- Keller Science Action Center, Field Museum, Chicago, IL, United States
Metropolitan areas play an undetermined role in supporting migratory monarch butterfly (Danaus plexippus) populations despite providing habitat areas rich with milkweed (Asclepias spp.), the obligate host plants for monarch larvae. Researchers from the US Geological Survey and collaborating institutions have called for an “all hands on deck” approach to establishing monarch butterfly habitat by focusing on potential contributions from all land use sectors at levels necessary to sustain the eastern migratory monarch butterfly population. To understand the current and potential contribution of milkweed stems in metropolitan areas, our research teams surveyed milkweed densities using a new “metro-transect” protocol and conducted interviews and surveys across a diverse set of stakeholder groups in four major metropolitan areas (Chicago, Minneapolis-St. Paul, Kansas City, and Austin). We developed Geographic Information System (GIS) tools that use these data to model existing milkweed stems in metropolitan areas, and to estimate the potential to add additional milkweed stems with the adoption of milkweed-friendly planting practices across different land use classes (e.g., residential, institutional, and commercial). By extrapolating metropolitan Chicago milkweed densities across US Census urbanized areas in the northern US range of the eastern monarch butterflies, we estimate that approximately 29.8 million stems of milkweed can be added under modest “enhanced” milkweed densities, and up to 271 million stems may be added under “exemplary” milkweed densities. Both estimates are derived from a two percent “adoption rate,” or landowner conversion of green spaces. These findings show that metropolitan areas provide important habitat opportunities and should be included prominently in monarch conservation strategies when working toward national goals to increase the amount of milkweed stems and monarch habitat across the Midwest. Municipal decision-makers and planners can estimate their capacity to add stems across the metropolitan landscape by identifying where the biggest opportunities exist with help from our Urban Monarch Conservation Planning Tools.
Introduction
Monarch butterflies (Danaus plexippus) east of the Rocky Mountains migrate annually between central Mexico and Canada (Flockhart et al., 2013). The overwintering population has decreased more than 80% over two decades (Brower et al., 2012; Semmens et al., 2016). One prevailing theory for this decline is the milkweed-limitation hypothesis (Pleasants and Oberhauser, 2012; Pleasants et al., 2017; but see Dyer and Forister, 2016). This hypothesis posits that increased efficiency in agricultural practices (i.e., universal use of glyphosate and Roundup Ready crops) and widespread conversion of grasslands to other land uses have resulted in a precipitous decline in milkweed stems (Asclepias spp.) that monarch larvae depend on as their obligate host plants (Zaya et al., 2017). The US Fish and Wildlife Service (USFWS) is in the process of assessing whether monarch butterflies should be listed as threatened or endangered1. This determination will be based in part on a national Species Status Assessment (SSA) underway by the USFWS, which takes into consideration current and pledged habitat conservation and restoration efforts across all sectors. Research at the national scale suggests that a fivefold increase in milkweed stems is needed to address extinction risks associated with the eastern monarch population (Pleasants, 2017; Thogmartin et al., 2017a). To accomplish this increase, Thogmartin et al. (2017b) recommend an “all hands on deck” approach, with participation in milkweed restoration efforts by five land-cover sectors including “perennial herbaceous vegetation on protected lands, land enrolled in Conservation Reserve Program (CRP), lands in rights-of-way status, land associated with agricultural practices, and the urban/suburban sector.” The USFWS and Monarch Joint Venture2 have expanded the “all hands on deck” initiative to recommend a collaborative partnership effort by organizations and individuals to increase pollinator habitat.
“Urban/suburban” areas are classified as “developed” areas in the National Land Cover Dataset and Cropland Data Layer. These lands make up the second largest footprint (45,313 km2) of the five land-cover sectors in the Midwest, but their importance and potential for breeding monarchs are not well understood. Efforts at the national scale are now underway to improve our understanding of milkweed and monarch butterfly densities. Some examples include the Integrated Monarch Monitoring Program (IMMP) (Cariveau et al., 2019), the Monarch Larva Monitoring Project (Prysby and Oberhauser, 2004; Kountoupes and Oberhauser, 2008), and sampling along roadside rights-of-way (Kasten et al., 2016). Based on our work in urban landscapes, we know milkweed is present in developed areas (Figure 1), and some studies have shown increased egg loading in urban area gardens (Cutting and Tallamy, 2015; Stenoien et al., 2015) and high potential in urban rights-of-way (Leston and Koper, 2016). However, published estimates of milkweed densities in urban landscapes are currently limited to the survey results compiled by Thogmartin et al. (2017b), which estimate 0.1 to 1.0 stems/acre in urban areas. Supplemental results from this US Geological Survey (USGS) publication are presently the sole data source used to inform the Monarch Conservation Database (MCD),3 a USFWS database system that tracks the current and anticipated monarch habitat contributions of participating organizations across the US to inform the SSA. If a main limiting factor in rebounding migratory monarch populations is the decreased availability of milkweed plants, then it is imperative to have accurate estimates about the existing density and the habitat potential of milkweed in urban landscapes, and, because of the urgency of the issue, it is necessary to have them soon.
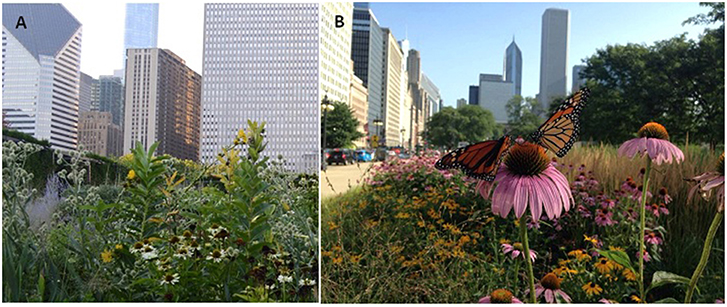
Figure 1. (A) Example of milkweed (tall green stems) in a highly urban setting (Source: Adriana Fernandez). Monarch butterflies (Danaus plexippus) are often observed in Chicago (B) within prime breeding habitat along their migratory path between Mexico and Canada (Source: Abigail Derby Lewis).
This paper addresses milkweed abundance across the various land use types that characterize US metropolitan areas—from their historic urban cores through suburbia and into the urban-rural fringe typical of federally-defined Metropolitan Areas4—and provides tools for estimating the potential for adding more habitat in these areas. In this study, we centered our analysis on “metropolitan areas” and “urbanized areas” as defined by the US Census, which provide established county-based boundaries for examining developed areas, rather than using more broadly defined terms such as “cities,” “urban,” and “suburban.” Our first goal was to estimate the current amount of milkweed available to monarch butterflies in Chicago, Minneapolis-St. Paul, Kansas City, and Austin as a basis for understanding the relative contribution of major metropolitan areas; our second goal was to understand the capacity of these metropolitan areas for increasing the amount of milkweed available for breeding monarch butterflies; our third goal was to provide geospatial planning tools that clarify opportunities for habitat expansion and support the development of conservation strategies in metropolitan areas; and our fourth goal was to estimate the potential for adding milkweed across other urbanized areas in the northern range of monarch butterflies in the United States. Based on our past work, we hypothesized that existing milkweed densities in metropolitan areas are higher than previously published by Thogmartin et al. (2017b), and that these areas have the potential to contribute a sizeable portion of the milkweed needed to support the eastern population of monarch butterflies.
Methods
Our study was conducted with support from local partners across four major metropolitan areas along the monarch's migratory route: Chicago, Minneapolis-St. Paul, Kansas City, and Austin (Figure 2). Selections were based on: their geographic location along the monarch flyway, organizations interested in partnering, geospatial data availability, and variation in environmental conditions (e.g., land use proportions and growing conditions).
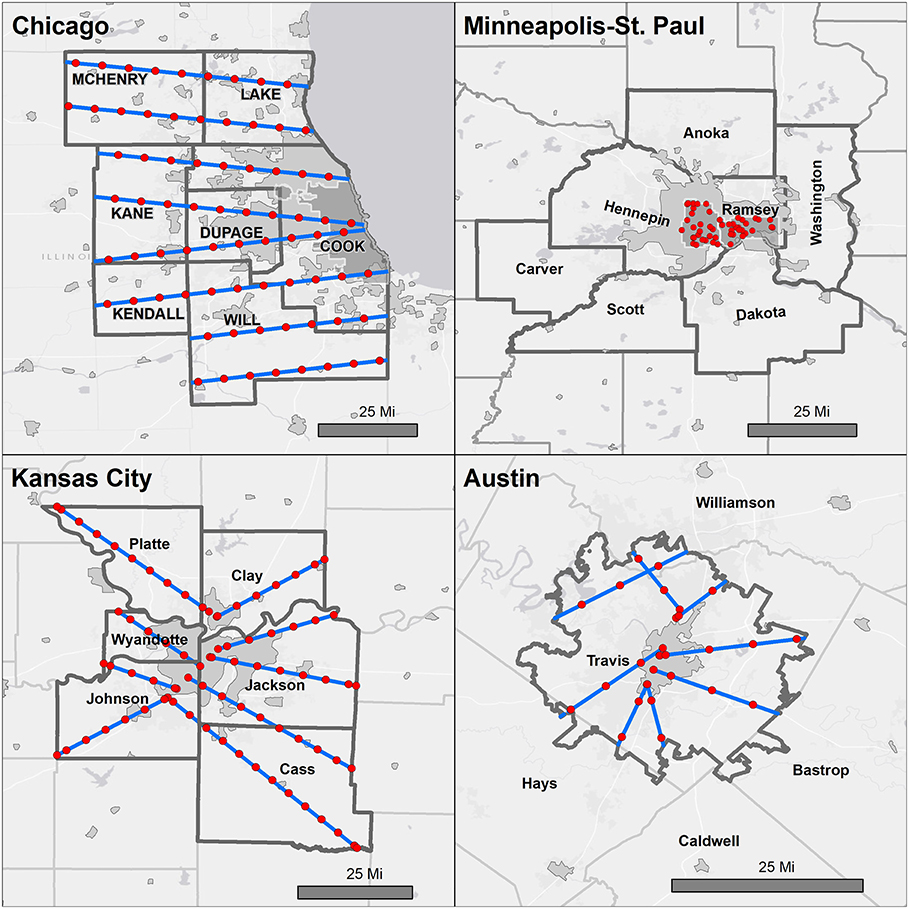
Figure 2. Analysis extent of four metropolitan areas: Chicago, Minneapolis-Saint Paul, Kansas City, and Austin, where field research was conducted to study ecological and social factors related to monarch butterfly habitat in urban/suburban areas. Shaded areas represent urbanized areas (US Census) within the metropolitan analysis extent (heavy outlined boundaries). Metro-transect lines shown in blue run from densely populated urban centers to rural sparsely populated areas. Evenly spaced sampling points (red) show where field teams were directed for sampling.
Our research methods are organized into three sections briefly summarized here and further described below (Figure 3). The Primary Data Sources and Data Calculations section includes both data sources and density calculations, which are used as inputs for the models and tools. In the Estimation Methods section, we walk through our Baseline Estimation Methods, which describe our geospatial analyses and tools used for estimating existing stems of milkweed on the landscape that are used as input for the User-Defined Scenario Estimation Methods, which describes the methods and tool used for estimating habitat potential. Outputs from the baseline and scenario planning tools were then used in the Extrapolation Methods section for estimating baseline, enhanced, and exemplary milkweed counts across urbanized areas in the eastern US monarch butterfly range.
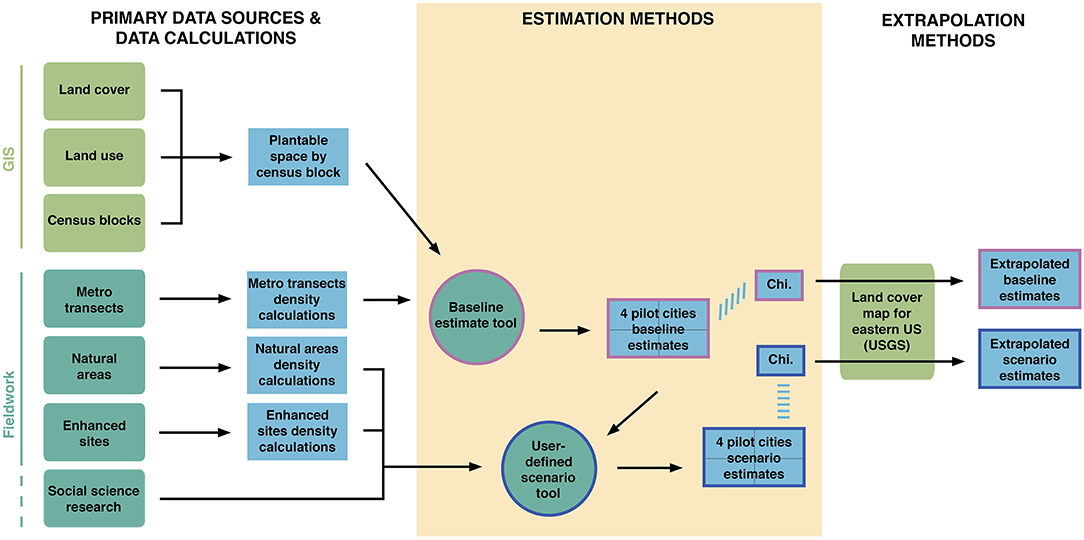
Figure 3. Organization of our methods and approach including data gathering and analysis. The Primary Data Sources and Data Calculations section addresses GIS data and fieldwork used to produce geospatial data which is combined for incorporation into our tools in Estimation Methods. We ran the Baseline tool for all cities which are then used as input to the Scenario tool. From both tools, Chicago estimates are ultimately used for superimposing onto the USGS land cover raster for extrapolation to regional scale estimates.
