- Department of Biology, Saint Louis University, St. Louis, MO, United States
Predicting how insects will react to future thermal conditions requires understanding how temperature currently affects insect behavior, from performance traits to those involved in mating and reproduction. Many reproductive behaviors are thermally-sensitive, but little is known how temperature affects the behaviors used to find mates and coordinate mating. Here, we investigate how temperature influences courtship activity in two sympatric species of Enchenopa treehoppers (Hemiptera: Membracidae). Enchenopa use substrate-borne vibrational signals exchanged in male-female duets to facilitate pair formation prior to mating. In a controlled laboratory setting, we assessed the likelihood of males and females to produce courtship signals across a range of ecologically relevant temperatures. We found that changes in courtship activity across temperatures differed between the two species. We also found sex differences within species: in one species males were more likely to signal at higher temperatures, while in the other species females were more likely to signal at higher temperatures. Our results suggest that sex-specific responses to temperature may constrain mating to narrower ranges of temperatures. Furthermore, sympatric species may respond differently to changes in thermal variation despite sharing similar climactic history.
Introduction
Insect biomass is rapidly declining worldwide in the wake of global warming (Hallmann et al., 2017). Due to the crucial role that insects play in nearly every ecological setting, these declines could have far-reaching implications for ecosystem functioning (Losey and Vaughan, 2006; Gallai et al., 2009; Ollerton et al., 2011). Rising temperatures pose a threat to insects because of the thermal sensitivity of a wide range of physiological, morphological, and behavioral traits (Kingsolver and Huey, 2008; Gibert et al., 2016; Abram et al., 2017). Many studies have focused on the thermal sensitivity of traits that are related to organismal performance, such as growth rates, heat shock, or flight performance (Deutsch et al., 2008; Frazier et al., 2008; King and MacRae, 2015). Additionally, some studies have investigated the effects of temperature on various aspects of reproduction, with a focus on life history traits like the timing and length of mating seasons and average clutch sizes, or the location of breeding sites (Fielding et al., 1999; García-Barros, 2000; Guarneri et al., 2003; Braschler and Hill, 2007; Katsuki and Miyatake, 2009). However, temperature variation can also affect activity levels related to courtship and the coordination of mating. Because courtship activity precedes all other aspects of reproduction (Eberhard, 1994; Ejima and Griffith, 2007), thermal constraints on these behaviors may profoundly impact overall patterns of reproduction in insect populations. Furthermore, given that temperature-related breakdowns in mating may quickly lead to insect population declines (Høye et al., 2013; Chinellato et al., 2014), the thermal sensitivity of behaviors related to the coordination of mating should be examined in the context of global warming.
Increased temperatures can generate breakdowns in the coordination of mating if daily temperatures shift such that the thermal window of mating for an insect is no longer common in the environment. Mating activity is often highest at intermediate temperatures, but physiological constraints limit activity as temperatures deviate from this optimal range (Huey and Stevenson, 1979; Deutsch et al., 2008; Kingsolver and Huey, 2008; Angilletta, 2009). Shifts in temperature due to global warming could limit the availability of temperatures within the range of high reproductive activity and lead to reduced opportunities for mating. Additionally, sex-specific thermal effects may also generate breakdowns in sexual communication in the wake of global warming. While males and females within a species are often assumed to react similarly to environmental stressors like temperature (Shreve et al., 2004), sex-specific thermal responses in courtship activity could arise through sex differences in body size and thermoregulatory behavior during reproduction (Brown and Weatherhead, 2000; Matzkin et al., 2009; Darnell et al., 2013; Baudier et al., 2015; Foley et al., 2019). If reproductive activity levels across temperatures are sex-specific, shifts in temperatures could accentuate mismatches in male and female activity levels and reduce overlap when both sexes are actively seeking mates.
In this study, we examine how temperature affects courtship activity in light of the challenges that global warming poses to the coordination of mating. We examined patterns of courtship activity of males and females in two sympatric species of Enchenopa treehoppers (Hemiptera: Membracidae) across a range of temperatures. We then placed these activity patterns within the context of contemporary warming to assess the potential for temperature-related breakdowns in the coordination of mating. Enchenopa treehoppers are ~1/2 cm sap-feeding insects that are highly host-plant-specific (Wood and Guttman, 1983; Wood, 1993; Rodríguez et al., 2004; Cocroft et al., 2008, 2010). They spend their entire lives, including coordinating mating, on their species-specific host plants. The complex is hypothesized to have diversified through shifts in host plant usage (Lin and Wood, 2002) and concurrent changes in sexual selection via female preferences on male signal traits (Rodríguez et al., 2004; Rodríguez and Cocroft, 2006). Multiple species can be found living in the same area (Wood, 1980), but are readily distinguished based on their use of host plant and courtship signal characteristics (Rodríguez and Cocroft, 2006; Cocroft et al., 2008). To find mates, sexually mature males will fly from stem to stem on their host plant and produce courtship advertisement signals in the form of plant-borne vibrations when they land (Hunt, 1994; Cocroft et al., 2008). If a female is receptive and finds a male signal attractive, she will respond with her own sex-specific vibrational courtship signals, which initiates an alternating signaling duet; this duet aids in mate localization and the initiation of pair formation prior to mating (Rodríguez et al., 2004; Rodríguez and Cocroft, 2006).
Enchenopa treehoppers are well-suited for studies of the effects of thermal variation on reproductive-related behaviors. The nature of the thermal environment for one of the species in the current study has been previously characterized (Jocson et al., 2019), and we know that temperature affects the expression of male advertisement signals and female mate preferences (Jocson et al., 2019). However, the likelihood of engaging in reproductive-related behaviors across temperatures is currently unknown. Aside from live male-female interactions, we are not aware of any studies that tested both males and females to determine how temperature affected each sex. The Enchenopa duetting system provides a mechanism with which to do so using vibrational playbacks to assay courtship activity without the other sex needing to be present (Rodríguez et al., 2004, 2012; Rodríguez and Cocroft, 2006).
Here, we focused on two sympatric species of Enchenopa treehopper (Hemiptera: Membracidae). One species lives on the host plant Ptelea trifoliata (Rutaceae) and the other on the host plant Viburnum prunifolium (Caprifoliaceae). Both host plants occur sympatrically at our study site. We expect the temperature where activity levels are highest and the range corresponding to high behavioral activity to reflect the thermal variation experienced by an organism in its historical habitat (Angilletta et al., 2002; Clusella-Trullas et al., 2011). While the biogeographic history of the two species under study is not known, they have overlapping distributions throughout their range (Wood, 1980), suggesting a somewhat shared thermal regime. Thus, we expect some similarity in behavioral responses to temperature variation. However, the scale of the thermal environment is important to consider (Logan et al., 2013) and thermal microclimates can vary between plant hosts (Suggitt et al., 2011; Pincebourde and Woods, 2012). Divergent selection from microclimates could result in different thermal optima and tolerances (Kellermann et al., 2012). Specifically for the two host plant species in our study, P. trifoliata is found primarily in semi-shaded areas and V. prunifolium tolerates full and partial sun. Thus, while we expected some similarity in activity patterns across temperatures due to the sympatry and close phylogenetic relatedness of the two Enchenopa species (Lin and Wood, 2002), it is not unreasonable to expect that the species on V. prunifolium may be more active at hotter temperatures as compared to the species on P. trifoliata.
