- 1Department of Biology, University of Toronto Mississauga, Mississauga, ON, Canada
- 2Ecology and Evolutionary Biology, University of Toronto, Toronto, ON, Canada
Salinization of freshwater ecosystems, due to the application of road salts, is recognized as a potential threat to aquatic communities. Much of the research on the impact of salinity has focused on performance metrics in vertebrates, including respiration and osmoregulation. Here we focus on immune function in larvae of the dragonfly Anax junius, a top predator in fishless aquatic habitats. Impacts on this top predator have the potential to cascade through the community, and immune function is known to be both plastic and sensitive to stress. We injected larvae with monofilaments (simulating a parasite) and placed them in one of three environmentally relevant concentrations of deicing road salt: control (dechlorinated tap water with no added salt), low (1,000 mgL−1), or high salt (3,000 mgL−1), for either acute (24 h) or chronic (96 h) exposure. We hypothesized that elevated salinity would suppress the immune response and that longer exposure magnifies this effect. As predicted, chronic exposure to high salt concentrations resulted in significantly reduced larval immune response compared to control conditions, however, there were no detectable treatment effects in larvae exposed to low concentrations of road salt or to acute high concentrations. Our results demonstrate that prolonged exposure to high levels of road salt can compromise the immune response of dragonfly larvae. Our findings suggest that insects in aquatic environments that experience sustained environmental salt pollution will be more susceptible to parasites, which in turn may affect the impact of this major predator on aquatic community dynamics.
Introduction
Salinization of freshwater ecosystems, resulting from the transfer of winter road salts into aquatic systems through meltwater (Kaushal et al., 2005; Novotny et al., 2008; Dugan et al., 2017), has become a major concern in freshwater conservation (Cañedo-Argüelles et al., 2013; Herbert et al., 2015). Previous research found that salinization of freshwater systems from the increasing use of deicing salt can negatively affect the performance of freshwater organisms (Bernabò et al., 2013; Kearney et al., 2014; Dananay et al., 2015; Hopkins et al., 2016), and threaten the viability of some populations (Silberbush et al., 2005; Petranka and Doyle, 2010; Van Meter et al., 2011; Searle et al., 2016; Castillo et al., 2018; Timpano et al., 2018). Salinization can impose physiological stresses for freshwater organisms including impaired osmoregulation (Marshall and Grosell, 2006; Griffith, 2016; Sala et al., 2016; Scheibener et al., 2016, 2017) and decreased respiratory performance (Roast et al., 1999; McAllen and Taylor, 2001; Tollefsen et al., 2015; Botwe et al., 2018). Consequently, road salt has been recognized as a potentially harmful substance and added to the “List of Toxic Substances” in Canada (Environment Canada, 2012).
The majority of studies examining the biological effects of salinization in freshwater systems have focused on vertebrates. Amphibians have been found to be negatively affected by increased salinity with reduced survival to metamorphosis and longer developmental times (Bernabò et al., 2013; Hopkins et al., 2013; Kearney et al., 2014; Wood and Welch, 2015), reduced activity levels (Wood and Welch, 2015; Jones et al., 2017), decreased sperm motility and velocity (Wilder and Welch, 2014), and physical abnormalities (Sanzo and Hecnar, 2006) including morphological alterations of gill epithelium following salinity exposure (Bernabò et al., 2013). Key fitness metrics, including reduced body length and mass, have also been reported in fish exposed to high salinity (e.g., Hintz and Relyea, 2017).
Although less extensively studied than the effects of salt on vertebrates, negative effects of salinization on freshwater invertebrates have also been reported. For example, exposure to dissolved salts resulted in slower growth rates of macroinvertebrate detrivores (Tyree et al., 2016) and reduced hatching rate of diapausing eggs of zooplankton species (Bailey et al., 2004). The stress induced by non-lethal levels of salt can interact with other stressors, such as parasite infections, to induce harmful effects in organisms. Daphnia magna infected with parasites and exposed to saline conditions produced fewer offspring than uninfected individuals exposed to the same range of salinities (Hall et al., 2013). These impacts may result from multiple stressors associated with salinization. For example, in addition to directly influencing osmoregulation, salinization reduces the capacity of water to hold dissolved oxygen and, thus, may lead to respiratory stress. Moreover, the increased investment required to maintain homeostasis during salt stress may lead to decreased investment in other crucial physiological functions and, thus, diffuse negative effects on organismal performance.
