- 1Université des Sciences et Techniques de Masuku, Franceville, Gabon
- 2Parasitology Laboratory, CHU Montpellier, Montpellier, France
- 3ISEM, University of Montpellier, CNRS, IRD, EPHE, Montpellier, France
Recent progress in designing non-invasive tools to monitor wildlife health offers promising perspectives in immune ecology. In this study, we investigated individual differences in fecal neopterin, a non-invasive marker closely associated with the activation of the cellular immune response, the biological and clinical relevance of which remains to be established. Specifically, we investigated the influence of gut parasites, injuries, environmental, and reproductive seasonality, and some individual traits (sex, age, social rank, reproductive state) on fecal neopterin in a natural population of mandrills (Mandrillus sphinx) in Southern Gabon. In this 46-month study, we analyzed neopterin levels from 372 fecal samples collected from 67 individuals of all sexes and ages, running separate models in three categories of individuals that may elicit different strategies of immune investment: (i) across individuals of all sexes and ages, (ii) in adult females, and (iii) in adult males. An increasing proportion of cycling females in the group, reflecting the mating season, positively influenced the concentrations of fecal neopterin across non-adult individuals. The origin of this effect remains unclear as it was unrelated to other ecological (rainfall) or social (rates of male-male aggression) seasonal variables. We did not detect any effects of injuries, parasitism or any other individual traits in these three categories of individuals. Fecal neopterin might be a useful immune marker in some clinical contexts, but our results raise questions regarding its relevance to reveal individual differences in the immune system of a healthy primate population.
Introduction
According to life history theory, maintaining a strong immune system is energetically costly (Janeway et al., 2005) and may compromise investment in other functions such as growth or reproduction (Sheldon and Verhulst, 1996). While important steps have been taken to understand the role of immune function in the evolution of life histories (Sheldon and Verhulst, 1996; Rolff, 2002; Nunn et al., 2009), major gaps remain in our knowledge. For example, we are only starting to appreciate the extent and evolutionary significance of sexual dimorphism in immune response (Sheldon and Verhulst, 1996; Rolff, 2002; Zuk and Stoehr, 2010). It is commonly hypothesized that males from polygynous species invest more in reproduction than in immunity and consequently show poor immune performances (Moore, 2002). But this paradigm has received mixed empirical support, generating results that are often contradictory depending on the immune phenotype examined and on the experimental conditions (Prall and Muehlenbein, 2014).
The adaptive significance of life history trade-offs relative to immune function can only be examined in naturally regulated populations where animals are exposed to injuries, infections, and natural stressors that reflect selective pressures encountered over the course of their evolutionary history. However, measuring variations in the immune function in the wild poses many practical challenges, as there is a general lack of non-invasive and ecologically relevant tools to monitor immune phenotypes (Adamo, 2004; Bradley and Jackson, 2008). Examining variations in the prevalence or intensity of natural infections (such as gut parasite loads) may reflect variations in individual exposure to infection rather than in immune capacity (Skorping and Jensen, 2004). Finally, the ecological relevance of standard in vivo immunoassays measuring the intensity of an adaptive response triggered by artificial antigens—such as phytohaemagglutinin—is largely debated (Siva-Jothy and Ryder, 2001; Adamo, 2004) and the same is true for in vitro stimulation of immune cells (Kidd, 2003).
The recent development of non-invasive tools to monitor wildlife health offers promising perspectives. For example, recent studies have assessed urinary (Heistermann and Higham, 2015; Behringer et al., 2017; Müller et al., 2017; Wu et al., 2018) or fecal (Campbell et al., 2004; Higham et al., 2015) levels of neopterin, a protein released by specific immune effectors (macrophages, monocytes, and dendritic cells) during particular steps of the innate response as well as during the activation of a Th1 specific immune response (Widner et al., 2000; Mosser and Edwards, 2008). After antigen recognition, the T-helper cells release interleukin 12 (IL-12), which activates the T-lymphocytes type 1 and natural killer cells to release interferon γ, which, in conjunction with other cytokines and endotoxins, stimulates the activation of monocytes, macrophages and dendritic cells (Murr et al., 2002; Mosser and Edwards, 2008). Therefore, the circulating level of neopterin is an efficient marker of the activation of the Th1 immune response, with the advantage that neopterin, in contrast to some other cytokines including interferon γ, is chemically stable. During the course of a viral infection (eliciting a Th1 response), neopterin levels initially increase and subsequently decline, when specific antibodies against the antigen become measurable, and then stay at baseline levels when the immune system has successfully defeated the infection (Murr et al., 2002). Therefore, changes in neopterin levels are closely associated with the activation of the early cellular immune response (Hamerlinck, 1999; Pingle et al., 2008). While urinary and serum levels of neopterin seem to be closely associated, less is known about fecal neopterin, which can be assayed in humans (Husain et al., 2013) and has, so far, never been used in wild animal populations. Blood and fecal levels of neopterin may reflect distinct immune processes, as a preliminary study suggests that there is no association between the two in non-human primates (Higham et al., 2015). Fecal neopterin may reflect local immune reactions affecting the gut mucosa rather than general immune processes, as suggested by some clinical studies, where levels of fecal neopterin were found to increase in patients with parasitic enteropathies and an associated inflammation of the gut mucosa (Husain et al., 2013). However, the only attempt to validate the biological relevance of fecal neopterin in non-human primates failed to find increased levels following either infection by SIV or a colonoscopy involving intestinal biopsy, a surgical procedure that may elicit mucosal inflammation (Higham et al., 2015).
Here we aim to test the relevance of fecal neopterin as a non-invasive marker of the immune function in a natural primate population. We focus on mandrills (Mandrillus sphinx) from Southern Gabon, for which detailed information on life history, behavior, physiology and health is available. Wild non-human primates represent interesting models in disease ecology studies because of their phylogenetic proximity to humans, which makes them a potential source of emerging zoonoses (Burgos-Rodriguez, 2011; Miller and Fowler, 2014; Levecke et al., 2015), but which also allows a direct transfer of methods from humans to primates. In addition, there is an over-representation of short-lived species such as rodents and passerines in the current record of eco-immunological studies (Norris and Evans, 2000; Sadd and Schmid-Hempel, 2009; Schulenburg et al., 2009; Girard et al., 2011; White and Perkins, 2012; Garnier and Graham, 2014). Yet, studying long-lived species is essential to understand how the pace of life may affect trade-offs involving the immune function. Finally, there is a wealth of detailed individually-based long-term studies on wild primates that can generate precious datasets to test predictions derived from life history theory, provided that non-invasive immune markers are available, including our field study of mandrills. Mandrills form large multimale-multifemale social groups in the wild (Brockmeyer et al., 2015) with multiple and complex socio-sexual strategies (Charpentier et al., 2005; Setchell et al., 2005). They live in polygynandrous societies where dominant males compete intensely and frequently fight over access to females during the reproductive season, which has led to the development of intense sexual signals and of extreme sexual size dimorphism (Setchell et al., 2001a). Consequently, individual investment in reproduction likely varies considerably in relation to sex, social status and season and we may expect similar variation in physiological and immune performances. In addition, this population harbors a diversity of gut parasites (Poirotte et al., 2016), which will allow us to measure immune variation in the context of natural diseases.
First, we investigate individual differences in fecal neopterin levels studying traits such as sex, age, and social rank and controlling for the season of sampling, to test whether fecal neopterin may serve as an immune phenotyping tool. This would ultimately allow to test hypotheses from life history theory. Second, we examine the influence of gut parasite richness and physical injuries on fecal neopterin levels. If fecal neopterin levels are sensitive to gut parasite or local infections, individuals carrying multiple species of amoebae (which trigger a mixed Th1 and Th2 response) and open wounds (which trigger a Th1 response), but not nematodes (which trigger a Th2 response), should exhibit higher neopterin levels.
