- 1Department Biology, California State University, Fresno, CA, United States
- 2National Socio-Environmental Synthesis Center (SESYNC), Annapolis, MD, United States
- 3Department of Environmental Conservation, University of Massachusetts, Amherst, MA, United States
- 4Tucson Audubon Society, Tucson, AZ, United States
- 5Department of Forestry and Environmental Resources, North Carolina State University, Raleigh, NC, United States
Urbanization presents novel challenges to native species by altering both the biotic and abiotic environment. Studies have attempted to make generalizations about how species with similar traits respond to urbanization, although existing results are idiosyncratic across cities and often fail to account for seasonality. Here, we present a comparative study in three US cities: Fresno, California; Tucson, Arizona; and Phoenix, Arizona. Using presence-absence data to define regional bird species pools and urban assemblages in non-breeding (winter) and breeding (spring) seasons, we tested whether urban avian assemblages were a random subset of regional assemblages on the basis of both traits and phylogeny, and whether urbanization was associated with homogenization among avian assemblages. We found evidence for non-random trait filtering into urban assemblages, including of diet guilds, migratory status, and primary habitat, but filtering differed across cities and seasons, being strongest for diet and in Fresno. There was no evidence for non-random phylogenetic-based filtering in urban avian assemblages. Dissimilarity in species and diet guild composition within each season was higher between cities than between regional species pools. These findings show the potential for biotic differentiation as opposed to homogenization as the outcome of environmental filtering processes operating on species traits across cities and seasons.
Introduction
The loss of biodiversity and ecosystem services due to urbanization has been well-documented across multiple taxa (Lockwood and McKinney, 2001). This decline in biodiversity is especially the case for native species (Aronson et al., 2014), making improved understanding of how native species respond to urbanization an urgent priority for the protection of biodiversity in urban landscapes.
A core focus of urban ecological research into the effect of urbanization on biodiversity has been the potential for ecological homogenization across cities. Although definitions of homogenization vary, they focus on two key features: homogenization of pattern and homogenization of process. The former describes increased similarity in the facets of biodiversity (species, trait, and phylogenetic composition) across cities whereas the latter addresses increased similarity in the operation of ecological processes, such as environmental filtering (Aronson et al., 2016; Pearse et al., 2018). Environmental filtering of species from a regional source pool (Keddy, 1992) has become a key conceptual framework for understanding the process generating patterns of urban biodiversity. Aronson et al. (2016) proposed a hierarchical set of filters at work in urban areas, from general biotic and abiotic filters including climate and land use, to characteristics of specific urban habitats, such as local scale human landscape management driven by socioeconomic and cultural factors. This hierarchical set of filters interacts with species traits to exclude certain species from the urban assemblage.
However, while numerous studies have found that urbanization leads to patterns of reduced species richness, increased biomass, and increased similarity in species composition between assemblages, these patterns are not consistent across cities or regions, because how biodiversity responds to urbanization may also be highly context-dependent (Chace and Walsh, 2006). For instance, Leveau et al. (2017) found the relationship between avian species richness and percent impervious land cover—a common metric of urbanization—varied across three cities depending on the habitat matrix surrounding each city. On a global scale, Aronson et al. (2014) analyzed patterns of bird species occurrence in 52 cities from across all biogeographic provinces. They found that urban avian assemblages strongly reflect their regional species pools, consisting largely of native species, with non-native “urban specialists” typically making up >5% of the number of species in urban bird assemblages, indicating a smaller role for homogenization than had been suggested by earlier work. Similarly, previous studies of bird traits in urban areas have failed to reach a consensus on which traits promote species occurrence in urban settings. Diet has often been found to be associated with species success in urban areas, but which diet types is inconsistent. For instance, insectivory has been found to have a negative (Leveau, 2013), positive (Lim and Sodhi, 2004; Kark et al., 2007), and no association with species occurrence (Croci et al., 2008; Lizée et al., 2011; Meffert and Dziock, 2013) in both tropical and temperate cities. Similarly, results on whether migratory habits are associated with tolerance to urbanization have been mixed (Kark et al., 2007; Leveau, 2013; Sol et al., 2014). Thus, homogenization of pattern, at least, may not be the rule or an inevitable result of urbanization.
One factor not considered in most previous studies that may in part drive these mixed results is seasonality (Leveau and Leveau, 2012, 2016). Typically, studies on urban bird communities focus on the breeding season, which is unsurprising given the prevalent northern temperate zone bias in cities where such studies are conducted. However, as in other terrestrial ecosystems, the species composition of bird communities in cities varies seasonally. Urban habitats support migratory birds in either breeding or non-breeding seasons, or both, depending on their latitude and regional context. Mid-latitude cities may be expected to see a significant turnover in species composition across seasons, with breeding migrants flying to lower latitudes even as non-breeding migrants move in for the winter from higher latitudes. These seasonal changes in species composition and community assembly have not been studied systematically in the urban context but it has been suggested that urbanization dampens temporal variation in bird communities compared to surrounding exurban habitats (Leveau et al., 2018). Thus, it remains unclear whether urban environmental filtering changes seasonally due to potential changes in the relative strength or specific mechanisms of filtering across seasons.
Here, we use 5 years (2011–2015) of point count data from three cities in the southwestern United States that differ in their surrounding habitat to compare homogenization of the pattern and process of environmental filtering of native urban bird assemblages, including across seasons. Specifically, we ask: (1) Does urbanization filter bird assemblages on the basis of traits?; (2) Does any trait-based filtering follow a phylogenetic pattern and lead to non-random loss of evolutionary history?; and (3) Do filtering processes operate similarly across different urban settings and seasons, and therefore lead to biotic homogenization?
We hypothesize that: (1) Urbanization does filter bird assemblages on the basis of traits, and that this will lead to significant over- or under-representations of traits in urban species assemblages; (2) Because traits are inherited, filtering will follow a phylogenetic pattern, and therefore result in significantly higher decreases in phylogenetic diversity (PD) metrics compared to random species loss; and (3) Urbanization will be associated with increased similarity in species and trait composition, including in both breeding and non-breeding seasons.
