- Department of Entomology, Cornell Agritech, Cornell University, Geneva, NY, United States
Urban soils differ from those in other managed ecosystems in many ways, including their heterogeneity, unique organic matter inputs and exposure to past and present anthropogenic activities. Soil processes in urban systems are influenced by the microbiome, specifically bacterial and fungal communities that are currently recognized as the primary drivers of soil organic matter dynamics. However, our understanding of biotic controls on microbial communities is incomplete, particularly in regard to the roles of invertebrates. We aim to highlight how invertebrates and their interactions with microbial communities may shape ecosystem processes in urban systems. We discuss three primary pathways through which invertebrates are known to influence the soil microbiome: dispersal of microorganisms throughout soils, grazing on microbial biomass, and mixing of organic inputs within soils and subsequently altering microbial resource accessibility. These invertebrate-mediated pathways may be particularly important because of their influence on soil microbiomes of urban systems. We also propose future research directions aimed at quantifying the influence of invertebrates on soil microbial processes to gain a more comprehensive understanding of urban microbiome function. Understanding the impact of invertebrates on the microbiome of urban systems can potentially lead to better management of microbiomes and enhance microbe-driven ecosystem services.
The Urban Soil Microbiome
Urban soils are typically defined first by the simple fact that they occur in urban areas. Beyond that, soils within urban areas are highly heterogeneous in their physical structure, chemical composition, temperature and moisture regimes, and land use among other characteristics (Lehmann and Stahr, 2007). Thus, urban soils can be defined in different ways and these definitions take into account the diverse impacts of human activities including physical disturbance and redistribution of the soil matrix, and chemical modification of soil by contaminants and other inputs (e.g., Lehmann and Stahr, 2007; Pouyat et al., 2009; Food Agriculture Organization of the United Nations, 2015; Morel et al., 2015). Despite the challenges this variability creates in defining an urban soil, urban soils are an important natural resource within urban ecosystems, supporting a wide variety of biotic communities and many ecosystem services (McIntyre, 2000; Tratalos et al., 2007; Gardiner et al., 2014; Ramirez et al., 2014; Setälä et al., 2014; Morel et al., 2015; Ossola et al., 2016; Herrmann et al., 2017; Pavao-Zuckerman and Pouyat, 2017; Anne et al., 2018; da Silva et al., 2018; Szlavecz et al., 2018).
As in other ecosystems, belowground processes in urban soils are heavily influenced by the communities of bacteria, fungi, and other microorganisms (i.e., the soil microbiome, Fierer, 2017) inhabiting them. These processes and ecosystem services include the breakdown and mineralization of nutrients, the formation of soil organic matter, and carbon sequestration (Van Der Heijden et al., 2008; Bradford et al., 2013; Cotrufo et al., 2013; Kallenbach et al., 2015, 2016). Such ecosystem services in urban soils are also critical for aboveground services of importance to human quality of life within cities, such as the maintenance of urban greenspaces. For example, soils in New York and Los Angeles, the United States' first and second largest cities by population, support roughly of one quarter and one third greenspace (parks and gardens), respectively (New York City Department of Planning, 2010; Los Angeles Department of Parks Recreation, 2016). Additionally, cities along the U.S. Rustbelt have tens to hundreds of square kilometers of vacant lots that contain soils that support other ecosystem services such as habitat and resources for urban wildlife and water filtration (Uno et al., 2010; Gardiner et al., 2013; Green et al., 2016; Herrmann et al., 2017).
While there is growing awareness of the potential importance of the soil microbiome within urban soils for maintaining belowground ecosystem services, studies of the factors that govern the urban soil microbiome, and its performance remain limited. Studies of the urban soil microbiome have focused primarily on the diversity of these communities and have found that abiotic factors such as pH and soil type are important drivers of microbial diversity (e.g., McGuire et al., 2013; Ramirez et al., 2014; Schmidt et al., 2017), as has been observed in non-urban systems (e.g., Fierer and Jackson, 2006; Lauber et al., 2009; Rousk et al., 2010). With a growing interest in urban soil ecosystem services, particularly for urban agriculture and responses to disturbances and global change, we must develop a more comprehensive understanding of the biotic controls on the urban soil microbiome. Plant communities have been identified as an important biotic control on par with the abiotic factors that control microbiomes such as moisture and nutrient availability (Fierer, 2017). However, our understanding of other biotic controls, especially the effects of soil-dwelling animals, is incomplete. Recent work has shown that the high variability observed in microbial community composition among different urban soil fragments (e.g., McGuire et al., 2013) is partly driven by soil invertebrate functional diversity, where increased invertebrate functional diversity can lead to increased microbial diversity (Bray et al., 2019). Yet, the actual mechanisms through which invertebrates affect microbial diversity and function have not been fully explored, especially in urban settings. In this perspective, we discuss how soil invertebrates have the potential to affect the diversity, biomass and activity of the microbiome and their influence on ecosystem services in urban soils.
Soil Invertebrate Community Composition and Function in Urban Soils
Invertebrate communities in urban soils can be taxonomically and functionally rich, containing detritivores, microbivores, predators and ecosystem engineers (e.g., Byrne and Bruns, 2004; Rochefort et al., 2006; Schrader and Böning, 2006; Byrne et al., 2008; Joimel et al., 2017). In some cases, urban soils even exhibit greater invertebrate abundances than in nearby natural systems (Philippot et al., 2013). Urban soil invertebrate communities are shaped by not only the inherent physical and chemical characteristics of urban soils but also heavily by past and present land use and management practices (Table 1). Many studies have highlighted that soil invertebrates are sensitive to many human activities and resulting soil conditions such as physical disturbance, metal contamination, pesticide inputs, site age and land use history (McIntyre, 2000; Pavao-Zuckerman, 2008; Pouyat et al., 2010; Jones and Leather, 2013; Table 1). While many anthropogenic activities have been shown to suppress the abundance and diversity of soil invertebrates, the direction and magnitude of the responses can also vary across different taxonomic groups. For instance, most invertebrates decrease in density in response to soil metal contamination (Nahmani and Lavelle, 2002; Santorufo et al., 2012; Pouyat et al., 2015); however, one group of soil macroinvertebrates, the isopods, has exhibited a positive density response to metal contamination in urban soils (Pouyat et al., 2015). Pesticides are also well-known to decrease microarthropod densities in urban soils; however, the effects can vary considerably with pesticide active ingredient, application rate, and frequency of use (Peck, 2009; Gan and Wickings, 2017). Other anthropogenic activities can have uniform positive effects on soil invertebrate communities. For example, the addition of organic matter is known to increase invertebrate densities across multiple taxonomic and functional groups in urban soils (Smith et al., 2006; Smetak et al., 2007; Byrne et al., 2008; Joimel et al., 2016, 2017).