In addition to species-specific responses to temperature, we might predict that males and females respond differently to temperature due to underlying sex-specific physiology (Brown and Weatherhead, 2000; Matzkin et al., 2009; Darnell et al., 2013; Baudier et al., 2015; Foley et al., 2019). However, courtship activity results from a complex interaction between physiology and behavioral decisions, and we currently lack a complete framework for making an informed prediction about sex-specific responses to temperature. The larger sex of a species—females in the case of Enchenopa (Hamilton and Cocroft, 2009)—is likely to have a higher thermal tolerance (Baudier et al., 2015; Foley et al., 2019). However, relative size does not always predict thermal preference. For example, males of some Drosophila species prefer hotter temperatures than females despite being smaller, but this is not always the case (Dillon et al., 2009). Furthermore, the costs of courtship can be higher at hotter temperatures (Teal, 1959), and these costs may be borne more by the typically more actively-courting males. Finally, even when thermal tolerance, thermal preference, and the costs of behaving at thermal extremes is known, these factors may not generate a straightforward prediction about when courtship occurs. For example, male jumping spiders are most active at low temperatures in the field while mating rates are greatest at high temperatures in a laboratory setting (Brandt et al., 2018) and male fiddler crabs court at the edge of their thermal limits (Allen and Levinton, 2014).
Here, we used vibrational playbacks to test the likelihood of male and female Enchenopa to produce courtship signals across a range of temperatures (18–36°C). This range reflects common temperatures experienced by Enchenopa treehoppers during the mating season for the species living on P. trifoliata (Jocson et al., 2019). Using a function-valued approach—treating the entire curve as the trait of interest (Meyer and Kirkpatrick, 2005; Kingsolver and Huey, 2008; Stinchcombe and Kirkpatrick, 2012; Hadjipantelis et al., 2013)—we compared the male versus female thermal courtship activity curves within species, and within-sex courtship activity curves across species. We found sex-specific responses to temperature, suggesting that changes in thermal regime could accentuate mismatches between males and females in optimal temperature for actively seeking mates. We also found species-specific responses to temperature, suggesting that warming could differentially affect the breakdown of mate coordination in two species with a shared historical temperature regime.
Materials and Methods
Study Organisms
We specifically studied the two species of treehoppers in the Enchenopa binotata species complex that live on P. trifoliata and V. prunifolium. Many species in the E. binotata species complex have yet to be formally described and share a common genus and species name (Hamilton and Cocroft, 2009). Thus, we refer to our study species using the name of their host plants: E. binotata “Ptelea” and E. binotata “V. prunifolium.” The two species are morphologically similar but easily distinguished by the mean signal frequency of the male advertisement signal (Wood and Guttman, 1983; Lin and Wood, 2002; Rodríguez et al., 2004; Cocroft et al., 2008, 2010). Enchenopa binotata “Ptelea” has a mean signal frequency of 350 Hz and E. binotata “V. prunifolium” has a mean signal frequency of 285 (Rodríguez and Cocroft, 2006) at a standardized 25°C. The data we present here includes an entirely new data set for E. binotata “V. prunifolium,” and data set from E. binotata “Ptelea” collected for a separate study conducted in the same year (Leith et al., in review) but significantly expanded for our current study.
In May 2018, we collected E. binotata nymphs living on V. prunifolium and P. trifoliata host plants from Stephens Lake Park (38.927133,−92.320419) in Columbia, Missouri as 2–3rd instars. We brought the nymphs back to a greenhouse on the campus of Saint Louis University in St. Louis, MO where we reared them on potted and netted host plant exemplars in groups of ~20. Upon the last molt to adulthood, males and females were separated to control for sexual/signaling experience (Fowler-Finn and Rodríguez, 2012). When the insects reached sexual maturity (about 2 weeks after the molt to adulthood for males and about 6 weeks after the molt to adulthood for females), we started our experimental trials testing the temperatures at which individuals were reproductively active. We conducted the experimental trials between June and July 2018.
General Approach
To determine the level of courtship activity of males and females across temperatures, we took advantage of the duetting system of E. binotata treehoppers: males signal to advertise to females and females respond (Rodríguez et al., 2004, 2012; Rodríguez and Cocroft, 2006). Reproductively receptive males readily signal when placed on a host plant or in response to a recording of a live duetting pair; similarly, females that are reproductively receptive will readily respond to recordings of live males (Rodríguez et al., 2004, 2012; Rodríguez and Cocroft, 2006). Thus, we used vibrational playbacks to test male and female receptivity. By repeating these vibrational playbacks across a range of temperatures in a controlled laboratory setting, we quantified variation in courtship activity across a range of temperatures. See below for details.
Vibrational Playback and Recording Set Up
We used the programs Audacity (v. 2.1.1; http://audacity.sourforge.net/) and MatLab (v.8.3 2014) to play vibrational primers from WAV files that were recorded from a live male-female duet at 25°C (for male primers) and a live male signaling at each specified temperature (for female primers, see below) from the populations tested. The recordings were selected based on representing an average signal type at the recording temperature. The WAV files were passed first through a Roland Duo-Capture USB interface (Model No. UA-11-MK2) and then transmitted to the plant through linear resonant actuators (LRA coin type Z-axis Model G0832012) affixed to the stem with beeswax, and played back at ~0.2 mm/s. We monitored and recorded vibrational signals produced by the insects using accelerometers (Vibra Metrics Model No. 9002A with signal conditioner and power supply Model P5000) connected to Roland Duo-Capture Ex USB interfaces (Model No. UA-22) and PreSonus Audiobox USB interfaces.
To isolate the testing set up from background vibrations, the testing incubator rested on either 1-inch steel plates or 2-inch concrete slabs that were floated on a heavy table with rubber casters using partially-inflated bicycle inner tubes. The experimental host plant was further isolated from the testing incubator with sorbothane pads.
Experimental Trials
We measured the likelihood of males and females to engage in courtship activity across seven target temperatures (18, 21, 24, 27, 30, 33, 36°C) using the above vibrational playback protocol. The selected temperatures span the range at which E. binotata has been found active during the mating season in the field (Jocson et al., 2019) with the upper limit corresponding to the point past which mortality starts occurring in the laboratory. We randomly assigned each individual to one of the seven testing temperatures and acclimated them in an incubator set to their assigned testing temperatures for at least 20 min prior to testing their courtship activity (following: Greenfield and Medlock, 2007). We then transferred the individual to the testing plant in the testing incubator set to their assigned temperature and allowed them to adjust to the plant for 2 min before playing back species- and sex-specific vibrational primers to the individual. Our methods follow well-established protocol for determining if male and female E. binotata are receptive (Rodríguez et al., 2004, 2012; Rodríguez and Cocroft, 2006; Cocroft et al., 2008).
