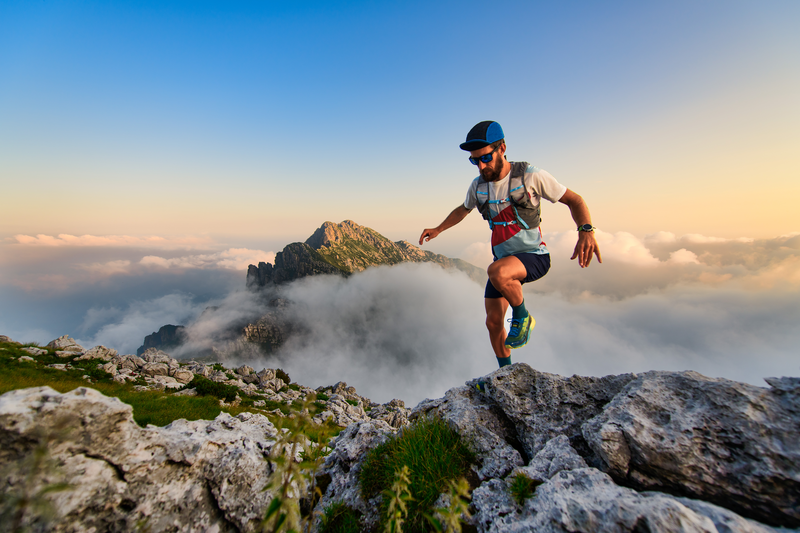
94% of researchers rate our articles as excellent or good
Learn more about the work of our research integrity team to safeguard the quality of each article we publish.
Find out more
ORIGINAL RESEARCH article
Front. Ecol. Evol. , 04 June 2019
Sec. Urban Ecology
Volume 7 - 2019 | https://doi.org/10.3389/fevo.2019.00201
This article is part of the Research Topic Patterns and Drivers of Urban Biodiversity View all 8 articles
Urban parks are biodiversity hotspots and are integral components of green infrastructure in urban areas. A variety of land use practices and environmental factors affect urban park biodiversity and vegetation structure, composition, and ecological function, but few studies have compared plant taxonomic composition, structural complexity, and species traits across different types of urban green spaces. The purpose of this study was to better understand the relationships between plant community composition, structural patterns, and environmental and species traits by using a standardized data collection method across different types of urban parks in Portland, Oregon. We examined the potential ways that different types of urban parks preserve native species and/or harbor non-native and invasive species. We used a stratified random sampling design to select 15 parks of different types based on use: (1) recreational-active use parks, (2) natural-passive use parks, and (3) multi-use parks. We found a total of 178 plant species belonging to 141 genera and 65 families. Multivariate analyses, including ordination with non-metric multidimensional scaling and cluster analysis were used to explore vegetation composition data and associations of different species assemblages with environmental variables. One-way analysis of variance was used to test hypotheses about variables associated with diversity. Statistically-significant differences in species richness and biodiversity indices were found between different park types. More native species were found in natural-passive use parks than other park types, more non-native species are found in multi-use parks than the other park types, and more invasive species were found in natural passive-use parks than in recreational-active use parks. Attributes such as natural-passive use park type, wetland habitat, steep slopes, native species origin, non-native species origin, and vine and tree plant forms were those most strongly correlated with the non-metric multidimensional scaling ordination, indicating that these attributes exert the strongest influence on species abundance and distribution with Portland's urban parks. The findings of this study can assist park managers in their aims to promote native species cover, reduce invasive species presence, or achieve additional management goals for urban parks.
Urban parks are important as biodiversity hotspots within cities. Not only do parks help preserve biodiversity in urban areas (Savard et al., 2000; Alvey, 2006; Nielsen et al., 2014; Threlfall et al., 2016), they also help to connect people with nature. These connections may be critical for public support of policies and actions whose goal is conservation of biodiversity. With over 55% of the world's population living in cities at present1, urban parks can allow opportunities for frequent nature interactions (Church, 2018). Urban parks can also harbor rare species and help protect important populations of vulnerable species. They may be critical components of corridors needed for habitat connectivity and dispersal among metapopulations (Ignatieva et al., 2011). Parks also play a role in people's efforts to fulfill ethical responsibilities such as being good stewards of the planet (Dearborn and Kark, 2010).
In addition to their role in biodiversity conservation, urban parks are also integral components of green infrastructure in urban areas (Savard et al., 2000; Cornelis and Hermy, 2004; Turner et al., 2005; Pataki et al., 2011; Nielsen et al., 2014). In the broad sense, green infrastructure has been defined as an interconnected system of natural areas, human-designed elements, and open spaces that not only conserves natural communities, biodiversity, and ecosystems, but also promotes clean water and air, and improves human physical and psychological well-being (Tzoulas et al., 2007; Benedict and McMahon, 2012; Lovell and Taylor, 2013). Natural communities can be described as assemblages of self-organizing and coevolved native species that are appropriate to local environmental conditions (Turner et al., 2005). While green infrastructure is designed to provide direct benefits such as stormwater management or recreation opportunities, it can also provide many co-benefits. It reduces energy use, increases economic growth, and assists with climate change adaptation and mitigation (European Commission, 2013). However, green infrastructure is not always considered an amenity. While some types of green infrastructure may increase land and property values (Bolitzer and Netusil, 2000; European Commission, 2013), other studies have noted a small, but significant decrease in property value directly adjacent to green street facilities (Netusil et al., 2014).
Multifunctional green infrastructure strategies aim to provide a holistic framework with flexible options that increase various co-benefits such as biodiversity conservation and social and ecological connectivity. These co-benefits extend beyond traditional green infrastructure uses that focus on a single benefit such as stormwater management2 (Lovell and Taylor, 2013; Meerow and Newell, 2017). However, there are often tradeoffs between maximizing these co-benefits, maximizing overall biodiversity, and increasing the presence of non-native and invasive species (Dearborn and Kark, 2010; Threlfall et al., 2016; Gaertner et al., 2017). For example, maximizing stormwater connectivity can result in decreases in landscape connectivity (Meerow and Newell, 2017). Additional studies are needed to better understand the role that different types of urban parks play in both promoting biodiversity and meeting other park management goals. In order to better inform and guide the multiple goals of park managers, such studies should include comparison of not only native species richness in parks, but also plant taxonomic composition, structural complexity, and species traits across different types of urban green spaces (Zhao et al., 2010;Threlfall et al., 2016).
While urban parks are some of the most species-rich urban green spaces (Savard et al., 2000; Cornelis and Hermy, 2004; Alvey, 2006; Nielsen et al., 2014), species richness tends to increase with moderate levels of urbanization, often as a result of the introduction of non-native species (Tait et al., 2005; McKinney, 2008). A variety of factors, including roads and impervious surface, distance to city center, human population size, and other land use activities are also associated with the success of non-native species (Pyšek, 1998; Kuhman et al., 2010; Gaertner et al., 2017). There is substantial controversy about whether the benefits of non-native species outweigh their negative impacts. On the one hand, many non-native species may increase urban biodiversity, provide a “sense of place” (e.g., Portland, Oregon is known as “The Rose City”), and a range of aesthetic and socioeconomic benefits for people (Gaertner et al., 2017; Zengeya et al., 2017). Introduced species may sometimes require less water than native species (i.e., species that evolved locally), and may survive the harsh conditions present in built green infrastructure facilities such as bioswales or detention ponds better than native species. Non-native species may also have a better chance of surviving fluctuations in climate (Morgenroth et al., 2016) and adapting to the altered physical conditions of urban environments (Alvey, 2006;McKinney, 2008).
