- 1Earth to Ocean Research Group, Department of Biological Sciences, Simon Fraser University, Burnaby, BC, Canada
- 2The Hakai Institute, Heriot Bay, BC, Canada
- 3Complex Environmental Systems Lab, Institute for Biodiversity, Resilience and Ecosystem Services, University of British Columbia-Okanagan, Kelowna, BC, Canada
The disproportionate effects of some species can drive ecosystem processes and shape communities. This study investigates how distributions of spawning Pacific salmon within streams, salmon consumers, and the surrounding landscape mediate the distribution of salmon carcasses to riparian forests and estuaries. This work demonstrates how carcass transfer can vary spatially, within and among watersheds, through differences in pink (Oncorhynchus gorbuscha) and chum (O. keta) salmon distributions within 16 streams on the central coast of British Columbia over a five-year period. Spawning pink salmon concentrated in the lower reaches of all streams, whereas chum salmon shifted from lower to upper stream reaches as the area of spawning habitat increased. Salmon carcasses transferred to riparian areas by gray wolves (Canis lupus) were concentrated in estuaries and lower stream reaches, particularly shallow reaches of larger streams surrounded by large meadow expanses. Black and grizzly bears (Ursus americanus and U. arctos) transferred higher numbers and proportions of salmon carcasses to riparian areas compared to wolves, transferred more carcasses in areas of higher spawning density, and tended to focus more on chum salmon. Riparian subsides were increasingly driven by bear-chum salmon associations in upper stream reaches. In addition, lower proportions of salmon carcasses were exported into estuaries when densities of spawning salmon were lower and spawning reaches of streams were longer. This study demonstrates how salmon subsidies vary between and within watersheds as a result of species associations and landscape traits, and provides a nuanced species-specific and spatially explicit understanding of salmon-subsidy dynamics.
Introduction
Cross-boundary resource exchanges in material, organisms and energy can form a major component of resource bases within ecosystems (Polis et al., 1997; Anderson et al., 2008). Some linkages can be driven by large-scale processes such as El Niño affecting ecosystems from the Galápagos Islands to Australia (Holmgren et al., 2001), or trans-oceanic winds bringing iron from African deserts to South American forests (Bristow et al., 2010). Other nutrient linkages can have more localized effects, such as reciprocal flows of invertebrates linking terrestrial and freshwater food webs (Power, 2001; Baxter et al., 2005). In some cases, landscape structure can play a key role in mediating the delivery of resources across ecosystems (Turner, 1989; Polis et al., 1997; Loreau and Holt, 2004).
Pacific salmon (Oncorhynchus spp.) provide one of the most ecologically important examples of broad-scale, cross-boundary life histories in the animal kingdom (Groot and Margolis, 1991; Janetski et al., 2009). Freshwater habitats mark the beginning and end of a life cycle mostly spent in productive oceanic feeding grounds. Semelparous salmon rear in and then return to natal streams as adults to reproduce, thereby importing mass quantities of marine-derived material into coastal ecosystems throughout the North Pacific Rim. A wide body of research has documented the importance of Pacific salmon in linking offshore marine productivity to coastal ecosystems (Cederholm et al., 1999; Gende et al., 2002; Naiman et al., 2002). After spawning, their nutrient-rich carcasses are dispersed along streams, estuaries, and into adjacent forests by consumers and the movement of water Cederholm et al., 1989; Payne and Moore, 2006; Quinn et al., 2009.
Black and grizzly bears (Ursus americanus and U. arctos) can transfer large quantities of salmon-derived material from streams to riparian forests (Frame, 1974; Hilderbrand et al., 1999; Reimchen, 2000, 2017). Bears exploit this predictable and accessible annual pulse of protein, which constitutes a crucial resource during their preparation for winter dormancy (Quinn et al., 2003; Hilderbrand et al., 2011). Gray wolves (Canis lupus) are another major consumer that depend on salmon and can transfer significant numbers of salmon carcasses to riparian areas (Darimont et al., 2003). Isotope evidence suggests that coastal wolves shift their diet from ungulates to salmon during fall spawning events (Darimont and Reimchen, 2002; Darimont et al., 2008). Salmon carcasses deposited in riparian forests by these consumers can increase soil organic content (Bartz and Naiman, 2005; Gende et al., 2007), elevate nutrient concentration, shift the diversity of riparian plant communities (Bilby et al., 2003; Hocking and Reynolds, 2011), and provide substantial resources to terrestrial invertebrate communities (Hocking et al., 2009, 2013). Although salmon play important roles in stream ecosystems as a source of nutrients and disturbance to stream beds through the action of digging redds (Janetski et al., 2009; Tiegs et al., 2009; Harding et al., 2014), considerable proportions of carcasses are also exported to estuaries (Gende et al., 2004b), which can elevate dissolved nutrient concentrations and provide substantial resource inputs into estuarine food webs (Cak et al., 2008; Harding and Reynolds, 2014a; Harding et al., 2015). However, differences in salmon nutrient input to estuaries likely vary as a function of spawner distributions and stream size as carcasses are usually transported limited distances downstream and are often retained within pools and organic debris within streams (Cederholm and Peterson, 1985; Minakawa and Gara, 2005; Strobel et al., 2009).
To date, Pacific salmon species have generally been grouped together by their effects as resource subsidies and sources of streambed disturbance despite inter-species variation in life histories (c.f. Service et al., 2018). While all Pacific salmon have the potential to subsidize coastal ecosystems to some extent, contrasts in how each species responds to different habitats may contribute to more complex relationships between carcass dispersal mechanisms and thus the subsidy potential of each salmon species (Hooper et al., 2005). A crucial consideration when assessing the subsidy potential of salmon is their distribution within streams. Variation in the distribution of live spawning salmon between species, or amongst streams, will influence the distribution of salmon-derived nutrients. The magnitude of salmon carcass transfer to riparian forests by consumers and the export of carcasses downstream may also vary spatially based on variability in spawning salmon distributions and landscape traits such as stream size and depth.
This paper tests how differences in the distribution of salmon species across heterogeneous landscapes can influence the dispersal of salmon carcasses in riparian and estuarine habitats. Analyses interpreted how patterns in the distribution of live salmon, and the dispersal of dead ones, varied across coastal watersheds of the Northeastern Pacific that span a natural gradient in size, salmon density and other characteristics. First, this paper tested for differences in how live spawning pink (O. gorbuscha) and chum (O. keta) salmon were distributed within streams. Focus was on these two salmon species because they constituted more than 95% of total salmon within our study area, and thus are most important as a resource subsidy. It was anticipated that spawning pink salmon would concentrate in lower stream reaches, and chum salmon in upper reaches, potentially because larger chum salmon can successfully navigate higher gradients and spawn in larger substrate sizes of upper stream reaches within these coastal watersheds (Hunter, 1959; Scott and Crossman, 1973; Hale et al., 1985; Raleigh and Nelson, 1985). Second, patterns in salmon carcass transfer to riparian areas from adjacent spawning reaches by black and grizzly bears and by gray wolves were assessed. Based on initial field observations and on the aforementioned prediction, it was expected that wolf-transferred carcasses would concentrate in lower reaches of larger streams and therefore consist disproportionately of pink salmon. It was also expected that bear-transferred carcasses would occur throughout spawning reaches, particularly in upper portions of streams, across all stream sizes and consist mainly of chum salmon given the bears' preference for the larger size of chum salmon (Frame, 1974). Based on previous work, it was predicted that the magnitude of consumer-transferred carcasses would correlate positively with salmon density (Quinn et al., 2003) and negatively with stream depth due to reduced consumer access to spawning salmon (Andersson and Reynolds, 2017a). The findings presented in this study illustrate how species-specific responses, functional associations between species, and habitat traits can mediate the subsidy effects of salmon across coastal landscapes.
Materials and Methods
Study Area
This study focused on 16 salmon-bearing watersheds within 45 km of Bella Bella (52°9′N, 128°8′W) on the central coast of British Columbia, Canada (Figure 1). This region lies within the Coastal Western Hemlock biogeoclimatic zone and receives some of the highest levels of precipitation on the continent (Pojar et al., 1991). Landscapes in this part of North America remain largely intact due to their remoteness, restricted access, governance by First Nations, and support from conservation coalitions (Price et al., 2009).
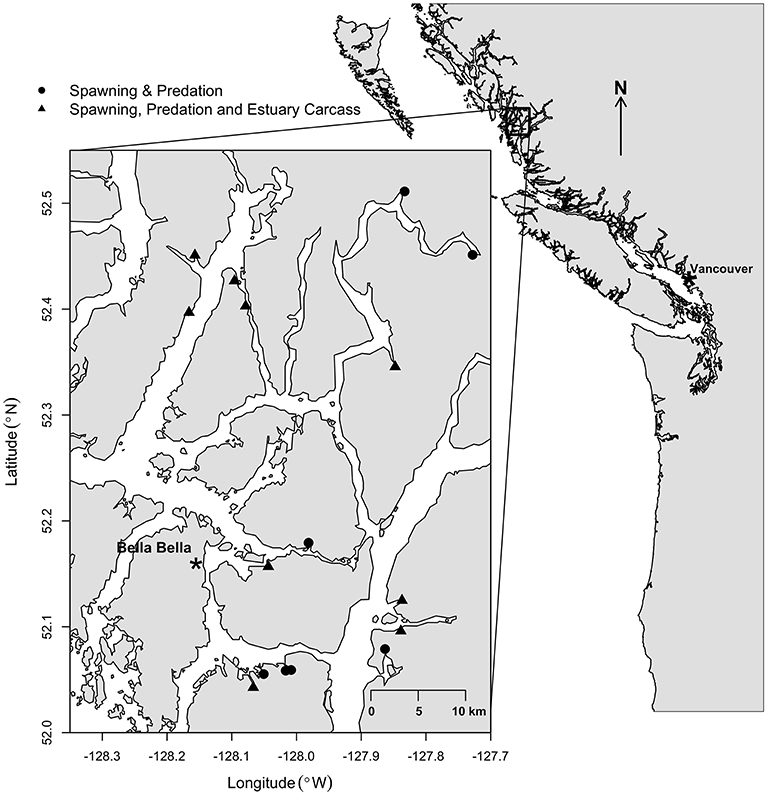
Figure 1. Study area in the vicinity of Bella Bella on British Columbia's central coast. Circles indicate spawning and carcass transfer study sites from 2009 to 2013; triangles indicate where estuary salmon carcasses were also counted in 2008 and 2009.