For males, we played a species-specific male-female duet primer (a male advertisement signal followed by a female response signal) twice in quick succession, every 2 min during a 10 min trial. If the male responded to the primer at any point during the trial, he was marked as reproductively active. For females, we played three species-specific male signal bouts, with each bout consisting of three (E. binotata “V. prunifolium”) or six (E. binotata “Ptelea”) signals to match the mean signals per bout for the species (Hunt, 1994; Rodríguez et al., 2004; Rodríguez and Cocroft, 2006). Each signal bout was separated from the next by 15 s of silence. To reduce the likelihood of confounding variation among females in preference for male signals either due to temperature (Jocson et al., 2019) or individual variation (Fowler-Finn et al., 2017) with whether or a not a female was receptive at a given temperature, we varied the fundamental frequency of the three signal bouts. The first signal bout consisted of a recorded male signaling at the mean frequency of the species range (recorded at 25°C: 285 Hz for E. binotata “V. prunifolium,” 350 Hz for E. binotata “Ptelea”; Rodríguez and Cocroft, 2006). The second primer we played to a female was a male signaling at the specific testing temperature (with the exception that signals recorded at 21°C were used for tests at 18°C in both species because we were unable to record males signaling at 18°C prior to the start of the experiment; and a signal recorded at 33°C was used for tests at 36°C in E. binotata “Ptelea” because we were unable to record males signaling at 36°C; see results). The third primer consisted of a male signal recorded at 21°C for females tested below 27°C, or recorded at 36°C (33°C for E. binotata “Ptelea”) for females tested at or above 27°C. After all three signal bouts were played in a random order, we waited 2 min and repeated the process of playing all three primers in a newly randomized order. If a female produced a vibrational response to any of the primers during the trial, we considered her reproductively receptive. Our design ensured that females not only heard the mean preferred signal frequency, but they also heard a range of other signal frequencies to help account for any variation in female preference across individuals. This aspect was important because females tend to be less active than males and also more discriminating (Rodríguez et al., 2012). Thus, our design minimized the likelihood of falsely classifying a female as unreceptive.
We tested individuals one to three times, never testing them at the same temperature more than once. At the conclusion of the experiment, we had 113 trials for E. binotata “V. prunifolium: (males N = 44, females N = 69) and 153 trials for E. binotata “Ptelea” (males N = 69, females N = 84).
We used the function-valued approach to compare courtship activity patterns across a range of temperatures between the two species, and between the sexes within each species. Function-valued traits use mathematical functions to describe responses to continuous environmental variation (Meyer and Kirkpatrick, 2005; Kingsolver and Huey, 2008; Stinchcombe and Kirkpatrick, 2012; Hadjipantelis et al., 2013). Here, the response is the likelihood of producing a courtship signal/response and the environmental gradient is temperature. The curves we derive from the raw data illustrate the likelihood of exhibiting courtship activity across temperature—herein “thermal courtship activity curve.”
Thermal Variation in Courtship Behavior Within and Across Species—Statistical Analyses
First, we tested for the effects of temperature on male and female courtship activity for each species independently by running nominal logistic regressions for each group (male and female of E. binotata “V. prunifolium” and male and female E. binotata “Ptelea”) separately. The dependent variable was whether an individual produced a vibrational signal in response to the vibrational playbacks. The independent variables were temperature and a temperature × temperature interaction term. The temperature term tests for a linear response of courtship activity to temperature (e.g., increased activity as temperature increases). The temperature × temperature interaction specifically tests the quadratic response of courtship activity to temperature because many thermally-sensitive traits have quadratic shapes (i.e., they peak at intermediate temperatures).
Next we compared variation in courtship activity across temperature for the following pairings: (i) male vs. female E. binotata “V. prunifolium,” (ii) male vs. female E. binotata “Ptelea,” (iii) male E. binotata “V. prunifolium,” vs. male E. binotata “Ptelea,” and (iv) female E. binotata “V. prunifolium,” vs. female E. binotata “Ptelea.” We used nominal logistic regressions with the dependent variable in each model being whether an individual produced a signal in response to the vibrational playback (as above). To compare courtship activity curves across sexes within species the independent variables were: temperature, sex, a temperature × temperature interaction, a temperature × sex interaction, and a temperature × temperature × sex interaction term. The temperature × temperature × sex interaction term was the key variable that tested whether the shape of the function varied between the sexes, which would indicate that courtship activity of the two sexes was affected by temperature in different ways. To compare courtship activity curves within sex across species, we used the same independent variables, substituting species for sex. We ran all statistical analyses in JMP Pro (14.1.0).
Describing Thermal Variation in Courtship Behavior—Qualitative Analyses
We next qualitatively compared the curves to each other using the following methodology. To visualize thermal courtship activity curves, we generated cubic splines in R (with “mgcv” package) using the open-source program PFunc (Kilmer et al., 2017; see Box 1). Cubic spline regressions are non-parametric curves which illustrate changes in the expression of a trait without making assumptions of the shape of the curve (Kilmer et al., 2017). We generated thermal courtship activity curves independently for male and female E. binotata “V. prunifolium,” and compared these to each other, as well as to thermal courtship activity curves for male and female E. binotata “Ptelea.” From each thermal courtship activity curve, we extracted the thermal activity peak, thermal activity breadth, and thermal activity window using the PFunc program (Box 1). Moreover, we qualitatively assessed variation among groups in these three parameters of the thermal courtship activity curves using the values extracted by the PFunc program.
Box 1. Key definitions of terminology used to describe courtship activity curves and illustration of how these activity curves are derived from raw data and are measured.
The following definitions can be applied to any curve describing how activity levels of any behavior vary across any environmental variable. Here, we refer to courtship activity across temperature.
Thermal Activity Peak (TAPk): The temperature at which activity is highest.
Thermal Activity Breadth (TABr): The temperature range at which the likelihood of activity is > 90% of the maximum activity level.
Thermal Activity Window (TAWn): The temperature range at which the likelihood of activity is 10%.
Individual data points represents the result of each test of a single individual and whether they produced a courtship signal or not at the testing temperature. The curve represents the likelihood of courtship activity occurring across environmental temperatures and is derived using a cubic spline regression. Cubic spline regressions make no assumptions about the shape of a curve other than that it is smooth (Schluter, 1988). To generate the curve, raw data (court yes or no) are input into the Pfunc module executed with a Python GUI (Kilmer et al., 2017). PFunc describes and analyzes functions by executing an R script that fits data using the gam function in the mgcv package (Wood, 2006). The script outputs measurements to users, which are then visualized through the interfacing Python GUI. The most recent version of the PFunc program can be downloaded from https://github.com/joccalor/pfunc/releases/latest).
Historical and Contemporary Weather Data
Typical approaches for predicting responses to global warming involve assessing current trait expression and projecting forward given estimated thermal changes (Hoffmann and Sgrò, 2011). We use a variant of this approach by mapping thermal courtship activity curves onto ranges of variation in historical and contemporary temperature data. The immense amount of weather data available at the study site we use for the two species allows us to visualize how temperature has changed over recent time at this locale and enables us to make predictions regarding how these species may cope with rising temperatures in the future. We obtained historical (1975–1978) and contemporary (2015–2018) daily low and daily high temperatures from June 1 through July 31—the primary mating season for E. binotata—recorded from Columbia Regional Airport weather station in Columbia, MO (38.8169°, −92.2183°), accessible via the National Oceanic and Atmospheric Administration online database (www.ncdc.noaa.gov).
Results
Thermal Effects on Courtship Activity
Courtship activity for males and females of both species showed both linear and quadratic responses to temperature (showing a peak at an intermediate temperature), except for female E. binotata “Ptelea” females, which showed only a linear response to temperature and thus were most active at the hottest testing temperatures (Table 1, Figure 1).
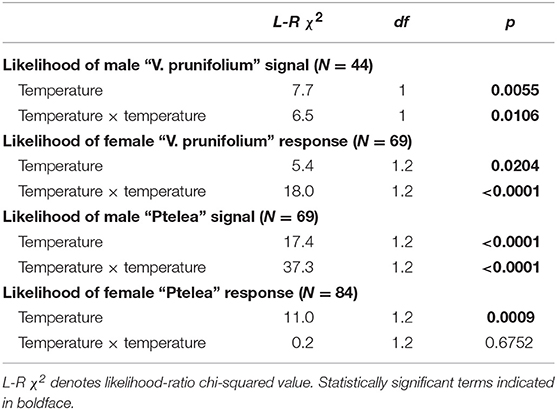
Table 1. The effects of temperature (linear and quadratic) on the likelihood of male signal production and female responses to male signals in E. binotata “Ptelea” and “V. prunifolium.”
