- 1NTNU University Museum, Norwegian University of Science and Technology, Trondheim, Norway
- 2Department of Biology, Dalhousie University, Halifax, NS, Canada
Despite that the study of individual repeatability is a common topic in behavioral ecology, virtually nothing is known about inter-annual variability in the marine migratory behavior of iteroparous salmonids that can complete multiple feeding migrations in their lifespan. Behavioral data from 38 anadromous brown trout (Salmo trutta), tracked by acoustic telemetry in 2–3 consecutive marine feeding migrations in two Norwegian fjord systems, were analyzed for intra-individual repeatability in key aspects of their marine migration. Individual brown trout displayed significant inter-annual consistency in marine area use and in the timing of marine exit (i.e. when they returned to spawning rivers), but not in the timing of marine entry or the time spent in the marine environment each year. Our study raises new questions about how anadromous brown trout respond to changing conditions and anthropogenic factors in the marine environment. Intra-individual repeatability of brown trout linked to changing environmental conditions should therefore be a focus for future studies.
Introduction
The post-spawning feeding migrations of iteroparous fish species have evolved to allow nutritionally depleted individuals the opportunity to exploit richer feeding habitats in an effort to recondition for future reproductive events. Needless to say, the mechanisms and patterns of migration can vary widely both within and among populations, as may the degree of individual flexibility and/or repeatability of migratory behavior in response to environmental fluctuations. Behavioral repeatability has been documented in various taxa (Bell et al., 2009), including species and populations of birds, mammals, and fish (e.g., Dias et al., 2010; Lea et al., 2015; Müller et al., 2015; Leclerc et al., 2016). For predatory fish feeding in the marine habitat, the availability, and distribution of resources in the marine environment can vary between years (Dragesund et al., 1997; Rikardsen and Amundsen, 2005), which should favor flexibility in traits like migration timing, distance, and duration of residency in various habitats if the organisms have reliable cues from the environment to adjust their behavior in response to the environmental changes (Reed et al., 2010).
The Salmonidae is a family of freshwater spawning fishes, where several of its species initiate feeding migrations to the marine environment (Pavlov and Savvaitova, 2008). Among these, brown trout Salmo trutta is a widely distributed, facultatively anadromous species known to display a continuum of migratory strategies ranging from freshwater residency and potamodromy to estuarine, short and long-distance marine migrations, both among and within populations (Cucherousset et al., 2005; Boel et al., 2014; del Villar-Guerra et al., 2014; Eldøy et al., 2015; Flaten et al., 2016; Bordeleau et al., 2018). As an iteroparous species, anadromous brown trout can undertake multiple annual marine feeding migrations during its lifetime (L'Abee-Lund et al., 1989; Thorstad et al., 2016), where the freshwater residency between marine migratory seasons is usually characterized by spawning and overwintering with opportunistic feeding (Davidsen et al., 2017) that have limited importance for somatic growth (Knutsen et al., 2001). While the drivers of the brown trout migratory continuum have remained somewhat mysterious, growing scientific evidence indicates a role of individual physiological and nutritional state, metabolic rate, and food availability (Olsson et al., 2006; Wysujack et al., 2009; Davidsen et al., 2014; Eldøy et al., 2015; Bordeleau et al., 2018). Despite high inter-individual variability in migratory behavior, the degree of intra-individual behavioral flexibility to changing environments and its consequences in terms of growth, survival, and ultimate fitness remain obscure. Beyond the role of environmental variability, the migratory behavior of anadromous brown trout can be influenced by anthropogenic impacts on coastal waterways, such as marine traffic, harbors and other near-shore infrastructure, renewable energy production, fisheries, and aquaculture (Thorstad et al., 2016; Aldvén and Davidsen, 2017). Importantly, recent work using acoustic telemetry has documented inter-annual shifts in the marine habitat use of different groups of anadromous brown trout in response to aquaculture-associated salmon lice abundances (Halttunen et al., 2018). However, due to logistical constraints imposed by battery life of acoustic transmitters, and relatively high mortality between spawning events (Fleming and Reynolds, 2004), no previous studies have yet assessed the inter-annual flexibility in the marine migrations of brown trout tracked through multiple years.
In order to investigate the degree of variation in behavior of brown trout individuals between consecutive marine feeding seasons, we extracted behavioral (movement) data from trout tagged in acoustic telemetry studies in two Norwegian fjord systems between 2012 and 2017 (e.g., Eldøy et al., 2015; Bordeleau et al., 2018). Studies of migratory species in various taxa have shown that individuals can exhibit both consistency and repeatability in behavior (Bell et al., 2009). Given the lack of previous studies on intra-individual repeatability in annual marine migratory behavior for salmonids, we chose not to make a priori predictions from specific hypotheses. Instead, we explored this unique dataset to investigate whether key behavioral aspects of the intra-individual marine behavior of anadromous brown trout was repeated among years. Specifically, we analyzed the degree of annual intra-individual behavioral repeatability in terms of (i) spatial dispersal, (ii) migratory timing, and (iii) duration of marine residency.
Materials and Methods
Study Area
The study was conducted in two fjord systems in central and northern parts of Norway (Figure 1). The Hemnfjord system consists of two interconnected fjords with more than 60 km2 surface area and about 65 km of shoreline and is connected to the open sea by a 36 km long strait (Figure 1, Eldøy et al., 2015). The Tosenfjord system consist of two interconnected fjords with about 150 km2 surface area and more than 270 km of shore line, connected to the open sea by a 15 km long strait (Figure 1). Several watercourses with partially anadromous populations of brown trout drain into both fjord systems. The Hemnfjord study area is described in detail by Eldøy et al. (2015, 2017) and Flaten et al. (2016), while the Tosenfjord study area is described by Bordeleau et al. (2018).
