- 1Department of Evolutionary Ecology, Museo Nacional de Ciencias Naturales, CSIC, Madrid, Spain
- 2Department of Conservation Biology, Estación Biológica de Doñana, CSIC, Seville, Spain
- 3Foundation for the Research and Conservation of Parrots in Bolivia, CLB, Santa Cruz de la Sierra, Bolivia
The dispersal of many large-seeded plants is thought to have been handicapped by the extinction of megafauna in the late Pleistocene, and due to the ongoing defaunation of the largest of the extant dispersers. Oversized fruits defined as “megafaunal” provide variable amounts of flesh even though many of them cannot be ingested entirely, nor their seeds defecated, by any extant vertebrate. This apparent mismatch lead to the hypothesis of anachronisms involving extinct megafauna as dispersal-mediated selective agents on fruit traits shaped through endozoochory. It has been suggested that free-ranging livestock partially supply the dispersal functions previously provided by those globally or regionally extinct species. However, there is little knowledge on the role of livestock as a surrogate for megafauna dispersal agents relative to living wild dispersers. Here, we focus on seed dispersal of six palm species (Attalea eichleri, Attalea barreirensis, Attalea speciosa, Attalea princeps, Mauritia flexuosa, Acrocomia totai) with large fruits that conform to the so-called “megafaunal syndrome”. Data on seed dispersal were obtained by observations and camera trapping in the Cerrado, Pantanal and Amazonia biomes in Bolivia and Brazil. Rich communities of wild seed dispersers differing among palm species and study areas were recorded, including rodents, monkeys, canids, and a wide variety of birds, especially parrots. Long-distance primary dispersal was mainly conducted by parrots, while multiple species acted as short- and medium-distance secondary dispersers. Among livestock, dispersal was limited to seeds of A. totai and A. princeps moved by several species through stomatochory and endozoochory (mainly regurgitation). These results show that the large seeds can be efficiently dispersed externally by a wide array of present-day vertebrates of variable size but much smaller than extinct megafauna and livestock. A knowledge gap of the natural history of these and other plants with oversized fruits assumed to be maladapted for contemporary dispersal may have been partially favored by neglecting some key disperser guilds (e.g., parrots) and dispersal mechanisms (e.g., ectozoochory). The evaluation of historic and ongoing defaunation of key external dispersers is advocated to understand the influence of actual (rather than putative) dispersers on contemporary frugivore-plant mutualistic interactions.
Introduction
Trade-offs between fruit size and seed dispersal ability have been repeatedly highlighted as governing plant-frugivore mutualistic interactions (Wheelwright, 1985; Lord, 2004, Bruun and Poschlod, 2006; Burns, 2013). A number of extant plants show fruit size, phenological patterns and other traits that have been argued to better reflect adaptations to past than present-day ecological conditions (Barlow, 2000). These “anachronisms” are generally suggested to explain traits that are unexpected or not understood based on their interactions with extant assemblages of fruit-eating vertebrates (Janzen and Martin, 1982; Barlow, 2000). In particular, the megafaunal seed dispersal hypothesis states that some extant plants show “overbuilt” fruits apparently adapted for seed dispersal by very large mammals such as elephant-like gomphotheres and giant ground sloths that went extinct during the Pleistocene in the Neotropics (Janzen and Martin, 1982). These “anachronistic” fruits were assumed to be ecologically ineffective today because of the lack of present-day seed dispersal mechanisms (Janzen and Martin, 1982). This argument implies that the extinction of megafrugivores resulted in marked shifts in the patterns of seed dispersal observed today in extant plants with oversized fruits (Janzen and Martin, 1982; Howe, 1985; Barlow, 2000), with important implications in the ecology, evolution, and conservation of biodiversity. However, this hypothesis has remained controversial given its vagueness, the discrepancies in its assumptions and the limited or contrary evidence for many of its predictions (Howe, 1985; Hunter, 1989).
A comparative study of Neotropical large-fruited plants revisited and refined the megafaunal seed dispersal hypothesis, introducing an operational definition and classification of megafaunal fruits, like oversized fruits with fleshy pulp packaging extremely large individual seeds or large numbers of moderately-sized seeds (Guimarães et al., 2008). In using these criteria, subsequent studies have adopted the conjecture that extinct megafauna acted as legitimate past dispersers of many oversized fruits (Onstein et al., 2018; van Zonneveld et al., 2018), while others critically tested its assumptions given knowledge on the effectiveness of smaller contemporary dispersal agents using variable dispersal mechanisms (Jansen et al., 2012; Boone et al., 2015; Baños-Villalba et al., 2017; Rebein et al., 2017; Carpenter et al., 2018; McConkey et al., 2018). Multiple variably-sized extant dispersers and abiotic factors have been recognized in present-day dispersal interactions with “megafaunal plants,” while assuming the existence of body-size trade-offs constraining dispersal ability via fruit ingestion and seed defecation (Donatti et al., 2007; Guimarães et al., 2008; Pires et al., 2018). Thus, the main challenge facing the megafaunal hypothesis remains the establishment of the disperser size that represents a functionally pervasive trait for each plant species (Howe, 1985, 2016; Jansen et al., 2012; Carpenter et al., 2018; McConkey et al., 2018), without succumbing to the circular rationale that assumes that large-sized vertebrates are major seed dispersers of large-fruited plants (Burns, 2013; Chen and Moles, 2015; Sebastián-González, 2017). Therefore, since Howe's (1985) critical review, the testing of the megafaunal seed dispersal hypothesis continues to be challenged by a general lack of knowledge on dispersers and dispersal strategies of many large-fruited plants (Fleming and Kress, 2013; Jordano, 2014).
Different fruit-exploiting and seed-dispersal mechanisms have important implications for seed viability, dispersal distance and recruitment (Howe and Smallwood, 1982). However, the definition of megafaunal fruit restrictively uses criteria matching African elephant-dispersed fruits, implying endozoochory as a dispersal mechanism (Guimarães et al., 2008; Bunney et al., 2017). Owing to their relatively large size, it has been suggested that domestic livestock serve as contemporary substitutes of extinct megafauna (Janzen, 1982; Janzen and Martin, 1982; Guimarães et al., 2008). The intensive contemporary harvesting of oversized fruits by large mammals, such as tapir and livestock acting as endozoochorous dispersers, has been highlighted to support this rationale (Janzen and Martin, 1982; Guimarães et al., 2008). This conflicts with evidence indicating that seeds of many of these fruits may not require gut passage to be efficiently dispersed by multiple legitimate external dispersers (Dominy and Duncan, 2005; Jansen et al., 2012; Blanco et al., 2018). Indeed, external dispersal may be the major mechanism exploited by large-fruited plants when a variable quantity of flesh is provided to dispersers (Dominy and Duncan, 2005; Baños-Villalba et al., 2017), and even when seed predators are the main dispersers (Jansen et al., 2012; Tella et al., 2016a,b, 2019). In this context, assessing the role of livestock as seed dispersers of oversized fruits was proposed as a feasible approach to test the megafaunal hypothesis (Janzen, 1982; Janzen and Martin, 1982; Howe, 1985). To date, however, there is still little information on seed dispersal by particular livestock species like cows, horses, sheep, goats, and pigs, on their external or internal seed dispersal mechanisms, as well as on their impact on seed germination and recruitment of particular large-fruited plants (Janzen, 1982; Boone et al., 2015; Baños-Villalba et al., 2017; Delibes et al., 2019). The ways in which different livestock species exploit large fruits and disperse their seeds can be especially informative for understanding their current role in plant population dynamics, and to assess the past role of extinct megafauna in the evolutionary ecology of their presumed food plants. This includes the determination of whether seeds are defecated (endozoochory), cleaned by stripping the pulp from the fruit in the mouth and then ejected (stomatochory), regurgitated after a variable period in the upper digestive system (endozoochory) or dispersed by a combination of these methods. This remains generally unknown for most livestock-large fruit interactions (Delibes et al., 2019).
Among large-fruited plants providing abundant food for multiple organisms, including human populations, palms (Arecaceae) have been highlighted as a keystone group of generally poor conservation status (Henderson, 2002; Smith, 2015). Information on the seed dispersal of large-fruited palms is limited (Zona and Henderson, 1989; Eiserhardt et al., 2011; Muñoz et al., 2019). In fact, previous studies attributed to extinct megafauna the dispersal of palm species because of the lack of essential natural history observations on the identity of current seed dispersers. Moreover, one of the main conclusions of a synthesis of palm-frugivore interactions published very recently is that “massive knowledge gaps of interaction diversity remain in the tropics” (Muñoz et al., 2019), which may be partially due to the recent regional and global declines and extinctions of many of their main primary dispersers (de Andrade et al., 2015; Lanes et al., 2016; Baños-Villalba et al., 2017).
Here, we focus on seed dispersal of six palm species (Attalea barreirensis, Attalea eichleri, Attalea speciosa, Attalea princeps, Mauritia flexuosa, Acrocomia totai) with large fruits that conform to the so-called “megafaunal syndrome” (Guimarães et al., 2008), and thus putatively anachronistic. We assessed whether contemporary wild vertebrates exert the seed dispersal function attributed to extinct megafauna in the past, and whether the assumption of endozoochory of large seeds by extinct megaherbivores can be supported by their presumed domestic substitutes, as a model to investigate past and ongoing seed dispersal strategies of these large-fruited plants. We hypothesized that seeds of large-fruited palms are currently dispersed by rich communities of wild vertebrates rather than by livestock acting as megafauna substitutes. This predicts (a) that multiple variable-sized contemporary fruit and seed consumers smaller than extinct megafauna and livestock act as reliable primary and secondary dispersers at variable distances using different dispersal mechanisms, not only endozoochory. In addition, (b) the largest among the extant potential dispersers, especially tapir, should act as reliable dispersal agents of these plants. Finally, if we assume that livestock act as disperser surrogates of extinct megafauna, we predict (c) that large seeds of the study palms are reliably dispersed by livestock using similar mechanisms (endoozochory implying defecation) as those presumably used by extinct megaherbivores.
Materials and Methods
Study Areas and Palm Species
Fieldwork was conducted in six different study areas within the Cerrado tropical dry forest, Pantanal and Amazonia biomes, including transitional habitats with Beni savannas and Chiquitano dry forest, according to the microhabitat requirements of the studied palm species (Lorenzi et al., 1996). Table 1 shows the details of the habitat and study periods in each locality in the departments of Beni and Santa Cruz in Bolivia, and the state of Piaui in Brazil (Figure 1). Sampling dates corresponded to the fruiting periods of the study palms. Given the long fructification periods of these palm species, which also greatly varied between years and regions, we attempted to cover several sampling periods in different areas to attain a more general view of the dispersers' communities.
