetically active radiation (Chen et al., 1999; Ries and Sisk, 2004; Savilaakso et al., 2009; Tuff et al., 2016; Agosta et al., 2017). These altered abiotic conditions and increased variability have been shown to increase canopy desiccation (Briant et al., 2010) which may alter plant traits that contribute to food quality for insect herbivores (e.g., Fortin and Mauffette, 2001).
Experimentally manipulated abiotic conditions have been shown to alter plant traits with consequences for insect herbivore performance (Buse et al., 1998; Levesque et al., 2002; Barber and Marquis, 2011). Winter Moth (Operophtera brumata) caterpillars fed leaves from Quercus robur grown in elevated temperatures grew less efficiently than caterpillars fed leaves grown in ambient temperatures (Buse et al., 1998). Additionally, the leaves of the tropical plant, Casearia nitida (Salicaceae) were tougher, thicker, and drier in young forests where ambient conditions were hotter, drier, and more variable, relative to leaves from mature forests with cooler, less variable ambient conditions (Agosta et al., 2017). Moreover, when caterpillars of the saturniid moth, Rothschildia lebeau, were fed leaves from Casearia nitida grown in early successional tropical forests, their growth and development were reduced relative to caterpillars fed leaves from mature tropical forests (Agosta et al., 2017). These examples suggest that altered abiotic conditions may indirectly alter food quality for insect herbivores through changes in plant traits. However, increased temperature may also directly influence insect herbivore performance and consumption rates; no clear general patterns have emerged because insect herbivores vary widely in their responses (Lemoine et al., 2014). Despite the possible mechanisms described above, whether or how landscape fragmentation affects insect herbivore performance through changes in intraspecific hostplant quality is a neglected topic of research.
Top-Down Mechanisms
Predator and parasitoid populations are predicted to decline in fragmented landscapes as sensitivity to habitat patch size increases with trophic position (Holt et al., 1999; Gravel et al., 2011) and degree of habitat specialization (Gravel et al., 2011; Murphy et al., 2016). The loss of natural enemies in fragmented landscapes may release prey populations from top-down control (Kruess and Tscharntke, 1994; Anton et al., 2007; Schüepp et al., 2014). However, the extent to which insect herbivores are released from predation may depend on the enemy's traits such as dispersal and competitive ability, and diet breadth (Roland and Taylor, 1997; Cagnolo et al., 2009; Nieminen and van Nouhuys, 2017). Release from top-down control may also depend on the herbivore's diet breadth because existing evidence from insect communities suggests that specialist predators and natural enemies tend to target dietary specialist herbivore prey (Dyer and Gentry, 2002; Stireman and Singer, 2003; Zvereva and Kozlov, 2016), and generalist predators and natural enemies tend to target generalist herbivores (Dyer, 1995; Singer et al., 2014; Zvereva and Kozlov, 2016), although there are exceptions to this pattern. Specifically, ecologically specialized predators and parasitoids that are highly dependent on ecologically specialized prey will be most susceptible to local extinction in fragmented landscapes when their prey is either reduced in abundance or entirely absent (Holt, 1996; Wimp et al., 2011). Therefore, communities in fragmented landscapes are likely to be deficient in interactions between ecologically specialized insect herbivores and their specialized natural enemies (Bagchi et al., 2018). There are many examples of predator declines in fragmented landscapes (Kruess and Tscharntke, 1994; Anton et al., 2007; Schüepp et al., 2014; Pfeifer et al., 2017), but the degree to which prey species of contrasting diet breadth are released from top-down control in a fragmented context remains understudied.
Here we investigate bottom-up and top-down mechanisms by which forest fragmentation alters tri-trophic interactions of dietary specialist and generalist herbivores in the same order (Lepidoptera) and feeding guild (exophytic leaf chewers). Theory on bottom-up mechanisms suggests that forest fragmentation could affect intraspecific hostplant quality, with distinct effects on dietary specialist and generalist herbivores. Both the trophic theory of island biogeography and the edge resource model postulate that forest fragmentation will render its strongest negative effects on ecologically specialized predators and natural enemies (Gravel et al., 2011; Murphy et al., 2016). To test these predictions, we assess (1) the effect of forest fragmentation on intraspecific hostplant quality in terms of caterpillar performance (growth efficiency) and leaf water content (a measure of food quality) and (2) parasitism rates of caterpillars collected from forest fragments of varying sizes.
Methods
Experimental Overview
We used a landscape-level experiment with manipulative and observational components to measure effects of forest fragmentation on intraspecific hostplant quality and caterpillar mortality due to parasitoids. Over the summers of 2017 and 2018, we collected caterpillars and leaves from temperate deciduous forest patches in Connecticut (U.S.A.) varying in size by two orders of magnitude. We compared leaf water content from plants grown in either small and heavily fragmented forests or large, continuous forests. Caterpillars were reared in laboratory environments to study their growth efficiency (performance in response to hostplant quality) on leaves collected from small and large forest patches. Additionally, laboratory-rearing also allowed us to quantify relative parasitism rates of caterpillars collected in forest patches of varying size.
Study Sites
Our study sites spanned a 3,500 km2 area in central and eastern Connecticut, U.S.A., in which the landscape is composed of patches of Northeastern Coastal Forest (Olson et al., 2001) surrounded by a matrix of suburban and agricultural land (Figure 1). We took advantage of existing forest patches ranging in area from 2.93 to 1,013 ha (Table 1). Forest patches were selected in blocks, with each block containing 2–3 fragments of contrasting area so that each small forest fragment (<50 ha) was paired with a large forest fragment (>200 ha) and sometimes an additional medium forest fragment (50–200 ha). The inclusion of fragments of contrasting area within each block ensured that fragment sizes were spatially interspersed to avoid issues with spatial autocorrelation in the statistical analyses. Forest boundaries varied and included two-lane roads, major highways, powerline clearings, and residential areas. Many of the sites in the small area class are bordered by major edges such as roads, multi-lane highways, power-line clearings, residential development, or industrial or commercial facilities. Within each forest patch, we restricted our collecting to upland areas that were similar in forest composition, comprising primarily mature Quercus alba (white oak), Quercus rubra (red oak), Acer rubrum (red maple), Acer saccharum (sugar maple), Carya spp. (hickories), and Betula lenta (black birch). Common woody understory species were Hamamelis virginiana (witch hazel), Kalmia latifolia (mountain laurel), Ostrya virginiana (American hophornbeam) and Carpinus caroliniana (American hornbeam). Plant communities were relatively homogeneous across sites. We did not collect data near patch edges, although both the small and large sites typically harbored more exotic plant species at the edge.
