- 1School of Natural Resources and Environment, University of Florida, Gainesville, FL, United States
- 2Institute for the Environment and Sustainability, Miami University, Oxford, OH, United States
- 3Conservation Department, Jacksonville Zoo and Gardens, Jacksonville, FL, United States
- 4Department of Wildlife Ecology and Conservation, University of Florida, Gainesville, FL, United States
- 5Frankfurt Zoological Society, National Park, Georgetown, Guyana
- 6Protected Areas Commission, National Park, Georgetown, Guyana
- 7Caiman House Research Station, Yupukari Village, Guyana
- 8South Rupununi Conservation Society, Wichabai Ranch, Guyana
- 9Lovett E. Williams Jr. Wildlife Research Laboratory, Fish and Wildlife Research Institute, Florida Fish and Wildlife Conservation Commission, Gainesville, FL, United States
Unsustainable harvest is driving population declines in tropical forest species across the globe. Despite maintaining the second highest percent forest cover in the world (85%), concern is increasing in Guyana that unmanaged commercial and subsistence hunting activities could result in defaunation, and the cascading ecological effects of “empty forests.” The Rupununi region of southwestern Guyana, home to the Kanuku Mountains Protected Area (KMPA), hosts one of the world's lowest human population densities (0.42 people/km2), as well as large, intact tracts of both Neotropical savanna and forest habitats, making it one of the country's most biodiverse regions. Indigenous Makushi and Wapichan communities that reside there have maintained subsistence lifestyles mediated by traditional beliefs and management practices for millennia. However, as human populations and access to markets increase, there is a corresponding increase in the harvest of natural resources. Protected areas have long been recognized for their role in biodiversity conservation, while also serving as a reserve for subsistence hunters. The KMPA, one of Guyana's newest protected areas, allows for the continued sustainable use of its resources by indigenous communities. It is critical to understand the patterns, impacts, and levels of hunting that are sustainable in and around the protected area so that biodiversity can be managed and conserved effectively. Our study shows that the impact of current hunting intensity in and around the KMPA remains relatively low and supports the hypothesis that Neotropical forests can support hunting pressure of <1 person/km2. While our results show that current levels of hunting in the region can be considered sustainable, small shifts in activity patterns and distribution of preferred game species were observed in sites subject to higher hunting intensity, which in turn appears to have had cascading effects on non-hunted species. Our results serve as a caution for the Kanuku Mountains region and an indication of the truly low levels of harvest that some species can sustain before populations begin to show declines. Further, we suggest a system-level approach to monitoring that incorporates both preferred game and non-hunted species, as well as indigenous knowledge of patterns of use and trends in populations of game species. This approach to monitoring would serve as an effective early warning system, allowing communities, managers, and policy makers to intervene before animal populations are significantly impacted by overhunting.
Introduction
Hunting intensity has reached unsustainable levels across much of the tropics, representing the most pressing threat to game mammal and bird populations after habitat loss (Redford, 1992; Fa and Peres, 2001; Nasi et al., 2011; Wilkie et al., 2011; Maxwell et al., 2016; Ripple et al., 2016; Young et al., 2016). Studies from across the global tropics assessing game mammal and bird species abundances under moderate and heavy hunting intensities have shown declines by an average of 83 and 58%, respectively (Wilkie et al., 2011). Hunting of long-lived, large-bodied species is only considered sustainable under low intensity harvest regimes—when exploitation is ≤ 20% of production (Fa et al., 2002). For tropical forest species, establishing sustainable harvest regimes has become increasingly important to ensure the long-term survival of hunted species, while at the same time maintaining a reliable, low-cost source of protein for subsistence-based communities. Determining the levels of harvest that are sustainable for tropical forest species is also particularly important for policy makers who are responsible for setting rules and regulations related to hunting, as well as for managers of conservation areas that allow for the continued use of resources by indigenous communities, which is typically the case in the Neotropics.
Large-bodied species tend to carry a higher risk of local extinction from overhunting when compared to smaller-bodied animals, due to a combination of particular biological traits (i.e., low reproductive rates, and naturally low population densities), behavioral traits (i.e., diurnally active, high visibility, slow moving, repeated use of den/resting sites), and external environmental factors (i.e., limited geographic range) (Cardillo et al., 2005; Fa and Brown, 2009). Studies have shown an overall preference for large-bodied frugivorous and herbivorous mammals among hunters in the Neotropics (Redford and Robinson, 1987; Jerozolimski and Peres, 2003), which has resulted in documented local population declines of lowland tapir (Tapirus terrestris), red brocket (Mazama americana) and white-tailed deer (Odocoileus virginianus), white-lipped peccary (Tayassu pecari), and giant armadillo (Priodontes maximus) (Cullen Jr. et al., 2000; Peres, 2001; Weber and Gonzalez, 2003; Di Bitetti et al., 2008; Superina et al., 2014).
Little of the substantial plant biomass present in tropical forests is readily available as food for the large, terrestrial frugivores and herbivores (DeWalt and Chave, 2004) that are preferred by hunters (Peres, 2001; Ripple et al., 2016), as it tends to be either out of reach in the upper canopy or indigestible (Waterman and McKey, 1989; Fa and Peres, 2001; Fa and Brown, 2009). Large, tropical forest mammals, therefore, exist naturally at relatively low densities (Arita et al., 1990), making them particularly vulnerable to overhunting even at moderate or low levels of hunting intensity (Wilkie et al., 2011). Further, omnivorous species (i.e., peccaries) achieve higher reproductive rates and crude and metabolic biomass than their purely herbivorous counterparts (Bodmer, 1989), putting herbivorous species like lowland tapir, red brocket and white-tailed deer at a higher risk of overhunting. Declines in the populations of these key species can have significant negative effects on tropical forest ecosystems through decreased seed dispersal and seedling survival, changes in vegetation cover and composition, and functional compensation (Peres and Dolman, 2000; Terborgh et al., 2001; Peres and van Roosmalen, 2002; Stoner et al., 2007; Wright et al., 2007; Beck et al., 2013). It is estimated that for communities dependent exclusively on wildlife for protein, tropical forests can support ~1 person/km2 (Robinson and Bennett, 2000), and therefore, a negative impact on the populations of preferred game species can still occur even if only a few animals are hunted per square kilometer per year (Mena et al., 2000).
In Guyana, the demand for wild meat is steadily increasing in the country's growing urban centers. An estimated 625 tons of wild meat per year (0.2 tons/km2/year) are consumed in Guyana's capital (Puran et al., 2017)—a rate of consumption comparable to the Amazon Basin (0.23 tons/km2/year) (Rushton et al., 2005; Nasi et al., 2011), but much lower than the Congo Basin (1.98 tons/km2/year) (Fa and Purvis, 1997; Fa et al., 2002; Nasi et al., 2011). Lack of employment opportunities, coupled with increasing access to markets (Wilkie et al., 2000; Laurance et al., 2006; Puran et al., 2017), means that many indigenous hunters in Guyana's interior have shifted from hunting as a predominately subsistence activity to hunting that yields enough to both meet subsistence needs and supplement incomes.
Protected areas have long been recognized as important refuges for biodiversity. They can serve as a key buffer against local extinctions driven by overhunting (Le Saout et al., 2013), but are also recognized for their ability to function as a reserve that can meet the continued needs of subsistence hunters (Robinson and Bennett, 2000). The Kanuku Mountains Protected Area (KMPA) is one of Guyana's richest protected areas in terms of biodiversity (Montambault and Massa, 2002), and, like all of Guyana's protected areas, the KMPA is categorized as an IUCN category VI protected area, which allows for the continued sustainable use of resources by surrounding indigenous communities. The KMPA is bordered by 11 titled villages and 10 satellite communities, located in the adjacent Rupununi savannas. These largely indigenous Makushi and Wapichan communities are dependent on the KMPA's forest resources for subsistence. Approximately 20 and 55% of Rupununi households reported that wild meat and wild-caught fish are their primary source of protein, respectively (Luzar et al., 2012). More recently, a 2016 survey showed that all 21 communities have at least some residents that hunt within the KMPA and adjacent indigenous titled lands, albeit to varying extents, intensities, and for different purposes (Protected Areas Commission, unpublished data).
The extent to which the Kanuku Mountains region is supplying the growing demand for wild meat in Guyana's urban centers is unknown; however, the local trade in wild meat currently fetches the highest return on investment for any food product in the region (FAO, 2015). Although this area supports one of the lowest human population densities in the world (0.42 people/km2), the indigenous population of the region has been increasing steadily (Bureau of Statistics (Guyana), 2016), while also transforming from primarily subsistence to increasingly commercial livelihoods. Demand for economic development, the opportunity presented by growing markets for wild meat, continued erosion of traditional beliefs and practices (Iwamura et al., 2016), and climate change models that predict this region will likely trend toward progressively hotter and drier conditions (Bovolo et al., 2012), creates the potential for significant negative effects on wild animal populations. The shift in hunting patterns raises particular concern among conservation managers and community leaders alike, with several communities already identifying overhunting as a driver of observed population declines, and even local extinctions, of some preferred game species (Protected Areas Commission, unpublished data).
Using a combination of household surveys, focus group discussions, community workshops, and camera-trap data, this study examines the type, level, and perceived and quantifiable impacts of low-intensity hunting on the occupancy, relative abundance, and activity patterns of important game species in the KMPA and surrounding indigenous titled lands.
Materials and Methods
Study Area
The Kanuku Mountains Protected Area (KMPA) encompasses 611,000 hectares of largely intact tropical forest in south-western Guyana (Figure 1). The mountains are divided into eastern and western ranges by the north-south course of the Rupununi River, a tributary of Guyana's largest river, the Essequibo. The protected area is largely composed of gallery, lowland, and montane, deciduous and evergreen tropical forest, with 1% comprising the surrounding Rupununi savannas. The Kanuku Mountains highest peak reaches 1,067 m asl, with a number of minor peaks in its western range above 900 m asl. The surrounding Rupununi savannas lie between 120 and 150 m asl, are ecologically connected to Brazil's Rio Branco savanna system (Montambault and Massa, 2002), and are analogous to the cerrado savannas of eastern Brazil (Eden and McGregor, 1992). The region experiences two rainy seasons, one long (May-August) and one short (December), and a long dry season (September–April), with an average annual rainfall of 1,500–2,000 mm.