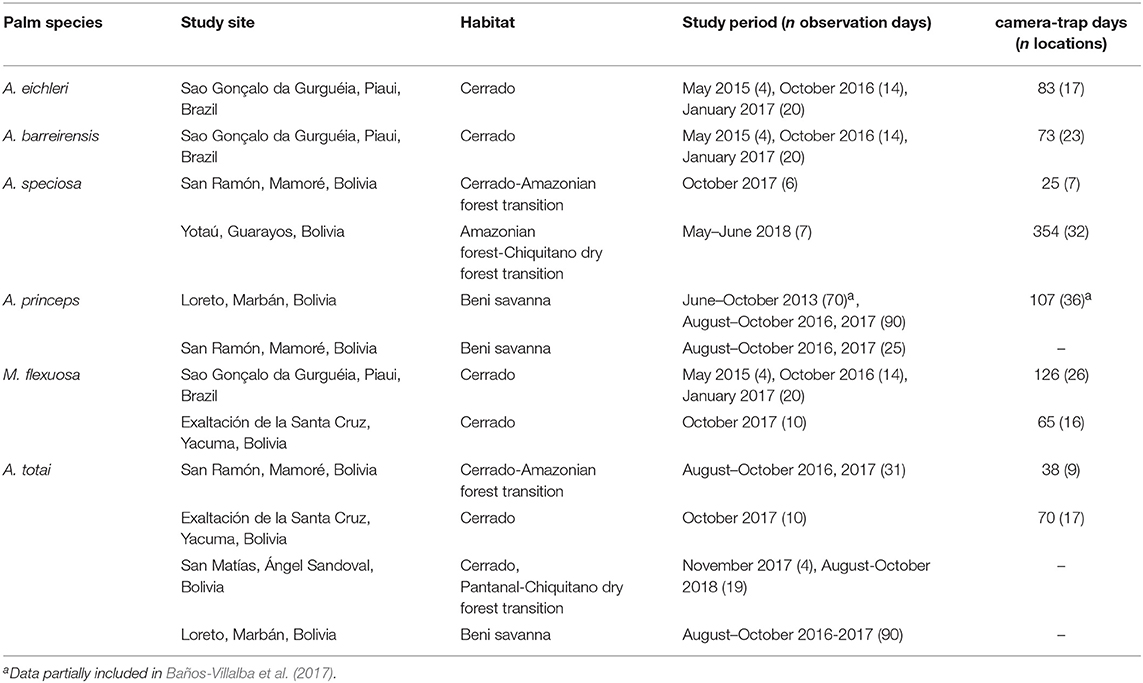
Table 1. Location, habitat, study periods, and sampling effort (number of days of observation, number of camera-trap days, and number of camera locations) for each palm species in each study area.
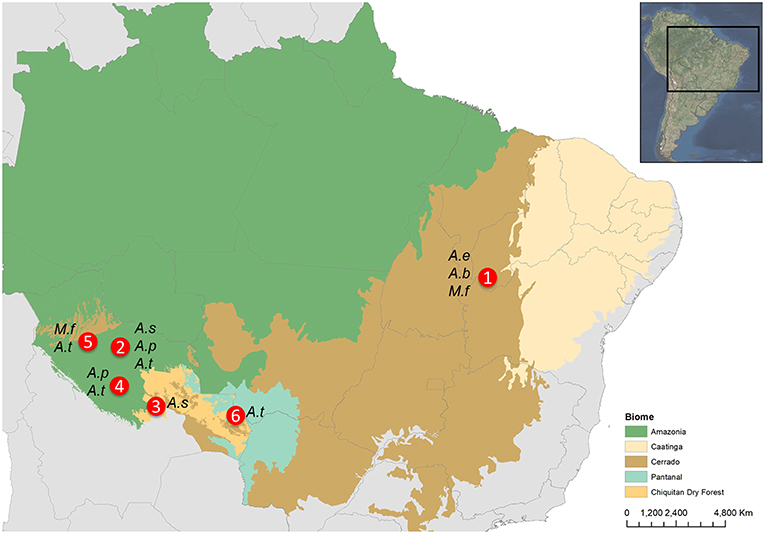
Figure 1. Map indicating the location of the study areas (1: Sao Gonçalo da Gurguéia, Piaui, Brazil; 2: San Ramón, Mamoré, Bolivia; 3: Yotaú, Guarayos, Bolivia; 4: Loreto, Marbán, Bolivia; 5: Exaltación de la Santa Cruz, Yacuma, Bolivia; 6: San Matías, Ángel Sandoval, Bolivia) for each palm species: A.e, Attalea eichleri; A.b, Attalea barreirensis; A.s, Attalea speciosa; A.p, Attalea princeps; M.f, Mauritia flexuosa; A.t, Acrocomia totai.
The study areas within the Cerrado biome and their transitional habitats with Amazonian forest and Chiquitano dry forest are characterized by a heterogeneous savanna landscape including grasslands, shrublands, gallery forests, and dry and humid forests, with palm patches of variable size in locations with suitable soil microclimate (Oliveira and Marquis, 2002; de Carvalho and Mustin, 2017). The habitat in these areas shows a variable degree of fragmentation and defaunation (especially hunting pressure for bush meat and parrot poaching for the pet trade) depending on its inclusion within protected areas, human population density, nearness to inhabited nuclei, and their influences on the frequency of fires and livestock density (Silva et al., 2006; de Carvalho and Mustin, 2017; Mistry et al., 2019; see Table S1 for details). The study area within the Beni savannas are characterized by wide areas of seasonal flooded grasslands dotted with forest islands dominated by palms and semi-deciduous groves used historically by indigenous human communities (Langstroth and Riding, 2011; Hordijk et al., 2019). The study area in San Matías, Ángel Sandoval (Bolivia) correspond to a transition between the Bolivian Pantanal, with roughly 80% of its floodplains submerged during the rainy season, and the Chiquitano dry forest characterized by shrublands, gallery forests, and dry forests (Power et al., 2016; de Carvalho and Mustin, 2017). Extensive ranching in large properties (generally >1,000 hectares) is the main livestock operation in these areas. Free-range cattle and equids move through the territory with temporal periods of grouping in corrals, while much less abundant herds of sheep and goats are locked in corrals every night to avoid predation. Pigs are restricted to the surroundings of small communities and isolated houses. The sampling areas where observations and camera trapping were conducted in each study site covered between 130 hectares (Yotaú, Guarayos, Bolivia) and about 15,000 hectares (San Matías, Ángel Sandoval, Bolivia; Table S1).
Table 2 shows the characteristics of the studied palm species, their fruits and seeds, which were obtained from the literature (Lorenzi et al., 1996) and our own measurements. The fruits of these palm species are categorized as “megafaunal fruits” Type I, defined as fleshy fruits 4–10 cm in diameter with up to 5 large seeds (generally 2.0 cm diameter; Guimarães et al., 2008) Although the mean size of fruit and seed of A. totai are smaller, the ranges can reach the values considered to be “megafaunal fruits” (Table 2).
Direct Observations on Wild Dispersers and Dispersal Mechanisms
In each study area, we actively searched for frugivorous and omnivorous wild vertebrates feeding on palm fruits. Table 1 shows the sampling effort, in days of observation, conducted by a team of 2–4 persons for each palm species in each study area. To increase the likelihood of encountering frugivorous dispersers and observing dispersal events, surveys were conducted between sunrise and midday and late in the afternoon by slowly driving along unpaved and secondary roads crossing or parallel to palm forests, or by walking in and around palm forests. Once frugivorous vertebrates were located foraging on the palms, observations were conducted with a telescope and binoculars to record foraging behavior while avoiding disturbance. Specifically, we focused on determining whether they moved the seed away from the parent or dropped it beneath the parent canopy, or secondarily after the seeds had been disseminated by primary dispersers or by abiotic factors coupled with physical fruit features (e.g., sphericity favoring rolling). We recorded whether the dispersal event occurred internally by swallowing seeds (endozoochory) or externally (ectozoochory) by transporting them in the beak or mouth (stomatochory or synzoochory), or by hand by monkeys, even when fruits were dropped beneath the palms thus promoting secondary dispersal by other organisms. When the foraging bout ended, remains of dispersed fruits and entire fruits dropped under the perching trees were inspected to assess which fruit parts were consumed and the form in which the consumer species consumed the fruits, including whether the pulp and seed were ingested and whether the discarded seeds were damaged by mastication or pecking. Random night surveys were conducted sporadically right after dawn along unpaved roads using flashlights to attempt to localize ground- and arboreal-dwelling frugivores feeding on fruits over or below fruiting palms and record seed dispersal events.
Camera Trapping
We used camera trapping to detect elusive, scarce or nocturnal potential dispersers like ground-dwelling mammals mainly acting as secondary dispersers of seeds from fruits passively falling from the palms or dispersed primarily by other frugivores. The cameras (model Bushnell 6 MP Trophy Cam Essential) were placed hidden at ground level close (3–5 m) to fleshy-fruited palms with presence of a variable number of fallen mature palm fruits (10–30) to increase the likelihood of detecting secondary dispersers (Baños-Villalba et al., 2017). The cameras were directed toward the fruits, and ran automatically for 5 consecutive days. Cameras were programmed to detect fruit consumers and dispersers with multiple instantaneous digital captures and short video recordings (video length = 10 s, interval between videos = 60 min) aimed to determine the manner in which fruits were used and moved; this time interval between videos or “trigger delay” was programed to prevent the card from filling up with too many redundant images of a single animal or group, also thus preventing the batteries from running out. Table 1 shows the sampling effort, as the number of camera-trap days (during daylight and night) and the number of camera locations, for each palm species in each study area.
Disperser Identification by Beak Marks and Feces
As part of the surveys, dispersed seeds found under perches (trees, cliffs, and fence poles) and foraging sites were attributed to disperser species based on distinctive beak marks found on fruits and seeds (see Baños-Villalba et al., 2017; Montesinos-Navarro et al., 2017 for parrots). Direct observations of fruit consumption allowed us to ascertain the types of marks produced by each species by examining fruit and seed remains and entire pecked and wasted fruits collected below fruiting palms after the foraging bouts. We searched for seeds dispersed by rodents and carnivorous mammals within and around palm patches. The identification of these disperser species were based on tooth marks on the pulp of partially consumed fruit and on seeds (see examples in Figure S1), and on the presence of other distinctive signs like traces, footprints, droppings and warrens (Eisenberg and Redford, 2000). This was enhanced in many cases by confirming presence, fruit consumption, and seed dispersal with camera traps, allowing us to subsequently search for dispersed fruits and seeds with typical tooth and beak marks of each species in the camera surroundings.
During the random surveys conducted in palm forests and their surroundings, we searched for feces of large-bodied vertebrates that could act as potential dispersers of palm seeds, especially carnivorous mammals, peccaries and Greater rhea (Rhea americana). These feces were examined in situ by breaking them up by hand to determine if they contained palm seeds. We paid particular attention to the qualitative role of South American tapir (Tapirus terrestris) as a reliable disperser of palm seeds. Given its elusive habits and scarcity in the study areas, we conducted specific and intensive searching with the help of local people in Yacuma Province, Beni Department, Bolivia to locate the latrines where this species generally defecate (Fragoso and Huffman, 2000; O'Farrill et al., 2013). This area was specifically selected to assess disperser assemblages of M. flexuosa and A. totai (Table 1), although small and very localized patches of A. princeps also occur there. Once latrines were located, we proceeded to determine the presence of seeds by opening each scat individually and searching for the presence of whole and partially eaten seeds, as well as other remains from palm fruits, specifically the presence of epicarp fragments (scales) of M. flexuosa. We recorded the presence of germinating palm seeds or saplings within and around latrines. The presence of seeds of other large fruited plants not systematically identified or quantified was also recorded in the latrines.
Dispersal Distances
Seed dispersal events that were directly observed allowed us to measure exact distances, from the location where the fruit was collected to where the fruit or its seeds were released, by using binoculars with a laser rangefinder (Leica Geovid 10 ×42) working in a range of 10–1,300 m. When the dispersers were lost from sight in the vegetation, we considered a minimum dispersal distance from the location where the fruit was collected to the location where the animal was no longer visible. For flying dispersers carrying fruits in the beak when they were first observed, we recorded the distance at which they were first detected by using reference points, and then followed them with binoculars. In these cases, we estimated minimum dispersal distances, as measured from the location of first sighting to where the disperser perched for handling the fruit, where they released the fruit in flight, or where they were lost from sight in flight or within the forest. When we observed frugivorous species feeding on palm fruits without knowledge of the location of the mother palm, we conservatively estimated the minimum dispersal distance as the distance to the closest fruiting palm. In addition, for dispersed seeds found under perches and foraging sites, and for those found in feces and warrens attributed to each disperser species, the minimum dispersal distance to the closest fruiting palm was considered. To avoid pseudoreplication, minimum dispersal distances were not estimated for seeds found in tapir latrines because they were not found at random but rather by focused intensive searching.