The perceived potential and actual benefits as compared to the negative impacts of particular invasive species can vary significantly depending on context and local perspectives (Zengeya et al., 2017). Invasive species are defined by U.S. Executive Order 13112 (Section 1. Definitions) as non-native species whose introduction causes or are likely to cause economic, environmental, or human health harm3. Many non-native species do not cause harm to the economy, environment, or human health, but some non-native species are redefined as invasive species if and when they homogenize communities, replace native species, and promote a range of other ecosystem disservices (Alvey, 2006; McKinney, 2008; Gaertner et al., 2017). Undesirable attributes of invasive species include increasing the intensity or frequency of fires and floods (Pejchar and Mooney, 2009), or degrading habitat for native fauna. Furthermore, even if urban areas are biologically diverse, important functions of vegetation can be lost when native species are replaced by non-native species. A synthesis of several plant studies showed that exotic species (i.e., both non-native and invasive) accounted for an average of 41.8% of urban park woody species and 42.6% of all vascular species (Nielsen et al., 2014). Native herbaceous species were more likely to be lost than native woody species, in part due to competition with non-native species. Urban parks can play a role in limiting the spread of invasive species, depending on their disturbance history and management practices. Invasive plant species are found more frequently in landscapes historically altered by human disturbances (Kuhman et al., 2010), while “semi-natural” areas can represent a barrier to invasions (Celesti-Grapow et al., 2006).
The City of Portland has an on-going investment in green infrastructure such as parks to improve the ecological health, community livability, and environmental equity in Portland, Oregon City of Portland Bureau of Environmental Services, 2010; Portland Parks Recreation, 2015. Although many of its sustainability successes are not distributed homogeneously throughout the city (Goodling et al., 2015), Portland is still an important place to study as an example of incorporating green infrastructure into urban environments because it was named as one of the most sustainable cities within the United States (Portney, 2005). There are some publicly-available plant community composition data resources for the Portland metropolitan area4 (City of Portland Bureau of Environmental Services, 2008; Christy et al., 2009; Oregon iMapInvasives Resources, 2018; Portland Parks Recreation, 2018, 2019). An extensive set of vegetation surveys (e.g., of community and site characteristics, ecological health, management concerns, etc.) were previously completed for 8,213 acres of natural area park properties in Portland between 2003 and 2008 to help with city-wide urban ecosystem planning and management (Portland Parks Recreation, 2018). However, these studies did not compare plant taxonomic composition or species traits across the different natural properties. The surveys also only included natural area parks and did not address the influence of park type on the conservation of plant biodiversity. More recently, an inventory is being completed for Portland's urban park trees. These inventory data are currently publicly available for 11 parks (Portland Parks Recreation, 2019). Although this inventory will extend across multiple park types in Portland, it will only include information for trees and not shrubs, herbaceous, or vine species.
Although numerous studies have identified a variety of land use practices and environmental factors that affect urban park biodiversity, there have been very few studies that use a standardized method to compare plant taxonomic composition, structural complexity, environmental variables, and species traits across different types of urban green spaces. The purpose of this study is to better understand the relationships between plant community composition, structural patterns, environmental variables, and species traits in different types of urban parks in Portland, Oregon. A systematic study can improve our understanding of the degree to which parks of different types fulfill the various goals that people have for urban parks. These goals may include conserving biodiversity, meeting the needs and desires of park users for access to nature, and enhancing community livability and environmental equity.
We used a stratified random sampling design to survey different types of urban parks, including natural-passive use parks (i.e., generally more passively used and do not have formal fields for active play), developed recreational-active use parks (i.e., highly developed with facilities that promote active play, and 3) multi-use parks with a general mix of active and passive uses (Weems, 2016). We characterized the different park types and evaluated their contribution to the conservation of plant biodiversity by describing the plant taxonomical composition and structural complexity in Portland's urban parks, as well as comparing species richness across the different park types. In this study, we addressed the following research questions:
1) To what extent do plant species richness, biodiversity, traits, and structural complexity vary across different park types?
2) How can different urban parks be classified by their plant community composition?
3) What are some of the relationships between parks and a range of environmental variables and plant species traits?
We examined potential ways in which different types of urban parks preserve native species and/or harbor non-native and invasive species. We hypothesized that:
• Hypothesis 1: Natural-passive use parks have the highest species richness and biodiversity, followed by multi-use and then recreational-active use parks. This hypothesis is based on the idea that larger, intact natural areas tend to have more biodiversity (Whittaker, 1970; Alvey, 2006; Celesti-Grapow et al., 2006; Dearborn and Kark, 2010), while more disturbed areas are likely to have lower species richness (Celesti-Grapow et al., 2006).
• Hypothesis 2: Natural-passive use parks have the most native species, followed by multi-use parks, and then recreational-active use parks. This is based on the premise the that more natural, remnant, and less disturbed sites tend to have increased native species richness (Celesti-Grapow et al., 2006; Taylor and Santelmann, 2014; Highland et al., 2015).
• Hypothesis 3: Multi-use parks have the most non-native species compared to the other park types, because areas with moderate levels of disturbance tend to be accompanied by the addition of non-native species (McKinney, 2008).
• Hypothesis 4: Recreational-active use parks have the most invasive species, followed by multi-use parks and then natural-passive use parks. This hypothesis is based on research that invasive species are more frequently found in historically-altered landscapes with human disturbances (Kuhman et al., 2010), which are most similar to recreational-active use parks. Additionally, “semi-natural” areas (i.e., which are most similar to natural-passive use parks) can oftentimes represent a barrier to invasions, and so are likely to have fewer invasive species (Celesti-Grapow et al., 2006).
In order to address these research questions and hypotheses, we sampled the vegetation cover and assembled relevant environmental data for fifteen urban parks, sampling five parks in each of three different park types within Portland. The data were then evaluated for plant taxonomic composition, species richness, species diversity, and structural complexity for each of the parks and across the three park types. We explored how the different urban parks can be grouped by their plant community composition, as well as how the relationship between parks, environmental variables, and plant species traits can be characterized using non-parametric statistical methods (Kruskal, 1964; McCune and Grace, 2002). Improved understanding of the vegetation structure associated with parks of different types will help characterize not only their contribution to urban biodiversity, but also their utility in providing access to nature and the contribution made by different park types to livability and environmental equity. The results of this study may assist Portland's urban planners and land managers by providing an assessment of urban park vegetation in relation to current and future management goals.