Primary Data Sources and Data Calculations
Geospatial Data
In developing our methods for sampling across metropolitan areas, we used precise land use data and high-resolution land cover data, typically provided by Metropolitan Planning Organizations (MPOs)—although data coverage, classification, and resolution can vary substantially across regions. For all four metropolitan areas, we sought data to cover the entire metropolitan area at the finest scale possible. In Austin, the regional planning agency serves Travis, Williamson, Bastrop, Caldwell, and Hays counties. Since available data only included Travis County, where Austin is located, we reduced our analysis extent to this central county. A list of key data sources used is found in Supplementary Table 1.
The National Land Cover Database covers the nation with 30-meter resolution cells sorted into 20 land cover classes, including low, medium, and high density developed land. Increasingly, higher resolution data are available for major urban areas, though typically with fewer land cover classes. For Chicago, Minneapolis-St. Paul, and Austin we had high-resolution land cover data (two-foot in Chicago, one-meter in Minneapolis-St. Paul, and three-foot in Austin), which included seven land cover classes for Chicago and Minneapolis-St. Paul (bare soil, buildings, grass/shrub, other paved surfaces, roads/railroads, tree canopy, and water) and only one land cover class (tree canopy) for Austin, which we combined with a “remaining pervious” GIS layer. Kansas City presented a significant data challenge, as the land cover layer was at a much coarser (eight-foot) resolution.
We created a dataset called “plantable space,” to describe both where pollinator habitat exists, and where non-forested green space exists that could potentially be converted to pollinator habitat. This layer was primarily derived from the grass/shrub land cover class. Although many green spaces may have a land use that is not compatible with conversion to pollinator habitat (e.g., recreational sport fields and airports), throughout much of metropolitan areas, these existing non-forested green spaces are where most of the opportunities to plant milkweed exist. While there are some exceptions where built up (hardscape) areas may be converted to habitat areas (e.g., the conversion of a rail line), the vast majority of opportunities are in existing vegetated areas due to lower conversion costs. We excluded the majority of tree canopy, because densely forested areas do not have enough light penetration to support the growth of most milkweed species, although Chaplin and Walker (1982) note that fourleaf milkweed (Asclepias quadrifolia) is commonly found in low-light forest understory. From expert opinion and estimates based on the proportion of our occurrence data located under tree canopy, we estimate that approximately 20% of forested areas provide suitable habitat for milkweed species capable of growing under the partial shade of solitary trees and along the woodland edge. In densely forested areas, plantable space calculations included only grass/shrub land cover, while in all other green spaces, plantable space calculations included grass/shrub as well as 20% of tree canopy. To examine how plantable space is distributed across land use categories, we isolated the grass/shrub land cover class and then reclassified it by the land use class where it occurs. This combined data layer is the basis for our modeling tools (Figure 4).
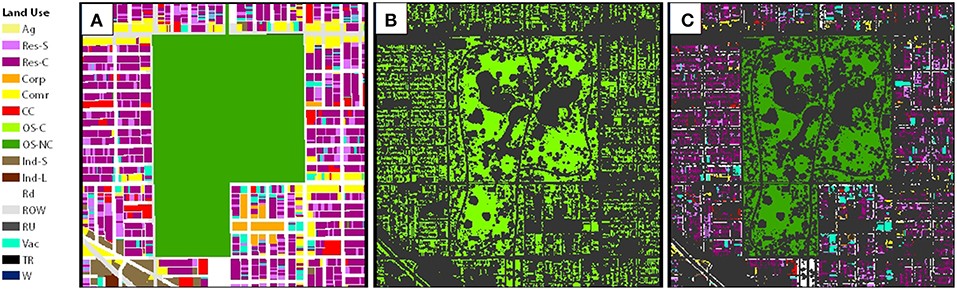
Figure 4. To estimate the basis and capacity metropolitan areas have for contributing to monarch habitat, we combine land use and land cover data to characterize what we call “plantable space.” Land use data (A) including Open space non-conservation (green), Residential (light and dark purple), and Commercial (yellow), are used to reclassify the grass/shrub land cover (B) to yield plantable space characterized by land use categories (C). Plantable space is a broad classification that includes everything from turf grass and shrubs to high quality prairie sites. In many cases only a fraction of plantable space might be reasonably converted into pollinator habitat due to land use restrictions (e.g., sports fields), but by eliminating built up areas (e.g., roads) we can improve our density estimates.
Inventories of existing land use are commonly divided into parent categories such as residential, commercial, and industrial. These datasets are developed by MPOs and—for the four metropolitan areas—originally contained between 22 and 60 land use classes. To facilitate comparisons across our study areas, we consolidated land use classes into 16 standardized categories, bearing in mind as we did so that land use in this case had much to do with how land users might manage their land for monarch conservation (Table 1). Both the creation of our 16 land use classes and the consolidation of each city's land use into these classes was done by our multidisciplinary team of ecologists, social scientists, and geospatial analysts. We relied heavily on the metadata provided with each dataset and consulted with local partners as we combined classes together. For more details see Supplementary Section 1.
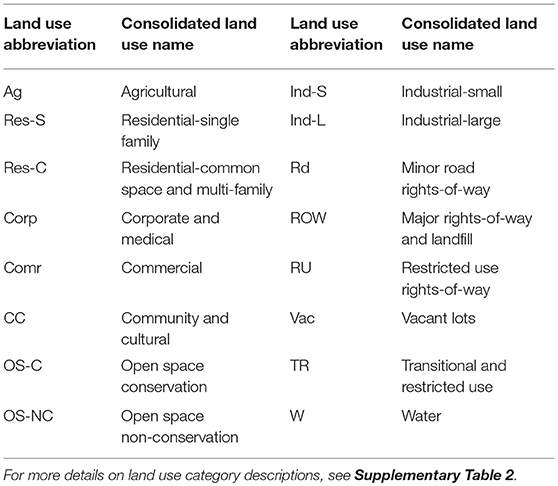
Table 1. Land use categories from Chicago (60 in total), Minneapolis-St. Paul (22), Kansas City (45), and Austin (38) were consolidated into 16 land use classes.
The way metropolitan areas classify open space within their land use is an important factor influencing both our plantable space and our final baseline calculations. In Austin and Chicago, the land use data sets differentiated between recreational open space and open space managed for conservation, whereas in Minneapolis-St. Paul and Kansas City, the land use data did not differentiate between these categories. For Minneapolis-St. Paul we migrated their combined “preserves and parks” land use into Open space conservation (OS-C) because it contained a large wildlife refuge, and in Kansas City we migrated their “parks and open space” land use into Open space non-conservation (OS-NC) in consultation with local partners.
Of the 16 defined land use classes, three encompass the land along rights-of-way, which makes up the third largest sector (43,148 km2) of the five examined by Thogmartin et al. (2017b). We extracted minor road rights-of-way to incorporate the strip of land or “greenway” between streets and sidewalks or drainage ditches, typically owned by the city or county in more rural areas. Often Minor right-of-way areas are not separated from Major rights-of-way. We isolated Minor right-of-way areas by applying a series of steps including buffering and extracting intersecting major roads, highways and ramps. For definitions of all land use categories see Supplementary Table 2.
To assist field teams conducting natural areas sampling in each metropolitan area, we used various geospatial protected area layers from the local landowner authority, or state/national protected land datasets5 to aid in planning field sampling locations. These boundaries were also used in calculating milkweed densities. US census blocks were used as geographic analysis units for aggregating land use and plantable space model inputs and outputs to/from our geospatial tools. Census blocks vary in size based on population density and allow for comparison of land use areas by demographic characteristics.
For extrapolation when estimating baseline and scenario estimates for urban areas at regional scales, we used a USGS-developed habitat raster developed for a national milkweed calculator which estimates the amount of milkweed present at the county scale. This tool uses as its basis for extrapolation a combined raster layer comprised of the Cropland Data Layer (CDL), the National Land Cover Database (NLCD), and US Census Bureau right-of-way data6.
Field Sampling and Density Calculation Methods
We used three field sampling methods to acquire information about current milkweed densities across different land use classes including: (1) randomized metro-transects for baseline density estimates, (2) targeted sampling of natural areas, and (3) targeted sampling of other “enhanced sites.” Both methods of targeted sampling were used for enhanced and exemplary density estimates. Sampling occurred throughout the 2016 growing season, approximately June through September of 2016 (and 2017 in Chicago). To estimate the baseline density of milkweed in each metropolitan land use area, milkweed stems were quantified using randomly sampled “metro-transects” running from more developed to less developed lands. While this approach allowed us to quickly and efficiently examine existing milkweed densities in most land use classes, this method was not practical for examining natural areas in Open space conservation and Open space non-conservation lands. Because sampling in natural areas required concentrated surveying in appropriate areas, advanced planning, and ample survey time, we conducted targeted rather than randomized sampling of natural areas. Since this approach was biased toward sites with higher milkweed densities, baseline densities for Open space conservation and Open space non-conservation areas were calculated using alternative methods described below. In addition to targeted sampling of natural areas, targeted “enhanced sites” sampling was conducted for all land use classes to get an understanding of the typical density of milkweed at sites where it is intentionally planted. The top ten percent of these enhanced sites were used to define “exemplary sites,” which set our upper limit on the amount of greenspace landowners might convert to habitat across different land uses.
Baseline densities were calculated on both a plantable space basis as well as on a total land use (LU) basis. For densely forested areas, we calculated plantable space on a grass/shrub (GS) basis and for all other green spaces, we calculated plantable space on a grass/shrub plus 20% tree canopy (GST) basis. To illustrate these density calculations, suppose 10 milkweed stems were counted in the agricultural component of a sampled site. For density calculations on an LU basis, we calculate the baseline density as the number of milkweed (10) divided by the acres of the site's agricultural component. For density calculations on a GST basis, we calculate the baseline density as the number of milkweed (10) divided by the acres of grass/shrub plus 20% of the acres of tree canopy that occur in that agricultural component as calculated from the plantable space land cover data reclassified by land use. Density calculations on a plantable space basis result in a higher number of milkweed stems per acre than calculations on an LU basis. This is because the total stem count is divided by a smaller area representing plantable space as opposed to dividing by the entire land use area.
Metro-transects sampling and milkweed density calculations
In the random sampling protocol called “metro-transects,” we sampled milkweed densities and habitat information across the metropolitan population gradient in Chicago, Kansas City, and Austin. Due to limitations in the number and time available of field crew members, Minneapolis-St. Paul restricted their sampling to 38 randomly selected neighborhood blocks inside the city limits. Metro-transects were run from highly urban areas through suburban and into rural areas at the outermost edge of the counties that comprise US Census-defined metropolitan areas. Transects used randomly staggered start distances between zero to four miles and were followed by evenly spaced sampling points every five miles in Chicago, and every four miles in Kansas City and Austin (Figure 2). The larger span between sampling points in Chicago was to accommodate the longer transect distances originating from the populated lakefront (on the eastern edge), as opposed to Kansas City and Austin where the urban cores are more centrally located within the metropolitan area. In all cases, we purposely angled our transect lines to avoid aligning with highly rectangular road networks which could otherwise have systematically skewed our sampling. Figure 5 shows examples of sampling locations along metro-transect lines in the Chicago metropolitan area.