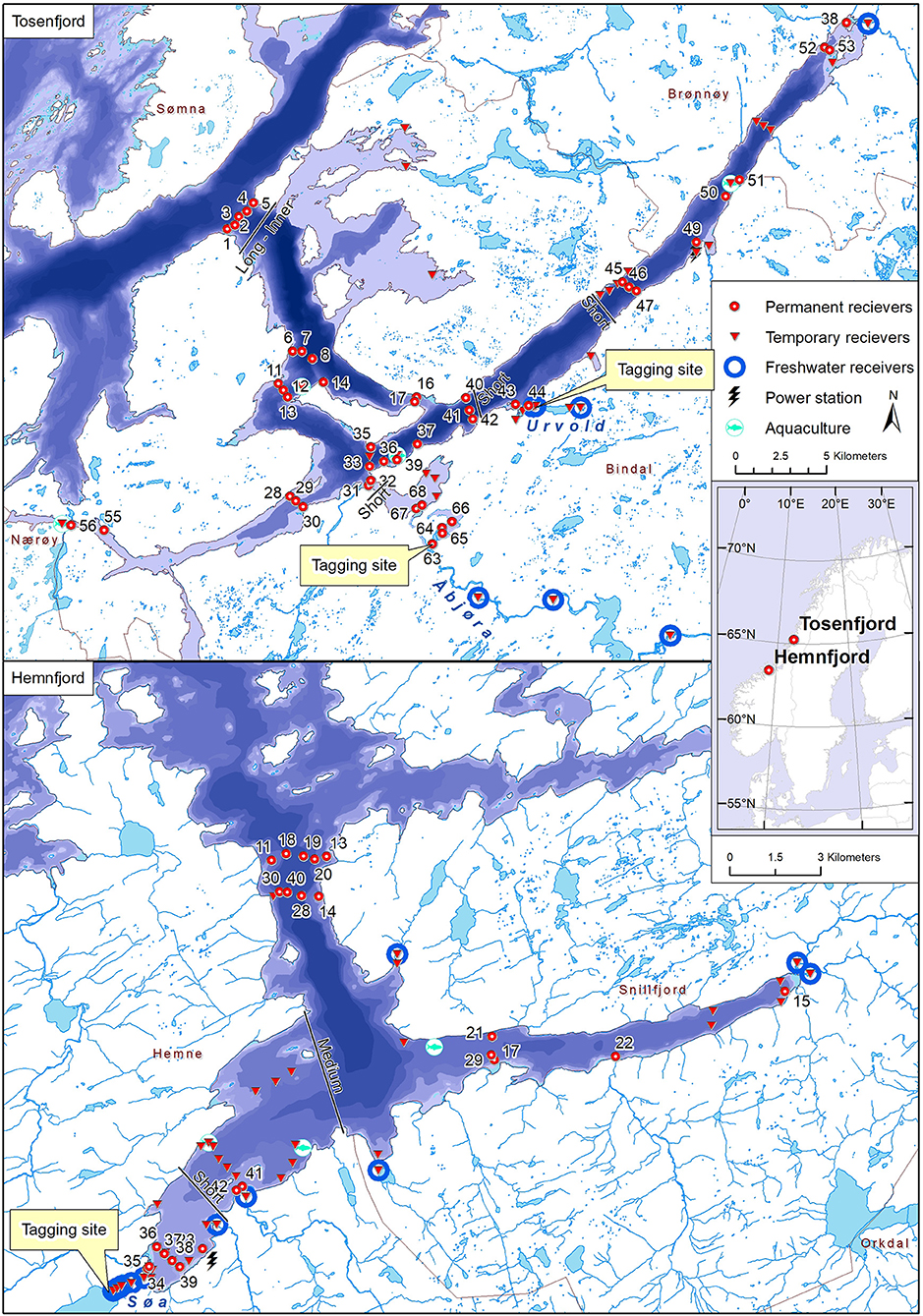
Figure 1. Map over study site Tosenfjord (upper) and Hemnfjord (lower), with site of tagging and locations of deployed receivers. Short-, medium-, and long-inner lines indicate the defined boundaries used to classify migratory behavior based on minimum travel distance. Two-digit numbers indicate receiver ID's included in the network analyses.
Environmental Variables
Both fjord systems had aquaculture facilities with farmed salmon in open net pens during the study periods. Sea temperature and salmon lice count data from the salmon farms was downloaded from the Norwegian Fish Health Database (www.barentswatch.no), and all available recordings from marine aquaculture locations in the two fjord systems were combined. Data on sea temperatures and salmon lice counts (here shown as counts of all life stages combined) in the farms located within each fjord system revealed seasonal and annual variations in both temperature (Figure 2) and salmon lice infestation levels (Figure 3).
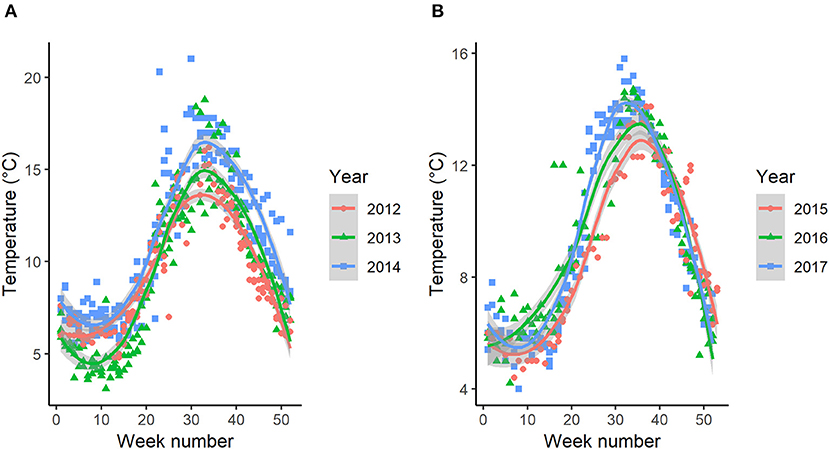
Figure 2. Sea temperatures reported by the salmon farms located within the study area in Hemnfjord (A) and Tosenfjord (B). Colored lines indicate the smoothed conditional mean and its pointwise 95% confidence interval (shaded bands).
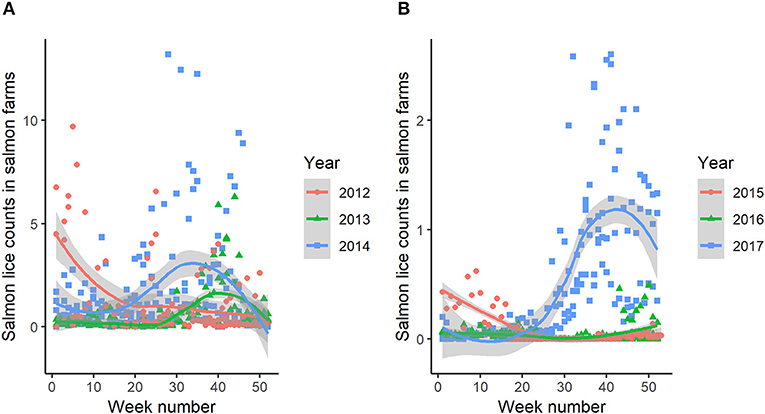
Figure 3. Salmon lice prevalence (all life stages combined) reported by the salmon farms located within the study area in Hemnfjord (A) and Tosenfjord (B). Colored lines indicate the smoothed conditional mean and its pointwise 95% confidence interval (shaded bands).