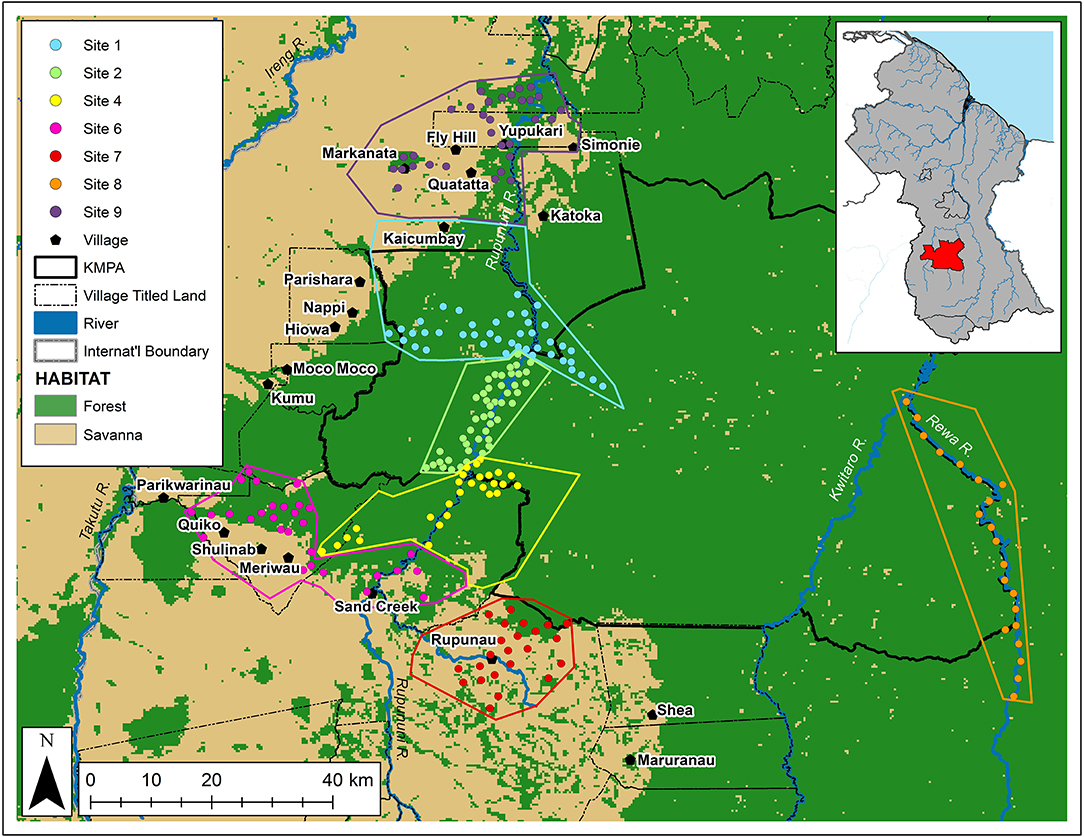
Figure 1. Map of the Kanuku Mountains region, showing camera-trap locations (circles), hunting sites (polygons), and villages (pentagons), as well as the boundaries of the KMPA (solid line) and indigenous community titled lands (dotted line) (Arino et al., 2012; Environmental Systems Research Institute (ESRI), 2015).
The Kanuku Mountains and associated savannas are extremely rich in biological diversity, hosting approximately 70% of mammal, 53% of bird (including 17 of the 25 bird species endemic to the Guiana Shield), and 26% of plant species recorded in Guyana (Montambault and Massa, 2002). The KMPA also harbors healthy populations of many species that are listed on the IUCN Red List of Threatened Species, such as the harpy eagle (Harpia harpyja), giant river turtle (Podocnemis expansa), arapaima (Arapaima arapaima), and giant river otter (Pteronura brasiliensis).
The 21 communities adjacent to the KMPA are composed primarily of indigenous Makushi and Wapichan people who maintain traditional livelihoods, including subsistence fishing, farming, and hunting. The Kanuku Mountains region supports populations of game species preferred by hunters, including lowland tapir, white-lipped peccary, red brocket deer, lowland paca (Cuniculus paca), and black curassow (Crax alector).
Assessing Hunting Patterns and Intensity
In 2015 and 2016 individual household surveys were carried out in all 21 communities surrounding the KMPA. A minimum of 50% of households were surveyed in each community, however in communities where the total number of households totaled <50, 100% of households were surveyed. In total, 815 households participated across the 11 villages (21 communities). The survey was designed to obtain a baseline of the Knowledge, Attitudes, and Practices (KAP) of participating communities as they relate to resource use in and around the protected area. Specific to this study, we calculated the percentage of households that engage in hunting activities in each community, as well as the species hunted. For each species identified as hunted, we also determined how frequently they were hunted and whether respondents perceived the abundance of each of the species as less, more, or the same as 10 years ago. Respondents were also asked their primary purpose for hunting (i.e., home use, selling, sharing, or multiple purposes).
Following these KAP surveys, in 2017, we carried out resource use mapping exercises in each of the 21 communities. Using participatory mapping and focus group discussions, each community worked with facilitators to create a sketch map of their resource use areas, and to identify hunting areas, hunting methods, the purpose of hunting, and hunting patterns using a seasonal calendar. Lists of hunted species developed from the KAP surveys were presented to focus groups and were verified or amended as necessary. Following the KAP surveys, communities were re-visited, and, at open village meetings, were asked similar questions to those in the KAP surveys, such as which species are hunted the most, which are getting less abundant, and which species villagers are most concerned about. A participatory voting approach was used in these meetings to verify trends in the individual responses from the KAP surveys. Once confirmed, maps of hunting areas were developed for each community.
Camera-Trap Surveys
Camera-trap photos were obtained as part of multi-species camera-trap studies of the Rupununi Region, following well-established methods for camera-trap research (Karanth and Nichols, 1998; Silver, 2004). Camera-traps (Bushnell Trophy Cam #119447C, #119734C, #119736C, and #119837C; Bushnell®, KS, USA) were set 2–3 km apart, with a single camera at each trap location, set 30–40 cm from the ground in proximity to observed animal sign. Cameras were active 24 h per day, with a 1 second delay between captures, recording the date and time with each 3-image sequence. Images of the species of interest that occurred at the same trap site within a period of 30 min were excluded to ensure that photo occasions were independent (Silver, 2004). In an effort to reduce wariness around cameras and avoid biased capture rates, no scents or lures were used, and all cameras employed were equipped with infrared flash.
Camera-trap sites were selected for inclusion in this study based on their proximity to hunting areas documented by KAP surveys and resource use mapping exercises. This included areas surveyed within the KMPA, as well as the adjacent titled lands of 14 indigenous communities. Camera-traps were set in clusters of 20–30 cameras and left in the field for 40–365 trap nights between May 2012 and October 2016. In order maximize the number of sampling locations across the study area, we integrated data from two different projects that employed identical methodologies, with the exception of the number of trap nights at each camera location. To standardize sampling effort and trap spacing, we selected the data from the first 40 trap nights at each camera location and removed overlapping trap locations with spacing <2 km.
In total, our sample includes 221 camera-trap locations (Figure 1) and 8,840 trap nights, which resulted in 51,036 photographs and 17,012 occasions. We detected a total of 102 species (46 mammals, 48 birds, 7 reptiles, 1 amphibian), of which 17 species were selected for inclusion in our analysis based on their status as either a game species targeted by local hunters, a terrestrial predator, or as a species of ecological importance/conservation interest.
Hunting Intensity Analysis
The boundaries of each site (Figure 1) were determined by the combination of (a) the locations of hunting areas identified by community members, (b) the number of people and number of villages using each hunting area, (c) the habitat type associated with hunting areas identified, and (d) the availability of overlapping camera trap data. Of 21 communities surveyed, data from 14 communities (7 of the 11 titled villages) were selected for inclusion in this study based on their overlap with camera-trap surveys. In cases of overlapping hunting sites between multiple communities, each combination of communities using a given area was recorded. Hunting intensity for each site, defined as the number of households hunting in a particular area (HH/km2), was determined by calculating the number of households from each community hunting in a given site, divided by the total area of that polygon.
We calculated the number of households by taking the percentage of respondents from the KAP surveys that hunted and extrapolating it to the total number of households known to occur in that village. For example, if the KAP surveys showed 10% of respondents said they hunted, and there was a total of 80 households in that village, we would calculate that eight households hunted. In this manner, the sum of all the households hunting would be combined to give an estimate of the total number of households hunting in a given site. Although we recognize that these are not an accurate reflection of the actual intensities for each site, calculating in a consistent and standardized manner across sites allows us to make inferences about their intensities relative to one another. Sites with the highest intensity value (HH/km2) were considered as the most intensely hunted sites.
GPS locations of hunting areas were used in determining the boundaries of each site, but intellectual property agreements with partner communities prohibits specific locations from being shown here. Variation in the overall size of each polygon (due to some communities hunting across larger areas than others) was standardized by considering camera trap density (traps/km2) at each site (Table 1). After excluding sites with insufficient camera-trap survey effort (<20 trap locations—Sites 3 and 5), seven sample sites were identified (Figure 1)—four in mixed lowland and upland tropical forest (largely within the protected area), and three within the savanna-forest mosaic (largely outside the protected area).
Camera-Trap Data Analysis
Occupancy, relative abundance, and activity patterns were calculated for each species of interest at each camera location to account for the influence of hunting intensity (as calculated above) on spatial and temporal distribution. To account for additional variation in detection probability on occupancy, we implemented season (rainy or dry) and trail type (anthropogenic or natural) as covariates in our model. Forest cover was determined by placing a 1-km buffer around each camera-trap and calculating the percent forest cover (Hansen et al., 2013) within each buffer using the Tabulate Area function. Distance to village was determined by calculating the Euclidean distance (in meters) from each camera-trap to the nearest village. Season was determined by the date range during which each camera was active and trail type was determined visually at each camera location. Cameras were placed into 15 spatial groups using the Grouping Analysis tool to control for spatial autocorrelation. We chose the K-nearest neighbors method and used trial and error to determine the optimum number of nearest neighbors (K = 20). All spatial analyses were conducted in ArcMap 10.3.1 (Environmental Systems Research Institute (ESRI), 2015).
We used a Bayesian multi-species hierarchical occupancy modeling approach (Dorazio and Royle, 2005) to assess the relationship between occupancy and hunting pressure, distance to the closest village, and percent forest cover. This approach enabled us to estimate species-specific and aggregate community occupancy while accounting for imperfect detection (MacKenzie et al., 2002; Dorazio et al., 2011). We recorded a binary measure of detection (1 = observed, 0 = not observed) for each species at each camera location for each 24 h period from when the cameras were deployed (n = 40). The number of trap nights available for each camera site varied, but subsetting the data to include the first 40 trap nights from each site allowed us to maximize the number of camera-trap sites included in our sample, while managing reasonable computation time. We investigated variables that we believed a priori would influence species-specific occurrence or detection using a generalized linear mixed modeling (GLMM) approach (Dorazio and Royle, 2005; Russell et al., 2009). We modeled the relationship of season (0 = rainy, 1 = dry) and trail type (0 = anthropogenic, 1 = natural) with species-specific and community detection probability. We modeled the relationship of hunting pressure, expressed as our hunting intensity index ranging from 0 to 1, distance to the closest village, and the percent of forest cover within a 1 km buffer around each camera, with species-specific and community occurrence probability. We standardized variables accounting for variability in detection and occurrence using a z-transformation and modeled them as random effects with species-level variation drawn from a common distribution inclusive of an estimated mean and variance (i.e., hyperparameters). Additionally, we modeled discrete spatial clusters of cameras as a random effect to account for spatial autocorrelation. We fitted a single full model inclusive of all a priori variables (Zipkin et al., 2010).