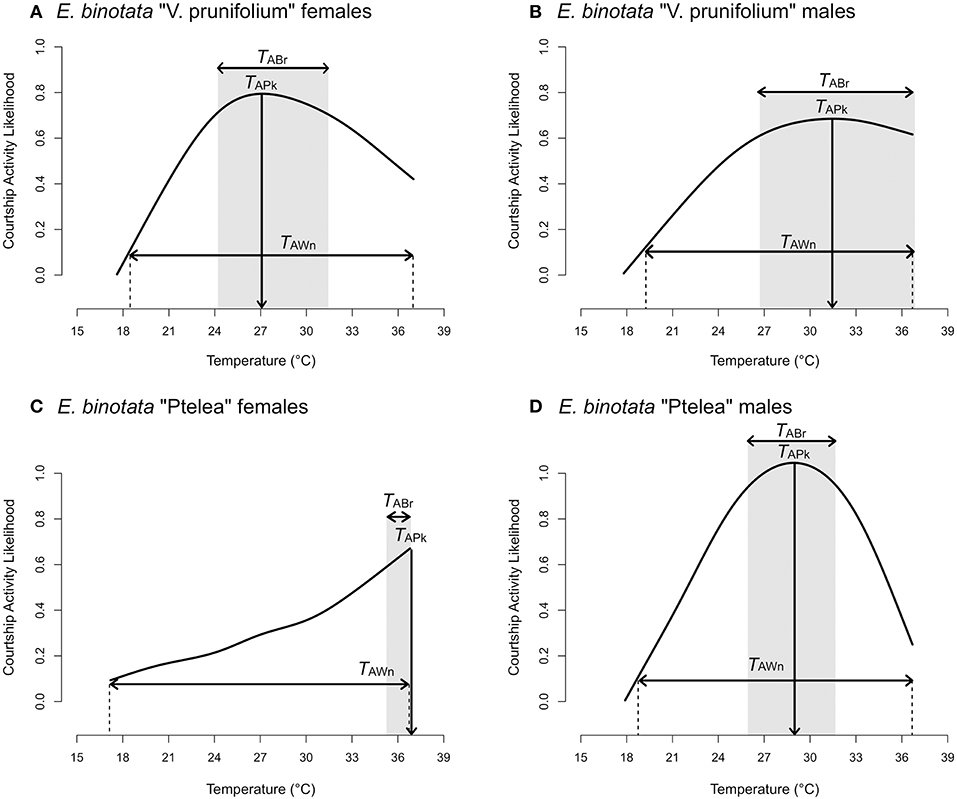
Figure 1. Courtship activity curves of (A) E. binotata “V. prunifolium” females, (B) E. binotata “V. prunifolium” males, (C) E. binotata “Ptelea” females, and (D) E. binotata “Ptelea” males. “TAPk” represents the thermal activity peak; the shaded region and “TABr” represents the thermal activity breadth; the vertical dashed lines and “TAWn” represent the thermal activity window.
Comparison of Thermal Effects on Courtship Activity Within Species
Male and female E. binotata “V. prunifolium” thermal courtship activity curves were statistically similar, including the change in activity across temperatures (lack of statistically significant temperature × sex and temperature × temperature × sex interaction terms; Table 2) and overall courtship activity levels (lack of a significant sex term; Table 2, Figure 2). In contrast, the thermal courtship activity curves for male and female E. binotata “Ptelea” differed in both overall shape (significant temperature × temperature × sex term; Table 2, Figure 2) and overall activity level (significant sex term due to higher male courtship activity; Table 2, Figure 2).
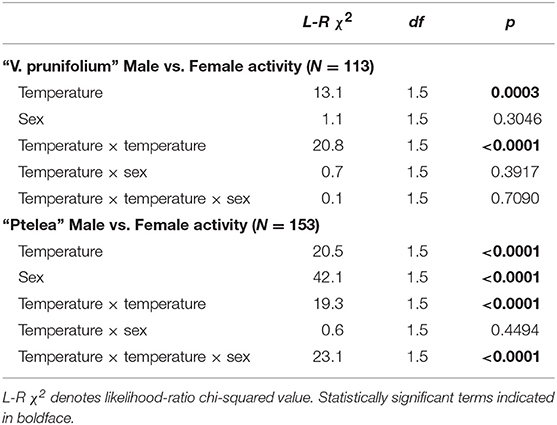
Table 2. Comparisons between the effects of temperature on activity level of males and females within E. binotata “V. prunifolium” and “Ptelea.”
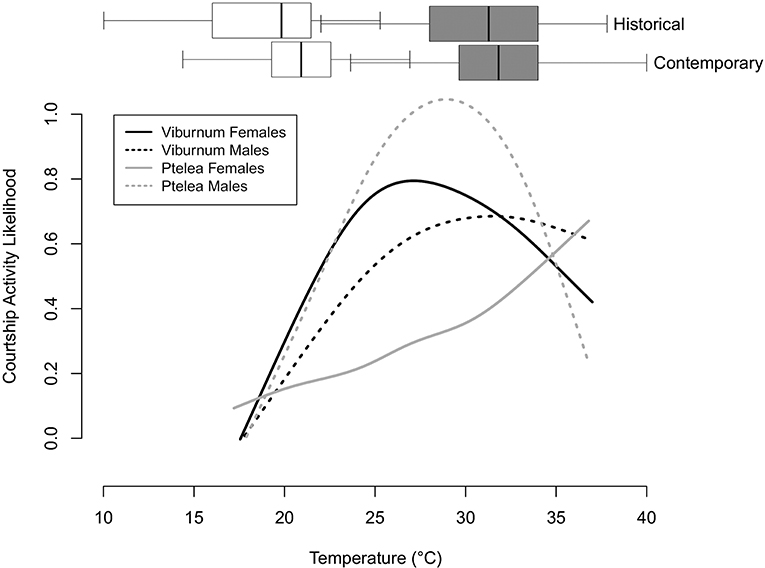
Figure 2. Each courtship activity curve represented in Figures 1A–D, featuring historical and contemporary daily high (shaded) and daily low temperatures (white) from June 1 through July 31 between 1975 and 1978 (historical), and June 1 through July 31 between 2015 and 2018 (contemporary). Temperature box plots are not affiliated with the Y-axis.
Comparison of Thermal Effects on Courtship Activity Across Species
For both males and females, the species differed in both the overall courtship activity levels (significant species term; Table 3), as well as the overall shape of the thermal courtship activity curve (significant temperature × temperature × species term, although this result is marginally not significant for males; p = 0.06; Table 3).
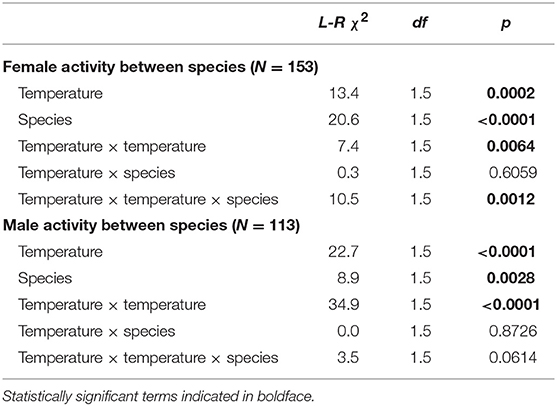
Table 3. Comparisons between the effects of temperature on activity level of males and females between E. binotata “V. prunifolium” and E. binotata “Ptelea.”