Acoustic Tracking
In the Søa watercourse in Hemnfjord, a total of 100 brown trout were tagged in freshwater or in the estuary and tracked with acoustic receiver arrays in the fjord system in 2012–2014 (Figure 1). In Tosenfjord, a total of 274 brown trout were tagged in freshwater and estuaries of River Åbjøra and Urvold watercourse and tracked with acoustic receiver arrays in the fjord during 2015–2017 (Figure 1). In general, anadromous brown trout in the two fjord systems migrate to sea each summer for feeding and return again to freshwater for spawning and/or overwintering during late summer (Eldøy et al., 2015; Bordeleau et al., 2018). The fish were either tagged during spring after spawning and prior to their marine migration, or in the autumn prior to potential spawning. For fish tagged during autumn, the tracking started at their outwards migration during the following spring. All fish included in this study were tagged following the same protocol. The fish were captured by rod fishing or gillnets that were continuously monitored and kept in holding nets for up to 4 h prior to tagging. The fish were sedated using 2-phenoxy ethanol for 4 min prior to making a 2 cm incision in the body cavity and inserting the sterilized acoustic tag. The incision was closed by 2–3 sutures, before the fish were placed in a recovery tank for up to 15 min and subsequently released at the site of tagging. The expected battery lifetime of the acoustic tags ranged from 15 to 24 months (Table 1). See Eldøy et al. (2015) and Bordeleau et al. (2018) for further details. Arrays of acoustic receivers (Vemco Inc., Canada models VR2, VR2W, and VR2-AR) were deployed at various locations in both freshwater, estuaries and saltwater in the two fjord systems to map the movements of the tagged brown trout (Figure 1). In Hemnfjord, receiver ID 34 and 35 were deployed in the estuary of River Søa and represented the transition zone between freshwater and saltwater. In Tosenfjord, receiver ID 44 was deployed in the estuary of River Urvold and receiver ID 63–68 were deployed in the estuarine parts of River Åbjøra. See Eldøy et al. (2015) and Bordeleau et al. (2018) for further details.
Prior to statistical analyses, the tracking data was filtered for false registrations resulting from noise in the surroundings of the receivers and/or code collision of simultaneously transmission from multiple transmitters (Pincock, 2012). Receivers found to contain frequently false detections were filtered by adding a filter that required at least two registrations within a 10-min time span to accept the registrations. The data was further visually inspected for false detections, and obviously false detections were removed prior to the statistical analyses. See Eldøy et al. (2015) and Bordeleau et al. (2018) for further details.
Data Analyses
All statistical analyses were conducted using R version 3.5.3 (R Core Team, 2019) and RStudio version 1.2.1335 (RStudio Team, 2019). Spearman's rank correlation was used to test the intra-individual correlation in migratory behavior between first and second year of tracking (Hanson et al., 2010; Taylor and Cooke, 2014; Nelson et al., 2015). Data on sea temperature and salmon lice prevalence were plotted using r-package ggplot2 (Wickham, 2016), using the “geom_smooth” function to produce smoothed trend lines. Marine migratory tactics were classified as short- medium- and long distance migration in Hemnfjord for each tracking season, based on how far out in the fjord system the fish was detected (Figure 1, Eldøy et al., 2015). Similarly, marine migratory tactics were defined as short, long-inner, and long-outer distance migrants based on migratory distance in Tosenfjord (Figure 1, Bordeleau et al., 2018), where fish remaining resident in the estuary of River Åbjøra were considered as short distance migrants. Only fish observed returning to freshwater the second season, being detected after 1 July in the second tracking, season or qualifying for the longest distance migratory tactic in the second tracking season were included in this analysis. The categorical variables of migration distance were transformed to ordinal structure according to relative migration distance (short = 1, medium/long-inner = 2, and long/long-outer = 3) prior to testing the intra-individual consistency using Spearman's correlation test.
Network analysis and bipartite graphs were made using the r-package igraph (Csardi and Nepusz, 2006), as previously demonstrated on acoustic telemetry data by Finn et al. (2014). Here, the individual's total yearly count of detections for each marine receiver that was operative through all the study years (numbered receivers in Figure 1) was used to compare the individual's marine area use among years. Only fish observed returning to freshwater the second season, being detected after 1 July in the second tracking season, or qualifying for the longest distance migratory tactic in the second tracking season were included in the network analysis. The number of detections was used as weights for the link (edges) between tracked fish and associated receivers. Receivers and tracked fish were grouped using the igraph “cluster_walktrap” function (using 6 steps for Hemnfjord and 11 steps for Tosenfjord), which uses a random-walk algorithm to try to find densely connected subgraphs (communities) within the network (Csardi and Nepusz, 2006; Finn et al., 2014). The results of the grouping within the network of each fjord system were organized as an ordinal variable according to distance in the network plot and geographic location of the receivers (Figure 1) prior to evaluating inter-individual consistency among years using the Spearman's correlation test. Timing of marine entry was defined as the time of the first detection on a receiver deployed in estuarine or marine waters preceding detection on a receiver deployed in freshwater. Timing of marine exit was defined as the time of the last detection on a receiver deployed in estuarine or marine waters prior to detection on a receiver deployed in freshwater. An exception was made for river Åbjøra, where the two outermost receivers in the river mouth (station 67 and 68, Figure 1) were defined as estuarine and receivers deployed further upstream in the large parts of the watercourse influenced by tidal water (station 63–66, Figure 1) were defined as “freshwater” in the marine timing and duration analyses. Marine duration was calculated as the yearly accumulated time spent in the marine environment, where periods of freshwater residency between the first marine entry and the last marine exit were excluded. Individuals that were only residing in tidally influenced parts of river Åbjøra were excluded from analyses of timing and duration of marine migration.
Results
Of the 374 tagged individuals from the two fjord systems, we could extract data from 36 individuals (Table 1) to explore the intra-individual repeatability in area use, timing of marine entry, timing of marine exit, and/or marine residence time among consecutive marine feeding seasons. Of these, 10 individuals from Hemnfjord and eight individuals from Tosenfjord were tracked throughout two full marine seasons (i.e., they migrated back to freshwater after the second feeding migration). For two individuals from Tosenfjorden, tracking data could be extracted from three consecutive marine feeding migrations. Generally, the tagged individuals displayed a relative consistent marine behavior on the evaluated aspects of their marine behavior between the two or three consecutive marine feeding seasons of tracking (Table 2). However, the degree of consistency varied among individuals, with some individuals displaying large variations in some of the measured behavioral aspects among years (Table 2).
