- 1Department of Crop and Soil Sciences, Washington State University, Pullman, WA, United States
- 2New Agronomics, Brooklyn, NY, United States
- 3Northwest Sustainable Agroecosystems Research Unit, United States Department of Agriculture, Agricultural Research Service, Pullman, WA, United States
- 4Department of Biological Systems Engineering, Washington State University, Pullman, WA, United States
Greenhouse gas (GHG) emissions result from short-term perturbations of agricultural systems such as precipitation and fertilization events. We hypothesized that those agricultural systems with contrasting management histories may respond differently to application events of water and N fertilizer with respect to GHG emissions. Studies with long-term management histories consisting of no-tillage (NT) and conventional tillage (CT) were coupled with high temporal resolution, automated chambers that monitored N2O and CO2 emissions for 22 h following treatments. Treatments applied to NT and CT were (a) control (no water or N additions), (b) simulated precipitation to achieve approximately 80% water-filled pore space, and (c) precipitation plus fertilizer additions of 150 kg N ha−1 as ammonium nitrate. Emissions of CO2 increased with increase in moisture and temperature and decreased under fertilizer application. Water and nitrogen treatments in CT at the sites with 2 and 12-year history produced N2O fluxes greater than NT by 142 and 68%, respectively. The site with 10-year history of NT produced similar amounts of N2O from CT and NT treatments. The same treatments at the site with 31 year-long NT history, despite being one of the lowest among all sites, demonstrated 380% higher N2O fluxes from the NT than CT, which was likely due to higher levels of labile organic matter present in NT treatments. GHG emissions data regressed on measured soil C and N properties, fractionation, and mineralization data showed that N2O flux increased with reduction of acid-hydrolyzable N and increase of NH4-N in soil, which suggested that N2O production in the short-term water and water and N additions events is mostly produced via nitrification process. This indicates that neither the length of NT treatment nor the fertilizer application rate define the rate of N2O emissions, but the soil N availability controlled by organic matter mineralization rate. The current study demonstrates the need for further research on the effects of the early stages of NT adoption as well as long-term NT on N2O spikes associated with artificial or natural rainfall events immediately following extended dry periods.
Introduction
Precipitation and soil N fertilization are factors that drive emissions of the greenhouse gases (GHG) nitrous oxide, a major contributor to climate change from agriculture (IPCC, 2007), and carbon dioxide. The C and N exchange with the atmosphere following rewetting by precipitation or irrigation after the period of sustained drought is known collectively as the Birch effect (Jarvis et al., 2011). Both N2O and CO2 emissions from soil are affected by factors such as temperature and water regimes, soil N status, and microbial activity due to carbon and nitrogen moving through ecosystems in coupled biogeochemical cycles (Li et al., 2005). Complexity of interactions between these factors results in high spatial and temporal variability of GHG emissions (Choudhary et al., 2002). Furthermore, management histories such as tillage practices can significantly impact all of these factors, as well as N and C cycling and storage by influencing inputs of N and C materials and the soil organic matter decomposition regime (Li et al., 2005; Smith et al., 2008). Assessment of factors affecting N2O flux from soils is therefore fundamental to determining the environmental conditions promoting N2O emissions (Forster et al., 2007).
Nitrogen fertilization and water additions have been reported to increase N2O emissions (Barton et al., 1999). Nitrogen fertilizer is often applied to meet the season-long demand of crops, consequently, N levels are commonly in excess of crop demand at the time of application. Application of N in excess of crop N requirements tends to exponentially increase N2O emissions (Shcherbak et al., 2014). Soil aeration status is related to the amount of water filled pore space (WFPS), as well as soil texture and structure, which regulate the movement of water and oxygen, thereby affecting the relative rates of nitrification and denitrification in soil (Wrage et al., 2001; Saggar et al., 2013). Emissions of N2O generally increase at 60–80% WFPS depending on soil texture and structure, with coarse-textured and well-structured soil requiring greater water additions than finer textured soils with little structure (Barton et al., 1999; Barton and Schipper, 2001). Emissions of CO2 increase immediately following water additions; however, N fertilization can have short-term reducing effects on CO2 emissions due to reduced enzyme activity and pH (Kowalenko et al., 1978; Wilson and Al-Kaisi, 2008; Gagnon et al., 2016). Previous research showed single events of very high fluxes of N2O and spikes of CO2 fluxes after N additions following rewetting, demonstrating importance of short-term events on overall GHG budgets (Nobre et al., 2001; Gelfand et al., 2015). Episodic production of N2O and CO2 associated with artificial or natural rainfall and N fertilization events emphasize the need to assess short-term emissions of N2O and CO2 from agricultural soils as influenced by different management histories.