Salmon Surveys
Live and dead pink and chum salmon were enumerated over a period of 5 years (2009–2013) between the months of August and October. Not all streams were surveyed every year (Table A1). Analyses were limited to pink and chum salmon as these species dominate our study region and account for >95% of total adult salmon spawners, with much smaller numbers of coho (O. kisutch) and a limited presence of sockeye (O. nerka) and Chinook salmon (O. tshawytscha).
For each site, only one live and dead salmon survey per year was used in analyses and occurred as close to peak spawning periods as time permitted. When more than one count was completed in a given year the count that had the highest number of live spawning pink and chum salmon combined was used in analyses. Peak spawning periods, when the total numbers of actively spawning salmon were the highest, were identified from sites where multiple live spawner surveys had been completed and from stock assessment surveys conducted by the Department of Fisheries and Oceans Canada and local First Nations fisheries programs in the same region. The first section counted in each stream was the spawning habitat in lower stream reaches below the highest extent of tidal coverage, which varied in length (Table A1). The remaining spawning areas upstream of the estuaries were divided into sections ranging 50–200 m in length depending on stream size, resulting in 3–10 sections per stream (excluding the tidal sections). Sections were measured in 50 m lengths or less using range finders accurate to the nearest meter. Streams were divided into sections starting at the stream mouth and ending at the upstream limit of salmon spawning to assess patterns in live salmon and carcass (bear and wolf-transferred) distributions within and between watersheds. Live spawning salmon were surveyed in an upstream direction and dead fish when returning back downstream. The entire spawning reach of each stream was surveyed. Most of these terminated at impassable barriers such as waterfalls or logjams. Sites that did not have barriers to fish migration were surveyed upstream until there were no longer salmon present. Enumeration of live and dead salmon was by visual estimation from riverbanks when possible, and from within streams when bankside vantage points were not present (e.g., in canyons). Typically a 5–10 m length of stream was estimated at a time and totals were tallied once the end of a section was reached. At high densities, salmon were estimated in groups of tens to hundreds at a time and counted individually at lower densities. If weather conditions or turbidity prevented accurate enumeration, counts were omitted from analyses. Due to large differences in coloration and size, pink and chum salmon can easily be distinguished during counts. All crews were experienced in salmon enumeration and Fisheries and Oceans Canada (DFO) has integrated this spawner survey data into regional salmon escapement estimates. Variation in salmon body mass among different spawning populations was accounted for by weighing 5 dead adult salmon of each sex for each species from a subset of streams in our study region. Carcasses that were selected for weight were moribund or fresh pre-spawn mortalities. These mean salmon masses were applied to the remaining study sites that shared island groups, channels or mainland inlets to calculate carcass biomass.
Salmon carcasses were counted individually when possible and estimated in groups when necessary (e.g., bottom of large pools). Carcass categories were: senescent (spawned out), bear transferred (see below), wolf transferred, and unknown (Table A2). Carcasses were enumerated for each section of stream including a 10 m band of the riparian zone on either side of the stream channel for wolf- and bear-transferred carcasses, the riparian area known to contain the highest numbers of consumed carcasses (Cederholm et al., 1989). Senescent carcasses were identified as those that had no sign of consumption and were within stream channels or along banks. If the level of decomposition prohibited species identification or confirmation of consumption by bears or wolves, it was categorized as unknown.
Large differences between bear and wolf eating habits enable a considerable degree of certainty in determining which animal has consumed a salmon carcass. Bears consume multiple parts of a salmon including the brain, eggs and muscle tissue (Reimchen, 2000; Gende et al., 2004a), while wolves almost exclusively consume the brain in a surgical manner (Darimont et al., 2003). While these patterns may not be universal, they are supported by our own observations of active predation and scavenging within our study region (Field and Reynolds, 2013). Salmon carcasses that had been preyed upon or scavenged were categorized as follows: wolf-transferred carcasses were counted as having their heads or brains surgically removed, occasionally with parts of the jaws still attached (Figure 2), with no other part of the carcass consumed. Wolf-transferred carcasses could have smaller bite marks but lacked major rips and tears to the rest of the body. Bear-transferred carcasses were categorized as those that showed evidence of consumed eggs, bites and tears to body cavity and trunk muscle tissue, large bites or claw marks in the dorsal hump, and consumed brains (Figure 3, Andersson and Reynolds, 2017a,b). For carcass-transfer analyses (wolf and bear), carcasses were recorded as unknown and omitted from analyses when signs of consumption by bears or wolves could not be confirmed (including advanced states of decomposition). On average this comprised 36% of pink salmon carcasses and 22% of chum salmon carcasses across all streams and years.
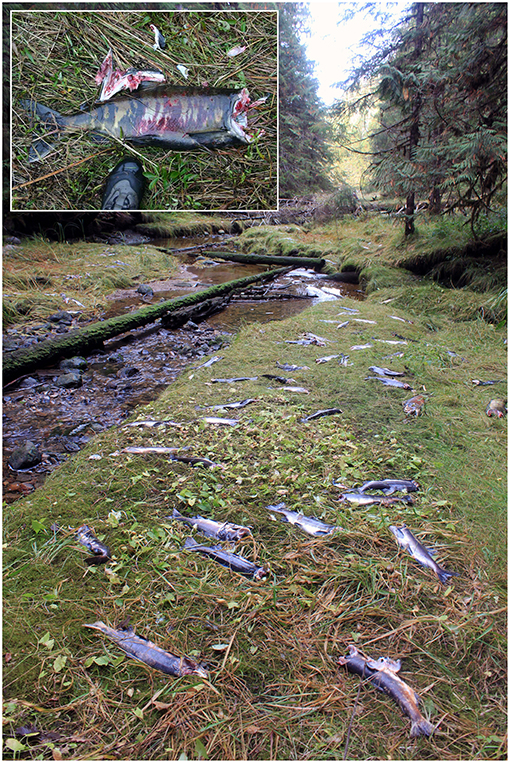
Figure 2. Wolf-transferred salmon carcasses with missing heads and minimal damage to body and trunk; no other parts of body consumed. Photo credit: Morgan Hocking (main), John Reynolds (inset).
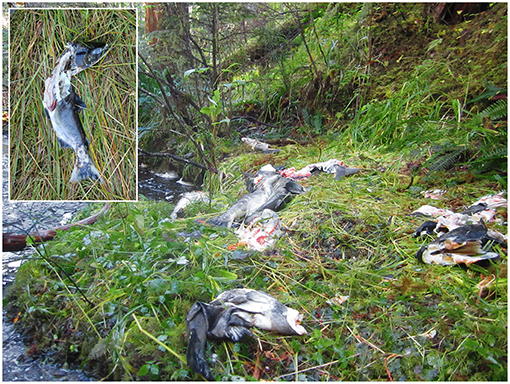
Figure 3. Bear-transferred carcasses with tears to body and trunk muscles tissue and eggs often consumed. Photo credit: Ben Rabinovitch (main), Morgan Hocking (inset).
Estuary Carcass Counts
In 2008 and 2009 intensive surveys were conducted for carcasses in the estuaries of a subset of nine of our study sites (Table A3). Intensive estuary surveys occurred separately from upstream counts because they were restricted to windows of low tide. All exposed carcasses and submerged carcasses to-2.4 m (below 0 m tide) were counted by species. Individual carcasses were counted whenever possible and estimated when there were large accumulations. For the latter, areas of carcass accumulations were measured and multiplied by mean carcass counts from several random 1 m2 quadrats subsampled from the accumulation. Wolves and bears were not considered in estuary carcass analyses, as it was difficult to discern whether a carcass was actually consumed within an estuary or had been flushed downstream from upstream reaches.
Habitat Characteristics
Habitat data were not collected every year as many of these metrics do not change substantially year-to-year. Habitat measurements included the area of spawning habitat, average stream depth, and estuary meadow area. Data that do vary annually, such as stream discharge and salmon spawning density, were measured each year. Spawning area (stream size) was calculated as the total length of spawning habitat within a stream multiplied by the mean wetted width. Water depth and wetted width were measured at 12 random transects along a study reach selected within each site. Water depth was measured at 11 systematic locations along the length of each transect and wetted width was measured as the distance along each transect (from bank to bank along the water's surface). Each transect ran perpendicular to streams bisecting flow. Each habitat study reach length was determined by multiplying the mean stream bankfull width (mean width of the stream channel at its highest point before flooding banks) by 30 (Bain and Stevenson, 1999). Estuary meadow area was measured by sketching meadow habitat over aerial photographs and calculating areas using the Government of British Columbia's mapping website iMapBC (Government of British Columbia, 2006). Stream discharge was measured during each of the peak salmon-spawning periods of 2008 and 2009 at three randomly selected transects (of the 12 established per site) in each stream using a Flo-Mate 2000™ portable flow meter. Flow measurements were recorded at 11 systematic locations along the length of each transect. Stream discharge, the cubic meters of water output per second, was calculated by multiplying stream flow by the cross-sectional areas of water at each transect location which was calculated from water depth measurements corresponding with each flow measurement location. Salmon densities were calculated as the total count of each salmon species divided by spawning reach or section area.