Marine Area Use of Tagged Anadromous Brown Trout
A wide range maximum migratory distances were observed, ranging from remaining resident in the estuarine areas of the watercourse where the fish were tagged—to utilizing large parts of the fjord system and spending a significant amount of their total marine residence time in areas outside the outermost receiver arrays. There was a strong and significant intra-individual correlation between the observed migratory tactic during the first and second year of tracking in both Hemnfjord (Spearman's rank-correlation; rs = 0.84, n = 12, P < 0.001) and Tosenfjord (rs = 0.96, n = 18, P < 0.001) when marine migratory behavior was classified as defined by Eldøy et al. (2015) and Bordeleau et al. (2018) for Tosenfjord (Figure 1). For Hemnfjord, 9 of 12 tracked fish were assigned to the same migratory tactic in both marine seasons. For Tosenfjord, 16 of 18 individuals were assigned to the same migratory tactic in both years. The annual consistency in marine area use of tagged individuals during the 2 consecutive years of tracking was further investigated by network analyses (Figures 4, 5; Table 2). There was a strong and significant intra-individual correlation between the assigned network group during the first and second year of tracking in both Hemnfjord (Spearman's rank-correlation; rs = 0.77, n = 12, P = 0.003) and Tosenfjord (rs = 0.89, n = 18, P < 0.001). The grouping analysis of network structure using a cluster walk trap algorithm on the network in Hemnfjorden resulted in two different groups; one containing receiver locations in the inner and central part of Hemnfjorden and one containing receiver locations in outer and eastern parts of the fjord system (Figure 4). In this fjord system, 11 of 12 of the tagged fish were assigned to the same community unit both years (Table 2). For Tosenfjord, the grouping analysis of network structure using a cluster walk trap algorithm resulted in seven different community with associated tagged fish and receiver locations (Figure 5). Here, 12 of 18 tagged fish that were followed for 2 years were assigned to the same community both years (Table 2). One of the two fish that was tracked and analyzed for three consecutive seasons was assigned to the same community all years. However, two of the fish tracked during two seasons, and the one fish tracked for three seasons that changed community, transitioned between communities that all had associated receivers in the estuarine areas of the Åbjøra watercourse.
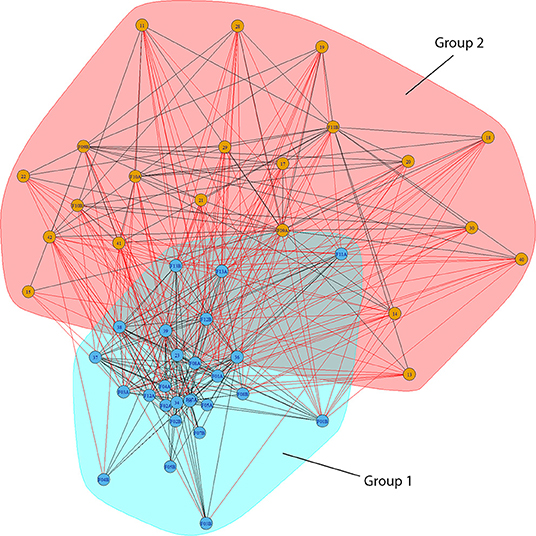
Figure 4. Bipartite graph of network analysis from Hemnfjord tracking data including marine receivers (two-digit numbers) that were operative from 2012 to 2014 and tagged fish tracked for two consecutive seasons (two-digit number with F as prefix and ending with A for first year of tracking, ending with B for second year of tracking). Lines indicate connections (edges) between receivers and the detected fish. Color indicate the different subgroups (communities) of receivers and associated tracked fish assigned by a random-walk-algorithm.
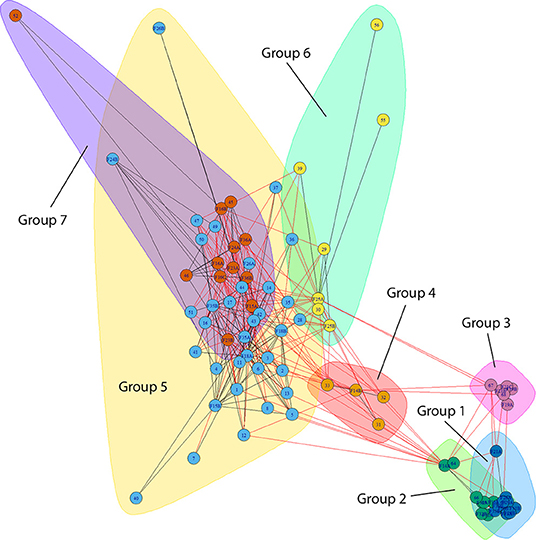
Figure 5. Bipartite graph of network analysis from Tosenfjord tracking data including marine receivers (two-digit numbers) that were operative from 2015 to 2017 tagged fish (two-digit number with F as prefix and ending with A for first year of tracking, ending with B for second year of tracking and C for third year of tracking). Lines indicate connections (edges) between receivers and the detected fish. Color indicate the different subgroups (communities) of receivers and associated tracked fish assigned by a random-walk-algorithm.
Timing of Start and End of Marine Feeding Migration
Average day of year for marine entry was 123.8 (n = 27, SD = 15.8, range 103.0–151.0) for the first year of tracking and 120.2 (n = 27, SD = 24.0, range 64.4–155.7) for the second year of tracking. There was a weak and non-significant intra-individual correlation of the timing of marine entry between the first and second year of tracking (Figure 6, Spearman rank-correlation; rs = 0.32, n = 27, P = 0.10). Average individual difference in the timing of sea entry between the consecutive years was 18.0 days (n = 27, SD = 15.0 days, range 0.2–50.6 days).