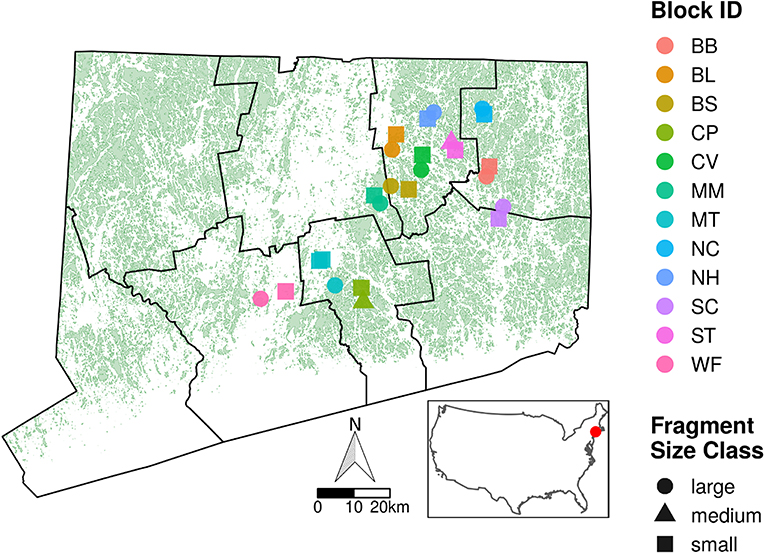
Figure 1. Map of Connecticut with forest fragments in green, county boundaries in black, and inset showing the position of Connecticut within the United States. Superimposed on the map of Connecticut are our 25 study sites spanning a 3,500 km2 area in east-central Connecticut. Experimental blocks are represented by different colors, and fragment area is indicated by shape. Large fragments are >201 ha, medium sites are 51–200 ha, and small sites are <50 ha. See Table 1 for further site and block information.
Plants
We studied growth performance and parasitism of caterpillars collected from A. rubrum and H. virginiana because these plant species were common in all forest sites and consistently harbored both dietary specialist and generalist caterpillars.
Caterpillars
Caterpillars of various larval stages were collected from A. rubrum and H. virginiana branches and saplings at 28 forest sites over the same 6-week period in June and early July of 2017 and 2018. In total, 882 caterpillars were collected, representing 49 species. We beat branches with a wooden dowel to shake caterpillars from branches onto a 1 m2 canvas sheet (Wagner, 2005). All caterpillars >1 cm in length were collected and individually placed into 33 ml plastic vials with a leaf from the host plant on which they were found. Caterpillars were collected with equal sampling effort in each patch as part of a concurrent study investigating the strength of bird and ant predation in forest patches of varying size. The community composition of caterpillar species was similar across patches of varying size as the host plants used harbor a typical and consistent community of dietary specialist caterpillars that feed exclusively on either A. rubrum or H. virginiana, respectively, and dietary generalist caterpillars that feed on many of the hardwood species found in these forests. For caterpillar species with >5 individuals collected, 94% (16/17) of dietary generalist species and 100% (9/9) of dietary specialist species were found in both large and small patches.
To characterize the diet breadth of caterpillar species, we identified every caterpillar to the species level so that we could compile host plant records for each caterpillar species using our own data and published sources (Wagner et al., 2002, 2011; Wagner, 2005). Based on these hostplant records, we calculated pairwise phylogenetic distances between woody hosts of each caterpillar species using a large phylogeny of seed plants (Smith and Brown, 2018). The mean of these pairwise phylogenetic distances was then used as a quantitative measure of diet breadth for each caterpillar species (Figure 2). Based on this quantitative measure of diet breadth, the caterpillar community studied here appears to fit discretely into two groups (specialist or generalist). Caterpillars that entirely or predominantly consume plants from one taxonomic family were considered dietary specialists, whereas caterpillars that commonly consume plants from >1 plant family were considered dietary generalists (Figure 2). Therefore, we evaluate all analyses using the discrete diet breadth descriptors, “specialist” and “generalist” to make analyses and interpretation more straightforward. Small sample sizes of caterpillar species precluded comparative analyses at the caterpillar species level.
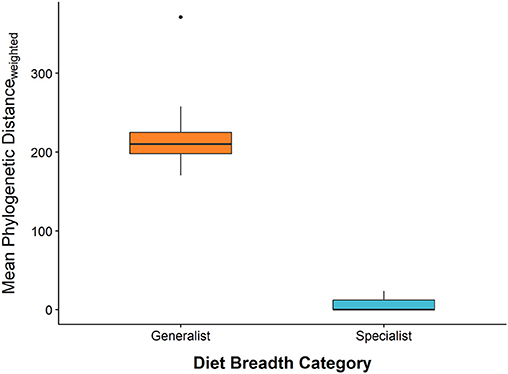
Figure 2. A comparison of each caterpillar species' continuous measure of diet breadth (mean phylogenetic distance among hostplant species) and categorically defined specialist and generalist subsets of the caterpillar community. Horizontal bars represent the median, box edges represent the 1st and 3rd interquartile range, whisker tips represent the minimum and maximum, and points are outliers. Outliers represent caterpillar species with small sample sizes.
Experimental Methods
Herbivore Performance Assay of Hostplant Quality
To test the hypothesis that forest fragmentation may alter the intraspecific quality of host plants as food for caterpillars, we reared all field-collected caterpillars from both years under controlled conditions in a laboratory setting. We measured variation in food quality for each hostplant species as the variation in growth efficiency of these lab-reared caterpillars. Upon laboratory arrival, each caterpillar was placed individually in a 160 ml plastic cup with a lid and starved for 24 h prior to initial weighing to avoid weighing gut contents and to account for disparities in larval instar among caterpillars at the time of collection. After starvation, each caterpillar was weighed to the nearest 1 mg and was randomly assigned a diet treatment group of either leaves from small (<50 ha) or large (>200 ha) forests. Each caterpillar in the assay received leaves only from its randomly assigned treatment group and from the hostplant species on which it was collected. Each day, each caterpillar in the assay received new leaves from a batch of field-collected leaves (see below). Leaves used in the assay were stored for no more than 5 days. Five days of sealed storage at 4°C had no effect on leaf water content for either hostplant species (linear regression, Pstorage.duration > 0.05, data not shown).
As a proxy for total food consumed, the fecal pellets of each caterpillar in the assay were collected daily and dried to constant mass at the end of the assay. Caterpillars completed the assay after pupating. Subsequently, pupae were sacrificed by overnight storage at −20°C, and then dried to constant mass. Many caterpillars brought to the laboratory for rearing either died from unknown causes (N = 468), pupated shortly after collection (N = 25), were parasitized (N = 71), or had incomplete data (N = 31) and were excluded from the assay. While none of the caterpillars that died from unknown causes had parasitoids emerge, it is possible that some of these individuals were parasitized. We did not dissect cadavers to confirm the cause of death. The proportion of caterpillars that died from unknown causes was similar among treatment groups (χ2 = 0.01, df = 1, P = 0.91). Similarly, whether caterpillars were collected from large or small forest fragments had little influence on unknown mortality (χ2 = 1.71, df = 1, P = 0.19).