The number of wolves or bears was not determined at any of our sites. Similar to Quinn et al. (2003), this study was not examining the responses of wolves or bears to salmon density, but rather the spatial patterns of wolf and bear consumption of salmon and resulting subsidies to riparian areas. Although the number of consumers in a watershed would affect the total number of salmon transferred to riparian areas, these analyses were focused on the spatial patterns and overall magnitude of such transfers, including differences between wolves and bears, contrasts in subsidies of pink and chum salmon carcasses, and the relationships with basic physical characteristics of streams. It is possible our surveys may have influenced the natural behavior of consumers in these systems. However, most of these streams have been surveyed for years for salmon stock assessment purposes. In addition, surveys consistently covered the entire spawning length of each stream which limits the degree to which our presence might have biased the results.
Statistical Analyses
Generalized linear mixed-models were used to estimate the number of live spawners, wolf-transferred carcasses, and bear-transferred carcasses per section of stream. This accounted for the hierarchical structure (sections within streams) and non-normal distribution of count data (Bolker et al., 2009; Zuur et al., 2009). Models tested for the effects of distance upstream, stream size, and salmon species, including two-way interactions between all three variables. Dependent variables in these analyses were the number of live spawners, the number of wolf-transferred carcasses, and the number of bear-transferred carcasses per stream section for both chum and pink salmon. For wolf- and bear-transferred carcass analyses we also included salmon spawner density at the section level, average stream depth and total estuary meadow area. Analyses were conducted using the glmmADMB package in R (Skaug et al., 2010) using a negative binomial distribution with two random effects to account for intrinsic differences between watersheds and years. All models were competed using Akaike Information Criterion corrected for small sample sizes (AICc), which selects the most parsimonious model of the candidate set of models given the data. Zero-inflation parameters were included in live spawner and bear, but not wolf, analyses based on visual inspection of the data and the resulting lower AICc values of the global model (the model containing all covariates considered). All covariates were centered in all analyses to avoid inaccuracies in slope estimates for main effects as they can vary considerably depending on the presence of interaction terms (Schielzeth, 2010). A binary “dummy” variable was included in all analyses to investigate the differences between pink and chum salmon species (0 = pink salmon, 1 = chum salmon) following the recommendations of Schielzeth (2010). Multicollinearity amongst all variables was generally low, with all variance inflation factors <3 and Pearson correlation coefficients <0.6 (Zuur et al., 2009, 2010). Goodness of fit was assessed using the coefficient of determination R2. For live spawner and for wolf- and bear-transferred carcass analyses (GLMM) R2 values are presented as both marginal (the proportion of variance described by fixed effects) and conditional (the proportion of variance described by both fixed and random effects; Nakagawa et al., 2017). For the estuary carcass analysis (GLM) a single coefficient of determination R2 is presented as no random effects were considered (Tjur, 2009; Zhang, 2018). The open-source statistical software R was used for all analyses (R Core Team, 2016).
Multi-model approaches were used for wolf and bear analyses as top model weights were below 0.95 (Burnham and Anderson, 2002). For multi-model inference, models were constructed with scaled covariates (mean of 0 and standard deviation of 2) to enable direct comparison of effect sizes amongst covariates between wolf and bear analyses (Gelman, 2008; Grueber et al., 2011; Barton, 2012). Two stream section counts of live salmon were omitted from wolf and bear analyses due to leverage of the effects of salmon density (Crawley, 2007). These were the two highest values of pink salmon section densities (6.4 and 7 salmon/m2; the range of remaining data for pink and chum salmon combined was 0–2.8 salmon/m2). Candidate models were limited to the subset of models with a ΔAICc <4 (Burnham and Anderson, 2002). Parameter estimates for each variable were averaged across the candidate model set using the natural average method. Top model weights for both live spawner and estuary carcasses analyses were 0.99 and did not require model averaging.
To quantify the magnitude of salmon carcass inputs in estuaries, generalized-linear models were used to estimate the ratio of salmon that terminated in estuaries as carcasses for each watershed. The total abundance of salmon within a stream was used in this analysis as opposed to section-specific data. Estimates of the total number of salmon in each stream were generated by DFO using the area-under-the-curve method (AUC) from salmon counts conducted by the Heiltsuk First Nation, Simon Fraser University and DFO (Irvine et al., 1992). When insufficient counts were completed for AUC estimation, peak abundance estimates were used, which are strongly correlated with AUC estimates in these streams (Hocking and Reynolds, 2011). A binomial distribution was used for proportional carcass data and re-fit with a quasibinomial to address over dispersion observed in model residuals. This did not change coefficient estimates but did increase standard errors around the estimates. Models were ranked using AICc and quasi-information criterion for small sample sizes (QICc) for binomial and quasibinomial models, respectively (Lee and Nelder, 1999; Anderson and Burnham, 2002; Bolker, 2017). Both AICc and QICc model rankings and weights were identical.
Results
In our study area, mean salmon weights in different streams for pink and chum salmon ranged from 0.9 to 1.3 kg and 2.5 to 3.6 kg, respectively. Over 5 years (2009–2013), a total of 718 sections of stream were surveyed for live and dead salmon. Salmon counts ranged from 0 to 7,200 and from 0 to 1,990 per section for live pink and chum salmon spawners, 0 to 333 and 0 to 22 for wolf-transferred pink and chum salmon carcasses, and 0 to 165 and 0 to 194 for bear-transferred pink and chum salmon carcasses, respectively. Estuary carcass counts (2008 and 2009) ranged from 0 to 21,909 and from 8 to 7,820 for pink and chum salmon carcasses, respectively. The supplementary material provides more detailed site-level summaries and model specifics.
Live Spawning Salmon
In small streams, both spawning pink and chum salmon were most abundant in lower stream reaches. However, as spawning area increased, pink salmon remained in lower reaches while chum salmon moved into upper stream reaches (Figure 4). The best model predicting spawner distributions had an Akaike weight >0.99, a marginal R2 of 0.22, a conditional R2 of 0.48, and contained all variables considered including distance upstream, total spawning area, and salmon species (Table A4).
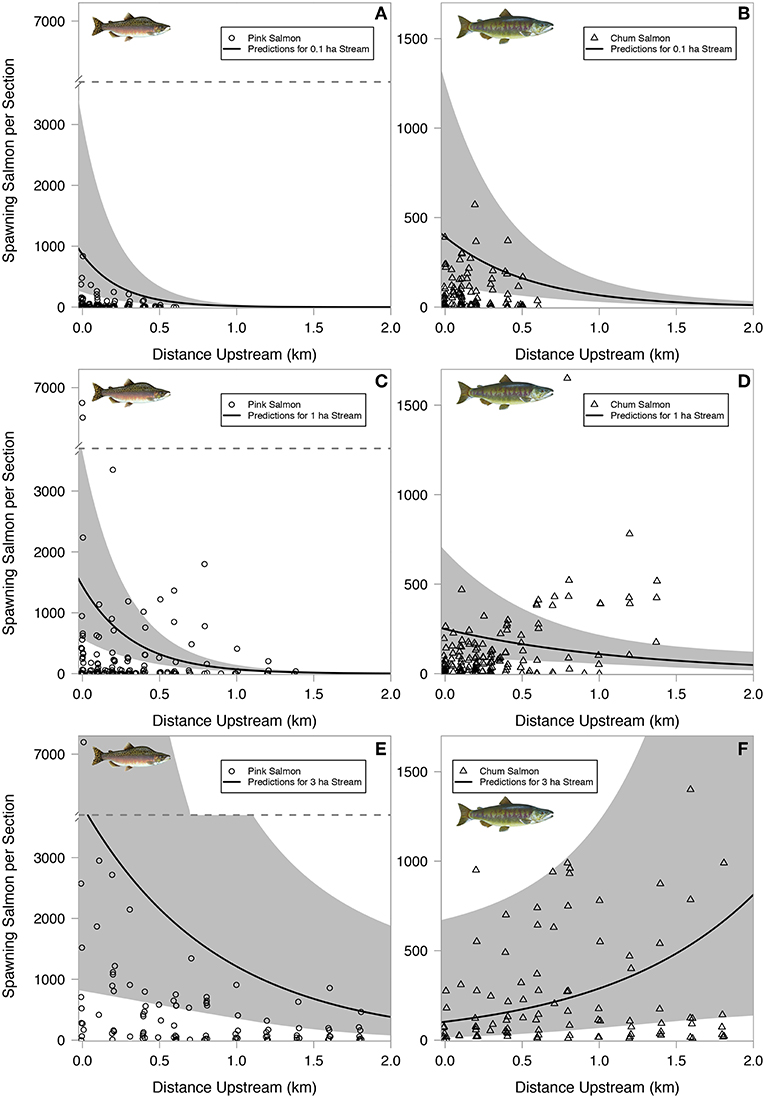
Figure 4. Live chum and pink salmon distributions. Pink salmon in left column, chum salmon in right column. The top row (A,B) shows observed data points for small streams, defined as the lower 25th percentile of stream areas. The prediction lines are for the top model and are based on a stream with 0.1 ha spawning area. The middle row (C,D) illustrates a medium-sized stream, with observed data points between the 25th and 75th percentile of stream areas, and prediction lines for a 1 ha spawning area stream. The bottom row (E,F) shows observed data points above the 75th percentile of stream sizes with prediction lines for a stream with a 3 ha spawning area. Shaded polygons indicate 95% confidence bands around model predictions.