Here, we conducted an experimental investigation of the effect of deicing road salt exposure on immune function in the aquatic larvae of the dragonfly, Anax junius (Odonata: Aeshnidae). Immune function of these top predators may be particularly sensitive to salt stress because of their relatively large body size and high activity levels (e.g., Folsom and Collins, 1984). Additionally, freshwater invertebrates such as dragonfly larvae that breathe using tracheal gills are more vulnerable to hypoxic conditions than invertebrates with other respiratory modes (Chapman et al., 2004; Novotny and Stefan, 2012). Immunity is known to be both highly plastic and sensitive to stress (Adamo, 2017) and its expression will also affect the ability of parasites and pathogens to infect hosts and take up resources from them, influencing host and pathogen populations and pathogen transmission dynamics (Schmid-Hempel, 2005a,b; Yourth et al., 2011). Finally, our focal species, A. junius larvae, is an important predator in aquatic habitats and can act as a top predator in fishless ponds (McPeek, 1990, 1998). Therefore, changes in the survival or performance of this species may have cascading effects throughout aquatic communities.
In this study we tested for an effect of salinity stress on the melanin immune response in larval A. junius dragonflies. We used sub-lethal concentrations of salt (not higher than 3,000 mgL−1) that are environmentally realistic for the region from which we collected our specimens (Environment Canada, 2001). Additionally we tested for an effect of acute vs. chronic salinity exposure on the larvae's melanization immune response.
Methods
Specimen Collection
Larvae were collected from a fishless pond at University of Toronto's Koffler Scientific Reserve in King City, Ontario, Canada (44° 01' 48” N, 79° 32' 01”W) in October 2017, and transferred in buckets to a laboratory at the University of Toronto (Mississauga, Ontario). Larvae were kept individually in plastic cups filled with aged, de-chlorinated water at room temperature for 3 weeks prior to experiments. Larvae were fed ad libitum with prey collected from a local pond. Waste and uneaten food were removed prior to feeding. Food types included zooplankton, smaller larval odonates and mayfly larvae that were given to A. junius larvae daily.
Experimental Set-Up
This study varied two conditions: concentration (low: 1,000 mgL−1 or high: 3,000 mgL−1) and duration (acute: 24 h or chronic: 96 h) of deicing salt exposure for larval dragonflies. These two conditions were fully crossed, and compared to A. junius larvae held in control conditions with no road salt exposure. Larvae were randomly allocated to the five treatments (four salt conditions and the control): low concentration-acute exposure (n = 16), low concentration-chronic exposure (n = 17), high concentration-acute exposure (n = 14), high concentration-chronic exposure (n = 16) and control (n = 15). We included head width (as a proxy for body size) in our analyses because melanization can vary with body size in some species (e.g., Rantala and Roff, 2005; Kelly and Jennions, 2009). The head width and body length (mm) of each larva was measured using digital calipers (accurate to 0.02 mm) and recorded prior to placing the larva into the experiment (mean head width ± SE: 5.02 ± 0.07; mean body length ± SE: 32.12 ± 1.86).
Salt water was prepared by dissolving Windsor Safe-T-Salt deicing salt, primarily composed of NaCl, in de-chlorinated water at experimental concentrations. These concentrations were chosen based on ecologically relevant chloride concentrations at peak periods of road salt influx into freshwater systems based on previous work (Environment Canada, 2001; Canadian Council of Ministers of the Environment (CCME), 2011). Aquarium heaters were added to heat up salt solutions to ~32°C, to speed up the process of dissolving these salts in water. The salt water was then cooled down to room temperature (~22°C) before the start of experimental trials. During trials, larvae were held individually in plastic cups filled with 250 mL of water with one of the three salt concentration treatments.
Data Collection
Like most insects, dragonfly larvae respond to infection with an internal cellular defense (Schmid-Hempel, 2005b), which includes encapsulating the pathogen and/or parasite with melanized hemocytes (Christensen et al., 2005). To test how different levels of salinization from road salt affected the level of melanization of a simulated parasite, A. junius larvae were injected with 1 mm sanded monofilaments (diameter 0.20 mm) using a single-shot tag injector (Northwest Marine Technology, Inc.). This approach provides a standard measure of this aspect of immune function (Köning and Schmid-Hempel, 1995; Rantala and Roff, 2007), and has been used successfully in odonate larvae (e.g., Nagel et al., 2011; Duong and McCauley, 2016; Ilvonen and Suhonen, 2016).