Leaf Water Content
To test the hypothesis that forest fragmentation alters phenotypic variation in plant traits, we compared the water content of leaves of A. rubrum and H. virginiana collected from 21 forest sites ranging in size from 3 to 1,013 ha in the summer of 2018. Leaf water content is a reliable measure of food quality for folivores of temperate trees, as it often correlates positively with herbivore growth rate, leaf nitrogen, and overall palatability (Mattson and Scriber, 1987). Leaves were transported to the laboratory in sealed plastic bags kept in a cooler with ice. In the laboratory, leaves were stored in sealed plastic bags containing a damp paper towel and refrigerated at 4°C. Upon laboratory arrival, 10–13 randomly selected leaves from each host plant at each site were weighed to the nearest 1 mg. Leaves were then dried at 40°C for 1 week, or until stable dry masses were reached. Leaf water content was calculated as the ratio of water mass to fresh mass. Although leaves used for water content measurements were haphazardly selected, we intentionally avoided leaves that were not representative of the leaves that would be fed to caterpillars. That is, leaves with necrotic edges, browning, extreme size, or other anomalies were not used in either the leaf water content study or the performance assay.
Parasitoid Emergence
Throughout the performance assay, all caterpillars that had parasitoids emerge were removed from the assay and recorded. In 2018, we supplemented these data by separately rearing 189 caterpillars that were field-collected from 26 sites within the same fragmentation network used in the performance assay. Only caterpillars from A. rubrum and H. virginiana were collected. These supplemental caterpillars were individually reared in 160 ml plastic cups in similar laboratory conditions as the performance assay.
Data Analysis
All analyses were performed in R, version 3.5.1 (R Core Team., 2018).
Growth efficiency, quantified as pupal dry mass per unit dry fecal mass, was used as a measure of hostplant quality from the herbivores' perspective. Growth efficiency was quantified as the slope of the relationship between Log10 (dry pupal mass) and Log10 (dry fecal mass) (Singer, 2001). Growth efficiency is the amount of growth per unit plant material consumed, with fecal mass as a proxy for the amount of plant material consumed. Variation in this slope indicates variation in caterpillar growth efficiency and interactions between covariates (e.g., leaf origin and caterpillar diet breadth) and the relationship between dry fecal mass on dry pupal mass measure the covariates' effect on growth efficiency. We used a linear mixed-effects model (Bates et al., 2015) to analyze variation in Log10 (dry pupal mass) across individual caterpillars as a function of Log10 (dry fecal mass), leaf origin (small or large forest patches), the year the caterpillar was reared (2017 or 2018), hostplant species, and caterpillar diet breadth. To quantify how caterpillar growth efficiency varies between dietary generalists and specialists, we included a two-way interaction between diet breadth and Log10 (dry fecal mass). To determine whether this efficiency is modified by factors such as leaf origin and year, we also included each of these factors in a three-way interaction with diet breadth and Log10 (dry fecal mass). We allowed the intercept and effect of caterpillar species to vary among individuals as normally-distributed random effects (random intercept and slope model) to account for the variation among species in both Log10 (dry pupal mass) and growth efficiency. In preliminary analyses, the initial mass of the caterpillars was added as covariate. However, this term did not modify growth efficiency in any iteration of the model and was subsequently dropped from further analyses. The caterpillars in this experiment were fed leaves from small or large forest patches from multiple blocks. As a result, we cannot separate the variance associated with block from the error variance.
To quantify the effect of forest fragmentation on leaf water content we used a generalized linear mixed-effects model with a Beta error distribution (Brooks et al., 2017) to model leaf water content as a function of forest fragment area, hostplant species, collection date and their two- and three-way interactions. Separate intercepts for collection site were modeled as normally distributed random effects. As refrigerated storage did not alter leaf water content, we pooled measurements from leaves taken across 5 days of storage.
The probability of mortality from parasitoids was modeled as a function of forest fragment area, diet breadth, and their two-way interaction using a generalized linear mixed-effects model (Bates et al., 2015) assuming a binomial error distribution. Separate intercepts for block and collection site (nested within block) were modeled as normally distributed random effects. All records of parasitism were pooled across years and tree species to increase sample sizes. Preliminary analyses confirmed that neither days from collection to pupation nor collection date had any effect on the probability of parasitism. Consequently, these terms were not included in the final model. We confirmed the lack of spatial autocorrelation in the residuals by examining semi-variograms of the residuals (no trend observed) and computing Moran's I auto-correlation coefficient (I = −0.03, P = 0.635).
Estimating confidence intervals and making formal inferences from generalized linear mixed-models (GLMMs) is complicated by the absence of a standard method to calculate the denominator degrees of freedom associated with reference distributions. One flexible and robust approach for constructing confidence intervals (CIs) around the parameter estimates from GLMMs is parametric bootstrapping (Davison and Hinkley, 1997; Gelman and Hill, 2007). We simulated 1,000 sets of response data from the posterior distribution of the parameter estimates, refitted the model to each simulated data set and calculated the 2.5 and 97.5% quantiles of the parameter estimates across the refitted models to obtain their 95% confidence intervals. We estimated approximate, two-tailed P-values following the methodology of Bagchi et al. (2011). We present parameter estimates on the scale of the linear predictor with 95% CIs and approximate P-values.
Results
Bottom-Up Effects
In total, 290 caterpillars completed the growth performance assay (dietary generalists: N = 161, 22 spp.; dietary specialists: N = 129, 8 spp.). Although there was substantial variation in final mass (dry pupal mass), dietary specialist caterpillars were smaller, on average, than generalists (βspecialists = −0.308, 95% CI = 0.529–0.098, P = 0.008). Dietary generalist caterpillars fed H. virginiana leaves were larger, on average, than those fed A. rubrum leaves (βhost plant species = 0.149, 95% CI = 0.076–0.223, P < 0.001). However, hostplant species did not modify growth efficiency in any two- or three-way interactions and these interaction terms were subsequently removed from further analyses.
As expected, dry pupal mass was strongly associated with dry fecal mass (βfrass = 0.216, 95% CI = 0.115–0.318, P < 0.001, Figure 3). This growth efficiency relationship was not dependent on leaf origin for either dietary specialist or generalist caterpillars (βfrass : diet breadth : leaf origin = −0.009, 95% CI = −0.121–0.100, P = 0.860; Figure 3), suggesting that intraspecific variation in hostplant quality is not associated with forest fragment area in this system.