Methods
Urban Bird Counts
Data from Fresno and Tucson both come from citizen science-based bird count projects. The Tucson Bird Count (Turner, 2003) started in 2001 and its replicate, the Fresno Bird Count, started in 2008. We used data from the years 2011 to 2015. Survey points in Fresno and Tucson were selected using a systematic randomized sampling design. 1 × 1 km grids were laid out over the metro area, and one survey site was randomly selected within each grid square, resulting in 460 total survey sites in Fresno and 1,385 survey sites in Tucson. In Fresno, a subset of 75 core sites were selected in a stratified sampling approach in order to reflect the socioeconomic gradient of the Fresno-Clovis Metropolitan Area (FCMA). Each core site was visited once by citizen scientists or trained observers during two annual count periods: spring breeding season (15 April to 15 May), and winter non-breeding season (15 December to 31 January). Other sites were surveyed during these periods as volunteers or time allowed. Counts occurred on mornings without inclement weather between one half hour before sunrise and 4 h after sunrise. Birds were counted for 5 min within 40 m of each survey point. In Tucson, sites were visited by citizen scientists or trained observers once during each season: spring (15 April−15 May), summer (1 July−31 July), autumn (1 September−30 September), and winter (15 January−15 February). We used only data from the spring and winter seasons to enable comparison across cities. Counts in Tucson occurred on mornings without inclement weather between one half hour before sunrise and 4 h after sunrise. Birds were counted for 5 min with an open radius.
Bird species occurrence data from Phoenix were collected as part of the Central Arizona Phoenix—Long Term Ecological Research (CAP LTER) project. Two hundred bird point count sites were selected by Hope et al. (2003), using a tessellation-stratified dual-density design. Point counts were conducted on mornings without inclement weather within 4 h of sunrise. Birds were counted for 15 min periods within an open radius (see Lerman and Warren, 2011 for entire protocol description). Again, we use only data from the spring and winter periods to control for season when comparing across the three cities.
To assess if differences in sampling effort between the three cities are responsible for differences in filtering results, we calculated species accumulation curves for each city in each season. We built species accumulation curves using the specaccum function in R package vegan (Oksanen et al., 2018). In each case, we built 100 species accumulation curves by randomly selecting survey sites. Species accumulation curves show a saturation in species richness for each sampling season in each city (Supplementary Figure 1), and we therefore did not use rarefaction in further comparisons among cities.
Non-Native Species
Given our focus was on how cities affect native North American avifauna we excluded non-native species from our analyses. Non-native species make up 8% of the number of species in Fresno, 4% in Tucson, and 3% in Phoenix. As such, this percentage is similar to the figure of 5% reported by Aronson et al. (2016) for urban bird assemblages globally. Only four non-native species exist in any of the species assemblages: European Starling (Sturnus vulgaris), House Sparrow (Passer domesticus), Rock Dove (Columba livia), and Eurasian Collared-Dove (Streptopelia decaocto). Starlings, sparrows, and Rock Doves are present in all regional and urban species assemblages, while Collared-Doves are present in all except for the Fresno winter assemblages (see Supplementary Material for a presence-absence matrix). Given the low percentage of non-native species in each of the three cities, including non-native species is unlikely to substantially change patterns of community structure reported for the three cities based on presence-absence data.
Regional Species Pools
Regional species pools were delimited using eBird data (Sullivan et al., 2009) from Pima County, AZ (Tucson), Maricopa County, AZ (Phoenix), and Fresno and Madera Counties, CA (Fresno). Tucson and Phoenix are each located in the middle of a county so only one county was used whereas Fresno is located on the border of two counties. We downloaded reporting frequency—defined as the proportion of all submitted checklists in which a taxon appears—for each county from 2011 to 2015. We generated a regional species pool for each city in each season by subsetting reporting frequency by season, keeping data only for the winter (15 December−31 January) and spring (15 April−15 May) periods. Next, we removed all non-specific taxa (e.g., “sparrow sp.”, or “Greater/Lesser Scaup”), as well as all species that do not occur naturally in western North America. We also removed species from all three regional species pools based on the location of a city relative to the range of habitats in each county. For example, Fresno County ranges in elevation from under 100 m on the floor of California's Central Valley to over 4,300 m in the Sierra Nevada, but Fresno sits at about 100 m of elevation. Consequently, the eBird data from Fresno county contains many high elevation species that would not be present in the vicinity of Fresno simply due to their habitat preferences. Using elevational range data from Birds of North American (Rodewald, 2015), we excluded all species that would not exist in the area of each city regardless of urbanization levels. Finally, to exclude observations of vagrant birds, we calculated the percentage of checklists in which each species was recorded during the time period of the urban bird counts in each city and season, and then removed from each regional species pool those species with reporting frequencies of >1% of checklists.
Urban Assemblages
Urban species assemblages were defined as all species detected during urban counts within each city and season between 2011 and 2015, that are also present in the regional species pool (hereafter referred to as the “total urban assemblage”). This was done to exclude non-native species from the urban assemblages. A second urban assemblage for each city (hereafter referred to as the “trimmed urban assemblage”) includes only species detected in at least 1% of urban counts. This “trimmed urban assemblage” was created to exclude those species that are potential vagrants in each city. We report relative species richness instead of absolute species richness when comparing urban assemblages to their regional species pool because relative species richness (defined as the percentage of the regional species pool present in the urban assemblage) accounts for the size of the regional species pool and is thus more informative than raw species richness for comparing the degree of filtering across cities (Cam et al., 2000).
Trait and Phylogenetic Data
Trait data were collected from published and online bird databases (BirdLife International, 2011; Rodewald, 2015; Cornell Lab of Ornithology., 2017). We collected trait data for main habitat, diet, foraging strategy, social behavior in the breeding season, social behavior in the non-breeding season (winter), migratory status, nest openness, body mass, number of molts per year in adults, plumage dimorphism, parental investment in nest building, parental investment in egg incubation, clutch size, number of clutches per year, incubation period, nestling period, and wingspan. Where available, values for regional populations or subspecies are used. Otherwise, species-wide data are used. Two species, the American Wigeon (Anas penelope), which is present only in the Phoenix winter regional species pool, and Clark's Grebe (Aechmophorus clarkii), present only in the Fresno winter regional species pool, have very little information published, so both were removed from trait-based analyses. For phylogenetic analyses, we downloaded 1,000 dated phylogenies containing all 283 species across the three regional species pools from birdtree.org (Jetz et al., 2012), using the Erickson backbone.