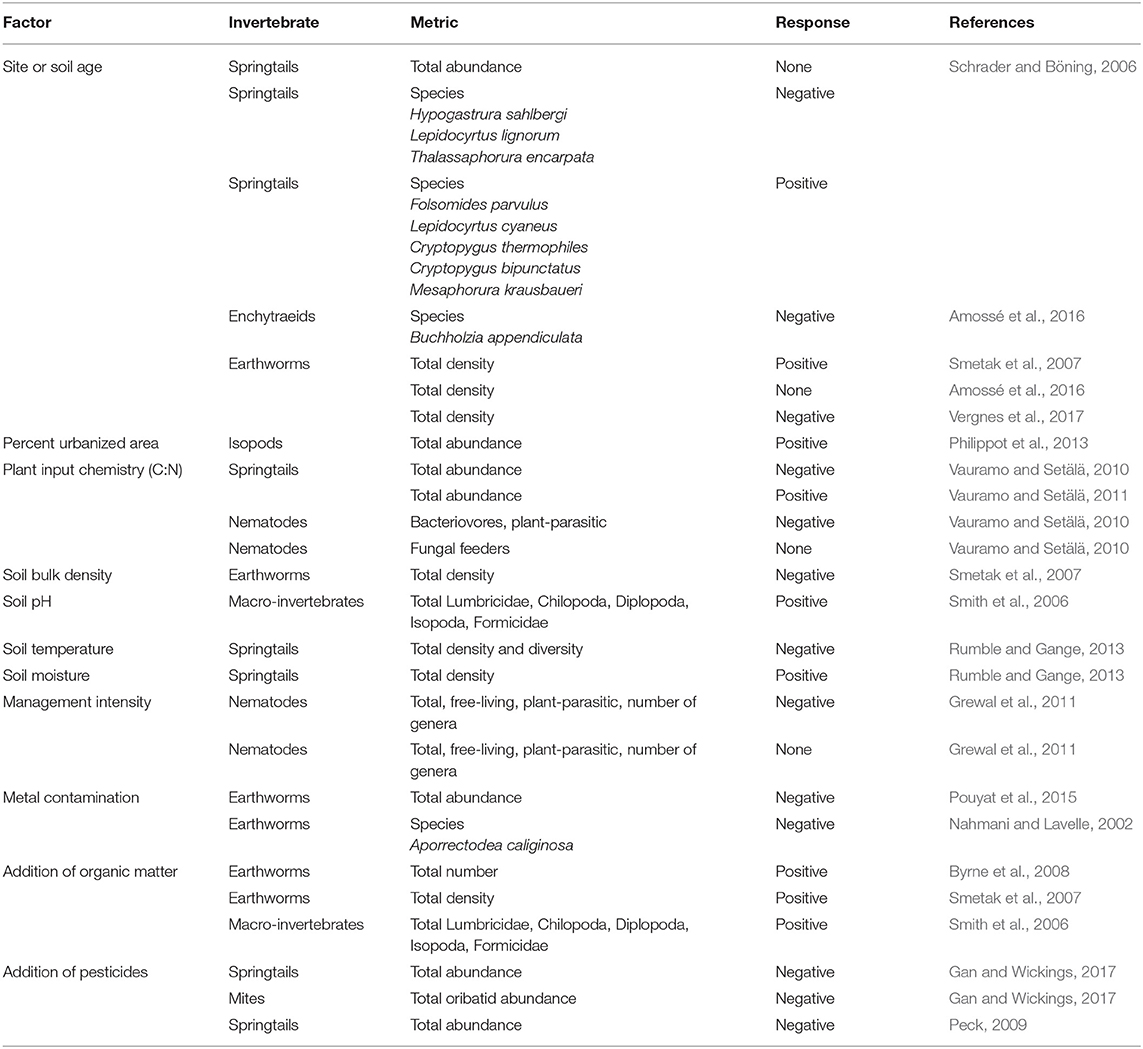
Table 1. Select factors affecting invertebrates within urban soils; the response of the invertebrate community is either to an increase in the factor or to the addition of the factor.
Anthropogenic influence, a defining feature of urban soils, is not always uniform, creating distinct pressures on invertebrate communities and site-specific variation across urban soil habitats and patches. If anthropogenic influences shaping invertebrate communities are in fact site-specific in urban soils, then their ecological function across different urban soils may be site-specific and therefore their effects on microbiomes may also be site-specific. It is unknown how altered invertebrate communities act as a biotic control on urban soil microbiomes and affect microbial community structure and function. Their importance may be high under some circumstances but may also vary considerably both within and among different urban areas such as lawns, gardens, vacant lots, and green roofs. This presents a challenge for assessing and predicting ecosystem services that are linked to soil invertebrates and the ecosystem services provided by soil microorganisms because of the multiple pathways through which invertebrates influence microbial community composition and function. Invertebrate effects on microbial function therefore require targeted investigations to fully gauge their impacts on the urban soil microbiome and the ecosystem services it provides.
To explore the roles of invertebrates in the urban soil microbiome, we selected the invertebrates classified as microbivores and/or detritivores given their potential to alter microbial communities. Furthermore, we identified three pathways through which invertebrates affect microbial processes: (1) microorganism dispersal, (2) grazing on microorganisms, and (3) fragmentation and mixing of organic matter resulting in altered resource accessbility for microorganisms (Figure 1). Through these direct and indirect pathways, invertebrates have been shown to exert strong controls on microbiome composition, activity and biomass, suggesting that invertebrates have the potential to influence microbial processes (Grandy et al., 2016). In the following sections, we highlight important future research directions to clarify the roles of invertebrates on microbial community composition and microbe-driven ecosystem services in the unique context of urban soil ecosystem.