Portland, Oregon is ~376 km2 and is located within Multnomah County in the Willamette Valley region of the Pacific Northwest at the confluence of the Columbia and Willamette Rivers. The physical geography of the Portland metropolitan region was shaped by a variety of geological events such as volcanic activity, catastrophic floods, ice ages, and thawing over hundreds of years, which contributed to the creation of sloughs, rivers with broad floodplains, various sizes of channels, rolling hills, steep mountainous areas, and marshy outlets (United States Department of Agriculture Soil Conservation Service, 1983). These landforms provide the basis for many of Portland's more than 4,047 hectares of parks, which include wetlands and river floodplains (e.g., Oaks Bottom Wildlife Refuge, Big Four Corners Natural Area, North Swan Island Boat Ramp, etc.), steep forested hillsides (e.g., Forest Park, Woods Memorial Natural Area, George Himes Park, etc.), and gentle rolling hillslopes (e.g., Ed Benedict Park, Brentwood Park, Rose City Park, etc.) (City of Portland, Oregon, 2018).
In addition to these physiographic features, the high yearly precipitation (~100–125 cm), moderate temperatures, and mix of nutrient-rich and hydric soil formations of the area provided conditions for a wide range of tree, shrub, vine, and herbaceous species to evolve in and/or inhabit in the area (United States Department of Agriculture Soil Conservation Service, 1983). Some of the common native plant species found in Portland's parks include Douglas fir (Pseudotsuga menziesii), western hemlock (Tsuga heterophylla), Oregon white oak (Quercus garryana), big-leaf maple (Acer macrophyllum), red alder (Alnus rubra), Pacific dogwood (Cornus sericea), vine maple (Acer circinatum), trailing blackberry (Rubus ursinus), Oregon oxalis (Oxalis oregana), Western sword fern (Polystichum munitum), willows (Salix sp.), and a variety of grasses (United States Department of Agriculture Soil Conservation Service, 1983; Christy et al., 2009; Portland Parks Recreation, 2011; Pojar and MacKinnon, 2016; Hitchcock and Cronquist, 2018). The vegetation, as well as the climate, physical geography, and natural disturbance (e.g., fire, wind, animal grazing, etc.) of the region act to support a variety of cultural and ethnobotanical uses (Pojar and MacKinnon, 2016), as well as ecosystem processes such as the flow of nutrients, water, and other materials (Swanson et al., 1988;Turner and Gardner, 2001).
A stratified random sampling design was used to select 12 urban parks within the Portland city boundary (Figure 1) (City of Portland, Oregon, 2018). The sites included four parks of each of three park types that were interspersed within each of the city's quadrants and had a range of sizes comparable to that of all Portland's parks. A park typology (Weems, 2016) was used to ensure that the examined parks were representative of Portland's parks. This typology included the following three general park types based on use: (1) recreational-active use parks that are highly developed with facilities that promote active play such as sports fields and play equipment, (2) natural-passive use parks that are generally more passively used with benches, viewing areas, and trails, but do not have formal fields for active play, and (3) multi-use parks with a general mix of active and passive uses (Weems, 2016). An additional natural-passive use park, Forest Park, was added to the list of sampled parks because it is the largest and most iconic park in Portland (Portland Parks Recreation, 2011). In order to have a more balanced study design, an additional recreational-active use park and a multi-use park were added to the list of sampled parks, for a total of fifteen parks with five parks of each type.
Within each of the selected parks, data were collected in five 400-m2 square plots that were identified using randomly selected pairs of coordinates placed at their southeastern corner. This allowed all areas within each park to be equally likely to be selected for sampling and to likely average any potential effects related to the variation of human management and/or landscape design within each park. Field data included the general vicinity, landform, slope, overall bare ground, tree species (diameter breast height [DBH] of at least 15 cm) and cover, sapling/shrub species (DBH < 15 cm) and cover, and woody vine species and cover. Species that occupied more than one plant form were recorded with their cover for each plant form. Additionally, five 1-m2 square subplots with randomly selected locations were sampled for herbaceous species and cover within each of the 400-m2 plots. Plant trait data were obtained from the Plants Database (United States Department of Agriculture, 2018) and other local guides (Christy et al., 2009; Hitchcock and Cronquist, 2018), and the wetlands data were collected from the US Fish and Wildlife Service Wetland Mapper (United States Fish and Wildlife Service, 2018). Field data were collected from June 28, 2017 to September 17, 2017, while much of the vegetation was at its peak phenological development for identification purposes. Information for the plant community composition by park, environmental variables, plant species and traits, and sample plot locations within each park can found in the Supplementary Material.
The species matrix (15 sample units x 189 species) contained percent cover data for trees, saplings/shrubs, and vines averaged for the five 400-m2 square plots within each park. There were 11 species that occupied both the tree and sapling/shrub plant forms and were counted as different taxonomic units for the multivariate analysis, but not as different species for the calculations of species richness and taxonomic composition. The herbaceous species cover data within the matrix were the average percent cover for all of the 1-m2 square plots sampled within each of the 400-m2 square plots of each park.
The environmental matrix (15 sample units x 17 variables) contained measurements of habitat variables and park characteristics such as park type, wetland presence (National Wetland Inventory [NWI]) within each park (United States Fish and Wildlife Service, 2018), landform, local relief, percent slope, percent bare ground, elevation, establishment date, irrigation, total park acreage, and beta diversity (Table 1). In order to calculate beta diversity (half changes), a measure of heterogeneity for the 400-m2 plots (total of 75 plots for all 15 parks) was calculated by finding the average within group distance (Sørensen) for each of the parks. Half-changes were then calculated (McCune and Grace, 2002) using the following formula:
The traits matrix (189 species x 16 traits) contained trait information for each of the plant species, which included: growth form, group (e.g., fern, horsetail, etc.), status (e.g., native, non-native, invasive, etc.), and growth duration (e.g., annual, perennial, etc.) (Table 2). In this study, we separately analyzed native species, non-native non-invasive species (hereon classified as non-native species), and non-native invasive species (hereon classified as invasive species). A SU x traits matrix was calculated using the original abundance data using weighted averages, and standardizing the traits by minimum to maximum in PC-ORD Version 7 (MjM Software, Gleneden Beach, Oregon). An outlier analysis was also performed to identify any potential outliers with an average Sørensen distance of more than two standard deviations from the grand mean of distances among sample units. No outliers were identified.
The data were evaluated for plant taxonomic composition, species richness, species diversity, and structural complexity for each of the parks and across the three park types. Species diversity is composed of species richness, which is the number of species in a sample unit or area, as well as evenness of abundance (Whittaker, 1970; McCune and Grace, 2002). In general, if two sample units have the same number of species, then the sample unit with a more even distribution of species would be considered to have higher species diversity. Diversity for each of the parks was measured using the Shannon-Weiner Index and Simpson Index (Whittaker, 1970). One-way analysis of variance was used to test hypotheses about variables associated with plant species traits and diversity.
Hierarchical agglomerative clustering was then used to construct groups of the parks by their plant community composition (McCune and Grace, 2002). The original data were transformed into presence-absence data, in which all occurrences received equal weighting regardless of abundance. This transformation was selected because it reduced skewness of species, beta diversity (half changes, βD), and coefficient of variation for columns as compared to the to the original data and a square root transformation. The hierarchical agglomerative clustering was performed in PC-ORD Version 7, using the Sørensen (Bray-Curtis) distance measure with Flexible Beta (beta of −0.25) and a group membership level of 5, which provided a good compromise between the interpretability of groups and information loss (~55% retained). The Sørensen distance measure was selected because it is one of the most effective measures for ecological community data (McCune and Grace, 2002). The Flexible Beta (beta of −0.25) was selected because it helped to avoid distortion in the clustering and it has less of a propensity to chain (McCune and Grace, 2002). The data were not relativized. Multi-response permutation procedure (MRPP), a non-parametric procedure, was then used to test the hypothesis of no difference between species composition by a priori park groups (e.g., natural-passive use, multi-use, and recreational-active use parks). The MRPP for this analysis included the Sørensen (Bray-Curtis) distance measure and the “n/sum(n)” weighting of groups.