Statistical Analyses
To determine if trait distributions in the urban species assemblages were a random subset of the trait distributions available in the regional pools, we performed bootstrapping tests with 999 simulations, using Chi-squared tests for categorical traits, and Kolmogorov-Smirnov tests (Gotelli and Ellison, 2004) for continuous traits. Bootstrap tests were performed using RStudio v1.1.383 (RStudio Team, 2015) and packages “Matching” (Sekhon, 2011) and “forcats” (Wickham, 2017).
We used three different phylogenetic metrics to assess effects of urbanization on bird assemblages: PD, mean pairwise distance (MPD), and mean nearest taxon distance (MNTD). PD estimates total independent evolutionary history of an assemblage based on species richness and the phylogenetic relationships between them (Veron et al., 2017). We used an unrooted PD measure (Faith, 1992). MPD measures the average distance between all pairs of species in an assemblage, while MNTD measures the average distance to the nearest relative of each species in an assemblage (Webb, 2000). All three metrics were calculated using all 1,000 dated phylogenies.
We used the ses.pd, ses.mpd, and ses.mntd functions from the R package “picante” (Kembel et al., 2010) to calculate the standardized effect size of PD, MPD, and MNTD, respectively. We tested for statistical significance by running 999 randomizations of urban assemblages for each of the 1,000 phylogenies by shuffling taxa labels across branch tips. Phylogenetic analyses were run in R v3.4.3 (R Core Team, 2018) with packages “picante” (Kembel et al., 2010) and “DescTools” (Signorell, 2017). Median p-values were used to assess statistical significance, with p < 0.01 taken as indicating significant departure from random.
To determine the strength of filtering in each city, we calculated nested Sorensen dissimilarity indices between each regional species pool and the respective urban species assemblage. We also calculated multi-site Sorensen dissimilarity indices between regional species pools and between urban species assemblages.
Results
Species Richness
Two-hundred eighty-three species were reported in at least 1% of eBird checklists across the three study cities (see Supplemental Table 1 for all species and trait info). Fresno had the most species poor urban assemblage in both the spring breeding (61 species) and winter, non-breeding (51 species) seasons (Table 1). In contrast, Phoenix had the most species rich urban assemblages in both spring (132) and winter (117), while Tucson had the largest trimmed urban assemblage (i.e., excluding vagrant species) in winter (84). Focusing on relative species richness, the Fresno urban assemblage had the lowest percentage of species from the regional pool (17–37%) in both seasons (Table 1), suggesting strong filtering. In contrast, urban assemblages in Tucson and Phoenix had a higher proportion of species from their respective regional pools (31–86%), with this percentage being higher in winter (8% in Phoenix, 13% in Tucson) than in spring indicating potentially stronger filtering of species from the regional pool in the spring breeding season.
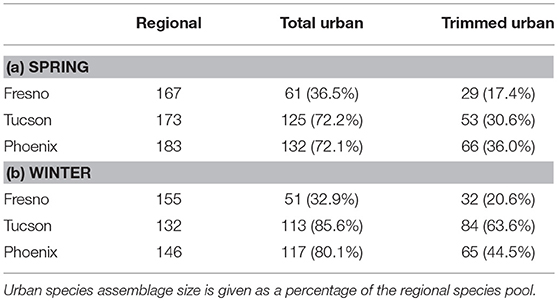
Table 1. Species richness for regional species pools and urban assemblages for spring (a) and Winter (b).
Traits
Does Urbanization Filter Birds on the Basis of Traits?
Three traits showed significant differences between urban assemblages and regional species pools, with results differing by city and season. First, the distribution of diet guilds within urban assemblages was significantly different from that within regional species pools in both spring and winter, but this pattern differed across cities. In Fresno, diet guild distribution differed from the regional species pool in both seasons in the total urban species assemblage (Spring: χ2 = 21.522, p = 0.027; Winter: χ2 = 29.695, p = 0.009) and the trimmed urban species assemblage (Spring: χ2 = 24.18, p = 0.018; Winter: χ2 = 35.075, p = 0.006). Relative to the regional species pool, the Fresno total urban assemblage had a 15% increase in omnivores, 6% increase in granivores, and 12% decrease in insectivores in spring (Figure 1 see Supplemental Figure 2 for absolute species numbers). These differences were larger for the trimmed urban assemblage, with a 24% increase in omnivores, a 10% increase in granivores, and a 28% decrease in insectivores relative to the spring regional species pool. Compared to the regional species pool in winter the Fresno total urban assemblage had increased granivores (5%) and omnivores (22%), but decreased insectivores by 24% (Figure 2 see Supplemental Figure 3 for absolute species numbers). Again, changes for the trimmed urban assemblage were larger, with a 12% increase in omnivores and a 36% decrease in insectivores. The diet distribution in Tucson's trimmed urban assemblage was significantly different from its regional pool in spring only (χ2 = 23.511, p = 0.012). Similar to spring species pools in Fresno, the trimmed urban assemblage had over-representation of granivores (10% increase) and omnivores (5% increase), and an under-representation of insectivores (14% decrease; Figures 1, 2). In Phoenix, no urban assemblages significantly differed from the regional pools.
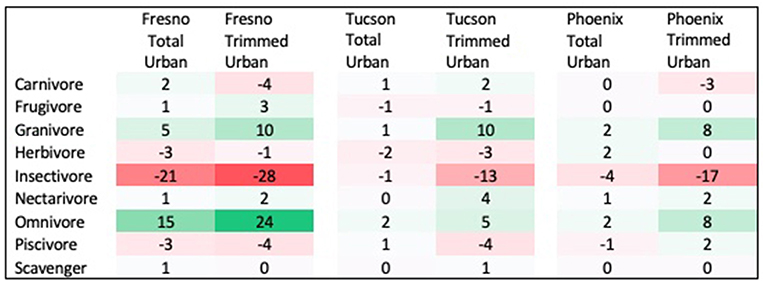
Figure 1. Percent change in prevalence of each diet guild in spring urban species assemblages compared to spring regional species pool. Guilds that are over-represented in urban species assemblages are shaded in green, while guilds that are under-represented in urban species assemblages are shaded in red.