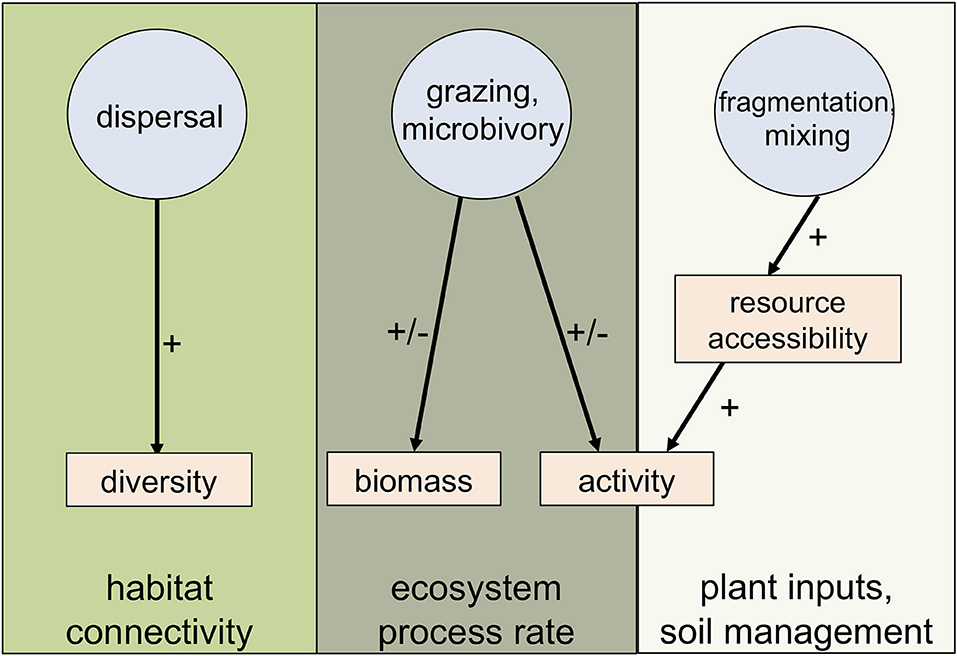
Figure 1. Three proposed invertebrate pathways that alter microbial communities in urban soils; invertebrates either have positive (+) and/or negative (−) effects on the different microbial response metrics and interactions between the different invertebrate pathways and the microbial metric affect distinct soil processes.
The Role of Invertebrates in Microbial Dispersal and in Shaping Urban Soil Microbiome Structure
The physical dispersal of microorganisms through soil may be a particularly important mechanism by which invertebrates influence community assembly and composition of the urban soil microbiome. Despite the potentially high cumulative area of urban greenspace soils, soil habitats in urban systems can be patchy and not well connected. Examples of urban patchy habitats include urban gardens, parks, green roofs, and street planters. Elucidating the relationships between invertebrate and microbial dispersal in urban habitats will help to determine whether invertebrates facilitate connectivity for less mobile soil microorganisms.
Studies have shown that soil invertebrates can transport both free-living and plant symbiotic microorganisms through gut passage, fecal deposition, and passive transport on their exoskeletons (McIlveen and Cole, 1976; Rabatin and Stinner, 1985; Gange, 1993; Moody et al., 1996; Lilleskov and Bruns, 2005). These studies were conducted in laboratory settings and non-urban soils; therefore, the roles of invertebrates in transporting microorganisms in urban soils and how this shapes microbial community composition in urban soils is an important future research direction. One key group of invertebrate-transported microorganisms are mycorrhizal fungi, which have positive effects on plant nutrient uptake, productivity and diversity (Van Der Heijden et al., 1998) and consequently soil ecosystem processes. In urban soils, factors such as habitat fragmentation, and pollution have the potential to negatively affect fungal diversity (Newbound et al., 2010). Therefore, exploring invertebrates as dispersal agents and promoters of these beneficial symbiotic relationships in urban soils may provide a unique ecosystem service, particularly in newly established soils. However, invertebrate transport of microorganism is not limited to beneficial symbiotic microorganisms. Invertebrates can transport other organisms such as seeds and plant pathogens (Thompson et al., 1994; Friberg et al., 2005; Eisenhauer et al., 2009). Limited work on this in urban soils has shown that invertebrates can play important roles in seedling recruitment and plant diversity in urban ecosystems (e.g., Sperling and Lortie, 2010) indicating potential for dispersal of weedy species. The net effects of plant-invertebrate-microbe interactions generally need to be further explored in urban soils, particularly if invertebrates are to be considered a tool for promoting microbial diversity.
Furthermore, to fully gauge the importance of this mechanism, a deeper understanding of invertebrate movement among urban soils is key to understanding their influence over soil microbiome formation and structure within urban soils and across urban soil fragments. While some work has shown that green spaces and corridors can help mobile invertebrates disperse across urban landscapes (Vergnes et al., 2012; Braaker et al., 2014), general principles regarding routes of colonization or constraints on soil invertebrate dispersal and population establishment within isolated urban soil fragments are lacking. This is relevant for not only the movement of native soil invertebrates but also for invasive soil fauna, such as the Asian jumping worms (Amynthas and Metaphire spp.), which disperse rapidly through urban habitats (Hale, 2008; Greiner et al., 2012; Qiu and Turner, 2017) and are known to alter soil microbial communities (Chang et al., 2018). Clarifying the relationships between invertebrate and microbial dispersal in urban habitats will help to determine whether invertebrates facilitate connectivity for less mobile soil microorganisms within urban soil habitats and across urban soil patches. This should be addressed by establishing long-term studies to characterize the colonization of newly established soils in urban ecosystems or re-colonization of defaunated soils of varying patch sizes and degrees of connectivity and identity surrounding source pools of potential colonizing invertebrates, both native and invasive, and assess changes in the soil microbiome.
The Effects of Invertebrate Grazing on the Size and Activity of the Urban Soil Microbiome
The size, or biomass, of the soil microbial community is a widely-used metric to assess the capacity of the microbiome to provision ecosystem services (Zak et al., 2003; Jangid et al., 2008; Van Der Heijden et al., 2008; Fierer et al., 2009; Rinkes et al., 2013; Serna-Chavez et al., 2013; Wagg et al., 2014) and there is growing interest in using it as a soil health metric (Gonzalez-Quiñones et al., 2011). While microbial biomass is influenced by many abiotic factors, biotic factors such as soil invertebrate grazing on bacteria and fungi can also impact it. Microbial grazing (a.k.a. microbivory) has been identified as one of the main pathways through which soil invertebrates can affect not only the microbial community but also soil biogeochemical processes (Grandy et al., 2016); however, its importance under field settings is unclear. We propose that investigating invertebrate effects on microbial biomass in urban soils under different invertebrate diversity and density scenarios will elucidate invertebrate controls on this important soil health metric and lead to better predictions of microbial biomass responses in recently disturbed or newly formed urban soils.