We estimated the posterior distributions of each parameter using Markov chain Monte Carlo (MCMC) implemented in JAGS (version 4.2.0) using the R2Jags R package (Plummer, 2011). We used uniform (uninformative) priors (Gelman et al., 1995; Gilks et al., 1996) and generated 3 chains of 50,000 iterations with a burn-in of 10,000 iterations and a thinning rate of 50, yielding 3,000 samples. We then assessed convergence of MCMC chains with trace plots and the Gelman-Rubin diagnostic (Rhat), where values <1.1 indicated convergence (Gelman and Hill, 2007). We considered model covariates with 95% Bayesian credibility intervals (CRI) not inclusive of zero to be relevant predictors of occurrence or detection.
We used the package “overlap” in R to estimate the percent overlap in the activity patterns of species of interest across all sites, as well as within pair-wise comparisons between sites with the highest and lowest hunting intensities (Ridout and Linkie, 2009). Package “overlap” observes capture times as random samples from a continuous distribution, and the “coefficient of overlap” as a non-parametric measurement of the overlap between the probability distribution functions of these underlying distributions estimated by bootstrapping (Ridout and Linkie, 2009). Avoidance of heat stress is a known factor influencing circadian rhythms of mammals in open habitats (Terrien et al., 2011), thus we excluded sites with <90% canopy cover (all savanna forest sites) from analysis of activity patterns to isolate effects related to hunting activity. Lastly, we calculated relative abundance indices (RAI's) of each species by dividing the number of occurrences of each species by the number of nights at each camera and standardizing for 100 trap nights (O'Brien, 2011). R code for each analysis can be found in Appendix 1.
Results
Communities' Hunting Activity and Patterns
All communities surveyed, but not all households in each community, indicated that they hunt. On average, 25% (range = 0.02–0.61) of households across all communities surveyed hunt. Of the 21 communities, all hunt on their village lands and 18 out the 21 also hunt inside the KMPA. Typically, hunting occurs throughout the year, with small groups of hunters going out for a few days at a time. Hunting activities increase in frequency and intensity, and hunting parties increase in size, around key celebrations, such as Amerindian heritage month (September), Easter, and Christmas. During these celebrations, certain large-bodied species, such as lowland tapir, are highly sought after for holiday meals.
Outside annual celebrations, two key hunting seasons were commonly identified across communities. The first is during the peak fruiting seasons (August and December), when animals are more accessible as they feed on fruits that drop to the forest floor. The second is during the peak dry season between January and March, when normally elusive animals gather around drying ponds, creeks, and springs, making them easier prey for hunters. Village residents mainly hunt for home or celebration (subsistence) purposes, but on average 62% of respondents across the 21 communities reported hunting for both home use and to generate income. Although hunting methods are highly variable depending on species and season, the most common method for hunting year-round across all villages was pursuit with dogs, bow and arrow, and/or firearms, with the level of offtake generally being consistent across hunters and villages.
Both KAP surveys and focus group discussions showed similar results in species hunted. The most commonly and frequently hunted species across all communities surveyed were (from most common to least) lowland paca, red brocket deer, red-rumped agouti (Dasyprocta leporina), collared peccary (Pecari tajacu), black curassow, lowland tapir, white-tailed deer, great long-nosed armadillo (Dasypus kappleri), nine-banded armadillo (Dasypus novemcinctus), yellow-footed tortoise (Chelonoidis denticulatus), red-footed tortoise (Chelonoidis carbonarius), capybara (Hydrochoerus hydrochoeris), and spectacled caiman (Caiman crocodilus). Lowland paca, red-rumped agouti, collared peccary, red brocket deer, and lowland tapir were the top five preferred mammal species across our survey sites (Table 2). Correlation analysis showed a very strong positive relationship between species most frequently hunted and those perceived to be less abundant than they were 10 years ago (r2 = 0.89; Figure 2).
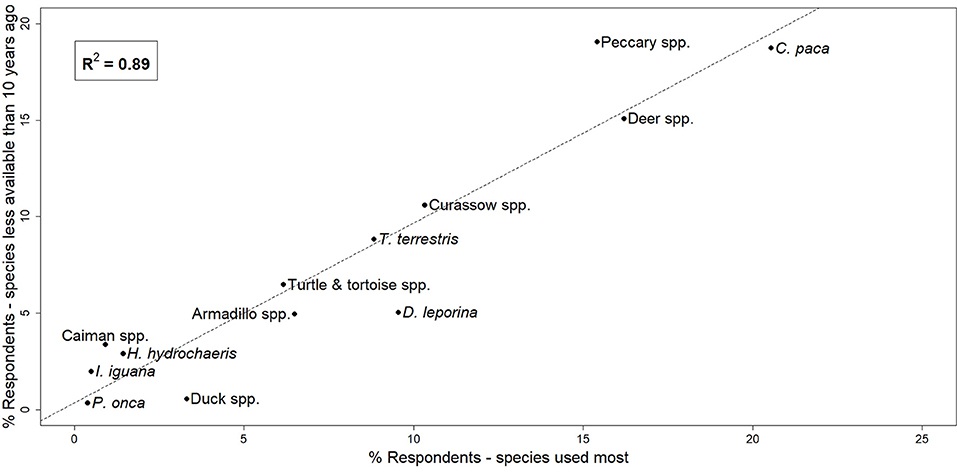
Figure 2. Correlation between species hunted most often and those perceived to be less available than 10 years ago, expressed as the percentage of respondents who identified each species during KAP surveys.
Hunting Intensity
Hunting intensity varied across sites, ranging from very low and low hunting intensity (Site 2 and Site 8) to higher intensity (Site 4 and Site 7) (Table 1). We found no correlation in any of the 21 communities surveyed between the proportion of households hunting in a village and the distance to the nearest market town, the nearest road, the protected area, or the number of shops available in their community. However, hunting intensities tended to be slightly higher in savanna forest habitats, with all sites experiencing medium and high hunting intensity compared to forested habitats where hunting intensity ranged from very low to high (Table 1).
Occurrence and Detection Probability
Our Bayesian multi-species hierarchical occupancy model indicated that although cumulative community detection probability was positively associated with dry season (β 0.07; CRI −0.17 to 0.33; Rhat 1.00) and natural (game) trails (β 0.05; CRI −0.08 to 0.18; Rhat 1.00), neither were a statistically significant predictor of detection. Similarly, we also found that cumulative community occurrence was positively (but not significantly) associated (did not exhibit a clear directional association) with hunting intensity (β 0.05; CRI −0.30 to 0.41; Rhat 1.00), and distance to nearest village (β 0.06; CRI −0.35 to 0.47; Rhat 1.00). Percent forest cover was found to be a significant predictor of community occurrence (β 0.56; CRI 0.18 to 0.95; Rhat 1.00), as occupancy increased with forest cover for most species included in our analyses.
Gelman-Ruben diagnostic (Rhat) results showed that all individual species models converged (Rhat <1), implying confidence in our estimates (Table 3). Trail type was not a good predictor of detection for any of the 17 species included in our analyses. Conversely, season proved a significant predictor of detection for several species, with lowland tapir (β 0.59; CRI 0.33–0.86), jaguar (Panthera onca) (β 0.90; CRI 0.40 to 1.44), and ocelot (Leopardus pardalis) (β 0.48; CRI 0.13 to 0.86) showing a positive correlation with detection in the dry season, while collared peccary (β −0.41; CRI −0.78 to −0.04), lowland paca (β −0.30; CRI −0.48 to −0.11), red acouchi (Myoprocta acouchy) (β −0.33; CRI −0.50 to −0.18), and gray-winged trumpeter (Psophia crepitans) (β −0.36; CRI −0.53 to −0.19) all had higher detection probability in the rainy season.
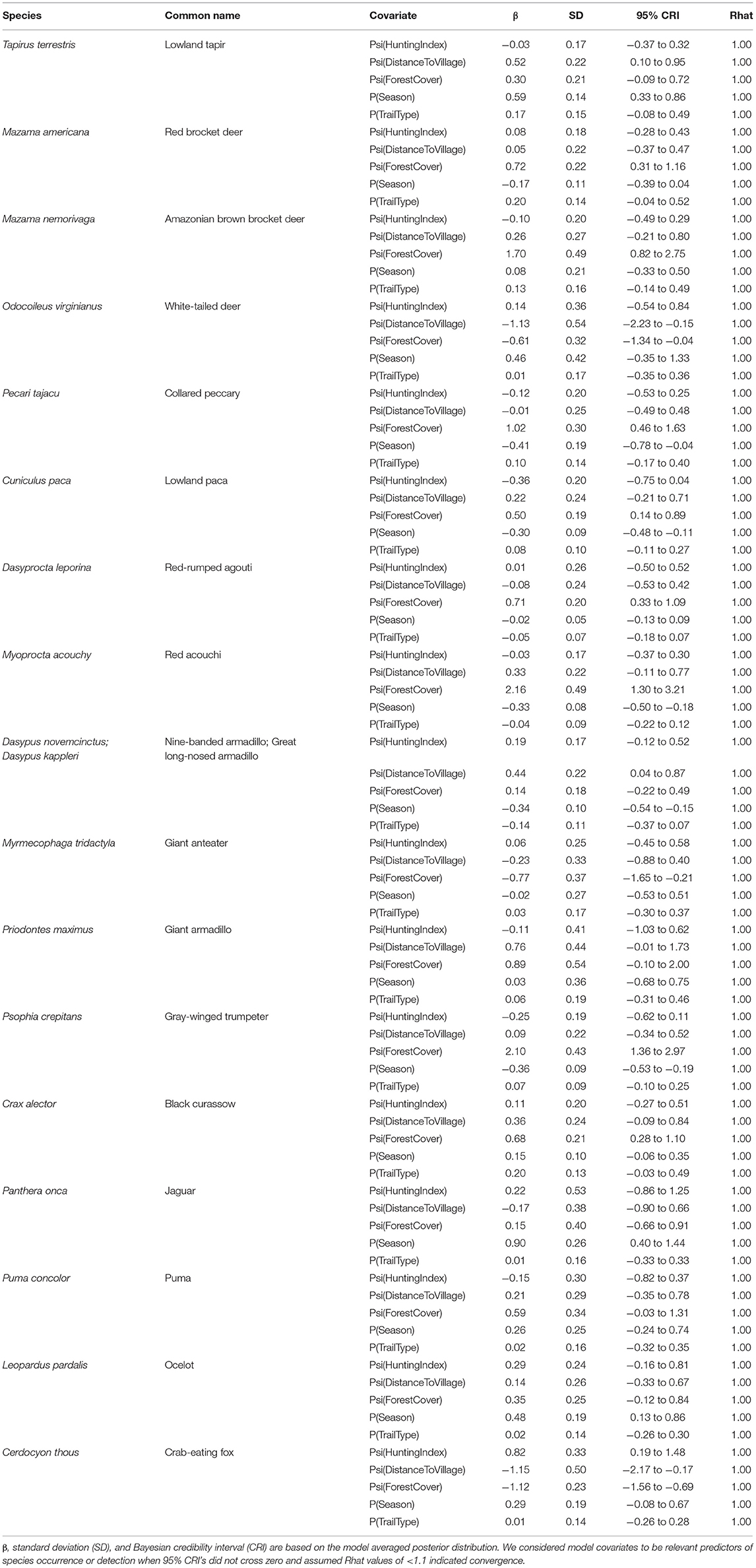
Table 3. Species-specific summaries of covariate effects on occupancy (psi) and detection (p) for 18 species of interest.