Monofilaments were injected into the seventh abdominal segment, dorsolaterally and perpendicular to the cuticle suture as in Duong and McCauley (2016). Larvae were then randomly assigned to one of the five treatments (control, Low-Acute, Low-Chronic, High-Acute, High-Chronic). After 24 h, larvae in the acute exposure treatment were removed from the salt water and placed in de-chlorinated tap water, and those under chronic exposure remained in their salt water treatment. Therefore, A. junius larvae in the acute exposure treatment were in the salt water for only 24 h, followed by a 72 h recovery period in non-salinized water, while those in chronic exposure experienced a total of 96 h in salt water conditions, and control treatment animals spent 96 h in non-salinized water. Ninety-six hours after the start of the experiment, injected monofilaments were removed from all larvae using tweezers, and placed in 2.0 mL microcentrifuge tubes and stored in a −20°C freezer. Two pictures of each monofilament were taken (rotated 180 degrees lengthwise) with an Infinity 1 camera mounted on an Olympus SZ61 microscope. Photos of monofilaments were then analyzed using ImageJ software, where pictures were converted into 32-bit grayscale images, and the melanin sections of the monofilament were measured. The mean level of melanization (expressed in pixel values) was recorded using a 0–255 scale (255 corresponds to white and 0 corresponds to black). The mean value of both sides of the monofilament was taken. We subtracted the measured melanization value from 255 to convert the scale so that lower values indicated less melanization.
Statistical Analyses
We standardized the continuous measure of melanization using the scale function in R (R Core Development Team). We fit a linear model to assess if there was a significant effect of deicing salt on melanization in A. junius larvae. The model fit melanization as the response, treatment (Control, Low-Acute, Low-Chronic, High-Acute, High-Chronic) and individual head width as fixed effects. Additionally, we performed an ANOVA that fit head width as well as concentration, exposure, and their interaction as predictors of melanization. All analyses were conducted in R version 3.1.2 (R Core Development Team).
Results
We found a significantly decreased melanization response in individuals chronically exposed to high (3,000 mgL−1) concentrations of deicing road salt compared to the control treatment (p = 0.034; Table 1, Figure 1). There was no significant decrease in melanization for individuals exposed to low salt concentrations regardless of exposure type, or for individuals exposed to acute, high concentrations compared to the control treatment (p > 0.05; Table 1). Larval head width was not a significant predictor of melanization response (Table 1). We did not see an effect of salinity treatment type (exposure or concentration) or their interaction on melanization (Table S1).
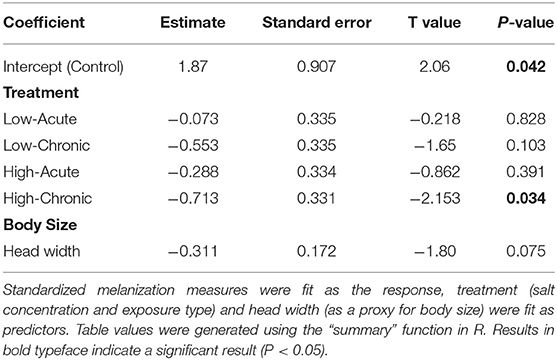
Table 1. Results from a linear model testing for an association of deicing salt treatment (exposure and salt concentration) and body size on the melanization immune response in larval Anax junius dragonflies.
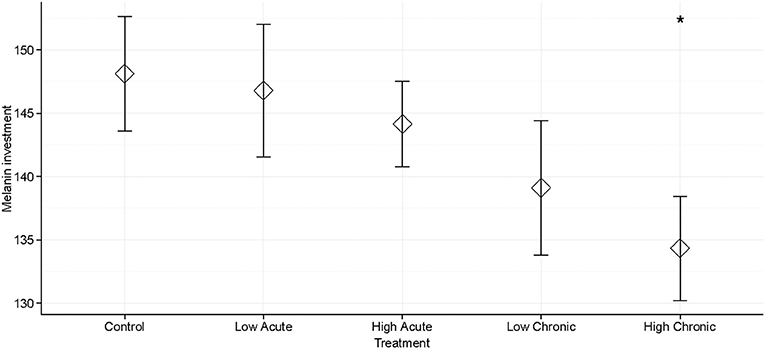
Figure 1. Mean melanization of monofilaments for Anax junius dragonfly larvae across five deicing salt stress treatments: control (no added salt treatment); low concentration, acute exposure; low concentration, chronic exposure; high concentration, acute exposure; high concentration, chronic exposure. Error bars show the standard error around the mean. Melanization was significantly decreased in the High Chronic treatment compared to the control treatment (*P < 0.05).