Materials and Methods
Study Site and Subjects
This study was performed on a free-ranging population of mandrills, an Old World primate living in the Equatorial forest of Central Africa from February 2014 to November 2017. The study population ranges in a 116 km2 private park (Lékédi Park) and its vicinity, near the village of Bakoumba located in Southern Gabon (570 m above sea level; 1°49′47″ S, 13°01′12″ E). This population originates from 65 captive-born mandrills housed at CIRMF (Center International de Recherches Médicales de Franceville, Gabon) and released into the park on two occasions (36 individuals were released in 2002 and 29 in 2006; see for details: Peignot et al., 2008). In the first years post-release, mandrills were provisioned three to four times a week with bananas and monkey chow in limited quantities to improve their natural diet. Food supplementation decreased progressively over time and completely ceased at the beginning of a long-term field project (Mandrillus Project) which started in early 2012. Within the framework of this project, trained field assistants follow the group year-round, from 6:00 a.m. to 6:00 p.m., to collect day-long behavioral, demographic, and group-living data as well as fecal material from identified and habituated animals. In early 2018, the study population was composed of ca. 150 habituated individuals, including almost 90% of wild-born animals (see for details: Charpentier et al., 2018).
Fecal Collection and Storage
During the study period, we collected a total of 1,082 fresh fecal samples (entire boluses, immediately after defecation) from identified individuals. All samples were brought back to the lab and thoroughly mixed at the end of the day of collection. A portion of each sample was stored at 4°C for a few days before subsequent parasitological analyses and, for a subset of fecal samples (N = 782), another portion was stored at −20°C for further experiments, including neopterin measurements.
Fecal Neopterin Concentrations
Just before extraction, 0.1 g of fecal material was thawed and mixed with half a milliliter of 0.9% saline solution (NaCl). Mixed solutions were subsequently vortexed for 30 min and centrifuged at 3.500 rpm for 20 min. The supernatant was then recovered for analysis following previously published procedures (Campbell et al., 2004). We assessed fecal neopterin concentrations using commercial neopterin ELISA kits (#RE59321; IBL International GmbH, Germany) and following the manufacturer's protocol. We deposited, in duplicates, 20 μL of standards and controls (with known concentrations) as well as fecal supernatants into wells of the microtiter plate coated with a goat anti-rabbit antibody. We added 100 μL of enzyme conjugate and 50 μL of neopterin antiserum into each well. We covered the microtiter plate with a black adhesive foil and incubated it by gently vortexing the plate in the dark for 90 min. After incubation, we removed the adhesive foil and washed the plate four times with 300 μL of wash buffer. We then added 150 μL of substrate solution into each well and incubated the plate for 10 min. We stopped the reaction by adding 150 μL of stop solution into each well. Finally, optical densities (OD) of the assayed samples were read using a microplate photometer with a 450 nm filter (Multiskan FC). Neopterin concentrations (in nmol/L) were measured 1–5 times (per sample) on a total of 379 fecal samples (122 replicates) stored at −20°C for 1–1,328 days. Concentrations were deduced by fitting averaged OD on standard curves.
Analytical Validation
First, we calculated parallelism using three serial dilutions of five mandrill fecal samples (Table S1). The slope of the standard curve was compared to the five slopes of the antibody binding using an ANCOVA (SAS v4). Slopes were found parallel for the five samples (Table S1). Second, we measured accuracy by mixing different dilutions of different concentrations of the same five fecal samples (Table S2). When plotting the expected concentrations of mixes with the observed concentrations, we found a correlation of 83.2% and a mean accuracy of 100.01 ± 10.00. For each plate, we then verified that the two internal quality controls yielded concentrations in acceptable ranges (control 1: 3.3–7.6 nmol/L; control 2: 13.1–27.1 nmol/L). We then estimated the precision of our analyses by measuring the coefficient of variation (CV) of 27 fecal samples run on a same plate in quadruplicates. However, for two samples, we discarded one of the four available OD which was obviously different from the others—meaning that these samples were eventually run in triplicates. Averaged intra-assay CV was 3.0%.
We also calculated inter-assay CV using two different methods. First, we measured CV using the repeated concentrations of each of the two internal quality controls run in each assay. CV were 12.1 and 21.3%, respectively. Second, 63 fecal samples were each run in 2–4 different assays (difference in storage time at −20°C ranged from 2 to 845 days across identical samples). We calculated the inter-assay CV using neopterin concentrations obtained from these repeated measurements. Averaged CV was found elevated (45.1%) and preliminary examinations of the data revealed combined, non-linear effects of both the storage time of the fecal samples at −20°C and the number of freeze-thaw cycles (mean ± SD: 1.3 ± 0.6, range: 1–4 across repeated measures of a single sample; Figure S1). When restricting inter-assay CV calculations to those samples that were frozen for comparable durations (2–8 days apart) and that underwent only 1–2 freeze-thaw cycles (N = 9 duplicates), inter-assay CV dropped to 22.8%. While this CV remained high, neopterin concentrations between these duplicates were, however, highly correlated (Spearman's correlation test: r = 0.92, p < 10−3). Consequently, we restricted our analyses to those samples that underwent only one freeze-thaw cycle (N = 406 measurements from 379 fecal samples). We further considered the time of storage as a fixed effect in all statistical models and included a random effect of the assay identity (“plate identity”; see below). In addition, all duplicated samples that showed an intra-assay CV higher than 10% were discarded from subsequent analyses (N = 9 measurements from 6 fecal samples). One sample was further removed because of labeling doubts. After applying these restrictions, we obtained neopterin concentrations for 373 fecal samples collected from 34 males and 33 females (aged 1.0–22.4 years), with a mean ± SD of 5.55 ± 5.89 samples per individual (median: 4, range: 1–31).
Parasitological Analyses
Using sedimentation techniques, we performed parasitological analyses from 1 to 3 g of stool material placed in a mixing chamber containing 6 ml of Bailenger solution (15 g sodium acetate, 3.60 mL acetic acid, and 1,000 mL distilled water). The solution was thoroughly vortexed for 15 s. A filter and a sedimentation chamber were attached to the mixing chamber and the solution was centrifuged at 1,500 rpm for 3 min. The supernatant was then removed and, after homogenization of the sediment, we examined a drop of the suspension using a microscope at 100x, followed by 400x, and 1,000x for parasite identification (see for details: Poirotte et al., 2016).
We identified nematode eggs and protozoan trophozoites and cystic stages by taxon according to morphological characteristics based on the shape, content, and size of the eggs and cystic stages (Deluol et al., 1998, 1999; and for the criteria used to identify protozoa, see Table 1 in Poirotte et al., 2016). In the following analyses, we considered protozoan richness as the total number of different protozoan taxa, and nematode richness as the total number of different nematode taxa retrieved from each sample (as per: Poirotte et al., 2016). Coprologies were performed 0–10 days following the day of collection, allowing accurate parasite identification (see for a rationale: Poirotte et al., 2016).
Ecological and Individual Traits
Environmental Seasonality
In Gabon, there are four climatic seasons characterized by a marked long rainy season (Feb-May) and a long dry season (Jun-Sept), in addition to a short rainy season (Oct-Nov), and a short dry season (Dec-Jan). The ecological seasonality was described by the cumulated rainfall (mm) within 30 days preceding sample collection. Daily rainfall records were downloaded from the NASA website (https://disc.gsfc.nasa.gov/) for the geographic region of interest (GPS coordinates: 1°45′27″S 12°57′22″E for the top left corner, and 1°50′35″S 13°01′19″E for the bottom right corner) during the study period.