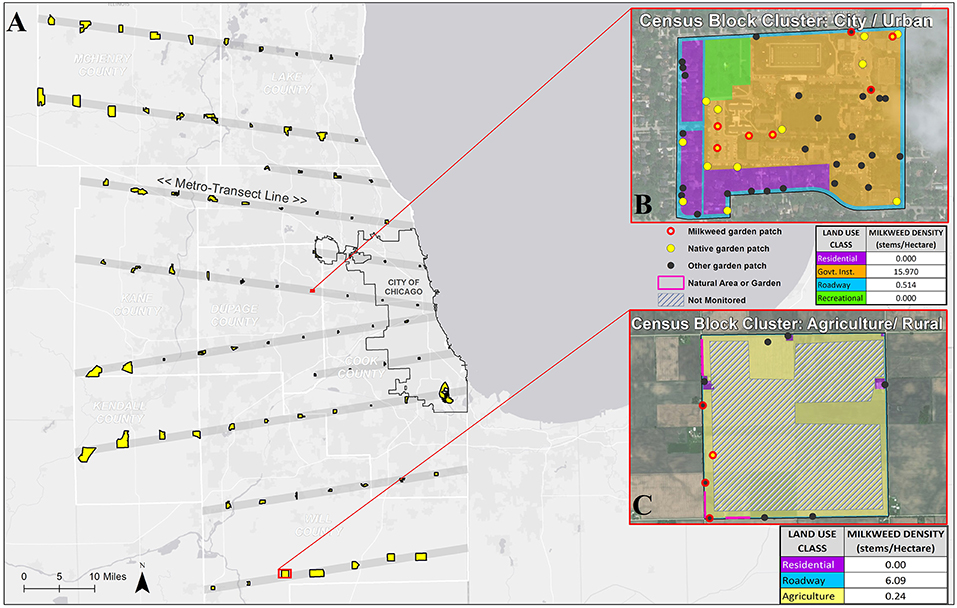
Figure 5. Example of metro-transect lines and sampling locations in Chicago. Milkweed and habitat data was collected along “metro-transects” which run from high to low human population density zones. Sampling areas in yellow on the main map (A) are clusters of US census blocks that intersect with the sampling points every five miles. In this example from the Chicago metropolitan area, we show the comparison between an urban census cluster (B) vs. a rural census cluster (C). Shading color indicates the land use, points are where habitat was encountered (red point contain milkweed), and hatching indicates areas that could not be sampled.
“Sampling clusters” were delineated around each sampling point by taking the intersection of all US census blocks within a 100-meter buffer from the sampling point. By using census block clusters, boundaries were clearly defined for the field team since the clusters align well with streets and property lines. Field teams conducted both walking and driving surveys. In Kansas City, 54 random sampling clusters were visited; in Austin, 66 sampling clusters were visited; and in Chicago, 65 were visited.
Within each sampling cluster, the field teams counted the number of stems of milkweed by species. Patches of land with plants in bloom were noted, and depending on the size of the patch, were recorded as either individual point data (typically small patches less than 25 square feet), or as a polygon within which plants were counted. Additional collected data included estimated patch size, percent native plants, percent volunteer/weed patch, percent ornamental plants, percent food garden, and number of native and blooming species; these categories were not mutually exclusive. We reserved the “native plants category” for areas that appeared to be intentional plantings and used the “volunteer/weed patch” category for unmanaged areas. These additional data attributes were not utilized for the present study. For field data collection, we used Android-based tablets with a built-in GPS and ESRI Collector application (version 18.0.1) pre-loaded with sampling locations and data templates. Field teams captured data for all land use classes that intersected the sampling cluster with the exception of Open space conservation and Open space non-conservation areas, since separate sampling methods were used for capturing stem densities across natural areas (see below).
Field visits were limited to a maximum of two to three hours for each sampling cluster. Areas that could not be observed from the public right-of-way were excluded from the analysis unless permission was granted by the landowner. This excluded most backyards in residential areas. Since residents may have planted or allowed milkweed plants to “volunteer” in their backyards, we recognize this may underestimate residential milkweed densities. Field technicians marked areas not sampled due to time limitations or inaccessibility and provided a reason why sampling did not occur. These areas were removed from our density calculations.
Each sampling cluster was divided into “components” based on land use type. For example, a single cluster could be made up of an Agricultural component, an Industrial-small component, etc. Within each survey cluster, if a recorded milkweed polygon crossed multiple land use types, the percentage of the polygon within each land use was calculated and the number of milkweed stems counted for the polygon was applied proportionally to each land use component based on that area percentage. Thus, the total stem count for a land use component within a sampling cluster is comprised of the total stem count from individual points that fall within the land use component in addition to the proportion of milkweed stems contributed by polygon sampling areas.
For more accurate estimates of the existing baseline and distribution of milkweed stems, the milkweed density for each land use component was calculated in stems per acre on a plantable space (or GST) basis for use with our geospatial tools, and on a total land use basis to align with other research standards in the literature. To calculate the average milkweed density for each land use class, we took all of the metro-transect components that shared the same land use and calculated the mean density (on both a GST and LU basis) along with the standard deviation and standard error. Because there was a large variation in component size within a given land use class (e.g., agricultural components ranged in land use size from 0.03 acres to 341 acres), we calculated both standard and weighted means. The latter allows larger areas to contribute more to the mean. We calculated the weight that would be applied to each component's GST and LU density by taking the area sampled for each component and dividing it by the sum of all land use components sampled. The density for each component was then multiplied by each component's weight. The mean weighted density was calculated for each land use class.
Targeted sampling of natural areas and milkweed density calculations
Milkweed densities from metro-transect surveys were calculated for all land use classes except natural areas. For this study, natural areas were divided into two categories: Open space conservation and Open space non-conservation. OS-C refers to lands which are managed primarily for conservation (such as a state park or a wilderness area) while OS-NC refers to lands with a primarily recreation or other land use, but which could have natural areas as a secondary land use (such as a city park or a municipal golf course).
To sample natural areas, we carried out a “targeted” sampling strategy distinct from metro-transects for several reasons: (1) natural areas are made up of many vegetation communities, some of which are not milkweed habitat, and we wanted to ensure that our field teams were collecting data within areas appropriate for milkweed habitat; (2) natural areas require advanced planning with randomized site level transects and landowner permits for scientific research; and (3) natural areas sampling often takes several hours, disrupting the workflow of metro-transect sampling. For both OS-NC and OS-C sites, scientific research permits were obtained from the land-owning agencies and land managers were consulted about the sites and units most likely to have milkweed habitat. This process allowed us to target our sampling to areas where we would likely find milkweed stems. Because we were unable to obtain site histories for many of our sites, we were not able to control for the restoration age of the places we sampled. In Austin, 51 natural areas were visited; in Chicago, 27 natural areas were visited. Natural areas in Minneapolis-St. Paul were not sampled due to time constraints, and in Kansas City, available GIS data was inadequate to follow our sampling protocol. The full sampling protocol for measuring milkweed density in natural areas can be found in Supplementary Section 2 and on the Field Museum's website7.
The milkweed density for each natural area sampling site was calculated based on the area surveyed. Due to the variable densities of milkweed within and among sites and our method of targeted sampling, we removed the statistical outliers using the 1.5 x Interquartile Range (IQR) Rule for both OS-NC and OS-C lands. After removing values above the third quartile + 1.5 IQR as sampling site outliers, the mean density of the remaining areas within each land use class was calculated along with the standard deviation and standard error. We termed these our “hotspot” densities, which represented the average density that was found in enhanced sites.
We know that not all OS-NC and OS-C lands will have the same milkweed density as their respective hotspot areas. These lands are managed for multiple uses that are not compatible with pollinator habitat, including developed areas and grassy areas that are regularly mowed. As a result, we sought to determine the proportion of open space lands that are maintained as non-forested natural areas and thus are compatible for milkweed habitat. We designated this compatible habitat as plantable non-cultural (PNC) land. Below we present equations used to calculate the percent of plantable non-cultural land, and the total milkweed stems within OS-C and OS-NC. We used GIS data and numbers provided by major landowning agencies in the Chicago area to determine what percent of OS-NC and OS-C land is typically managed as natural areas (defined as non-cultural land), as opposed to areas maintained for recreational activities such as sports fields and picnic areas (defined as cultural land). For OS-NC, the Chicago Park District reports that of its 8,832 acres, 1,850 acres are managed as natural areas8. To get this on a plantable space basis, we calculated the acreage of grass/shrub area and added 20% of the tree canopy area within the park district's natural areas. Based on consultation with land managers, we characterize trees in parks as being dispersed, allowing enough light penetration for milkweed habitat. We used GIS analysis to determine the percentage of natural areas within OS-NC lands that contain plantable space for a known sample of natural areas (defined as GST in Sampled Natural Areas), and applied that proportion to the 1,850 acres of park land managed for natural areas to get a more accurate estimate of plantable space in all OS-NC natural areas (GST in NA). The result, when divided by the calculated acres of plantable space within all OS-NC lands (Total GST in LU), produced the percentage of plantable space within OS-NC lands that are within natural areas (defined as %PNC land).
The percent of plantable non-cultural land was applied to the amount of land in a given land use that is plantable space to derive the amount of land that is available for milkweed (Total PNC area in LU).
This was then multiplied by different milkweed densities (for baseline, enhanced, and exemplary estimations) to calculate the total number of milkweed stems in the land use:
To apply this equation for baseline estimates, we used the milkweed density from Thogmartin et al. (2017b, Supplement table 3.1) for Conservation Reserve Program-Non-wet (CRP-NW, 112.14 milkweed stems/acre), which we estimate as the closest baseline approximation for natural areas. For enhanced density estimates, we used the hotspot milkweed density from our targeted surveys. For exemplary density estimates, we applied the mean milkweed density observed in the top ten percent of OS-NC sites, including outliers.
We were unable to calculate the percent of plantable non-cultural land for OS-C using the same methodology as OS-NC, because the GIS data we had for lands within the Forest Preserve District of Cook County (FPDCC) was not recommended for this purpose. Thus, for our density calculations, we used their published information. In their Natural and Cultural Resources Master Plan, the FPDCC describes 27.6% of their land as “cultural,” which includes developed and heavily altered vegetation (including mowing)9. From this, we derived that 72.4% of their land is managed as natural areas (Non-cultural land). We estimate that this percentage approximates the proportion of plantable space managed in natural areas (Percent of Plantable non-cultural land). As a check to this percentage, we also used the GIS data provided by FPDCC to calculate the percentage of natural areas that were within OS-C plantable space. We calculated the acreage of natural areas that could be considered suitable milkweed habitat, which included Eurasian meadow, prairie, savanna, sedge meadow, and shrubland, and divided it by the acres of grass/shrub land within OS-C. The result (73.4%) was similar; therefore, we decided to apply the more established 72.4% estimate. Using the same formula above, we applied the 72.4% (%PNC land) to the acres of plantable space in OS-C land to derive the total plantable non-cultural area in OS-C. Plantable space was calculated on a grass/shrub basis to account for the densely forested nature in this land use category, which would not provide adequate sunlight for milkweed. To apply this equation for baseline estimates, we used the national milkweed density from Thogmartin et al. (2017b, Supplement table 3.1) for CRP-NW (112.14 milkweed stems/acre). For enhanced density estimates, we used the hotspot milkweed density from our targeted surveys. For exemplary density estimates, we applied the mean milkweed density observed in the top ten percent of OS-C sites, including outliers.
The baseline density estimates used for OS-C and OS-NC in Chicago were also used in Minneapolis-St. Paul and Kansas City. For Austin's baseline, we applied the sample percentages of plantable non-cultural land to the milkweed densities recorded in Austin for OS-C and OS-NC hotspots, since we used the same sampling approach as in Chicago. While this assumes that the percent of natural areas in OS-C and OS-NC in Austin are about the same as they are in Chicago, we lack the GIS data on natural areas in Austin to test this.
Enhanced and exemplary sites sampling and milkweed density calculation
To run the scenario planning tool, we used estimated milkweed densities at sites with intentionally planted milkweed and sampled densities from OS-C and OS-NC lands. We divided those sites into two categories: enhanced sites, which refer to the average density of milkweed at sites with over five total stems of intentionally planted milkweed, along with exemplary sites, which refers to the average of the milkweed densities observed in the top ten percent of sites in each land use category, including outliers. As previously mentioned, the purpose of the targeted sampling of exemplary sites is to understand the upper threshold of what people are willing to plant on their land. These sites were located based on a snowball sampling method (Schensul and LeCompte, 2010), in which we contacted people through our networks and through participants in our other monarch work to find patches of intentionally planted milkweed across the 16 land use categories. For every site with at least five milkweed stems, the milkweed density was calculated on a grass/shrub and 20% tree canopy basis for the surveyed area, and the mean density, standard deviation, and standard error were calculated for each land use class. For land use classes where we were unable to find sites with more than five stems of milkweed, we used parcels with over five stems of milkweed collected through our metro-transect surveys. We used the average density found at enhanced sites to estimate the milkweed density likely to be found at sites where milkweed is intentionally planted, and we use the exemplary site density for the realistic maximum that landowners will likely implement. We assume that targeted future installations and restorations will on average have similar milkweed densities as those measured at these sites. Enhanced and exemplary densities are used in combination with “adoption rates” which estimate the percent land area that landowners will convert into similar milkweed densities for a given geographic extent.
Municipal case study sampling
Municipalities have increasingly taken steps to enhance monarch and pollinator habitat within their jurisdiction, such as signing the Mayors' Monarch Pledge10. Such places offer a glimpse into what “enhanced” and “exemplary” scenarios might look like, should a wide variety of actions be taken. In the Chicago region, we worked with two local municipalities, Glenview and Schaumburg, to estimate milkweed densities at localized scales for comparison with regional estimates, and as case studies for testing the application of our monarch conservation planning tools. We partnered with the Natural Resources Manager for the Village of Glenview to deploy a field team in summer 2017 to comprehensively survey all of Glenview visible from the public right-of-way, using similar data gathering techniques deployed in metro-transect sampling. As with metro-transect sampling, areas that were not accessible/visible (e.g., most backyards) were digitally captured by the field team and later removed from density calculations. In the Village of Schaumburg, we partnered with the Landscape and Sustainability Planner for the Village to survey a random selection of census blocks. In all other aspects, the field sampling methods were the same.