Carcass Transfers to Riparian Areas
A total of 1,424 and 3,871 wolf- and bear-transferred carcasses were identified over 5 years, respectively. Wolves transferred over three times more pink salmon (1,125) than chum salmon (299) carcasses. Bears transferred approximately half the number of pink salmon (1,079) compared to chum salmon (2,792) carcasses (Figures 5A,B). The proportion of salmon carcasses transferred by wolves within each section did not vary by salmon species, and bears transferred higher overall proportions of salmon than wolves, in particular chum salmon (Figures 5C,D). Stream-level proportions of wolf-transferred carcasses ranged from 0 to 3.6% and from 0 to 8.1% for pink and chum salmon, respectively. Total proportions of bear-transferred carcasses ranged between 0 and 9.6% and 0 and 23.3% for pink and chum salmon, respectively. These estimates are low given the limited 10 m band of riparian area that was surveyed and the discounting of unknown carcasses. The number of wolf-transferred carcasses decreased with increasing distance upstream for both pink and chum salmon (Figure 6A). Bear-transferred pink salmon carcasses decreased similarly, but to a lesser degree than wolf carcasses, while bear-transferred chum salmon carcasses increased in upstream sections (Figure 6B). Salmon density had a much lower effect on the magnitude of wolf-transferred carcasses than those transferred by bears. Wolf-transferred pink salmon carcasses increased only slightly at higher pink salmon densities (Figure 6C). The number of bear-transferred carcasses for both salmon species increased with spawner density (Figure 6D).
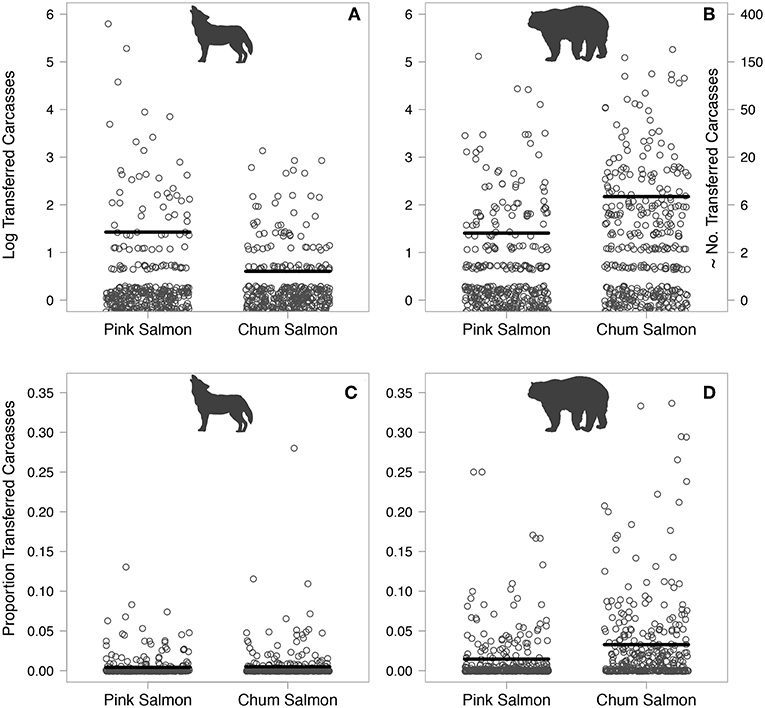
Figure 5. Log-transformed counts of wolf- (A) and bear-transferred (B) carcasses for each salmon species per stream section. Proportion of total salmon consumed by wolves (C) and bears (D) per stream section. Proportions were calculated as the number of wolf- or bear-consumed carcasses relative to total salmon (sum of wolf and bear consumed, senescent and live) per stream section. Horizontal lines indicate mean values. Data points are jittered horizontally for display purposes. Gaps in data are a result of log-transformation.
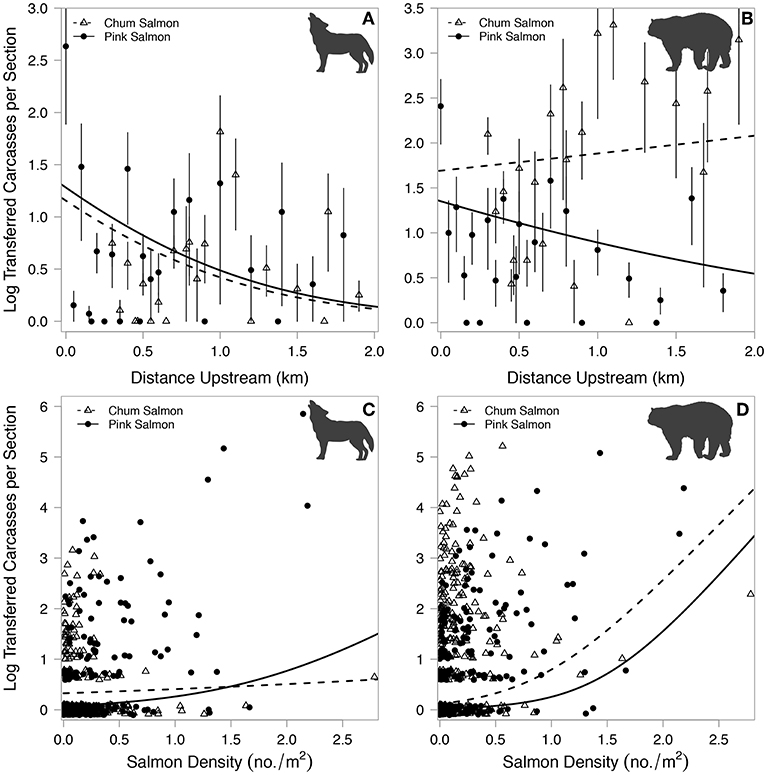
Figure 6. Log-transformed counts of wolf- (A) and bear-transferred (B) carcasses for each salmon species per stream section with increasing distance upstream. Log-transformed counts of wolf- (C) and bear-transferred (D) carcasses for each salmon species per stream section with increasing salmon density. Data points are mean values with 95% confidence intervals for panels (A,B) and raw data points for (C,D). Lines represent model predictions for each species with all other covariates held at mean values.
The strongest correlates of wolf-transferred salmon were habitat characteristics; riparian areas adjacent to larger and shallower spawning areas and surrounded by larger estuary meadows contained the largest numbers of wolf-transferred carcasses (Figure 7A). The negative correlation between distance upstream and the number of wolf-transferred carcasses did not change with stream size but did strengthen at higher salmon densities (Figure 7A). For bear-transferred carcasses the negative correlation with distance was only notable when associated with pink salmon carcasses (Figure 7B). Bear consumption of salmon increased in streams with larger spawning areas but stream depth and estuary meadow area had negligible or uncertain effects (Figure 7B). General patterns suggest that habitat traits drive wolf transfer of salmon carcasses while salmon density and species drive patterns in bear carcass transfers to riparian areas. Akaike weights for the top wolf- and bear-transferred carcass models were 0.59 (marginal R2 of 0.32 and a conditional R2 of 0.50) and 0.10 (marginal R2 of 0.17 and a conditional R2 of 0.38), with candidate sets consisting of 3 and 30 models, respectively (Anderson and Burnham, 2002; Burnham and Anderson, 2002; Table A5).
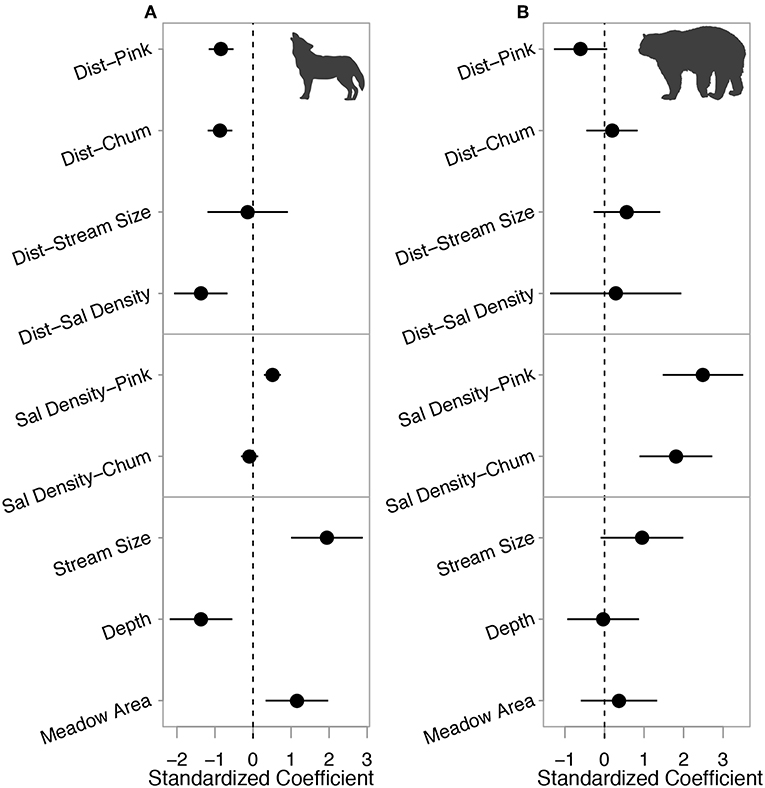
Figure 7. Standardized coefficients (mean = 0, standard deviation = 2) with 95% confidence intervals for all covariates considered in the (A) wolf candidate model set and (B) bear candidate model set. Coefficient values indicate the average change in number of transferred carcasses per stream section as the associated covariate values increase by 2 standard deviations. Dist = distance upstream (km), Sal Density = salmon density (no./m2), Stream Size = total spawning area (m2). The top two rows show the relative effects of distance upstream on both wolf- and bear-transferred pink and chum carcasses as presented in Figures 6A,B. The two middle rows, isolated by horizontal lines, show the relative effect sizes of salmon density as presented in Figures 6C,D. Two variables separated by a hyphen indicate the effect of the interaction between those two covariates on wolf- or bear-transferred carcasses.