Seed Dispersal by Livestock
Direct observations were conducted on livestock foraging on palm fruits to determine the form of fruit exploitation and seed dispersal of each palm species in each study area. This was complemented with video-recordings during daylight and at night by camera traps placed at ground level under fleshy-fruited trees with mature palm fruits fallen passively or discarded by primary dispersers (Table 1). A random sample of feces of free-ranging livestock found in the palm forests and their surroundings was shredded in situ to determine the presence of seeds. The presence of seeds was also recorded at outdoor resting sites used during daylight (cows) or in corrals used at night (sheep, goat) depending on livestock operations in each study area. In addition to feces, we also recorded the presence of seeds in regurgitations, especially cud-chewing regurgitations produced during rumination (Delibes et al., 2019). Dispersal distances were determined as detailed above for wild animals when seeds were found at random, but not when they were searched for specifically at resting sites.
To further assess the patterns of palm seed dispersal by livestock, we conducted an intensive sampling in two monotypic stands of A. totai (3 and 9 hectares of extension) regularly used by extensive cows and horses in Yacuma Province, Beni Department, Bolivia. This area includes well-conserved Cerrado vegetation where this palm grows patchily due to a localized humid soil microclimate. Walking transects were conducted at random from the palm patch border to about 700 m in the Cerrado vegetation to record the location of livestock feces and regurgitations with palm seeds using a GPS device. A random sampling of the numbers of seeds regurgitated by cows was conducted in 1 m2 plots (n = 9) within A. totai patches. In addition, we recorded the location of excreta at greater distances (3–8 km) from the palm patch to confirm the presence of livestock and potential seed dispersal at longer distances. For each excreta, we recorded the livestock species (cattle or horse), and the presence and number of palm seeds. The minimum distance from each excreta to the border of the palm grove was calculated with ArcGIS 10.5 (ESRI; Redlands, California, USA).
Data Analysis
Factors affecting dispersal distance by wild animals were analyzed by Generalized Linear Models (GLM) using a negative binomial distribution and a log-link function. Palm species, disperser species nested within dispersal mode (primary or secondary) and the precision of the distance measurement (minimum or exact) were considered as predictor variables (fixed factors).
The patterns of A. totai seed dispersal by livestock were assessed with a GLM (binomial error, logit function) where the presence or lack of seeds in excreta was the response variable. The type of excreta (fecal or regurgitated), livestock species (cow or horse), and the identity (a or b) of each palm grove sampled were considered as explanatory factors, while the distance from each excreta to the border of the palm patch was included as a covariate.
Statistical analyses and checking of model assumptions were performed using SPSS software v. 25 (IBM SPSS Statistics).
Results
Disperser Assemblages and Dispersal Mechanisms
A total of 54 vertebrate species were confirmed as seed dispersers of the study palms (Table S2). Wild dispersers ranged from very small species like the bat Artibeus sp. (about 65 g) and a small parakeet (Brotogeris chriri, 72 g) to larger species like the tapir and livestock of variable sizes, but generally larger than wild dispersers (Table S2). The number of dispersers varied among palm species; parrots (Psittaciformes) and monkeys (Primates) were recorded as seed dispersers for all of the studied palm species, while other Orders varied in their impact as dispersers of each palm species (Figure 2, Table S2). Parrots (13 species) dominated among the primary dispersers for all palm species, ranging from one species (A. hyacinthinus for A. eichleri and A. barreirensis) to 12 species for A. totai (Figures 2, 3, Table S2). Several tree- and ground-dwelling primates and rodents acted as primary and secondary dispersers of A. princeps.
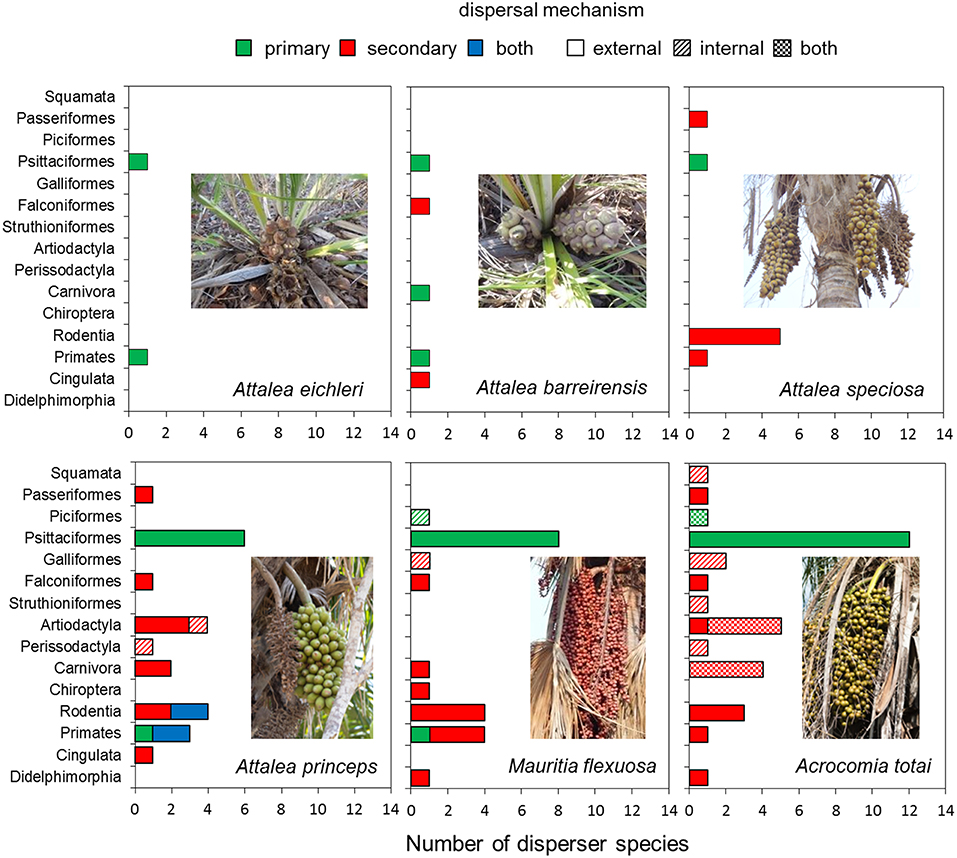
Figure 2. Number of seed disperser species of each vertebrate Order for each palm species according to dispersal mechanism.
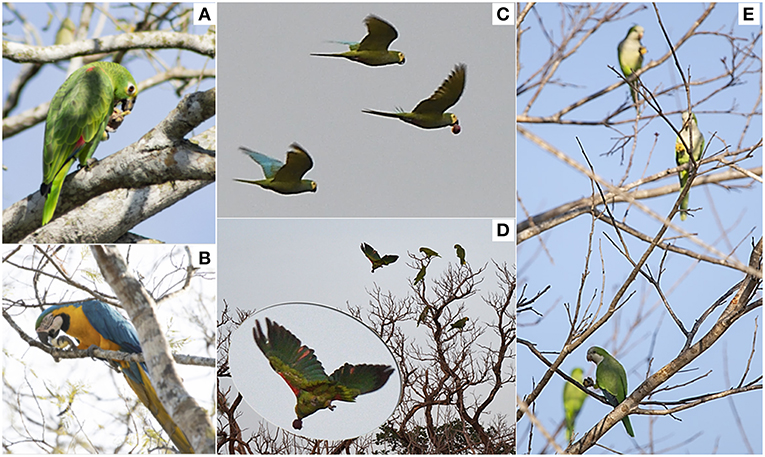
Figure 3. Photographs illustrating examples of primary external dispersal of palm seeds by parrots: (A) Amazona ochrocephala and (B) Ara ararauna feeding on the pulp of Attalea princeps fruits moved from mother palms to perching trees; (C) Orthopsittaca manilatus flying with a Mauritia flexuosa fruit; (D) Amazona aestiva departing from a perching tree with a Mauritia flexuosa fruit (a two-phase dispersal); (E) three Myiopsitta monachus individuals feeding on the pulp of Acrocomia totai fruits moved from mother palms to a perching tree. Photographs taken by J. Salguero (A,B,E) and M. de la Riva (C,D).
Most disperser species disseminated palm seeds externally by transporting them in the beak and mouth (stomatochory). Only external dispersers were recorded for A. eichleri, A. barreirensis, and A. speciosa (Figures 2, 4, Table S2). Among wild dispersers, only tapirs acted as internal dispersers of A. princeps, while two bird species (Mitu tuberosum and Ramphastos toco) were observed swallowing entire fruits of M. flexuosa. The seeds of the smallest-fruited palm (A. totai) were dispersed externally (20 disperser species), internally (5 dispersers), and by both methods (9 dispersers, including wild and domestic species). Most dispersers (40 of 54 species, 74%) acted exclusively as external dispersers of 1–4 palm species each, while 5 of 54 (9%) were exclusively internal dispersers of 1–2 palm species each; the remaining species (9 of 54, 17%) acted both as primary and secondary dispersers of A. totai (Figure 2, Table S2). Overall, 49 of 54 species (91%) acted as external dispersers of 1–4 palm species, while 14 of 54 species (26%) acted as internal dispersers of 1–2 palm species.
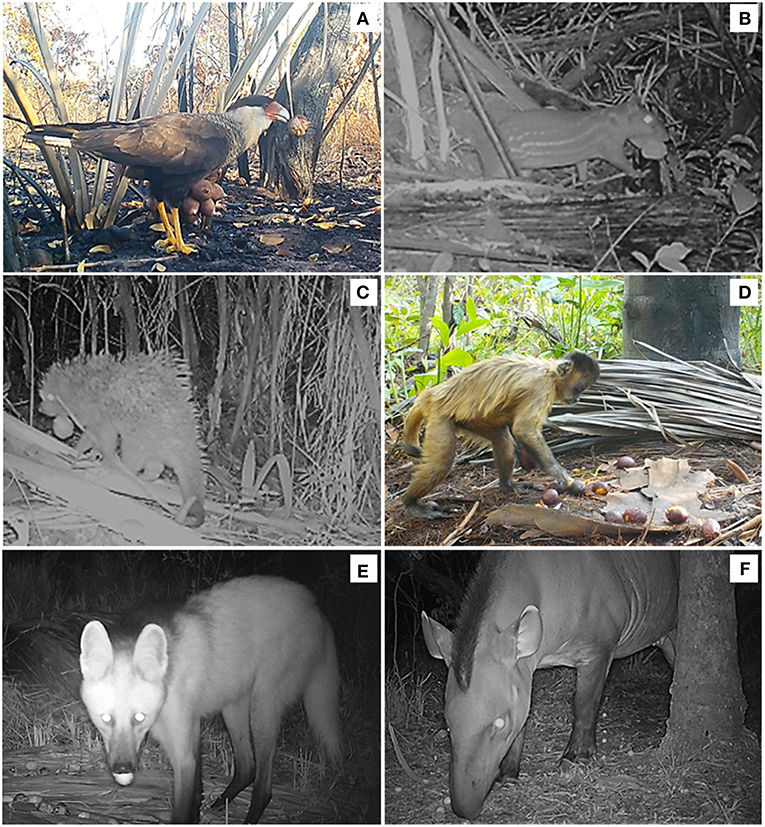
Figure 4. Photographs illustrating examples of secondary external dispersal of palm seeds: (A) Caracara plancus dispersing Attalea barreirensis, (B) Cuniculus paca and (C) Coendou prehensilis dispersing Attalea speciosa; (D) Sapajus libidinosus and (E) Chrysocyon brachyurus dispersing Mauritia flexuosa; (F) Tapirus terrestris consuming and potentially dispersing Acrocomia totai. Photographs taken by camera traps.