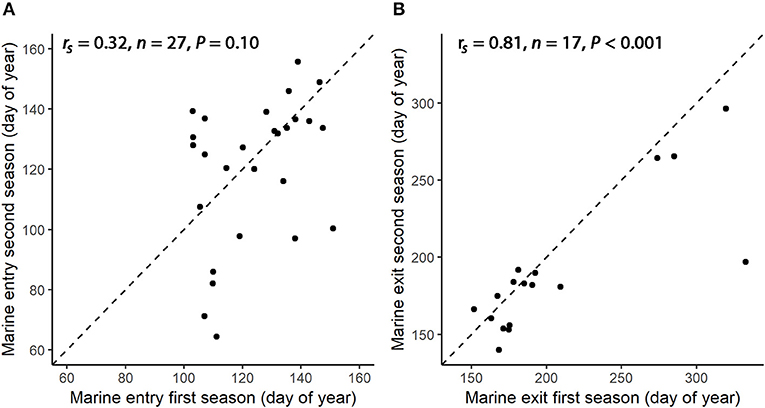
Figure 6. Timing (day of year) of tagged individual's marine entry (A) and marine exit (B) in first year of tracking (x-axis) and second year of tracking (y-axis). Stippled line indicates the 1:1 line. Summary statistics (rs and P-values) are calculated by Spearman's correlation test.
Average day of year for marine exit was 207.1 (n = 17, SD = 57.5, range 151.8–332.8) for the first year of tracking and 190.5 (n = 17, SD = 43.9, range 140.0–296.4) for the second year of tracking. There was a strong and significant intra-individual correlation of marine exit timing between the first and second year of tracking (Figure 6, Spearman rank-correlation; rs = 0.81, n = 17, P < 0.001). Average difference in the timing of exit between the consecutive years was 21.1 days (n = 17, SD = 30.8 days, range 2.1–135.8 days).
Migratory Duration
Average marine residency was 91.6 days (n = 18, SD = 66.4, range 30.3–262.8) for the first year of tracking and 74.2 (n = 18, SD = 56.7, range 13.1–263.4) for the second year of tracking. There was no significant correlation of marine residence time between the first and second year of tracking (Figure 7, Spearman's rank-correlation; rs = 0.31, n = 18, P = 0.20). The difference in the duration of the marine migration between the two seasons varied greatly among the individuals, ranging from 0.7 days to 152.6 days (n = 18, mean = 30.3 days, SD = 44.4 days).
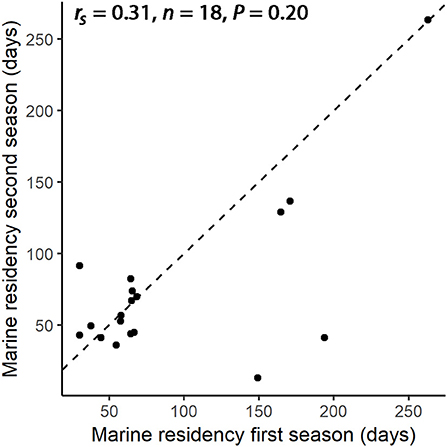
Figure 7. Duration of tagged individual marine residence (days) during first (x-axis) and second (y-axis) year of tracking. Stippled line indicates the 1:1 line. Summary statistics (rs and P-values) are calculated by Spearman's correlation test.
Discussion
This study revealed that some key aspects of the annual marine feeding migration of anadromous brown trout tend to be repeatable between years. Repeatable behavior is a common phenomenon in nature, but this is to our knowledge the first study to illustrate repeatable behavior by anadromous brown trout, and is among the few to evaluate behavioral repeatability in salmonid fishes more generally (Taylor and Cooke, 2014). Although large phenotypic and behavioral variability has been observed among brown trout in previous studies (Klemetsen et al., 2003; Thorstad et al., 2016; Halttunen et al., 2018), and previous studies suggest pre-migratory nutritional state as a driver for the migratory continuum of brown trout (Bordeleau et al., 2018), this study suggests that the intra-individual behavioral flexibility during the marine migration is low. However, despite the general repeatability of marine migration behavior, the degree of repeatability varied greatly among individuals, with some individuals displaying large intra-individual variance among the 2 years of tracking.
Variation in behavioral traits among years can be divided into an individual effect and a residual effect, where the individual effect is thought to be determined by genetics and previous experiences (Bell et al., 2009; Biro and Stamps, 2010; Conrad et al., 2011). This study was not designed in a way that allowed us to evaluate the importance of environmental experience to the observed trends of behavioral repeatability. Due to low number of tracking years (only 2 years for most individuals, Table 2), low number of different environments, and the relatively low sample size, it was not possible to evaluate how yearly variation in environmental factors affected the behavior of anadromous brown trout. Sea temperature and salmon lice abundances reported by salmon farms in the two fjord systems suggest some degree of environmental fluctuation throughout the season and among years, and previous studies have in fact reported that the abundance of certain prey in Norwegian fjords can vary greatly among years (Dragesund et al., 1997; Rikardsen and Amundsen, 2005). It is therefore likely that the anadromous brown trout in our study experienced somewhat varying conditions interannually during their marine migrations, despite the observed individual consistency in their marine behavior. Alternatively, the observed differences in environmental conditions experienced by the tagged fish might not have been drastic enough to trigger intra-individual changes in marine habitat use patterns.
Inter-annual variation in individual marine area use was evaluated with two different approaches; subjectively defined lines by the distance from watercourse of tagging, and by performing network analyses to investigate the relationship between every tagged fish and receivers deployed in the fjord and grouping them by using a random-walk-algorithm. Using both methods, the fish from both fjord systems showed a strong and significant individual consistency in marine area use between the two tracking seasons. Previous studies have revealed that the area use of individuals in anadromous brown trout populations can vary greatly (Thorstad et al., 2016). Migratory tactics within brown trout populations have previously been linked with nutritional status in spring prior to seasonal marine feeding migrations, where fish in low body condition Eldøy et al. (2015) and low nutritional physiological state Bordeleau et al. (2018) were more likely to migrate further out in the fjord system. Bordeleau et al. (2018) suggested that individuals with poor body condition in spring may be more prone toward feeding in the distant, outer areas, where potentially better feeding conditions occur, in order to regain their energy reserves. The observed consistency in marine area use raises questions about whether there could be causal factors that act over longer time-frames, which might cause individual pre-migratory nutritional status during spring to be maintained across years. For example, it could be speculated that marine habitat or prey preferences have the potential to affect energy storage, energy investment into reproduction, and post-spawning nutritional state prior to the next feeding season (Bordeleau, 2019). However, the links between marine migratory behavior and prey choice, growth, reconditioning of body condition and subsequent spawning investment is poorly understood.