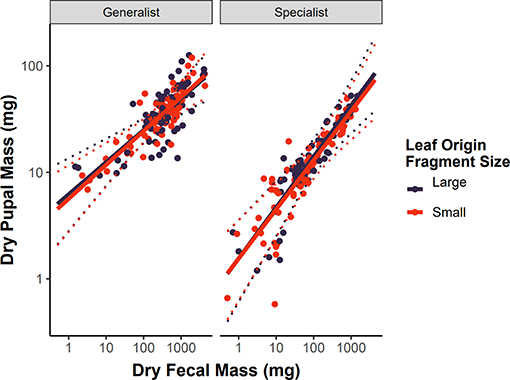
Figure 3. Growth efficiency of dietary specialist and generalist subsets of the caterpillar community on leaves collected from small (<50 ha) or large (>200 ha) forest patches. We define growth efficiency as the relationship between dry fecal mass (a proxy for total food consumed) and dry pupal mass (finishing mass). Variation in the slope of these relationships would indicate variation in intraspecific hostplant quality. Solid lines represent the predicted values from LMMs, dashed lines represent 95% confidence intervals around the predictions, and points are the raw data reshaped to account for the random effects of caterpillar species.
Variation in growth efficiency between dietary generalist and specialist caterpillars was highly dependent on year. Generalists had approximately equal growth efficiencies across years (βfrass : generalist : year = 0.049, 95% CI = −0.061–0.155, P = 0.408, Figure 4), while specialists grew significantly more efficiently in 2018 (βfrass : specialist : year = 0.282, 95% CI = 0.130–0.446, P < 0.001, Figure 4). Notably, in 2017, dietary specialist caterpillars grew less efficiently than generalists while the opposite was true in 2018 (Figure 4).
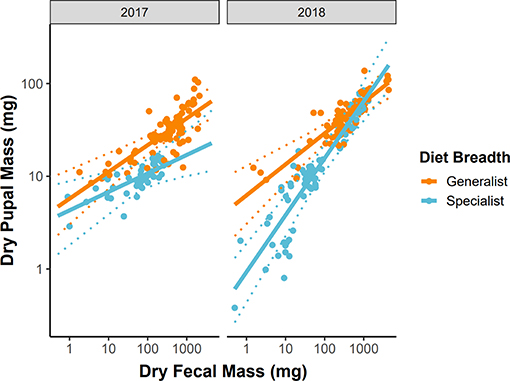
Figure 4. Growth efficiency across years for dietary specialist and generalist subsets of the caterpillar community. Variation in the slope of these relationships indicates variation in growth efficiency between dietary specialist and generalist caterpillars. Solid lines represent the predicted values from LMMs, dashed lines represent 95% confidence intervals around the predictions, and points are the raw data reshaped to account for the random effects of caterpillar species.
Additionally, to confirm that our analytical approach of using all species in a single model had not masked species-specific results, we ran the same models described above (without the random effect terms) for each of the nine (4 dietary specialists and 5 dietary generalists) most abundant species individually and found results consistent with the model that includes all caterpillar species. That is, growth efficiency was not modified by the leaf origin treatment for any of the nine most abundant species (P > 0.10each species). In individual analyses, year similarly modified the growth efficiency of dietary specialist caterpillars (P < 0.05each species) but not dietary generalist caterpillars (P > 0.10each species).
In 2018, we quantified leaf water content from 1,077 leaves of A. rubrum and 1,063 leaves of H. virginiana. There was substantial variation in leaf water content among leaves within sites. H. virginiana leaves had consistently more water than A. rubrum leaves (βhost plant species = 0.260, 95% CI = 0.249–0.271, P < 0.001, Figure 5). Consistent with the herbivore growth performance assay results, forest fragment area had no effect on leaf water content for A. rubrum (βfragment area : A. rubrum = 0.006, 95% CI = −0.004–0.048, P = 0.756). However, for H. virginiana there was a slight increase in leaf water content with forest fragment area (βfragment area : H. virginiana = 0.025, 95% CI = 0.013–0.036, P < 0.001). As expected, leaf water content declined throughout the summer (βcollection date = −0.042, 95% CI = −0.050–0.035, P < 0.001, Figure 5), with no noticeable difference between the slopes for A. rubrum and H. virginiana (βcollection date : H. virginiana = 0.006, 95% CI = −0.004–0.017, P = 0.240, Figure 5). Leaf water content declined more rapidly over time in smaller forest fragments (βcollection date : fragment area = 0.024, 95% CI = 0.016–0.031, P < 0.001, Figure 5), regardless of host plant species.
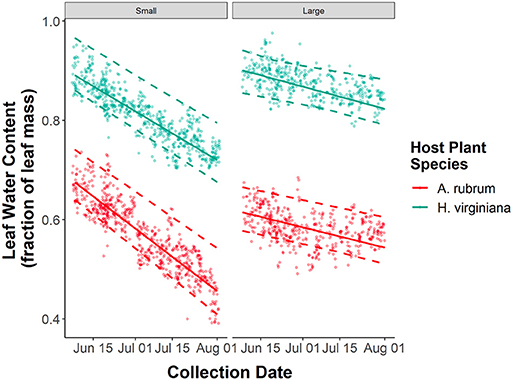
Figure 5. Seasonal declines in leaf water content of Acer rubrum and Hamamelis virginiana trees in relation to forest patch size. Solid lines represent the predicted values from GLMMs, dashed lines represent 95% confidence intervals around the predictions, and points are the raw data.
Top-Down Effects
Of the 1071 caterpillars collected (dietary specialists: N = 401, 13 spp.; dietary generalists: N = 670, 40 spp.), 12.0% (129) were parasitized. Parasitoids emerged from 71 dietary specialist caterpillars (17.7%) across 3 species and 58 generalist caterpillars (8.7%) across 17 species. Dietary specialist caterpillars had a higher probability of parasitism than did generalist caterpillars (βspecialist = 0.9706, 95% CI = 0.610–1.341, P < 0.001). Additionally, the probability of parasitism for specialist caterpillars increased with forest fragment area (βfragment area : specialist = 0.501, 95% CI = 0.112–0.964, P = 0.022, Figure 6). In the largest fragments, dietary specialist caterpillars were 1.8 times as likely to be parasitized relative to those in the smallest fragments (Figure 6). The probability of parasitism for generalist caterpillars decreased slightly with increased forest fragment area (βfragment area : generalist = −0.273, 95% CI = −0.673–0.036, P = 0.088, Figure 6).
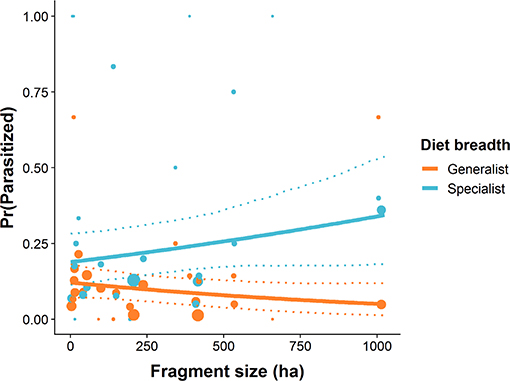
Figure 6. The probability of parasitism for dietary specialist and generalist subsets of the caterpillar community in relation to forest patch size. Solid lines represent the predicted values from GLMMs, dashed lines represent 95% confidence intervals around the predictions, points are the raw data, and the size of the point represents the sample size.