Forest cover had the most significant relationship with species occurrence, with 11 out of the 17 species showing a significant relationship with this variable (Appendix II). Increased forest cover was positively associated with occurrence of the red acouchi (β 2.16; CRI 1.30 to 3.21), black curassow (β 0.68; CRI 0.28 to 1.10), collared peccary (β 1.02; CRI 0.46 to 1.63), Amazonian brown brocket deer (Mazama nemorivaga) (β 1.70; CRI 0.82 to 2.75), gray-winged trumpeter (β 2.10; CRI 1.36 to 2.97), lowland paca (β 0.50; CRI 0.14 to 0.89), red brocket deer (β 0.72; CRI 0.31 to 1.16), and red-rumped agouti (β 0.71; CRI 0.33 to 1.09), and a significant negative association with the occurrence of crab-eating fox (Cerdocyon thous) (β −1.12; CRI −1.56 to −0.69), giant anteater (Myrmecophaga tridactlya) (β −0.77; CRI −1.65 to −0.21), and white-tailed deer (β −0.61; CRI −1.34 to −0.04). Lowland tapir (β 0.52; CRI 0.10–0.95) and both species of long-nosed armadillos (β 0.44; CRI 0.04 to 0.87) demonstrated a significant positive correlation with increased distance from the nearest village, while white-tailed deer (β −1.13; CRI −2.23 to −0.15) and crab-eating fox (β −1.15; CRI −2.17 to −0.17) exhibited significant negative relationships (Appendix II). Hunting intensity did not appear to have a significant impact on species occurrence for any of our 17 species (Appendix II), with the exception of the crab-eating fox (β 0.82; CRI 0.19 to 1.48) which showed a significant positive relationship with hunting intensity (Table 3).
Relative Abundance Index
Given the influence of forest cover and season in predicting species occurrence, we compared the RAI of species between lower and higher hunting intensity sites post analysis, when habitat type and season were similar. In this manner, we compared Site 2 (very low intensity) with Site 4 (high intensity), both in tropical forest habitat sampled during the rainy season, as well as Site 6 (medium intensity) and Site 7 (high intensity), which represented savanna-forest mosaic habitat sampled in the dry season (Table 1). In forested habitats in the rainy season, and in savanna-forest mosaic in the dry season, nearly all species showed differences in their RAI's between low and high intensity sites (Table 4), however none of these differences proved statistically significant (Wilcoxon's test: forest: z = −0.37, n = 15, p = 0.71; savanna: z = −1.48, n = 15, p = 0.14). Lowland tapir, all three species of deer, red-rumped agouti, puma (Puma concolor), and ocelot consistently showed higher RAI's in sites that had lower hunting intensity levels regardless of habitat type, while lowland paca, black curassow, gray-winged trumpeter, and jaguar showed the same trend within forested habitats only. Conversely, collared peccary and red acouchi consistently showed higher RAI's in both higher hunting intensity sites, while lowland paca, black curassow, gray-winged trumpeter, crab-eating fox, and jaguar showed this trend in savanna-forest habitat only.
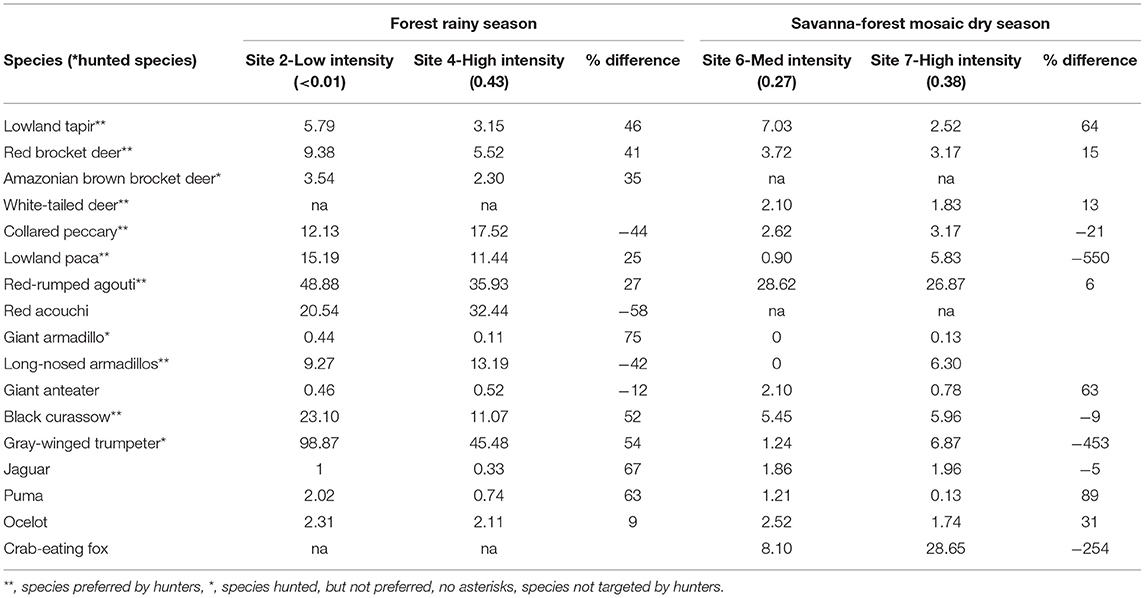
Table 4. Comparison of relative abundance indices (RAI) of species of interest between sites with “low” and “high” hunting intensity, keeping habitat type and season constant.
Activity Patterns
Although we plotted the activity patterns of 13 of the species included in the analyses above (Appendix III), we applied more detailed analyses to the top five hunted species as indicated by our KAP surveys, as well as two non-hunted species—red acouchi and ocelot. Plots of general activity patterns showed that red-rumped agouti and collared peccary were primarily diurnal, lowland paca and both long-nosed armadillos exclusively nocturnal, lowland tapir were mostly nocturnal with some activity during the day, and red brocket deer were active both day and night (Figure 3). In non-hunted species, the red acouchi were crepuscular and ocelots were active both day and night with peak activities occurring at dawn, dusk, and midday.
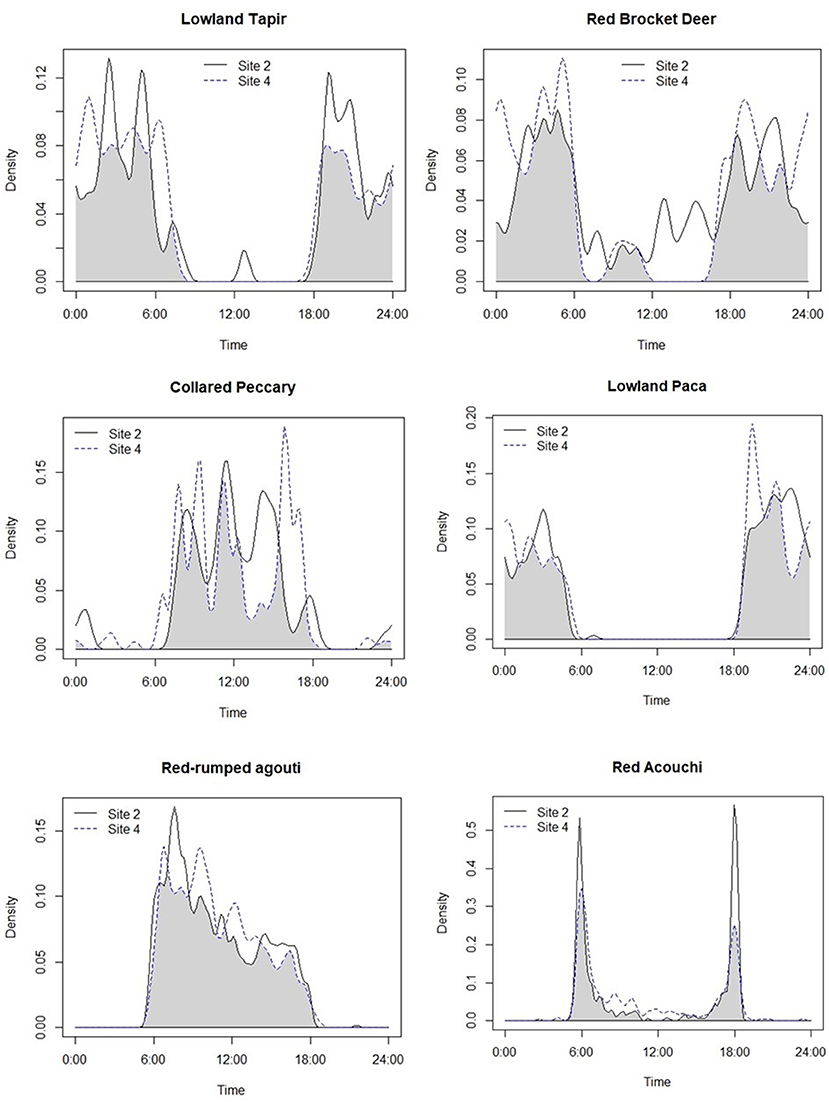
Figure 3. Overlap in the activity patterns of species of interest at Site 2 (very low hunting intensity) and Site 4 (high intensity sites), keeping habitat (tropical forested) and season (rainy) constant.
In pair-wise comparisons of activity patterns between the lowest (Site 2) and highest intensity hunting sites (Site 4) where both habitat and season were constant, we observed shifts in activity patterns consistent with temporal avoidance of human (hunting) activity. Large-bodied game species that are targeted by hunters showed a decrease in diurnal activity at the more intensively hunted site (Figure 3), with activity patterns of lowland tapir, red brocket deer, and collared peccary shifting by 18.1, 19.8, and 20% respectively (Table 5). Medium-sized game animals showed more subtle shifts in activity patterns (Figure 3), with lowland paca (10.9%), red-rumped agouti (10%), and the long-nosed armadillos (10.1%) each shifting away from peak hunting times early in the morning (Table 5). Pair-wise comparisons of the overlap of activity patterns of medium-sized non-hunted species showed much larger shifts in activity, with red acouchi and ocelot shifting toward increased activity in the morning by 29 and 27%, respectively.
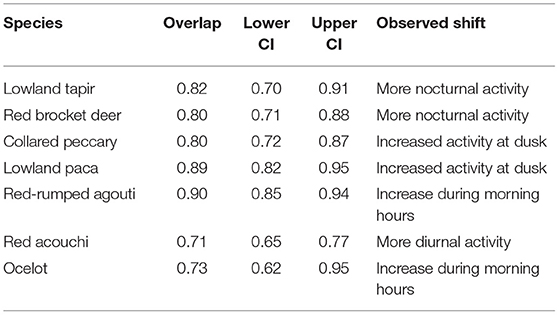
Table 5. Percent overlap and overall trends in activity patterns of species of interest between Site 2 (very low hunting intensity) and Site 4 (high hunting intensity).