Qualitative Analyses of Thermal Courtship Activity Curves
Male and female thermal courtship activity curves were more similar to each other in E. binotata “V. prunifolium” than in E. binotata “Ptelea.” We found that thermal activity peak was 4.4°C higher in males vs. females in E. binotata “V. prunifolium,” while thermal activity window was barely 1°C wider in females, yet thermal activity breadth was 3°C wider in males than females (Table 4). Interestingly the thermal activity peak in E. binotata “Ptelea” was 7.9°C higher in females vs. males, and female thermal activity window was 1.3°C wider than in males. However, thermal activity breadth was 4.5°C wider in males vs. females (Table 4).
Historical and Contemporary Weather Data
The historical and contemporary temperature ranges differ: the average daily minimum temperature increased significantly from 17.99 to 19.94°C (F ratio = 42.6551, Prob > F = <0.0001) and the average daily high temperature also increased significantly from 30.08 to 30.78°C (F ratio = 4.5387, Prob > F = 0.0336). When comparing thermal courtship activity curves to contemporary thermal conditions during the mating season, we found that the thermal activity breadth of all the thermal courtship activity curves except E. binotata “Ptelea” females either completely or nearly overlap with intermediate daily temperatures (24–27°C) during the active mating season (Figure 2). We also found that the thermal activity peak for E. binotata “V. prunifolium” males aligns with the contemporary mean daily high temperature (~32°C), with females exhibiting courtship activity rates of >60% (Figure 2). In contrast, while male E. binotata “Ptelea” are still active at the contemporary mean daily high, females exhibit closer to ~40% activity levels at this temperature (Figure 2).
Discussion
We assessed two potential ways in which temperature-related breakdowns in the coordination of mating could occur in two sympatric species of E. binotata treehopper: reduced availability of optimal temperature ranges for actively finding mates and the accentuation of mismatches in activity between sexes. To do so, we quantified patterns of thermal sensitivity in male and female courtship activity rates and then compared these activity patterns with contemporary temperatures. We found that male and female E. binotata “V. prunifolium” differ in optimal temperature for courtship activity, but overall show similar changes in courtship activity across temperatures. Thus, E. binotata “V. prunifolium” shows high overlap between the sexes in activity levels at the mean daily temperature range during the mating season. In contrast, male and female E. binotata “Ptelea” not only differed in optimal temperatures for courtship activity, but also exhibited strikingly different thermal courtship activity curves. In fact, male and female E. binotata “Ptelea” overlap in activity in a narrower range of temperatures than the other species and at temperatures lower than mean daily temperatures.
If we extrapolate mating rates from courtship activity curves—mating rates correspond more closely with male activity curves in E. binotata “Ptelea” (Leith et al., in review)—we predict that E. binotata “Ptelea” will experience greater disruption of the coordination of mating with global warming. Male E. binotata “Ptelea” peak in courtship activity at a temperature lower than contemporary mean daily high temperatures and nearly 8°C lower than females. Furthermore, E. binotata “Ptelea” appear quite restricted in the range at which both males and females are actively signaling, with males only being minimally active at temperatures for which females are most active. On the other hand, E. binotata “V. prunifolium” appears to be more of a thermal generalist, with higher courtship activity across a broad range of temperatures; furthermore, while the male thermal activity peak was 4.4°C higher than females, male activity patterns were broad and overlapped substantially with that of females. Given the extent to which daily temperatures at our study site have risen the past 40 years, windows for mating may be greatly reduced for E. binotata “Ptelea” unless they can adapt to a broader and hotter range of temperatures (Hoffmann and Sgrò, 2011; Sinclair et al., 2012; Austin and Moehring, 2013). Adaptation to local conditions will be particularly important for species like those in the E. binotata complex. These insects have limited mobility and patchy habitats, reducing the likelihood of dispersal to more suitable thermal habitats, which is a common response to global warming (Sinervo et al., 2010; Sunday et al., 2012).
Divergence in the thermal response of the two species we studied could involve many underlying ecological and physiological mechanisms (Kleynhans et al., 2014). The two species could vary in historical patterns of environmental conditions (Sih et al., 2011; Tuomainen and Candolin, 2011; Foster, 2013). For example, species that have experienced historically high fluctuations in temperature will show patterns of being more temperature generalists (Kingsolver, 2009). While we do not know how the thermal microclimates of the two host plant species compare, it is possible that V. prunifolium plants experience greater thermal variation, shaping a more generalist response. Other environmental and/or demographic properties could also shape adaptation to temperature variation in addition to selection from historical thermal regimes (Foster, 2013; Tuff et al., 2016) that may account for the differences between females of the two species. For example, the two treehopper species may differ in the temperatures at which they are most active at to reduce competition or avoid predators (Greenfield, 2002; Bailey, 2003) on their respective host plants. Regardless of mechanism, our results indicate that host plant species and other ecological interactions could potentially influence the vulnerability of insect species to thermal change.
As we predicted based on host plant microhabitat, E. binotata “V. prunifolium” males were active at hotter temperatures than E. binotata “Ptelea” males. However, females did not follow the same pattern. E. binotata “V. prunifolium” females peaked at the same temperature at which E. binotata “Ptelea” female leveled off in their activity levels. Furthermore, E. binotata “Ptelea” females maintained high courtship activity through the highest testing temperature 36°C. This high testing temperature is also the point past which we start to see mortality in the laboratory (Jocson et al., 2019). It is notable that the E. binotata “Ptelea” female thermal courtship activity curve diverges from a typical performance curve. We do not have an explanation for why the patterns of sex-specific responses to temperature differ between species, or why E. binotata “Ptelea” females show such a distinct curve. However, more generally, potential causes of sex-specific thermal responses include differences between males and females in body size, physiological/metabolic costs of activity at hotter temperatures, or thermal by-products of sexual signaling (Block, 1994; Beaupre and Duvall, 1998; Cullum, 1998; Brown and Weatherhead, 2000; Kingsolver and Huey, 2008).
Differences among sexes in thermal courtship activity curves may affect mate coordination if sex-specific responses to temperature result in different daily activity patterns for males and females. Alternatively, sex-specific thermal courtship activity curves may have little effect on the coordination of mating if males and females utilize different thermal niches. For example, in crickets, males preferentially signal from warmer locations (Hedrick et al., 2002). In the host plant P. trifoliata, temperatures can vary over 5°C on a plant at a single point in time (Jocson et al., 2019), though how males and females utilize these temperatures is not yet known. Finally, it is also possible that patterns of activity in one sex may not predict the likelihood of mating occurring (e.g., Brandt et al., 2018). However, we found in E. binotata “Ptelea” that mating rates peak at the temperatures at which males are most likely to engage in courtship behavior (Leith et al., in review).
Sex-specific thermal courtship activity curves may also affect the mate selection process. In fiddler crabs, males court at very hot temperatures (Allen and Levinton, 2014), but also experience greater physiological stress at hotter temperatures when courting (Darnell et al., 2013). If only those males best able to withstand the stress can court at hot temperatures, females active at hotter temperatures may select the highest quality males (i.e., the handicap hypothesis; Cotton et al., 2004). However, for E. binotata “Ptelea,” variability in male courtship signals and female mate preferences are more variable at hotter temperatures (Jocson et al., 2019), suggesting activity at hotter temperatures may result in a reduced ability to discriminate high quality mates. Further studies on the effects of temperature on mating-related behaviors will be necessary to generate a broader framework for understanding how temperature affects mate coordination and mate selection processes.