Breeding Seasonality
In the studied mandrills, most females are sexually receptive from April to September, corresponding to an overall increase in male-male aggression (Figure 1). The monthly proportion of cycling females in the group was used as a continuous proxy of the reproductive seasonality, whereas monthly rates of male-male aggression were used as a proxy of the associated social instability due to an influx of competing immigrant males in the group (Abernethy et al., 2002; Brockmeyer et al., 2015; Hongo et al., 2016). Monthly proportions of cycling females were calculated by dividing the total number of days mature females were seen cycling by the total number of days mature females were observed in any given month, whatever their reproductive status. Monthly rates of male-male aggression were obtained from focal sampling by dividing all occurrences of male-male aggression recorded a given month by the total time of focal sampling of these males this month (in hours). Agonistic interactions included e.g., chases, bites, head-bobs, ground slaps. Both male-male aggression and the monthly proportion of cycling females were significantly, though moderately correlated (Pearson's test, N = 40 months, r = 0.43, t = 2.98, p = 0.005).
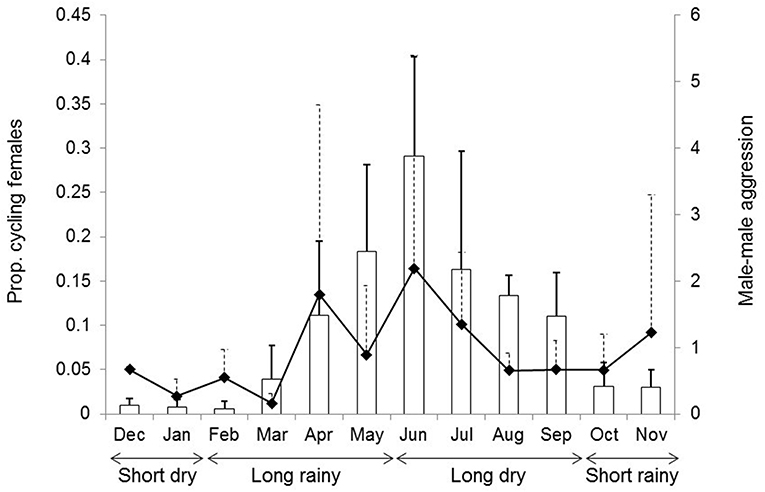
Figure 1. Temporal variation in the proportion of cycling females in the study group (bar plots) and in the rate of male-male aggression during the annual cycle (filled diamonds). In this figure, the monthly proportions of cycling females and the monthly rates of male-male aggression were averaged across the years (mean ± SD are shown).
Age and Reproductive Status
Individual birth dates were exactly known for 18 animals or approximated with a possible error estimated to be inferior to a few days/weeks for 9 mandrills. For all other studied individuals (N = 40), dates of birth were estimated based on body condition and patterns of tooth eruption and wear (Galbany et al., 2014). For these later mandrills, the error was estimated to be less than a year for 22 individuals; and <5 years for 18 mandrills that we saw for the first time as adults. Females were considered adult after 4 years of age, when most females reach sexual maturity, while males were considered adult after 9 years of age, when they have reached adult size and their secondary sexual characters are progressively developing (Setchell et al., 2001b).
Female reproductive status was monitored daily. Females were considered cycling if they showed a visible perineal swelling of any size (Dixson, 1983). Pregnancies were deduced from a combination of clues including the presence of a small pink swelling visible in the two last months of pregnancy (MJEC, pers. obs.) as well as patterns of sexual cycles and births. Females with an infant younger than 6 months were considered lactating, except when they resumed cycling before their infant reached that age (two cases). Non-cycling females were not pregnant, not lactating and did not show any visible perineal swelling. In subsequent analyses, female reproductive status was therefore described as a class variable with four modalities: cycling, non-cycling, pregnant, and lactating.
Dominance Rank
In adult males and females, hierarchical ranks were deduced from patterns of approach-avoidance interactions. We used the corrected David's score to rank individuals (David, 1987; as per: Charpentier et al., 2018). Dominance rank is mostly linear for both males and females but cannot be compared between sexes. In females, rank is stable and maternally inherited, with daughters acquiring a rank just below their mothers (Setchell et al., 2001b). We classified females in three categories of rank with a balanced sample size across categories: high-ranking, medium-ranking, low-ranking females. In males, rank regularly changes over time. We therefore established a monthly dominance rank per male and classified males into two categories: the alpha male vs. all subordinate males. Indeed, the alpha male displays clear behavioral and phenotypic traits that are distinct from other males. In particular, the alpha male exhibits bright facial colors (Setchell and Dixson, 2001) and faces frequent behavioral challenges from subordinates (MJEC, pers. obs.).
Injuries
We recorded the occurrence, type of wounds (open cuts, punctures of the skin, abnormal skin swellings, limbs), freshness (presence of fresh/dry blood) and body location of any injury on a daily basis. During the study period, we recorded a total of 25 injuries for which feces were collected within the 3 weeks following injury. Superficial scratches, limbs and lameness (14 injuries) were discarded from the analysis, as these injuries may not be associated with any detectable Th1-immune reaction. The effect of injuries on levels of fecal neopterin was only investigated in adult males (N = 11 open injuries from 7 males, with a mean ± SD of 12.45 ± 6.77 days between the first record of injury and sample collection) because females or juveniles did not show any open injuries during the study period.
Statistical Analyses
We performed three sets of Linear Mixed Models (LMM) to study the relationships between fecal neopterin concentration (response variable) and different predictors in three groups of individuals who may elicit different strategies of immune investment: (1) individuals of all sexes and ages, (2) sexually mature females and (3) adult males. In all models, fecal neopterin concentrations were log-transformed in order to obtain residuals that were normally distributed. All three sets of LMMs had a same structure of random effects, including individual and plate (i.e., assay) identity. Fixed effects fitted in all models included cumulated rainfall in the 30 days preceding sample collection (in mm), proportion of cycling females in the group as well as rates of male-male aggression (number of events per hour) in the month of data collection, age (in years), as well as nematode richness and protozoa richness. All quantitative fixed effects were standardized (transformed to obtain a mean of 0 and a variance of 1) to facilitate model convergence. Variance inflation factor was below 2 across all models suggesting that model stability was not compromised by multicolinearity among co-variables. Model fit was evaluated by checking the Gaussian distribution of the residuals, and plotting them against the predicted values to verify their homogeneity across the range of these predicted values.
In a first model, we considered all samples excluding one extreme value (N = 372 samples collected from 67 individuals of all sexes and ages; mean number of samples per individual ± SD: 5.6 ± 5.9; range: 1–31), in order to compare neopterin concentrations between sexes and across different age classes. In addition to the above-mentioned fixed effects, we included individual sex, a quadratic term for age to account for the possibility of non-linear age effects, as well as storage time (i.e., time elapsed between collection and extraction of the fecal samples, in days) with a quadratic term to control for potentially confounding effects of storage time on neopterin levels. Here and below, we did not fit any interactions to avoid over-parameterization and facilitate model convergence.
We restricted the second model to adult females only (>4 years, N = 149 samples collected from 32 females; mean number of samples per female ± SD: 4.7 ± 4.5; range: 1–23) to test whether neopterin levels vary with female social rank and reproductive state. In addition to the fixed effects included in all models, we included as extra fixed effects: female dominance rank (class variable with three modalities: high, middle or low rank), and reproductive status (class variable with four modalities: pregnant, lactating, cycling and non-cycling). For this model as well as for adult males, we omitted simple and quadratic effects of storage time as well as the quadratic effect of age, which were all found non-significant on the extended sample using the first model to limit risks of over-parameterization.
In a third model, we restricted our sample to adult males only (>9 years old, N = 113 samples collected from 13 males) to test whether neopterin levels vary with the presence of injuries. In addition to the fixed effects included in all models, we included as extra fixed effects: male dominance rank (alpha male vs. other males), and the presence of a visible open wound at the time of sample collection (class variable with two modalities: no wound vs. open wound).