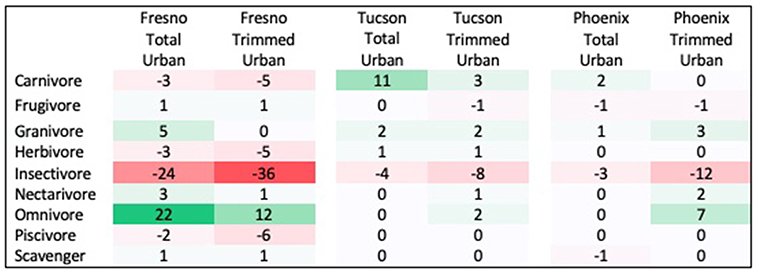
Figure 2. Percent change in prevalence of each diet guild in winter urban species assemblages compared to winter regional species pool. Guilds that are over-represented in urban species assemblages are shaded in green, while guilds that are under-represented in urban species assemblages are shaded in red.
Second, the proportion of migratory species differed from the regional pool only in the trimmed urban species assemblage in Phoenix during the spring season (χ2 = 5.2833, p = 0.033), with a 22% decrease in migratory species.
Third, the distribution of species' main habitat preferences in the trimmed urban assemblage differed significantly from the regional pool in all three cities during the spring (Fresno: χ2 = 24.68, p = 0.005; Tucson: χ2 = 30.549, p = 0.003; Phoenix: χ2 = 18.437, p = 0.029), but only in Fresno during the winter (χ2 = 17.199, p = 0.041). In spring, Fresno's trimmed urban assemblage showed a 22% decrease in forest species. In Tucson, the trimmed urban assemblage had a higher proportion of forest species (8%), marsh species (10%), desert species (7%) and scrub species (15%) than the surrounding regional pool. Desert and scrub species were also higher by 5 and 3 percent, respectively, in Phoenix in the trimmed urban assemblage, but forest species decreased by 8%. In winter, the Fresno trimmed urban assemblage shows only a 4% decrease in forest species, but a 21% increase in open woodland species.
Does Trait-Based Filtering Lead to Non-random Loss of Evolutionary History?
None of the three phylogenetic metrics for either the total or trimmed urban assemblages differed significantly from random community assembly simulations in either season (Table 2).
Does Trait- or Phylogeny-Based Filtering in Each City and Season Result in Homogenization Among Cities?
Nested Sorensen dissimilarities showed that urban assemblages in Fresno had high dissimilarity to the regional species pool for both winter and spring (Table 3). Tucson and Phoenix both showed substantially lower dissimilarity between urban assemblages and their regional species pools in both seasons.
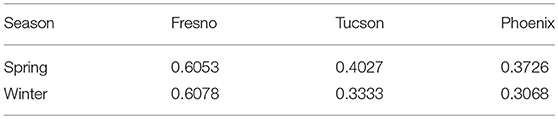
Table 3. Nested Sørensen dissimilarities between regional species pools and urban assemblages for each city and season.
Multi-site Sorensen dissimilarities showed that urban species assemblages were more dissimilar to each other than regional pools were from each other, in both seasons (Table 4). These differences in dissimilarities were driven almost entirely by increases in nestedness between urban species assemblages compared to regional species pools.
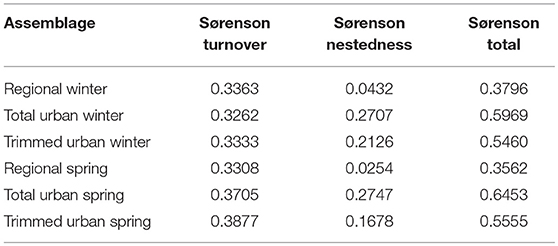
Table 4. Multi-site Sørensen dissimilarities between the regional species pools, total urban species assemblages, and trimmed urban species assemblages in each season.
The diet guild composition of trimmed urban assemblages differed significantly for all pairwise city comparisons in the spring season (Fresno-Tucson: χ2 = 45.799, p = 0.001; Fresno-Phoenix: χ2 = 51.558, p = 0.001; Tucson-Phoenix: χ2 = 34.985, p = 0.001), and in foraging strategy between Tucson and Phoenix in the spring season (χ2 = 29.142, p = 0.002). In contrast, no comparisons between regional species pools and winter urban assemblages in these traits produced significant results.
Discussion
Trait-based environmental filtering of avian communities in Fresno, Tucson and Phoenix was based on diet and species' habitat preferences in both breeding and non-breeding seasons, but this did not result in non-random changes in urban phylogenetic community structure relative to regional species pools. Although we found evidence for the operation of trait-based environmental filtering processes in each city, there was no pattern of biotic homogenization of native avifauna among the three cities, with consistently higher dissimilarity between cities than between regional species pools for both species and trait composition, including across seasons. As such, this study shows the potential for individual cities to retain the local character of their avian assemblages despite similar community assembly processes operating across cities.
Does Urbanization Filter Birds on the Basis of Their Biological Traits?
Species' habitat preference of the trimmed urban assemblage was the only trait showing significant differences from regional species pools in all three cities. This agrees with the vast majority of previous studies of this type, which found significant habitat filtering in response to urbanization. The pattern of increased prevalence of omnivores and granivores, with decreased prevalence of insectivores in Fresno and Tucson also matches a general consensus reached by prior research (Lim and Sodhi, 2004; Kark et al., 2007; Croci et al., 2008; Conole and Kirkpatrick, 2011; Lizée et al., 2011; Leveau, 2013; Cristaldi et al., 2017).