A proportion of dispersers moved seeds of multiple palm species (21 of 54, 38.9%). Therefore, we pooled all palm species to summarize the role of primary and secondary dispersal, or both, in relation to the mechanism (endozoochory, ectozoochory, or both) used by each disperser species (Figure 5). All primary dispersers moved palm seeds externally (100%, n = 16 species), most being parrots (13 of 16 species, 81%). Other dispersers acting exclusively as primary dispersers included the black howler (Alouatta caraya), an unidentified Artibeus bat and the toco toucan (R. toco) for M. flexuosa and A. totai (Table S2). The toucan was considered both an external and internal primary disperser of A. totai (Figure 5, Table S2), because this species can fly with fruit in the beak regardless of whether the fruit is later swallowed or lost. Most secondary dispersers move seeds only externally (especially rodents), or both externally and internally (especially canids and livestock), while exclusively endozoochorous species included the tapir, a variety of large birds and the single reptile recorded. Finally, versatile ground- and tree-dwelling primary and secondary dispersers such as squirrels and primates moved palm seeds only externally (Figure 5).
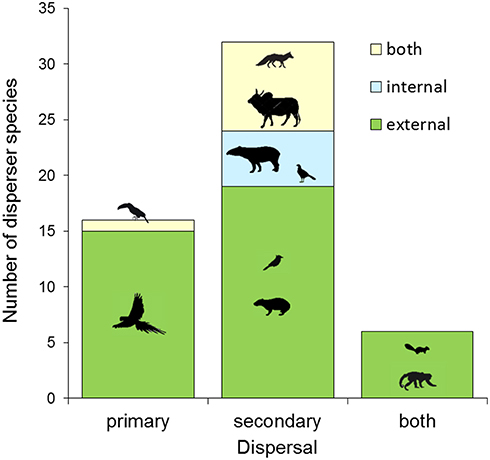
Figure 5. Summary of the number of seed disperser species (pooling all palm species) according to dispersal mode (primary, secondary, or both) and mechanisms (external, internal, or both).
Dispersal Distances
Overall, we recorded the distances of seed dispersal events (n = 2,504, Table S3) conducted by 21 wild disperser species for the six study palms (A. barreirensis, n = 385, A. eichleri, n = 253 A. speciosa, n = 719, A. princeps, n = 363, M. flexuosa, n = 400, A. totai n = 384). Seed dispersal distance was influenced by palm species (binomial negative GLM, χ2 = 434.4, df = 5, P < 0.0001, Figure 6A), and dispersal mode being higher when seeds were moved primarily (n = 1,609) than secondarily (n = 895) while controlling for disperser species (χ2 = 1579.1, df = 20, P < 0.0001, Figures 6A,B). The precision of the distance measurement (minimum, n = 1,591 or exact, n = 913) was not significant (χ2 = 0.08, df = 1, P = 0.77). The dispersal mechanism was not included as a predictor because internal dispersal distance was only recorded for A. totai seeds found in a single scat of an unidentified carnivorous mammal. Dispersal distances ranged from a few meters when seeds were moved by a variety of rodents acting as secondary dispersers, to several kilometers when primarily dispersed by macaws (Figure 6B).
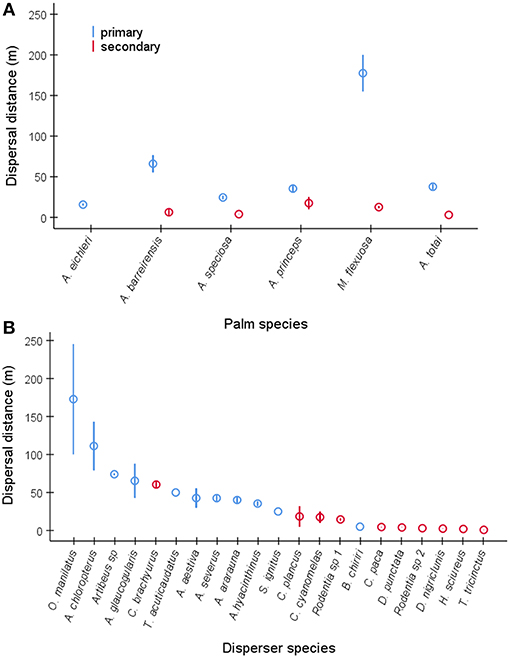
Figure 6. Mean ± SE dispersal distances (in m) for (A) primary and secondary dispersions of seeds of each palm species, pooling disperser species, and for (B) dispersions conducted by each primary and secondary disperser species, pooling palm species. The complete scientific name of each disperser species is shown in Table S2.
Dispersal by Tapir
Half of the tapir latrines (n = 20) contained seeds of A. totai, while about 26% of feces (n = 744) showed between 1 and 5 A. totai seeds. The number of seeds was not recorded in all feces but at least 493 A. totai seeds were counted, pooling all latrines. Sixty-five seeds of A. princeps were found in two old latrines where the number of feces could not be determined (Table 3). Multiple epicarpus scales of M. flexuosa were found in a large proportion of latrines, but no intact seeds were found after examining all individual feces and the compacted unquantified fecal material found in the bottom of latrines (Table 3).
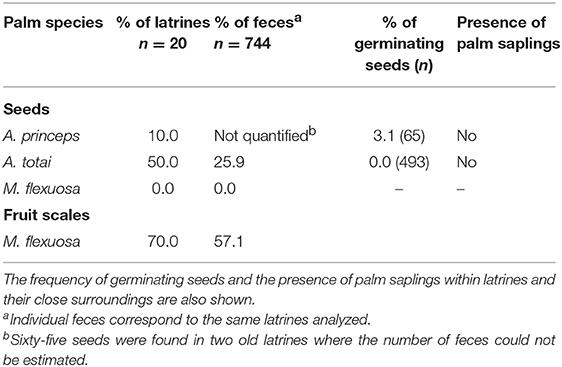
Table 3. Frequency of latrines and individual feces of South American tapir with palm seeds and epicarp scales of M. flexuosa.
One dead seedling and two germinating seeds of A. princeps were found in a single latrine. A sample of the remaining seeds found in the same latrine (n = 18) were opened to confirm their apparent viability (7 seeds, 38.9%) or unviability due to predation by invertebrate larvae (11 seeds, 61.1%). No A. totai seeds showed signs of germination, nor were any seedlings or saplings of old palm seeds found within the latrines or their surroundings (Table 3).
A variable number of seeds from other large-fruited plants were found in the latrines but not systematically identified or quantified. Among them, all seeds examined by opening and checking the endosperm were unviable (Cariocar brasiliensis, n = 10; Mauritella armata, n = 3; Artocarpus sp., n = 2) or were found germinating at high densities (Euterpe precatoria, estimated n > 1,500) or low densities (Pouteria sp., n = 2) in the latrines. We found no older saplings of these species in the latrines and their close surroundings.
Palm Exploitation by Livestock
Palm exploitation by each livestock species is shown in Table 4. Ruminants consumed saplings of all palm species, the leaves of mature bush layer palms and, when accessible, immature palms of the taller species. Cows also exploited flowers of the bush layer species. Most livestock species present in each study area consumed the fleshy pulp of the palm species with smaller fruits (A. princeps, M. flexuosa, A. totai), but none consumed the scarce pulp of the larger-fruited palms (Table 4). Pigs also consumed, or at least destructively masticated, the seeds of A. princeps and A. totai, and likely those of M. flexuosa although this was not confirmed in this study.
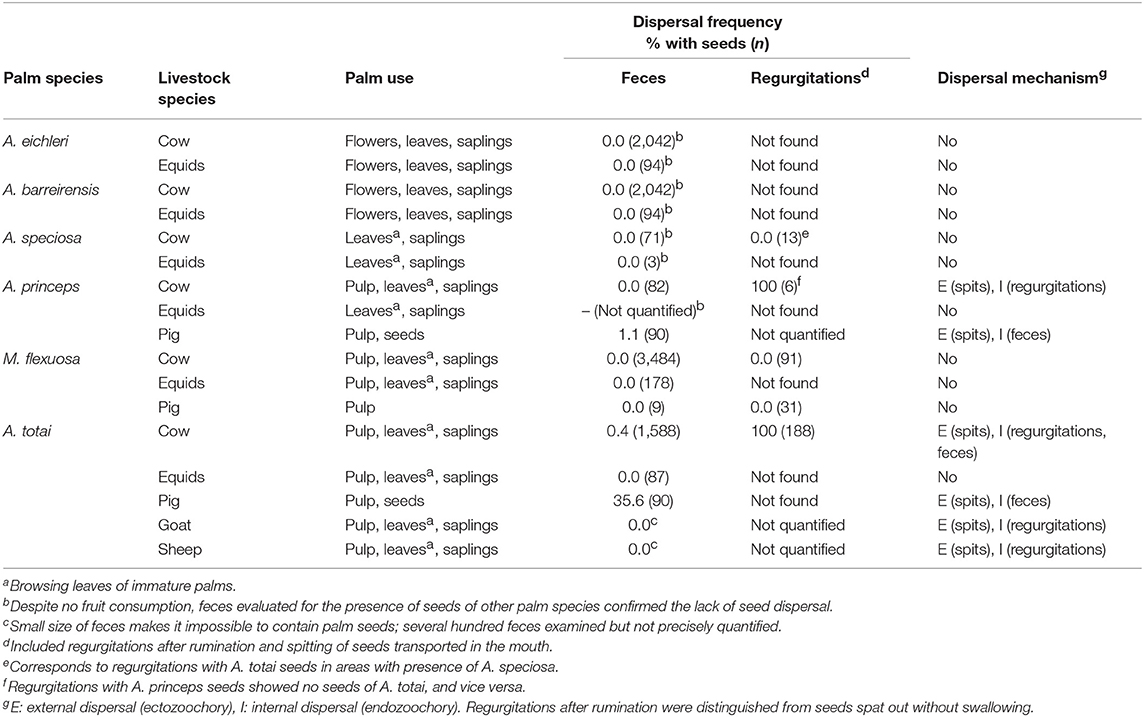
Table 4. Palm parts consumed, frequency of seeds dispersed in feces and regurgitations, and dispersal mechanism by each livestock species.
Seed Dispersal by Livestock
Livestock dispersal was restricted to the seeds of A. totai and A. princeps moved by endozoochory and ectozoochory. Specifically, cows, sheep and goats regurgitated large quantities of A. totai seeds after a period of rumination but never (sheep, goat) or rarely (cow) defecated seeds (Table 4). In addition, these livestock also exploited fleshy pulp without ingesting seeds, but transported and ejected them after a generally short period in the mouth. Pigs also chewed and sucked the pulp and spit out the seeds or ingested and defecated them. In other cases, livestock exploited the pulp and discarded the seeds of A. totai (equids) and M. flexuosa (cow, equids) without dispersing them. This was specifically confirmed by recording large numbers of M. flexuosa seeds stripped from the pulp (n = 1,507) or partially stripped (n = 187) after consumed by cows, compared with whole fruits (n = 211) below the canopy of particular fruiting palms (n = 16), but not in their surroundings.
Seed Dispersal Frequency by Livestock
Estimating the frequency of each of these dispersal mechanisms was challenging because of the logistic difficulty in determining each seed's fate (i.e., whether or not seeds were ingested, and subsequent spitting, regurgitation, or defecation). This was further complicated by a likely combination of these dispersal mechanisms occurring for seeds consumed in a single feeding bout. The presence of palm seeds in feces was limited to a low proportion of A. totai in cow and pig feces (Table 4). Estimating the frequency of seed dispersal in regurgitations was hindered because seed regurgitation after rumination was apparently promoted by the ingestion of large quantities of palm fruits. In fact, all cow regurgitations recorded contained seeds of A. totai or A. princeps (Table 4). The mean number of A. princeps seeds per cow regurgitation was 3.3 (SD: 1.8, range: 1–6, n = 6).