The results of our study predict that species occupying similar habitats may experience divergent effects of global warming on the coordination of mating. We found both species-specific and sex-specific patterns of courtship activity across temperatures. While we focused on patterns of courtship activity, shifts in temperature outside of contemporary ranges could impact several aspects of reproduction, ranging from mate selection and mating duration to egg viability (Huey and Stevenson, 1979; Shreve et al., 2004; Katsuki and Miyatake, 2009; Conrad et al., 2017; Jocson et al., 2019). A better understanding of how animals alter courtship and other reproductive behaviors across adverse thermal conditions can provide critical insight as to whether certain populations are at increased risk of decline. In particular, clear sex differences in responses to temperature indicate that understanding the effects of temperature on reproduction for a broad range of taxa may require testing sex-specific effects of thermal variation. It is too early to determine the long-term effects on insect populations due to temperature-related breakdowns in the coordination of mating. However, the consequences of global warming for sexual communication and the coordination of mating deserves more attention and we hope this study inspires more research into mating under variable thermal conditions.
Data Availability Statement
The datasets generated for this study are available on request to the corresponding author.
Author Contributions
AM and KF-F designed the study. AM, NL, and DS collected data. AM, NL, and KF-F processed and analyzed the data. AM and KF-F were the primary authors with significant contributions from NL and DS.
Funding
Funding was provided by NSF IOS-1656818 to KF-F.
Conflict of Interest
The authors declare that the research was conducted in the absence of any commercial or financial relationships that could be construed as a potential conflict of interest.
Acknowledgments
We would like to thank E. Miller, C. Ballman, and S. Pidalta for helping with data collection, and M. T. Gonzales for generating the MATLAB script for playbacks. We would also like to thank M. FowlerFinn for design and construction of custom testing incubators and temperature sensors. Finally, we would like to thank two reviewers and S. Murphy for thoughtful feedback on the manuscript.
References
Abram, P. K., Boivin, G., Moiroux, J., and Brodeur, J. (2017). Behavioural effects of temperature on ectothermic animals: unifying thermal physiology and behavioural plasticity. Biol. Rev. 92, 1859–1876. doi: 10.1111/brv.12312
Allen, B. J., and Levinton, J. S. (2014). Sexual selection and the physiological consequences of habitat choice by a fiddler crab. Oecologia 176, 25–34. doi: 10.1007/s00442-014-3002-y
Angilletta, M. J. (2009). Thermal Adaptation: A Theoretical and Empirical Synthesis. Oxford University Press. Available online at: https://www.oxfordscholarship.com/view/10.1093/acprof:oso/9780198570875.001.1/acprof-9780198570875 (accessed August 13, 2019).
Angilletta, M. J., Niewiarowski, P. H., and Navas, C. A. (2002). The evolution of thermal physiology in ectotherms. J. Therm. Biol. 27, 249–268. doi: 10.1016/S0306-4565(01)00094-8
Austin, C. J., and Moehring, A. J. (2013). Optimal temperature range of a plastic species, Drosophila simulans. J. Anim. Ecol. 82, 663–672. doi: 10.1111/1365-2656.12041
Bailey, W. J. (2003). Insect duets: underlying mechanisms and their evolution. Physiol. Entomol. 28, 157–174. doi: 10.1046/j.1365-3032.2003.00337.x
Baudier, K. M., Mudd, A. E., Erickson, S. C., and O'Donnell, S. (2015). Microhabitat and body size effects on heat tolerance: implications for responses to climate change (army ants: Formicidae, Ecitoninae). J. Anim. Ecol. 84, 1322–1330. doi: 10.1111/1365-2656.12388
Beaupre, S. J., and Duvall, D. J. (1998). Integrative Biology of RattlesnakesContributions to biology and evolution. Bioscience 48, 531–538. doi: 10.2307/1313315
Block, B. A. (1994). Thermogenesis in muscle. Annu. Rev. Physiol. 56, 535–577. doi: 10.1146/annurev.ph.56.030194.002535
Brandt, E. E., Kelley, J. P., and Elias, D. O. (2018). Temperature alters multimodal signaling and mating success in an ectotherm. Behav. Ecol. Sociobiol. 72:191. doi: 10.1007/s00265-018-2620-5
Braschler, B., and Hill, J. K. (2007). Role of larval host plants in the climate-driven range expansion of the butterfly Polygonia c-album. J. Anim. Ecol. 76, 415–423. doi: 10.1111/j.1365-2656.2007.01217.x
Brown, G. P., and Weatherhead, P. J. (2000). Thermal ecology and sexual size Dimorphism in Northern Water Snakes, Nerodia Sipedon. Ecol. Monogr. 70, 311–330. doi: 10.1890/0012-9615(2000)070[0311:TEASSD]2.0.CO;2
Chinellato, F., Battisti, A., Finozzi, V., and Faccoli, M. (2014). Better today but worse tomorrow: how warm summers affect breeding performance of a Scots pine pest. Agrochimica 58, 133–145. doi: 10.1400/226737
Clusella-Trullas, S., Blackburn, T. M., and Chown, S. L. (2011). Climatic predictors of temperature performance curve parameters in ectotherms imply complex responses to climate change. Am. Nat. 177, 738–751. doi: 10.1086/660021
Cocroft, R. B., RodrÍGuez, R. L., and Hunt, R. E. (2008). Host Shifts, the Evolution of Communication, and Speciation in the Enchenopa binotata Species Complex of Treehoppers. University of California Press. Available online at: http://california.universitypressscholarship.com/view/10.1525/california/9780520251328.001.0001/upso-9780520251328-chapter-7 (accessed April 18, 2019).
Cocroft, R. B., Rodríguez, R. L., and Hunt, R. E. (2010). Host shifts and signal divergence: mating signals covary with host use in a complex of specialized plant-feeding insects. Biol. J. Linn. Soc. 99, 60–72. doi: 10.1111/j.1095-8312.2009.01345.x
Conrad, T., Stöcker, C., and Ayasse, M. (2017). The effect of temperature on male mating signals and female choice in the red mason bee, Osmia bicornis (L.). Ecol. Evol. 7, 8966–8975. doi: 10.1002/ece3.3331
Cotton, S., Fowler, K., and Pomiankowski, A. (2004). Do sexual ornaments demonstrate heightened condition-dependent expression as predicted by the handicap hypothesis? Proc. Biol. Sci. 271, 771–783. doi: 10.1098/rspb.2004.2688
Cullum, A. J. (1998). Sexual dimorphism in physiological performance of whiptail lizards (Genus Cnemidophorus). Physiol. Zool. 71, 541–552. doi: 10.1086/515961
Darnell, M. Z., Fowler, K. K., and Munguia, P. (2013). Sex-specific thermal constraints on fiddler crab behavior. Behav. Ecol. 24, 997–1003. doi: 10.1093/beheco/art006
Deutsch, C. A., Tewksbury, J. J., Huey, R. B., Sheldon, K. S., Ghalambor, C. K., Haak, D. C., et al. (2008). Impacts of climate warming on terrestrial ectotherms across latitude. Proc. Natl. Acad. Sci. U.S.A. 105, 6668–6672. doi: 10.1073/pnas.0709472105
Dillon, M. E., Wang, G., Garrity, P. A., and Huey, R. B. (2009). Review: thermal preference in Drosophila. J. Therm. Biol. 34, 109–119. doi: 10.1016/j.jtherbio.2008.11.007
Eberhard, W. (1994). Evidence for widespread courtship during copulation in 131 species of insects and spiders, and implications for cryptic female choice. Evolution 48, 711–733. doi: 10.1111/j.1558-5646.1994.tb01356.x
Ejima, A., and Griffith, L. C. (2007). Measurement of courtship behavior in Drosophila melanogaster. Cold Spring Harb. Protoc. 2007:pdb.prot4847. doi: 10.1101/pdb.prot4847
Fielding, C. A., Whittaker, J. B., Butterfield, J. E. L., and Coulson, J. C. (1999). Predicting responses to climate change: the effect of altitude and latitude on the phenology of the Spittlebug Neophilaenus lineatus. Funct. Ecol. 13, 65–73. doi: 10.1046/j.1365-2435.1999.00009.x
Foley, H. B., Sun, P. Y., Ramirez, R., So, B. K., Venkataraman, Y. R., Nixon, E. N., et al. (2019). Sex-specific stress tolerance, proteolysis, and lifespan in the invertebrate Tigriopus californicus. Exp. Gerontol. 119, 146–156. doi: 10.1016/j.exger.2019.02.006
Foster, S. A. (2013). Evolutionary insights from behavioural geography: plasticity, evolution, and responses to rapid environmental change. Evol. Ecol. Res. 15, 705–731.