Increases in prevalence of omnivores (e.g., American Crow) are likely due to these species' habits of scavenging human refuse, enabling them to exploit new niches present in urban habitats (Kark et al., 2007). Lim and Sodhi (2004) concluded that omnivores possess advantages in urban areas mainly at higher latitudes where food resources are seasonally limited. This meshes with the observed increases in omnivore prevalence and seasonal nature of climates of the cities in the present study. Increases in prevalence of native granivores (e.g., White-crowned Sparrow) are likely due to elevated levels of human bird feeding in urban areas. Multiple studies have shown that bird feeders increase species richness (Daniels and Kirkpatrick, 2006; Parsons et al., 2006), and Fuller et al. (2008) found significant effects of bird feeding on total bird abundance, driven mainly by changes in abundances of species known to use feeders. Bird feeders likely have positive influences on omnivores as well (Jokimäki and Suhonen, 1998). The decrease in insectivore (e.g., Blue-gray Gnatcatcher) prevalence in urban species assemblages may be due to the scarcity of insects in urban habitats. Changes in herbivorous invertebrates can translate to higher trophic levels (Flückiger et al., 2002), so habitat fragmentation, elevated pollutant levels, anthropogenic maintenance of yards, and planting of ornamental exotic species in urban habitats may drive declines in invertebrate diversity and abundance, which in turn cause decreasing prevalence of insectivorous birds (Tallamy, 2004; Burghardt et al., 2009).
Interestingly, in Phoenix, no difference was observed in diet guild composition between the urban assemblages and regional species pool, implying that food resources in the urban habitats of Phoenix match those present in natural habitats of the area. This may be a result of the large size of Phoenix, which presents ample opportunity for heterogeneity in food resources across the city. Of the three cities in the study, Phoenix is the only one that encompasses large tracts of undisturbed natural habitats: North Mountain Park and the Phoenix Mountains Preserve reside within the urban matrix of the city. These areas may harbor food sources for species that may not find appropriate food sources in the more disturbed areas of the city, enabling them to persist in urban conditions they could not otherwise endure. Alternatively, avian populations in the urban matrix of Phoenix may be supplemented through source-sink dynamics (Pulliam, 1988) with populations in the more natural settings of the parks. So, the heterogeneous nature of habitats in Phoenix may be offsetting filtering effects induced by its large size and high human population density. Multiple studies have shown that bird populations and communities respond positively to the presence of urban greenery, including parks (Melles et al., 2003; de Toledo et al., 2012).
The use of presence-absence data may also be contributing to a lack of significant patterns in trait filtering for specific traits. The hyper-abundance of certain urbanophilic species can drive significant differences in community trait composition and biotic homogenization. Paz Silva et al. (2016) found that the biotic homogenization results in their study were largely driven by changes in abundance of three species, two of which were concentrated in urban habitats (House Sparrow and Chilean Swallow, Tachycineta meyeni), and one which was concentrated in rural habitats (Southern Lapwing, Vanellus chilensis). In the American context these abundance changes may often be most-pronounced for non-native, urban exploiter species, such as Rock Pigeon (Paz Silva et al., 2016). High densities of synurbic exotic species such as Rock Pigeon, House Sparrow, and European Starling could be outcompeting native species for resources in urban areas as a result of their heightened foraging efficiency in urban habitats (Shochat et al., 2010).
It is also possible that species loss in the three cities of this study is not due to trait-environment interactions, but to some other biotic factor. Species occurrence has been shown to respond to abiotic and biotic factors simultaneously (Paine, 1966; Patterson, 1980; Thompson et al., 1996), and there are many biotic factors that may be influencing species occupancy of urban habitats in the present study. Cadotte and Tucker (2017) called for consideration of biotic factors in studies of environmental filtering, and for careful experimentation to tease apart these mechanisms. They also note that observational data can still be useful, such as that presented here, as long as the environmental filter is not strictly dependent on abiotic factors.
Does Trait-Based Filtering Lead to Non-Random Loss of Evolutionary History?
The lack of significant differences in phylogenetic metrics between observed urban assemblages and those simulated by drawing species at random from regional pools indicates that patterns of PD, MPD, and MNTD loss in the Tucson, Fresno and Phoenix do not differ from those that would result from random loss of species. If traits with high phylogenetic signal had shown shifts due to filtering, phylogenetic filtering would be expected as related species possessing those traits would drive phylogenetic patterns.
This is an interesting finding, because previous studies of avian phylogenetics in urban areas have convincingly demonstrated loss of PD (Sol et al., 2014; La Sorte et al., 2018), phylogenetic beta diversity (La Sorte et al., 2018), and a reduction in evolutionary distinctiveness (Ibáñez-Álamo et al., 2017). Because these phylogenetic metrics are not independent of species richness (Faith, 1992), decreases in urban areas are not surprising in the light of widespread species loss in urban assemblages. (Ibáñez-Álamo et al., 2017) evaluated loss of evolutionary distinctiveness in urban areas globally, noting differences in the magnitude of loss on different continents. Of the five continents in the study, North America exhibits the smallest losses in both bird species richness and community evolutionary distinctiveness. This may explain why the current study of urban avian phylogenetics at a local scale was unable to find significant filtering patterns. The phylogenetic effects of urbanization at continental or global scales (Sol et al., 2014; La Sorte et al., 2018) may be emergent patterns due to accumulation of small effects on local scales. This scalar dependence of evolutionary patterns deserves more detailed research, and may indicate that efforts to ameliorate adverse effects of urbanization on avian phylogenies will need to be coordinated over large regions instead of within individual cities.
Does Filtering Lead to Biotic Homogenization Among the Three Cities?