Discussion
Larval dragonflies exposed to high concentrations of deicing salt for 4 days significantly decreased their melanin immune response to a simulated parasite compared with larvae exposed to control conditions in a laboratory setting (Table 1, Figure 1). We did not see a significant effect of either treatment type (salt concentration or exposure time) or their interaction in an ANOVA (Table S1), and there was no effect of head width on larval melanization (Table 1, Table S1). Our findings show that exposure to salt pollution at environmentally realistic concentrations for less than a week can compromise dragonfly larvae's ability to initiate a cellular defense against a foreign body (Figure 1). Depression of the melanization response could make larval dragonflies more vulnerable to parasites and suggests that salinity may induce a generalized stress response, which has previously been associated with decreased immune responses in insects (Adamo, 2017).
Studies of laboratory salinity tolerance (survival) have been shown to correlate with natural salinity tolerances across species (Kefford et al., 2004). These studies primarily focus on determining the lethal levels of salinity exposure. Many salt tolerance studies (both in the lab and the field), however, have not examined salinity levels that cause sub-lethal effects, which can also have dramatic consequences for organisms (but see Hintz and Relyea, 2019). The depressed melanization in the High-Chronic salt treatment that we observed is likely part of a larger suite of sub-lethal effects of salinity on freshwater invertebrates. While we did not see overall effects of exposure time and concentration, understanding how these two important salinity pollution factors interact on a larger time scale (longer chronic exposure time) will be important for understanding the long-term consequences of even low levels of road salt pollution for freshwater organisms and the functioning of these ecosystems.
Sub-lethal effects of salinity pollution, such as those observed in this study, likely have consequences for higher levels of ecological organization (Hintz and Relyea, 2019). The physiological stress of salinization in freshwater ecosystems can affect the performance of many aquatic organisms at varying trophic levels (reviewed in Castillo et al., 2018), and have cascading effects that influence the structure of aquatic communities (Silberbush et al., 2005; Petranka and Doyle, 2010; Van Meter et al., 2011; Searle et al., 2016; Timpano et al., 2018; Hintz and Relyea, 2019). By favoring salt tolerant species, salinization could not only alter community structure and affect ecosystem function but will likely drive down biodiversity in places that are particularly at risk of salinization, such as road-adjacent habitats (Collins and Russell, 2009; Stoler et al., 2018; Hintz and Relyea, 2019). Future mesocosm studies looking at how sub-lethal salt pollution affects species abundance, local diversity and community structure will be important for predicting changes to permanent and temporary aquatic habitats.
We compared animals from a relatively small range of larval instars and body sizes, however, the effects of salinization have been shown previously to vary considerably across life stages (e.g., amphibians: Kearney et al., 2014; fish: Hintz and Relyea, 2017). Future studies investigating immunity in the context of road salt contamination across life stages in insects (egg through adult) will be important for beginning to understand some of the evolutionary consequences of long-term salt exposure. Specifically, studies could assess how the developmental stage that insects experience periods of peak salt pollution (e.g., during the spring melt) affects their performance (including growth rate, parasite immunity, metamorphic and reproductive success).
Our study shows that insect hosts will be vulnerable to parasites at relatively short salt exposure times. Within this study “chronic salt exposure” was 4 days long, and we did not see an effect of exposure itself (Table S1), however we know that some freshwater habitats experience heightened salinity throughout the year (see Hintz and Relyea, 2019). Future studies looking at the effect of long-term exposure to salt pollution on host parasite dynamics will be critical to our understanding of how multiple stressors (i.e., salt and parasites) interact to affect insect populations. For example, do hosts and parasites respond similarly to road salt contamination, or are some parasites tolerant to salinity stress? Surveys of parasite loads on aquatic insects in sites that vary in road salt inputs would be useful to assess the impacts of this contaminant on interactions between hosts and parasites in nature.
In addition to affecting host-parasite interactions, freshwater salinization may influence the structure and functioning of freshwater communities and ecosystems through indirect effects on other members of the food web (Silberbush et al., 2005; Petranka and Doyle, 2010; Van Meter et al., 2011; Searle et al., 2016; Castillo et al., 2018; Timpano et al., 2018). Given the high trophic position of A. junius in fishless environments (Crumrine, 2010; Hopkins et al., 2011), depressed immune function in these animals could have important consequences for the community structure of ponds exposed to road salt contamination. Even when the salt levels are not high enough to induce mortality in this species, by facilitating infections by parasites and pathogens, road salt may affect the abundance or behavior of this top predator, altering community structure in both permanent and temporary fishless habitats (McPeek, 1990, 1998).