All LMMs were run using the lmer function of the lme4 package (Bates et al., 2014) in R version 3.5.2 (R Core Team, 2017). The significance of the fixed factors was tested by computing their 95% bootstrap confidence intervals (using confint.merMod) and checking that they did not cross zero. Their p-values were calculated by using the PBmodcomp function from the pbkrtest package (Halekoh and Højsgaard, 2014), which compares a model with and without the variable using a parametric bootstrap method.
Results
Variation of Fecal Neopterin Concentrations in All Individuals
Fecal neopterin concentrations varied between 1.0 and 11.1 nmol/L (mean ± SD: 3.4 ± 1.7) but individual identity did not explain any significant part of such variation (comparison of a model with and without this random effect: = 1.45, p = 0.23). There was, therefore, no consistency across repeated measures of a same individual. In contrast, samples measured in a same assay were more homogeneous than samples measured in different assays (comparison of a model with and without the random effect “plate identity”: = 120.85, p < 10−4). Fecal neopterin concentrations were significantly influenced by the temporal variation in the proportion of cycling females in the group (Table 1; Figure 2). In contrast, none of the other fixed effects, including sex (Figure S2) and variation in parasite richness (Figure 3), explained any variation in fecal neopterin levels. An additional LMM was run on non-adults only (females aged <4 years old and males aged <9 years old) to investigate whether the proportion of cycling females was driven by the consideration of adult females. This effect remained significant in non-adults, suggesting that this seasonal effect did affect juveniles (Table S3).
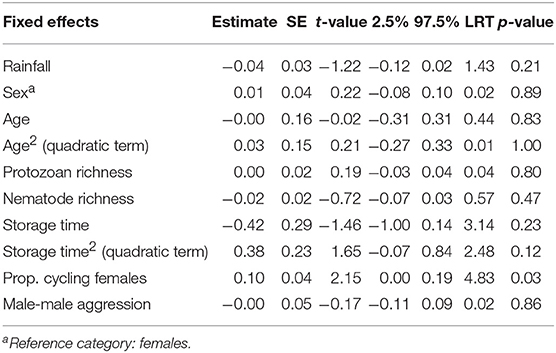
Table 1. Estimates, standard errors (SE), and confidence intervals of fixed effects predicting variations in fecal neopterin concentrations across males and females of all ages (N = 67 individuals and 372 samples; mean number of samples per individual ± SD: 5.6 ± 5.9; range: 1–31).
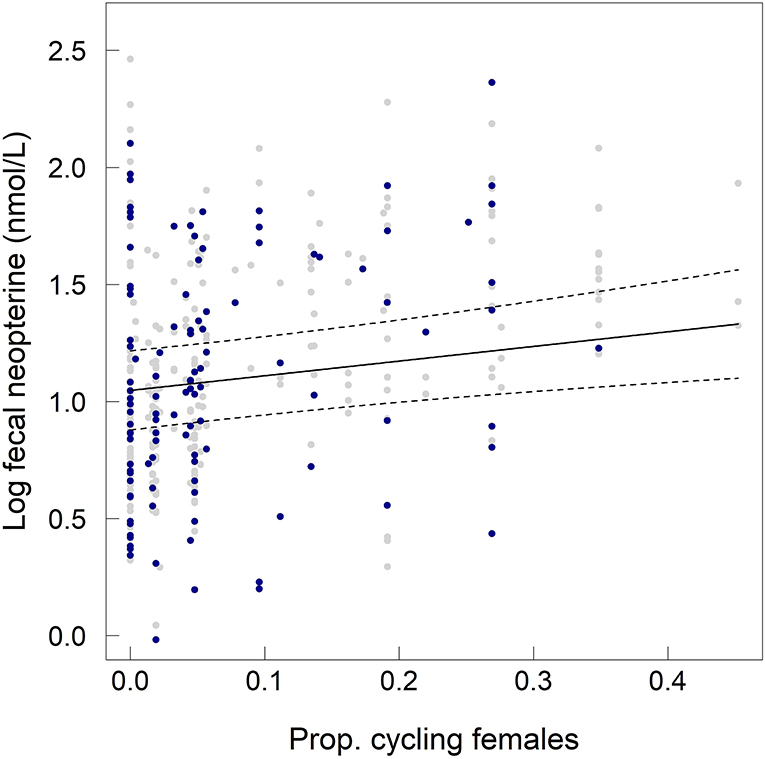
Figure 2. Influence of the proportion of cycling females in the group on fecal neopterin concentrations (log-transformed) across individuals of both sexes and all ages. Gray dots represent the raw values for adult individuals, blue dots represent the raw values for non-adult individuals and the solid line represents the predicted values of the model including all individuals. The dashed lines represent the 95% confidence intervals of the predicted values.
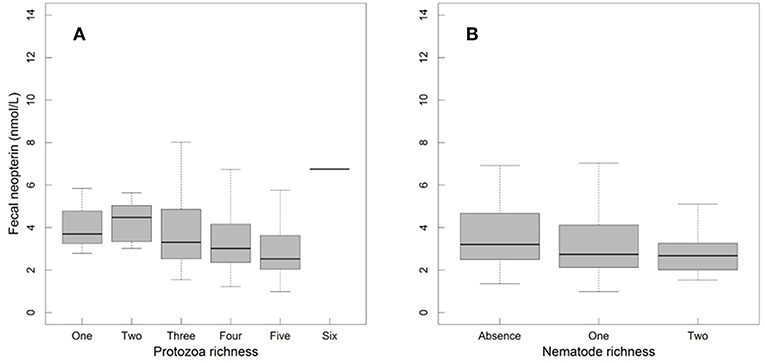
Figure 3. Fecal neopterin concentrations (raw data) across male and female mandrills of all ages in relation to protozoa richness (A) and nematode richness (B). Sample sizes for panel A included 67 individuals and 372 samples broken as follows: 3 samples for the category of 1 parasite, 12 for two, 44 for three, 123 for four, 189 for five and 1 for six. Sample sizes for panel B included 67 individuals and 372 samples broken as follows: 111 samples for the category of zero parasite, 213 for one and 30 for two. For both panels, the bottom and top of the box, respectively, represent the 25th and 75th quartiles, and the bold horizontal line represents the median.
Variation of Fecal Neopterin Concentrations in Females
In sexually mature females, fecal neopterin concentrations varied between 1.3 and 8.7 nmol/L (mean ± SD: 3.4 ± 1.5). As for the model above, female identity did not significantly explain this variation ( = 0.41, p = 0.52), in contrast to plate identity ( = 50.52, p < 10−4). There was no significant influence of the fixed effects investigated (Table 2). Although the variable “reproductive state” was globally non-significant, the pairwise comparison between two categories of this factor revealed that lactating females had lower neopterin levels than pregnant females (the confidence intervals did not include 0: Table 2; Figure 4).
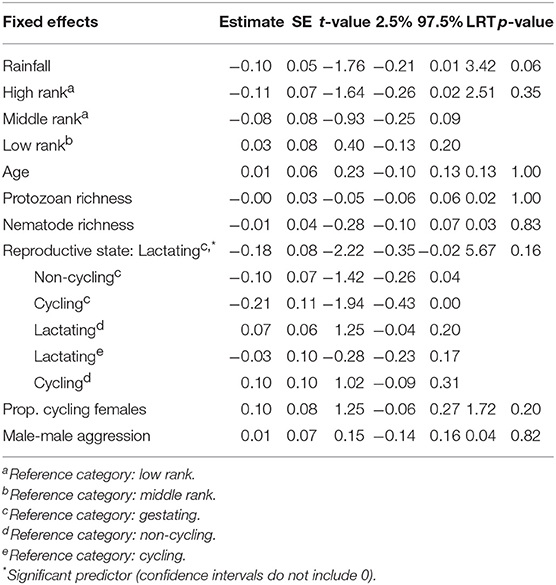
Table 2. Estimates, standard errors (SE) and confidence intervals of fixed effects predicting variations in fecal neopterin concentrations in sexually mature females (N = 32 individuals and 149 samples; mean number of samples per female ± SD: 4.7 ± 4.5; range: 1–23).