Seed Dispersal Sites and Distance by Livestock
Dispersal of A. totai seeds by cattle regurgitation mostly occurred below the source plant, especially along paths and at resting sites within the limits of the palm patches. The number of seeds counted in 1 m2 plots within A. totai patches showed a high density of seeds regurgitated by cows (mean ± SD = 170 ± 82 seeds per m2, range = 108–385, n = 9). Minimum dispersal distances were necessarily short in these cases because of the small size of the palm patches, but these distances were not measured to avoid introducing statistical bias related to the large number of seeds excreted as a consequence of artificially high cow densities. Pigs dispersed seeds of A. totai by spitting them out (all estimated minimum distances, mean ± SD distances = 27.8 ± 44.5 m, range = 4–185, n = 215) and defecation (17.7 ± 11.3 m, range = 6–35, n = 9). In several cases, we recorded sheep collecting A. totai fruits and spitting out seeds without ingestion at exact distances (45.0 ± 20.7 m, range = 20–60, n = 8). In addition, we observed thousands of A. totai seeds regurgitated during rumination in different sheep and goat corrals at minimum distances of 110 and 106 m, respectively, from the nearest palm patch.
Intensive Sampling of A. totai Seed Dispersal by Livestock
The intensive sampling of livestock excreta conducted around two A. totai forest patches showed that seed dispersal depends mainly on the type of cow excreta (binomial GLM, χ2 = 227.37, df = 1, P < 0.0001). In fact, all regurgitations recorded contained seeds (100%, n = 75), while only one of the sampled feces contained seeds (0.9%, n = 113). The mean number of seeds per regurgitation was 24.1 (SD: 15.9, range: 4–68, n = 30), while the single cow scat with seeds contained five seeds. The presence or absence of seeds in cow excreta did not differ between palm patches (χ2 = 0.96, df = 1, P = 0.33) nor did it depend on the distance from each excreta to the palm patch (χ2 = 1.83, df = 1, P = 0.18). However, when the type of excreta (regurgitation or scat) was the response variable, a clear effect of the distance from the palm patch border was found (binomial GLM χ2 = 15.18, df = 1, P < 0.0001; palm patch effect: χ2 = 1.07, df = 1, P = 0.30). The frequency of regurgitations (all with seeds) decreased as the distance from the palms increased (Figure 7; the mean ± SD minimum seed dispersal distance in regurgitations was 107 ± 114, range = 7–358, n = 75), despite the fact that cows were present at larger distances as confirmed by the presence of their feces (Figure 7). No seeds of A. totai were found in horse feces sampled within and around the two palm patches (n = 58), and no regurgitations of horses were recorded.
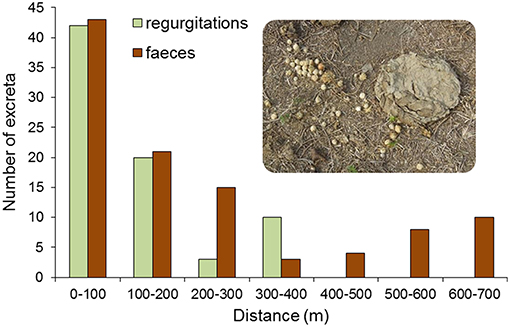
Figure 7. Frequency of regurgitations containing seeds of A. totai regurgitated by cows during rumination, and frequency of cow droppings according to the distance from the border of the palm patch (two A. totai stands pooled). Both regurgitations and droppings were recorded in intensive walking surveys in the Cerrado vegetation around palm patches. The picture shows a cow regurgitation containing multiple A. totai seeds (left) close to a cow dropping without palm seeds.
Discussion
External Dispersal as a Key Mechanism for Large-Fruited Plants
The large-fruited palms studied depend on a rich variety of variably sized vertebrates to efficiently disseminate their seeds externally at variable distances, both primarily and secondarily. External dispersal has been generally under-appreciated as a major mechanism in the evolution of plants with large fleshy fruits (Fleming and Kress, 2013). Oversized fruits defined as “megafaunal” provide variable amounts of flesh even though many of them cannot be ingested entirely, nor their seeds defecated, by any extant vertebrate. This apparent mismatch lead to the hypothesis of anachronisms involving extinct megafauna as dispersal-mediated selective agents on fruit traits shaped through endozoochory (Janzen and Martin, 1982; Guimarães et al., 2008). Our results clearly challenge this contention by showing that large seeds of palms were dispersed externally by a wide array of vertebrates of variable size but much smaller than extinct megafauna and livestock.
The primary dispersers were generally birds and monkeys extracting fruits directly from the palm bunches and flying or moving over the canopy with them in the beak or mouth (stomatochory). In particular, rich communities of variably sized parrots (weighing between 70 and 1,500 g) were recorded dispersing seeds of all the study palms, with most species dispersing several palm species. This supports the key role of parrots as efficient dispersal agents of many large-fruited plants (reviewed in Blanco et al., 2018). Owing to their general wasteful feeding behavior, parrots disperse seeds at distances ranging from below the fruiting palm where secondary dispersers act, to long distances (up to several kilometers) that are generally underestimated due to logistic challenges of measurement (Tella et al., 2016a, 2019; Baños-Villalba et al., 2017). Among secondary dispersers, rich assemblages of frugivorous and omnivorous vertebrates mostly disseminated seeds externally, although some also ingested and defecated or regurgitated the seeds of the smallest-fruited palm species. As for other large-fruited palms, rodent species were especially frequent among the short-distance secondary dispersers (e.g., Jansen et al., 2012), while ground- and tree-dwelling squirrels and primates moved palm seeds externally, both primarily and secondarily (e.g., Chapman and Onderdonk, 1998; Wright and Duber, 2001).
Overall, external dispersal emerges as the major mechanism exploited by these large-fruited plants to disseminate their seeds at variable distances by an array of dispersers. Information on disperser assemblages of the study palms in other geographical areas (Zona and Henderson, 1989; Eiserhardt et al., 2011; Virapongse et al., 2017), as well as on other large-fruited plants (Jansen et al., 2012; Tella et al., 2015, 2019; Blanco et al., 2016; Rebein et al., 2017) support these mixed redundant and complementary dispersal systems by ectozoochory. Indeed, studies revisiting and adopting the megafaunal seed dispersal hypothesis recognized guilds of external dispersers with a role in population dynamics of these plants, although overlooked their potential impacts as selective agents shaping large size and other fruit traits (Guimarães et al., 2008; McConkey et al., 2018). More generally, it has often been emphasized that fleshy pulp evolved as a reward to gulpers ingesting entire fruits and to fruit mashers swallowing tiny seeds, both constrained by gape and gut size (Fleming and Kress, 2013). This assumes endozoochory as the main process driving trade-offs between body size and dispersal ability of contemporary dispersers (Fleming and Kress, 2013; Jordano, 2014) and extinct megafauna (Guimarães et al., 2008; Pires et al., 2018). These assumptions underestimated potential selective pressures imposed by frugivores moving large fleshy fruits to consume the pulp without ingesting or predating the seeds (Stevenson et al., 2005; Baños-Villalba et al., 2017; Delibes et al., 2019), or to predate and disperse seeds while ingesting or discarding the pulp (Jansen et al., 2012). This is striking because stomatochory allows the movement of fruits and seeds that are much larger than those dispersed by endozoochory (Lambert, 1999; Stevenson et al., 2005; Blanco et al., 2016; Castañeda et al., 2018; Fuzessy et al., 2018), thus promoting selective forces other than gape or gut size in the evolution of large-sized fleshy fruit and their seeds. Each particular species can be variably efficient in seed dispersal and recruitment, which deserves further investigation. However, whole rich assemblages of dispersers overlapping among palm species and study areas would guarantee the future dispersal-dependent survival of their populations. Therefore, the results of this study suggest that fruits of these palms cannot be further supported as anachronistic. These findings add to growing evidence indicating that assumptions related to extinct megafauna because of their large size are not necessary to understand all past and current mutualistic frugivore-large fruit interactions (Howe, 1985; Jansen et al., 2012; Baños-Villalba et al., 2017; Rebein et al., 2017; Carpenter et al., 2018).
In summary, our results agree with Howe's (1985) critical view that the megafaunal syndrome hypothesis suffers from a general lack of knowledge on the natural history of seed dispersal of many large-fruited plants. A knowledge gap in the number of interactions between Neotropical palms and vertebrates has been recently highlighted (Muñoz et al., 2019). For instance, Donatti et al. (2007) expressed their surprise about the survival of some plant species, exemplified by the apparent lack of contemporary dispersers and dispersal mechanisms in A. speciosa, and only three interactions with frugivorous species have been recorded for this palm in a recent review (Muñoz et al., 2019). However, we found that this palm is extensively dispersed primarily by a large macaw (Ara ararauna) and at least by seven secondary ground- and tree-dwelling dispersers, including rodents (see Figures 4B,C), a primate, a squirrel and a corvid species. This highlight how particular animal groups, traditionally neglected as reliable seed dispersers, can promote a crucial mutualistic role for palms and other large-fruited plants (Jansen et al., 2012; Blanco et al., 2015, 2018; Montesinos-Navarro et al., 2017; Albert-Daviaud et al., 2018).
Role of Tapir as Dispersers of “Megafaunal Fruits”
Tapirs have been highlighted as the largest extant seed dispersers of large-fruited plants in the Neotropics, and thus their role as endozoochorous dispersers could resemble that of extinct non-ruminant megafauna (Fragoso and Huffman, 2000; Guimarães et al., 2008). We found that tapirs consume large amounts of palm fruits and defecate a few seeds of A. princeps and many seeds of the smallest-fruited palm species (A. totai). However, most seeds were unviable or lacked germination, and no saplings were found in abandoned or used latrines of variable size or their surroundings. In addition, although tapirs readily consumed fruits of M. flexuosa, as confirmed by abundant epicarp scales in a high proportion of excrement and latrines, the seeds were masticated and never survived gut passage. Thus, tapirs can be particularly destructive to seeds when feeding on these fruits (see also Bodmer, 1990; Fragoso and Huffman, 2000). Several studies have highlighted that different tapir species can be effective dispersers of small-medium seeds by passive browsing and defecation (Salas and Fuller, 1996; Fragoso and Huffman, 2000; Talamoni and Cançado, 2009; Campos-Arceiz et al., 2012; Barcelos et al., 2013; O'Farrill et al., 2013). However, seed recruitment has been generally evaluated under laboratory or greenhouse conditions rather than by monitoring the fate of seeds where they are excreted in the wild. Our results suggest that tapirs can be considered frequent consumers, effective predators, and poor dispersers of large-fruited palms, at least when their seeds are defecated in latrines where high densities of fecal nitrogen, pathogens and predators, as well as seed overcrowding and competition, may preclude or abort germination and seedling growth (Salas and Fuller, 1996; Campos-Arceiz et al., 2012). This was supported by the high number of old dead and unviable seeds of other plants with “megafaunal fruits” (Guimarães et al., 2008) found in the latrines. In conclusion, contrary to previous suggestions, the dispersal role of tapirs, as representative large-bodied non-ruminant frugivores, seems weak regarding large-seeded plants. Further research is required to confirm our results by considering these and other large-fruited plants dispersed outside latrines.
Livestock as Substitutes of Extinct Megafauna for Dispersal of Large Seeds
Our results suggest that ruminants are poor seed dispersers of large-fruited palm species by endozoochory implying seed defecation. After examining hundreds of cattle, equids, goat and sheep scats, only a few seeds of the palm species with the smallest fruits and seeds were found at very low frequency in cow feces. Domestic pigs show a higher frequency of seeds of the same palm in feces, although they intensively masticate their food and can be particularly destructive to a much larger proportion of seeds of these and other species, similar to peccaries (Beck, 2006). Rather, livestock can regurgitate large quantities of seeds of A. princeps and A. totai after an unknown period of rumination, or spit out the seeds without swallowing them. Under normal browsing conditions on pastures, leaves, and twigs, rumen vomiting in livestock is a rare pathologic process associated with the accidental ingestion of unusually large items such as stones and artifacts causing blockages and injuries to the digestive system (Van Soest, 1994). This agrees with our results showing that palm seed regurgitation from the cud while ruminating was apparently provoked by the ingestion of large numbers of palm fruits, for which seed defecation appears anatomically impossible for smaller livestock (sheep, goat) or unnecessary due to nutritional and physiological costs for larger livestock (Van Soest, 1994; Feer, 1995; Clauss et al., 2009; Delibes et al., 2019).