The contamination of aquatic ecosystems by deicing road salts and how it acts both directly and indirectly to affect populations and communities requires further investigation. We have a growing, but still limited, understanding of the sub-lethal effects of salt pollution on freshwater invertebrates. Our understanding of the more subtle ways that relatively low levels of salt pollution affect freshwater systems is critical to developing predictions about the effects of road salt on community structure and for developing measures to facilitate freshwater conservation and minimize the influence of this contaminant.
Data Availability Statement
The raw data supporting the conclusions of this manuscript will be made available by the authors, without undue reservation, to any qualified researcher.
Author Contributions
RLM and SM designed the study. RSM and RLM collected the data, and RLM analyzed the data. All authors contributed to the writing and revising of the manuscript.
Funding
This research was supported by funding from the Natural Science and Engineering Research Council (RGPIN 435614 to SM) as well as funding from the Department of Biology at UTM to support undergraduate research by RSM.
Conflict of Interest
The authors declare that the research was conducted in the absence of any commercial or financial relationships that could be construed as a potential conflict of interest.
Acknowledgments
Locke Rowe provided extensive support and feedback on this research and manuscript. Two reviewers and SM and Rowe labs provided useful feedback on this study. The Koffler Scientific Reserve provided research support for collecting insects used in these experiments.
Supplementary Material
The Supplementary Material for this article can be found online at: https://www.frontiersin.org/articles/10.3389/fevo.2019.00376/full#supplementary-material
References
Adamo, S. A. (2017). The stress response and immune system share, borrow, and reconfigure their physiological network elements: evidence from the insects. Horm. Behav. 88, 25–30. doi: 10.1016/j.yhbeh.2016.10.003
Bailey, S. A., Duggan, I. C., van Overdijk, C. D. A., Johengen, T. H., Reid, D. F., and MacIsaac, H. J. (2004). Salinity tolerance of diapausing eggs of freshwater0020zooplankton. Freshw. Biol. 49, 286–295. doi: 10.1111/j.1365-2427.2004.01185.x
Bernabò, I., Bonacci, A., Coscarelli, F., Tripepi, M., and Brunelli, E. (2013). Effects of salinity stress on Bufo balearicus and Bufo bufo tadpoles: tolerance, morphological gill alterations and Na+/K+-ATPase localization. Aquat. Toxicol. 132–133, 119–133. doi: 10.1016/j.aquatox.2013.01.019
Botwe, P. K., Carver, S., Magierowski, R., McEvoy, P., Goonan, P., Madden, C., et al. (2018). Effects of salinity and flow interactions on macroinvertebrate traits in temporary streams. Ecol. Indicat. 89, 74–83. doi: 10.1016/j.ecolind.2018.01.036
Canadian Council of Ministers of the Environment (CCME) (2011). Canadian Water Quality Guidelines for the Protection of Aquatic Life: Chloride. Gatineau: Environment Canada.
Cañedo-Argüelles, M., Kefford, B. J., Piscart, C., Prat, N., Schäfer, R. B., and Schulz, C. (2013). Salinisation of rivers: an urgent ecological issue. Environ. Pollut. 173, 157–167. doi: 10.1016/j.envpol.2012.10.011
Castillo, A. M., Sharpe, D. M. T., Ghalambor, C. K., and De Léon, L. F. (2018). Exploring the effects of salinization on trophic diversity in freshwater ecosystems: a quantitative review. Hydrobiologia 807, 1–17. doi: 10.1007/s10750-017-3403-0
Chapman, L. J., Schneider, K. R., Apodaca, C., and Chapman, C. A. (2004). Respiratory ecology of macroinvertebrates in a swamp-river system of east Africa. Biotropica 36, 572–585. doi: 10.1111/j.1744-7429.2004.tb00351.x
Christensen, B. M., Li, J., Chen, C., and Nappi, A. J. (2005). Melanization immune responses in mosquito vectors. Trends Parasitol. 21, 192–199. doi: 10.1016/j.pt.2005.02.007
Collins, S. J., and Russell, R. W. (2009). Toxicity of road salt to Nova Scotia amphibians. Environ. Pollut. 157, 320–324. doi: 10.1016/j.envpol.2008.06.032
Crumrine, P. W. (2010). Body size, temperature, and seasonal differences in size structure influence the occurrence of cannibalism in larvae of the migratory dragonfly, Anax junius. Aquat. Ecol. 44, 761–770. doi: 10.1007/s10452-010-9314-z
Dananay, K. L., Krynak, K. L., Krynak, T. J., and Benard, M. F. (2015). Legacy of road salt: apparent positive larval effects counteracted by negative postmetamorphic effects in wood frogs. Environ. Toxicol. Chem. 34, 2417–2424. doi: 10.1002/etc.3082
Dugan, H. A., Bartlett, S. L., Burke, S. M., Doubek, J. P., Krivak-Tetley, F. E., Skaff, N. K., et al. (2017). Salting our freshwater lakes. Proc. Natl. Acad. Sci. U.S.A. 114, 4453–4458. doi: 10.1073/pnas.1620211114
Duong, T., and McCauley, S. J. (2016). Predation risk increases immune response in a larval dragonfly (Leucorrhinia intacta). Ecology 97, 1605–1610. doi: 10.1890/15-1964.1
Environment Canada (2001). Canadian Environmental Protection Act, 1999; Priority Substances List Assessment Reports. Kingston upon Hull: Environment Canada.