The relationships between the sample units, measured environmental variables, and plant species traits were then characterized with non-metric multidimensional scaling (NMS). NMS is a non-parametric ordination technique that iteratively searches for positions of entities on axes to minimize the stress of the configuration (Kruskal, 1964; McCune and Grace, 2002). This technique was selected for this study because it does not have normality or linearity assumptions. The NMS of the sample units in species space was completed in PC-ORD Version 7, using the “autopilot mode,” slow and thorough speed, a random starting configuration, 200 runs with real data, Sørensen (Bray-Curtis) distance measure, and penalizing unequal ordination distance. The final solution for the NMS ordination of the parks in species space was determined by plotting final stress vs. the number of dimensions and choosing the number of axes beyond which stress reductions were small (McCune and Grace, 2002). Overlays of the environmental variables and trait distances as joint plots were examined to evaluate the direction and strength of linear relationships with the axes. Hilltop plots were created to show multiple non-linear response surfaces on a single graph and display their maximums (settings: flexibility—optimize, contour fitting of 2 standard deviations, top 20% of range) (Nelson et al., 2015).
The urban parks within the study were all managed landscapes, but had varying intensity of human management and landscape design. The highest levels horticultural design appeared to be in the recreational-active use parks, while more naturalistic landscape approaches were typically observed in the natural-passive use parks. Varying combinations of these management approaches were observed in the multi-use parks, which oftentimes had both horticultural beds and patches of more naturalistic areas within the same park. The plots within the parks also tended to reflect some of these patterns of varying intensity for human management and design, with the most heavily designed plots in the recreational-active use parks, more naturalistic approaches in the plots of the natural-passive use parks, and generally a combination of these approaches within the multi-use park plots. While no park typology can capture all of the variation in landscape design, management, and visitor use, the typology used in this study allowed for a diverse range of urban parks to be sampled. The following sections provide the taxonomic composition, species richness, diversity indices, structural complexity, and community composition and multivariate analysis results of the study.
The data set included a total of 178 plant species belonging to 141 genera and 65 families (Figure 2). The families with the greatest number of plant species included Rosaceae (e.g., Crataegus douglasii, Rubus armeniacus, etc.), Poaceae (e.g., Poa pratensis, Holcus lanatus, etc.), Asteraceae (e.g., Achillea millefolium, Bellis perennis, etc.), Berberidaceae (e.g., Mahonia aquifolium, Achlys triphylla, etc.), and Cyperaceae (e.g., Carex obnupta, Carex unilateralis, etc.). Of the 65 plant families, 71% had only one to two species.
Figure 3 shows the number of native, non-native, invasive, unknown, and total number of species by park. The parks with the highest total number of species were relatively large, natural passive-use parks such as Big Four Corners Natural Area and Woods Memorial Natural Area, while the parks with the lowest number of species were recreational-active use parks such as Jamison Park and Wallace Park. Of the 178-total species, there were mostly native species (43 percent), followed by non-native (31 percent), invasive (21 percent), and unknown species (5 percent) (e.g., unidentifiable in the field). The average number of native, non-native, invasive, unknown, and total plant species by park type are shown in Figures 4A–D. More native species were found in natural-passive use parks than the other park types, F(2, 12) = 68.86, p < 0.01) (Figure 4A), and more non-native species were found in multi-use parks than the other park types F(2, 12) = 10.63, p < 0.01) (Figure 4B). However, a higher number of invasive species were found in natural passive-use parks (M = 10.8, SD = 3.8) than in recreational-active use parks (M = 3.8, SD = 1.8) (Figure 4C). There was not a significant difference in the number of unknown species by park type. Overall, there was a significant difference in total species richness was found between the different park types, F(2, 12) = 31.83, p < 0.001 (Figure 4D). Species richness was significantly higher in natural passive-use parks (M = 43, SD = 8.4) than multi-use parks (M = 23.2, SD = 5) and recreational-active use parks (M = 13.2, SD = 3.6).
Figure 4. Average number of plant species that were: (A) native, (B) non-native, (C) invasive, and (D) total for each park type. N, natural-passive use; M, multi-use; R, recreational-active use. Error bars represent ± 1 standard deviation.
The diversity indices for each park are shown in Table 3. In general, the parks with highest Shannon-Weiner and Simpson indices were the natural-passive use parks such as Big Four Corners Natural Area and Woods Memorial Natural Area, followed by multi-use parks such as Patton Square Park, and then recreational-active use parks such as Khunamokwst Park. However, one of the multi-use parks, Plaza Blocks, had lower diversity indices than some of the recreational-active use parks. A significant difference between park types was also found in the Shannon-Weiner biodiversity index F(2, 12) = 18.1, p < 0.001). The Shannon-Weiner index was significantly higher in the natural-passive use parks (M = 2.8, SD = 0.36), than in the multi-use parks (M = 2, SD = 0.28) and recreational-active use parks (M = 1.7, SD = 0.2). Using the Simpson Index results, there was a significant different between park types F(2, 12) = 7.49, p < 0.01), with the natural-passive use parks Simpson Index being significantly higher than the recreational-active use parks (M = 0.74, SD = 0.07).
Table 3. Diversity indices for each park and park type (N, natural-passive use; M, multi-use; R, recreational-active use).
In relation to plant structural complexity, the largest number of species were the herbaceous species, followed by the sapling/shrub species (11 of which were also tree species), tree species, and vine species (Table 4). Table 4 shows the number of plant species and percentage of invasive, native, non-native, and unknown species by plant form. The largest number native and invasive species were found in the herbaceous plant form, while the most non-native species were found in the sapling/shrub plant form. However, the vines had the largest percentage of invasive species of any plant form and trees had the largest percentage of non-natives of any plant form.
Table 4. Number of species and percentage of invasive, native, non-native, unknown, and total species by plant form (herbaceous, sapling/shrub, tree, vine).
The hierarchical agglomerative clusters of the parks can be interpreted in the context of their characteristics and habitat associations (Figure 5). The cluster analysis retained ~50–55% of the data with five groups and ~12% chaining. A cluster of five parks, including Big Four Corners Natural Area, Oaks Bottom Wildlife Refuge, Woods Memorial Natural Area, George Himes Park, and Forest Park can be generally described as natural-passive use parks with more native species. Other clusters included multi-use parks with more non-native species (e.g., Plaza Blocks, etc.), multi-use and recreational-active use parks with many non-native species and more herbaceous cover (e.g., Khunamokwst Park, Brentwood Park, etc.), a park with more bare ground and beta diversity between plots (North Swan Island Boat Ramp), and a park with more non-native trees (Jamison Square). It is important to note that beta diversity was high at the North Swan Island Boat Ramp primarily because one of its plots was in vegetation and most of the others were located in primarily impervious concrete.