Multi-site Sørensen dissimilarity indices (Table 4) indicate that urban species assemblages are more dissimilar than regional species pools based on presence-absence of native species. This result of “biotic differentiation,” as opposed to homogenization, is further supported by higher dissimilarity among cities than among regional species pools in the distributions of diet guilds. This points to urbanization potentially driving a functional divergence in native bird assemblages, including across seasons. These results point to the potential for urban assemblages to retain, or even increase, their local character. With more thoughtful design of urban areas, these habitats could become important sites for native biodiversity, despite being thought of as heavily disturbed. This finding contrasts with previous studies that have demonstrated biotic homogenization due to urbanization (Blair and Launer, 1997; Aronson et al., 2014). We propose that this dichotomy is due to two aspects of the present study: (1) the use of presence-absence data instead of abundance or density estimates, and (2) the exclusion of exotic bird species. We used presence-absence data in order to minimize the effects of different survey procedures across the three cities, but this excludes a potential signal of homogenization due to any changing bird abundances. Introduced exotic species are often hyper-abundant in urban habitats (Blair, 1996; Lim and Sodhi, 2004; van Heezik and Adams, 2016), so they can be a strong driver of biotic homogenization. For example, Paz Silva et al. (2016) found that biotic homogenization between three South American urban bird assemblages was mostly driven by changing abundances of three species, one of which was an exotic species.
There is some evidence here for a seasonal nature of the effects of urbanization on bird assemblages. Urban environmental filtering may vary in strength by season (Figures 1, 2). Nested Sorenson dissimilarities (Table 3) also support this, although Fresno shows little variation between spring and winter nested dissimilarities. Multi-site Sorenson dissimilarities show that while dissimilarity between regional species pools slightly decreases from winter to spring, dissimilarity between urban assemblages increases from winter to spring. Seasonal dynamics of the effects of urbanization on birds are little-studied to this point, and deserve more attention.
Conclusions
The results presented here show some interesting departures from the existing body of literature on urban environmental filtering. Mainly, our findings show no evidence of biotic homogenization. Instead, the three cities in this study exhibit signs of biotic differentiation in urban avian assemblages. Thus, cities may have the capability to retain the local character of their native avifauna. We also show that seasonality has effects on differentiation between urban avian assemblages. This study shows the effects of urbanization specifically on native birds, where the majority of previous studies included exotic species, which may have caused results to be skewed in the direction of how exotics respond to urbanization. This study has also filled a gap in the literature by taking a comparative approach to the study of how bird assemblages respond to urbanization.
Data Availability
The datasets analyzed for this study can be found in the possession of the researchers who generated them. Requests to access the Fresno Bird Count database should be directed to MK, bWthdHRpQG5jc3UuZWR1. Requests to access the Tucson Bird Count database should be directed to Jennie MacFarland, am1hY2ZhcmxhbmRAdHVjc29uYXVkdWJvbi5vcmc=. Requests to access the CAP-LTER database can be submitted through the CAP-LTER online data portal at https://sustainability.asu.edu/caplter/data/
Author Contributions
This publication is a result of the MS thesis work of CH. CH collected and organized all trait data, performed all statistical analyses, and wrote the original manuscript draft. MK and CT contributed significantly to the direction and design of the study, and wrote portions of the manuscript. CT also gave substantial assistance with statistical analysis and R coding. PW provided data from Phoenix, and JM provided data from Tucson. SB and JR provided guidance and advice throughout the thesis and manuscript process, and provided helpful comments on the manuscript.
Funding
Funding for this project was provided by the Fresno State Student Research Award and the Fresno State Graduate and Creative Activities Support Award. National Science Foundation grants (ULTRA-Ex # 0949036 and DEB-9714833) supported the establishment of projects that contributed data to this research. CT was supported by the National Socio-Environmental Synthesis Center (SESYNC) under funding received from the US National Science Foundation DBI-1052875.
Conflict of Interest Statement
The authors declare that the research was conducted in the absence of any commercial or financial relationships that could be construed as a potential conflict of interest.
Acknowledgments
Thanks to Dr. Michael Rosenzweig for founding the Tucson Bird Count, and to Stevan Earl for curating an enormous amount of data from Phoenix. Thanks also to all the volunteer citizen scientists who have collected data in Fresno and Tucson.
Supplementary Material
The Supplementary Material for this article can be found online at: https://www.frontiersin.org/articles/10.3389/fevo.2019.00071/full#supplementary-material
References
Aronson, M. F. J., LaSorte, F. A., Nilon, C. H., Katti, M., Goddard, M. A., Lepczyk, C. A., et al. (2014). A global analysis of the impacts of urbanization on bird and plant diversity reveals key anthropogenic drivers. Proc. R. Soc. B 281. doi: 10.1098/rspb.2013.3330
Aronson, M. F. J., Nilon, C. H., Lepczyk, C. A., Parker, T. S., Warren, P. S., Cilliers, S. S., et al. (2016). Hierarchical filters determine community assembly of urban species pools. Ecology. 97, 2952–2963. doi: 10.1002/ecy.1535
BirdLife International (2011). Species Accounts. Cambridge: BirdLife International. Available online at: www.birdlife.org (Accessed January 2015 - March 2018).
Blair, R. B. (1996). Land use and avian species diversity along an urban gradient. Ecol. Appl. 6, 506–519.
Blair, R. B., and Launer, A. E. (1997). Butterfly diversity and human land use: species assemblages along an urban gradient. Biol. Conserv. 80, 113–125. doi: 10.1016/S0006-3207(96)00056-0
Burghardt, K. T., Tallamy, D. W., and Shriver, W. G. (2009). Impact of native plants on bird and butterfly biodiversity in suburban landscapes. Conserv. Biol. 23, 219–224. doi: 10.1111/j.1523-1739.2008.01076.x
Cadotte, M. W., and Tucker, C. W. (2017). Should environmental filtering be abandoned? Trends Ecol. Evol. 32, 429–437. doi: 10.1016/j.tree.2017.03.004
Cam, E., Nichols, J. D., Sauer, J. R., Hines, J. E., and Flather, C. H. (2000). Relative species richness and community completeness: birds and urbanization in the Mid-Atlantic States. Ecol. Appl. 10, 1196–1210. doi: 10.1890/1051-0761(2000)010[1196:RSRACC]2.0.CO;2
Chace, J. J., and Walsh, J.J. (2006). Urban effects on native avifauna: a review. Landsc. Urban Plan. 74, 46–69. doi: 10.1016/j.landurbplan.2004.08.007
Conole, L. E., and Kirkpatrick, J. B. (2011). Functional and spatial differentiation of urban bird assemblages at the landscape scale. Landsc. Urban Plan. 100, 11–23. doi: 10.1016/j.landurbplan.2010.11.007
Cornell Lab of Ornithology. (2017). All About Birds. Ithaca, NY: Cornell Laboratory of Ornithology. Available online at: https://allaboutbirds.org (Accessed January 2015 - March 2018).