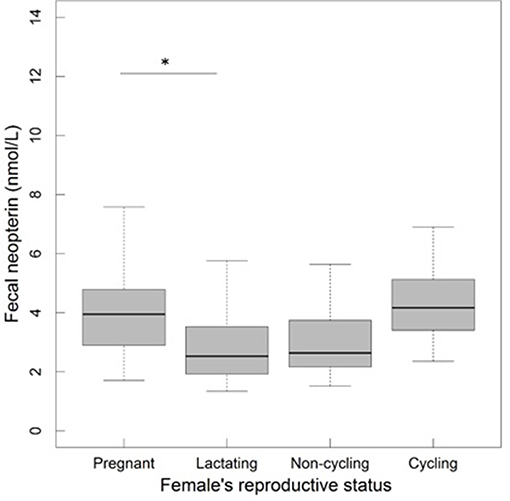
Figure 4. Fecal neopterin concentrations (raw data) in relation to female reproductive state (N = 32 individuals and 149 samples including 25 from pregnant females, 48 from lactating females, 62 from non-cycling females and 14 from cycling females; mean number of samples per female ± SD: 4.7 ± 4.5; range: 1–23). The bottom and top of the box, respectively, represent the 25th and 75th quartiles, and the bold horizontal line represents the median. The significance of the comparisons was evaluated by changing contrasts in each LMM. Comparisons are denoted by “*” if significant (p < 0.05).
Variation of Fecal Neopterin Concentrations in Males
In adult males, fecal neopterin concentrations varied between 1.4 and 11.1 nmol/L (mean ± SD = 3.4 ± 1.9). Male identity significantly explained some of this variation ( = 5.66, p = 0.02), in addition to plate identity ( = 36.68, p < 10−4). However, none of the tested fixed effects were significant, including social rank (Figure S3) and injuries—despite a graphical trend suggesting higher fecal neopterin levels in injured individuals (Table 3; Figure 5).
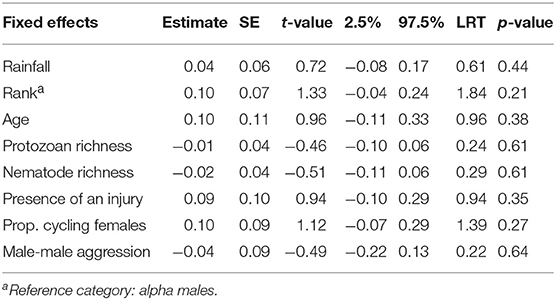
Table 3. Estimates, standard errors (SE) and confidence intervals of fixed effects predicting variations in fecal neopterin concentrations in adult males (N = 13 individuals and 113 samples; mean number of samples per male ± SD: 8.7 ± 10.1; range: 1–31).
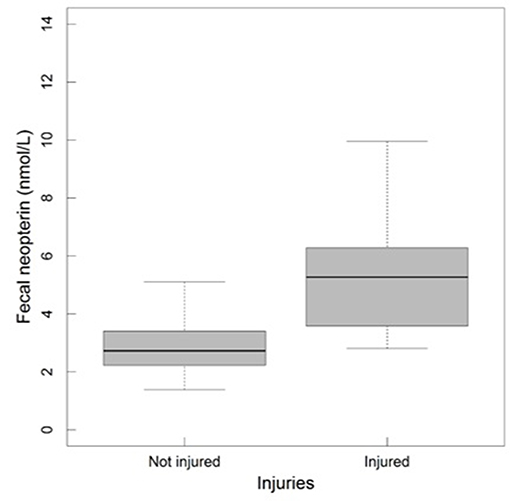
Figure 5. Fecal neopterin concentrations (raw data) in relation to injuries in adult males (N = 13 individuals and 113 samples including 102 samples from the category not injured and 11 from the category injured; mean number of samples per male ± SD: 8.7 ± 10.1; range: 1–31). The bottom and top of the box, respectively, represent the 25th and 75th quartiles, and the bold horizontal line represents the median.
Discussion
In this 46-month study, we investigated longitudinal intra- and inter-individual variation of fecal neopterin concentrations, a marker closely associated with the activation of the cellular immune response, across 67 male and female free-ranging mandrills of all ages. In particular, we were interested to know whether neopterin varied with individual traits, as well as if this marker was sensitive to gut parasitism or local infections. However, individual traits did not explain a significant amount of variation in fecal neopterin levels, and our predictions regarding either gut parasitism or injuries were not supported.
The lack of social and individual effects on fecal neopterin concentrations may be related to methodological limitations in our analyses. Indeed, we observed elevated coefficients of variation (CVs) across identical samples assayed on different plates, partly due to the sample storage time (time spent in the freezer) and freeze-thaw cycles. Controlling for these effects lowered the inter-assay CV, which nevertheless remained relatively high. Although we thoroughly mixed all samples before any extraction, a possible explanation is that neopterin was not homogeneously distributed within fecal samples, resulting in high CVs across plates. In addition, measurements were obtained from wet fecal material and were not corrected by the dry weight of the fecal pellet. If water is not homogeneously distributed in a fecal sample, this may also contribute to these elevated CVs. We considered the identity of the plate as a random factor in our analyses in order to control for unexplained variation across plates that may be related to our methodological approaches. Applying such a conservative statistical method nevertheless presents a drawback. Indeed, because of field constraints related to data collection, we performed our analyses in batches. For example, we tended to assay samples collected during a same season on a same plate to avoid long storage times. Considering a random effect of the plate could thus result in an under-estimation of seasonal effects—even though samples from two or more seasons still commonly co-occur on a same plate. However, this scenario does not explain the absence of individual effects, as males and females were randomly distributed across plates, as were individual age, rank, or parasite status.
We further failed to detect effects of open injuries or parasite loads on fecal neopterin levels, possibly due to the limitations highlighted above. In addition, all fecal samples collected within 3 weeks post-injury were included in the “injured” category, at a time where potential increases in neopterin levels following the fresh injury might already be on their way down to baseline levels. In addition, fecal neopterin is probably predominantly a signal of gut infection and inflammation, and might not reflect any other local or general inflammatory process. Yet, we did not detect an effect of gut parasite richness on fecal neopterin levels either. This lack of parasite effect on fecal neopterin might be due to immune tolerance, where most gut parasites would fail to elicit a strong inflammatory response. However, the studied mandrills are infected by parasites that are known to be pathogenic (e.g., Entamoeba histolytica) and that affect their behavior (Poirotte et al., 2017a,b), which might not favor the immune tolerance hypothesis. It is also possible that immune response to such parasites activate alternative immune pathways that do not involve neopterin, such as a typical Th2 response. Finally, our estimates of parasite infestation, which simply counts the number of parasite species present while omitting the relative and absolute abundance of each parasite species, may not adequately reflect the complexity of pathogenic pressures, and of immune responses elicited in response to these pressures.