Discussion
As the demand for wild animal meat continues to increase, maintaining healthy populations of game mammals and birds represents a significant challenge facing conservation managers around the world. Understanding shifts in hunting preferences, patterns, and intensities, as well as the potential negative impacts of hunting, is critical for policy makers who are responsible for setting rules and regulations related to hunting. This is particularly true in regions that allow for the continued use of resources within conservation areas by indigenous communities, as is typically the case in the Neotropics. Boasting one of the world's lowest deforestation rates and human population densities, Guyana's interior still hosts healthy populations of many globally and locally important species. However, indigenous communities surrounding the Kanuku Mountains Protected Area, one of the country's most biodiversity rich protected areas with a human population density in adjacent communities of only 0.42/km2, are reporting declines, and even local extinctions, of some preferred game species.
Hunting is typically considered sustainable when the number of individuals removed from the population is the same as the number added through normal population growth (Clark, 1990; Milner-Gulland et al., 2009). Our results indicate that current levels of hunting in and around the KMPA may currently be sustainable, however small shifts detected in the behavior and distribution of species preferred by hunters match the perceived changes observed by KMPA's indigenous communities, suggesting that species under elevated hunting pressure may be changing their behavior to evade predation by humans.
Species Occurrence
Our results showed that hunting intensity, distance to village, forest cover, and season all serve as significant predictors of species occurrence and detectability, depending on the species. Interestingly, trail type did not have a significant relationship with detection for any of the species included in our model, despite previous research indicating that this was an important predictor for some species (Harmsen et al., 2010). We believe that this contradictory result can be attributed to the fact that none of the camera-traps in our sample were set along roads, and that the human-made trails that were utilized for camera-trapping were only lightly used.
Of the variables examined, forest cover was the best predictor of occurrence across species, showing a significant relationship with 11 out of the 17 species examined. Red and Amazonian brown brocket deer, collared peccary, lowland paca, red-rumped agouti, red acouchi, black curassow, and gray-winged trumpeter (includes four out of the top five species preferred by indigenous communities surrounding the KMPA), all showed a significant positive association with increased forest cover. On the other hand, white-tailed deer, giant anteater, and crab-eating fox showed a significant negative association with percent forest cover. These results are not surprising and reflect the general habitat preference typical for each species. White-tailed deer, giant anteater, and crab-eating fox are considered savanna-dwelling species (with giant anteater also inhabiting tropical forest but in lower densities), while the remaining species listed above are typically associated with dense tropical forests.
Forest cover was not a significant predictor of occurrence for lowland tapir and the long-nosed armadillos, however, suggesting that these species may use the savanna-forest mosaic more readily than other “forest-dwelling” game species. This result is not unexpected, as both species are known inhabitants of savanna, grassland, and shrubland habitats of the Cerrado, Chaco, Llanos, and Pantanal regions of South America (Loughry et al., 2014; Varela et al., 2019). Similarly, forest cover was not a good predictor of occurrence for jaguars, pumas, or ocelots, perhaps unsurprisingly as the savanna-forest mosaic hosts sufficient prey (including domestic animals) and the distribution and movement of these species is well-documented to be a function of prey availability (Weckel et al., 2006). Season was also a key variable in detection probability for many species, with lowland tapir and jaguar more detectable in the dry season, while probability of detection for collared peccary, lowland paca, red acouchi, and the long-nosed armadillos was higher in the rainy season. As with our interpretation of species relationships with forest cover, we suggest that these findings are more likely attributed to behavior and responses to the availability of water and prey/food, than for any other reason.
While not associated with forest cover, occurrence of both lowland tapir and the long-nosed armadillos showed a significant positive correlation with increased distance to villages. Studies show that distance to village is an important predictor for other elusive species, particularly in areas where hunting pressure is high (Phan et al., 2019). Lowland tapirs, in particular, are known to prefer less disturbed areas further away from human presence (Tobler, 2002; Licona et al., 2011). Generally, hunters use areas close to settlements more frequently than more distant areas (De Souza-Mazurek et al., 2000) and the communities in our study are no exception, with all 21 villages hunting more frequently in the savanna-forest mosaic close to villages (Protected Areas Commission unpublished data). KAP surveys and mapping exercises also showed that hunting intensities were higher in savanna-forest mosaic habitat near to villages, compared to forested habitats which are typically further from villages and closer to or within the KMPA (Table 2). So, while tapir and armadillos more readily utilize savanna forest habitats than other “forest-dwelling” species, distance from human settlement seems to be a more influential factor in determining the distribution of these species. Interestingly, lowland tapir and the long-nosed armadillos were ranked as the fifth and sixth most preferred forest game mammal species across the 21 communities surveyed. Both were also frequently cited as a species that communities were most worried about with regard to their availability in the future (Protected Areas Commission unpublished data), indicating that even relatively low hunting pressure may affect the distribution of species that are particularly sensitive to human activity.
Our study generally supports Robinson and Bennett (2000) hypothesis that hunting intensity <1 hunter/km2 is sustainable. Though a number of hunted species showed a negative association, hunting intensity was not a significant predictor of occurrence for any of the species that we examined, with the exception of the crab-eating fox which showed a significant positive relationship with hunting intensity. The crab-eating fox is not hunted within our study area, and we suggest that this relationship is likely the result of its preference for scavenging, as well as the reduction in niche occupancy of competing species. Although omnivorous, studies have shown that fruit is the most prevalent item in the crab-eating fox's diet (Rocha et al., 2008), and thus there is likely significant dietary overlap with a number of the species that were identified as hunted by the KAP surveys. Occurrence of crab-eating fox also showed a significant negative relationship with increased distance to villages, which is not surprising as crab-eating fox frequently occur in human-dominated areas where they are known to scavenge (Rocha et al., 2008) and likely feed on fruits from cultivated trees often found in association with homesteads (mango, cashew, citrus).
Relative Abundance
Although not statistically significant, our results show that the RAI's of nearly all hunted species were higher in sites with low hunting intensity when compared to those with higher hunting intensity, with habitat (tropical forest) and season (rainy) kept constant. These trends are synonymous with what would be expected if hunting is having an impact on species, in so far as there are higher RAI's in the low hunting intensity site for species targeted by hunters, with the largest differences in those species that have a greater predisposition to overhunting—i.e., lowland tapir and deer (>40% increase in RAI in low hunting intensity sites) (Bodmer, 1989; Cardillo et al., 2005; Fa and Brown, 2009; De Thoisy et al., 2010). Smaller-bodied, more prolific breeding species such as lowland paca and red-rumped agouti, species known to be somewhat resistant to the effects of harvesting (Mayor et al., 2007), showed much smaller differences (~25% increase in RAI in low hunting intensity sites) in RAI between sites.
For species whose movements and distribution are largely determined by prey availability, such as jaguar and puma (Weckel et al., 2006), trends in RAI mirrored those for most potential prey species. Conversely, we found that collared peccary and red acouchi showed higher RAI's in more intensely hunted sites. We suggest that this may be a result of these species occupying niches that have become available as competition for resources is reduced (Brown and Davidson, 1977). Previous studies (Fragoso et al., 2016) and anecdotal reports indicate that white-lipped peccaries were once relatively abundant across our study area. The lack of white-lipped peccary occurrences in our data suggests that the rapid, unexplained population declines that have transpired across the range of this species (Altrichter et al., 2012) may have also occurred in and around the KMPA. The disappearance of a superior competitor with which there is a high degree of niche overlap (Desbiez et al., 2009), in combination with their generalist nature and high reproductive rates, may explain increased RAI observed in collared peccaries. In rodents, this effect tends to be more prevalent in specialized species (Brown and Davidson, 1977). Red acouchi diets overlap with lowland paca and red-rumped agouti but comprises only about 50% of the diversity of their superior competitors filling the same niche (Dubost and Henry, 2006). As expected, in species such as ocelot, which is not hunted nor has much dietary overlap with other species included in our analysis, we saw little difference in RAI's between low and high intensity hunting sites.
In savanna-forest habitat in the dry season, while we see similar patterns in lowland tapir and jaguar, the potential impacts of hunting on RAI's are less pronounced. We suggest therefore, that additional confounding variables are likely at play. Firstly, the difference in hunting intensity between the two sites compared is relatively small (0.11 compared to >0.42 in forest habitats). Secondly, the site with the highest hunting intensity in our study, Site 7, is in close proximity to a major river, while Site 6, a site with medium hunting intensity, is located far from any major water source (Figure 1). We suspect that, particularly in the dry season, the presence of a permanent water source may have a greater influence in species' RAI's than the impacts of hunting. This hypothesis is further supported by the fact that we see a >500% increase in the RAI of lowland paca in Site 7—a species that is known to remain close to permanent water sources (Hutchins et al., 2003).
Activity Patterns
As with RAI's, we compared the activity patterns of a variety of hunted and non-hunted species at sites with high and low hunting intensity, keeping habitat and season constant. We predicted that hunted species would shift their activity away from peak hunting times while unhunted species, such as the red acouchi, would shift its activities to avoid superior competitors (red-rumped agouti and lowland paca), and that ocelot would show no change in activity patterns. Ocelot are not hunted, there is little dietary overlap with large carnivores, and they have shown not to shift activity patterns even in areas of very high human disturbance (Kolowski and Alonso, 2010).
Activity patterns generally reflected those observed in other studies for large game species, as well as ocelot. Collared peccary were typically diurnal, lowland tapir primarily nocturnal, and red brocket deer (Tobler et al., 2009) and ocelot (Kolowski and Alonso, 2010) active day or night. Among medium-sized hunted species, red-rumped agouti were largely diurnal, following similar activity patterns to those found in previous studies (Dubost, 1988). However, we observed variation in the activity patterns of lowland paca and red acouchi when compared to those previously documented in the literature. Typically, lowland paca are largely crepuscular (Hutchins et al., 2003) and red acouchi predominantly diurnal (Dubost, 1988). However, our data showed lowland paca as exclusively nocturnal, a pattern typically observed in this species in heavily hunted areas (Hutchins et al., 2003). Additionally, our data showed that red acouchi were predominantly crepuscular, suggesting temporal avoidance of the diurnal red-rumped agouti and nocturnal lowland paca.
Shifts in circadian rhythms are considered important for species targeted by hunters, as they may alter reproductive success and survival (Gaynor et al., 2018). Activity pattern overlap analysis showed that large-bodied game species, like lowland tapir, red brocket deer, and collared peccary showed ~20% shifts in activity patterns in the more intensely hunted site, with tapir and deer shifting toward increasingly nocturnal and collared peccary toward increasingly crepuscular activity. Although relatively small, these shifts away from peak hunting times are synonymous with temporal avoidance of human (hunting) activity (Kilgo et al., 1998). Red-rumped agouti and lowland paca also showed minor shifts away from peak hunting times (~10%), with red-rumped agouti showing greater activity around dawn and lowland paca after dusk in hunted sites.