Salmon Carcass Inputs Into Estuaries
The proportion of salmon carcasses that reached estuaries decreased with longer spawning reaches in streams. This negative correlation was stronger for pink salmon than chum salmon, opposite to our predictions (Figures 8A,B). The most parsimonious model describing the proportion of salmon carcasses in estuaries had a weight of evidence of 0.99, a R2 of 0.67, and included upstream salmon density, spawning length, and an interaction between spawning length and salmon species (Table A6). Stream discharge and year were absent from this model. Estuaries below streams with spawning reaches < ~1 km had higher proportions of pink salmon carcasses while those below longer streams had higher proportions of chum salmon carcasses (Figure 8B). Higher upstream salmon densities increased the proportion of carcasses in estuaries. However, this relationship was related to the length of spawning reach (Figure 8C). Total numbers of estuary carcasses were highly variable between the 2 years. Carcass numbers, total carcass biomass, and biomass density (kg of salmon carcass per m2) were much lower in 2008 than 2009, the latter of which was a high pink salmon-return year (Figures 9A–C). Chum salmon comprised the majority of 2008 carcass inputs into estuaries but pink salmon comprised the majority of carcasses in 2009, and for both years combined, even when correcting for differences in salmon size (carcass biomass) and estuary size (biomass density).
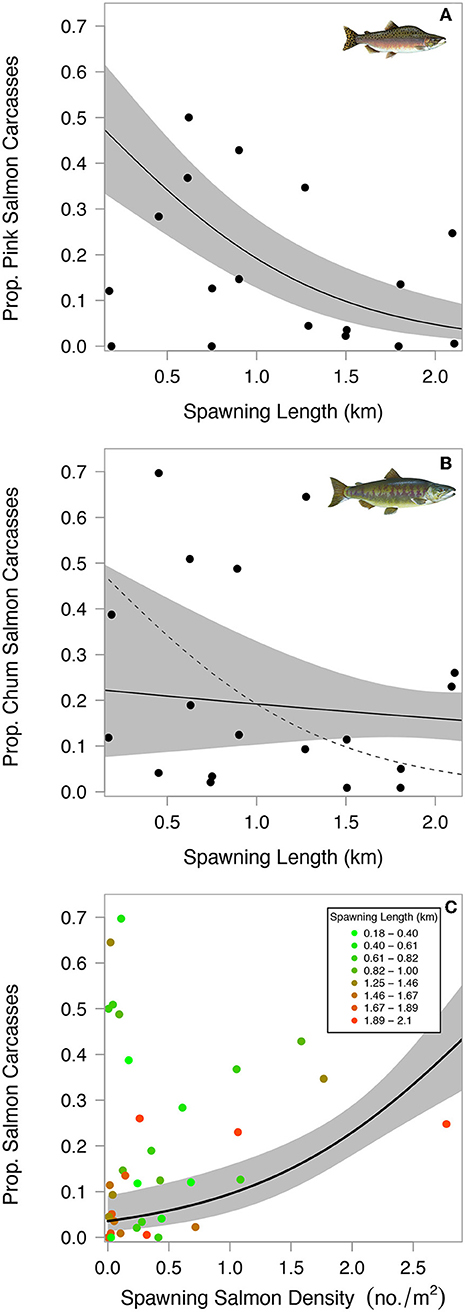
Figure 8. Proportion of salmon carcasses in estuaries relative to total spawning channel length for pink salmon (A) and chum salmon (B). Proportion of salmon carcasses in estuaries as a function of total salmon density (C). Raw data points are colored according to spawning channel length. Solid lines and shaded polygons show model predictions and 95% confidence bands with all other covariates held at mean values. The dotted line in panel B shows pink model trend line for visual comparison between salmon species, indicating higher proportions of chum carcasses end up in estuaries when the total length of spawning channel exceeds approximately 1 km.
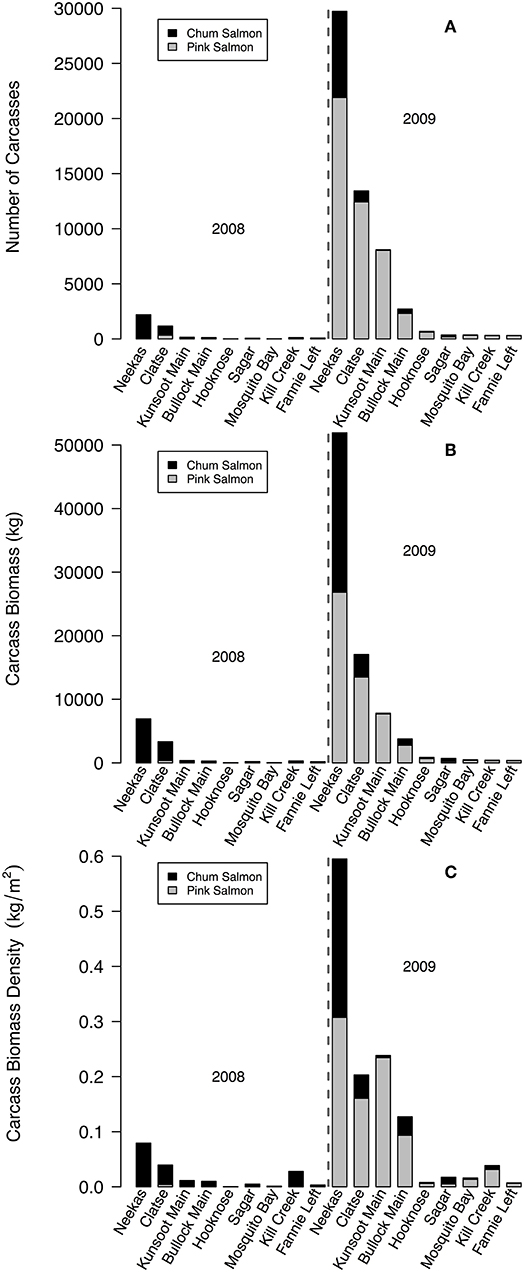
Figure 9. Contributions of salmon carcasses to each estuary for 2008 (a low pink return year) and 2009 (a high pink return year), indicating (A) total number of carcasses; (B) total biomass of carcasses (kg); and (C) biomass density of carcasses (kg/m2). Salmon biomass was calculated by measuring the weight of 5 dead adult salmon of each sex for each species in a subset of study streams covering our study area in 2009. Biomass densities are calculated for the total area of exposed estuary at a 0 m tide.
Discussion
The distributions of live pink and chum salmon were mediated by stream size, with the two species diverging in spawning distributions as stream size increased. Although these salmon species share similar spawning habitat requirements (Nelson et al., 2015), larger chum salmon may not be as limited in terms of suitable spawning habitat types. The larger size of chum salmon may enable them to access and successfully spawn in reaches with more variable discharge (Neave, 1966a), higher water velocities (Hale et al., 1985; Raleigh and Nelson, 1985), and larger spawning-substrate sizes (Hunter, 1959; DeVries, 1997). Chum salmon may occupy upper reaches in larger streams as a density-dependent response to saturated habitat by pink salmon in lower reaches or because offspring can survive periods of high substrate scour in larger substrate sizes (Montgomery et al., 1996). Alternatively, higher peak discharge, or scour depths in upper reaches of larger catchments could constrain pink salmon to downstream areas. It is also possible that pink salmon prefer smaller particle sizes and plane-bed channels of lower reaches while chum salmon prefer upstream pool-riffle channel structures (Neave, 1966b; Montgomery et al., 1999). These contrasts in distribution suggest variation in subsidy potential among salmon species in adjacent riparian and estuarine landscapes, and have not been considered previously.
The expectation that there would be more wolf-transferred salmon in estuaries and lower stream reaches was validated, but the prediction that pink salmon would constitute the majority of wolf- transferred carcasses was not supported. Wolf transfer of salmon carcasses was strongly driven by habitat characteristics, such as shallow reaches of larger streams surrounded by large open meadows, and not solely by the density or species of salmon. This could be a result of habitat preference as salmon consumption by gray wolves has been observed to be concentrated in estuaries (Darimont et al., 2003, 2008). Habitat partitioning between wolves and bears may also explain this pattern as dietary partitioning has been documented among these terrestrial consumers (Merkle et al., 2017).
Bear-transferred carcasses showed a different pattern. Distance upstream correlated negatively with the number of bear-transferred pink salmon carcasses but showed a negligible relationship with chum salmon carcasses. Bears exhibited a strong foraging preference for chum salmon, possibly due to their larger size as suggested by Frame (1974). This bias could also be an artifact of the observation that bears consume salmon throughout stream reaches, of which chum salmon increasingly dominate in upper reaches of larger streams. Our analyses suggest that, on average, bears transfer more chum salmon than pink salmon carcasses to riparian zones throughout stream reaches, regardless of stream size. In addition, the disparities between bear-transferred pink and chum salmon carcasses in riparian areas increased with distance upstream. This, supported by previous work showing that bear carcass transfers are density-dependent (Quinn et al., 2003), suggests that bears are going to areas with the highest spawning salmon densities, particularly of chum salmon, and that stream habitat traits are of less importance. Our expected effect of stream depth differed from work in Alaska by Quinn et al. (2009), who reported larger percentages of carcass transfers in deeper streams. In our study, depth did not influence amounts of bear carcass transfers, but deeper streams did correlate with reduced numbers of wolf transfers. Our contrasting results could relate to differences in stream depth, fish distribution or other habitat characteristics between Alaska and British Columbia. Reductions in wolf carcass transfers may reflect their limited ability to catch salmon in deeper stream reaches. Andersson and Reynolds (2017b) also found that bears are more likely to selectively consume higher quality portions of salmon carcasses in narrow, shallow streams, which may play an indirect role in the amount of salmon material that is deposited in riparian areas to some degree.