Social Science Surveys and Interview Methods
Our research team included social scientists, who conducted interviews and surveys to understand the current and potential contribution of cities from a more qualitative approach. Based on our prior ethnographic work with Chicago communities, the team's social scientists began this project knowing that many people in and around cities care about monarchs and/or nature more broadly11. The goal of the social science research was to understand what this enthusiasm and interest adds up to; how these activities connect to other issues; and which engagement strategies are most effective with which groups. To this end, social scientists collected 734 online surveys and conducted 76 phone or in-person semi-structured interviews across the four pilot metropolitan areas, asking participants about their conservation beliefs and practices with extra attention given to monarchs and milkweed. Study participants included members of faith-based organizations, universities, elementary schools, community gardens, parks and recreation departments, utility companies, conservancies, departments of transportation, as well as citizen scientists, home gardeners, land managers, landscape designers, and others. Researchers employed snowball sampling to recruit participants through their organization's conservation community connections, and thus reached a population more conservation-oriented than the general population. Of particular relevance to the geospatial estimates discussed in this paper are the questions we asked participants about the land they manage, such as: how much of the total plantable open space on this site is made up of native plants?; how much milkweed is there at this site?; and, in the next 5 years, would you be willing and able to convert more of the plantable open space of this site to native plants and/or native milkweed?
Social science surveys and interviews were used in part to validate our ecological methods and model assumptions (e.g., enhanced and exemplary concepts and methods), and helped to inform our understanding about adoption rates used in our scenario planning tool. Surveys and interviews were also used to identify landowners with enhanced and exemplary sites across land use sectors. Knowing the landowners helped our field teams locate enhanced sites to visit.
Estimation Methods
Baseline Estimation Methods
We developed an Urban Milkweed Baseline Tool for estimating the total amount of existing milkweed and the average stem density for each census block in the metropolitan area. This tool, along with our Scenario-Based Planning Tool, Urban Monarch Guidebook, and Urban Monarch Conservation Planning Toolsets, is freely available via our website12. The baseline and scenario-based planning tools are designed to allow municipal and regional planning agencies, major landowners, and federal, state, and non-profit conservation organizations to estimate their current milkweed contribution in support of monarchs, and to assist in goal-setting for monarch butterfly conservation planning. The tools also allow users to examine potential co-benefits and opportunities available through the combined application of establishing pollinator habitat areas while addressing other goals and infrastructure issues such as stormwater runoff, flooding, and compliance issues. These geospatial tools were developed in collaboration with USGS staff by modifying a national Milkweed Calculator Tool developed by the Monarch Conservation Science Partnership13. GIS users are encouraged to download and utilize the tools, manual, and guidebook for these and other planning purposes.
Our baseline milkweed estimation tool uses US census blocks with a modified attribute table (for the four metropolitan areas where we sampled). This modified table includes, for every census block, the total amount of plantable space by each of our 16 consolidated land use classes. These values were calculated for each census block by summing the total amount of grass/shrub and 20% tree canopy using the Tabulate Area and Spatial Join tools (ArcMap Spatial Analyst extension version 10.6). We recorded these values in square feet both to be consistent with the unit of measurement from the layer (Illinois State Plane, US survey feet), and to use a more accurate measure than fractions of acres for the many small patches surveyed. Ultimately, our geospatial tools convert all measures in square feet to acres. All densities are calculated on a stems per acre basis.
Using the weighted mean milkweed densities by land use class, the calculator applies these densities to each census block and provides both a numeric and graphic output showing the estimated number of milkweed stems occurring in each census block and the average stem density. We used this tool for estimating the total stem count for each of the four metropolitan areas. Using the attribute tables from these model outputs, it is possible to summarize stem contributions by land use class.
User-Defined Scenario Estimation Methods
We use the output from the Urban Milkweed Baseline Tool along with user-supplied adoption rates as inputs for running our Scenario-Based Planning Tool. This tool estimates milkweed stem counts and density by census blocks after applying user scenarios across land use types. More specifically, users apply an estimated adoption rate (see below) for one or more land use class(es) and can apply an “enhanced,” “exemplary,” or user-supplied milkweed density for the land use classes of interest. Since these tools use small geographic units (census blocks) as their basis, they can be combined with other data layers (e.g., public parks, vacant lots, utility corridors, or planning project areas) to give area and project-specific milkweed stem density and total count estimates. For more information about the tool see our Urban Monarch Guidebook, or for information on how to access and use the tool itself, see the Urban Monarch Conservation Planning Toolsets manual on our website14.
Estimating Adoption Rates
Adoption rate is defined as the rate at which landowners convert their plantable space to habitat with a milkweed density akin to what we have observed and calculated from either enhanced or exemplary sites. In referring to a two percent adoption rate, for example, for a given land use class (e.g., Residential-single family), we are estimating that two percent of all grass/shrub area within that land use class will have a stem density that is equivalent to the target density specified by the user (e.g., enhanced or exemplary stem density) when running the scenario tool. Since our exemplary stem densities are meant to be aspirational, these can be used for setting the upper limit of goal-setting over a longer term, while enhanced densities are meant to reflect a starting point for goal-setting over a shorter term.
When using our tool, the user inputs the adoption rate for their analysis extent. For the purpose of exploring what may be possible at local and regional scales, we use two, five, and ten percent adoption rates at enhanced densities, as well as a two percent adoption rate at exemplary densities. To determine appropriate adoption rates, we would ideally conduct a longitudinal study on the conversion of green space into habitat over time while also tracking changes in milkweed density. However, since our study did not directly research adoption rates, we used a space-for-time comparison15 to examine reasonable adoption rates. To do this we compared case studies in Glenview and Schaumburg where pollinator work has been recently focused, to the Chicago region where much less pollinator work has been done. Thus, we consider the larger regional area as time zero (t0), and the local density in Glenview and Schaumburg as time one (t1). For each land use class, we then used a pairwise comparison of our baseline milkweed density from the Chicago region (Rd) with the local-baseline milkweed density (Ld) over a period of time (t1-t0) in years of focused monarch activity. The following equation shows the relationship between the calculated adoption rate (AR) and the enhanced or exemplary milkweed density (Ed):
where the adoption rate (AR) is equal to the difference in local density (at t1) and regional densities (at t0), divided by time in years (t1-t0) over which this transformation in density took place, multiplied by the enhanced or exemplary density (Ed). The adoption rate for enhanced and exemplary densities are calculated separately. The assumption is that the main driver of higher observed densities is known and is not due to environmental or geographic factors. Our sampling in Glenview and Schaumburg indicates that a two percent adoption rate at exemplary densities is a reasonable scenario, given aggressive local action (see Supplementary Section 3).
Extrapolation Methods
The USGS has delineated several core monarch model regions (Rohweder and Thogmartin, 2016). Based on our findings for the Chicago metropolitan area, we extrapolate across the North Central and Northeast monarch regions, and these regions combined with the South monarch region, to estimate what a two percent adoption rate might look like for all urbanized areas in these core areas using our baseline, enhanced, and exemplary milkweed densities (Supplementary Figure 1). The analysis areas within these core regions are US Census urbanized areas, which are defined as urban areas with a population of 50,000 or more people16.
There are significant model assumptions in this extrapolation, including: environmental growing ranges and conditions of milkweeds, similar socioeconomic conditions to Chicago, social momentum and desire to support monarchs, accuracy of our milkweed density estimates, and translation of our model onto the USGS habitat raster17 and its applicability across the eastern range. In addition, when considering extrapolations to other metropolitan areas, it is worth knowing that the Chicago region has long been engaged in open space preservation and ecological restoration that may not be as prevalent elsewhere and is among the many local circumstances that should be borne in mind when making broad generalizations across land cover categories (Heneghan et al., 2012; Crane et al., 2014; Watkins et al., 2015). Therefore, we consider these extrapolation results as useful in goal-setting and as aspirational particularly for our exemplary results. This approach relies on an areas-of-overlap matrix between our 16 consolidated land use classes and the 35 land cover classes used in the USGS habitat raster (see Supplementary Section 4 for detailed methods). We applied this matrix to our baseline milkweed densities (calculated on a total land use basis) to translate our densities into coefficients for use with the USGS habitat raster. This process was then repeated for both enhanced and exemplary densities. For our Open space conservation and non-conservation baseline densities, we used the CRP-NW values from Thogmartin et al. (2017b, Supplement), which is further explained in the discussion.
After calculating all of the USGS habitat coefficients for baseline, enhanced, and exemplary densities, we summarized the total acres for the 35 USGS habitat land cover classes within all urbanized areas across the US eastern range. We then multiplied each land use coefficient by the acreage of each land use class and totaled the number of estimated stems for the baseline. The process for enhanced and exemplary estimates used the following formula:
Where the estimated total number of additional milkweed stems (S) for a given land use class, is equal to the total land use area (L) for a given class, multiplied by the adoption rate (AR)—this gives us the proportion of land that is being converted—times the difference between the enhanced or exemplary density (E) minus the baseline density (B). Note that enhanced and exemplary are calculated separately, and the total estimate of additional stems is the sum of estimated stems across all land use classes.
Results
Plantable Space
Across the four metropolitan areas, we found that about half of all plantable space is found in agricultural areas. The proportion was lower in Austin because our study area was limited to the central county in the 5-county area. After Agricultural land, Residential-single family was the second largest land use category by area for three of the four metropolitan areas, and the third largest for Minneapolis-St. Paul (Figure 6). In Minneapolis-St. Paul, Vacant lots made up the second largest land use category because it included land with buildings, whereas in other metropolitan areas Vacant lots did not include land with buildings. Other land use classes making up significant portions within metropolitan areas included Open space conservation, Rights-of-way categories, and Vacant lots (without buildings).
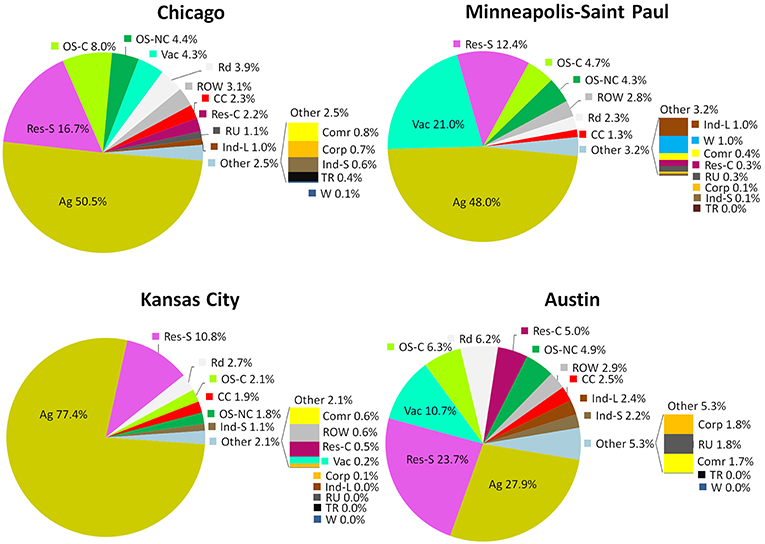
Figure 6. Proportion of grass/shrub land cover (“plantable space”) categorized by land use category for each metropolitan area. Agriculture and Residential-single family make up the highest and second-highest proportions in almost all of the metropolitan areas. Austin was limited to one county.
Milkweed Densities
Chicago Baseline Milkweed Density Estimates
Metro-transect sampling methods were effective at capturing milkweed densities across major metropolitan areas despite the low detectability of milkweed across such a vast area. Of the 54 random sampling clusters visited in Kansas City, 25 contained milkweed; in Austin, 50 of the 66 clusters visited contained milkweed; and in Chicago, 53 of the 66 visited clusters contained milkweed. In Chicago, Residential-common space and multi-family (Res-C) had the highest stem density (weighted mean = 18.9 stems/acre), followed by Minor road rights-of-way (Rd, 7.4), Restricted use rights-of-way (RU, 5.4), Vacant lots (Vac, 4.8), and Industrial-small (Ind-S, 3.9) (Figure 7). Due to variability in milkweed densities among Res-C and Ind-S sites, these land use classes had large standard errors. It is also worth mentioning that Residential-single family (Res-S, 1.4), while lower than many land use classes, carries a lot of influence on the final milkweed stem counts due to the sizable extent occupied by residential properties in metropolitan areas. Several of the remaining land use classes were either not observed within our randomized sampling clusters due to their scarcity across the landscape, or milkweed was rarely or never observed. These classes include Commercial (Comr), Industrial-large (Ind-L), Transitional and restricted use (TR), and Water (W). Consequently, these land use categories have an existing milkweed stem density that is approximated as zero stems per acre. Milkweed density values for all land use classes from both metro-transect sampling and natural area sampling are combined in Table 2. This table is also available with calculations on a total land use basis rather than plantable space in Supplementary Table 3. To examine whether there is a difference in milkweed densities in more densely vs. less densely populated parts of the metropolitan area, we compared milkweed densities in two population density size classes. These results are in Supplementary Section 5.