The red acouchi demonstrated a large shift in activity patterns (29%), with increased activity during the day in our most intensively hunted site. We infer that this result demonstrates a cascading shift in activity, with red acouchi shifting toward peak hunting times to avoid peak red-rumped agouti activity around dawn. In this case, hunting activity may have reduced competition with red-rumped agouti and lowland paca, thus opening a temporal niche to this smaller-bodied, inferior competitor. Surprisingly, ocelots also showed a large shift in activity (30%) and appeared to reduce their activity in the middle of the day—similar to the shifts in activity observed in tapir and deer. Shift in ocelot activity may be a response to increased human activity, which would contradict previous studies that suggest ocelots are not impacted by human disturbance (Kolowski and Alonso, 2010). However, competition is also known to be an important influence over the structure of Neotropical carnivore communities (de Oliveira et al., 2010; de Oliveira and Pereira, 2014)—one that cannot be ruled out here and needs to be examined further.
Perceptions vs. Camera-Trap Data
Data from the KAP surveys showed a strong positive correlation between the species most frequently hunted and those perceived by hunters to be less abundant than they were 10 years ago (Figure 2). Our camera-trap data supports these perceptions, with observed shifts in the behavior and distribution of species preferred by hunters that are consistent with what would be expected if species were demonstrating avoidance behavior in hunted areas. Indigenous knowledge (IK) is accumulated over a lifetime of traditional use of species (Gilchrist et al., 2005), and has long been recognized as an important and reliable source of information when used in modeling the abundance and distribution of species (Walters and Holling, 1990; Zabel et al., 2002; Anadón et al., 2009). We suggest, therefore, that a combination of indigenous knowledge and participation, as well as quantifiable data should be applied to informed decision-making on species management and the identification of levels of sustainable hunting for species of interest.
Conclusion
Most previous studies on hunting in the tropics have focused on areas where levels of hunting are already unsustainable (Wilkie et al., 2011), therefore limiting our understanding of the level of harvest that is sustainable for a number of species of conservation concern. In lowland tapirs, it is well-documented that hunting is generally unsustainable even at very low levels as a result of their low population growth rate and density (Tobler et al., 2014). Although our study showed that current hunting levels are not having a statistically significant impact on the occurrence of species preferred by hunters, and this result supports previous studies that conclude that hunting intensity of <1 person/km2 is sustainable, we treat this conclusion with caution. Our models did detect negative relationships with hunting intensity in a number of the preferred game species (lowland paca, collared peccary, lowland tapir, giant armadillo) in our study area. We view this result as an early warning for the Kanuku Mountains region and, considering the low overall hunting intensity at our site when compared to much of the rest of the Neotropics, an indication of the truly low levels of harvest that some species can sustain before populations begin to show declines. Once a significant relationship is found between species occurrence and hunting intensity, its likely hunting has already exceeded sustainable levels, and thus provides little insight for managers and policy makers tasked with preventing or reversing population decline.
Fa et al. (2002) suggests that harvest is no longer sustainable when species exploitation is ≥20% of its productivity. Sustainability therefore is largely attributed to balancing individual removal with population recovery (Clark, 1990) and only accounts for impacts on the populations of hunted species. Our study shows that even with relatively low intensity hunting, shifts in the distribution and behavior of hunted species can trigger cascading effects on non-hunted species, which in turn could have an impact on ecosystems as a whole. Our results support the notion, therefore, that levels of sustainable hunting should not only consider population trends in hunted species but should also include the structure and function of the community as a whole (Milner-Gulland et al., 2009).
We suggest that determining occupancy, relative abundance, and activity patterns of hunted and non-hunted species, as well as incorporating indigenous knowledge of trends in use and populations over time, would be a more effective early alarm system than monitoring the occurrence of hunted species alone. Further, as the human population in the Rupununi region and market demand for wild meat outside of the region grows, and infrastructure developments make the region more accessible to outsiders, our data can serve as a baseline that can be monitored over time. Monitoring can be done alongside surveys of changes in hunting practices, thus allowing for preventative measures to be adopted by managers and indigenous communities to ensure that the level of hunting in the region remains sustainable into the future.
Ethics Statement
Research methodologies received approval from the IACUC and IRB offices at Miami University (#870, #E00547) and the University of Florida (#201408378, #2015-U-400). Methodologies were also reviewed and approved by the Frankfurt Zoological Society, the Protected Areas Commission, and the Ministry of Indigenous People's Affairs (MoIPA) prior to obtaining a research permit from the Environmental Protection Agency (#011315 BR 002) in Guyana. Permission to conduct research within indigenous titled lands was only granted by MoIPA following the submission of individual letters of support signed by the councils of each village who chose to participate in this project.
Author Contributions
MH determined study design, primary facilitator of camera-trap data collection, and co-primary author and editor of paper. AK determined study design, facilitated KAP surveys and community mapping, co-facilitated implementation of camera-trap data collection in some sites, and co-primary author and editor of paper. RM was responsible for KAP survey data management, facilitated analysis of KAP survey data, and contributed to writing and paper editing. TBag informed design and facilitated implementation of KAP surveys and community mapping, co-facilitated implementation of camera-trap data collection, and contributed to writing and paper editing. TBab facilitated spatial analysis of KAP survey data, GIS, and map making. HB contributed to design and implementation of camera-trap data collection, coordinated research teams, contributed to camera-trap data management, and reviewed and provided feedback to paper draft. AW contributed to design and implementation of camera-trap data collection, coordinated research teams, contributed to camera-trap data management, and reviewed and provided feedback to paper draft. FL was a official local collaborator, contributed to design and implementation of camera-trap data collection, coordinated research teams, contributed to camera-trap data management, and reviewed and provided feedback to paper draft. WB was responsible for writing code and running updated Bayesian models, wrote portions of the methods and results sections pertaining to these models, and worked with the team to interpret results. BB contributed to design and implementation of camera-trap data analysis, writing up of statistical methodology, R coding, production of figures, and contributed to writing and paper editing.
Funding
Funding for this project provided through between the Protected Areas Commission, Frankfurt Zoological Society, and Jacksonville Zoo & Gardens, as well as the Ron Magill Conservation Award (2015), Phoenix Zoo Field Conservation and Science Grant (2013), and Field Research Grants from Project Dragonfly (2012), the Institute for the Environment and Sustainability (2012) and the Graduate School (2013) at Miami University, and the Tropical Conservation and Development Program (2014), School of Natural Resources and Environment (2016) and Department of Wildlife Ecology and Conservation (2016) at the University of Florida.
Conflict of Interest
The authors declare that the research was conducted in the absence of any commercial or financial relationships that could be construed as a potential conflict of interest.
Acknowledgments
The authors are grateful to the whole team who made the collection of data possible for this study, particularly PAC staff, Francisco Gomes, Jessica George, Octavious Hendricks, Jenkins Lawrence, Vercida Gomes, Norbert Atkinson, Sara Henry, and Tana Yussuff and the community-based team from the Rupununi Wildlife Research Unit—Ashley Holland, Ramlal Roberts, Brian Duncan, Jose George, Bernard Lawrence, Herman Phillips, Manuel Mandook, Anthony Roberts, Gerry Bell, Henry Clement, Nicholas Mandook, Magnus David, Flavian Thomas, Micah Davis, Martin Carter, Angus Jackman, Rhomayne Li, and Kyle Hallett. We are grateful to the Guyana Environmental Protection Agency, Ministry of Indigenous People's Affairs, Protected Areas Commission and the Kanuku Mountains Community Representative Group for issuing permission and permits to carry out this study. Lastly, we ever grateful to the leadership and residents of Katoka (Simonie), Maruranau, Moco Moco, Nappi (Parishara, Hiowa), Parikwaranau, Rupunau, Sand Creek, Shulinab (Meriwau, Quiko), Shea, St. Ignatius (Kumu, Quarrie), and Yupukari (Fly Hill, Quatatta, Kaicumbay) villages for their overwhelming kindness, hospitality, generosity, cooperation, support, and participation, without which none of this study could have been carried out.
Supplementary Material
The Supplementary Material for this article can be found online at: https://www.frontiersin.org/articles/10.3389/fevo.2019.00412/full#supplementary-material
References
Altrichter, M., Taber, A., Beck, H., Reyna-Hurtado, R., Lizarraga, L., Keuroghlian, A., et al. (2012). Range-wide declines of a key Neotropical ecosystem architect, the near threatened white-lipped peccary Tayassu pecari. Oryx 46, 87–98. doi: 10.1017/S0030605311000421
Anadón, J. D., Giménez, A., Ballestar, R., and Pérez, I. (2009). Evaluation of local ecological knowledge as a method for collecting extensive data on animal abundance. Conserv. Biol. 23, 617–625. doi: 10.1111/j.1523-1739.2008.01145.x
Arino, O., Ramos Perez, J. J., Kalogirou, V., Bontemps, S., Defourny, P., and Van Bogaert, E. (2012). Global Land Cover Map for 2009 (GlobCover 2009). European Space Agency (ESA); Université Catholique de Louvain (UCL). doi: 10.1594/PANGAEA.787668
Arita, H. T., Robinson, J. G., and Redford, K. H. (1990). Rarity in Neotropical forest mammals and its ecological correlates. Conserv. Biol. 4, 181–192. doi: 10.1111/j.1523-1739.1990.tb00107.x
Beck, H., Snodgrass, J. W., and Thebpanya, P. (2013). Long-term exclosure of large terrestrial vertebrates: implications of defaunation for seedling demographics in the Amazon rainforest. Biol. Conserv. 163, 115–121. doi: 10.1016/j.biocon.2013.03.012
Bodmer, R. E. (1989). Ungulate biomass in relation to feeding strategy within Amazonian forests. Oecologia 81, 547–550. doi: 10.1007/BF00378967
Bovolo, C. I., Pereira, R., Parkin, G., Kilsby, C., and Wagner, T. (2012). Fine-scale regional climate patterns in the Guianas, tropical South America, based on observations and reanalysis data. Int. J. Climatol. 32, 1665–1689. doi: 10.1002/joc.2387
Brown, J., and Davidson, D. (1977), Competition between seed-eating rodents ants in desert ecosystems. Science 196, 880–882. doi: 10.1126/science.196.4292.880
Bureau of Statistics (Guyana). (2016). 2012 Population & Housing Census. Georgetown, Guyana: Bureau of Statistics, 1–66.
Cardillo, M., Mace, G. M., Jones, K. E., Bielby, J., Bininda-Emonds, O. R., Sechrest, W., et al. (2005). Multiple causes of high extinction risk in large mammal species. Science 309, 1239–1241. doi: 10.1126/science.1116030
Clark, C. W. (1990). Mathematical Bioeconomics: The Optimal Management of Renewable Resources. New York, NY: John Wiley.