Overall, the total quantity of salmon carcasses transferred to riparian zones, by wolves and bears combined, was highest in estuaries and declined upstream. This upstream decline was a result of decreases in wolf-transferred carcasses and corresponding reductions in the number of pink salmon carcasses transferred by bears. Therefore, the magnitude of salmon subsidies per stream section was roughly equal between salmon species in lower reaches, with contributions from both wolves and bears, but shifted to predominance of bear-transferred chum salmon as distance upstream increased. Overall, subsidy potential may be higher with chum salmon carcasses given their larger size, but this is contingent on the amount of carcass left by consumers. Somewhat surprisingly, a study in the same region by Andersson and Reynolds (2017b) showed that selective consumption of carcasses by bears does not depend on the abundance of spawning salmon in these streams. Future studies that investigate how abundances of bears and wolves drive the magnitude of riparian salmon subsidies would be helpful. Studies that attempt to connect the amount of salmon transferred out of streams by consumers to how many carcasses ultimately reach estuaries, or consider differences in riparian subsides between streams that are dominated by grizzly bears vs. black bears, would also be of interest. Notably, the use of a 10 m-wide riparian band to assess carcass transfers is appropriate as the majority of carcass transfers are thought to occur within the first 10 m of riparian area adjacent to streams (Cederholm et al., 1989); however, bears also transport salmon farther into adjacent forests, and this distance can vary with the salmon density, fish freshness, and bear social hierarchies (Reimchen, 2000; Gende and Quinn, 2004; Quinn et al., 2009).
The prediction that salmon carcass inputs into estuaries were dominated by pink salmon as a result of spawning pink salmon concentrating in lower stream reaches was observed. However, the prediction that, as stream length increased, proportions of pink salmon carcasses in estuaries would remain relatively stable while the proportion of chum salmon carcasses would decrease was not supported by the data. Results showed that pink salmon carcass proportions decreased more than chum salmon carcasses as stream length increased. Although carcasses have been shown to travel short distances (Cederholm and Peterson, 1985; Cederholm et al., 1989; Minakawa and Gara, 2005; Strobel et al., 2009) high discharge events can cause longer distance carcass transport (Glock et al., 1980). Thus, high rainfall and variable discharge regimes in our study region, which were not necessarily captured by our spot flow measurements, may export larger proportions of carcasses from upstream reaches. However, lower than expected proportions of pink salmon carcasses below longer streams may be explained if portions of pink salmon carcasses are washed seaward out of intertidal areas due to their smaller size. Alternative salmon metrics, such as biomass per unit stream discharge, may be more appropriate for other salmon inputs such as particulate matter or dissolved nutrients (Johnston et al., 2004; Cak et al., 2008).
Total carcass inputs into estuaries differed greatly between the 2 years. This could be driven by differences in pink salmon abundances that cycle between even and odd years. In 2008, a low pink salmon-return year, carcass inputs were dominated by chum salmon and total numbers were much lower than the following pink salmon-dominant year. Over both years combined, total carcass inputs were dominated by pink salmon. This suggests that pink salmon populations could drive longer-term patterns in estuarine responses to salmon carcass subsidies (Harding et al., 2015). It is also possible that the comparatively prolonged spawning seasons of chum salmon could bias our carcass counts if the majority of chum salmon were still alive during surveys. However, the higher numbers of live pink salmon observed in lower reaches of these streams during surveys, and the fact that chum salmon generally start spawning earlier than pink salmon within our study region, support our confidence in these results (Neave, 1966a). Further, notable separation of spawning periods between salmon species was not observed within sites during data collection.
This study has demonstrated how the potential effects of salmon nutrient subsidies in coastal ecosystems vary within and across landscapes, by species of salmon, and through associations with major terrestrial consumers. Studies do not currently consider taxonomic variability in salmon-subsidy potential or spatial variability beyond average, site-level salmon density metrics and comparisons of above and below salmon migration barriers (Hocking and Reimchen, 2002; Mathewson et al., 2003; Harding and Reynolds, 2014b). Our analyses could provide a framework to guide future studies that investigate productivity responses to salmon subsidies in coastal systems, and specifically those that consider how subsidy effects on recipient ecosystems might be influenced by patterns in spawning salmon density and distribution in concert with the presence of terrestrial consumers and habitat characteristics. Such studies will further improve our understanding of complex, multi-scale ecosystem dynamics and processes. This work also highlights the importance of sound management decisions in the conservation and protection of salmon and populations of large terrestrial consumers to maintain ecologically important functional associations and mechanistic processes that link offshore marine productivity with coastal forests and estuaries (Chapin et al., 1997; Helfield and Naiman, 2006; Artelle et al., 2013).
Ethics Statement
This study was carried out in accordance with the recommendations of Canadian Council on Animal Care Guidelines, Simon Fraser University Animal Care Committee. The protocol was approved by the Simon Fraser University Animal Care Committee.
Author Contributions
JMSH and JR conceptualized the study. JMSH, JNH, RF, JP, NS, and MW collected the data. JMSH conducted and interpreted analyses and drafted the paper. JNH, RF, JP, NS, MW, and JR critically reviewed and revised the manuscript.
Funding
This research was supported by the Natural Sciences and Engineering Research Council and the Tom Buell BC Leadership Chair endowment funded by the Pacific Salmon Foundation and the BC Leading Edge Endowment Fund. Support was also received from the Tula Foundation, including a scholarship to JMSH through the Hakai Institute.
Conflict of Interest Statement
The authors declare that the research was conducted in the absence of any commercial or financial relationships that could be construed as a potential conflict of interest.
Acknowledgments
We are grateful to everyone who provided countless hours of field support including Kyle Emslie, Johanna Gordon-Walker, Tess Grainger, Ryan Midgley, Ralph Nelson, Danny O'Farrell, Heather Recker, Michelle Segal, Mark Spoljaric, Morgan Stubbs, and Dan Wagner. We thank the Heiltsuk First Nation and Fisheries and Oceans Canada (DFO) for providing salmon count data, valuable input, and logistical support. Finally, we thank Sean Anderson, Michael Beakes, Doug Braun, Brendan Connors, Nick Dulvy, Rick Routledge, the Earth to Ocean Research Group and Stats-beerz for help with statistical analyses and insightful comments. Salmon, wolf, and bear images used under Public Domain Dedication 1.0 license (Details in Table A7). Content in this manuscript first appeared in the lead author's doctoral dissertation (Harding, 2015).
Supplementary Material
The Supplementary Material for this article can be found online at: https://www.frontiersin.org/articles/10.3389/fevo.2019.00192/full#supplementary-material
References
Anderson, D. R., and Burnham, K. (2002). Avoiding pitfalls when using information-theoretic methods. J Wildlife Manage. 66, 912–918. doi: 10.2307/3803155
Anderson, W. B., Wait, D. A., and Stapp, P. (2008). Resources from another place and time: responses to pulses in a spatially subsidized system. Ecology 89, 660–670. doi: 10.1890/07-0234.1
Andersson, L. C., and Reynolds, J. D. (2017a). Effects of habitat features on size-biased predation on salmon by bears. Oecologia 184, 101–114. doi: 10.1007/s00442-017-3845-0
Andersson, L. C., and Reynolds, J. D. (2017b). Habitat features mediate selective consumption of salmon by bears. Can. J. Fish. Aquatic Sci. 75, 955–963. doi: 10.1139/cjfas-2017-0055
Artelle, K. A., Anderson, S. C., Cooper, A. B., Paquet, P. C., Reynolds, J. D., and Darimont, C. T. (2013). Confronting uncertainty in wildlife management: performance of grizzly bear management. PLoS ONE 8:e78041. doi: 10.1371/journal.pone.0078041
Bain, M. B., and Stevenson, N. J. (1999). Aquatic Habitat Assessment. Maryland: American Fisheries Society.
Bartz, K., and Naiman, R. J. (2005). Effects of salmon-borne nutrients on riparian soils and vegetation in southwest Alaska. Ecosystems 8, 529–545. doi: 10.1007/s10021-005-0064-z
Baxter, C. V., Fausch, K. D., and Saunders, C. W. (2005). Tangled webs: reciprocal flows of invertebrate prey link streams and riparian zones. Freshwater Biol. 50, 201–220. doi: 10.1111/j.1365-2427.2004.01328.x
Bilby, R. E., Beach, E. W., Fransen, B. R., Walter, J. K., and Bisson, P. A. (2003). Transfer of nutrients from spawning salmon to riparian vegetation in western Washington. T. Am. Fish. Soc. 132, 733–745. doi: 10.1577/T02-089
Bolker, B. (2017). Dealing With Quasi-Models in R.: 1–5. Available online at: https://mirror.its.sfu.ca/mirror/CRAN/web/packages/bbmle/vignettes/quasi.pdf
Bolker, B. M., Brooks, M. E., Clark, C. J., Geange, S. W., Poulsen, J. R., Stevens, M. H. H., et al. (2009). Generalized linear mixed models: a practical guide for ecology and evolution. Trends Ecol. Evol. 24, 127–135. doi: 10.1016/j.tree.2008.10.008
Bristow, C. S., Hudson-Edwards, K. A., and Chappell, A. (2010). Fertilizing the amazon and equatorial atlantic with west african dust. Geophys. Res. Lett. 37:L14807. doi: 10.1029/2010GL043486
Burnham, K. P., and Anderson, D. R. (2002). Model Selection and Multimodel Inference: A Practical Information-Theoretic Approach. New York, NY: Springer Science and Business Media.