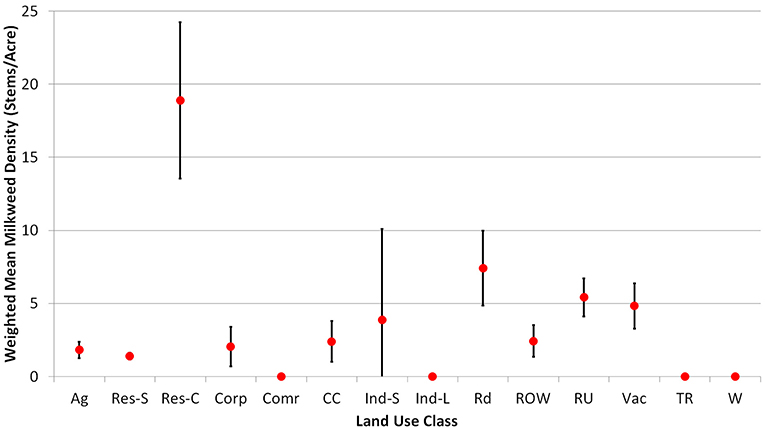
Figure 7. Chicago baseline weighted mean milkweed densities (calculated on a grass/shrub and 20% tree basis) for the metro-transect sampling results. Standard error around the mean are indicated by lines. Metro-transect sampling was used for all land use classes except for open space which used density values provided by Thogmartin et al. (2017b).
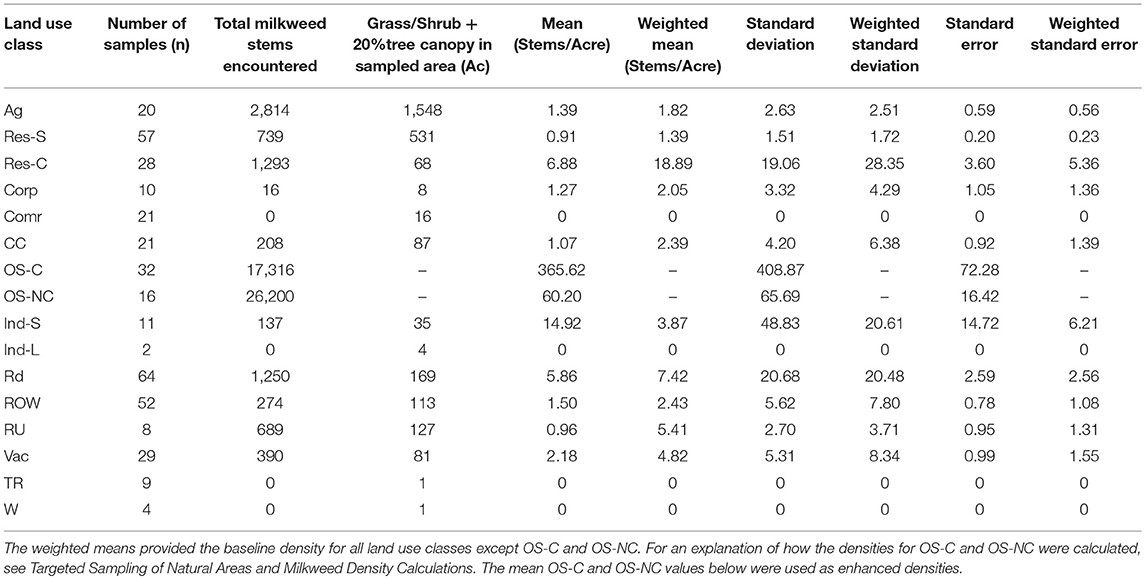
Table 2. Chicago milkweed density survey results by land use class calculated on a “plantable space” basis, which leverages high-resolution land cover.
Enhanced and Exemplary Sites in Chicago
Through targeted sampling, we were able to capture enhanced and exemplary milkweed densities for most land use classes. In Figure 8, the highest enhanced density average was found in the OS-C land uses class (365.6 stems/acre). The second highest enhanced density average was 195.4 stems/acre for Ind-S based on three highly variable site densities ranging from 2.0 to 321.7 stems/acre. For exemplary sites, the highest density average was found in OS-C at 4,109 stems/acre, followed by Res-S (330.4 stems/acre) and OS-NC (304 stems/acre). In the Res-S category, we sampled 50 sites, where the lowest density was 1.3 stems/acre and the top five densities (ten percent of samples) ranged from 225 to 419.5.
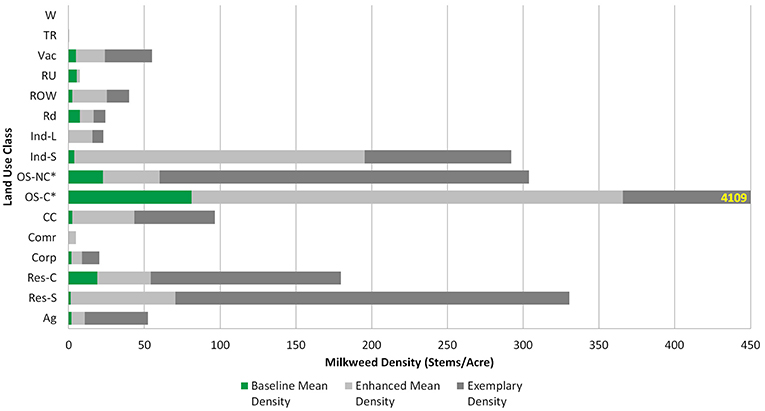
Figure 8. Chicago average milkweed stem densities by consolidated land use categories are shown for existing “baseline” densities (green bars), “enhanced” densities (light gray), and “exemplary” densities (dark gray). *For open space conservation and non-conservation, the baseline density values were provided by Thogmartin et al. (2017b). The mean exemplary density for open space conservation extends well beyond the plotted space.
Comparing Baseline Densities Across Metropolitan Areas
Using metro-transects, we evaluated milkweed densities randomly in Chicago, Kansas City, and Austin (Figure 9). In general, average milkweed density values were low in both Res-S (1.4 in Chicago, 0.01 in Kansas City, and 0.6 in Austin) and Res-C (0.0 in Kansas City and 1.8 in Austin). However, in Chicago, there was a high density in Res-C (18.9) and in Minneapolis-St. Paul, the Res-S density was 30.0 stems per acre. This is over 20 times higher than the other metropolitan areas studied. While this density may be associated with the different sampling protocol used in Minneapolis-St. Paul that targeted neighborhood blocks inside the city limits, results from our social science survey also found that Minneapolis-St. Paul had higher milkweed stem counts. Also notable are elevated baseline densities in Ind-S (in Chicago and Kansas City), Rd (especially in Chicago), and Vac (in Chicago and Kansas City).
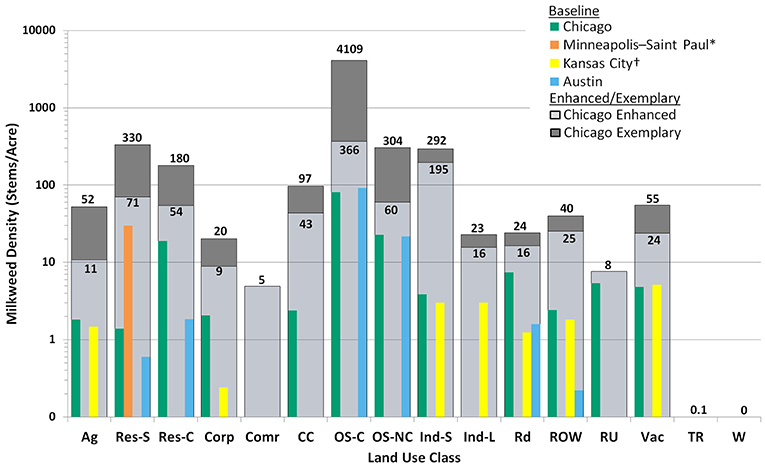
Figure 9. Average milkweed stem densities by consolidated land use categories for existing conditions in Chicago (green), Minneapolis-St. Paul (orange), Kansas City (yellow), Austin (blue). *Baseline densities for Minneapolis-St. Paul were only collected for Res-S, so Chicago baseline values were used for all other land use classes. †Baseline densities for Kansas City were not collected for OS-C and OS-NC, so Chicago baseline values were used for these land use classes. Densities from Chicago are also shown for enhanced sites (light-gray bars), and exemplary conditions (dark-gray bars). All densities shown are on a plantable space basis. Density values are displayed in log space to fit all values.
When we applied national density values for Open space land use classes as described above, areas such as nature preserves and city parks contributed the greatest number of milkweed stems compared with all other land use classes. We used national milkweed densities (for Open space in Chicago, Minneapolis-St. Paul, and Kansas City) and metro-transect milkweed densities (for all others) to run our baseline calculator for the four metropolitan areas. The resulting maps show areas of high to low densities (Figure 10). The corresponding total estimated stem counts by land use are shown in Table 3.
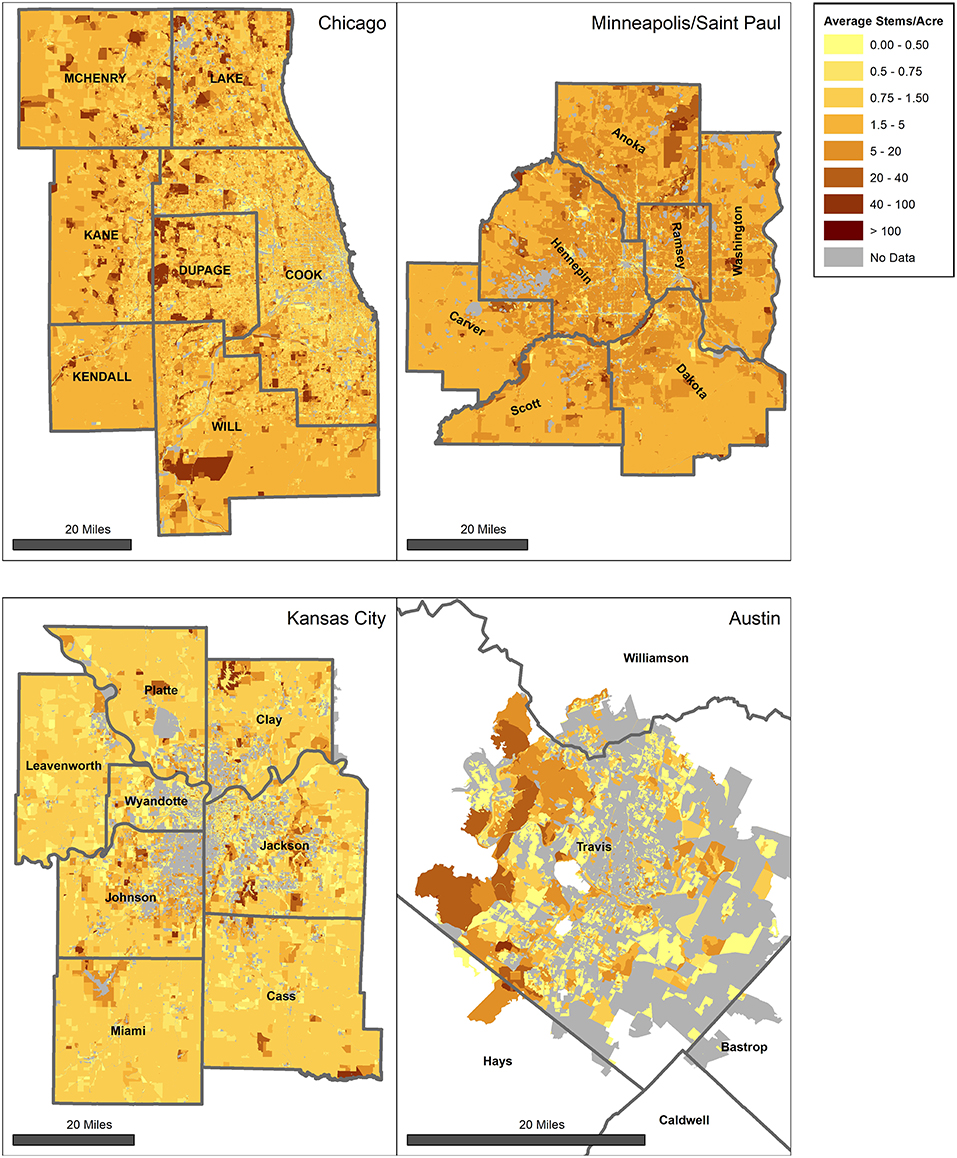
Figure 10. Baseline average milkweed densities by US census blocks for all four metropolitan areas. Darker areas indicate a higher milkweed density than lighter areas. The highest observed densities for all four metropolitan areas were in natural areas. These natural areas are more present and evenly dispersed within the 7 counties that make up Chicago's metropolitan area. Limitations in available land use data and sampling resulted in areas within the analysis extent where there was no calculated milkweed densities, shown in gray.