Discussion
Bottom-Up Mechanisms
The hypothesis that forest fragmentation alters intraspecific hostplant quality was not supported. Neither dietary specialist nor generalist caterpillars varied in growth efficiency when fed leaves from forests of contrasting area. As food quality is best measured by the growth response of the herbivore, these findings suggest that intraspecific hostplant quality is not affected by forest fragmentation, at least in this system and at these scales. To our knowledge, no other studies have directly measured insect herbivore performance in the context of area effects associated with forest fragmentation. Alternatively, edge effects in similar forest types have been shown to alter larval performance in polyphagous Malacosoma disstria (Lasiocampidae) caterpillars fed leaves from Acer saccharum (sugar maple) (Fortin and Mauffette, 2001). Compared to those fed leaves from forest interiors, M. disstria caterpillars fed leaves from forest edges were larger and had greater survival; these effects were attributed to higher levels of nitrogen, soluble sugars, and total phenolics in leaves grown at the forest edge despite having lower leaf water content (Fortin and Mauffette, 2001). Another explanation for the lack of an effect of forest fragment size on caterpillar growth performance is that lab-rearing conditions may attenuate differences in abiotic conditions that the herbivore experiences in field settings. For example, abiotic factors such as temperature may also mediate herbivore performance more directly. Although not tested here, higher temperature can increase consumption rates (Buse et al., 1998), which could enable herbivores to compensate for reduced food quality. However, whether and the degree to which temperature and food quality interact is difficult to predict because herbivores vary widely in their responses to increased temperature (Lemoine et al., 2014).
Although there was no effect of forest fragmentation on growth efficiency in our study, dietary generalist caterpillars grew larger on leaves of H. virginiana, which had a higher water content than A. rubrum. This result is consistent with previous evidence that leaves of H. virginiana offer higher food quality to dietary generalist caterpillars than do those of A. rubrum (Singer et al., 2012). That dietary generalist caterpillars found on both hostplant species grew larger on H. virginiana lends support for leaf water content contributing to food quality. Along with leaf nitrogen content, leaf water content has been shown to be highly predictive of herbivore growth performance (Scriber and Slansky, 1981), although the disparities may also be attributable to other differences in fiber or secondary metabolites (Mattson and Scriber, 1987).
Interestingly, the community of dietary specialist caterpillars grew much less efficiently in 2017, while the community of dietary generalist caterpillars grew with equivalent efficiency in both years. While forest fragment area cannot explain the decrease in growth efficiency of dietary specialist caterpillars, other landscape variables may be at play. In 2017, the forest region in this study experienced an outbreak of Gypsy moth (Lymantria dispar, a dietary generalist) caterpillars, which resulted in large-scale defoliation (Pasquarella et al., 2018). We hypothesize that the magnitude of this defoliation may have induced anti-herbivore resistance via increased mechanical defenses such as leaf toughness, which has been shown to reduce insect herbivore performance (Clissold et al., 2009). Induced mechanical defenses such as leaf thickness or toughness may disproportionately affect dietary specialist species that are often smaller (Davis et al., 2013, this study; Figures 3, 4) and may not have the ability to efficiently chew tough leaves. Dietary generalists, however, often have to cope with substantial resource variation across many host plants (Michaud, 1990). Consequently, they may have superior physiological mechanisms that buffer them against year-to-year variation in host plant quality.
The effects of forest fragment area on leaf water content were nuanced. Consistent with other studies of forest fragmentation that have measured leaf water content (Fáveri et al., 2008; Rossetti et al., 2014), we found that leaf water content varied independently of fragment area for A. rubrum. There was a slight increase in water content in H. virginiana leaves from large fragments, although the magnitude of this effect was small and did not translate into better quality food for caterpillars. In our study, the rate at which leaf water content declined throughout the summer was dependent on fragment area with leaves from the small forest fragments showing the sharpest decline in water content throughout the summer (Figure 4). Leaf water content declined in the large forest fragments as well, but at a lower rate (Figure 4). To our knowledge, this is the first example of temporal dependence in the relationship between leaf water content and forest fragment area. Similar studies on leaf water content have found either no effect of area (Fáveri et al., 2008), only edge effects (Briant et al., 2010; Rossetti et al., 2014), or edge by area interactive effects (Briant et al., 2010).
It is possible that the herbivore performance assay did not reflect these temporal differences in leaf water content because the majority of caterpillars in our assay pupated by early July, before which the disparities in leaf water content between large and small fragments were small. As the majority of Lepidoptera in these forests complete their larval development in late spring or early summer (Wagner et al., 2002, 2011; Wagner, 2005), it is possible that the faster desiccation rate of leaf water in small forest fragments may only affect insect herbivores that are multivoltine or have prolonged development times that carry late into the summer.
Top-Down Mechanisms
Theoretical frameworks based on area and isolation effects and on edge effects predict that species' persistence in fragmented habitats is inversely related to degree of ecological specialization (Ries et al., 2004; Gravel et al., 2011). Trophic island biogeography theory (Holt et al., 1999; Gravel et al., 2011) additionally predicts that trophic rank will contribute to sensitivity to fragmentation. That is, ecologically specialized predators are most likely to become locally extinct in small habitat patches. Our results are consistent with these predictions, although they cannot distinguish among these alternative theoretical frameworks. That the probability of parasitism for dietary specialist caterpillars increased with forest fragment area while the probability of parasitism for generalist caterpillars varied independently of fragment area suggests to us that host-specific parasitoids were poorly represented in the communities of small forest fragments. Parasitoids that emerged from caterpillars in this study were not identified, thus we cannot conclude explicitly that the lower parasitism rates are due to a loss of host-specific parasitoids in small forest fragments. However, caterpillar-parasitoid interaction data from both tropical (Dyer and Gentry, 2002) and temperate (Stireman and Singer, 2003) communities suggest a widespread pattern of host-specific parasitoids attacking host-specific caterpillars. If the caterpillar-parasitoid interactions in the community studied here show this same pattern, the observed decline in parasitism of dietary specialist caterpillars in small forest patches would support the prediction that specialized parasitoids are especially sensitive to landscape fragmentation (Gravel et al., 2011; Murphy et al., 2016). A key test of both area/isolation and edge effects comes from studies of the leafminer-parasitoid network in Chaco-Serrano dry forest remnants of Argentina (Valladares et al., 2006; Cagnolo et al., 2009). Parasitism rates of leafminers declined with decreasing area of forest remnants, but increased along forest edges despite negative edge responses of parasitoid abundance (Valladares et al., 2006). It is important to note that the area/isolation effects were independent of edge effects on parasitism rate. Further study revealed that species richness of leaf-miner parasitoids declined in the smallest forest remnants with host-specific parasitoids showing sharper species-area relationships than their generalist counterparts (Valladares et al., 2012). Together, these results and others (e.g., Aizen et al., 2012) suggest that heavily fragmented landscapes are deficient in specialized interactions between parasitoids and hosts.