Previous studies have demonstrated that microbivory can either increase or decrease microbial biomass and activity and a review by Crowther et al. (2012) suggests that observed differences in the direction and magnitude of microbial responses to microbivory are linked to grazer identity and density. For example, the effects of microarthropods (e.g., springtails) stimulate fungal growth, whereas macroinvertebrates such as isopods decrease fungal growth (Crowther et al., 2011; Crowther and A'Bear, 2012). Grazer density also determines the responses of microbial communities to microbivory with low-intensity grazing leading to higher microbial biomass and activity and high-intensity grazing leading to decreased microbial biomass and activity (Lenoir et al., 2007; Crowther and A'Bear, 2012; Crowther et al., 2012). This is not only true for free-living saprotrophic microorganisms, but also for root-symbiotic microorganisms such as mycorrhizal fungi (Klironomos and Kendrick, 1996). Root-symbiotic microorganisms can be altered by the presence of invasive earthworms in urban soils leading to changes in organic matter in the rhizosphere and fine root growth (Baxter et al., 1999). Despite the variability in invertebrate effects on microbial biomass, one of the primary consequences of invertebrate grazing on microbial biomass is an increase in nitrogen availability (Anderson, 1988; Osler and Sommerkorn, 2007), indicating that by grazing on microorganisms, soil invertebrates play important roles in soil nitrogen cycles and potentially promote or reduce soil fertility.
Given that changes in microbial biomass and activity have implications for soil microbiome-derived ecosystem services, understanding the biotic interactions that contribute to changes in microbial biomass and activity is essential. Integrated studies exploring concurrent patterns of both microbial and invertebrate population dynamics specifically in urban soils would provide more insight into how the microbiome may change with invertebrate community composition and density. Additionally, manipulative field studies using fauna exclusions or fauna additions and assessing microbiome responses in urban soils can provide important insights into community-level changes due to soil invertebrates. This could potentially elucidate an important pathway through which invertebrates mediate microbially-driven ecosystem services through their effects on the urban soil microbiome.
The Roles of Invertebrates in Organic Matter Fragmentation and Mixing in Urban Soils
Many soil invertebrates are involved in the mixing and breakdown of plant residue inputs to soil (Chamberlain et al., 2006). As such, invertebrates alter the physical and chemical composition and accessibility of organic matter in soil, which can influence microbial resource acquisition and resource use efficiency (Scheu and Wolters, 1991; Wardle, 2002; Wieder et al., 2014). Yet, the impact of invertebrates on plant residue breakdown is known to vary with residue chemistry (Garcia-Palacios et al., 2013; Suzuki et al., 2013) suggesting that their importance as moderators of resource inputs for the urban soil microbiome may depend heavily on the type or amount of organic matter input a system receives.
Organic matter inputs across urban soil habitats can range widely in physical and chemical composition (Lorenz and Lal, 2009) from tree leaf litter in urban forests to grass clippings and root exudates in lawns. Organic matter is also frequently added to urban soils in the form of mulches, compost, biosolids, biostimulants, and high-organic matter topsoil. Along with their addition, it is also common practice to remove organic matter from some urban soils (e.g., lawn and leaf waste) which can reduce organic matter inputs to soils (Craul, 1985; Byrne et al., 2008). Such notable modifications to organic matter within urban soils can alter microbial activity and decomposition (Byrne et al., 2008; Carlson et al., 2015), modify plant nutrient availability (Beniston et al., 2016) and impact carbon storage (Beesley, 2012). There is general evidence that the composition of the decomposer community can affect the rate of decomposition in urban soils through interactions between inputs, invertebrates and microorganism (Ossola et al., 2016; Jusselme et al., 2019; Tresch et al., 2019); however, the importance of invertebrates in shaping organic matter-microbe interactions in urban soils is not fully understood. We therefore need to account for all the potential consequences of alterations to organic matter while supporting management goals within urban soils. Future work should focus on targeted approaches to assess invertebrate contributions to microbial resource accessibility and how resource accessibility alters the structure and function of the urban soil microbiome. This could include tracking microbiome responses in field studies with wide-ranging input chemistries such as labile grass clippings to compost to tree leaves in which invertebrate communities are present or excluded.
Conclusions
Urban soils are a unique habitat for microorganisms and invertebrates, and there is ample evidence that biota can be both abundant and diverse within urban soils (e.g., McIntyre, 2000; Tratalos et al., 2007; Gardiner et al., 2014; Ramirez et al., 2014; Setälä et al., 2014; Ossola et al., 2016; Szlavecz et al., 2018). Yet, going beyond characterization and moving to management of microbiomes to increase plant productivity and bolster other ecosystem services is an important future direction for urban soil ecology research. Therefore, identifying and assessing all the biotic pathways that can change microbiomes is key to achieving this management goal. Through the three pathways identified here, soil invertebrates may be particularly important drivers of soil microbiome function in urban soils. Invertebrate-microbe interactions in urban soil may be site-specific given the variability within and across urban areas. Advancing knowledge of invertebrate-microbe interactions in urban soils has the potential to improve our ability to make more accurate predictions about belowground biogeochemical processes across diverse urban soils. Many unknowns remain surrounding the relative importance of invertebrates for predicting soil microbial processes under different urban soil conditions but targeted approaches that investigate invertebrate-microbe interactions in urban soils may eventually increase our ability to manage microbiomes and the ecosystem services they provide.
Author Contributions
Both authors contributed to the writing of this work and have approved it for publication.
Funding
NB and KW were supported by funding from the College of Agricultural and Life Sciences at Cornell University.
Conflict of Interest
The authors declare that the research was conducted in the absence of any commercial or financial relationships that could be construed as a potential conflict of interest.
Acknowledgments
The authors would like to thank the two reviewers for their feedback and suggestions that improved the paper.