Environment Canada (2012). Five-Year Review Of Progress: Code of Practice for the Environmental Management of Road Salts. Ottawa, ON: Environment Canada.
Folsom, T. C., and Collins, N. C. (1984) The diet foraging behavior of the larval dragonfly Anax Junius (Aeshnidae), with an assessment of the role of refuges prey activity. Oikos 42, 105–113. doi: 10.2307/3544615
Griffith, M. B. (2016). Toxicological perspective on the osmoregulation and ionoregulation physiology of major ions by freshwater animals: teleost fish, crustacean, aquatic insects and mollusca. Environ. Toxicol. Chem. 36, 576–600. doi: 10.1002/etc.3676
Hall, M. D., Vettiger, A., and Ebert, D. (2013). Interactions between environmental stressors: the influence of salinity on host-parasite interactions between Daphnia magna and Pasteuria ramosa. Oceologia 171, 189–796. doi: 10.1007/s00442-012-2452-3
Herbert, E. R., Boon, P., Burgin, A. J., Neubauer, S. C., Franklin, R. B., Ardón, M., et al. (2015). A global perspective on wetland salinization: ecological consequences of a growing threat to freshwater wetlands. Ecosphere 6, 1–43. doi: 10.1890/ES14-00534.1
Hintz, W. D., and Relyea, R. A. (2017). Impacts of road deicing salts on the early-life growth and development of a stream salmonid: salt type matters. Environ. Pollut. 223, 409–415. doi: 10.1016/j.envpol.2017.01.040
Hintz, W. D., and Relyea, R. A. (2019). A review of the species, community, and ecosystem impacts of road salt salinisation in fresh waters. Freshw. Biol. 64, 1081–1097. doi: 10.1111/fwb.13286
Hopkins, G. R., Brodie, E. D. Jr., Neuman-Lee, L. A., Mohammadi, S., Brusch, I. V. G. A., Hopkins, Z. M., et al. (2016). Physiological responses to salinity vary with proximity to the ocean in a coastal amphibian. Physiol. Biochem. Zool. 89, 322–330. doi: 10.1086/687292
Hopkins, G. R., French, S. S., and Brodie, E. D. Jr. (2013). Potential for local adaptation in response to an anthropogenic agent of selection: effects of road deicing salts on amphibian embryonic survival and development. Evol. Appl. 6, 384–392. doi: 10.1111/eva.12016
Hopkins, G. R., Gall, B. G., and Brodie, E. D. Jr. (2011). Ontogenetic shift in efficacy of antipredator mechanisms in a top aquatic predator, Anax junius (Odonata: Aeshnidae). Ethology 117, 1093–1100. doi: 10.1111/j.1439-0310.2011.01963.x
Ilvonen, J. J., and Suhonen, J. (2016). Phylogeny affects host's weight, immune response and parasitism in damselflies and dragonflies. R. Soc. Open Sci. 3:160421. doi: 10.1098/rsos.160421
Jones, D. K., Mattes, B. M., Hintz, W. D., Schuler, M. S., Stoler, A. B., Lind, L. A., et al. (2017). Investigation of road salts and biotic stressors on freshwater wetland communities. Environ. Pollut. 221, 159–167. doi: 10.1016/j.envpol.2016.11.060
Kaushal, S. S., Groffman, P. M., Likens, G. E., Belt, K. T., Stack, W. P., Kelly, V. R., et al. (2005). Increased salinization of fresh water in the northeastern United States. Proc. Natl. Acad. Sci. U.S.A. 102, 13517–13520. doi: 10.1073/pnas.0506414102
Kearney, B. D., Pell, R. J., Byrne, P. G., and Reina, R. D. (2014). Anuran larval developmental plasticity and survival in response to variable salinity of ecologically relevant timing and magnitude. J. Exp. Zool. 321A, 541–549. doi: 10.1002/jez.1887
Kefford, B. J., Papas, P. J., Metzeling, L., and Nugegoda, D. (2004). Do laboratory salinity tolerances of freshwater animals correspond with their field salinity? Environ. Pollut. 129, 355–362. doi: 10.1016/j.envpol.2003.12.005
Kelly, C. D., and Jennions, M. D. (2009). Sexually dimorphic immune response in the harem polygynous Wellington tree weta Hemideina crassidens. Physiol. Entomol. 34, 174–179. doi: 10.1111/j.1365-3032.2009.00671.x
Köning, C., and Schmid-Hempel, P. (1995). Foraging activity and immunocompetence in workers of the bumble bee, Bombus terrestris. Proc. R. Soc. Lond. B 260, 225–227. doi: 10.1098/rspb.1995.0084
Marshall, W. S., and Grosell, M. (2006). “Ion transport, osmoregulation and acid-base balance,” in The Physiology of Fishes (CRC Press), 177–230.