The clusters differ in terms of park type (MRPP: A = 0.17, p < 0.001). A more positive A statistic indicates that groups have more agreement than what is expected by chance. The MRPP data analysis has a low p-value, which indicated stronger evidence to reject the null hypothesis that there is no difference in plant community composition between park groups. Pairwise comparisons for each of the clusters in terms of park type revealed that natural-passive use parks were different compared to multi-use and recreational-active use parks (p < 0.05), but that multi-use and recreational-active use parks were only marginally different from each other (p < 0.1).
The NMS ordination shows the parks (sample units) as triangles in species space with overlays of the environmental variables and traits as joint plots to reflect the direction and strength of linear relationships with the axes (Figures 6–9). The plot of final stress vs. the number of dimensions showed that a 3-dimensional solution (3 axes) was best for this dataset with a final stress of 5.0 (raw stress multiplied by 100) and a R2n (non-metric fit) of 0.99. In NMS, ecological community data sets with a final stress of 5.0 are generally considered to be “good” (McCune and Grace, 2002). The final NMS solution with the environmental variables as joint plots are shown in Figure 6 (Axes 1 and 2) and Figure 7 (Axes 1 and 3). Most of the variation is shown in the first two axes (r2 = 0.82). The most strongly correlated environmental variables with Axis 1 are natural-passive use park type (r = −0.95), wetland presence (r = −0.93), hillslope landform (r = 0.78), slope percent (r = −0.78), and irrigation (r = 0.68). The most strongly correlated environmental variables for Axis 2 are bare ground percent (r = 0.85) and beta diversity (half changes) (r = 0.82), and then multi-use park type (r = −0.49) and flat local relief (r = −0.48) for Axis 3. The final NMS solution with plant species traits as joint plots are provided in Figure 8 (Axes 1 and 2) and Figure 9 (Axes 1 and 3). The Pearson correlations show that the most strongly correlated trait variables with Axis 1 are native species (r = −0.95) (e.g., Sambucus racemosa, Corylus cornuta, Acer macrophyllum, and Alnus rubra, etc.), non-native species (r = 0.83) (e.g., Lolium perenne and Trifolium repens, etc.), vines (r = −0.75) (e.g., Rubus ursinus and Hedera helix, etc.), and saplings/shrubs (r = −0.75) (e.g., Symphoricarpos albus, etc.). The most strongly correlated variable with Axis 2 is tree (r = 0.61), and then mixed growth pattern (r = 0.44) for Axis 3. One of the weakest correlations of the ordination is invasive species (r = 0.16) for Axis 1.
Figure 6. NMS ordination Axes 1 and 2 for the parks in species space. Environmental variables that are strongly related to the individual ordination axes (r2 > 0.2) are shown as joint plots (radiated red lines).
Figure 7. NMS ordination Axes 1 and 3 for the parks in species space. Environmental variables that are strongly related to the individual ordination axes (r2 > 0.2) are shown as joint plots (radiated red lines).
Figure 8. NMS ordination Axes 1 and 2 for the parks in species space. Trait variables that are strongly related to the individual ordination axes (r2 > 0.2) are shown as joint plots (radiated red lines).
Figure 9. NMS ordination Axes 1 and 3 for the parks in species space. Trait variables that are strongly related to the individual ordination axes (r2 > 0.2) are shown as joint plots (radiated red lines).
Various hilltop plots for plant traits and select plant species are shown in Figures 10A–D. They depict the optima of native, non-native, and invasive species (Figure 10A), different plant forms (Figure 10B), and various plant groups (e.g., dicot, monocot, gymnosperm, fern, and horsetail) (Figure 10C), plant groups. The optima for native species and several plant groups are within the natural-passive use parks, while the optimum for non-native species overlaps with both multi-use and recreational-active use parks. Figure 10D indicates that some invasive plant species are not acting in concert. For example, the optimum for Poa annua is in the multi-use and recreational-active use parks, while the optima for Geranium robertianum, Hedera helix, Phalaris arundinacea, and Rubus armeniacus are among natural-passive use parks.
Figure 10. Hilltop plots for the presence/absence NMS ordination. Each colored area is a hilltop that shows the optima of a variable, and the multiple partially transparent hilltops show the overlap of variable optimas for (A) native, non-native, and invasive species, (B) herb, vine, sapling/shrub, and tree strata, (C) dicot, monocot, gymnosperm, fern, and horsetail, and (D) select invasive species. Park code information can be found in the Supplementary Material.
Improved understanding of plant community composition patterns in urban parks can help communities better manage green infrastructure investments, which are important for improving ecological health, community livability, and environmental equity within urban areas City of Portland Bureau of Environmental Services, 2010; Portland Parks Recreation, 2015. Our study was unique for Portland in that it used a stratified random sampling method to address the influence of park type on the conservation plant biodiversity and also included information for tree, shrub, herbaceous, and vine species. This type of research extends beyond past sampling efforts that included only natural-passive use parks (Portland Parks Recreation, 2018) or surveys that include only information on tree species within Portland parks (Portland Parks Recreation, 2019). The stratified-random sampling design allowed us to investigate the extent to which plant species composition, biodiversity, environmental variables, and species traits vary across different urban park types in Portland, Oregon. Although this study used a sampling protocol rather than attempting a complete floristic survey of all parks due to time and resource constraints, a total of 178 species from 141 genera and 65 families were found in the sampled parks. The largest number of species were herbaceous species, followed by sapling/shrub species, tree species, and vine species.
This study provided information on the ways in which different types of urban parks can contribute to the conservation of native species and/or harbor non-native and invasive species. The total species richness was highest in the natural-passive use parks, followed by multi-use, and then recreational-active use parks, which was consistent with Hypothesis 1. The results also showed that more native species were found in natural-passive use parks than other types of parks, which aligns with Hypothesis 2, and is consistent with other studies which have reported that more natural, remnant, and less disturbed sites tend to have increased native species richness (Celesti-Grapow et al., 2006; Taylor and Santelmann, 2014; Highland et al., 2015). It is not surprising that the average species richness and biodiversity indices were highest in the natural-passive use parks, because areas that are natural have a tendency to be more diverse than other areas (Whittaker, 1970; Alvey, 2006; Dearborn and Kark, 2010) and provide a range and diversity of resources and habitat structures for species (Threlfall et al., 2016). In order to further promote biodiversity conservation in urban areas, park managers and/or land use planners can use the results of this study to justify limiting recreational development, land use change, and other human disturbances in natural-passive use parks. Conservation of more natural, remnant sites is likely to increase plant and animal biodiversity within cities. The outcomes of this study may also be used to further promote the creation of native habitat patches in multi-use and recreational-active use parks for the purposes of increasing native biodiversity and its associated benefits (Portland Parks Recreation, 2015).
The results of the study also showed that more non-native species were found in multi-use parks than in natural-passive use or recreational-active use parks, which aligns with Hypothesis 3, and supports the notion that moderate levels of disturbance tend to be accompanied by the addition of non-native species (McKinney, 2008). However, a surprising result was that more invasive species were found in natural-passive use parks than in recreational-active use parks, a result which is inconsistent with Hypothesis 4. Although “semi-natural” areas (i.e., which are most similar to natural-passive use parks) can often represent a barrier to invasions (Celesti-Grapow et al., 2006), species invasions of natural, forested areas may simply develop more slowly under natural disturbance regimes (Martin et al., 2009). These species invasions can then be accelerated with the increase of human influence such as horticultural practices and intentional introduction of plant species (Martin et al., 2009). In addition to these explanations, it is also possible that the natural-passive use parks in this study may have experienced a high enough level of disturbance that they were vulnerable to invasive species colonization. Another confounding issue may be that invasive species management can be more challenging in more remote and larger natural-passive use parks than in multi-use and recreational-active use parks, which tend to be more accessible and smaller in size.