Cristaldi, M. A., Giraudo, A. R., Arzamendia, V., Bellini, G. P., and Claus, J. (2017). Urbanization impacts on the trophic guild composition of bird communities. J. Nat. Hist. 51, 2385–2404. doi: 10.1080/00222933.2017.1371803
Croci, S., Butet, A., and Clergeau, P. (2008). Does urbanization filter birds on the basis of their biological traits? Condor. 110, 223–240. doi: 10.1525/cond.2008.8409
Daniels, G. D., and Kirkpatrick, J. B. (2006). Does variation in garden characteristics influence the conservation of birds in suburbia? Biol. Conserv. 133, 326–335. doi: 10.1016/j.biocon.2006.06.011
de Toledo, M. C. B., Donatelli, R. J., and Batista, G. T. (2012). Relation between green spaces and bird community structure in an urban area in Southeast Brazil. Urban Ecosyst. 15, 111–131. doi: 10.1007/s11252-011-0195-2
Faith, D. P. (1992). Conservation evaluation and phylogenetic diversity. Biol. Conserv. 61, 1–10. doi: 10.1016/0006-3207(92)91201-3
Flückiger, W., Braun, S., and Hiltbrunner, E. (2002). “Effects of air pollution on biotic stress,” in Air Pollution and Plant Life. eds J.N.B. Bell, and M. Treshow (Chichester: John Wiley and Sons), 379–406.
Fuller, R. A., Warren, P. H., Armsworth, P. R., Barbosa, O., and Gaston, K. J. (2008). Garden bird feeding predicts the structure of urban avian assemblages. Divers. Distrib. 14, 131–137. doi: 10.1111/j.1472-4642.2007.00439.x
Gotelli, N. J., and Ellison, A. M. (2004). A Primer of Ecological Statistics. Sunderland, MA: Sinauer Associates, Inc.
Hope, D., Gries, C., Zhu, W., Fagan, W. F., Redman, C. L., Grimm, N. B., et al. (2003). Socioeconomics drive urban plant diversity. PNAS 100, 8788–8792. doi: 10.1073/pnas.1537557100
Ibáñez-Álamo, J. D., Rubio, E., Benedetti, Y., and Morelli, F. (2017). Global loss of avian evolutionary uniqueness in urban areas. Glob. Chang. Biol. 23, 2990–2998. doi: 10.1111/gcb.13567
Jetz, W., Thomas, G. H., Joy, J. B., Hartmann, K., and Mooers, A. O. (2012). The global diversity of birds in time and space. Nature 491, 444–448. doi: 10.1038/nature11631
Jokimäki, J., and Suhonen, J. (1998). Distribution and habitat selection of wintering birds in urban environments. Landsc. Urban Plan. 39, 253–263. doi: 10.1016/S0169-2046(97)00089-3
Kark, S., Iwaniuk, A., Schalimtzek, A., and Banker, E. (2007). Living in the city: can anyone become an ‘urban exploiter’? J. Biogeogr. 34, 638–651. doi: 10.1111/j.1365-2699.2006.01638.x
Keddy, P. A. (1992). Assembly and response rules: two goals for predictive community ecology. J. Veg. Sci. 3, 157–164. doi: 10.2307/3235676
Kembel, S. W., Cowan, P. D., Helmus, M. R., Cornwell, W. K., Morlon, H., Ackerly, D. D., et al. (2010). Picante: R tools for integrating phylogenies and ecology. Bioinformatics 26, 1463–1464. doi: 10.1093/bioinformatics/btq166
La Sorte, F. A., Lepczyk, C. A., Aronson, M. F. J., Goddard, M. A., Katti, M., MacGregor-Fors, I., et al. (2018). The phylogenetic and functional diversity of regional breeding assemblages is reduced and constricted through urbanization. Divers. Distrib. 24, 928–938. doi: 10.1111/ddi.12738
Lerman, S. B., and Warren, P. S. (2011). The conservation value of residential yards: linking birds and people. Ecol. Appl. 21, 1327–1339. doi: 10.1890/10-0423.1
Leveau, L. M. (2013). Bird traits in urban-rural gradients: how many functional groups are there? J. Ornithol. 154, 655–662. doi: 10.1007/s10336-012-0928-x
Leveau, L. M., Isla, F. I., and Bellocq, M. I. (2018). Predicting the seasonal dynamics of bird communities along an urban-rural gradient using NDVI. Landsc. Urban Plan. 177, 103–113. doi: 10.1016/j.landurbplan.2018.04.007
Leveau, L. M., and Leveau, C. M. (2012). The role of urbanization and seasonality on the temporal variability of bird communities. Landsc. Urban Plan. 106, 271–276. doi: 10.1016/j.landurbplan.2012.03.008
Leveau, L. M., and Leveau, C. M. (2016). Does urbanization affect the seasonal dynamics of bird communities in urban parks? Urban Ecosyst. 19, 1461–1476. doi: 10.1007/s11252-016-0525-5
Leveau, L. M., Leveau, C. M., Villegas, M., Cursach, J. A., and Suazo, C. G. (2017). Bird communities along urbanization gradients: a comparative analysis among three Neotropical cities. Ornitol. Neotrop. 28, 77–87.
Lim, H. C., and Sodhi, N. S. (2004). Responses of avian guilds to urbanisation in a tropical city. Landsc. Urban Plan. 66, 199–215. doi: 10.1016/S0169-2046(03)00111-7
Lizée, M. H., Mauffrey, J. F., Tatoni, T., and Deschamps-Cottin, M. (2011). Monitoring urban environments on the basis of biological traits. Ecol. Indic. 11, 353–361. doi: 10.1016/j.ecolind.2010.06.003
Lockwood, J. L., and McKinney, M. L. (2001). Biotic Homogenization. New York, NY: Kluwer Academic/Plenum.