McAllen, R., and Taylor, A. (2001). The effect of salinity change on the oxygen consumption and swimming activity of the high-shore rockpool copepod Tigriopus brevicornis. J. Exp. Mar. Biol. Ecol. 263, 227–240. doi: 10.1016/S0022-0981(01)00308-2
McPeek, M. A. (1990). Behavioral differences between Enallagma species (Odonata) influencing differential vulnerability to predators. Ecology 5, 1714–1726. doi: 10.2307/1937580
McPeek, M. A. (1998). The consequences of changing the top predator in a food web: a comparative experimental approach. Ecol. Monogr. 68, 1–23.
Nagel, L., Mlynarek, J. J., and Forbes, M. R. (2011). Immune responses to nylon filaments in two damselfly species that differ in their resistance to ectoparasitic mites. Ecol. Entomol. 36, 736–743. doi: 10.1111/j.1365-2311.2011.01323.x
Novotny, E. V., Murphy, D., and Stefan, H. G. (2008). Increase of urban lake salinity by road deicing salt. Sci. Tot. Environ. 406, 131–144. doi: 10.1016/j.scitotenv.2008.07.037
Novotny, E. V., and Stefan, H. G. (2012). Road salt impact on lake stratification and water quality. J. Hydraulic Eng. 138, 1069–1080. doi: 10.1061/(ASCE)HY.1943-7900.0000590
Petranka, J. W., and Doyle, E. J. (2010). Effects of road salts on the composition of seasonal pond communities: can the use of road salts enhance mosquito recruitment? Aquat. Ecol. 44, 155–166. doi: 10.1007/s10452-009-9286-z
Rantala, M. J., and Roff, D. A. (2005). An analysis of trade-offs in immune function, body size and development time in the Mediterranean Field Cricket, Gryllus bimaculatus. Funct. Ecol. 19, 323–330. doi: 10.1111/j.1365-2435.2005.00979.x
Rantala, M. J., and Roff, D. A. (2007). Inbreeding and extreme outbreeding cause sex differences in immune defence and life history traits in Epirrita autumnata. Heredity 98, 329–336. doi: 10.1038/sj.hdy.6800945
Roast, S. D., Widdows, J., and Jones, M. B. (1999). Respiratory responses of the esturarine mysid Neomysis integer (Peracarida: Mysidacea) in relation to a variable environment. Mar. Biol. 133, 643–649. doi: 10.1007/s002270050504
Sala, M., Faria, M., Sarasúa, I., Barata, C., Bonada, N., Brucet, S., et al. (2016). Choride and sulphate toxicity to Hydropsyche exocellata (Trichoptera, Hydropsychidae): exploring intraspecific variation and sub-lethal endpoints. Sci. Tot. Environ. 566–567, 1032–1041. doi: 10.1016/j.scitotenv.2016.05.121
Sanzo, D., and Hecnar, S. J. (2006). Effects of road de-icing salt (NaCl) on larval wood frogs (Rana sylvatica). Environ. Pollut. 140, 247–256. doi: 10.1016/j.envpol.2005.07.013
Scheibener, S., Conley, J. M., and Buchwalter, D. (2017). Sulfate transport kinetics and toxicity are modulated by sodium in aquatic insects. Aquat. Toxicol. 190, 62–69. doi: 10.1016/j.aquatox.2017.06.027
Scheibener, S. A., Richardi, V. S., and Buchwalter, D. B. (2016). Comparative sodium transport patterns provide clues for understanding salinity and metal responses in aquatic insects. Aquat. Toxicol. 171, 20–29. doi: 10.1016/j.aquatox.2015.12.006
Schmid-Hempel, P. (2005a). Natural insect host-parasite systems show immune priming and specificity: puzzles to be solved. BioEssays 27, 1026–1034. doi: 10.1002/bies.20282
Schmid-Hempel, P. (2005b). Evolutionary ecology of insect immune defenses. Annu. Rev. Entomol. 50, 529–551. doi: 10.1146/annurev.ento.50.071803.130420