Livestock ruminants chew their cud and ferment their food for variable periods of time (Van Soest, 1994), thus variably limiting seed survival through the upper gut passage (Janzen, 1982; Bodmer and Ward, 2006; Schwarm et al., 2009). In addition, pulp is often chewed and the cleaned seeds driven out without ingestion just below (without dispersal) or at a short distance from the fruiting palms. Although the viability of palm seeds dispersed after rumination or spitting without swallowing remains unknown, these different mechanisms for exploiting fruit can have important implications on seed dispersal distances (Castañeda et al., 2018; Delibes et al., 2019). Dispersal of A. totai seeds by cows mostly occurred below the source plant, as demonstrated the high density of seeds regurgitated in resting places within palm patches where plant recruitment is hindered by trampling, grazing and soil compaction (Montúfar et al., 2011; Smith, 2015; Baños-Villalba et al., 2017; Hordijk et al., 2019), or in locations unsuitable for germination outside palm patches within the dry Cerrado vegetation matrix. The frequency of regurgitations (all of A. totai seeds) decreased as the distance from the palms increased, with maximum dispersal distances of up to about 400 m in unsuitable sites, even though cow movement capability allows much longer daily travel distances. Cattle readily clean and spit out fruits of M. flexuosa below fruiting palms, without dispersing seeds, while they and other livestock can use this mechanism to disperse other palm seeds short distances. In any case, stomatochory leads to seed dispersal of large seeds at shorter distances than those dispersed by regurgitation after rumination, meriting focused research to adequately evaluate the dispersal role of livestock (Delibes et al., 2019). Seeds regurgitated by livestock can be potentially dispersed by other organisms. For instance, large macaws (Anodorhynchus hyacinthinus and A. leari) can act in two-phased (or tertiary) dispersal by moving palm seeds regurgitated by cattle and goats (authors' unpubl. data).
Our results did not agree with the prediction that seeds of large-fruited plants should be reliably dispersed by livestock exploiting similar mechanisms to those presumably used by extinct megaherbivores (Janzen and Martin, 1982; Guimarães et al., 2008). The logic of livestock as surrogate dispersers of seeds presumably disseminated by extinct megafauna implies gut passage through the digestive system and defecation of viable seeds (Janzen and Martin, 1982; Guimarães et al., 2008, Pires et al., 2018). Following this rationale, our results indicate that the complex digestive process of ruminants (Van Soest, 1994) hinders complete gut passage of large seeds (Feer, 1995; Clauss et al., 2009; Delibes et al., 2019). Therefore, like wild herbivorous ungulates (Feer, 1995; Bodmer and Ward, 2006; Castañeda et al., 2018), livestock are not effective long-distance endozoochorous dispersers of large seeds by defecation. Similar anatomical and physiological constraints and trade-offs governing fruit swallowing and seed defecation may also have operated in potential past interactions between large fruits and extinct megafauna (e.g., Carpenter et al., 2018). However, stomatochory by seed spitting without ingestion and endozoochory through regurgitation after a variable retention period in the upper digestive tract have been cited, but not comprehensively evaluated, as mechanisms used by extinct megafauna to exploit large fruits (Guimarães et al., 2008; Pires et al., 2018). In sum, livestock cannot be considered as ecological surrogates of extinct megafauna by exclusively assuming seed defecation as a dispersal mechanism.
Rethinking the Megafaunal Seed Dispersal Hypothesis
This study negates the idea that overbuilt, fleshy fruits of the study palms are today maladapted for dispersal by contemporary fauna. Since the work of Janzen and Martin (1982), evidence has been building in support of seed dispersal of putative anachronistic plants by contemporary fauna (e.g., Jansen et al., 2012; Baños-Villalba et al., 2017; Rebein et al., 2017; McConkey et al., 2018), whose members existed in the same or much earlier geological periods as the extinct Pleistocene megafauna (Koch and Barnosky, 2006; Wright et al., 2008; Eriksson, 2016). Therefore, efforts are encouraged to rethink whether fruits assumed to have adjusted to a “megafaunal syndrome” actually required the past participation of extinct megafauna and to consider the contemporary function of domestic surrogates for seed dispersal.
Contrary to the assertion that seeds of many oversized fruits go undispersed due to the extinction of their legitimate megafaunal endozoochorous dispersers (Janzen and Martin, 1982; Guimarães et al., 2008), evidence suggests that mixed strategies combining dispersal mechanisms with asymmetric outcomes can be modulated depending on a complementary and/or redundant and rich variety of variably sized vertebrate groups (Wheelwright and Orians, 1982; Howe, 1985; Chapman and Chapman, 2002; McConkey et al., 2018). Therefore, oversized fruits can be better understood within a continuum ranging from species depending on few co-evolved dispersal partners to those depending on an array of unspecialized dispersers that can also exert selective impacts on plant fitness (for dry-fruited plants see Tella et al., 2016a,b, 2019; Gómez et al., 2018).
In conclusion, many oversized fruits assumed to be maladapted for contemporary dispersal are, in fact, misunderstood. This knowledge gap may have been partially favored by neglecting some key disperser guilds (e.g., parrots) and dispersal mechanisms (e.g., ectozoochory). By exclusively focusing in endozoochory via seed defecation as the mechanism shaping oversized fruits, the megafaunal dispersal hypothesis can fail to be adequately tested. This appealing hypothesis could attract renewed interest by considering ectozoochorous fruit mashers, thus reversing the view of past frugivore-plant mutualistic interactions markedly biased toward fruit gulpers. Alternative hypotheses are encouraged that incorporate regurgitation and spitting, and especially ectozoochory so commonly used by many vertebrates to get rid of large seeds (Fleming and Kress, 2013; Delibes et al., 2019). Thus, rather than (or before) relying on “ghosts of the past” in an attempt to explain traits that are unexpected or misunderstood, these refinements could provide a path to new fruitful research on frugivore-plant mutualistic interactions governing past and contemporary evolution of fruit traits.
Conservation Implications
A proportion of species with “unfit” overbuilt fruits show restricted distributions and low genetic variability linked to reduced gene flow, which have been associated with the extinction of megafaunal dispersers (Guimarães et al., 2008; Johnson, 2009; Doughty et al., 2016). However, by relying on extinct megafauna for seed dispersal, the anachronism hypothesis overlooks the possibility that distribution patterns and genetic variability of many plants may be affected by historic defaunation of many large-sized vertebrates (other than extinct Pleistocene mammals) exploited by humans since their arrival to the Americas (Peres, 2000; Koch and Barnosky, 2006; Muller-Landau, 2007). Deforestation and defaunation have increased since then, further reducing distribution ranges and genetic variability of many plant species to the point that these patterns could be directly associated with the recent and ongoing extinction and decline of many contemporaneous dispersers (Peres and Roosmalen, 2002; Kurten, 2013). Among primary dispersers, several macaws and primates have become globally or regionally extinct recently, while most surviving species have suffered strong declines from much of their original distribution (Wich and Marshall, 2016; Berkunsky et al., 2017; Forshaw and Knight, 2017). The ecological functions of these organisms have been undervalued or neglected in population dynamics and genetic variability of their food plants (Chapman and Onderdonk, 1998; Blanco et al., 2015, 2018; Baños-Villalba et al., 2017; Montesinos-Navarro et al., 2017, Andresen et al., 2018). This applies specifically to plant populations and communities that are now limited by low abundance in impoverished assemblies of parrots and other large-sized dispersers (Dirzo et al., 2007; Galetti et al., 2013; Hall and Walter, 2013). Unfortunately, most reviews on the loss of plant dispersal function in forests due to defaunation and other human activities have excluded parrots (Farwig and Berens, 2012; McConkey et al., 2012; Fleming and Kress, 2013; Sebastián-González et al., 2015; Peres et al., 2016), even though these key long-distance dispersers are among the vertebrates most threatened by habitat loss, hunting for bush meat and pet trade (Berkunsky et al., 2017).
We thus advocate the evaluation of historic and ongoing defaunation of key external dispersers to attempt to understand the influence of actual (rather than putative) dispersers on contemporary frugivore-plant mutualistic interactions.
Ethics Statement
This study relies on observational data obtained in areas unrestricted to people and thus did not require special permits.
Author Contributions
GB, JT, JD-L, and FH designed and conducted field work. GB analyzed the data and wrote a first draft of the manuscript. All authors contributed to improve it.
Funding
We acknowledge support for this research from Fundación Repsol and Loro Parque Fundación.
Conflict of Interest Statement
The authors declare that the research was conducted in the absence of any commercial or financial relationships that could be construed as a potential conflict of interest.
The handling editor and author, JT, declared their involvement as co-editors in the Research Topic, and confirm the absence of any other ongoing collaboration.
Acknowledgments
We thank L. Lima, M. de la Riva, A. Montesinos-Navarro, J. Salguero, D. Parada, T. Rojas, W. Montaño, and Ch. Dolan for their help in the fieldwork, P. Salinas and F. Dénes for help with the figures.
Supplementary Material
The Supplementary Material for this article can be found online at: https://www.frontiersin.org/articles/10.3389/fevo.2019.00328/full#supplementary-material
References
Albert-Daviaud, A., Perillo, S., and Stuppy, W. (2018). Seed dispersal syndromes in the Madagascan flora: the unusual importance of primates. Oryx 52, 418–426. doi: 10.1017/S0030605317001600
Andresen, E., Arroyo-Rodríguez, V., and Ramos-Robles, M. (2018). Primate seed dispersal: old and new challenges. Int. J. Primatol. 39:443. doi: 10.1007/s10764-018-0024-z
Baños-Villalba, A., Blanco, G., Díaz-Luque, J. A., Dénes, F. V., Hiraldo, F., and Tella, J. L. (2017). Seed dispersal by macaws shapes the landscape of an Amazonian ecosystem. Sci. Rep. 7:7373. doi: 10.1038/s41598-017-07697-5
Barcelos, A. R., Bobrowiec, P. E. D., Sanaiotti, T. M., and Gribel, R. (2013). Seed germination from lowland tapir (Tapirus terrestris) fecal samples collected during the dry season in the northern Brazilian Amazon. Integr. Zool. 8, 63–73. doi: 10.1111/1749-4877.12003
Barlow, C. (2000). The Ghosts of Evolution: Nonsensical Fruit, Missing Partners, and Other Ecological Anachronisms. New York, NY: Basic Books.