The individual migratory behavior of the tagged individuals was relative consistent among years despite some observed yearly variation in sea water temperature and salmon lice prevalence in salmon farms. Previous studies have linked both horizontal and vertical marine responses of anadromous brown trout to variation in seawater temperature (Rikardsen et al., 2007; Jensen et al., 2014; Eldøy et al., 2017; Kristensen et al., 2018). It has been thoroughly documented that open cage salmon farming can lead to the unnaturally high infestation of wild salmonids and alter their marine behavior (Thorstad et al., 2015; Finstad et al., 2017). Halttunen et al. (2018) documented shifts in the marine area use of different groups of anadromous brown trout in response to salmon lice abundance in Hardangerfjord, Southern Norway, and observed that brown trout utilized outer areas less in years when the risk of salmon lice infestation was high, compared with years with lower infestation risk. However, the variation in salmon lice infestation levels in the study by Halttunen et al. (2018) was probably greater than in our study, as they investigated the behavior of brown trout in years when salmon production cycles were active vs. the behavior of brown trout in years when all salmon farms in the inner fjord were fallow. There was a marginally non-significant, intra-individual correlation in timing of marine entry between the 2 years of tracking. Previous studies have documented that previous life history, morphology and physiology affect the timing of seaward migrations in salmonid populations (Halttunen et al., 2013; Thorstad et al., 2016), and so intra-individual consistency in timing of marine entry is thus expected. However, environmental conditions such as the timing of ice melting and increased water temperature and discharge has also been found to influence the timing of migration (Thorstad et al., 2016). Inter-annual variation in environmental conditions in the freshwater habitat is therefore likely to have influenced the annual timing of marine entry in the present study.
In contrast to timing of marine entry, a strong and significant intra-individual correlation was found for the timing of marine exit between the 2 years of tracking. This suggests that life history, physiological state and/or individual effects have some influence on the timing of when individuals end their marine feeding season. Marine exit was in the present study defined as the last detection at a marine or estuarine receiver prior to detection at a receiver in freshwater. Because marine exit timing varied so little between years, brown trout were probably able to migrate into freshwater under most water discharge conditions, and so inter-annual environmental conditions in the rivers probably had little influence on the timing of their movement into freshwater. More likely, the timing of marine exit and freshwater entry was probably more influenced by life history, stage of maturity, and sex, as previously shown (Thorstad et al., 2016). Regarding the inter-annual marine residence time of brown trout, we found no significant intra-individual relationship. If the timing of marine entry is mainly influenced by the environmental conditions in the freshwater habitat, and there is strong consistency in marine exit timing, the varying environmental conditions in freshwater prior to marine entry are likely the main determinants of the marine residence time of brown trout. Alternatively, marine residence time in brown trout has been inversely correlated with individuals nutritional state (i.e., plasma triglycerides) prior to migration, such that depleted fish spend more time at sea reconditioning (Bordeleau et al., 2018).
In summary, this study revealed a strong tendency for individual, inter-annual repeatability in anadromous brown trout with respect to migratory decisions and marine habitat use patterns. While the causes remain obscure, this is the first study assessing the intra-individual behavioral repeatability of a salmonid fish species in relation to aspects of their spatiotemporal marine habitat use during consecutive annual feeding migrations. The findings of this study may have strong potential implications for management purposes. The role of intra-individual repeatability and the inter-annual behavioral response of anadromous brown trout to changing environmental conditions should therefore be a focus for future studies.
Data Availability Statement
The datasets generated for this study are available on request to the corresponding author.
Ethics Statement
The experimental procedures followed national ethical requirements and were approved by the Norwegian National Animal Research Authority (permit No. 2012/22965 & 2015/8518).
Author Contributions
SE was as the lead author responsible for the main part of the data analyses and on writing the paper. SE has further contributed considerable in field work and obtaining the dataset. XB was central in the initial idea of the paper and contributed significantly in terms of study design and contributed on feedback and input in the process of writing the paper. XB has further contributed in field work in the Tosenfjord study site and have during his work in this fjord system also contributed in the analyses these data. GC has contributed significantly in planning, study design, and the writing of the paper. JD was the PI of the two tracking studies that this paper is based on and has through this role significantly contributed to study design, field work, and project management. Further, he has contributed to this specific study with planning, study design, and writing of the paper.
Funding
This study was part of the CHASES project funded by the Research Council of Norway (ref: 255110/E50). The tracking study in Tosenfjord was financed or supported by contributions from Sinkaberg-Hansen AS, the County Governor of Nordland, Nordland County Authority, The Norwegian Environment Agency, The river Åbjøra landowners' association, Phlates Eiendommer, The Natural Sciences and Engineering Research Council of Canada, Ocean Tracking Network, and the NTNU University Museum. The tracking study in Hemnfjord was financed or supported by contributions from the Hemne municipality, the County Governor of Sør-Trøndelag, Sør-Trøndelag County Authority, The Norwegian Environment Agency, AquaGen AS, the Norwegian institute for Nature Research, the Lake Rovatnet landowner's association, TrønderEnergi AS, DTU aqua, UiT the Arctic University of Norway, Ocean Tracking Network, and the NTNU University Museum.
Conflict of Interest
The authors declare that the research was conducted in the absence of any commercial or financial relationships that could be construed as a potential conflict of interest.
The handling editor and reviewer, NS, declared their involvement as co-editors in the Research Topic, and confirm the absence of any other collaboration.
Acknowledgments
The crew of RV Gunnerus, Lars Rønning, Jan Ivar Koksvik, Aslak Darre Sjursen, Martin Georg Hansen, Ola Magne Taftø, Hans Erlandsen, Vegard Pedersen Sollien, Paul Skarsvåg, Stein Hugo Hemmingsen, Kristian Lian, Embla Østebrøt, Hilde Dørum, Kristina Johansen and Ashley Ann, Ole Johan Hornenes, Torjus Haukvik, and Charlotte Hallerud are all thanked for their extensive help during fieldwork. The experimental procedures were approved by the Norwegian National Animal Research Authority (permission number 2012/22965 & 2015/8518).
References
Aldvén, D., and Davidsen, J. (2017). “Marine migrations of sea trout (Salmo trutta),” in Sea Trout: Science & Management: Proceedings of the 2nd International Sea Trout Symposium, ed G. Harris (Leicester: Troubador Publishing Ltd., 288–297.