References
Amossé, J., Dózsa-Farkas, K., Boros, G., Rochat, G., Sandoz, G., Fournier, B., et al. (2016). Patterns of earthworm, enchytraeid and nematode diversity and community structure in urban soils of different ages. Eur. J. Soil Biol. 73, 46–58. doi: 10.1016/j.ejsobi.2016.01.004
Anderson, J. M. (1988). Spatiotemporal effects of invertebrates on soil processes. Biol. Fertil. Soils 6, 216–227. doi: 10.1007/BF00260818
Anne, B., Geoffroy, S., Cherel, J., Warot, G., Marie, S., Noël, C. J., et al. (2018). Towards an operational methodology to optimize ecosystem services provided by urban soils. Landsc. Urban Plan. 176, 1–9. doi: 10.1016/j.landurbplan.2018.03.019
Baxter, J. W., Pickett, S. T. A., Carreiro, M. M., and Dighton, J. (1999). Ectomycorrhizal diversity and community structure in oak forest stands exposed to contrasting anthropogenic impacts. Can. J. Bot. 77, 771–782. doi: 10.1139/b99-039
Beesley, L. (2012). Carbon storage and fluxes in existing and newly created urban soils. J. Environ. Manage. 104, 158–165. doi: 10.1016/j.jenvman.2012.03.024
Beniston, J. W., Lal, R., and Mercer, K. L. (2016). Assessing and managing soil quality for urban agriculture in a degraded vacant lot soil. Land Degrad. Dev. 27, 996–1006. doi: 10.1002/ldr.2342
Braaker, S., Ghazoul, J., Obrist, M. K., and Moretti, M. (2014). Habitat connectivity shapes urban arthropod communities: the key role of green roofs. Ecology 95, 1010–1021. doi: 10.1890/13-0705.1
Bradford, M. A., Keiser, A. D., Davies, C. A., Mersmann, C. A., and Strickland, M. S. (2013). Empirical evidence that soil carbon formation from plant inputs is positively related to microbial growth. Biogeochemistry 113, 271–281. doi: 10.1007/s10533-012-9822-0
Bray, N., Kao-Kniffin, J., Frey, S. D., Fahey, T. J., and Wickings, K. (2019). Soil macroinvertebrate presence alters microbial community composition and activity in the rhizosphere. Front. Microbiol. 10:256. doi: 10.3389/fmicb.2019.00256
Byrne, L. B., and Bruns, M. A. (2004). The effects of lawn management on soil microarthropods. J. Agric. Urban Entomol. 21, 150–156.
Byrne, L. B., Bruns, M. A., and Kim, K. C. (2008). Ecosystem properties of urban land covers at the aboveground–belowground interface. Ecosystems 11, 1065–1077. doi: 10.1007/s10021-008-9179-3
Carlson, J., Saxena, J., Basta, N., Hundal, L., Busalacchi, D., and Dick, R. P. (2015). Application of organic amendments to restore degraded soil: effects on soil microbial properties. Environ. Monit. Assess. 187:109. doi: 10.1007/s10661-015-4293-0
Chamberlain, P. M., McNamara, N. P., Chaplow, J., Stott, A. W., and Black, H. I. J. (2006). Translocation of surface litter carbon into soil by Collembola. Soil Biol. Biochem. 38, 2655–2664. doi: 10.1016/j.soilbio.2006.03.021
Chang, C.-H., Johnston, M. R., Görres, J. H., Dávalos, A., McHugh, D., and Szlavecz, K. (2018). Co-invasion of three Asian earthworms, Metaphire hilgendorfi, Amynthas agrestis and Amynthas tokioensis in the USA. Biol. Invasions 20, 843–848. doi: 10.1007/s10530-017-1607-x
Cotrufo, M. F., Wallenstein, M. D., Boot, C. M., Denef, K., and Paul, E. (2013). The Microbial Efficiency-Matrix Stabilization (MEMS) framework integrates plant litter decomposition with soil organic matter stabilization: do labile plant inputs form stable soil organic matter? Glob. Change Biol. 19, 988–995. doi: 10.1111/gcb.12113
Craul, P. J. (1985). A description of urban soils and their desired characteristics. J. Arboric. 11, 330–339.
Crowther, T. W., and A'Bear, A. D. (2012). Impacts of grazing soil fauna on decomposer fungi are species-specific and density-dependent. Fungal Ecol. 5, 277–281 doi: 10.1016/j.funeco.2011.07.006
Crowther, T. W., Boddy, L., and Jones, T. H. (2011). Outcomes of fungal interactions are determined by soil invertebrate grazers. Ecol. Lett. 14, 1134–1142. doi: 10.1111/j.1461-0248.2011.01682.x
Crowther, T. W., Boddy, L., and Jones, T. H. (2012). Functional and ecological consequences of saprotrophic fungus–grazer interactions. ISME J. 6, 1992–2001. doi: 10.1038/ismej.2012.53
da Silva, R. T., Fleskens, L., van Delden, H., and van der Ploeg, M. (2018). Incorporating soil ecosystem services into urban planning: status, challenges and opportunities. Landsc. Ecol. 33, 1087–1102. doi: 10.1007/s10980-018-0652-x
Eisenhauer, N., Schuy, M., Butenschoen, O., and Scheu, S. (2009). Direct and indirect effects of endogeic earthworms on plant seeds. Pedobiologia 52, 151–162. doi: 10.1016/j.pedobi.2008.07.002
Fierer, N. (2017). Embracing the unknown: disentangling the complexities of the soil microbiome. Nat. Rev. Microbiol. 15, 579–590. doi: 10.1038/nrmicro.2017.87
Fierer, N., and Jackson, R. B. (2006). The diversity and biogeography of soil bacterial communities. Proc. Natl. Acad. Sci. U.S.A. 103, 626–631 doi: 10.1073/pnas.0507535103
Fierer, N., Strickland, M. S., Liptzin, D., Bradford, M. A., and Cleveland, C. C. (2009). Global patterns in belowground communities. Ecol. Lett. 12, 1238–1249. doi: 10.1111/j.1461-0248.2009.01360.x
Food Agriculture Organization of the United Nations (2015). World Reference Base for Soil Resources 2014. Available online at: http://www.fao.org/3/i3794en/I3794en.pdf (accessed February 1, 2018).