Cak, A. D., Chaloner, D. T., and Lamberti, G. A. (2008). Effects of spawning salmon on dissolved nutrients and epilithon in coupled stream-estuary systems of southeastern Alaska. Aquat. Sci. 70, 169–178. doi: 10.1007/s00027-008-8090-5
Cederholm, C. J., Houston, D. B., Cole, D. L., and Scarlett, W. J. (1989). Fate of coho salmon (Oncorhynchus kisutch) carcasses in spawning streams. Can. J. Fish. Aquat. Sci. 46, 1347–1355. doi: 10.1139/f89-173
Cederholm, C. J., Kunze, M. D., Murota, T., and Sibatani, A. (1999). Pacific salmon carcasses: essential contributions of nutrients and energy for aquatic and terrestrial ecosystems. Fisheries 24, 6–15. doi: 10.1577/1548-8446(1999)024<0006:PSC>2.0.CO;2
Cederholm, C. J., and Peterson, N. P. (1985). The retention of coho salmon (Oncorhynchus kisutch) carcasses by organic debris in small streams. Can. J. Fish. Aquat. Sci. 42, 1222–1225. doi: 10.1139/f85-150
Chapin, I. I. I. F. S, Walker, B. H., Hobbs, R. J., Hooper, D. U., Lawton, J. H., Sala, O. E., et al. (1997). Biotic control over the functioning of ecosystems. Science 277, 500–504. doi: 10.1126/science.277.5325.500
Darimont, C. T., Paquet, P. C., and Reimchen, T. E. (2008). Spawning salmon disrupt trophic coupling between wolves and ungulate prey in coastal British Columbia. BMC Ecol. 8:14. doi: 10.1186/1472-6785-8-14
Darimont, C. T., and Reimchen, T. E. (2002). Intra-hair stable isotope analysis implies seasonal shift to salmon in gray wolf diet. Can. J. Zool. 80, 1638–1642. doi: 10.1139/z02-149
Darimont, C. T., Reimchen, T. E., and Paquet, P. C. (2003). Foraging behaviour by gray wolves on salmon streams in coastal British Columbia. Can. J. Zool. 81, 349–353. doi: 10.1139/z02-246
DeVries, P. (1997). Riverine salmonid egg burial depths: review of published data and implications for scour studies. Can. J. Fish. Aquat. Sci. 54, 1685–1698. doi: 10.1139/f97-090
Field, R. D., and Reynolds, J. D. (2013). Ecological links between salmon, large carnivore predation, and scavenging birds. J. Avian Biol. 44, 009–016. doi: 10.1111/j.1600-048X.2012.05601.x
Frame, G. W. (1974). Black bear predation on salmon at olsen creek, Alaska. Ethology 35, 23–38. doi: 10.1111/j.1439-0310.1974.tb00430.x
Gelman, A. (2008). Scaling regression inputs by dividing by two standard deviations. Statist. Med. 27, 2865–2873. doi: 10.1002/sim.3107
Gende, S. M., Edwards, R. T., Willson, M. F., and Wipfli, M. S. (2002). Pacific salmon in aquatic and terrestrial ecosystems. BioScience 52, 917–928. doi: 10.1641/0006-3568(2002)052[0917:PSIAAT]2.0.CO;2
Gende, S. M., Miller, A. E., and Hood, E. (2007). The effects of salmon carcasses on soil nitrogen pools in a riparian forest of southeastern Alaska. Can. J. Forest Res. 37, 1194–1202. doi: 10.1139/X06-318
Gende, S. M., and Quinn, T. P. (2004). The relative importance of prey density and social dominance in determining energy intake by bears feeding on Pacific salmon. Can. J. Zool. 82, 75–85. doi: 10.1139/z03-226
Gende, S. M., Quinn, T. P., Hillborn, R., Hendry, A. P., and Dickerson, B. (2004a). Brown bears selectively kill salmon with higher energy content but only in habitats that facilitate choice. Oikos 104, 518–528. doi: 10.1111/j.0030-1299.2004.12762.x
Gende, S. M., Quinn, T. P., Willson, M. F., Heintz, R., and Scott, T. M. (2004b). Magnitude and fate of salmon-derived nutrients and energy in a coastal stream ecosystem. J. Freshwater Ecol. 19, 149–160. doi: 10.1080/02705060.2004.9664522
Glock, J. W., Hartman, G., and Conquest, L. (1980). Skagit River Chum Salmon Carcass Drift Study. Seattle: University of Washington Center for Quantitative Science.
Government of British Columbia (2006). iMapBC. Integrated Land Management Buereau. Available online at: https://www2.gov.bc.ca/gov/content/data/geographic-data-services/web-based-mapping/imapbc
Grueber, C. E., Nakagawa, S., Laws, R. J., and Jamieson, I. G. (2011). Multimodel inference in ecology and evolution: challenges and solutions. J. Evol. Biol. 24, 699–711. doi: 10.1111/j.1420-9101.2010.02210.x
Hale, S. S., McMahon, T. E., and Nelson, P. C. (1985). Habitat Suitability Index Models and Instream Flow Suitability Curves: Chum Salmon. U.S. Fish and Wildlife Service. Biological Report, 82.
Harding, J. M., Segal, M. R., and Reynols, J. D. (2015). Location is everything: evaluating the effects of terrestrial and marine resource subsidies on an estuarine bivalve. PLoS ONE 10:e0125167. doi: 10.1371/journal.pone.0125167
Harding, J. M. S. (2015). From earth and ocean: Investigating the importance of cross-ecosystem resource linkages in estuaries of the Pacific Northwest. (dissertation). Burnaby, BC: Simon Fraser University
Harding, J. M. S., and Reynolds, J. D. (2014a). From earth and ocean: investigating the importance of cross-ecosystem resource linkages to a mobile estuarine consumer. Ecosphere 5:art54. doi: 10.1890/ES14-00029.1
Harding, J. N., Harding, J. M. S., and Reynolds, J. D. (2014). Movers and shakers: nutrient subsidies and benthic disturbance predict biofilm biomass and stable isotope signatures in coastal streams. Freshw. Biol. 59, 1361–1377. doi: 10.1111/fwb.12351
Harding, J. N., and Reynolds, J. D. (2014b). Opposing forces: evaluating multiple ecological roles of Pacific salmon in coastal stream ecosystems. Ecosphere 5:art157. doi: 10.1890/ES14-00207.1
Helfield, J. M., and Naiman, R. J. (2006). Keystone interactions: salmon and bear in riparian forests of Alaska. Ecosystems 9, 167–180. doi: 10.1007/s10021-004-0063-5
Hilderbrand, G. V., Hanley, T. A., Robbins, C. T., and Schwartz, C. C. (1999). Role of brown bears (Ursus arctos) in the flow of marine nitrogen into a terrestrial ecosystem. Oecologia 121, 546–550. doi: 10.1007/s004420050961
Hilderbrand, G. V., Schwartz, C. C., Robbins, C. T., Jacoby, M. E., Hanley, T. A., Arthur, S. M., et al. (2011). The importance of meat, particularly salmon, to body size, population productivity, and conservation of North American brown bears. Can. J. Zool. 77, 132–138. doi: 10.1139/cjz-77-1-132
Hocking, M. D., Dulvy, N. K., Reynolds, J. D., Ring, R. A., and Reimchen, T. E. (2013). Salmon subsidize an escape from a size spectrum. Proc. R. Soc. B 280:20122433. doi: 10.1098/rspb.2012.2433
Hocking, M. D., and Reimchen, T. E. (2002). Salmon-derived nitrogen in terrestrial invertebrates from coniferous forests of the Pacific Northwest. BMC Ecol. 2:4. doi: 10.1186/1472-6785-2-4
Hocking, M. D., and Reynolds, J. D. (2011). Impacts of salmon on riparian plant diversity. Science 331, 1609–1612. doi: 10.1126/science.1201079
Hocking, M. D., Ring, R. A., and Reimchen, T. E. (2009). The ecology of terrestrial invertebrates on Pacific salmon carcasses. Ecol. Res. 24, 1091–1100. doi: 10.1007/s11284-009-0586-5
Holmgren, M., Scheffer, M., Ezcurra, E., Gutiérrez, J. R., and Mohren, G. M. J. (2001). El Niño effects on the dynamics of terrestrial ecosystems. Trends Ecol. Evol. 16, 89–94. doi: 10.1016/S0169-5347(00)02052-8
Hooper, D. U., Chapin, I. I. I. F. S, Ewel, J. J., Hector, A., Inchausti, P., Lavorel, S., et al. (2005). Effects of biodiversity on ecosystem functioning: a consensus of current knowledge. Ecol. Monogr. 75, 3–35. doi: 10.1890/04-0922
Hunter, J. G. (1959). Survival and production of pink and chum salmon in a coastal stream. J. Fish. Res. Bd. Can. 16, 835–886. doi: 10.1139/f59-061
Irvine, J. R., Bocking, R. C., English, K. K., and Labelle, M. (1992). Estimating coho salmon (Oncorhynchus kisutch) spawning escapements by conducting visual surveys in areas selected using stratified random and stratified index sampling designs. Can. J. Fish. Aquat. Sci. 49, 1972–1981. doi: 10.1139/f92-219
Janetski, D. J., Chaloner, D. T., Tiegs, S. D., and Lamberti, G. A. (2009). Pacific salmon effects on stream ecosystems: a quantitative synthesis. Oecologia 159, 583–595. doi: 10.1007/s00442-008-1249-x
Johnston, N. T., MacIsaac, E. A., Tschaplinski, P. J., and Hall, K. J. (2004). Effects of the abundance of spawning sockeye salmon (Oncorhynchus nerka) on nutrients and algal biomass in forested streams. Can. J. Fish. Aquat. Sci. 61, 384–403. doi: 10.1139/f03-172
Lee, Y., and Nelder, J. A. (1999). The robustness of the quasilikelihood estimator. Can. J. Stat. 27, 321–327. doi: 10.2307/3315642
Loreau, M., and Holt, R. (2004). Spatial flows and the regulation of ecosystems. Am. Nat. 163, 606–615. doi: 10.1086/382600
Mathewson, D. D., Hocking, M. D., and Reimchen, T. E. (2003). Nitrogen uptake in riparian plant communities across a sharp ecological boundary of salmon density. BMC Ecol. 3:4. doi: 10.1186/1472-6785-3-4
Merkle, J. A., Polfus, J. L., Derbridge, J. J., and Heinemeyer, K. S. (2017). Dietary niche partitioning among black bears, grizzly bears and wolves in a multiprey ecosystem. Can. J. Zool. 95, 663–671.