Despite these limitations, we found that fecal neopterin concentrations were significantly influenced by the proportion of cycling females in the group. This effect was observed across all animals, but neither in adult females nor in adult males. In these females, some variation linked to the proportion of cycling females may be absorbed by the reproductive state variable, as lactating females had lower neopterin levels than pregnant females, and a substantial proportion of females are in early pregnancy during the mating season. Nevertheless, none of these two fixed effects (reproductive state and the monthly proportion of cycling females) were significant in the female model, suggesting that such an explanation is not sufficient. In addition, the proportion of cycling females was also found significant in a model containing only non-adults, pointing to an environmental or social determinant that varies seasonally and affects juveniles as well as, or preferentially than adults. The potential nature of this effect is hard to uncover as two other seasonal covariables, namely cumulated rainfall in the 30 days preceding data collection and rates of male-male aggression, were non-significant either. We would expect most environmental seasonality to correlate with seasonal variations in rainfall in this equatorial region, where other aspects of environmental seasonality such as variations in day length or in temperature are tenuous. In addition, any social effect broadly linked with mandrill breeding seasonality, such as the influx of immigrant males during the mating season that induces a strong instability in the male hierarchy and may cause associated stress to other group members (Charpentier et al., 2018), is expected to co-vary with our male-male aggression variable. It remains possible that a physiological phenomenon affecting juveniles and restricted to the long dry season is responsible for this effect, and better captured by the proportion of cycling females than by rainfall patterns, which shows a bimodal distribution with two rainfall peaks a year.
Knowledge on immune and disease ecology from previous studies in this population is however not sufficient to shed light on the nature of such a phenomenon, though data available reveal that the mating season appears physiologically stressful for the mandrills. First, as the driest season of the year, it is likely that food availability is lower than in other seasons, though such an association is hard to test in a generalist forest species that regularly consumes over 150 plant species (Nsi Akoue et al., 2017). Second, individuals exhibited higher nematode richness during the long dry season, especially the juveniles (Poirotte et al., 2016)—although our analyses show that gut parasites do not explain fecal neopterin levels. Third, the oxidative damages of females, including juvenile females, were found to be higher during the long dry season (Beaulieu et al., 2014), whereas their leucocyte profiles also varied seasonally, with lower lymphocyte proportion being observed during the long dry season, presumably because of the stress at that time of the year (Beaulieu et al., 2017) which is also characterized by increases in cortisol production compared to the long rainy season—although the highest cortisol concentrations are in fact observed during the short dry season (Charpentier et al., 2018). It is therefore likely that the long dry season poses a particular challenge on the immune system of juvenile mandrills, due to combined effects of nutritional stress and higher disease risk, which is reflected by the effect of the mating season (or proportion of cycling females) on neopterin levels.
Overall, fecal neopterin did not meet our expectations while it may have been a promising non-invasive tool relevant for eco-epidemiological studies. This may be due to the methodological limitations of our study, and further research based on additional data may accordingly reach different conclusions. Some prior studies interested in the clinical relevance of fecal neopterin found substantial changes in neopterin levels in relation to the inflammatory status of the gut mucosa. For example, in humans, one study showed that elevated neopterin concentrations were associated with acute gastritis (Ledjeff et al., 2001). Other examples include a field study who detected increases in fecal neopterin in children infested with Giardia cysts compared to healthy children (Campbell et al., 2004) as well as the usefulness of fecal neopterin levels to monitor disease progression in patients suffering from Crohn's disease or from active ulcerative colitis (Husain et al., 2013). In such cases, the extent of increases in neopterin levels was 10–1,000-fold higher than in our own study. In non-human primates, the only published study investigating variations in fecal neopterin levels was conducted in captive macaques and failed to detect consistent effects of the retroviral status of individuals, as well as a correlation with serum or urinary neopterin levels—though this was not unexpected given that fecal neopterin only appears to increase in response to gut inflammatory processes in humans, rather than to general inflammatory states (Higham et al., 2015). In addition, fecal neopterin did not increase after a colonoscopy involving a colon biopsy, which was more surprising, raising further doubts on the biological relevance of such marker to assess an inflammation of the gut mucosa. Overall, more remains to be done to validate the biological and clinical relevance of fecal neopterin in humans and non-human primates.
In mandrills, the small range of fecal neopterin concentrations (1.0–11.1 nmol/L) that we found may only reflect the baseline levels of a healthy population, where inter-individual variation is essentially undetectable and does not have any functional significance. It is therefore possible that fecal neopterin is of limited interest to test predictions derived from life history theory by investigating inter- or intra-individual variations in strategies of immune investment. Serum and urinary levels of neopterin may be more promising for eco-immunology studies (Behringer et al., 2017; Müller et al., 2017), as previous studies reported between-individual variations related to individual traits including age (Heistermann and Higham, 2015; Behringer and Deschner, 2017) and health, such as the retroviral status of individuals (Souquière et al., 2014; Higham et al., 2015) and respiratory infection (Behringer et al., 2017; Wu et al., 2018), autoimmune process (Balázs et al., 2012), viral gastroenteritis (Plata-Nazar et al., 2010) or for pathology of depression (Tang et al., 2016). A rigorous biological validation of the clinical relevance of fecal neopterin now appears suitable before any further study in immune ecology.
Data Availability Statement
The datasets generated during the current study are available from the corresponding author on reasonable request.
Ethics Statement
All applicable international, national, and/or institutional guidelines for the care and use of animals were followed. This study further complied with ethical protocols approved by the CENAREST institute (authorization number: AR0042/17/MESRS/CENAREST/CG/CST/CSAR) and adhered to the legal requirements of Gabon for the ethical treatment of non-human primates.
Author Contributions
EH and MC conceived the ideas and designed methodology. EH, MC, and SD analyzed the data and led the writing of the manuscript. AS read the final manuscript. DB conceived parasitological methods. All authors contributed critically to the drafts and gave final approval for publication.
Conflict of Interest
The authors declare that the research was conducted in the absence of any commercial or financial relationships that could be construed as a potential conflict of interest.
Acknowledgments
We thank past and present field assistants of the Mandrillus Project for their help in data collection. We are also grateful to the SODEPAL-COMILOG society (ERAMET group) for their logistical contribution. This study was funded by a Station d'Etudes en Ecologie Globale (INEE-CNRS) and a Laboratoire International Associé (CIRMF and INEE-CNRS) to MC; a PEPS program to EH (MI-CNRS); a Conservation Action Research Network—Congo Basin Grant Program and Bourses SUD (ISEM) to SD, EH, and MC. Data used in this manuscript are part of long-term data collected within the framework of the Mandrillus Project, funded by a grant of the Deutsche Forschungsge meinschaft (DFG, KA 1082-20-1; 2012-2015) and two grants from the Agence Nationale de la Recherche (ANR SLEEP 17-CE02-0002, 2017-2020 to MC; ANR ERS-17-CE02-0008, 2018-2021 to EH). This study was approved by an authorization from the CENAREST institute (permit number: AR0042/17/MESRS/CENAREST/CG/CST/CSAR). This is a Mandrillus Project publication number 18 and # ISEM 2019-188-SUD.
Supplementary Material
The Supplementary Material for this article can be found online at: https://www.frontiersin.org/articles/10.3389/fevo.2019.00368/full#supplementary-material
References
Abernethy, K. A., White, L. J. T., and Wickings, E. J. (2002). Hordes of mandrills (Mandrillus sphinx): extreme group size and seasonal male presence. J. Zool. 258, 131–137. doi: 10.1017/S0952836902001267
Adamo, S. (2004). How should behavioural ecologists interpret measurements of immunity? Anim. Behav. 68, 1443–1449. doi: 10.1016/j.anbehav.2004.05.005
Balázs, C., Türke, B., and Vámos, A. (2012). Determination of serum neopterin levels in patients with autoimmune thyroid diseases. Orvosi Hetilap. 153, 1127–1131. doi: 10.1556/OH.2012.29405
Bates, D., Maechler, M., Bolker, B., and Walker, S. (2014). Lme4: Linear Mixed-Effects Models Using Eigen and S4. R Package Version 1.1-7. R Package Version 1.1-7.