In terms of overall plant species composition, most of the species in the parks were native (43 percent), followed by non-native species (31 percent), and with a smaller percentage of invasive species (21 percent). The largest number of native and invasive species were herbaceous species, while the most non-native species were saplings/shrubs. The lower proportion of invasive species relative to native species may indicate some progress toward invasive species management in Portland (City of Portland Bureau of Environmental Services, 2008). However, the combined number of non-native and invasive species in our plots accounted for 52% of the total species richness, which is slightly higher than a previous synthesis of a few plant studies, which showed an average of 41.8% exotics for urban park woody species and 42.6% exotics for vascular species (Nielsen et al., 2014). The results presented here also indicate that the herbaceous plant form has the largest number of invasive species, while the vines have the largest percentage of invasive species of any plant form. These results, among others, may assist managers by providing an assessment of urban park vegetation in relation to existing and future management goals.
For our second research question, we used the sampled data to examine how different urban parks in Portland can be classified by their plant community composition, as well as some of the relationships between parks and a range of environmental variables and plant species traits. The hierarchical agglomerative cluster analysis of the parks in the study included a cluster of five parks that can be generally described as natural-passive use parks with more native species. Other clusters included two multi-use parks with more non-native species, five multi-use and recreational-active use parks with many non-native species and more herbaceous cover, a park with more bare ground and beta diversity between plots, and a park with more non-native trees. Natural-passive use parks could be distinguished from multi-use and recreational-active use parks, but species composition of plots from multi-use and recreational-active use parks were only marginally different from each other. These results indicate that there may be a continuum between the vegetation community composition of the multi-use and recreational-active parks in Portland, which makes clear separation of these two park types difficult. Other studies have also noted that multi-use and recreational-active use parks can be difficult to distinguish (e.g., Weems, 2016).
To answer our third research question, we found that attributes such as natural-passive use park type, wetland presence, hillslope landform, steep slopes, and irrigation were those most strongly correlated with the NMS ordination, indicating that these attributes exert the strongest influence on species abundance and distribution with Portland's urban parks. Some of the most strongly correlated plant trait attributes are related to native and non-native species origin and vine and tree physiognomy. Portland Parks and Recreation already considers attributes such as whether species are native or invasive in management of urban parks. However, invasive species cover was only weakly correlated with the ordination axes, which indicates that in addition to park type, other factors are likely to influence the occurrence of invasive species. Land and water management of nearby and surrounding areas can have a strong impact on the diversity of urban green infrastructure (Hostetler et al., 2011). For example, the use of urban parks with wetlands to help manage nutrient-laden stormwater runoff can lead to conversion of wetlands established to help preserve native vegetation from a more natural vegetation type to one more dominated by invasive species such as reed canary grass (Phalaris arundinacea) (Maurer et al., 2003; Taylor and Santelmann, 2014). Future studies could investigate the effect of park context as well as park type in applying this methodology to Portland and other cities, to further investigate the relative influence of park type and neighborhood context as influences on plant community composition and biodiversity patterns.
Our findings are consistent with other studies that have identified parks as important for conserving biodiversity in urban areas. In a study of 15 urban and suburban park of Flanders, Belgium, the parks had higher amounts of species richness especially if they contained semi-natural habitat and were larger in terms of area (Cornelis and Hermy, 2004). Other studies have also shown that areas with the greatest biodiversity tend to be large and have intact natural habitat (Cornelis and Hermy, 2004; Rosenzweig, 2005; Alvey, 2006). However, in a study of plant composition in different types of urban green spaces in Melbourne, Australia, urban parks had less species diversity than golf courses, residential neighborhoods, and vegetation remnants in urban areas (Threlfall et al., 2016). This was attributed to the urban parks having reduced structural diversity with low tree density, sparse understory, and high amounts of imported mulch, which are managed for basic amenities, low maintenance costs, and safety rather than for biodiversity or habitat. Even so, improved management of plant community composition, structure, and cover of all types urban green spaces, including urban parks, has the potential to greatly influence habitat quality for vertebrates, invertebrates, microorganisms, and fungi (Threlfall et al., 2016). Greater structural diversity of the vegetation also tends to enhance faunal species richness and biodiversity (Rosenzweig, 2005; Fontana et al., 2011).
By using a standardized method applied consistently across different park types, we were able to identify the influence of urban park type on plant community composition and biodiversity, including tree, sapling/shrub, herbaceous, and vine species. This research builds upon the urban biodiversity research examinof others to examine plant taxonomic composition, structural complexity, and species traits across different types of urban green spaces and extends it toward the examination of different urban park types in Portland, Oregon. The results of this study may assist urban park managers in their aims to promote native species cover, reduce invasive species cover, or achieve other outcomes for Portland's urban parks (City of Portland Bureau of Environmental Services, 2008; Portland Parks Recreation, 2015). The cluster analysis groups, NMS ordinations with joint plots, and hilltop plots can be used to highlight particular parks and/or plant species, as well as provide additional information for potential management actions. For example, park managers can use the results to see that natural-passive use parks are more correlated with native species cover, while multi-use parks tend to be more correlated with non-native species. If park managers are interested in conserving particular plant groups such as ferns, then they can observe how these are more correlated with natural-passive use parks and focus their conservation efforts in these areas. These results may also be used to address environmental equity concerns such as the spatial distribution and accessibility of different types of urban parks (City of Portland Bureau of Environmental Services, 2010; Weems, 2016), as well as accessibility to a range of native habitats and plant biodiversity. Finally, the hilltop plots may be especially useful for identifying species of interest (e.g., rare native species, invasive species, etc.) at their optimum locations to target specific management actions. More in-depth studies and comparisons of plant community composition and biodiversity patterns in urban parks of Portland with other cities would provide greater understanding of the influence of park type on urban biodiversity. Ultimately, improved management of plant community composition, structure, and cover within all park types has the potential to improve habitat quality for animals, microorganisms, and fungi, as well as a range of economic, health, social, and cultural benefits for people who live in urban areas.
Although many studies have identified a variety of land use practices and environmental factors that may affect urban park biodiversity, there have been very few studies that use a standardized method to compare plant taxonomic composition, biodiversity, structural complexity, and species traits across different types of urban green spaces. This research builds upon the urban biodiversity research of others to examine taxonomic composition, structural complexity, and species traits across different types of urban park in Portland, Oregon. By using a standardized method applied consistently across different park types, we were able to identify the influence of urban park type on plant community composition and biodiversity, including tree, sapling/shrub, vine, and herbaceous species. Improved understanding of plant community composition patterns in urban parks can help communities better manage green infrastructure investments, which are important for improving ecological health, community livability, and environmental equity within urban areas. The results of this study may assist urban park managers in their aims to promote native species cover, reduce invasive species cover, or achieve other outcomes for urban parks.