In conclusion, our results show evidence of both bottom-up and top-down effects of forest fragmentation on the plant-insect community. However, the bottom-up effect we studied, intraspecific variation in hostplant quality, did not support the hypothesis that leaves of trees in relatively fragmented forest were of such reduced food quality as to limit caterpillar growth performance. It is still possible that plant community changes wrought by forest fragmentation do have significant effects upon the insect herbivore community in this system, and we are actively investigating this possibility. By contrast, the top-down effect we studied, mortality of caterpillars due to parasitoids, was altered by landscape fragmentation in a manner that accorded with theoretical predictions (area/isolation effects, edge effects). Our results provide preliminary evidence that forest fragmentation can alter ecological communities by disrupting trophic interactions.
Data Availability Statement
All data used in the analyses presented here can be found in the Supplemental Material. Please contact the corresponding author for access to R code.
Author Contributions
RA, MS, RB, and CC conceived the ideas. MS and RB obtained funding. RA, ND, NP, and CC carried out the experiments. RA drafted the initial manuscript. MS, RB, and JM contributed sections and revisions. RA, RB, and JM performed statistical analyses and developed the figures.
Funding
This work was provided by the NSF (DEB: 1557086, REU: 1820555), Wesleyan University and the University of Connecticut.
Conflict of Interest
The authors declare that the research was conducted in the absence of any commercial or financial relationships that could be construed as a potential conflict of interest.
Acknowledgments
We thank David L. Wagner (U. Connecticut) for help with caterpillar identification and rearing; thanks to Adrianna Perez, Hanna Holcomb, Christopher White, Riley Hawkins-Hecock, Justin Zhao, Hooman Musavi, Courtney Marren, Caitlin Garvey, Daniel Charron, Samuel Erickson, and Ian Barron for their assistance with field and lab work.
Supplementary Material
The Supplementary Material for this article can be found online at: https://www.frontiersin.org/articles/10.3389/fevo.2019.00452/full#supplementary-material
References
Agosta, S. J., Hulshof, C. M., and Staats, E. G. (2017). Organismal responses to habitat change: herbivore performance, climate and leaf traits in regenerating tropical dry forests. J. Anim. Ecol. 86, 590–604. doi: 10.1111/1365-2656.12647
Aizen, M. A., Sabatino, M., and Tylianakis, J. M. (2012). Specialization and rarity predict nonrandom loss of interactions from mutualist networks. Science 335, 1486–1489. doi: 10.1126/science.1215320
Anton, C., Zeisset, I., Musche, M., Durka, W., Boomsma, J. J., and Settele, J. (2007). Population structure of a large blue butterfly and its specialist parasitoid in a fragmented landscape. Mol. Ecol. 16, 3828–38. doi: 10.1111/j.1365-294X.2007.03441.x
Bagchi, R., Brown, L. M., Elphick, C. S., Wagner, D. L., and Singer, M. S. (2018). Anthropogenic fragmentation of landscapes: mechanisms for eroding the specificity of plant–herbivore interactions. Oecologia 187, 521–533. doi: 10.1007/s00442-018-4115-5
Bagchi, R., Philipson, C. D., Slade, E. M., Hector, A., Phillips, S., Villanueva, J. F., et al. (2011). Impacts of logging on density-dependent predation of dipterocarp seeds in a south east Asian rainforest. Philos. Trans. R Soc. Lond. B Biol. Sci. 366, 3246–3255. doi: 10.1098/rstb.2011.0034
Barber, N. A., and Marquis, R. J. (2011). Light environment and the impacts of foliage quality on herbivorous insect attack and bird predation. Oecologia 166, 401–409. doi: 10.1007/s00442-010-1840-9
Bates, D., Machler, M., Bolker, B., and Walker, S. (2015). Fitting linear mixed-effects models using lme4. J. Stat. Softw. 67, 1–48. doi: 10.18637/jss.v067.i01
Briant, G., Gond, V., and Laurance, S. G. W. (2010). Habitat fragmentation and the desiccation of forest canopies: a case study from eastern Amazonia. Biol. Conserv. 143, 2763–2769. doi: 10.1016/j.biocon.2010.07.024
Brooks, M. E., Kristensen, K., van Benthem, K. J., Magnusson, A., Berg, C. W., Nielsen, A., et al. (2017). GlmmTMB balances speed and flexibility among packages for zero-inflated generalized linear mixed modeling. R J. 9, 378–400. doi: 10.32614/RJ-2017-066
Brown, L. M., and Crone, E. E. (2016). Minimum area requirements for an at-risk butterfly based on movement and demography. Conserv. Biol. 30, 103–112. doi: 10.1111/cobi.12588
Buse, A., Good, J. E. G., Dury, S., and Perrins, C. M. (1998). Effects of elevated temperature and carbon dioxide on the nutritional quality of leaves of oak (Quercus robur L.) as food for the winter moth (Operophtera brumata L.). Funct. Ecol. 12, 742–749. doi: 10.1046/j.1365-2435.1998.00243.x
Cagnolo, L., Valladares, G., Salvo, A., Cabido, M., and Zak, M. (2009). Habitat fragmentation and species loss across three interacting trophic levels: effects of life-history and food-web traits. Conserv. Biol. 23, 1167–1175. doi: 10.1111/j.1523-1739.2009.01214.x
Chen, J., Saunders, S. C., Crow, T. J., Naiman, R. J., Kimberley, D., Mroz, G. D., et al. (1999). In forest microclimate and ecosystem ecology landscape the effects of different management regimes. BioScience 49, 288–297. doi: 10.2307/1313612