Beck, H. (2006). A review of peccary-palm interactions and their ecological ramifications across the Neotropics. J. Mammal. 87, 519–530. doi: 10.1644/05-MAMM-A-174R1.1
Berkunsky, I., Quillfeldt, P., Brightsmith, D. J., Abbud, M. C., Aguilar, J. M. R. E., Alemán-Zelaya, U., et al. (2017). Current threats faced byNeotropical parrot populations. Biol. Conserv. 214, 278–287. doi: 10.1016/j.biocon.2017.08.016
Blanco, G., Bravo, C., Pacifico, E., Chamorro, D., Speziale, K., Lambertucci, S., et al. (2016). Internal seed dispersal by parrots: an overview of a neglected mutualism. PeerJ 4:e1688. doi: 10.7717/peerj.1688
Blanco, G., Hiraldo, F., Rojas, A., Dénes, F. V., and Tella, J. L. (2015). Parrots as key multilinkers in ecosystem structure and functioning. Ecol. Evol. 18, 4141–4160. doi: 10.1002/ece3.1663
Blanco, G., Hiraldo, G., and Tella, J. L. (2018). Ecological functions of parrots: an integrative perspective from plant life cycle to ecosystem functioning. Emu 118, 36–49. doi: 10.1080/01584197.2017.1387031
Bodmer, R., and Ward, D. (2006). “Frugivory in large mammalian herbivores,” in Large Herbivore Ecology, Ecosystem Dynamics and Conservation, eds K. Danell, P. Duncan, R. Bergstrom, and J. Pastor (Cambridge: Cambridge University Press), 232–260. doi: 10.1017/CBO9780511617461.010
Bodmer, R. E. (1990). Fruit patch size and frugivory in the lowland tapir (Tapirus terrestris). J. Zool. 222, 121–128. doi: 10.1111/j.1469-7998.1990.tb04034.x
Boone, M. J., Davis, C. N., Klasek, L., del Sol, J. F., Roehm, K., and Moran, M. D. (2015). A test of potential Pleistocene mammal seed dispersal in anachronistic fruits using extant ecological and physiological analogs. Southeast. Nat. 14, 22–32. doi: 10.1656/058.014.0109
Bruun, H. H., and Poschlod, P. (2006). Why are small seeds dispersed through animal guts: large numbers or seed size per se? Oikos 113, 402–411. doi: 10.1111/j.2006.0030-1299.14114.x
Bunney, K., Bond, W. J., and Henley, M. (2017). Seed dispersal kernel of the largest surviving megaherbivore—the African savanna elephant. Biotropica 49, 395–401. doi: 10.1111/btp.12423
Burns, K. C. (2013). What causes size coupling in fruit–frugivore interaction webs? Ecology 94, 295–300. doi: 10.1890/12-1161.1
Campos-Arceiz, A., Traeholt, C., Jaffar, R., Santamaria, L., and Corlett, R. T. (2012). Asian tapirs are no elephants when it comes to seed dispersal. Biotropica 44, 220–227. doi: 10.1111/j.1744-7429.2011.00784.x
Carpenter, J. K., Wood, J. R., Wilmshurst, J. M., and Kelly, D. (2018). An avian seed dispersal paradox: New Zealand's extinct megafaunal birds did not disperse large seeds. Proc. R. Soc. B 285:20180352. doi: 10.1098/rspb.2018.0352
Castañeda, I., Fedriani, J. M., and Delibes, M. (2018). Potential of red deer (Cervus elaphus) to disperse viable seeds by spitting them from the cud. Mamm. Biol. 90, 89–91. doi: 10.1016/j.mambio.2017.10.004
Chapman, C. A., and Chapman, L. J. (2002). “Plant-animal coevolution: Is it thwarted by spatial and temporal variation in animal foraging?” in Seed Dispersal and Frugivory: Ecology, Evolution and Conservation, eds D. J. Levey, W. R. Silva, and M. Galetti (New York, NY: CABI Publishing), 275–290. doi: 10.1079/9780851995250.0275
Chapman, C. A., and Onderdonk, D. A. (1998). Forests without primates: primate/plant codependency. Am. J. Primatol. 45, 127–141. doi: 10.1002/(SICI)1098-2345(1998)45:1<127::AID-AJP9>3.0.CO;2-Y
Chen, S. C., and Moles, A. T. (2015). A mammoth mouthful? A test of the idea that larger animals ingest larger seeds. Glob. Ecol. Biogeogr. 24, 1269–1280. doi: 10.1111/geb.12346
Clauss, M., Nunn, C., Fritz, J., and Hummel, J. (2009). Evidence for a tradeoff between retention time and chewing efficiency in large mammalian herbivores. Comp. Biochem. Physiol. A 154, 376–382. doi: 10.1016/j.cbpa.2009.07.016
de Andrade, W. M., Ramos, M. A., Souto, W. M. S., and Bento-Silva, J. S. (2015). Knowledge, uses and practices of the licuri palm (Syagrus coronata (Mart.) Becc.) around protected areas in northeastern Brazil holding the endangered species Lear's Macaw (Anodorhynchus leari). Trop. Conserv. Sci. 8, 893–911. doi: 10.1177/194008291500800403
de Carvalho, W. D., and Mustin, K. (2017). The highly threatened and little known Amazonian savannahs. Nat. Ecol. Evol. 1:0100. doi: 10.1038/s41559-017-0100
Delibes, M., Castañeda, I., and Fedriani, J. M. (2019). Spitting seeds from the cud: a review of an endozoochory exclusive to ruminants. Front. Ecol. Evol. 7:265. doi: 10.3389/fevo.2019.00265
Dirzo, R., Mendoza, E., and Ortíz, P. (2007). Size-related differential seed predation in a heavily defaunated neotropical rain forest. Biotropica 39, 355–362. doi: 10.1111/j.1744-7429.2007.00274.x
Dominy, N. J., and Duncan, B. W. (2005). Seed-spitting primates and the conservation and dispersion of large-seeded trees. Int. J. Primatol. 26, 631–649. doi: 10.1007/s10764-005-4370-2
Donatti, C. I., Galetti, M., Pizo, M. A., Guimaraes, P. R. J., and Jordano, P. (2007). “Living in the land of ghosts: fruit traits and the importance of large mammals as seed dispersers in the Pantanal, Brazil,” in Seed Dispersal: Theory and Its Application in a Changing World, eds A. J. Dennis, R. J. Green, E. W. Schupp, and D. A. Westcott (Wallingford, CT: CAB International), 104–123. doi: 10.1079/9781845931650.0104
Doughty, C. E., Wolf, A., Morueta-Holme, N., Jørgensen, P. M., Sandel, B., Violle, C., et al. (2016). Megafauna extinction, tree species range reduction, and carbon storage in Amazonian forests. Ecography 39, 194–203. doi: 10.1111/ecog.01587
Eisenberg, J. F., and Redford, K. H. (2000). Mammals of the Neotropics, Volume 3: Ecuador, Bolivia, Brazil. Chicago, IL: University of Chicago Press.
Eiserhardt, W. L., Svenning, J. C., Kissling, W. D., and Balslev, H. (2011). Geographical ecology of the palms (Arecaceae): determinants of diversity and distributions across spatial scales. Ann. Bot. 108, 1391–1416. doi: 10.1093/aob/mcr146
Eriksson, O. (2016). Evolution of angiosperm seed disperser mutualisms: the timing of origins and their consequences for coevolutionary interactions between angiosperms and frugivores. Biol. Rev. 91, 168–186. doi: 10.1111/brv.12164
Farwig, N., and Berens, D. G. (2012). Imagine a world without seed dispersers: a review of threats, consequences and future directions. Basic Appl. Ecol. 13, 109–115. doi: 10.1016/j.baae.2012.02.006
Feer, F. (1995). Seed dispersal in African forest ruminants. J. Trop. Ecol. 11, 683–689. doi: 10.1017/S0266467400009238
Fleming, T. H., and Kress, W. J. (2013). The Ornaments of Life. Chicago, IL: University of Chicago Press. doi: 10.7208/chicago/9780226023328.001.0001
Forshaw, J., and Knight, F. (2017). Vanished and Vanishing Parrots: Profiling Extinct and Endangered Species. Melbourne, VIC: CSIRO Publishing. doi: 10.1071/9780643106499
Fragoso, J. M., and Huffman, J. M. (2000). Seed-dispersal and seedling recruitment patterns by the last Neotropical megafaunal element in Amazonia, the tapir. J. Trop. Ecol. 16, 369–385. doi: 10.1017/S0266467400001462
Fuzessy, L. F., Janson, C., and Silveira, F. A. (2018). Effects of seed size and frugivory degree on dispersal by Neotropical frugivores. Acta Oecol. 93, 41–47. doi: 10.1016/j.actao.2018.10.004
Galetti, M., Guevara, R., Côrtes, M. C., Fadini, R., Von Matter, S., Leite, A. B., et al. (2013). Functional extinction of birds drives rapid evolutionary changes in seed size. Science 340,1086–1090. doi: 10.1126/science.1233774
Gómez, J. M., Schupp, E. W., and Jordano, P. (2018). Synzoochory: the ecological and evolutionary relevance of a dual interaction. Biol. Rev. 94, 874–902. doi: 10.1111/brv.12481
Guimarães, P. R., Galetti, M., and Jordano, P. (2008). Seed dispersal anachronisms: rethinking the fruits extinct megafauna ate. PLoS ONE 3:e1745. doi: 10.1371/journal.pone.0001745
Hall, J. A., and Walter, G. H. (2013). Seed dispersal of the Australian cycad Macrozamia miquelii (Zamiaceae): are cycads megafauna-dispersed “grove forming” plants? Am. J. Bot. 100,1127–1136. doi: 10.3732/ajb.1200115
Henderson, A. (2002). Evolution and Ecology of Palms. New York, NY: The New York Botanical Garden Press.
Hordijk, I., Meijer, F., Nissen, E., Boorsma, T., and Poorter, L. (2019). Cattle affect regeneration of the palm species Attalea princeps in a Bolivian forest–savanna mosaic. Biotropica 51, 28–38. doi: 10.1111/btp.12613
Howe, H. F. (2016). Making dispersal syndromes and networks useful in tropical conservation and restoration. Glob. Ecol. Conserv. 6, 152–178. doi: 10.1016/j.gecco.2016.03.002
Howe, H. F., and Smallwood, J. (1982). Ecology of seed dispersal. Annu. Rev. Ecol. Syst. 13, 201–228. doi: 10.1146/annurev.es.13.110182.001221
Hunter, J. R. (1989). Seed dispersal and germination of Enterolobium cyclocarpum (Jacq.) Griseb. (Leguminosae: Mimosoideae): are megafauna necessary? J. Biogeogr. 16, 369–378. doi: 10.2307/2845228
Jansen, P. A., Hirsch, B. T., Emsens, W. J., Zamora-Gutierrez, V., Wikelski, M., and Kays, R. (2012). Thieving rodents as substitute dispersers of megafaunal seeds. Proc. Natl. Acad. Sci. U.S.A. 109, 12610–12615. doi: 10.1073/pnas.1205184109
Janzen, D. H. (1982). Differential seed survival and passage rates in cows and horses, surrogate Pleistocene dispersal agents. Oikos 38,150–156. doi: 10.2307/3544014
Janzen, D. H., and Martin, P. S. (1982). Neotropical anachronisms: the fruits the gomphotheres ate. Science 215, 19–27. doi: 10.1126/science.215.4528.19
Johnson, C. N. (2009). Ecological consequences of Late Quaternary extinctions of megafauna. Proc. R. Soc. B Biol. Sci. 276, 2509–2519. doi: 10.1098/rspb.2008.1921
Jordano, P. (2014). “Fruits and frugivory,” in Seeds: The Ecology of Regeneration of Plant Communities, 3rd Edn, ed R. S. Gallagher (Wallingford: CABI Publishing), 18–61. doi: 10.1079/9781780641836.0018
Koch, P. L., and Barnosky, A. D. (2006). Late Quaternary extinctions: state of the debate. Annu. Rev. Ecol. Evol. Syst. 37, 215–250. doi: 10.1146/annurev.ecolsys.34.011802.132415
Kurten, E. L. (2013). Cascading effects of contemporaneous defaunation on tropical forest communities. Biol. Conserv. 163, 22–32. doi: 10.1016/j.biocon.2013.04.025
Lambert, J. E. (1999). Seed handling in chimpanzees (Pan troglodytes) and redtail monkeys (Cercopithecus ascanius): implications for understanding hominoid and cercopithecine fruit-processing strategies and seed dispersal. Am. J. Phys. Anthropol. 109, 365–386. doi: 10.1002/(SICI)1096-8644(199907)109:3<365::AID-AJPA6>3.0.CO;2-Q
Lanes, É. C., Motoike, S. Y., Kuki, K. N., Resende, M. D., and Caixeta, E. T. (2016). Mating system and genetic composition of the macaw palm (Acrocomia aculeata): implications for breeding and genetic conservation programs. J. Hered. 107, 527–536. doi: 10.1093/jhered/esw038
Langstroth, R., and Riding, S. (2011). Biogeography of the Llanos de Moxos: natural and anthropogenic determinants. Geogr. Helv. 66, 183–192. doi: 10.5194/gh-66-183-2011
Lord, J. M. (2004). Frugivore gape size and the evolution of fruit size and shape in Southern Hemisphere floras. Austral. Ecol. 29, 430–436. doi: 10.1111/j.1442-9993.2004.01382.x
Lorenzi, H., Souza, H. M., Medeiros-Costa, J. T., Cerqueira, L. S. C., and Von Behr, N. (1996). Palmeiras no Brasil. Nova Odessa: Ed. Plantarum.