All datasets generated for this study are included in the manuscript and/or the Supplementary Files.
MT designed the study, performed the field data collection, completed statistical analysis, and wrote the manuscript. MS assisted in study design, provided guidance for and review of field data collection and statistical analysis, and assisted in preparation of the manuscript.
Urban Water Innovation Network (UWIN) National Science Foundation Grant Award #1444758.
The authors declare that the research was conducted in the absence of any commercial or financial relationships that could be construed as a potential conflict of interest.
We thank Hattie Greydanus and Michael Tchintcharauli-Harrison for their help with field data collection and Dr. Bruce McCune for his assistance with the multivariate statistical analysis. Financial support was provided from the Urban Water Innovation Network (UWIN) National Science Foundation Grant Award #1444758. The City of Portland authorized research permit #1484773 for our data collection on park properties.
The Supplementary Material for this article can be found online at: https://www.frontiersin.org/articles/10.3389/fevo.2019.00201/full#supplementary-material
1. ^United Nations Division of Economic and Social Affairs. (2018). Revision of World Urbanization Prospects. Retrieved from: https://www.un.org/development/desa/en/news/population/2018-revision-of-world-urbanization-prospects.html.
2. ^Environmental Protection Agency. (2018). What is Green Infrastructure? Retrieved from: https://www.epa.gov/green-infrastructure/what-green-infrastructure.
3. ^U.S. Department of Agriculture. (2019). What are Invasive Species? Retrieved from: https://www.invasivespeciesinfo.gov/what-are-invasive-species.
4. ^Oregon Biodiversity Information Center. (2018). About ORBIC. Retrieved from: https://inr.oregonstate.edu/orbic/about-orbic.
Alvey, A. A. (2006). Promoting and preserving biodiversity in the urban forest. Urban Forestry Urban Greening 5, 195–201. doi: 10.1016/j.ufug.2006.09.003
Benedict, M. A., and McMahon, E. T. (2012). Green Infrastructure: Linking Landscapes and Communities. Washington, DC: Island Press.
Bolitzer, B., and Netusil, N. R. (2000). The impact of open spaces on property values in Portland, Oregon. J. Environm. Manag. 59, 185–193. doi: 10.1006/jema.2000.0351
Celesti-Grapow, L., Pyšek, P., Jarošík, V., and Blasi, C. (2006). Determinants of native and alien species richness in the urban flora of Rome. Diversity Distributions 12, 490–501. doi: 10.1111/j.1366-9516.2006.00282.x
Christy, J. A., Kimpo, A., Marttala, V., Gaddis, P. K., and Christy, N. L. (2009). Urbanizing Flora of Portland, Oregon, 1806–2008. Available online at: https://ir.library.oregonstate.edu/concern/technical_reports/j6731792f
Church, S. P. (2018). From street trees to natural areas: retrofitting cities for human connectedness to nature. J. Environ. Planning Manag. 61, 878–903. doi: 10.1080/09640568.2018.1428182
City of Portland Bureau of Environmental Services (2008). City of Portland Invasive Plant Strategy Report 2008.
City of Portland Bureau of Environmental Services (2010). Portland's Green Infrastructure: Quantifying the Health, Energy and Community Livability Benefits.
City of Portland, Oregon. (2018). Parks. Retrieved from: http://gis-pdx.opendata.arcgis.com/datasets/parks (accessed November 4, 2018).
Cornelis, J., and Hermy, M. (2004). Biodiversity relationships in urban and suburban parks in Flanders. Landscape Urban Planning 69, 385–401. doi: 10.1016/j.landurbplan.2003.10.038
Dearborn, D. C., and Kark, S. (2010). Motivations for Conserving Urban Biodiversity. Conserv. Biol. 24, 432–440. doi: 10.1111/j.1523-1739.2009.01328.x
European Commission (2013). Green Infrastructure (GI)—Enhancing Europe's Natural Capital—Communication from the Commission to the European Parliament, The Council, The European Economic and Social Committee and the Committee of the Regions.
Fontana, S., Sattler, T., Bontadina, F., and Moretti, M. (2011). How to manage the urban green to improve bird diversity and community structure. Landscape Urban Planning 101, 278–285. doi: 10.1016/j.landurbplan.2011.02.033
Gaertner, M., Wilson, J. R. U., Cadotte, M. W., MacIvor, J. S., Zenni, R. D., and Richardson, D. M. (2017). Non-native species in urban environments: patterns, processes, impacts and challenges. Biol. Invasions 19, 3461–3469. doi: 10.1007/s10530-017-1598-7
Goodling, E., Green, J., and McClintock, N. (2015). Uneven development of the sustainable city: shifting capital in Portland, Oregon. Urban Geography 36, 504–527. doi: 10.1080/02723638.2015.1010791
Highland, S. A., Santelmann, M. V., and Schwindt, R. A. (2015). Vegetation dynamics of restored and remnant willamette valley, OR Prairie Wetlands. Ecol. Restoration 33, 156–170. doi: 10.3368/er.33.2.156
Hitchcock, C. L., and Cronquist, A. (2018). Flora of the Pacific Northwest: An Illustrated Manual, 2nd Edn. New York, NY: University of Washington Press.
Hostetler, M., Allen, W., and Meurk, C. (2011). Conserving urban biodiversity? Creating green infrastructure is only the first step. Landscape Urban Planning 100, 369–371. doi: 10.1016/j.landurbplan.2011.01.011
Ignatieva, M., Stewart, G. H., and Meurk, C. (2011). Planning and design of ecological networks in urban areas. Landscape Ecol. Eng. 7, 17–25. doi: 10.1007/s11355-010-0143-y
Kruskal, J. B. (1964). Multidimensional scaling by optimizing goodness of fit to a nonmetric hypothesis. Psychometrika 29, 1–27
Kuhman, T. R., Pearson, S. M., and Turner, M. G. (2010). Effects of land-use history and the contemporary landscape on non-native plant invasion at local and regional scales in the forest-dominated southern Appalachians. Landscape Ecol. 25, 1433–1445. doi: 10.1007/s10980-010-9500-3
Lovell, S. T., and Taylor, J. R. (2013). Supplying urban ecosystem services through multifunctional green infrastructure in the United States. Landscape Ecol. 28, 1447–1463. doi: 10.1007/s10980-013-9912-y
Martin, P. H., Canham, C. D., and Marks, P. L. (2009). Why forests appear resistant to exotic plant invasions: intentional introductions, stand dynamics, and the role of shade tolerance. Front. Ecol. Environ. 7, 142–149. doi: 10.1890/070096
Maurer, D. A., Lindig-Cisneros, R., Werner, K. J., Kercher, S., Miller, R., and Zedler, J. B. (2003). The Replacement of Wetland Vegetation by Reed Canarygrass (Phalaris arundinacea). Ecol. Restoration 21, 116. doi: 10.3368/er.21.2.116
McCune, B., and Grace, J. B. (2002). Analysis of Ecological Communities. MjM Software Design. Gleneden Beach, OR.