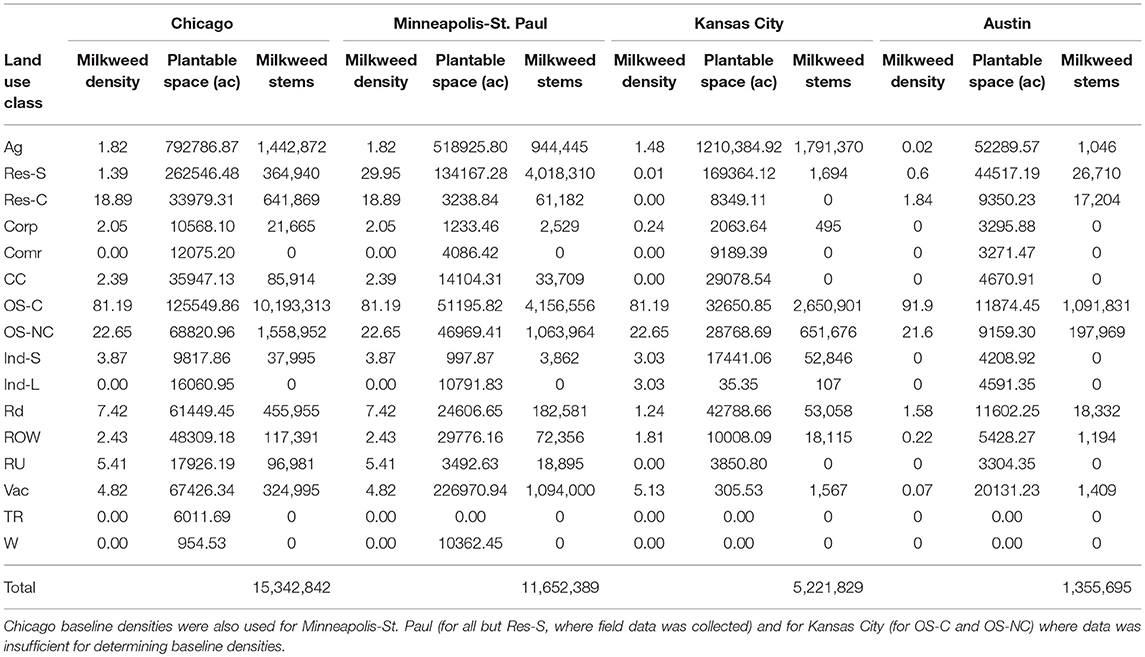
Table 3. Baseline milkweed densities (stems/acre) used as input for the Urban Milkweed Baseline Tool for each metropolitan area and the resulting outputs including acres of plantable space and estimated count of current milkweed stems.
Results from our baseline geospatial tool estimate that Chicago has 15.3 million stems of existing milkweed. Approximately 66% of these stems are from OS-C areas, followed by 10% from OS-NC areas. Our findings in other cities were similar, in that OS-C dominated all other land use classes (36% in Minneapolis-St. Paul, 51% in Kansas City, and 81% in Austin). These were followed by Res-S in Minneapolis-St. Paul (35%), Agriculture in Kansas City (34%), and OS-NC in Austin (15%). In Minneapolis-St. Paul, we estimate a baseline of 11.7 million stems, in Kansas City we estimate 5.2 million, and in Austin we estimate 1.3 million stems. As noted above, the geographic extent of Austin only reflects the central county (Travis) or this estimate would be much higher. These data should also be interpreted with some caution since we draw from national densities for the Open space baseline density estimates in Chicago, Kansas City and Minneapolis-St. Paul.
Municipal Case Studies
When looking at our results from sampling at the municipal-scale in Glenview and Schaumburg, it is helpful to compare these localized milkweed densities with our Chicago regional densities. We found that the density for some land use classes were higher, while others were similar or lower (see Supplementary Section 3 for more details). Average density values for Glenview differed from the greater Chicago region especially for Community and cultural (CC, +9.1 stems/ acre), Major rights-of-way and landfill (ROW, +5.5 stems/acre), and Res-S (+0.7). In Schaumburg the higher density values were much less pronounced; ROW was slightly greater (+0.4), as was Corporate and medical (Corp, +0.3), and Res-S (+0.3).
Social Science Research Results
The social science research covered a wide range of topics relevant to monarch conservation, including both open-ended and closed-ended questions, and it generated both qualitative and quantitative data. Of the most relevance here are the quantitative online survey results having to do with how many people already are growing milkweed, how much milkweed is on their property, and how much more they would be willing to add in the future. Of the 734 people surveyed in the four pilot metropolitan areas, 226 indicated that they “manage plantable open space on one or multiple sites” (most, but not all, were residential sites, i.e., home gardens). Of those 226 respondents, 184 or 81% answered that they had milkweed growing at their site. Of the 184 with milkweed, almost half had 1–10 milkweed plants at their site and a little over a third had 11–50 milkweed plants. Just 17% said that all or almost all of their site's plantable space was already taken up by native plants, i.e., many indicated that there was more plantable space at their site. When asked what they would be willing to plant in the next five years, 63% said they would be willing to convert more of the plantable space at their site to native milkweed. These additional data, while not representative of the general population, did function to provide a check on the baseline and scenario estimates the geospatial team generated.
Scenario-Modeling Results by Metro Area
After running the baseline tool, the total estimated number of milkweed stems for each metropolitan area ranged from 1.3 million in Austin to 15.3 million in Chicago (Table 3). With a two percent adoption rate across all sectors, at enhanced densities, Chicago would add an estimated 1.4 million stems to the 15.3 million current stems for a total of about 16.7 million stems (Supplementary Table 4). We also applied a five and ten percent adoption rate across all land use classes for Chicago, based on enhanced densities, which result in an estimated 3.6 million additional stems and 7.2 million additional stems, respectively. When a two percent adoption rate across all sectors is applied at exemplary densities, Chicago would add an estimated 13.4 million stems to the 15.3 million current stems for a total of 28.7 million stems (Table 4).
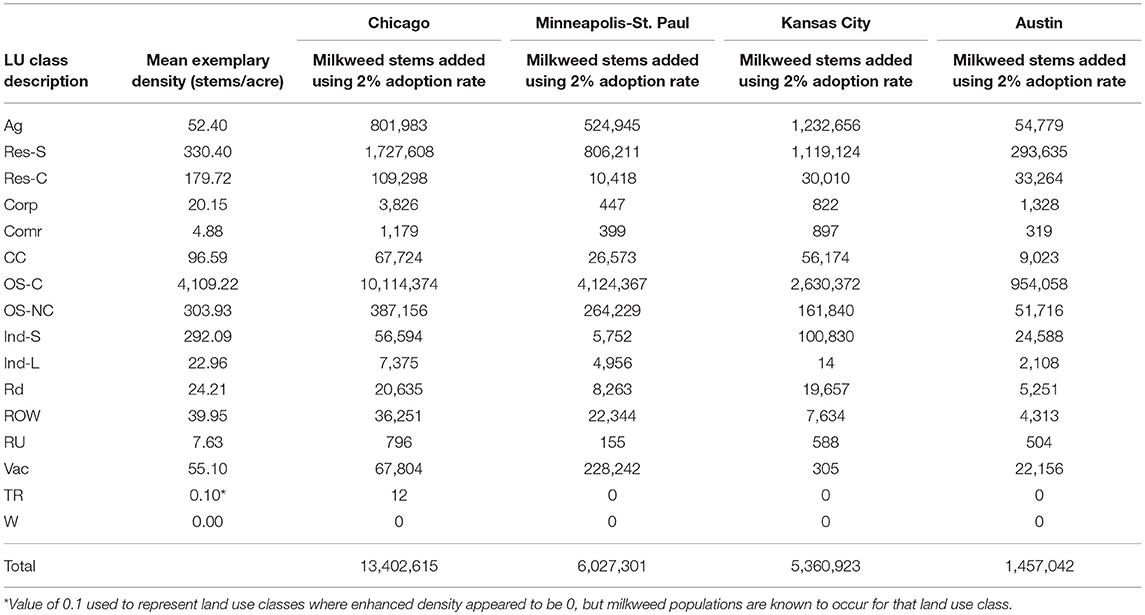
Table 4. Estimated number of milkweed stems that would be added to the baseline density for each metropolitan area based on using the Scenario-Based Planning Tool with Chicago's exemplary milkweed density and a two percent adoption rate.
Estimates for Baseline and Projected Stem Counts When Extrapolating Across the Eastern Range of the Monarch Butterfly
Bearing in mind the previously identified assumptions, when extrapolating across urbanized areas, we estimate that 312 million stems currently exist on the landscape in the North Central and Northeast monarch regions as delineated by the USGS (Supplementary Figure 1) utilized by Oberhauser et al. (2017, Figure 1). We also estimate that an additional 29.8 million stems could be added in this area with modest effort, based on a two percent adoption rate using our Chicago enhanced milkweed densities. Our more ambitious estimate, based on exemplary densities, adds 271 million stems of milkweed in urbanized areas for this same geography at a two percent adoption rate. It is important to note that common milkweed (Asclepias syriaca), which comprises approximately 74% of milkweed occurrence along our metro-transect lines (Supplementary Section 6), has a species distribution that roughly matches the USGS North Central and Northeast monarch regions (see Lemoine, 2015). We therefore have more confidence in extrapolating across this region, since the species distribution and ecotype have a greater affinity to the Chicago region. However, for regional comparison purposes, if we extrapolate across the USGS North Central, Northeast, and South monarch regions, a total of 56.1 million stems may be added under an enhanced scenario, and 517 million stems may be added under an exemplary scenario. For the entire region east of the Rockies, the total contribution reaches 31%, or nearly one third of the national goal. For all of the above estimates, we use baseline densities from the literature for Open space conservation and non-conservation land use classes, and Chicago densities for all other land use classes.
Discussion
This research is the first in-depth examination into understanding the role that metropolitan areas play in supporting monarch butterflies, and what their capacity may be for supporting breeding populations. Our three main goals of this study were to (1) estimate the current and potential contribution that metropolitan areas provide for monarch butterfly habitat, (2) provide geospatial planning tools to support the development of conservation strategies, and (3) estimate the potential for adding milkweed within urbanized areas across the monarch butterfly's eastern range. Our study offers several key findings and outcomes about metropolitan areas and monarch conservation.
One key finding is that a large presence of milkweed occurs across most land use classes in metropolitan areas, especially in Open space conservation, Open space non-conservation, Residential-single family, Residential-common space and multi-family, Vacant lots, and the Rights-of-way land use classes. In the metropolitan areas where we sampled both open space and residential areas, we found that these land use categories had the highest potential for increasing the number of estimated milkweed stems. Targeting people and organizations in these classes may result in some of the best opportunities for focused engagement strategies aimed at bolstering additional habitat. However, excellent large-scale opportunities may also exist across other land use classes particularly where cumulative land areas have few or a single owner such as rights-of-way and vacant lots owned by a city or municipality.
Another important outcome from our research was the development of geospatial tools that allow landowners and planners to evaluate the potential for adding pollinator habitat to large land areas and to measure the success of planting efforts by comparing baseline estimates over time. These tools are also useful for evaluating the co-benefits that exist when combining green infrastructure improvements with conservation planning for monarchs. Our tools are dependent on the accuracy of field sampling results. We found that sampling milkweed across vast metropolitan areas is difficult, but possible. Our metro-transect sampling method was successful in detecting milkweed across the diverse mix and patterns of land use classes, even at low densities. Sampling across open space natural areas was challenging but was successful in locating milkweed stems through targeted sampling. These sampling methods were effective for understanding more about the density of milkweed where it occurs across the landscape, but further study is needed for establishing more precise metropolitan-scale baseline density estimates particularly for Open space conservation and non-conservation land use classes in urban areas (see Methodological Considerations below).
Our geospatial extrapolations indicate that if all urbanized areas in northern and northeastern US were engaged, it may be possible to add an estimated 271 million stems of milkweed, or over 15% of the projected milkweed stems needed to rebound the eastern monarch population. This projection increases to 31% if we extend this extrapolation across all urbanized areas in the entire US eastern range. These findings suggest that urbanized areas should figure prominently in monarch conservation planning (see Implications for Monarch Conservation below).