Beaulieu, M., Benoit, L., Abaga, S., Kappeler, P. M., and Charpentier, M. J. E. (2017). Mind the cell: seasonal variation in telomere length mirrors changes in leucocyte profile. Mol. Ecol. 26, 5603–5613. doi: 10.1111/mec.14329
Beaulieu, M., Mboumba, S., Willaume, E., Kappeler, P. M., and Charpentier, M. J. E. (2014). The oxidative cost of unstable social dominance. J. Exp. Biol. 217, 2629–2632. doi: 10.1242/jeb.104851
Behringer, V., and Deschner, T. (2017). Non-invasive monitoring of physiological markers in primates. Horm. Behav. 91, 3–18. doi: 10.1016/j.yhbeh.2017.02.001
Behringer, V., Stevens, J. M. G., Leendertz, F. H., Hohmann, G., and Deschner, T. (2017). Validation of a method for the assessment of urinary neopterin levels to monitor health status in non-human-primate species. Front. Physiol. 8:41. doi: 10.3389/fphys.2017.00051
Bradley, J. E., and Jackson, J. A. (2008). Measuring immune system variation to help understand host-pathogen community dynamics. Parasitology 135, 807–823. doi: 10.1017/S0031182008000322
Brockmeyer, T., Kappeler, P. M., Willaume, E., Benoit, L., Mboumba, S., and Charpentier, M. J. E. (2015). Social organization and space use of a wild mandrill (Mandrillus sphinx) group: Mandrill Social Organization and Space Use. Am. J. Primatol. 77, 1036–1048. doi: 10.1002/ajp.22439
Burgos-Rodriguez, A. G. (2011). Zoonotic diseases of primates. Vet. Clin. North Am. Exot. Anim. Pract. 14, 557–575. doi: 10.1016/j.cvex.2011.05.006
Campbell, D. I., McPhail, G., Lunn, P. G., Elia, M., and Jeffries, D. J. (2004). Intestinal inflammation measured by fecal neopterin in Gambian children with enteropathy: association with growth failure, Giardia lamblia, and intestinal permeability: J. Pediatri. Gastroenterol. Nutr. 39, 153–157. doi: 10.1097/00005176-200408000-00005
Charpentier, M., Peignot, P., Hossaert-McKey, M., Gimenez, O., Setchell, J. M., and Wickings, E. J. (2005). Constraints on control: factors influencing reproductive success in male mandrills (Mandrillus sphinx). Behav. Ecol. 16, 614–623. doi: 10.1093/beheco/ari034
Charpentier, M. J. E., Givalois, L., Faurie, C., Soghessa, O., Simon, F., and Kappeler, P. M. (2018). Seasonal glucocorticoid production correlates with a suite of small-magnitude environmental, demographic, and physiological effects in mandrills. Am. J. Phys. Anthropol. 165, 20–33. doi: 10.1002/ajpa.23329
David, H. A. (1987). Ranking from unbalanced paired-comparison data. Biometrika 74, 432–436. doi: 10.1093/biomet/74.2.432
Deluol, A., Hellegouarc'h, A., Lebras, P., and Ricq, G. (1998). Colour Atlas of Parasitology, Vol. 1. Amoebae. St. Maur: Varia.
Deluol, A., Lebras, P., and Ricq, G. (1999). Colour atlas of parasitology, Vol. 2, Flagellates - Ciliates - Coccidia - Microsporidia - Blastocystis hominis - Trichomonas vaginalis. St. Maur: Varia.
Dixson, A. F. (1983). Observations on the evolution and behavioral significance of “Sexual Skin” in female primates. Adv. Study Behav. 13, 63–106. doi: 10.1016/S0065-3454(08)60286-7
Galbany, J., Romero, A., Mayo-Alesón, M., Itsoma, F., Gamarra, B., Pérez-Pérez, A., et al. (2014). Age-related tooth wear differs between forest and savanna primates. PLoS ONE 9:e94938. doi: 10.1371/journal.pone.0094938
Garnier, R., and Graham, A. L. (2014). Insights from parasite-specific serological tools in eco-immunology. Integ. Comp. Biol. 54, 363–376. doi: 10.1093/icb/icu022
Girard, J., Goldberg, T. L., and Hamer, G. L. (2011). Field investigation of innate immunity in passerine birds in suburban Chicago, Illinois, USA. J. Wildl. Dis. 47, 603–611. doi: 10.7589/0090-3558-47.3.603
Halekoh, U., and Højsgaard, S. (2014). A Kenward-Roger approximation and parametric bootstrap methods for tests in linear mixed models—The R package pbkrtest. J. Stat. Softw. 59, 1–30. doi: 10.18637/jss.v059.i09
Hamerlinck, F. F. V. (1999). Neopterin: a review. Exp. Dermatol. 8, 167–176. doi: 10.1111/j.1600-0625.1999.tb00367.x
Heistermann, M., and Higham, J. P. (2015). Urinary neopterin, a non-invasive marker of mammalian cellular immune activation, is highly stable under field conditions. Sci. Rep. 5:16308. doi: 10.1038/srep16308
Higham, J. P., Kraus, C., Stahl-Hennig, C., Engelhardt, A., Fuchs, D., and Heistermann, M. (2015). Evaluating noninvasive markers of nonhuman primate immune activation and inflammation: evaluating noninvasive markers of nonhuman primate immune activation and inflammation: noninvasive markers of immune activation. Am. J. Phys. Anthropol. 158, 673–684. doi: 10.1002/ajpa.22821
Hongo, S., Nakashima, Y., Akomo-Okoue, E. F., and Mindonga-Nguelet, F. L. (2016). Female reproductive seasonality and male influxes in wild mandrills (Mandrillus sphinx). Intern. J. Primatol. 37, 416–437. doi: 10.1007/s10764-016-9909-x
Husain, N., Tokoro, K., Popov, J. M., Naides, S. J., Kwasny, M. J., and Buchman, A. L. (2013). Neopterin concentration as an index of disease activity in Crohn's Disease and Ulcerative Colitis. J. Clin. Gastroenterol. 47, 246–251. doi: 10.1097/MCG.0b013e3182582cdb
Janeway, C. A. Jr., and Travers, P. (eds.). (2005). Immunobiology: The Immune System in Health and Disease. London: Current Biology, Ltd.; New York, NY: Garland Publishing Inc., 622.
Kidd, P. (2003). Th1/Th2 balance: the hypothesis, its limitations, and implications for health and disease. Altern. Med. Rev. 8, 223–246.
Ledjeff, E., Artner-Dworzak, E., Witasek, A., Fuchs, D., and Hausen, A. (2001). Neopterin concentrations in colon dialysate. Pteridines 12, 155–160. doi: 10.1515/pteridines.2001.12.4.155
Levecke, B., Dorny, P., Vercammen, F., Visser, L. G., Van Esbroeck, M., Vercruysse, J., et al. (2015). Transmission of Entamoeba nuttalli and Trichuris trichiura from nonhuman primates to humans. Emerg. Infects. Dis. 21, 1871–1872. doi: 10.3201/eid2110.141456
Miller, R. E., and Fowler, M. E. (2014). Fowler's Zoo and Wild Animal Medicine, Volume 8—E-Book. Elsevier Health Sciences.