Friberg, H., Lagerlöf, J., and Rämert, B. (2005). Influence of soil fauna on fungal plant pathogens in agricultural and horticultural systems. Biocontrol Sci. Technol. 15, 641–658. doi: 10.1080/09583150500086979
Gan, H., and Wickings, K. (2017). Soil ecological responses to pest management in golf turf vary with management intensity, pesticide identity, and application program. Agric. Ecosyst. Environ. 246, 66–77. doi: 10.1016/j.agee.2017.05.014
Gange, A. C. (1993). Translocation of mycorrhizal fungi by earthworms during early succession. Soil Biol. Biochem. 25, 1021–1026. doi: 10.1016/0038-0717(93)90149-6
Garcia-Palacios, P., Maestre, F. T., Kattge, J., and Wall, D. H. (2013). Climate and litter quality differently modulate the effects of soil fauna on litter decomposition across biomes. Ecol. Lett. 16, 1045–1053. doi: 10.1111/ele.12137
Gardiner, M. M., Burkman, C. E., and Prajzner, S. P. (2013). The value of urban vacant land to support arthropod biodiversity and ecosystem services. Environ. Entomol. 42, 1123–1136. doi: 10.1603/EN12275
Gardiner, M. M., Prajzner, S. P., Burkman, C. E., Albro, S., and Grewal, P. S. (2014). Vacant land conversion to community gardens: influences on generalist arthropod predators and biocontrol services in urban greenspaces. Urban Ecosyst. 17, 101–122. doi: 10.1007/s11252-013-0303-6
Gonzalez-Quiñones, V., Stockdale, E. A., Banning, N. C., Hoyle, F. C., Sawada, Y., Wherrett, A. D., et al. (2011). Soil microbial biomass interpretation and consideration for soil monitoring. Soil Res. 49, 287–304. doi: 10.1071/SR10203
Grandy, A. S., Wieder, W. R., Wickings, K., and Kyker-Snowman, E. (2016). Beyond microbes: are fauna the next frontier in soil biogeochemical models? Soil Biol. Biochem. 102, 40–44. doi: 10.1016/j.soilbio.2016.08.008
Green, O. O., Garmestani, A. S., Albro, S., Ban, N. C., Berland, A., Burkman, C. E., et al. (2016). Adaptive governance to promote ecosystem services in urban green spaces. Urban Ecosyst. 19, 77–93. doi: 10.1007/s11252-015-0476-2
Greiner, H. G., Kashian, D. R., and Tiegs, S. D. (2012). Impacts of invasive Asian (Amynthas hilgendorfi) and European (Lumbricus rubellus) earthworms in a North American temperate deciduous forest. Biol. Invasions 14, 2017–2027. doi: 10.1007/s10530-012-0208-y
Grewal, S. S., Cheng, Z., Masih, S., Wolboldt, M., Huda, N., Knight, A., et al. (2011). An assessment of soil nematode food webs and nutrient pools in community gardens and vacant lots in two post-industrial American cities. Urban Ecosyst. 14, 181–194. doi: 10.1007/s11252-010-0146-3
Hale, C. M. (2008). Evidence for human-mediated dispersal of exotic earthworms: support for exploring strategies to limit further spread. Mol. Ecol. 17, 1165–1167. doi: 10.1111/j.1365-294X.2007.03678.x
Herrmann, D. L., Shuster, W. D., and Garmestani, A. S. (2017). Vacant urban lot soils and their potential to support ecosystem services. Plant Soil 413, 45–57. doi: 10.1007/s11104-016-2874-5
Jangid, K., Williams, M. A., Franzluebbers, A. J., Sanderlin, J. S., Reeves, J. H., Jenkins, M. B., et al. (2008). Relative impacts of land-use, management intensity and fertilization upon soil microbial community structure in agricultural systems. Soil Biol. Biochem. 40, 2843–2853. doi: 10.1016/j.soilbio.2008.07.030
Joimel, S., Cortet, J., Jolivet, C. C., Saby, N. P. A., Chenot, E.-D., Branchu, P., et al. (2016). Physico-chemical characteristics of topsoil for contrasted forest, agricultural, urban and industrial land uses in France. Sci. Total Environ. 545, 40–47. doi: 10.1016/j.scitotenv.2015.12.035
Joimel, S., Schwartz, C., Hedde, M., Kiyota, S., Krogh, P. H., Nahmani, J., et al. (2017). Urban and industrial land uses have a higher soil biological quality than expected from physicochemical quality. Sci. Total Environ. 584, 614–621. doi: 10.1016/j.scitotenv.2017.01.086
Jones, E. L., and Leather, S. R. (2013). Invertebrates in urban areas: a review. EJE 109, 463–478. doi: 10.14411/eje.2012.060
Jusselme, M. D., Pruvost, C., Motard, E., Giusti-Miller, S., Frechault, S., Alphonse, V., et al. (2019). Increasing the ability of a green roof to provide ecosystem services by adding organic matter and earthworms. Appl. Soil Ecol. 143, 61–69. doi: 10.1016/j.apsoil.2019.05.028
Kallenbach, C. M., Frey, S. D., and Grandy, A. S. (2016). Direct evidence for microbial-derived soil organic matter formation and its ecophysiological controls. Nat. Commun. 7:13630. doi: 10.1038/ncomms13630
Kallenbach, C. M., Grandy, A. S., Frey, S. D., and Diefendorf, A. F. (2015). Microbial physiology and necromass regulate agricultural soil carbon accumulation. Soil Biol. Biochem. 91, 279–290. doi: 10.1016/j.soilbio.2015.09.005
Klironomos, J. N., and Kendrick, W. B. (1996). Palatability of microfungi to soil arthropods in relation to the functioning of arbuscular mycorrhizae. Biol. Fertil. Soils 21, 43–52. doi: 10.1007/BF00335992
Lauber, C. L., Hamady, M., Knight, R., and Fierer, N. (2009). Pyrosequencing-based assessment of soil pH as a predictor of soil bacterial community structure at the continental scale. Appl. Environ. Microbiol. 75, 5111–5120. doi: 10.1128/AEM.00335-09
Lehmann, A., and Stahr, K. (2007). Nature and significance of anthropogenic urban soils. J. Soils Sediments 7, 247–260. doi: 10.1065/jss2007.06.235
Lenoir, L., Persson, T., Bengtsson, J., Wallander, H., and Wiren, A. (2007). Bottom–up or top–down control in forest soil microcosms? Effects of soil fauna on fungal biomass and C/N mineralisation. Biol. Fertil. Soils 43, 281–294. doi: 10.1007/s00374-006-0103-8
Lilleskov, E. A., and Bruns, T. D. (2005). Spore dispersal of a resupinate ectomycorrhizal fungus, Tomentella sublilacina, via soil food webs. Mycologia 97, 762–769. doi: 10.1080/15572536.2006.11832767
Lorenz, K., and Lal, R. (2009). Biogeochemical C and N cycles in urban soils. Environ. Int. 35, 1–8. doi: 10.1016/j.envint.2008.05.006
Los Angeles Department of Parks Recreation (2016). Los Angeles Countywide Comprehensive Parks and Recreation Needs Assessment. Available online at: https://lacountyparkneeds.org/final-report/ (accessed February 1, 2018).