McKinney, M. L. (2008). Effects of urbanization on species richness: a review of plants and animals. Urban Ecosystems 11, 161–176. doi: 10.1007/s11252-007-0045-4
Meerow, S., and Newell, J. P. (2017). Spatial planning for multifunctional green infrastructure: growing resilience in Detroit. Landscape Urban Planning 159, 62–75. doi: 10.1016/j.landurbplan.2016.10.005
Morgenroth, J., Östberg, J., Konijnendijk van den Bosch, C., Nielsen, A. B., Hauer, R., Sjöman, H., et al. (2016). Urban tree diversity—Taking stock and looking ahead. Urban Forestry Urban Greening 15, 1–5. doi: 10.1016/j.ufug.2015.11.003
Nelson, P. R., McCune, B., Roland, C., and Stehn, S. (2015). Non-parametric methods reveal non-linear functional trait variation of lichens along environmental and fire age gradients. J. Veget. Sci. 26, 848–865. doi: 10.1111/jvs.12286
Netusil, N. R., Levin, Z., Shandas, V., and Hart, T. (2014). Valuing green infrastructure in Portland, Oregon. Landscape Urban Planning 124, 14–21. doi: 10.1016/j.landurbplan.2014.01.002
Nielsen, A. B., van den Bosch, M., Maruthaveeran, S., and van den Bosch, C. K. (2014). Species richness in urban parks and its drivers: a review of empirical evidence. Urban Ecosyst. 17, 305–327. doi: 10.1007/s11252-013-0316-1
Oregon iMapInvasives Resources (2018). Oregon iMapInvasives Resources. Retrieved from: https://sites.google.com/site/orimapresources/ (accessed November 20, 2018).
Pataki, D. E., Carreiro, M. M., Cherrier, J., Grulke, N. E., Jennings, V., Pincetl, S., et al. (2011). Coupling biogeochemical cycles in urban environments: ecosystem services, green solutions, and misconceptions. Front. Ecol. Environ. 9, 27–36. doi: 10.1890/090220
Pejchar, L., and Mooney, H. A. (2009). Invasive species, ecosystem services and human well-being. Trends Ecol. Evol. 24, 497–504. doi: 10.1016/j.tree.2009.03.016
Pojar, J., and MacKinnon, A. (2016). Plants of the Pacific Northwest: Washington, Oregon, British Columbia, and Alaska. Partners Publishing.
Portland Parks Recreation (2018). Natural Area Vegetation. The City of Portland. Retrieved from: https://www.portlandoregon.gov/parks/39872 (accessed November 17, 2018).
Portland Parks Recreation (2019). Park Tree Inventory. Retrieved from: https://www.portlandoregon.gov/parks/article/629112
Portney, K. (2005). Civic Engagement and Sustainable Cities in the United States. Public Administration Rev. 65, 579–591.
Pyšek, P. (1998). Alien and native species in Central European urban floras: a quantitative comparison. J. Biogeography 25, 155–163. doi: 10.1046/j.1365-2699.1998.251177.x
Rosenzweig, M. L. (2005). Species Diversity in Space and Time. Cambridge: Cambridge University Press.
Savard, J.-P. L., Clergeau, P., and Mennechez, G. (2000). Biodiversity concepts and urban ecosystems. Landscape Urban Planning 48, 131–142. doi: 10.1016/S0169-2046(00)00037-2
Swanson, F. J., Kratz, T. K., Caine, N., and Woodmansee, R. G. (1988). Landform effects on ecosystem patterns and processes. Bioscience 38, 92–98. doi: 10.2307/1310614
Tait, C. J., Daniels, C. B., and Hill, R. S. (2005). Changes in species assemblages within the Adelaide metropolitan area, Australia, 1836–2002. Ecol. Appl. 15, 346–359. doi: 10.1890/04-0920
Taylor, S. M., and Santelmann, M. V. (2014). Comparing vegetation and soils of remnant and restored wetland prairies in the Northern Willamette Valley. Northwest Sci. 88, 329–343. doi: 10.3955/046.088.0407
Threlfall, C. G., Ossola, A., Hahs, A. K., Williams, N. S. G., Wilson, L., and Livesley, S. J. (2016). Variation in vegetation structure and composition across urban green space types. Front. Ecol. Evol. 4:66. doi: 10.3389/fevo.2016.00066
Turner, K., Lefler, L., and Freedman, B. (2005). Plant communities of selected urbanized areas of Halifax, Nova Scotia, Canada. Landscape Urban Planning 71, 191–206. doi: 10.1016/j.landurbplan.2004.03.003
Turner, M. G., and Gardner, R. H. (2001). Landscape Ecology in Theory and Practice. New York, NY: Springer-Verlag.
Tzoulas, K., Korpela, K., Venn, S., Yli-Pelkonen, V., Kazmierczak, A., Niemela, J., et al. (2007). Promoting ecosystem and human health in urban areas using Green Infrastructure: a literature review. Landscape Urban Planning 81, 167–178. doi: 10.1016/j.landurbplan.2007.02.001
United States Department of Agriculture (2018). Welcome to the PLANTS Database. USDA PLANTS. Retrieved from: https://plants.sc.egov.usda.gov/java/ (accessed November 5, 2018).
United States Department of Agriculture Soil Conservation Service (1983). Soil Survey of Multnomah County, Oregon.
United States Fish Wildlife Service (2018). Wetlands Mapper. Retrieved from: https://www.fws.gov/wetlands/Data/Mapper.html (accessed November 5, 2018).
Weems, C. M. (2016). Examining the Spatial Distribution of Park Access and Trajectories of Gentrification in Seattle, Washington 1990–2010.
Whittaker, R. H. (1970). Communities and Ecosystems, 2nd Edn. New York, NY: University of Washington Press.
Zengeya, T., Ivey, P., Woodford, D. J., Weyl, O., Novoa, A., Shackleton, R., et al. (2017). Managing conflict-generating invasive species in South Africa: challenges and trade-offs. Bothalia 47:2160. doi: 10.4102/abc.v47i2.2160
Keywords: plant communities, biodiversity, urban ecology, urban parks, green infrastructure, native species, invasive species, multivariate analysis
Citation: Talal ML and Santelmann MV (2019) Plant Community Composition and Biodiversity Patterns in Urban Parks of Portland, Oregon. Front. Ecol. Evol. 7:201. doi: 10.3389/fevo.2019.00201
Received: 21 February 2019; Accepted: 15 May 2019;
Published: 04 June 2019.
Edited by:
Bryan Lyle Brown, Virginia Tech, United StatesReviewed by:
Andrea Olive, University of Toronto, CanadaCopyright © 2019 Talal and Santelmann. This is an open-access article distributed under the terms of the Creative Commons Attribution License (CC BY). The use, distribution or reproduction in other forums is permitted, provided the original author(s) and the copyright owner(s) are credited and that the original publication in this journal is cited, in accordance with accepted academic practice. No use, distribution or reproduction is permitted which does not comply with these terms.
*Correspondence: Michelle L. Talal, bWljaGVsbGUudGFsYWxAZ21haWwuY29t
Disclaimer: All claims expressed in this article are solely those of the authors and do not necessarily represent those of their affiliated organizations, or those of the publisher, the editors and the reviewers. Any product that may be evaluated in this article or claim that may be made by its manufacturer is not guaranteed or endorsed by the publisher.
Research integrity at Frontiers
Learn more about the work of our research integrity team to safeguard the quality of each article we publish.