Bell, A. M., Hankison, S. J., and Laskowski, K. L. (2009). The repeatability of behaviour: a meta-analysis. Anim. Behav. 77, 771–783. doi: 10.1016/j.anbehav.2008.12.022
Biro, P. A., and Stamps, J. A. (2010). Do consistent individual differences in metabolic rate promote consistent individual differences in behavior? Trends Ecol. Evol. 25, 653–659. doi: 10.1016/j.tree.2010.08.003
Boel, M., Aarestrup, K., Baktoft, H., Larsen, T., Sondergaard Madsen, S., Malte, H., et al. (2014). The physiological basis of the migration continuum in brown trout (Salmo trutta). Physiol. Biochem. Zool. 87, 334–345. doi: 10.1086/674869
Bordeleau, X. (2019). The post-spawning ecology of iteroparous salmonids: basis of variability in migratory behaviour and survival, ecological importance and conservation implications (Ph.D. thesis). Department of Biology, Dalhousie University, Halifax, Canada.
Bordeleau, X., Davidsen, J. G., Eldøy, S. H., Sjursen, A. D., Whoriskey, F. G., and Crossin, G. T. (2018). Nutritional correlates of spatiotemporal variations in the marine habitat use of brown trout (Salmo trutta) veteran migrants. Can. J. Fish. Aquatic Sci. 75, 1–11. doi: 10.1139/cjfas-2017-0350
Conrad, J. L., Weinersmith, K. L., Brodin, T., Saltz, J., and Sih, A. (2011). Behavioural syndromes in fishes: a review with implications for ecology and fisheries management. J. Fish Biol. 78, 395–435. doi: 10.1111/j.1095-8649.2010.02874.x
Csardi, G., and Nepusz, T. J. I. (2006). The igraph software package for complex network research. Int. J. Complex Systems 1695, 1–9. Available online at: https://igraph.org (accessed April 04, 2019).
Cucherousset, J., Ombredane, D., Charles, K., Marchand, F., and Baglinière, J.-L. (2005). A continuum of life history tactics in a brown trout (Salmo trutta) population. Can. J. Fish. Aquatic Sci. 62, 1600–1610. doi: 10.1139/f05-057
Davidsen, J. G., Daverdin, M., Sjursen, A. D., Rønning, L., Arnekleiv, J. V., and Koksvik, J. I. (2014). Does reduced feeding prior to release improve the marine migration of hatchery brown trout Salmo trutta smolts? J. Fish Biol. 85, 1992–2002. doi: 10.1111/jfb.12485
Davidsen, J. G., Knudsen, R., Power, M., Sjursen, A. D., Rønning, L., Hårsaker, K., et al. (2017). Trophic niche similarity among sea trout Salmo trutta in central Norway investigated using different time-integrated trophic tracers. Aquat. Biol. 26, 217–227. doi: 10.3354/ab00689
del Villar-Guerra, D., Aarestrup, K., Skov, C., and Koed, A. (2014). Marine migrations in anadromous brown trout (Salmo trutta). Fjord residency as a possible alternative in the continuum of migration to the open sea. Ecol. Freshw. Fish 23, 594–603. doi: 10.1111/eff.12110
Dias, M. P., Granadeiro, J. P., Phillips, R. A., Alonso, H., and Catry, P. (2010). Breaking the routine: individual Cory's shearwaters shift winter destinations between hemispheres and across ocean basins. Proc. R. Soc. B Biol. Sci. 278, 1786–1793. doi: 10.1098/rspb.2010.2114
Dragesund, O., Johannessen, A., and Ulltang, Ø. (1997). Variation in migration and abundance of norwegian spring spawning herring (Clupea harengus L.). Sarsia 82, 97–105. doi: 10.1080/00364827.1997.10413643
Eldøy, S. H., Davidsen, J. G., Thorstad, E. B., Whoriskey, F., Aarestrup, K., Næsje, T. F., et al. (2015). Marine migration and habitat use of anadromous brown trout Salmo trutta. Can. J. Fish. Aquatic Sci. 72, 1366–1378. doi: 10.1139/cjfas-2014-0560
Eldøy, S. H., Davidsen, J. G., Thorstad, E. B., Whoriskey, F. G., Aarestrup, K., Næsje, T. F., et al. (2017). Marine depth use of sea trout Salmo trutta in fjord areas of central Norway. J. Fish Biol. 91, 1268–1283. doi: 10.1111/jfb.13463
Finn, J. T., Brownscombe, J. W., Haak, C. R., Cooke, S. J., Cormier, R., Gagne, T., et al. (2014). Applying network methods to acoustic telemetry data: modeling the movements of tropical marine fishes. Ecol. Modell. 293, 139–149. doi: 10.1016/j.ecolmodel.2013.12.014
Finstad, B., Thorstad, E. B., Falkegård, M., Fiske, P., Forseth, T., Mo, T. A., et al. (2017). The major threats to Atlantic salmon in Norway. ICES J. Marine Sci. 74, 1496–1513. doi: 10.1093/icesjms/fsx020
Flaten, A. C., Davidsen, J. G., Thorstad, E. B., Whoriskey, F., Rønning, L., Sjursen, A. D., et al. (2016). The first months at sea: marine migration and habitat use of sea trout Salmo trutta post-smolts. J. Fish Biol. 89, 1624–1640. doi: 10.1111/jfb.13065
Fleming, I. A., and Reynolds, J. D. (2004). “Salmonid breeding systems,” in Evolution Illuminated: Salmon and Their Relatives, eds A. Hendry and S. Stearns (Oxford: Oxford University Press, 264–294.