McGuire, K. L., Payne, S. G., Palmer, M. I., Gillikin, C. M., Keefe, D., Kim, S. J., et al. (2013). Digging the New York City Skyline: soil fungal communities in green roofs and city parks. PLoS ONE 8:e58020. doi: 10.1371/journal.pone.0058020
McIlveen, W. D., and Cole, H. Jr. (1976). Spore dispersal of Endogonaceae by worms, ants, wasps, and birds. Can. J. Bot. 54, 1486–1489. doi: 10.1139/b76-161
McIntyre, N. E. (2000). Ecology of urban arthropods: a review and a call to action. Ann. Entomol. Soc. Am. 93, 825–835. doi: 10.1603/0013-8746(2000)093[0825:EOUAAR]2.0.CO;2
Moody, S. A., Piearce, T. G., and Dighton, J. (1996). Fate of some fungal spores associated with wheat straw decomposition on passage through the guts of Lumbricus terrestris and Aporrectodea longa. Soil Biol. Biochem. 28, 533–537. doi: 10.1016/0038-0717(95)00172-7
Morel, J. L., Chenu, C., and Lorenz, K. (2015). Ecosystem services provided by soils of urban, industrial, traffic, mining, and military areas (SUITMAs). J. Soils Sediments 15, 1659–1666. doi: 10.1007/s11368-014-0926-0
Nahmani, J., and Lavelle, P. (2002). Effects of heavy metal pollution on soil macrofauna in a grassland of Northern France. Eur. J. Soil Biol. 38, 297–300. doi: 10.1016/S1164-5563(02)01169-X
New York City Department of Planning (2010). New York City Department of Planning NYC Open Data. Available online at: https://data.cityofnewyork.us/browse (accessed February 1, 2018)
Newbound, M., Mccarthy, M. A., and Lebel, T. (2010). Fungi and the urban environment: a review. Landsc. Urban Plan. 96, 138–145. doi: 10.1016/j.landurbplan.2010.04.005
Osler, G. H. R., and Sommerkorn, M. (2007). Toward a complete soil C and N cycle: incorporating the soil fauna. Ecology 88, 1611–1621. doi: 10.1890/06-1357.1
Ossola, A., Hahs, A. K., Nash, M. A., and Livesley, S. J. (2016). Habitat complexity enhances comminution and decomposition processes in urban ecosystems. Ecosystems 19, 927–941. doi: 10.1007/s10021-016-9976-z
Pavao-Zuckerman, M., and Pouyat, R. V (2017). “The effects of urban expansion on soil health and ecosystem services: an overview,” in Urban Expansion, Land Cover and Soil Ecosystem Services, ed C. Gardi (London: Routledge), 123–145. doi: 10.4324/9781315715674-6
Pavao-Zuckerman, M. A. (2008). The nature of urban soils and their role in ecological restoration in cities. Restor. Ecol. 16, 642–649. doi: 10.1111/j.1526-100X.2008.00486.x
Peck, D. C. (2009). Comparative impacts of white grub (Coleoptera: Scarabaeidae) control products on the abundance of non-target soil-active arthropods in turfgrass. Pedobiologia 52, 287–299. doi: 10.1016/j.pedobi.2008.10.003
Philippot, L., Raaijmakers, J. M., Lemanceau, P., and Van Der Putten, W. H. (2013). Going back to the roots: the microbial ecology of the rhizosphere. Nat. Rev. Microbiol. 11, 789–799. doi: 10.1038/nrmicro3109
Pouyat, R. V., Carreiro, M. M., Groffman, P. M., and Zuckerman, M. (2009). “Investigative approaches to urban biogeochemical cycles: New York metropolitan area and Baltimore as case studies,” in Ecology of Cities and Towns: A Comparative Approach, eds M. J. McDonnell, A. K. Hahs, and J. Breuste (Cambridge: Cambridge University Press), 329–354. doi: 10.1017/CBO9780511609763.021
Pouyat, R. V., Szlavecz, K., Yesilonis, I. D., Groffman, P. M., and Schwarz, K. (2010). Chemical, physical, and biological characteristics of urban soils. Urban Ecosyst. Ecol. 119–152.
Pouyat, R. V., Szlavecz, K., Yesilonis, I. D., Wong, C. P., Murawski, L., Marra, P., et al. (2015). Multi-scale assessment of metal contamination in residential soil and soil fauna: a case study in the Baltimore–Washington metropolitan region, USA. Landsc. Urban Plan. 142, 7–17. doi: 10.1016/j.landurbplan.2015.05.001
Qiu, J., and Turner, M. G. (2017). Effects of non-native Asian earthworm invasion on temperate forest and prairie soils in the Midwestern US. Biol. Invasions 19, 73–88. doi: 10.1007/s10530-016-1264-5
Rabatin, S. C., and Stinner, B. R. (1985). Arthropods as consumers of vesicular-arbuscular mycorrhizal fungi. Mycologia 77, 320–322. doi: 10.1080/00275514.1985.12025103
Ramirez, K. S., Leff, J. W., Barberán, A., Bates, S. T., Betley, J., Crowther, T. W., et al. (2014). Biogeographic patterns in below-ground diversity in New York City's Central Park are similar to those observed globally. Proc. R. Soc. B 281:20141988. doi: 10.1098/rspb.2014.1988
Rinkes, Z. L., Sinsabaugh, R. L., Moorhead, D. L., Grandy, A. S., and Weintraub, M. N. (2013). Field and lab conditions alter microbial enzyme and biomass dynamics driving decomposition of the same leaf litter. Front. Microbiol. 4:260. doi: 10.3389/fmicb.2013.00260
Rochefort, S., Therrien, F., Shetlar, D. J., and Brodeur, J. (2006). Species diversity and seasonal abundance of Collembola in turfgrass ecosystems of North America. Pedobiologia 50, 61–68. doi: 10.1016/j.pedobi.2005.10.007
Rousk, J., Bååth, E., Brookes, P. C., Lauber, C. L., Lozupone, C., Caporaso, J. G., et al. (2010). Soil bacterial and fungal communities across a pH gradient in an arable soil. ISME J. 4, 1340–1351. doi: 10.1038/ismej.2010.58
Rumble, H., and Gange, A. C. (2013). Soil microarthropod community dynamics in extensive green roofs. Ecol. Eng. 57, 197–204. doi: 10.1016/j.ecoleng.2013.04.012
Santorufo, L., Van Gestel, C. A. M., Rocco, A., and Maisto, G. (2012). Soil invertebrates as bioindicators of urban soil quality. Environ. Pollut. 161, 57–63. doi: 10.1016/j.envpol.2011.09.042
Scheu, S., and Wolters, V. (1991). Influence of fragmentation and bioturbation on the decomposition of 14C-labelled beech leaf litter. Soil Biol. Biochem. 23, 1029–1034. doi: 10.1016/0038-0717(91)90039-M
Schmidt, D. J. E., Pouyat, R., Szlavecz, K., Setäl,ä, H., Kotze, D. J., Yesilonis, I., et al. (2017). Urbanization erodes ectomycorrhizal fungal diversity and may cause microbial communities to converge. Nat. Ecol. Evol. 1:123. doi: 10.1038/s41559-017-0123