McConkey, K. R., Nathalang, A., Brockelman, W. Y., Saralamba, C., Santon, J., Matmoon, U., et al. (2018). Different megafauna vary in their seed dispersal effectiveness of the megafaunal fruit Platymitra macrocarpa (Annonaceae). PLoS ONE 13:e0198960. doi: 10.1371/journal.pone.0198960
McConkey, K. R., Prasad, S., Corlett, R. T., Campos-Arceiz, A., Brodie, J. F., Rogers, H., et al. (2012). Seed dispersal in changing landscapes. Biol. Conserv. 146, 1–13. doi: 10.1016/j.biocon.2011.09.018
Mistry, J., Schmidt, I. B., Eloy, L., and Bilbao, B. (2019). New perspectives in fire management in South American savannas: the importance of intercultural governance. Ambio 48, 172–179. doi: 10.1007/s13280-018-1054-7
Montesinos-Navarro, A., Hiraldo, F., Tella, J. L., and Blanco, G. (2017). Network structure embracing mutualism-antagonism continuums increases community robustness. Nat. Ecol. Evol. 1, 1661–1669. doi: 10.1038/s41559-017-0320-6
Montúfar, R., Anthelme, F., Pintaud, J. C., and Balslev, H. (2011). Disturbance and resilience in tropical American palm populations and communities. Bot. Rev. 77, 426–461. doi: 10.1007/s12229-011-9085-9
Muller-Landau, H. C. (2007). Predicting the long-term effects of hunting on plant species composition and diversity in tropical forests. Biotropica 39, 372–384. doi: 10.1111/j.1744-7429.2007.00290.x
Muñoz, G., Trøjelsgaard, K., and Kissling, W. D. (2019). A synthesis of animal-mediated seed dispersal of palms reveals distinct biogeographical differences in species interactions. J. Biogeogr. 46, 466–484. doi: 10.1111/jbi.13493
O'Farrill, G., Galetti, M., and Campos-Arceiz, A. (2013). Frugivory and seed dispersal by tapirs: an insight on their ecological role. Integr. Zool. 8, 4–17. doi: 10.1111/j.1749-4877.2012.00316.x
Oliveira, P. S., and Marquis, R. J. (2002). The Cerrados of Brazil: Ecology and Natural History of a Neotropical Savanna. New York, NY: Columbia University Press. doi: 10.7312/oliv12042
Onstein, R. E., Baker, W. J., Couvreur, T. L., Faurby, S., Herrera-Alsina, L., Svenning, J. C., et al. (2018). To adapt or go extinct? The fate of megafaunal palm fruits under past global change. Proc. R. Soc. B 285:20180882. doi: 10.1098/rspb.2018.0882
Peres, C. A. (2000). Effects of subsistence hunting on vertebrate community structure in Amazonian forests. Conserv. Biol. 14, 240–253. doi: 10.1046/j.1523-1739.2000.98485.x
Peres, C. A., Emilio, T., Schietti, J., Desmoulière, S. J., and Levi, T. (2016). Dispersal limitation induces long-term biomass collapse in overhunted Amazonian forests. Proc. Natl. Acad. Sci. U.S.A. 113, 892–897. doi: 10.1073/pnas.1516525113
Peres, C. A., and Roosmalen, M. G. M. (2002). “Primate frugivory in two species-rich neotropical forests: implications for the demography of large-seeded plants in overhunted areas,” in Seed Dispersal and Frugivory: Ecology, Evolution and Conservation, eds D. Levey, W. R. Silva, and M. Galetti (Oxon: CAB International), 407–421. doi: 10.1079/9780851995250.0407
Pires, M. M., Guimarães, P. R., Galetti, M., and Jordano, P. (2018). Pleistocene megafaunal extinctions and the functional loss of long-distance seed-dispersal services. Ecography 41,153–163. doi: 10.1111/ecog.03163
Power, M. J., Whitney, B. S., Mayle, F. E., Neves, D. M., de Boer, E. J., and Maclean, K. S. (2016). Fire, climate and vegetation linkages in the Bolivian Chiquitano seasonally dry tropical forest. Philos. Trans. R. Soc. B Biol. Sci. 371:20150165. doi: 10.1098/rstb.2015.0165
Rebein, M., Davis, C. N., Abad, H., Stone, T., del Sol, J., Skinner, N., et al. (2017). Seed dispersal of Diospyros virginiana in the past and the present: evidence for a generalist evolutionary strategy. Ecol. Evol. 7, 4035–4043. doi: 10.1002/ece3.3008
Salas, L. A., and Fuller, T. K. (1996). Diet of the lowland tapir (Tapirus terrestris L.) in the Tabaro River valley, southern Venezuela. Can. J. Zool. 74,1444–1451. doi: 10.1139/z96-159
Schwarm, A., Ortmann, S., Wolf, C., Streich, W. J., and Clauss, M. (2009). More efficient mastication allows increasing intake without compromising digestibility or necessitating a larger gut: comparative feeding trials in banteng (Bos javanicus) and pygmy hippopotamus (Hexaprotodon liberiensis). Comp. Biochem. Physiol. A Mol. Integr. Physiol. 152, 504–512. doi: 10.1016/j.cbpa.2008.12.006
Sebastián-González, E. (2017). Drivers of species' role in avian seed-dispersal mutualistic networks. J. Anim. Ecol. 86, 878–887. doi: 10.1111/1365-2656.12686
Sebastián-González, E., Dalsgaard, B., Sandel, B., and Guimaraes, P. R. Jr. (2015). Macroecological trends in nestedness and modularity of seed-dispersal networks: human impact matters. Glob. Ecol. Biogeogr. 24, 293–303. doi: 10.1111/geb.12270
Silva, J. F., Felfili, J. M., Klink, C. A., Farin, M. R., and Farinas, M. R. (2006). Spatial heterogeneity, land use and conservation in the Cerrado region of Brazil. J. Biogeogr. 33, 536–548. doi: 10.1111/j.1365-2699.2005.01422.x
Stevenson, P. R., Pineda, M., and Samper, T. (2005). Influence of seed size on dispersal patterns of woolly monkeys (Lagothrix lagotricha) at Tinigua Park, Colombia. Oikos 110, 435–440. doi: 10.1111/j.0030-1299.2005.12898.x
Talamoni, S. A., and Cançado, M. A. (2009). Feeding habit of the Brazilian tapir, Tapirus terrestris (Perissodactyla: Tapiridae) in a vegetation transition zone in south-eastern Brazil. Zoologia 26, 251–254. doi: 10.1590/S1984-46702009000200007
Tella, J. L., Baños-Villalba, A., Hernández-Brito, D., Rojas, A., Pacífico, E., Díaz-Luque, J. A., et al. (2015). Parrots as overlooked seed dispersers. Front. Ecol. Environ. 13, 338–339. doi: 10.1890/1540-9295-13.6.338
Tella, J. L., Blanco, G., Dénes, F. V., and Hiraldo, F. (2019). Overlooked parrot seed dispersal in Australia and South America: insights on the evolution of dispersal syndromes and seed size in Araucaria trees. Front. Ecol. Evol. 7:82. doi: 10.3389/fevo.2019.00082
Tella, J. L., Dénes, F. V., Zulian, V., Prestes, N. P., Martínez, J., Blanco, G., et al. (2016a). Endangered plant-parrot mutualisms: seed tolerance to predation makes parrots pervasive dispersers of the Parana pine. Sci. Rep. 6:31709. doi: 10.1038/srep31709
Tella, J. L., Lambertucci, S., Speziale, K., and Hiraldo, F. (2016b). Large-scale impacts of multiple co-occurring invaders on monkey puzzle forest regeneration, native seed predators and their ecological interactions. Glob. Ecol. Conserv. 6, 1–15. doi: 10.1016/j.gecco.2016.01.001
Van Soest, P. J. (1994). Nutritional Ecology of the Ruminant, 2nd Edn. Ithaca, NY: Cornell University Press.
van Zonneveld, M., Larranaga, N., Blonder, B., Coradin, L., Hormaza, J. I., and Hunter, D. (2018). Human diets drive range expansion of megafauna-dispersed fruit species. PNAS 115, 3326–3331. doi: 10.1073/pnas.1718045115
Virapongse, A., Endress, B.A., Gilmore, M. P., Horn, C., and Romulo, C. (2017). Ecology, livelihoods, and management of the Mauritia flexuosa palm in South America. Glob. Ecol. Conserv. 10, 70–92. doi: 10.1016/j.gecco.2016.12.005
Wheelwright, N. T. (1985). Fruit size, gape width, and the diets of fruit-eating birds. Ecology 66, 808–818. doi: 10.2307/1940542
Wheelwright, N. T., and Orians, G. H. (1982). Seed dispersal by animals: contrasts with pollen dispersal, problems of terminology, and constraints on coevolution. Am. Nat. 119, 402–413. doi: 10.1086/283918
Wich, S. A., and Marshall, A. J. (eds.). (2016). An Introduction to Primate Conservation. Oxford: Oxford University Press. doi: 10.1093/acprof:oso/9780198703389.001.0001
Wright, S. J., and Duber, H. C. (2001). Poachers and forest fragmentation alter seed dispersal, seed survival, and seedling recruitment in the palm Attalea butyracea, with implications for tropical tree diversity. Biotropica 33, 583–595. doi: 10.1111/j.1744-7429.2001.tb00217.x
Wright, T. F., Schirtzinger, E. E., Matsumoto, T., Eberhard, J. R., Graves, G., Sanchez, J. J., et al. (2008). A multi-locus molecular phylogeny of the parrots (Psittaciformes): support for a Gondwanan origin during the Cretaceous. Mol. Biol. Evol. 25, 2141–2156. doi: 10.1093/molbev/msn160
Keywords: disperser assemblages, ectozoochory, extinct megafauna, livestock, oversized fruits, parrots, palms, tapir
Citation: Blanco G, Tella JL, Díaz-Luque JA and Hiraldo F (2019) Multiple External Seed Dispersers Challenge the Megafaunal Syndrome Anachronism and the Surrogate Ecological Function of Livestock. Front. Ecol. Evol. 7:328. doi: 10.3389/fevo.2019.00328
Received: 04 January 2019; Accepted: 16 August 2019;
Published: 29 August 2019.
Edited by:
Casper H. A. Van Leeuwen, Netherlands Institute of Ecology (NIOO-KNAW), NetherlandsReviewed by:
Jo Carpenter, Manaaki Whenua, Landcare Research, New ZealandRens Willem Vaessen, Utrecht University, Netherlands
Copyright © 2019 Blanco, Tella, Díaz-Luque and Hiraldo. This is an open-access article distributed under the terms of the Creative Commons Attribution License (CC BY). The use, distribution or reproduction in other forums is permitted, provided the original author(s) and the copyright owner(s) are credited and that the original publication in this journal is cited, in accordance with accepted academic practice. No use, distribution or reproduction is permitted which does not comply with these terms.
*Correspondence: Guillermo Blanco, Zy5ibGFuY28mI3gwMDA0MDtjc2ljLmVz