Cullen, L Jr, Bodmer, R. E., and Pádua, C. V. (2000). Effects of hunting in habitat fragments of the Atlantic forests, Brazil. Biol. Conserv. 95, 49–56. doi: 10.1016/S0006-3207(00)00011-2
de Oliveira, T. G., and Pereira, J. A. (2014). Intraguild predation and interspecific killing as structuring forces of carnivoran communities in South America. J. Mammal. Evol. 21, 427–436. doi: 10.1007/s10914-013-9251-4
de Oliveira, T. G., Tortato, M. A., Silveira, L., Kasper, C. B., Mazim, F. D., Lucherini, M., et al. (2010). “Ocelot ecology and its effect on the small-felid guild in the lowland neotropics,” in Biology and Conservation of Wild Felids, eds D. W. Macdonald and A. J. Loveride (Oxford, UK: Oxford University Press, 559–580.
De Souza-Mazurek, R. R., Pedrinho, T., Feliciano, X., Hilário, W., Gerôncio, S., and Marcelo, E. (2000). Subsistence hunting among the Waimiri Atroari Indians in central Amazonia, Brazil. Biodivers. Conserv. 9, 579–596. doi: 10.1023/A:1008999201747
De Thoisy, B., Da Silva, A. G., Ruiz-García, M., Tapia, A., Ramirez, O., Arana, M., et al. (2010). Population history, phylogeography, and conservation genetics of the last Neotropical mega-herbivore, the lowland tapir (Tapirus terrestris). BMC Evol. Biol. 10:278. doi: 10.1186/1471-2148-10-278
Desbiez, A. L. J., Santos, S. A., Keuroghlian, A., and Bodmer, R. E. (2009). Niche partitioning among white-lipped peccaries (Tayassu pecari), collared peccaries (Pecari tajacu), and feral pigs (Sus scrofa). J. Mammal. 90, 119–128. doi: 10.1644/08-MAMM-A-038.1
DeWalt, S. J., and Chave, J. (2004). Structure and biomass of four lowland Neotropical forests. Biotropica 36, 7–19. doi: 10.1111/j.1744-7429.2004.tb00291.x
Di Bitetti, M. S., Paviolo, A., Ferrari, C. A., De Angelo, C., and Di Blanco, Y. (2008). Differential responses to hunting in two sympatric species of brocket deer (Mazama americana and M. nana). Biotropica 40, 636–645. doi: 10.1111/j.1744-7429.2008.00413.x
Dorazio, R. M., Gotelli, N. J., and Ellison, A. M. (2011). “Modern methods of estimating biodiversity from presence-absence surveys,” in Biodiversity Loss in a Changing Planet, eds G. Venora, O. Grillo, and J. Lopez-Pujol (Rijeka: InTech, 277–302.
Dorazio, R. M., and Royle, J. A. (2005). Estimating size and composition of biological communities by modeling the occurrence of species. J. Am. Stat. Assoc. 100, 389–398. doi: 10.1198/016214505000000015
Dubost, G. (1988). Ecology and social life of the red acouchi, Myoprocta exilis; comparison with the orange-rumped agouti, Dasyprocta leporine. J. Zool. Lond. 214, 107–123. doi: 10.1111/j.1469-7998.1988.tb04990.x
Dubost, G., and Henry, O. (2006). Comparison of diets of the acouchi, agouti and paca, the three largest terrestrial rodents of French Guianan forests. J. Trop. Biol. 22, 641–651. doi: 10.1017/S0266467406003440
Eden, M. J., and McGregor, D. F. M. (1992). “Dynamics of the forest-savanna boundary in the Rio Branco-Rupununi region of northern Amazonia,” in Nature and Dynamics of Forest-Savanna Boundaries, eds P. A. Furley, J. Proctor, and J. A. Ratter (London: Chapman and Hall, 77–88.
Environmental Systems Research Institute (ESRI). (2015). ArcGIS Release 10.3.1. Redlands, CA: Environmental Systems Research Institute.
Fa, J. E., and Brown, D. (2009). Impacts of hunting on mammals in African tropical moist forests: a review and synthesis. Mamm. Rev. 39, 231–264. doi: 10.1111/j.1365-2907.2009.00149.x
Fa, J. E., and Peres, C. A. (2001). “Game vertebrate extraction in African and Neotropical forests: an intercontinental comparison,” in Conservation of Exploited Species, eds J. Reynolds, G. Mace, J. G. Robinson, and K. Redford (Cambridge, UK: Cambridge University Press, 203–241.
Fa, J. E., Peres, C. A., and Meeuwig, J. (2002). Bushmeat exploitation in tropical forests: an intercontinental comparison. Conserv. Biol. 16, 232–237. doi: 10.1046/j.1523-1739.2002.00275.x
Fa, J. E., and Purvis, A. (1997). Body size, diet and population density in Afrotropical forest mammals: a comparison with neotropical species. J. Anim. Ecol. 66, 98–112. doi: 10.2307/5968
FAO (2015). The State of Food & Agriculture in the Rupununi, Guyana: Imperatives for Sustainable Livelihoods and Development. Georgetown: Conservation International Guyana.
Fragoso, J. M., Levi, T., Oliveira, L. F., Luzar, J. B., Overman, H., Read, J. M., et al. (2016). Line transect surveys underdetect terrestrial mammals: implications for the sustainability of subsistence hunting. PLoS ONE 11:e0152659. doi: 10.1371/journal.pone.0152659
Gaynor, K. M., Hojnowski, C. E., Carter, N. H., and Brashares, J. S. (2018). The influence of human disturbance on wildlife nocturnality. Science 360, 1232–1235. doi: 10.1126/science.aar7121
Gelman, A., Carlin, J. B., Stern, H. S., and Rubin, D. B. (1995). Bayesian Data Analysis. New York, NY: Chapman and Hall. doi: 10.1201/9780429258411
Gelman, A., and Hill, J. (2007). Data Analysis Using Regression and Multilevel/Hierarchical Models. New york, NY: Cambridge University Press. doi: 10.1017/CBO9780511790942
Gilchrist, G., Mallory, M., and Merkel, F. (2005). Can local ecological knowledge contribute to wildlife management? Case studies of migratory birds. Ecol. Soc. 10:20. doi: 10.5751/ES-01275-100120
Gilks, W. R., Rochardson, S., and Spiegelhalter, D. J. (1996). “Introducing Markov chain Monte Carlo,” in Markov Chain Monte Carlo Methods in Practice, eds W. R. Gilks, S. Rochardson, and D. J. Spiegelhalter (New York, NY: Chapman and Hall), 1–20. doi: 10.1201/b14835
Hansen, M. C., Potapov, P. V., Moore, R., Hancher, M., Turubanova, S. A. A., Tyukavina, A., et al. (2013). High-resolution global maps of 21st-century forest cover change. Science 342, 850–853. doi: 10.1126/science.1244693
Harmsen, B. J., Foster, R. J., Silver, S., Ostro, L., and Doncaster, C. P. (2010). Differential use of trails by forest mammals and the implications for camera-trap studies: a case study from Belize. Biotropica 42, 126–133. doi: 10.1111/j.1744-7429.2009.00544.x
M.. Hutchins, D. G.. Kleiman, V.. Geist, and M. C.. McDade (eds.). (2003). Grzimek's Animal Life Encyclopedia, 2nd Edn, Vol. 12–16. Farmington Hills, MI: Gale Group.
Iwamura, T., Lambin, E. F., Silvius, K. M., Luzar, J. B., and Fragoso, J. M. (2016). Socio–environmental sustainability of indigenous lands: simulating coupled human–natural systems in the Amazon. Front. Ecol. Environ. 14, 77–83. doi: 10.1002/fee.1203
Jerozolimski, A., and Peres, C. A. (2003). Bringing home the biggest bacon: a cross-site analysis of the structure of hunter-kill profiles in Neotropical forests. Biol. Conserv. 111, 415–425. doi: 10.1016/S0006-3207(02)00310-5
Karanth, K. U., and Nichols, J. D. (1998). Estimation of tiger densities in India using photographic captures and recaptures. Ecology 79, 2852–2862. doi: 10.1890/0012-9658(1998)079[2852:EOTDII]2.0.CO;2
Kilgo, J. C., Labisky, R. F., and Fritzen, D. E. (1998). Influences of hunting on the behavior of white-tailed deer: implications for conservation of the Florida panther. Conserv. Biol. 12, 1359–1364. doi: 10.1046/j.1523-1739.1998.97223.x
Kolowski, J. M., and Alonso, A. (2010). Density and activity patterns of ocelots (Leopardus pardalis) in northern Peru and the impact of oil exploration activities. Biol. Conserv. 143, 917–925. doi: 10.1016/j.biocon.2009.12.039
Laurance, W. F., Croes, B. M., Tchignoumba, L., Lahm, S. A., Alonso, A., Lee, M. E., et al. (2006). Impacts of roads and hunting on central African rainforest mammals. Conserv. Biol. 20, 1251–1261. doi: 10.1111/j.1523-1739.2006.00420.x
Le Saout, S., Hoffmann, M., Shi, Y., Hughes, A., Bernard, C., Brooks, T. M., et al. (2013). Protected areas and effective biodiversity conservation. Science 342, 803–805. doi: 10.1126/science.1239268
Licona, M., McCleery, R., Collier, B., Brightsmith, D. J., and Lopez, R. (2011). Using ungulate occurrence to evaluate community-based conservation within a biosphere reserve model. Anim. Conserv. 14, 206–214. doi: 10.1111/j.1469-1795.2010.00416.x
Loughry, J., McDonough, C., and Abba, A. M. (2014). Dasypus novemcinctus. The IUCN Red List of Threatened Species 2014: e.T6290A47440785. Downloaded on 20 September 2019.
Luzar, J. B., Silvius, K. M., and Fragoso, J. M. (2012). Church affiliation and meat taboos in indigenous communities of Guyanese Amazonia. Hum. Ecol. 40, 833–845. doi: 10.1007/s10745-012-9521-4
MacKenzie, D. I., Nichols, J. D., Lachman, G. B., Droege, S., Royle, J. A., and Langtimm, C. A. (2002). Estimating site occupancy rates when detection probabilities are less than one. Ecology 83, 2248–2255. doi: 10.1890/0012-9658(2002)083[2248:ESORWD]2.0.CO;2
Maxwell, S. L., Fuller, R. A., Brooks, T. M., and Watson, J. E. M. (2016). Biodiversity: the ravages of guns, nets and bulldozers. Nature 536, 143–145. doi: 10.1038/536143a
Mayor, P., Guimaraes, D. A., Le Pendu, Y., Da Silva, J. V., Jori, F., and Lopez-Béjar, M. (2007). Reproductive performance of captive collared peccaries (Tayassu tajacu) in the eastern Amazon. Anim. Reprod. Sci. 102, 88–97. doi: 10.1016/j.anireprosci.2006.10.015
Mena, V. P., Stallings, J. R., Regalado, J. B., and Cueva, R. L. (2000). “The sustainability of current hunting practices by the Huaorani,” in Hunting for Sustainability in Tropical Forests, eds J. G. Robinson and E. L. Bennett (New York, NY: Columbia University Press, 57–78.