Schrader, S., and Böning, M. (2006). Soil formation on green roofs and its contribution to urban biodiversity with emphasis on Collembolans. Pedobiologia 50, 347–356. doi: 10.1016/j.pedobi.2006.06.003
Serna-Chavez, H. M., Fierer, N., and Van Bodegom, P. M. (2013). Global drivers and patterns of microbial abundance in soil. Glob. Ecol. Biogeogr. 22, 1162–1172. doi: 10.1111/geb.12070
Setälä, H., Bardgett, R. D., Birkhofer, K., Brady, M., Byrne, L., De Ruiter, P. C., et al. (2014). Urban and agricultural soils: conflicts and trade-offs in the optimization of ecosystem services. Urban Ecosyst. 17, 239–253. doi: 10.1007/s11252-013-0311-6
Smetak, K. M., Johnson-Maynard, J. L., and Lloyd, J. E. (2007). Earthworm population density and diversity in different-aged urban systems. Appl. Soil Ecol. 37, 161–168. doi: 10.1016/j.apsoil.2007.06.004
Smith, J., Chapman, A., and Eggleton, P. (2006). Baseline biodiversity surveys of the soil macrofauna of London's green spaces. Urban Ecosyst. 9:337. doi: 10.1007/s11252-006-0001-8
Sperling, C. D., and Lortie, C. J. (2010). The importance of urban backgardens on plant and invertebrate recruitment: a field microcosm experiment. Urban Ecosyst. 13, 223–235. doi: 10.1007/s11252-009-0114-y
Suzuki, Y., Grayston, S. J., and Prescott, C. E. (2013). Effects of leaf litter consumption by millipedes (Harpaphe haydeniana) on subsequent decomposition depends on litter type. Soil Biol. Biochem. 57, 116–123. doi: 10.1016/j.soilbio.2012.07.020
Szlavecz, K., Yesilonis, I., and Pouyat, R. (2018). “Soil as a foundation to urban biodiversity,” in Urban Biodiversity, eds A. Ossola and J. Niemela (Abingdon: Routledge in Association with GSE Research), 18–35. doi: 10.9774/GLEAF.9781315402581_3
Thompson, K., Green, A., and Jewels, A. M. (1994). Seeds in soil and worm casts from a neutral grassland. Funct. Ecol. 8, 29–35. doi: 10.2307/2390108
Tratalos, J., Fuller, R. A., Warren, P. H., Davies, R. G., and Gaston, K. J. (2007). Urban form, biodiversity potential and ecosystem services. Landsc. Urban Plan. 83, 308–317. doi: 10.1016/j.landurbplan.2007.05.003
Tresch, S., Frey, D., Le Bayon, R.-C., Zanetta, A., Rasche, F., Fliessbach, A., et al. (2019). Litter decomposition driven by soil fauna, plant diversity and soil management in urban gardens. Sci. Total Environ. 658, 1614–1629. doi: 10.1016/j.scitotenv.2018.12.235
Uno, S., Cotton, J., and Philpott, S. M. (2010). Diversity, abundance, and species composition of ants in urban green spaces. Urban Ecosyst. 13, 425–441. doi: 10.1007/s11252-010-0136-5
Van Der Heijden, M. G. A., Bardgett, R. D., and Van Straalen, N. M. (2008). The unseen majority: soil microbes as drivers of plant diversity and productivity in terrestrial ecosystems. Ecol. Lett. 11, 296–310. doi: 10.1111/j.1461-0248.2007.01139.x
Van Der Heijden, M. G. A., Klironomos, J. N., Ursic, M., Moutoglis, P., Streitwolf-Engel, R., Boller, T., et al. (1998). Mycorrhizal fungal diversity determines plant biodiversity, ecosystem variability and productivity. Nature 396, 69–72. doi: 10.1038/23932
Vauramo, S., and Setälä, H. (2010). Urban belowground food-web responses to plant community manipulation–impacts on nutrient dynamics. Landsc. Urban Plan. 97, 1–10. doi: 10.1016/j.landurbplan.2010.04.004
Vauramo, S., and Setälä, H. (2011). Decomposition of labile and recalcitrant litter types under different plant communities in urban soils. Urban Ecosyst. 14, 59–70. doi: 10.1007/s11252-010-0140-9
Vergnes, A., Blouin, M., Muratet, A., Lerch, T. Z., Mendez-Millan, M., Rouelle-Castrec, M., et al. (2017). Initial conditions during Technosol implementation shape earthworms and ants diversity. Landsc. Urban Plan. 159, 32–41. doi: 10.1016/j.landurbplan.2016.10.002
Vergnes, A., Le Viol, I., and Clergeau, P. (2012). Green corridors in urban landscapes affect the arthropod communities of domestic gardens. Biol. Conserv. 145, 171–178. doi: 10.1016/j.biocon.2011.11.002
Wagg, C., Bender, S. F., Widmer, F., and van der Heijden, M. G. A. (2014). Soil biodiversity and soil community composition determine ecosystem multifunctionality. Proc. Natl. Acad. Sci. U.S.A. 111, 5266–5270. doi: 10.1073/pnas.1320054111
Wardle, D. A. (2002). Communities and Ecosystems: Linking the Aboveground and Belowground Components. Princeton, NJ: Princeton University Press.
Wieder, W. R., Grandy, A. S., Kallenbach, C. M., and Bonan, G. B. (2014). Integrating microbial physiology and physio-chemical principles in soils with the MIcrobial-MIneral Carbon Stabilization (MIMICS) model. Biogeosciences 11, 3899–3917. doi: 10.5194/bg-11-3899-2014
Keywords: urban, soil, invertebrates, microbial communities, soil microbiome
Citation: Bray N and Wickings K (2019) The Roles of Invertebrates in the Urban Soil Microbiome. Front. Ecol. Evol. 7:359. doi: 10.3389/fevo.2019.00359
Received: 05 February 2019; Accepted: 09 September 2019;
Published: 25 September 2019.
Edited by:
Peter Groffman, The Graduate Center, City University of New York, United StatesReviewed by:
Loren B. Byrne, Roger Williams University, United StatesKatalin Szlavecz, Johns Hopkins University, United States
Copyright © 2019 Bray and Wickings. This is an open-access article distributed under the terms of the Creative Commons Attribution License (CC BY). The use, distribution or reproduction in other forums is permitted, provided the original author(s) and the copyright owner(s) are credited and that the original publication in this journal is cited, in accordance with accepted academic practice. No use, distribution or reproduction is permitted which does not comply with these terms.
*Correspondence: Kyle Wickings, a2d3MzcmI3gwMDA0MDtjb3JuZWxsLmVkdQ==