Clissold, F. J., Sanson, G. D., Read, J., and Simpson, S. J. (2009). Gross vs. net income: how plant toughness affects performance of an insect herbivore. Ecology 90, 3393–3405. doi: 10.1890/09-0130.1
Connor, E. F., and McCoy, E. D. (1979). The statistics and biology of the species-area relationship. Am. Nat. 113, 791–833. doi: 10.1086/283438
Davis, R. B., Ounap, E., Javoiš, J., Gerhold, P., and Tammaru, T. (2013). Degree of specialization is related to body size in herbivorous insects. Evolution 67, 583–589. doi: 10.1111/j.1558-5646.2012.01776.x
Davison, A. C., and Hinkley, D. V. (1997). Bootstrap Methods and Their Application. 1st Edn. Cambridge: Cambridge University Press. doi: 10.1017/CBO9780511802843
Dyer, L. (1995). Tasty generalists and nasty specialists? Antipredator mechanisms in tropical Lepidopteran larvae. Ecology 76, 1483–1496. doi: 10.2307/1938150
Dyer, L., and Gentry, G. (2002). On the conditional nature of neotropical caterpillar defenses against their natural enemies. Ecology 83, 3108–3119. doi: 10.1890/0012-9658(2002)083[3108:OTCNON]2.0.CO;2
Fáveri, S. B., Vasconcelos, H. L., and Dirzo, R. (2008). Effects of Amazonian forest fragmentation on the interaction between plants, insect herbivores, and their natural enemies. J. Trop. Ecol. 24, 57–64. doi: 10.1017/S0266467407004592
Fortin, M., and Mauffette, Y. (2001). Forest edge effects on the biological performance of the forest tent caterpillar (Lepidoptera: Lasiocampidae) in sugar maple stands. Ecoscience 8, 164–172. doi: 10.1080/11956860.2001.11682642
Fourcade, Y., Ranius, T., and Öckinger, E. (2017). Temperature drives abundance fluctuations, but spatial dynamics is constrained by landscape configuration: implications for climate-driven range shift in a butterfly. J. Anim. Ecol. 86, 1339–1351. doi: 10.1111/1365-2656.12740
Fox, L. R., and Morrow, P. A. (1981). Specialization: species property or local phenomenon. Science 211, 887–893. doi: 10.1126/science.211.4485.887
Gelman, A., and Hill, J. (2007). Data Analysis Using Regression and Multilevel/Hierarchical Models. New York, NY: Cambridge University Press. doi: 10.1017/CBO9780511790942
Gravel, D., Massol, F., Canard, E., Mouillot, D., and Mouquet, N. (2011). Trophic theory of island biogeography. Ecol. Lett. 14, 1010–1016. doi: 10.1111/j.1461-0248.2011.01667.x
Gripenberg, S., Mayhew, P. J., Parnell, M., and Roslin, T. (2010). A meta-analysis of preference-performance relationships in phytophagous insects. Ecol. Lett. 13, 383–393. doi: 10.1111/j.1461-0248.2009.01433.x
Holt, R. D. (1996). “Food webs in space: an island biogeographic perspective,” in Food Webs, Integration of Patterns and Dynamics, eds G. A. Polis and K. O. Winemiller (London: Chapman & Hall), 313–323. doi: 10.1007/978-1-4615-7007-3_30
Holt, R. D. (2010). “Toward a trophic theory of island biogeography,” in The Theory of Island Biogeography Revisted, eds J. B. Losos and R. E. Ricklefs (Princeton, NJ: Princeton University Press), 143–185. doi: 10.1515/9781400831920.143
Holt, R. D., Lawton, J. H., Polis, G. A., and Martinez, N. D. (1999). Trophic rank and the species–area relationship. Ecology 80, 1495–1504. doi: 10.1890/0012-9658(1999)080[1495:TRATSA]2.0.CO;2
Honnay, O., Jacquemyn, H., Bossuyt, B., and Hermy, M. (2005). Forest fragmentation effects on patch occupancy and population viability of herbaceous plant species. New Phytol. 166, 723–736. doi: 10.1111/j.1469-8137.2005.01352.x
Hull-Sanders, H. M., and Eubanks, M. D. (2005). Plant defense theory provides insight into interactions involving inbred plants and insect herbivores. Ecology 86, 897–904. doi: 10.1890/04-0935
Kittelson, P. M., Wagenius, S., Nielsen, R., Qazi, S., Howe, M., Kiefer, G., et al. (2015). How functional traits, herbivory, and genetic diversity interact in echinacea: implications for fragmented populations. Ecology 96, 1877–1886. doi: 10.1890/14-1687.1
Kruess, A., and Tscharntke, T. (1994). Habitat fragmentation, species loss, and biological control. Science 264, 1581–1584. doi: 10.1126/science.264.5165.1581
Leimu, R., Vergeer, P., Angeloni, F., and Ouborg, N. J. (2010). Habitat fragmentation, climate change, and inbreeding in plants. Ann. N. Y. Acad. Sci. 1195, 84–98. doi: 10.1111/j.1749-6632.2010.05450.x
Lemoine, N. P., Burkepile, D. E., and Parker, J. D. (2014). Variable effects of temperature on insect herbivory. PeerJ 2:e376. doi: 10.7717/peerj.376
Levesque, K. R., Fortin, M., and Mauffette, Y. (2002). Temperature and food quality effects on growth, consumption and post-ingestive utilization efficiencies of the forest tent caterpillar Malacosoma disstria (Lepidoptera: Lasiocampidae). Bull. Entomol. Res. 92, 127–136. doi: 10.1079/BER2002153
MacArthur, R. H., and Wilson, E. O. (1967). The Theory of Island Biogeography. Princetown, NJ: Princeton University Press.
Martinson, H. M., and Fagan, W. F. (2014). Trophic disruption: a meta-analysis of how habitat fragmentation affects resource consumption in terrestrial arthropod systems. Ecol. Lett. 17, 1178–1189. doi: 10.1111/ele.12305
Mattson, W. J., and Scriber, J. M. (1987). “Nutritional ecology of insect folivores of woody plants: nitrogen, water, fiber, and mineral considerations,” in Nutritional Ecology of Insects, Mites, Spiders, and Related Invertebrates, eds F. Jr. Slansky and J. G. Rodrigues (New York, NY: John Wiley and Sons), 105–146.