Milner-Gulland, E. J., Bunnefeld, N., and Proaktor, G. (2009). “The science of sustainable hunting,” in Recreational Hunting, Conservation and Rural Livelihoods: Science and Practice, eds B. Dickson, J. Hutton, and W. M. Adams. (Oxford: Blackwell Publishing Ltd., 75–93.
J. R.. Montambault, and O.. Massa (eds.). (2002). “A biodiversity assessment of the Eastern Kanuku Mountains, Lower Kwitaro River, Guyana,” in Conservation International Rapid Assessment Program (RAP) Working Papers 26 (Georgetown), 1–86.
Nasi, R., Taber, A., and Van Vliet, N. (2011). Empty forests, empty stomachs? Bushmeat and livelihoods in the Congo and Amazon Basins. Int. Forestry Rev. 13, 355–368. doi: 10.1505/146554811798293872
O'Brien, T. G. (2011). “Abundance, density and relative abundance: a conceptual framework,” in Camera-Traps in Animal Ecology, eds A. F. O'Connell, J. D. Nichols, and K. U. Karanth (Tokyo: Springer), 71–96. doi: 10.1007/978-4-431-99495-4_6
Peres, C. A. (2001). Synergistic effects of subsistence hunting and habitat fragmentation on Amazonian forest vertebrates. Conserv. Biol. 15, 1490–1505. doi: 10.1046/j.1523-1739.2001.01089.x
Peres, C. A., and Dolman, P. M. (2000). Density compensation in neotropical primate communities: evidence from 56 hunted and nonhunted Amazonian forests of varying productivity. Oecologia 122, 175–189. doi: 10.1007/PL00008845
Peres, C. A., and van Roosmalen, M. (2002). “Primate frugivory in two species-rich Neotropical forests: implications for the demography of large-seeded plants in overhunted areas,” in Seed Dispersal and Frugivory: Ecology, Evolution and Conservation, eds D. J. Levey, W. R. Silva, and M. Galetti (Wallingford: CABI Publishing), 407–421. doi: 10.1079/9780851995250.0407
Phan, T. D., Baxter, G. S., Phan, H. A., Mai, L. S., and Trinh, H. D. (2019). An integrated approach for predicting the occurrence probability of an elusive species - the Southwest China serow. Wildl. Res. 4, 386–397. doi: 10.1071/WR18116
Plummer, M. (2011). JAGS: A Program for the Statistical Analysis of Bayesian Hierarchical Models by Markov Chain Monte Carlo. Available online at: http://sourceforge.net/projects/mcmc-jags/ (accessed April 2019).
Puran, A., Paemelaere, E. A. D, and Ali, R. (2017). “Wildmeat consumption on the coast of Guyana,” in 28th International Congress for Conservation Biology (Cartagena, Colombia).
Redford, K. H., and Robinson, J. G. (1987). The game of choice: patterns of Indian and colonist hunting in the Neotropics. Am. Anthropol. 89, 650–667. doi: 10.1525/aa.1987.89.3.02a00070
Ridout, M. S., and Linkie, M. (2009). Estimating overlap of daily activity patterns from camera-trap data. J. Agric. Biol. Environ. Stat. 14, 322–337. doi: 10.1198/jabes.2009.08038
Ripple, W. J., Abernethy, K., Betts, M. G., Chapron, G., Dirzo, R., Galetti, M., et al. (2016). Bushmeat hunting and extinction risk to the world's mammals. R. Soc. Open Sci. 3:160498. doi: 10.1098/rsos.160498
Robinson, J. G., and Bennett, E. L. (2000). “Carrying capacity limits to sustainable hunting in tropical forests,” in Hunting for Sustainability in Tropical Forests, eds J. G. Robinson and E. L. Bennett (New York, NY: Columbia University Press, 13–30.
Rocha, V. J., Aguiarll, L. M., Silva-Pereirall, J. E., Moro-Riosll, R. F., and Passosll, F. C. (2008) Feeding habits of the crab-eating fox, Cerdocyon thous (Carnivora: Canidae), in a mosaic area with native exotic vegetation in Southern Brazil. Rev. Bras. Zool. 25:594–600. doi: 10.1590/S0101-81752008000400003
Rushton, J., Viscarra, R., Viscarra, C., Basset, F., Baptista, R., and Brown, D. (2005). How important is bushmeat consumption in South America: now and in the future. Odi Wildl. Policy Brief. 11, 1–4. Available online at: http://www.odi.org.uk/resources/download/2418.pdf (accessed March 10, 2019).
Russell, R. E., Royle, J. A., Saab, V. A., Lehmkuhl, J. F., Block, W. M., and Sauer, J. R. (2009). Modeling the effects of environmental disturbance of wildlife communities: avian responses to prescribed fire. Ecol. Appl. 19, 1253–1263. doi: 10.1890/08-0910.1
Silver, S. (2004). Assessing Jaguar Abundance Using Remotely Triggered Cameras. New York, NY: Wildlife Conservation Society. Available online at: https://s3.amazonaws.com/academia.edu.documents/32342673/Manual_for_jaguar_phototraping_WCS.pdf (accessed December 15, 2018).
Stoner, K. E., Vulinec, K., Wright, S. J., and Peres, C. A. (2007). Hunting and plant community dynamics in tropical forests: a synthesis and future directions. Biotropica 39, 385–392. doi: 10.1111/j.1744-7429.2007.00291.x
Superina, M., Pagnutti, N., and Abba, A. M. (2014). What do we know about armadillos? An analysis of four centuries of knowledge about a group of South American mammals, with emphasis on their conservation. Mamm. Rev. 44, 69–80. doi: 10.1111/mam.12010
Terborgh, J., Lopez, L., Nuñez, P., Rao, M., Shahabuddin, G., Orihuela, G., et al. (2001). Ecological meltdown in predator-free forest fragments. Science 294, 1923–1926. doi: 10.1126/science.1064397
Terrien, J., Perret, M., and Aujard, F. (2011). Behavioral thermoregulation in mammals: a review. Front. Biosci. 16, 1428–1444. doi: 10.2741/3797
Tobler, M. V, Carrillo-Percastegui, S. E., and Powell, G. (2009). Habitat use, activity patterns and use of mineral licks by five species of ungulate in south-eastern Peru. J. Trop. Ecol. 25, 261–270. doi: 10.1017/S0266467409005896
Tobler, M. V. (2002). Habitat use and diet of Baird's tapirs (Tapirus bairdii) in a montane cloud forest of the Cordillera de Talamanca, Costa Rica. Biotropica 34, 468–474. doi: 10.1111/j.1744-7429.2002.tb00563.x
Tobler, M. V., Hibert, F., Debeir, L., and Richard-Hansen, C. (2014). Estimates of density and sustainable harvest of the lowland tapir (Tapirus terrestris) in the Amazon of French Guiana using a Bayesian spatially explicit capture–recapture model. Oryx 48, 410–419. doi: 10.1017/S0030605312001652
Varela, D., Flesher, K., Cartes, J. L., de Bustos, S., Chalukian, S., and Ayala, G. Richard-Hansen, C. (2019). Tapirus Terrestris. The IUCN Red List of Threatened Species 2019: e.T21474A45174127.en. Downloaded on 20 September 2019.
Walters, C. J., and Holling, C. S. (1990). Large-scale management experiments and learning by doing. Ecology 71, 2060–2068. doi: 10.2307/1938620
Waterman, P. G., and McKey, D. (1989) “Herbivory secondary compounds in rain-forest plants,” in Tropical Rain Forest Ecosystems: Biogeographical and Ecological Studies, eds H. Lieth M. J. A. Erger (Amsterdam/New York, NY: Elsevier), 513–536. doi: 10.1016/B978-0-444-42755-7.50035-3
Weber, M., and Gonzalez, S. (2003). Latin American deer diversity and conservation: a review of status and distribution. Ecoscience 10, 443–454. doi: 10.1080/11956860.2003.11682792
Weckel, M., Giuliano, W., and Silver, S. (2006). Jaguar (Panthera onca) feeding ecology: distribution of predator and prey through time and space. J. Zool. 270, 25–30. doi: 10.1111/j.1469-7998.2006.00106.x
Wilkie, D. S., Bennett, E. L., Peres, C. A., and Cunningham, A. A. (2011). The empty forest revisited. Ann. N. Y. Acad. Sci. 1223, 120–128. doi: 10.1111/j.1749-6632.2010.05908.x
Wilkie, D. S., Shaw, E., Rotberg, F., Morelli, G., and Auzel, P. (2000). Roads, development, and conservation in the Congo Basin. Conserv. Biol. 14, 1614–1622. doi: 10.1111/j.1523-1739.2000.99102.x
Wright, S. J., Hernandez, A., and Condit, R. (2007). The bushmeat harvest alters seedling banks by favoring lianas, large seeds, and seeds dispersed by bats, birds, and wind. Biotropica 39, 363–371. doi: 10.1111/j.1744-7429.2007.00289.x
Young, H. S., McCauley, D. J., Galetti, M., and Dirzo, R. (2016). Patterns, causes, and consequences of anthropocene defaunation. Annu. Rev. Ecol. Evol. Syst. 47, 333–358. doi: 10.1146/annurev-ecolsys-112414-054142
Zabel, C. J., Roberts, L. M., Mulder, B. S., Stauffer, H. B., Dunk, J. R., Wolcott, K., et al. (2002). “A collaborative approach in adaptive management at a large landscape scale,” in Predicting Species Occurrence: Issues of Accuracy and Scale, eds J. M. Stott, P. J. Heglund, M. L. Morrison, J. B. Haufler, M. G. Raphael, W. A. Wall, and F. B. Samson (Washington, DC: Island Press, 241–253.
Keywords: bushmeat, hunting intensity, protected areas, indigenous lands, Kanuku Mountains, Guyana, large mammals, Rupununi
Citation: Hallett MT, Kinahan AA, McGregor R, Baggallay T, Babb T, Barnabus H, Wilson A, Li FM, Boone WW and Bankovich BA (2019) Impact of Low-Intensity Hunting on Game Species in and Around the Kanuku Mountains Protected Area, Guyana. Front. Ecol. Evol. 7:412. doi: 10.3389/fevo.2019.00412
Received: 05 January 2019; Accepted: 15 October 2019;
Published: 08 November 2019.
Edited by:
Robert Nasi, Center for International Forestry Research, IndonesiaReviewed by:
Charlotte H. Chang, National Institute for Mathematical and Biological Synthesis (NSF), United StatesFiona Maisels, Wildlife Conservation Society, United States
Copyright © 2019 Hallett, Kinahan, McGregor, Baggallay, Babb, Barnabus, Wilson, Li, Boone and Bankovich. This is an open-access article distributed under the terms of the Creative Commons Attribution License (CC BY). The use, distribution or reproduction in other forums is permitted, provided the original author(s) and the copyright owner(s) are credited and that the original publication in this journal is cited, in accordance with accepted academic practice. No use, distribution or reproduction is permitted which does not comply with these terms.
*Correspondence: Matthew T. Hallett, bWhhbGxldHQyMzIwQHVmbC5lZHU=