Fowler-Finn, K. D., Cruz, D. C., and Rodríguez, R. L. (2017). Local population density and group composition influence the signal-preference relationship in Enchenopa treehoppers (Hemiptera: Membracidae). J. Evol. Biol. 30, 13–25. doi: 10.1111/jeb.12994
Fowler-Finn, K. D., and Rodríguez, R. L. (2012). Experience-mediated plasticity in mate preferences: mating assurance in a variable environment. Evolution 66, 459–468. doi: 10.1111/j.1558-5646.2011.01446.x
Frazier, M. R., Harrison, J. F., Kirkton, S. D., and Roberts, S. P. (2008). Cold rearing improves cold-flight performance in Drosophila via changes in wing morphology. J. Exp. Biol. 211, 2116–2122. doi: 10.1242/jeb.019422
Gallai, N., Salles, J.-M., Settele, J., and Vaissière, B. E. (2009). Economic valuation of the vulnerability of world agriculture confronted with pollinator decline. Ecol. Econom. 68, 810–821. doi: 10.1016/j.ecolecon.2008.06.014
García-Barros, E. (2000). Body size, egg size, and their interspecific relationships with ecological and life history traits in butterflies (Lepidoptera: Papilionoidea, Hesperioidea). Biol. J. Linn. Soc. 70, 251–284. doi: 10.1111/j.1095-8312.2000.tb00210.x
Gibert, J. P., Chelini, M.-C., Rosenthal, M. F., and DeLong, J. P. (2016). Crossing regimes of temperature dependence in animal movement. Glob. Change Biol. 22, 1722–1736. doi: 10.1111/gcb.13245
Greenfield, M. D. (2002). Signalers and Receivers: Mechanisms and Evolution of Arthropod Communication. Oxford, NY: Oxford University Press.
Greenfield, M. D., and Medlock, C. (2007). Temperature coupling as an emergent property: parallel thermal effects on male song and female response do not contribute to species recognition in an acoustic moth. Evolution 61, 1590–1599. doi: 10.1111/j.1558-5646.2007.00140.x
Guarneri, A. A., Lazzari, C., Xavier, A. A. P., Diotaiuti, L., and Lorenzo, M. G. (2003). The effect of temperature on the behaviour and development of Triatoma brasiliensis. Physiol. Entomol. 28, 185–191. doi: 10.1046/j.1365-3032.2003.00330.x
Hadjipantelis, P. Z., Jones, N., John, M., Springate, D., and Knight, C. G. (2013). Function-valued traits in evolution. J. R. Soc. Interface 10:20121032. doi: 10.1098/rsif.2012.1032
Hallmann, C. A., Sorg, M., Jongejans, E., Siepel, H., Hofland, N., Schwan, H., et al. (2017). More than 75 percent decline over 27 years in total flying insect biomass in protected areas. PLoS ONE 12:e0185809. doi: 10.1371/journal.pone.0185809
Hamilton, K. G. A., and Cocroft, R. B. (2009). Establishing the identity of existing names in the North American Enchenopa binotata species complex of treehoppers (Hemiptera: Membracidae). Entomol. News 120, 554–565. doi: 10.3157/021.120.0513
Hedrick, A., Perez, D., Lichti, N., and Yew, J. (2002). Temperature preferences of male field crickets (Gryllus integer) alter their mating calls. J. Comp. Physiol. A 188, 799–805. doi: 10.1007/s00359-002-0368-9
Hoffmann, A. A., and Sgrò, C. M. (2011). Climate change and evolutionary adaptation. Nature 470, 479–485. doi: 10.1038/nature09670
Høye, T. T., Post, E., Schmidt, N. M., Trøjelsgaard, K., and Forchhammer, M. C. (2013). Shorter flowering seasons and declining abundance of flower visitors in a warmer Arctic. Nat. Clim. Change 3, 759–763. doi: 10.1038/nclimate1909
Huey, R. B., and Stevenson, R. D. (1979). Integrating thermal physiology and ecology of ectotherms: a discussion of approaches. Integr. Comp. Biol. 19, 357–366. doi: 10.1093/icb/19.1.357
Hunt, R. E. (1994). Vibrational signals associated with mating behavior in the treehopper, Enchenopa binotata Say (Hemiptera: Homoptera: Membracidae). J. N. Y. Entomol. Soc. 102, 266–270.
Jocson, D. I., Smeester, M., Leith, N. T., Macchiano, A., and Fowler-Finn, K. D. (2019). Temperature coupling of mate attraction signals and female mate preferences in four populations of Enchenopa treehoppers (Hemiptera: Membracidae). J. Evol. Biol. doi: 10.1111/jeb.13506. [Epub ahead of print].
Katsuki, M., and Miyatake, T. (2009). Effects of temperature on mating duration, sperm transfer and remating frequency in Callosobruchus chinensis. J. Insect Physiol. 55, 113–116. doi: 10.1016/j.jinsphys.2008.10.012
Kellermann, V., Overgaard, J., Hoffmann, A. A., Fløjgaard, C., Svenning, J.-C., and Loeschcke, V. (2012). Upper thermal limits of Drosophila are linked to species distributions and strongly constrained phylogenetically. Proc. Natl. Acad. Sci. U.S.A. 109, 16228–16233. doi: 10.1073/pnas.1207553109
Kilmer, J. T., Fowler-Finn, K. D., Gray, D. A., Höbel, G., Rebar, D., Reichert, M. S., et al. (2017). Describing mate preference functions and other function-valued traits. J. Evol. Biol. 30, 1658–1673. doi: 10.1111/jeb.13122
King, A. M., and MacRae, T. H. (2015). Insect heat shock proteins during stress and Diapause. Annu. Rev. Entomol. 60, 59–75. doi: 10.1146/annurev-ento-011613-162107
Kingsolver, J. G. (2009). The well-temperatured biologist: (American Society of Naturalists Presidential Address). Am. Nat. 174, 755–768. doi: 10.1086/648310
Kingsolver, J. G., and Huey, R. B. (2008). Size, temperature, and fitness: three rules. Evol. Ecol. Res. 10, 251–268.