Halttunen, E., Gjelland, K. O., Hamel, S., Serra-Llinares, R. M., Nilsen, R., Arechavala-Lopez, P., et al. (2018). Sea trout adapt their migratory behaviour in response to high salmon lice concentrations. J. Fish Dis. 41, 953–967. doi: 10.1111/jfd.12749
Halttunen, E., Jensen, J. L. A., Næsje, T. F., Davidsen, J. G., Thorstad, E. B., Chittenden, C. M., et al. (2013). State-dependent migratory timing of postspawned Atlantic salmon (Salmo salar). Can. J. Fish. Aquatic Sci. 70, 1063–1071. doi: 10.1139/cjfas-2012-0525
Hanson, K. C., Hasler, C. T., Donaldson, M. R., and Cooke, S. J. (2010). Stability of swimming performance and activity hierarchies among wild largemouth bass at multiple temporal scales: evidence for context-dependent shuffling between seasons. Can. J. Zool. 88, 324–333. doi: 10.1139/Z10-006
Jensen, J. L. A., Rikardsen, A. H., Thorstad, E. B., Suhr, A. H., Davidsen, J. G., and Primicerio, R. (2014). Water temperatures influence the marine area use of Salvelinus alpinus and Salmo trutta. J. Fish Biol. 84, 1640–1653. doi: 10.1111/jfb.12366
Klemetsen, A., Amundsen, P.-A., Dempson, J. B., Jonsson, B., Jonsson, N., O'Connell, M. F., et al. (2003). Atlantic salmon Salmo salar L., brown trout Salmo trutta L., and Arctic charr Salvelinus alpinus (L.): a review of aspects of their life histories. Ecol. Freshw. Fish 12, 1–59. doi: 10.1034/j.1600-0633.2003.00010.x
Knutsen, J. A., Knutsen, H., Gjøsæter, J., and Jonsson, B. (2001). Food of anadromous brown trout at sea. J. Fish Biol. 59, 533–543. doi: 10.1111/j.1095-8649.2001.tb02359.x
Kristensen, M. L., Righton, D., del Villar-Guerra, D., Baktoft, H., and Aarestrup, K. (2018). Temperature and depth preferences of adult sea trout Salmo trutta during the marine migration phase. Mar. Ecol. Prog. Ser. 599, 209–224. doi: 10.3354/meps12618
L'Abee-Lund, J. H., Jonsson, B., Jensen, A. J., Saettem, L. M., Heggberget, T. G., Johnsen, B. O., et al. (1989). Latitudinal variation in life-history characteristics of sea-run migrant brown trout Salmo trutta. J. Anim. Ecol. 58, 525–542. doi: 10.2307/4846
Lea, J. S. E., Wetherbee, B. M., Queiroz, N., Burnie, N., Aming, C., Sousa, L. L., et al. (2015). Repeated, long-distance migrations by a philopatric predator targeting highly contrasting ecosystems. Sci. Rep. 5:11202. doi: 10.1038/srep11202
Leclerc, M., Vander Wal, E., Zedrosser, A., Swenson, J. E., Kindberg, J., and Pelletier, F. J. O. (2016). Quantifying consistent individual differences in habitat selection. Oecologia 180, 697–705. doi: 10.1007/s00442-015-3500-6
Müller, M. S., Massa, B., Phillips, R. A., and Dell'omo, G. (2015). Individual consistency and sex differences in migration strategies of Scopoli's shearwaters Calonectris diomedea despite year differences. Curr. Zool. 60, 631–641. doi: 10.1093/czoolo/60.5.631
Nelson, J. A., Atzori, F., and Gastrich, K. R. (2015). Repeatability and phenotypic plasticity of fish swimming performance across a gradient of urbanization. Environ. Biol. Fishes 98, 1431–1447. doi: 10.1007/s10641-014-0369-x
Olsson, I. C., Greenberg, L. A., Bergman, E., and Wysujack, K. (2006). Environmentally induced migration: the importance of food. Ecol. Lett. 9, 645–651. doi: 10.1111/j.1461-0248.2006.00909.x
Pavlov, D. S., and Savvaitova, K. A. (2008). On the problem of ratio of anadromy and residence in salmonids (Salmonidae). J. Ichthyol. 48, 778–791. doi: 10.1134/S0032945208090099
Pincock, D. G. (2012). False Detections: What They Are and How to Remove Them From Detection Data. AMIRIX Systems Inc., Hali-fax, NS, 1–11. DOC-004691-03.
R Core Team (2019). R: A Language and Environment for Statistical Computing. R Foundation for Statistical Computing, Vienna. Availble online at: http://www.R-project.org/
Reed, T. E., Waples, R. S., Schindler, D. E., Hard, J. J., and Kinnison, M. T. (2010). Phenotypic plasticity and population viability: the importance of environmental predictability. Proc. R. Soc. B Biol. Sci. 277, 3391–3400. doi: 10.1098/rspb.2010.0771
Rikardsen, A. H., and Amundsen, P.-A. (2005). Pelagic marine feeding of Arctic charr and sea trout. J. Fish Biol. 66, 1163–1166. doi: 10.1111/j.0022-1112.2005.00655.x
Rikardsen, A. H., Diserud, O. H., Elliott, J. M., Dempson, J. B., Sturlaugsson, J., and Jensen, A. J. (2007). The marine temperature and depth preferences of Arctic charr and sea trout, as recorded by data storage tags. Fish. Oceanogr. 16, 436–447. doi: 10.1111/j.1365-2419.2007.00445.x
RStudio Team (2019). RStudio: Integrated Development for R. RStudio, Inc., Boston, MA. Available online at: http://www.rstudio.com/
Taylor, M. K., and Cooke, S. J. (2014). Repeatability of movement behaviour in a wild salmonid revealed by telemetry. J. Fish Biol. 84, 1240–1246. doi: 10.1111/jfb.12334
Thorstad, E. B., Todd, C. D., Uglem, I., Bjørn, P. A., Gargan, P. G., Vollset, K. W., et al. (2015). Effects of salmon lice Lepeophtheirus salmonis on wild sea trout Salmo trutta—a literature review. Aquacult. Environ. Interact. 7, 91–113. doi: 10.3354/aei00142
Thorstad, E. B., Todd, C. D., Uglem, I., Bjørn, P. A., Gargan, P. G., Vollset, K. W., et al. (2016). Marine life of the sea trout. Marine Biol. 163:47. doi: 10.1007/s00227-016-2820-3
Keywords: behavioral repeatability, habitat use, marine migration, migratory timing, Salmo trutta, sea trout, migratory continuum
Citation: Eldøy SH, Bordeleau X, Crossin GT and Davidsen JG (2019) Individual Repeatability in Marine Migratory Behavior: A Multi-Population Assessment of Anadromous Brown Trout Tracked Through Consecutive Feeding Migrations. Front. Ecol. Evol. 7:420. doi: 10.3389/fevo.2019.00420
Received: 01 May 2019; Accepted: 18 October 2019;
Published: 01 November 2019.
Edited by:
Yolanda E. Morbey, University of Western Ontario, CanadaReviewed by:
Thomas Reed, University College Cork, IrelandNathan R. Senner, University of South Carolina, United States
Copyright © 2019 Eldøy, Bordeleau, Crossin and Davidsen. This is an open-access article distributed under the terms of the Creative Commons Attribution License (CC BY). The use, distribution or reproduction in other forums is permitted, provided the original author(s) and the copyright owner(s) are credited and that the original publication in this journal is cited, in accordance with accepted academic practice. No use, distribution or reproduction is permitted which does not comply with these terms.
*Correspondence: Sindre H. Eldøy, c2luZHJlLmVsZG95JiN4MDAwNDA7bnRudS5ubw==