Meffert, P. J., and Dziock, F. (2013). The influence of urbanisation on diversity and trait composition of birds. Landsc. Ecol. 28, 943–957. doi: 10.1007/s10980-013-9867-z
Melles, S., Glenn, S., and Martin, K. (2003). Urban bird diversity and landscape complexity: species-environment associations along a multiscale habitat gradient. Conserv. Ecol. 7:5. doi: 10.5751/ES-00478-070105
Oksanen, J., Blanchet, F. G., Friendly, M., Kindt, R., Legendre, P., McGlinn, D., et al. (2018). vegan: Community Ecology Package. R package version 2.4-6. Available online at: https://CRAN.R-project.org/package=vegan
Paine, R. T. (1966). Food web complexity and species diversity. Am. Nat. 100, 65–75. doi: 10.1086/282400
Parsons, H., Major, R. E., and French, K. (2006). Species interactions and habitat associations of birds inhabiting urban areas of Sydney, Australia. Austral Ecol. 31, 217–227. doi: 10.1111/j.1442-9993.2006.01584.x
Patterson, B. D. (1980). Montane mammalian biogeography in New Mexico. Southwest. Nat. 25, 33–40. doi: 10.2307/3671209
Paz Silva, C., Sepulveda, R. D., and Barbosa, O. (2016). Nonrandom filtering effect on birds: species and guilds response to urbanization. Ecol. Evol. 6, 3711–3720. doi: 10.1002/ece3.2144
Pearse, W. D., Marcia Barbosa, A., Fritz, S. A., Keith, S. A., Harmon, L. J., Harte, J., et al. (2018). Building up biogeography: pattern to process. J. Biogeogr. 45, 1223–1230. doi: 10.1111/jbi.13242
Pulliam, R. H. (1988). Sources, sinks, and population regulation. Am. Nat. 132, 652–661. doi: 10.1086/284880
R Core Team (2018). R: A Language and Environment for Statistical Computing. R Foundation for Statistical Computing, Vienna.
Rodewald, P. (Ed). (2015). The Birds of North America. Ithaca, NY: Cornell Laboratory of Ornithology. Available online at: https://birdsna.org (Accessed January 2015 - March 2018).
RStudio Team (2015). RStudio: Integrated Development for R. Boston, MA: RStudio, Inc. Available online at: http://www.rstudio.com/
Sekhon, J. S. (2011). Multivariate and propensity score matching software with automated balance optimization: the matching package for R. J. Stat. Softw. 42, 1–52. doi: 10.18637/jss.v042.i07
Shochat, E., Lerman, S. B., Anderies, J. B., Warren, P. S., Faeth, S. H., and Nilon, C. H. (2010). Invastion, competition, and biodiversity loss in urban ecosystems. Bioscience 60, 199–208. doi: 10.1525/bio.2010.60.3.6
Sol, D., Gonzalez-Lagos, C., Moreira, D., Maspons, J., and Lapiedra, O. (2014). Urbanisation tolerance and the loss of avian diversity. Ecol. Lett. 17, 942–950. doi: 10.1111/ele.12297
Sullivan, B. L., Wood, C. L., Iliff, M. J., Bonney, R. E., Fink, D., and Kelling, S. (2009). eBird: a citizen-based bird observation network in the biological sciences. Biol. Conserv. 142, 2282–2292. doi: 10.1016/j.biocon.2009.05.006
Tallamy, D. W. (2004). Do alien plants reduce insect biomass? Conserv. Biol. 18, 1689–1692. doi: 10.1111/j.1523-1739.2004.00512.x
Thompson, J. D., Weiblen, G., Thomson, B. A., Alfaro, S., and Legendre, P. (1996). Untangling multiple factors in spatial distributions: Lilies, gophers, and rocks. Ecology 77, 1698–1715. doi: 10.2307/2265776
Turner, W. R. (2003). Citywide biological monitoring as a tool for ecology and conservation in urban landscapes: the case of the Tucson Bird Count. Landsc. Urban Plan. 65, 149–166. doi: 10.1016/S0169-2046(03)00012-4
van Heezik, Y., and Adams, A. L. (2016). Vulnerability of native and exotic urban birds to housing densification and changing gardening and landscaping trends. Urban Ecosyst. 19, 1551–1563. doi: 10.1007/s11252-014-0379-7
Veron, S., Davies, T. J., Cadotte, M. W., Clergeau, P., and Pavoine, S. (2017). Predicting loss of evolutionary history: where are we? Biol. Rev. 92, 271–291. doi: 10.1111/brv.12228
Webb, C. O. (2000). Exploring the phylogenetic structure of ecological communities: an example for rain forest trees. Am. Nat. 156, 145–155. doi: 10.1086/303378
Wickham, H. (2017). forcats: Tools for Working with Categorical Variables (Factors). R package version 0.2.0. Available online at: https://CRAN.R-project.org/package=forcats
Keywords: bird-habitat association, urbanization, traits, biotic homogenization, environmental filtering
Citation: Hensley CB, Trisos CH, Warren PS, MacFarland J, Blumenshine S, Reece J and Katti M (2019) Effects of Urbanization on Native Bird Species in Three Southwestern US Cities. Front. Ecol. Evol. 7:71. doi: 10.3389/fevo.2019.00071
Received: 31 July 2018; Accepted: 25 February 2019;
Published: 22 March 2019.
Edited by:
Darryl Jones, Griffith University, AustraliaReviewed by:
Iryna Dronova, University of California, Berkeley, United StatesWayne C. Zipperer, United States Forest Service (USDA), United States
Loren B. Byrne, Roger Williams University, United States
Copyright © 2019 Hensley, Trisos, Warren, MacFarland, Blumenshine, Reece and Katti. This is an open-access article distributed under the terms of the Creative Commons Attribution License (CC BY). The use, distribution or reproduction in other forums is permitted, provided the original author(s) and the copyright owner(s) are credited and that the original publication in this journal is cited, in accordance with accepted academic practice. No use, distribution or reproduction is permitted which does not comply with these terms.
*Correspondence: Christopher B. Hensley, Y2hyaXMuaGVuc2xleTE5QGdtYWlsLmNvbQ==