Minakawa, N., and Gara, R. (2005). Spatial and temporal distribution of coho salmon carcasses in a stream in the Pacific Northwest, USA. Hydrobiologia 539, 163–166. doi: 10.1007/s10750-004-3367-8
Montgomery, D. R., Beamer, E. W., Pess, G. R., and Quinn, T. P. (1999). Channel type and salmonid spawning distribution and abundance. Can. J. Fish. Aquat. Sci. 56, 377–387. doi: 10.1139/cjfas-56-3-377
Montgomery, D. R., Buffington, J. M., Peterson, N. P., Schuett-Hames, D., and Quinn, T. P. (1996). Stream-bed scour, egg burial depths, and the influence of salmonid spawning on bed surface mobility and embryo survival. Can. J. Fish. Aquat. Sci. 53, 1061–1070. doi: 10.1139/cjfas-53-5-1061
Naiman, R. J., Bilby, R. E., Schindler, D. E., and Helfield, J. M. (2002). Pacific salmon, nutrients, and the dynamics of freshwater and riparian ecosystems. Ecosystems 5, 399–417. doi: 10.1007/s10021-001-0083-3
Nakagawa, S., Johnson, P. C. D., and Schielzeth, H. (2017). The coefficient of determination R2 and intra-class correlation coefficient from generalized linear mixed-effects models revisited and expanded. J. R. Soc. Interface 14:20170213. doi: 10.1098/rsif.2017.0213
Neave, F. (1966a). Salmon of the north pacific. Part III. A review of the life history of North Pacific salmon. 6: Chum salmon in British Columbia. Int. North Pacif. Fish. Comm. Bull. 18, 81–85.
Neave, F. (1966b). Salmon of the North Pacific. Part III. A review of the life history of North Pacific salmon. 5: Pink salmon in British Columbia. Int. North. Pacif. Fish. Comm. Bull. 18, 71–79.
Nelson, M. C., Hocking, M. D., Harding, J. N., Harding, J. M. S., and Reynolds, J. D. (2015). Quantifying the effects of stream habitat on populations of breeding Pacific salmon. Can. J. Fish. Aquat. Sci. 72, 1469–1476. doi: 10.1139/cjfas-2014-0253
Payne, L. X., and Moore, J. W. (2006). Mobile scavengers create hotspots of freshwater productivity. Oikos 115, 69–80. doi: 10.1111/j.2006.0030-1299.14899.x
Pojar, J., Klinka, K., and Demarchi, D. A. (1991). “Coastal western hemlock zone,” in Ecosystems of British Columbia, eds D. V. Meidinger, and J. Pojar (Victoria, BC: Research Branch, Ministry of Forests), 95–111.
Polis, G. A., Anderson, W. B., and Holt, R. D. (1997). Toward an integration of landscape and food web ecology: the dynamics of spatially subsidized food webs. Annu. Rev. Ecol. Syst. 28, 289–316. doi: 10.1146/annurev.ecolsys.28.1.289
Power, M. (2001). Prey exchange between a stream and its forest watershed elevates predator densities in both habitats. Proc. Natl. Acad. Sci. U.S.A. 98, 14–15. doi: 10.1073/pnas.98.1.14
Price, K., Roburn, A., and MacKinnon, A. (2009). Ecosystem-based management in the Great Bear Rainforest. For. Ecol. Manage. 258, 495–503. doi: 10.1016/j.foreco.2008.10.010
Quinn, T. P., Carlson, S. W., Gende, S. M., and Rich Jr., H. B. (2009). Transportation of Pacific salmon carcasses from streams to riparian forests by bears. Can. J. Zool. 87, 195–203. doi: 10.1139/Z09-004
Quinn, T. P., Gende, S. M., Ruggerone, G. T., and Rogers, D. E. (2003). Density-dependent predation by brown bears (Ursus arctos) on sockeye salmon (Oncorhynchus nerka). Can. J. Fish. Aquat. Sci. 60, 553–562. doi: 10.1139/f03-045
R Core Team (2016). R: A Language and Environment for Statistical Computing. Vienna: R Foundation for Statistical Computing.
Raleigh, R. F., and Nelson, P. C. (1985). Habitat Suitability Index Models and Instream Flow Suitability Curves: Pink Salmon. U.S. Fish and Wildlife Service. Biological Report 82.
Reimchen, T. E. (2000). Some ecological and evolutionary aspects of bear-salmon interactions in coastal British Columbia. Can. J. Zool. 78, 448–457. doi: 10.1139/z99-232
Reimchen, T. E. (2017). Diverse ecological pathways of salmon nutrients through an intact marine-terrestrial interface. Can. Field Natural. 131, 350–368. doi: 10.22621/cfn.v131i4.1965
Schielzeth, H. (2010). Simple means to improve the interpretability of regression coefficients. Methods Ecol. Evol. 1, 103–113. doi: 10.1111/j.2041-210X.2010.00012.x
Scott, W. B., and Crossman, E. J. (1973). Freshwater Fishes of Canada. Ottawa, ON: Fisheries Research Board of Canada.
Service, C. N., Bateman, A. W., Adams, M. S., Artelle, K. A., Reimchen, T. E., Paquet, P. C., and Darimont, C. T. (2018). Salmonid species diversity predicts salmon consumption by terrestrial wildlife. J. Anim. Ecol. 88, 392–404. doi: 10.1111/1365-2656.12932
Skaug, J. H., Fournier, D., Nielsen, A., Magnusson, A., and Bolker, B. (2010). glmmADMB: Generalized Linear Mixed Models Using AD Model Builder. R package version 0.6. 5. r143.
Strobel, B., Shively, D. R., and Roper, B. B. (2009). Salmon Carcass Movements in Forest Streams. North Amer. J. of Fish. Manage. 29, 702–714. doi: 10.1577/M08-144.1
Tiegs, S. D., Campbell, E. Y., Levi, P. S., Rüegg, J., Benbow, M. E., Chaloner, D. T., et al. (2009). Separating physical disturbance and nutrient enrichment caused by Pacific salmon in stream ecosystems. Freshw. Biol. 54, 1864–1875. doi: 10.1111/j.1365-2427.2009.02232.x
Tjur, T. (2009). Coefficients of determination in logistic regression models - a new proposal: The coefficient of discrimination. Am. Stat. 63, 366–372. doi: 10.1198/tast.2009.08210
Turner, M. G. (1989). Landscape ecology: the effect of pattern on process. Annu. Rev. Ecol. Syst. 20, 171–197. doi: 10.1146/annurev.es.20.110189.001131
Zuur, A. F., Ieno, E. N., and Elphick, C. S. (2010). A protocol for data exploration to avoid common statistical problems. Methods Ecol. Evol. 1, 3–14. doi: 10.1111/j.2041-210X.2009.00001.x
Keywords: cross-ecosystem, fisheries, landscape ecology, nutrient subsidies, pacific salmon
Citation: Harding JMS, Harding JN, Field RD, Pendray JE, Swain NR, Wagner MA and Reynolds JD (2019) Landscape Structure and Species Interactions Drive the Distribution of Salmon Carcasses in Coastal Watersheds. Front. Ecol. Evol. 7:192. doi: 10.3389/fevo.2019.00192
Received: 02 November 2018; Accepted: 13 May 2019;
Published: 07 June 2019.
Edited by:
M. Eric Benbow, Michigan State University, United StatesReviewed by:
Scott D. Tiegs, Oakland University, United StatesMichael J. Anteau, United States Geological Survey, United States
Copyright © 2019 Harding, Harding, Field, Pendray, Swain, Wagner and Reynolds. This is an open-access article distributed under the terms of the Creative Commons Attribution License (CC BY). The use, distribution or reproduction in other forums is permitted, provided the original author(s) and the copyright owner(s) are credited and that the original publication in this journal is cited, in accordance with accepted academic practice. No use, distribution or reproduction is permitted which does not comply with these terms.
*Correspondence: John D. Reynolds, cmV5bm9sZHNAc2Z1LmNh