Moore, S. L. (2002). Parasites as a viability cost of sexual selection in natural populations of mammals. Science 297, 2015–2018. doi: 10.1126/science.1074196
Mosser, D. M., and Edwards, J. P. (2008). Exploring the full spectrum of macrophage activation. Nat. Rev. Immunol. 8, 958–969. doi: 10.1038/nri2448
Müller, N., Heistermann, M., Strube, C., Schülke, O., and Ostner, J. (2017). Age, but not anthelmintic treatment, is associated with urinary neopterin levels in semi-free ranging Barbary macaques. Sci. Rep. 7:41973. doi: 10.1038/srep41973
Murr, C., Widner, B., Wirleitner, B., and Fuchs, D. (2002). Neopterin as a marker for immune system activation. Cur. Drug Metab. 3, 175–187. doi: 10.2174/1389200024605082
Norris, K., and Evans, M. R. (2000). Ecological immunology: life history trade-offs and immune defense in birds. Behav. Ecol. 11, 19–26. doi: 10.1093/beheco/11.1.19
Nsi Akoue, G., Mbading-Mbading, W., Willaume, E., Souza, A., Mbatchi, B., and Charpentier, M. J. E. (2017). Seasonal and individual predictors of diet in a free-ranging population of mandrills. Ethology 123, 600–613. doi: 10.1111/eth.12633
Nunn, C. L., Lindenfors, P., Pursall, E. R., and Rolff, J. (2009). On sexual dimorphism in immune function. Philos. Trans. R. Soc. B 364, 61–69. doi: 10.1098/rstb.2008.0148
Peignot, P., Charpentier, M. J. E., Bout, N., Bourry, O., Massima, U., Dosimont, O., et al. (2008). Learning from the first release project of captive-bred mandrills (Mandrillus sphinx) in Gabon. Oryx 42, 122–131. doi: 10.1017/S0030605308000136
Pingle, S., Tumane, R., and Jawade, A. (2008). Neopterin: biomarker of cell-mediated immunity and potent usage as biomarker in silicosis and other occupational diseases. Indian J. Occup. Environ. Med. 12, 107–111. doi: 10.4103/0019-5278.44690
Plata-Nazar, K., Luczak, G., Gora-Gebka, M., Liberek, A., and Kaminska, B. (2010). Serum neopterin concentration in children with viral gastroenteritis. Pteridines 21, 11–16. doi: 10.1515/pteridines.2010.21.1.11
Poirotte, C., Basset, D., Willaume, E., Makaba, F., Kappeler, P. M., and Charpentier, M. J. E. (2016). Environmental and individual determinants of parasite richness across seasons in a free-ranging population of Mandrills (Mandrillus sphinx). Am. J. Phys. Anthropol. 159, 442–456. doi: 10.1002/ajpa.22888
Poirotte, C., Benhamou, S., Mandjembe, A., Willaume, E., Kappeler, P. M., and Charpentier, M. J. E. (2017a). Gastro-intestinal parasitism and recursive movements in free-ranging mandrills. Anim. Behav. 134, 87–98. doi: 10.1016/j.anbehav.2017.10.013
Poirotte, C., Massol, F., Herbert, A., Willaume, E., Bomo, P. M., Kappeler, P. M., et al. (2017b). Mandrills use olfaction to socially avoid parasitized conspecifics. Sci. Adv. 3:e1601721. doi: 10.1126/sciadv.1601721
Prall, S. P., and Muehlenbein, M. P. (2014). Testosterone and immune function in primates: a brief summary with methodological considerations. Intern. J. Primatol. 35, 805–824. doi: 10.1007/s10764-014-9752-x
R Core Team (2017). R: A Language and Environment for Statistical Computing. Vienna: R Foundation for Statistical Computing.
Rolff, J. (2002). Bateman's principle and immunity. Proc. R. Soc. B 269, 867–872. doi: 10.1098/rspb.2002.1959
Sadd, B. M., and Schmid-Hempel, P. (2009). Principles of ecological immunology. Evol. Applic. 2, 113–121. doi: 10.1111/j.1752-4571.2008.00057.x
Schulenburg, H., Kurtz, J., Moret, Y., and Siva-Jothy, M. T. (2009). Introduction. Ecological immunology. Philos. Trans. R. Soc.Lond. B Biol. Sci. 364, 3–14. doi: 10.1098/rstb.2008.0249
Setchell, J. M., Charpentier, M., and Wickings, E. J. (2005). Sexual selection and reproductive careers in mandrills (Mandrillus sphinx). Behav. Ecol. Sociobiol. 58, 474–485. doi: 10.1007/s00265-005-0946-2
Setchell, J. M., and Dixson, A. F. (2001). Changes in the secondary sexual adornments of male mandrills (Mandrillus sphinx) are associated with gain and loss of alpha status. Horm. Behav. 39, 177–184. doi: 10.1006/hbeh.2000.1628
Setchell, J. M., Lee, P. C., Wickings, E. J., and Dixson, A. F. (2001a). Growth and ontogeny of sexual size dimorphism in the mandrill (Mandrillus sphinx). Am. J. Phys. Anthropol. 115, 349–360. doi: 10.1002/ajpa.1091
Setchell, J. M., Lee, P. C., Wickings, E. J., and Dixson, A. F. (2001b). Reproductive parameters and maternal investment in Mandrills (Mandrillus sphinx). Int. J. Primatol. 23, 51–68. doi: 10.1023/A:1013245707228
Sheldon, B. C., and Verhulst, S. (1996). Ecological immunology: costly parasite defences and trade-offs in evolutionary ecology. Trends Ecol. Evol. 11, 317–321. doi: 10.1016/0169-5347(96)10039-2
Siva-Jothy, M. T., and Ryder, J. J. (2001). Assaying PHA-induced mitosis: out of control? Funct. Ecol. 15, 813–814. doi: 10.1046/j.0269-8463.2001.00573.x
Skorping, A., and Jensen, K. H. (2004). Disease dynamics: all caused by males? Trends Ecol. Evol. 19, 219–220. doi: 10.1016/j.tree.2004.02.006
Souquière, S., Makuwa, M., Sallé, B., Lepelletier, Y., Mortreux, F., Hermine, O., et al. (2014). Immunological alterations and associated diseases in mandrills (Mandrillus sphinx) naturally co-infected with SIV and STLV. Virology 454, 184–196. doi: 10.1016/j.virol.2014.02.019
Tang, C.-Z., Zhang, Y.-L., Wang, W.-S., Li, W.-G., and Shi, J.-P. (2016). Elevated serum levels of neopterin at admission predicts depression after acute ischemic stroke: a 6-Month follow-up study. Mol. Neurobiol. 53, 3194–3204. doi: 10.1007/s12035-015-9220-4
White, T. A., and Perkins, S. E. (2012). The ecoimmunology of invasive species. Funct. Ecol. 26, 1313–1323. doi: 10.1111/1365-2435.12012
Widner, B., Wirleitner, B., Baier-Bitterlich, G., Weiss, G., and Fuchs, D. (2000). Cellular immune activation, neopterin production, tryptophan degradation and the development of immunodeficiency. Arch. Immunol. Ther. Exp. 48, 251–258.
Wu, D. F., Behringer, V., Wittig, R. M., Leendertz, F. H., and Deschner, T. (2018). Urinary neopterin levels increase and predict survival during a respiratory outbreak in wild chimpanzees (Taï National Park, Côte d'Ivoire). Sc. Rep. 8:13346. doi: 10.1038/s41598-018-31563-7
Keywords: immunity, fecal neopterin, non-invasive sampling, primates, Mandrillus sphinx
Citation: Dibakou SE, Basset D, Souza A, Charpentier M and Huchard E (2019) Determinants of Variations in Fecal Neopterin in Free-Ranging Mandrills. Front. Ecol. Evol. 7:368. doi: 10.3389/fevo.2019.00368
Received: 29 March 2019; Accepted: 17 September 2019;
Published: 04 October 2019.
Edited by:
Romain Garnier, Georgetown University, United StatesReviewed by:
Laurence R. Gesquiere, Duke University, United StatesNicole A. Thompson, University of New Mexico, United States
Copyright © 2019 Dibakou, Basset, Souza, Charpentier and Huchard. This is an open-access article distributed under the terms of the Creative Commons Attribution License (CC BY). The use, distribution or reproduction in other forums is permitted, provided the original author(s) and the copyright owner(s) are credited and that the original publication in this journal is cited, in accordance with accepted academic practice. No use, distribution or reproduction is permitted which does not comply with these terms.
*Correspondence: Elise Huchard, elise.huchard@umontpellier.fr
†These authors have contributed equally to this work