Implications for Monarch Conservation
Although agricultural land had the largest amount of plantable space in all four metropolitan areas, the majority of this land is not available as plantable space for milkweed stems. Agricultural land is primarily found in the outer fringes of the metropolitan areas studied. Most of this land is composed of intensively farmed row crops (cereals and soybeans primarily). The loss of milkweeds stems from this habitat resulting from GMO crops followed by extensive herbicide use is a big part of the monarchs' plight (Brower et al., 2012; Pleasants et al., 2017). Community gardens and other small-scale agriculture in the urban and suburban core are treated as part of the land use category in which they are imbedded, rather than in the agricultural land use class. After Agriculture, Residential-single family land use made up the second largest amount of plantable space in three of the four metropolitan areas.
While existing milkweed densities were typically low (<2 stems/acre) in Residential-single family land use, because this land use category is so massive in total size, low densities can still have a big contribution. Our results likely underestimate the density of milkweed in this land use, because we were unable to survey in backyards. Results also show that densities within city limits can be 20–30 fold higher, as was observed in Minneapolis-St. Paul. Residential-single family also had the third highest enhanced density and the second highest exemplary density (Figure 8). Further, results from our online surveys of the interested public suggest over 60% of people surveyed in residential areas would be willing to plant more milkweed in the next 5 years. With the large amount of plantable space, pronounced interest of residents, and high measured densities at enhanced and exemplary sites, Residential-single family areas have the potential to add a considerable number of stems even at a two or five percent adoption rate.
In achieving a two or five percent adoption rate, the highly fractured ownership of residential land poses both a challenge and an opportunity. While the acreage-to-landowner ratio is generally low, the high level of enthusiasm and capacity among monarch-friendly gardeners (Derby Lewis et al., 2018) makes the task of converting additional residential land to monarch habitat less daunting and demonstrates real conversion potential in one of the largest land use classes in metropolitan and urbanized areas. While working with a greater number of landowners, each with a relatively small amount of land, may intuitively seem like an inefficient approach, our social science research shows this is not necessarily the case. The higher population density in cities and their surroundings tends to bring people in contact with one another, encouraging the adoption and spread of new practices. One's neighbors (and their gardens) are often more visible, and organizations can achieve a critical mass of involvement to move conservation initiatives forward.
Within the public sector, agencies own or manage a high proportion of land in rights-of-way, open space, and vacant lots and have the potential to improve and convert large areas through management and policy changes (Anderson and Minor, 2017). We estimate, for example, that right-of-way areas, which occupy 128,000 acres of plantable space in the Chicago region, could add approximately 577,000 stems by converting 20% of the green space to habitat at exemplary levels in the Chicago region alone. Similarly, converting 20% of vacant lots, which occupy over 67,000 acres of plantable space in the Chicago region, could add approximately 678,000 stems. By default, our projections use a two percent adoption rate. However, a higher adoption rate may be reasonable for vacant lots, rights-of-way, and open space natural areas, since existing green space on these lands can more often be augmented with habitat without changing how the land is currently utilized. In addition, public land owners can couple management practices and funding opportunities, such as stormwater and green infrastructure improvement programs and grants, with pollinator improvements to further enhance milkweed populations.
OS-C and OS-NC are another example of publicly owned land with a relatively low landowner-to-acre ratio. Stem densities for OS-C and OS-NC in metropolitan areas where our teams sampled (Chicago and Austin) were much higher when compared with other land use classes. Much of this is due to our use of targeted sampling, however our results suggest that milkweed can be present at very high densities in these land use classes. These high densities coupled with the large amount of plantable space means these areas can be important opportunities for adding stems even with relatively low adoption rates.
While OS-C and OS-NC provided some of our highest sampled milkweed densities, some land use classes in metropolitan areas appear to have low milkweed densities and lower potential for adoption, based on current conditions. Of our 16 consolidated land use classes, several had no milkweed occurrence at all. Some of these classes were scarce in our study areas (e.g., Transitional and restricted use, Industrial-large, and land classified as Water). Commercial land, on the other hand, was well represented in our sampling, but had zero random milkweed occurrences. In fact, we actively tried to locate commercial sites with milkweed during our targeted sampling of enhanced sites and were only able to find one site in Chicago. Future research could assess the potential for increasing commercial adoption rates with different engagement practices.
Methodological Considerations
Our findings, comparisons, and extrapolations rely more heavily on Chicago data, as it was more complete and consistent. Our local knowledge of Chicago and that of our partner organizations allowed us to conduct “targeted” sampling of known milkweed locations. This was an important part of the effort to determine the typical milkweed densities where milkweed occurs, which was key to estimating the potential capacity to add habitat in metropolitan areas.
Fieldwork across the four metropolitan areas highlights the difficulty of sampling the urban environment. Urban areas are highly heterogeneous landscapes, and there is a complex matrix of landowners and policies. Nearly all of our land use field data, other than the Open space categories, comes from randomized sampling of what is visible from the public right-of-way (i.e., roads and sidewalks) except when our team was explicitly invited onto private land (e.g., targeted sampling on enhanced sites). In contrast, for OS-C and OS-NC, our field teams obtained permits and conferred with land managers to conduct more intensive sampling of known milkweed sites. This was necessary because little was known about milkweed densities in urban natural areas. Both OS-C and OS-NC are comprised of many plant communities, including large areas devoid of milkweed. However, we knew these land use classes would be important in determining densities where milkweed is found (enhanced sites) and their upper threshold (exemplary sites). Using targeted sampling ensured we would collect data on these important land use categories.
For Open space baseline densities, we used values taken from the supplement of Thogmartin et al. (2017b) as it is the only source estimating milkweed densities across a wide geographic extent and across a full range of land use types. Because our land use classes and the land cover classes used in their supplement are not the same, we chose classes that were the best representation of the management approaches within OS-C and OS-NC. For OS-C, we selected CRP-NW, with a value of 112.14 stems/acre, as the best match. Although CRP land is based on a very different program than are lands like state parks, national wildlife refuges, forest preserves and other protected lands with a primary conservation goal, they share a management goal of biodiversity conservation. Since this value was provided on a land use basis, we applied our estimated proportion of the amount of OS-C land that is non-cultural plantable space (72.4%) to this amount, resulting in a baseline of 81.19 stems/acre. We also applied the CRP-NW value of 112.14 stems/acre to the proportion of OS-NC land that is non-cultural plantable space (20.2%), resulting in a baseline of 22.65 stems/acre. This category may not appear to be the best match and possibly overestimates the baseline density for OS-NC; an alternative option would be to use the Protected Grasslands category, however, in applying this value (3.09) to the proportion of natural areas in OS-NC (20.2%), the result is a density of 0.62 stems per acre, lower than nearly all measured land use densities in Chicago, which we believe would greatly under-represent the presence of milkweed in this land use class.
We expect that the actual milkweed density present across all Open space conservation and Open space non-conservation land is somewhere between the estimate provided by Thogmartin et al. (2017b) and the densities observed in the targeted sampling areas. More research is needed to better approximate a baseline density of milkweed stems in this land use category. In addition, another of our biggest challenges was determining how much of the plantable space within land in OS-C and OS-NC is actually plantable space as opposed to land set aside for recreation or other uses. As a future project we would like to better delineate those lands.
Our methods may need to be adapted for other metropolitan areas, towns, and municipalities that wish to utilize our geospatial tools, or to replicate this study. In particular, we leverage high-resolution land cover data to estimate stem densities on a plantable space basis. Using estimations on a total land use basis is an acceptable alternative when these data are not available. We also recognize that when extrapolating from our findings, the milkweed species and growing conditions present in Chicago differ from other parts of the country. For example, common milkweed (Asclepias syriaca) is found throughout the north and northeast but is not common in the south.
Due to qualitative differences in the data available and in the methodological approach, inferences are challenging; however, some clear distinctions are apparent. Minneapolis-St. Paul, for example, achieved a much higher residential milkweed density as compared to its peers, and in Schaumburg, where they are actively engaging residents through community planting programs, the milkweed density was moderately higher as compared to the citywide density. In Glenview where they have a 10-year history of pollinator friendly institutional projects, there was a large boost over the regional density. These are encouraging results that may be indicators of effective social and institutional engagement; however, we also caution that it is difficult to tease apart cultural and environmental factors that may be at play.
Lastly, it's important to note that our study only looked at the current and potential contributions of milkweed stems in metropolitan areas, and not at actual monarch productivity. While our approach in Chicago reveals both a higher baseline where milkweed was found and a higher potential for milkweed stems in metropolitan areas than previously reported, it is not known whether larval survivorship is similar to what has been observed in other sectors such as rights-of-way or agriculture. With such high public awareness and interest in creating pollinator habitat in metropolitan areas, it is important to have a better understanding of what influences monarch productivity (e.g., patch size, milkweed density, floral diversity, distance to green space, etc.) (Nail et al., 2015) in this landscape in order to inform best planting practices for a variety of stakeholder groups who we see clamoring for this information.
Recommendations and Next Steps
Although urban areas cover only three percent of land in the United States, they are home to 80% of the country's population18. We show that there is more milkweed on the ground in metropolitan areas than previously published by Thogmartin et al. (2017b) (0.1–1.0 milkweed stems/acre in developed areas). If there is a higher baseline density of milkweed in urban areas than was previously thought, this could mean that the existing goal of 1.8 billion stems is not adequate to boost overwintering populations to sustainable levels. However, much of our data is drawn from the Midwest and all of the cities in our study had populations in excess of one million people. More study of small to mid-size cities and those outside of the Midwest is needed before conclusions can be drawn. Our findings clearly indicate that the urban sector can make important contributions to monarch recovery and that the diverse landscape of urban areas requires careful attention to both ecological and social differences across land use classes and the engagement strategies employed for getting additional habitat on the ground. This includes establishing planning objectives that prioritize appropriate engagement strategies for key decision makers to harness social momentum for milkweed adoption. Some of the biggest potential occurs in Residential land use classes, which require successful engagement of residents (Derby Lewis et al., 2018). Researchers from the US Geological Survey and collaborating institutions have called for an “all hands on deck” approach across all sectors, including urban and suburban areas (Thogmartin et al., 2017b). We suggest a targeting and engagement approach in metropolitan and urbanized areas to complement the “all hands on deck” strategy for fulfilling the goals of increasing planted milkweed by 1.8 billion stems to support monarch butterflies (Thogmartin et al., 2017a).
Our Urban Monarch Conservation Planning Tools are designed to help municipal decision-makers and planners estimate their capacity to add stems across the metropolitan landscape by identifying where the biggest opportunities exist and what the best practices are to engage different stakeholder groups. Our tools can be applied to a land use class, for example, estimating how many stems could be added if five percent of homeowners adopted our enhanced site numbers; and they can be used spatially to estimate the potential of particular neighborhoods, municipalities, or parts of a city. One active area of research has been applying our tools to estimate the potential addition of milkweed stems within areas set aside for stormwater management, thus capturing the co-benefits of increased habitat along with water infiltration.
While our research has largely been successful at accomplishing our objectives, we acknowledge that further study is needed. Our research is based on metropolitan areas ranging in size from 1.3 million people in Austin to 9.7 million in Chicago. We recommend further study into small and medium-sized metropolitan areas across Middle America to understand differences and similarities to these findings, and to test the replicability of our methods. Smaller cities and towns may show different social and ecological trends, which could impact both regional assumptions and social engagement strategies. Studies into how social networks affect the transfer and spread of information resulting in on-the-ground habitat are also critical to driving future restoration efforts at larger scales. Also, due to the urgency needed to support monarch butterflies with on-the-ground resources, we are aware that we are addressing an ecological problem with an “engineering solution” focused on getting milkweed stems into the ground vs. what would be a more nuanced approach of producing healthy diversified ecosystems that can support pollinator networks capable of resisting disturbances such as localized effects of climate change. We recommend research into several topics that would increase our understanding of how monarchs and other pollinators perform under different environmental conditions such as patch size, habitat diversity needs and efficacy at producing monarchs, connectivity to other patches and resources, and the effect all these factors may have on monarch butterfly fecundity and predation (especially at the egg and larval stages).
Ethics Statement
This study was carried out in accordance with the recommendations of The Field Museum Institutional Review Board Policy and Procedures. The IRB granted the team a waiver of written informed consent based on the fact that participation in the study presented very minimal risk of harm to subjects. Subjects were informed of study confidentiality procedures and told how their answers would be used before they took part in an interview or survey. The protocol was approved by The Field Museum Institutional Review Board.
Author Contributions
MJ: primary contributing author, geospatial team manager, primary on research and tool design. AH: contributed content, editing, research advice, GIS analysis and field coordination. KK: contributed GIS analysis, statistics, content, and editing. ML: GIS analysis and research advice. AD: contributed content, editing, research advice and project management. DS: contributed research advice, editing, and content. AW: contributed social research design, analysis, and team coordination, content, and graphic design. MB: contributed content, editing, and research advice. IR: contributed editing and research advice.
Funding
This research was funded by a US FWS Cooperative Agreement No. F11AC01062 awarded for the period September 1, 2015 to May 31, 2017; and US FWS Cooperative Agreement No. F17AC01018 awarded for the period October 1, 2017 to September 30, 2018.