Michaud, J. P. (1990). Conditions for the evolution of polyphagy in herbivorous insects. Oikos 57, 278–279. doi: 10.2307/3565951
Morante-Filho, J. C., Arroyo-Rodríguez, V., Lohbeck, M., Tscharntke, T., and Faria, D. (2016). Tropical forest loss and its multitrophic effects on insect herbivory. Ecology 97, 3315–3325. doi: 10.1002/ecy.1592
Murphy, S. M., Battocletti, A. H., Tinghitella, R. M., Wimp, G. M., and Ries, L. (2016). Complex community and evolutionary responses to habitat fragmentation and habitat edges: what can we learn from insect science? Curr. Opin. Insect Sci. 14, 61–65. doi: 10.1016/j.cois.2016.01.007
Nieminen, M., and van Nouhuys, S. (2017). The roles of trophic interactions, competition and landscape in determining metacommunity structure of a seed-feeding weevil and its parasitoids. Ann. Zool. Fenn. 54, 83–95. doi: 10.5735/086.054.0109
Öckinger, E., Schweiger, O., Crist, T. O., Debinski, D. M., Krauss, J., Kuussaari, M., et al. (2010). Life-history traits predict species responses to habitat area and isolation: a cross-continental synthesis. Ecol. Lett. 13, 969–979. doi: 10.1111/j.1461-0248.2010.01487.x
Olson, D. M., Dinerstein, E., Wikramanayake, E. D., Burgess, N. D., Powell, G. V. N., Underwood, E. C., et al. (2001). Terrestrial ecoregions of the world: a new map of life on earth. BioScience 51, 933–938. doi: 10.1641/0006-3568(2001)051[0933:TEOTWA]2.0.CO;2
Pasquarella, V. J., Elkinton, J. S., and Bradley, B. A. (2018). Extensive gypsy moth defoliation in southern New England characterized using Landsat satellite observations. Biol. Invasions 20, 3047–3053. doi: 10.1007/s10530-018-1778-0
Pfeifer, M., Lefebvre, V., Peres, C. A., Banks-Leite, C., Wearn, O. R., Marsh, C. J., et al. (2017). Creation of forest edges has a global impact on forest vertebrates. Nature 551, 187–191. doi: 10.1038/nature.24457
R Core Team. (2018). R: A Language and Environment for Statistical Computing. Vienna: R Foundation for Statistical Computing. https://www.r-project.org/
Ridley, C. E., Hangelbroek, H. H., Wagenius, S., Stanton-Geddes, J., and Shaw, R. G. (2011). The effect of plant inbreeding and stoichiometry on interactions with herbivores in nature: Echinacea angustifolia and its specialist aphid. PLoS ONE 6:e24762. doi: 10.1371/journal.pone.0024762
Ries, L., Fletcher, R. J. Jr., Battin, J., and Sisk, T. (2004). Ecological responses to habitat edges: mechanisms, models, variability explained. Ann. Rev. Ecol. Evol. Syst. 35, 491–522. doi: 10.1146/annurev.ecolsys.35.112202.130148
Ries, L., and Sisk, T. (2004). A predictive model of edge effects. Ecology 85, 2917–2926. doi: 10.1890/03-8021
Roland, J., and Taylor, P. D. (1997). Insect parasitoid species respond to forest structure at different spatial scales. Nature 386, 710–713. doi: 10.1038/386710a0
Rossetti, M. R., González, E., Salvo, A., and Valladares, G. (2014). Not all in the same boat: trends and mechanisms in herbivory responses to forest fragmentation differ among insect guilds. Arthropod-Plant Inte. 8, 593–603. doi: 10.1007/s11829-014-9342-z
Savilaakso, S., Koivisto, J., Veteli, T. O., and Roininen, H. (2009). Microclimate and tree community linked to differences in Lepidopteran larval communities between forest fragments and continuous forest. Divers. Distrib. 15, 356–365. doi: 10.1111/j.1472-4642.2008.00542.x
Schüepp, C., Uzman, D., Herzog, F., and Entling, M. H. (2014). Habitat isolation affects plant-herbivore-enemy interactions on cherry trees. Biol. Control 71, 56–64. doi: 10.1016/j.biocontrol.2014.01.007
Scriber, J. M., and Slansky, F. Jr. (1981). The nutritional ecology of immature insects. Ann. Rev. Entomol. 26, 183–211. doi: 10.1146/annurev.en.26.010181.001151
Singer, M. S. (2001). Determinants of polyphagy by a woolly bear caterpillar: a test of the physiological efficiency hypothesis. Oikos 93, 194–204. doi: 10.1034/j.1600-0706.2001.930203.x
Singer, M. S., Farkas, T. E., Skorik, C. M., and Mooney, K. A. (2012). Tritrophic interactions at a community level: effects of host plant species quality on bird predation of caterpillars. Am. Nat. 179, 363–374. doi: 10.1086/664080
Singer, M. S., Lichter-Marck, I. H., Farkas, T. E., Aaron, E., Whitney, K. D., and Mooney, K. A. (2014). Herbivore diet breadth mediates the cascading effects of carnivores in food webs. Proc. Nat. Acad. Sci. U. S.A. 111, 9521–9526. doi: 10.1073/pnas.1401949111
Smith, S. A., and Brown, J. M. (2018). Constructing a broadly inclusive seed plant phylogeny. Am. J. Bot. 105, 302–314. doi: 10.1002/ajb2.1019
Stireman, J. O., and Singer, M. S. (2003). Determinants of host-parasitoid associations: insights from a natural Tachinid-Lepidopteran community. Ecology 84, 296–310. doi: 10.1890/0012-9658(2003)084[0296:DOPHAI]2.0.CO;2
Tuff, K. T., Tuff, T., and Davies, K. F. (2016). A framework for integrating thermal biology into fragmentation research. Ecol. Lett. 19, 361–374. doi: 10.1111/ele.12579
Valladares, G., Cagnolo, L., and Salvo, A. (2012). Forest fragmentation leads to food web contraction. Oikos 121, 299–305. doi: 10.1111/j.1600-0706.2011.19671.x
Valladares, G., Salvo, A., and Cagnolo, L. (2006). Habitat fragmentation effects on trophic processes of insect-plant food webs. Conserv. Biol. 20, 212–217. doi: 10.1111/j.1523-1739.2006.00337.x
Vidal, M. C., and Murphy, S. M. (2018). Bottom-up vs. top-down effects on terrestrial insect herbivores: a meta-analysis. Ecol. Lett. 21, 138–150. doi: 10.1111/ele.12874
Wagner, D. L. (2005). Caterpillars of Eastern North America. Princeton, NJ: Princeton University Press.
Wagner, D. L., Ferguson, D., McCabe, T., and Reardon, R. (2002). Geometriod Caterpillars of Northeastern and Appalachian Forests. FHTET-2001. Morgantown, WV: USFS Technology Transfer Bulletin. doi: 10.5962/bhl.title.150204
Wagner, D. L., Schweitzer, D. F., Sullivan, J. B., and Reardon, R. C. (2011). Owlet Caterpillars of Eastern North America. Princeton, NJ: Princeton University Press.
Wimp, G. M., Murphy, S. M., Lewis, D., and Ries, L. (2011). Do edge responses cascade up or down a multi-trophic food web? Ecol. Lett. 14, 863–870. doi: 10.1111/j.1461-0248.2011.01656.x
Keywords: habitat modification, herbivore performance, hostplant quality, Lepidoptera, parasitoid, tri-trophic interactions
Citation: Anderson RM, Dallar NM, Pirtel NL, Connors CJ, Mickley J, Bagchi R and Singer MS (2019) Bottom-Up and Top-Down Effects of Forest Fragmentation Differ Between Dietary Generalist and Specialist Caterpillars. Front. Ecol. Evol. 7:452. doi: 10.3389/fevo.2019.00452
Received: 28 February 2019; Accepted: 11 November 2019;
Published: 26 November 2019.
Edited by:
Lora A. Richards, University of Nevada, Reno, United StatesReviewed by:
Freerk Molleman, Adam Mickiewicz University, PolandJohn Thomas Lill, George Washington University, United States
Copyright © 2019 Anderson, Dallar, Pirtel, Connors, Mickley, Bagchi and Singer. This is an open-access article distributed under the terms of the Creative Commons Attribution License (CC BY). The use, distribution or reproduction in other forums is permitted, provided the original author(s) and the copyright owner(s) are credited and that the original publication in this journal is cited, in accordance with accepted academic practice. No use, distribution or reproduction is permitted which does not comply with these terms.
*Correspondence: Riley M. Anderson, rmanderson@wesleyan.edu