Searle, C. L., Shaw, C. L., Hunsberger, K. K., Prado, M., and Duffy, M. A. (2016). Salinization decreases population densities of the freshwater crustacean, Daphnia dentifera. Hydrobiologia 770, 165–172. doi: 10.1007/s10750-015-2579-4
Silberbush, A., Blaustein, L., and Margalith, Y. (2005). Influence of salinity concentration on aquatic insect community structure: a mesocosm experiment in the Dead Sea Basin Region. Hydrobiologia 548, 1–10. doi: 10.1007/s10750-004-8336-8
Stoler, A., Sudol, K., Mruzek, J., and Relyea, R. (2018). Interactive effects of road salt and sediment disturbance on the productivity of seven common aquatic macrophytes. Freshw. Biol. 63, 709–720. doi: 10.1111/fwb.13110
Timpano, A. J., Schoenholtz, S. H., Soucek, D. J., and Zipper, C. E. (2018). Benthic macroinvertebrate community response to salinization in headwater streams in Appalachia USA over multiple years. Ecol. Indic. 91, 645–656. doi: 10.1016/j.ecolind.2018.04.031
Tollefsen, K. E., Song, Y., Kleiven, M., Mahrosh, U., Meland, S., Rosseland, B. O., et al. (2015). Transcriptional changes in Atlantic salmon (Salmo salar) after embryonic exposure to road salt. Aquat. Toxicol. 169, 58–68. doi: 10.1016/j.aquatox.2015.10.003
Tyree, M., Clay, N., Polaskey, S., and Entrekin, S. (2016). Salt in our streams: even small sodium additions can have negative effects on detritivores. Hydrobiologia 775, 109–122. doi: 10.1007/s10750-016-2718-6
Van Meter, R. J., Swan, C. M., Leips, J., and Snodgrass, J. W. (2011). Road salt stress induces novel food web structure and interactions. Wetlands 31, 843–851. doi: 10.1007/s13157-011-0199-y
Wilder, A. E., and Welch, A. M. (2014). Effects of salinity and pesticide on sperm activity and oviposition site selection in green treefrogs, Hyla cinerea. Copeia 4, 659–667. doi: 10.1643/CE-14-053
Wood, L., and Welch, A. M. (2015). Assessment of interactive effects of elevated salinity and three pesticides on life history and behavior of southern toad (Anaxyuris terrestris) tadpoles. Environ. Toxicol. Chem. 34, 667–676. doi: 10.1002/etc.2861
Keywords: insect immunity, melanization, anthropogenic stressor, road salt, salinization, odonates, salt pollution
Citation: Mangahas RS, Murray RL and McCauley SJ (2019) Chronic Exposure to High Concentrations of Road Salt Decreases the Immune Response of Dragonfly Larvae. Front. Ecol. Evol. 7:376. doi: 10.3389/fevo.2019.00376
Received: 18 May 2019; Accepted: 19 September 2019;
Published: 11 October 2019.
Edited by:
Laurentiu Rozylowicz, University of Bucharest, RomaniaReviewed by:
Coy St. Clair, Iowa State University, United StatesDan Cogalniceanu, Ovidius University, Romania
Copyright © 2019 Mangahas, Murray and McCauley. This is an open-access article distributed under the terms of the Creative Commons Attribution License (CC BY). The use, distribution or reproduction in other forums is permitted, provided the original author(s) and the copyright owner(s) are credited and that the original publication in this journal is cited, in accordance with accepted academic practice. No use, distribution or reproduction is permitted which does not comply with these terms.
*Correspondence: Rosalind L. Murray, cm9zYWxpbmQubXVycmF5QHV0b3JvbnRvLmNh
†These authors have contributed equally to this work