Conflict of Interest Statement
The authors declare that the research was conducted in the absence of any commercial or financial relationships that could be construed as a potential conflict of interest.
Acknowledgments
Funding for this project came from the US Fish and Wildlife Service. We would like to thank the deeply committed and creative team of individuals whose work is reflected in this study, including Tim Bodeen, Katie Boyer, Brittany Buckles, Wendy Caldwell, Louise Clemency, Caitlin Dix, Martha Dooley, Ryan Drum, Jill Erickson, Adriana Fernandez, Robyn Flakne, Nigel Golden, Jessica Hellman, Kyle Kasten, Susan Lenz, Laura Lukens, Cora Lund-Preston, Patrick Martin, Katie Maxwell, Tom Melius, Kelley Myers, Rosie Nguyen, Karen Oberhauser, Zach Paolillo, John Rogner, Jason Rowader, Glen Salmon, Kristin Shaw, Wayne Thogmartin, Michelle Thompson, Chuck Traxler, Kristin Voorhies, Gwen White, and Barbara Willy. We also thank our reviewers and Amy Rosenthal, Ellen Woodward, Katherine Moore Powell, and Nigel Pitman for their review and comments on this paper.
Supplementary Material
The Supplementary Material for this article can be found online at: https://www.frontiersin.org/articles/10.3389/fevo.2019.00210/full#supplementary-material
Footnotes
1. ^Assessing the status of the monarch butterfly https://www.fws.gov/savethemonarch/SSA.html
2. ^2018 Monarch Conservation Implementation Plan https://monarchjoint venture.org/images/uploads/documents/2018_Monarch_Conservation_Implementation_Plan_FINAL_1.pdf
3. ^The Monarch Conservation Database https://www.fws.gov/savethemonarch/mcd.html
4. ^As deployed by the US Census Bureau using the 2010 OMB definitions found in the June 28, 2010 Federal Register; https://www.census.gov/programs-surveys/metro-micro.html
5. ^e.g., Protected Areas Database of the US (PADUS) https://gapanalysis.usgs.gov/padus
6. ^A detailed methodology for how this raster was developed can be obtained by downloading the user manual for the desktop Monarch Conservation Tools: http://www.umesc.usgs.gov/management/dss/monarch/desktop_monarch_conservation_planning_tools.html
7. ^http://www.fieldmuseum.org/monarchs
8. ^https://assets.chicagoparkdistrict.com/s3fs-public/documents/departments/budget/2019%20Budget%20Summary.pdf
9. ^http://fpdcc.com/downloads/plans/FPCC-Natural-Cultural-Resources-Master-Plan_3-9-15_WEB.pdf.
10. ^https://www.nwf.org/Garden-For-Wildlife/About/National-Initiatives/Mayors-Monarch-Pledge.aspx
11. ^http://climatechicago.fieldmuseum.org/pilsen
12. ^http://www.fieldmuseum.org/monarchs
13. ^https://www.umesc.usgs.gov/management/dss/monarch/desktop_monarch_conservation_planning_tools.html
14. ^www.fieldmuseum.org/monarchs
15. ^Substituting space for time is a common practice when studying ecological systems with a component of time [e.g., climate change (Blois et al., 2013), and ecological forecasting (Banet and Trexler, 2013)].
16. ^https://www.census.gov/geo/reference/urban-rural.html
17. ^https://www.umesc.usgs.gov/management/dss/monarch/desktop_monarch_conservation_planning_tools.html
18. ^United States Census Bureau 2010 https://www2.census.gov/library/publications/decennial/2010/cph-2/cph-2-1.pdf
References
Anderson, E. C., and Minor, E. S. (2017). Vacant lots: an underexplored resource for ecological and social benefits in cities. Urban Forest. Urban Green. 21, 146–152. doi: 10.1016/j.ufug.2016.11.015
Banet, A. I., and Trexler, J. C. (2013). Space-for-time substitution works in everglades ecological forecasting models. PLoS ONE 8:e81025. doi: 10.1371/journal.pone.0081025
Blois, J. L., Williams, J. W., Fitzpatrick, M. C., Jackson, S. T., and Ferrier, S. (2013). Space can substitute for time in predicting climate-change effects on biodiversity. Proc. Natl. Acad. Sci. U.S.A. 110, 9374–9379. doi: 10.1073/pnas.1220228110
Brower, L. P., Taylor, O. R., Williams, E. H., Slayback, D. A., Zubieta, R. R., and Ramírez, M. I. (2012). Decline of monarch butterflies overwintering in Mexico: Is the migratory phenomenon at risk? Decline of monarch butterflies in Mexico. Insect Conserv. Divers. 5, 95–100. doi: 10.1111/j.1752-4598.2011.00142.x
Cariveau, A. B., Holt, H. L., Ward, J. P., Lukens, L., Kasten, K., Thieme, J., et al. (2019). The integrated monarch monitoring program: from design to implementation. Front. Ecol. Evol. 7:167. doi: 10.3389/fevo.2019.00167
Chaplin, S. J., and Walker, J. L. (1982). Energetic constraints and adaptive significance of the floral display of a forest milkweed. Ecology 63, 1857–1870. doi: 10.2307/1940126
Crane, P., Heneghan, L., Muraski-Stotz, F., Pruett-Jones, M., Ross, L., Wali, A., et al. (2014). “Chicago wilderness: integrating biological and social diversity in the urban garden,” in The Social Lives of Forests, eds S. B. Hecht, K. D. Morrison, and C. Padoch (Chicago, IL: University of Chicago Press), 362–368. doi: 10.7208/chicago/9780226024134.003.0033
Cutting, B. T., and Tallamy, D. W. (2015). An evaluation of butterfly gardens for restoring habitat for the monarch butterfly (Lepidoptera: Danaidae). Environ. Entomol. 44, 1328–1335. doi: 10.1093/ee/nvv111
Derby Lewis, A., Winter, A., Czarnecki, C., and Bouman, M. (2018). “Connecting nature and society to increase conservation relevance: a case study of the monarch butterfly in urban areas,” in Wildlife Management Institute, Transactions of the 82nd North American Wildlife and Natural Resources Conference (Spokane, WA).
Dyer, L. A., and Forister, M. L. (2016). Wherefore and whither the modeler: understanding the population dynamics of monarchs will require integrative and quantitative techniques. Ann. Entomol. Soc. Am. 109, 172–175. doi: 10.1093/aesa/sav160
Flockhart, D. T., Wassanaar, L. I., Martin, T. G., Hobson, K. A., Wunder, M. B., and Norris, D. R. (2013). Tracking multi-generational colonization of the breeding grounds by monarch butterflies in eastern North America. Proc. R. Soc. B Biol. Sci. 280:20131087. doi: 10.1098/rspb.2013.1087
Heneghan, L., Mulvaney, C., Ross, K., Umek, L., Watkins, C., Westphal, L. M., et al. (2012). Lessons learned from Chicago Wilderness—Implementing and sustaining conservation management in an urban setting. Diversity 4, 74–93. doi: 10.3390/d4010074
Kasten, K., Stenoien, C., Caldwell, W., and Oberhauser, K. S. (2016). Can roadside habitat lead monarchs on a road to recovery. J. Insect. Conserv. 20, 1047–1057. doi: 10.1007/s10841-016-9938-y
Kountoupes, D. L., and Oberhauser, K. S. (2008). Citizen science and youth audiences: educational outcomes of the monarch larva monitoring program. J. Commun. Engagem. Scholarsh. 1, 10–20. Available online at: http://jces.ua.edu/wp-content/uploads/2012/10/JCES_Vol1No1.pdf#page=12
Lemoine, N. P. (2015). Climate change may alter breeding ground distributions if eastern migratory monarchs (Danaus plexippus) via range expansion of Asclepias host plants. PLoS ONE 10:e0118614. doi: 10.1371/journal.pone.0118614
Leston, L., and Koper, N. (2016). Urban rights-of-way as reservoirs for tallgrass prairie plants and butterflies. Environ. Manage. 57, 543–557. doi: 10.1007/s00267-015-0631-9
Nail, K. R., Stenoien, C., and Oberhauser, K. S. (2015). Immature monarch survival: effects of site characteristics, density, and time. Ann. Entomol. Soc. Am. 108, 690–690. doi: 10.1093/aesa/sav047
Oberhauser, K., Wiederholt, R., Diffendorfer, J., Semmens, D., Ries, L., Thogmartin, W., et al. (2017). A trans-national monarch butterfly population model and implications for regional conservation priorities. Eco. Entomol. 42, 51–60. doi: 10.1111/een.12351
Pleasants, J. M. (2017). Milkweed restoration in the Midwest for monarch butterfly recovery: estimates of milkweeds lost, milkweeds remaining and milkweeds that must be added to increase the monarch population. Insect Conserv. Divers. 10, 42–53. doi: 10.1111/icad.12198
Pleasants, J. M., and Oberhauser, K. S. (2012). Milkweed loss in agricultural fields because of herbicide use: effect on the monarch butterfly population. Insect Conserv. Divers. 6, 135–144. doi: 10.1111/j.1752-4598.2012.00196.x
Pleasants, J. M., Zalucki, M. P., Oberhauser, K. S., Brower, L. P., Taylor, O. R., and Thogmartin, W. E. (2017). Interpreting surveys to estimate the size of the monarch butterfly population: pitfalls and prospects. PLoS ONE 12:e0181245. doi: 10.1371/journal.pone.0181245
Prysby, M., and Oberhauser, K. S. (2004). “Temporal and geographical variation in monarch densities: citizen scientists document monarch population patterns,” in The Monarch Butterfly: Biology and Conservation, eds K. S. Oberhauser and M. J. Solensky (Ithaca, NY: Cornell University Press, 9–20.
Rohweder, J., and Thogmartin, W. E. (2016). Monarch Conservation Planning Tools. US Geological Survey. Available online at: https://www.umesc.usgs.gov/management/dss/monarch/desktop_monarch_conservation_planning_tools.html
Schensul, J. J., and LeCompte, M. D. (2010). Essential Ethnographic Methods. Ethnographer's Toolkit. New York, NY: AltaMira Press.
Semmens, B. X., Semmens, D. J., Thogmartin, W. E., Wiederholt, R., López-Hoffman, L., Diffendorfer, J. E., et al. (2016). Quasi-extinction risk and population targets for the Eastern, migratory population of monarch butterflies (Danaus plexippus). Sci. Rep. 6:23265. doi: 10.1038/srep23265
Stenoien, C., Nail, K. R., and Oberhauser, K. S. (2015). Habitat productivity and temporal patterns of monarch butterfly egg densities in the eastern United States. Ann. Entomol. Soc. Am. 108, 670–679. doi: 10.1093/aesa/sav054
Thogmartin, W. E., Diffendorfer, J. E., López-Hoffman, L., Oberhauser, K., Pleasants, J., Semmens, BX, et al. (2017a). Density estimates of monarch butterflies overwintering in central Mexico. PeerJ 5:e3221. doi: 10.7717/peerj.3221
Thogmartin, W. E., López-Hoffman, L., Rohweder, J., Diffendorfer, J., Drum, R., Semmens, D., et al. (2017b). Restoring monarch butterfly habitat in the Midwestern US: 'all hands on deck'. Environ. Res. Lett. 12:074005. doi: 10.1088/1748-9326/aa7637
Watkins, C., Westphal, L. M., Gobster, P. H., Vining, J., Wali, A., and Tudor, M. (2015). Shared principles of restoration practice in the Chicago Wilderness region. Hum. Ecol. Rev. 21, 155–177. doi: 10.22459/HER.21.01.2015.07
Keywords: milkweed, monarch butterfly, urban, metropolitan, habitat sampling, GIS tools, conservation
Citation: Johnston MK, Hasle AF, Klinger KR, Lambruschi MP, Derby Lewis A, Stotz DF, Winter AM, Bouman MJ and Redlinski I (2019) Estimating Milkweed Abundance in Metropolitan Areas Under Existing and User-Defined Scenarios. Front. Ecol. Evol. 7:210. doi: 10.3389/fevo.2019.00210
Received: 01 February 2019; Accepted: 21 May 2019;
Published: 21 June 2019.
Edited by:
Wayne E. Thogmartin, United States Geological Survey, United StatesReviewed by:
Carl Stenoien, University of Minnesota Twin Cities, United StatesKaren Tuerk, Monarch Joint Venture, United States
Copyright © 2019 Johnston, Hasle, Klinger, Lambruschi, Derby Lewis, Stotz, Winter, Bouman and Redlinski. This is an open-access article distributed under the terms of the Creative Commons Attribution License (CC BY). The use, distribution or reproduction in other forums is permitted, provided the original author(s) and the copyright owner(s) are credited and that the original publication in this journal is cited, in accordance with accepted academic practice. No use, distribution or reproduction is permitted which does not comply with these terms.
*Correspondence: Mark K. Johnston, bWpvaG5zdG9uQGZpZWxkbXVzZXVtLmVkdQ==