Kleynhans, E., Conlong, D. E., and Terblanche, J. S. (2014). Direct and indirect effects of development temperature on adult water balance traits of Eldana saccharina (Lepidoptera: Pyralidae). J. Insect Physiol. 68, 69–75. doi: 10.1016/j.jinsphys.2014.06.018
Lin, C. P., and Wood, T. K. (2002). Molecular phylogeny of the North American Enchenopa binotata (Homoptera: Membracidae) species complex. Ann. Entomol. Soc. Am. 95, 162–171. doi: 10.1603/0013-8746(2002)095[0162:MPOTNA]2.0.CO;2
Logan, M. L., Huynh, R. K., Precious, R. A., and Calsbeek, R. G. (2013). The impact of climate change measured at relevant spatial scales: new hope for tropical lizards. Glob. Change Biol. 19, 3093–3102. doi: 10.1111/gcb.12253
Losey, J. E., and Vaughan, M. (2006). The economic value of ecological services provided by insects. Bioscience 56:311. doi: 10.1641/0006-3568(2006)56[311:TEVOES]2.0.CO;2
Matzkin, L. M., Watts, T. D., and Markow, T. A. (2009). Evolution of stress resistance in Drosophila: interspecific variation in tolerance to desiccation and starvation. Funct. Ecol. 23, 521–527. doi: 10.1111/j.1365-2435.2008.01533.x
Meyer, K., and Kirkpatrick, M. (2005). Restricted maximum likelihood estimation of genetic principal components and smoothed covariance matrices. Genet. Sel. Evol. 37, 1–30. doi: 10.1186/1297-9686-37-1-1
Ollerton, J., Winfree, R., and Tarrant, S. (2011). How many flowering plants are pollinated by animals? Oikos 120, 321–326. doi: 10.1111/j.1600-0706.2010.18644.x
Pincebourde, S., and Woods, H. A. (2012). Climate uncertainty on leaf surfaces: the biophysics of leaf microclimates and their consequences for leaf-dwelling organisms. Funct. Ecol. 26, 844–853. doi: 10.1111/j.1365-2435.2012.02013.x
Rodríguez, R. L., and Cocroft, R. B. (2006). Divergence in female duetting signals in the Enchenopa binotata species complex of treehoppers (Hemiptera: Membracidae). Ethology 112, 1231–1238. doi: 10.1111/j.1439-0310.2006.01285.x
Rodríguez, R. L., Haen, C., Cocroft, R. B., and Fowler-Finn, K. D. (2012). Males adjust signaling effort based on female mate-preference cues. Behav. Ecol. 23, 1218–1225. doi: 10.1093/beheco/ars105
Rodríguez, R. L., Sullivan, L. E., and Cocroft, R. B. (2004). Vibrational communication and reproductive isolation in the Enchenopa Binotata species complex of treehoppers (hemiptera: Membracidae). Evolution 58, 571–578. doi: 10.1111/j.0014-3820.2004.tb01679.x
Schluter, D. (1988). Estimating the form of natural selection on a quantitative trait. Evolution 42, 849–861. doi: 10.1111/j.1558-5646.1988.tb02507.x
Shreve, S. M., Kelty, J. D., and Lee, R. E. Jr. (2004). Preservation of reproductive behaviors during modest cooling: rapid cold-hardening fine-tunes organismal response. J. Exp. Biol. 207, 1797–1802. doi: 10.1242/jeb.00951
Sih, A., Ferrari, M. C. O., and Harris, D. J. (2011). Evolution and behavioural responses to human-induced rapid environmental change. Evol. Appl. 4, 367–387. doi: 10.1111/j.1752-4571.2010.00166.x
Sinclair, B. J., Williams, C. M., and Terblanche, J. S. (2012). Variation in thermal performance among insect populations. Physiol. Biochem. Zool. 85, 594–606. doi: 10.1086/665388
Sinervo, B., Méndez-de-la-Cruz, F., Miles, D. B., Heulin, B., Bastiaans, E., Cruz, M. V.-S., et al. (2010). Erosion of lizard diversity by climate change and altered thermal niches. Science 328, 894–899. doi: 10.1126/science.1184695
Stinchcombe, J. R., and Kirkpatrick, M. (2012). Genetics and evolution of function-valued traits: understanding environmentally responsive phenotypes. Trends Ecol. Evol. 27, 637–647. doi: 10.1016/j.tree.2012.07.002
Suggitt, A. J., Gillingham, P. K., Hill, J. K., Huntley, B., Kunin, W. E., Roy, D. B., et al. (2011). Habitat microclimates drive fine-scale variation in extreme temperatures. Oikos 120, 1–8. doi: 10.1111/j.1600-0706.2010.18270.x
Sunday, J. M., Bates, A. E., and Dulvy, N. K. (2012). Thermal tolerance and the global redistribution of animals. Nat. Clim. Change 2, 686–690. doi: 10.1038/nclimate1539
Teal, J. M. (1959). Respiration of crabs in Georgia salt marshes and its relation to their ecology. Physiol. Zool. 32, 1–14. doi: 10.1086/physzool.32.1.30152287
Tuff, K. T., Tuff, T., and Davies, K. F. (2016). A framework for integrating thermal biology into fragmentation research. Ecol. Lett. 19, 361–374. doi: 10.1111/ele.12579
Tuomainen, U., and Candolin, U. (2011). Behavioural responses to human-induced environmental change. Biol. Rev. Camb. Philos. Soc. 86, 640–657. doi: 10.1111/j.1469-185X.2010.00164.x
Wood, S. N. (2006). Generalized Additive Models: An Introduction With R. Boca Raton, FL: Chapman and Hall/CRC Available at: https://www.crcpress.com/Generalized-Additive-Models-An-Introduction-with-R-Second-Edition/Wood/p/book/9781498728331 (accessed August 13, 2019).
Wood, T. K. (1980). Divergence in the Enchenopa binotata say complex (Homoptera: membracidae) effected by host plant adaptation. Evolution 34, 147–160. doi: 10.1111/j.1558-5646.1980.tb04800.x
Wood, T. K. (1993). Diversity in the new World Membracidae. Annu. Rev. Entomol. 38, 409–433. doi: 10.1146/annurev.en.38.010193.002205
Keywords: plasticity, vibrational communication, sex-specific responses, sympatry, mating behavior
Citation: Macchiano A, Sasson DA, Leith NT and Fowler-Finn KD (2019) Patterns of Thermal Sensitivity and Sex-Specificity of Courtship Behavior Differs Between Two Sympatric Species of Enchenopa Treehopper. Front. Ecol. Evol. 7:361. doi: 10.3389/fevo.2019.00361
Received: 27 May 2019; Accepted: 11 September 2019;
Published: 26 September 2019.
Edited by:
Shannon Murphy, University of Denver, United StatesReviewed by:
Erin Brandt, University of California, Berkeley, United StatesLaurel Symes, Cornell University, United States
Copyright © 2019 Macchiano, Sasson, Leith and Fowler-Finn. This is an open-access article distributed under the terms of the Creative Commons Attribution License (CC BY). The use, distribution or reproduction in other forums is permitted, provided the original author(s) and the copyright owner(s) are credited and that the original publication in this journal is cited, in accordance with accepted academic practice. No use, distribution or reproduction is permitted which does not comply with these terms.
*Correspondence: Anthony Macchiano, anthony.macchiano@slu.edu