No-tillage (NT) management is a conservation practice, which can sequester soil carbon, act as a sink of atmospheric CO2, preserve soil water and reduce erosion, while its effects on greenhouse gas emissions is less well-known. The N2O emissions were shown to generally stay the same following conversion from conventional tillage (CT) to NT in coarse-textured and fine-textured well-aerated soils, but tend to increase in fine-textured, poorly aerated soils, primarily due to denitrification (Six et al., 2002; Rochette, 2008). Longer-term adoption of NT has been reported to result in reduced contributions to GHGs through lower N2O emissions from soil (Six et al., 2004). Effects of tillage on CO2 emissions vary (BilandŽija et al., 2016), while being mostly dependent on soil moisture and temperature (Chavez et al., 2009). Due to existing controversy on the long-term effects of no-till on climate mitigation (Halvorson et al., 2008; Rochette, 2008; Stockle et al., 2012; Sainju et al., 2014; Bayer et al., 2016), field-level analysis is needed to address the vulnerability of GHG emissions to precipitation and N fertilization events as impacted by tillage management.
We conducted a study on short-term GHG emissions (CO2 and N2O) immediately following water and N additions in long-term CT and NT research sites representing dryland cropping as well as a more recently established irrigated site within the inland Pacific Northwest (iPNW). Our objectives were to (i) compare short-term N2O and CO2 emissions in CT and NT immediately following water and N fertilization additions; and (ii) assess factors affecting event-driven N2O emissions in cropping systems representative of the iPNW.
Materials and Methods
Field experiments were conducted between July 3 and July 28, 2013 in the long-term studies with tillage treatments, located in dryland and irrigated cropping regions of the iPNW. The summer timing for experiments was chosen as the highest levels of soluble soil C and total C are found in soils during that period, as well as potential denitrifier activity is increased (Shrewsbury et al., 2016). Summers are typically hot and dry in this Mediterranean-like climate and soils are vulnerable to high spikes of GHG emissions during rewetting events, which maximize expected treatment effects in contrasting long term tillage sites. The sites were located in Sherman Research Station, located in Moro, OR (45.48° N, 120.69° W), Columbia Basin Agricultural Research Center in Pendleton, OR (45.44° N, 118.37° W), Irrigated Agricultural Research Center in Prosser, WA (46.29° N, 119.74° W), and Kambitsch Farm of the University of Idaho in Genesee, ID (46.58° N, 116.95° W). The sites represent four agro-ecological classes (Kaur et al., 2017): (i) annual cropping at Kambitsch Farm in Genessee, ID, (ii) annual crop-fallow transition at Columbia Basin Agricultural Research Center in Pendleton, OR, (iii) grain-fallow at Sherman Research Station in Moro, OR, and (iv) irrigated at Irrigated Agricultural Research Center in Prosser, WA, and included tillage and no-tillage treatments established between 1982 and 2011 (Morrow et al., 2016) and were all planted to winter wheat (Triticum aestivum L.) at the time of monitoring.
We implemented an automated CO2 and N2O measurement mobile setup, which allowed the flexibility of moving equipment between sites. The system included Li-Cor 8100A automatic chambers coupled with LGR 23r N2O analyzer for continuous monitoring of CO2 and N2O emissions in a short-term micro-plot study. More details on the instrumentation setup can be found in Kostyanovsky et al. (2017). The experimental design at each site was a split-plot design with 3–4 replications. The main plot was the tillage treatment (CT and NT), and subplots 1 × 1 m2 were water addition and fertilizer treatments. The treatments applied at each site were: (1) no water (DRY); (2) water added approximately to 80% WFPS (H2O); and (3) water added to 80% WFPS and amended with 150 kg N ha−1 as NH4NO3 (H2O+N). The water addition mimicked a 59 mm rainfall event, achieved by distributing 59 L of water over the 1 × 1 m plot area. No observable runoff occurred during the water addition. Ammonium nitrate fertilizer was applied to ensure ammonium and nitrate are not limiting factors to the N2O emissions from treatments by allowing nitrification and denitrification processes to occur at the same time. Application of N and water took place at 9:00 a.m. and the measurements continued from that time until 7:00 a.m. the following day for a total of 22 h at the 2 h interval, for the total of 11 measurement cycles per plot. Our studies showed that GHG emissions were highest during the first day and decreased on the second and third days following water and N additions (Figure 1); therefore, the first 22 h appeared adequate for capturing diurnal dynamics and for treatment comparison, as it captured the highest N2O flux (Trost et al., 2013; Leitner et al., 2017; Mumford et al., 2019). Soil temperature (T, °C) and volumetric water content (θ, m3 m−3) at the depth of 20 cm was measured continuously at each plot with 5TM temperature and moisture sensors (METER, Pullman, WA, USA) to monitor any changes in soil conditions that could affect results. The study was conducted at the end of the vegetative period for wheat (Triticum aestivum, L) during the dry summer period with high daytime temperatures typical of the Mediterranean climate in July 2013. Greenhouse gas response to applied N and water would be expected to be maximal at this time (Shrewsbury et al., 2016).
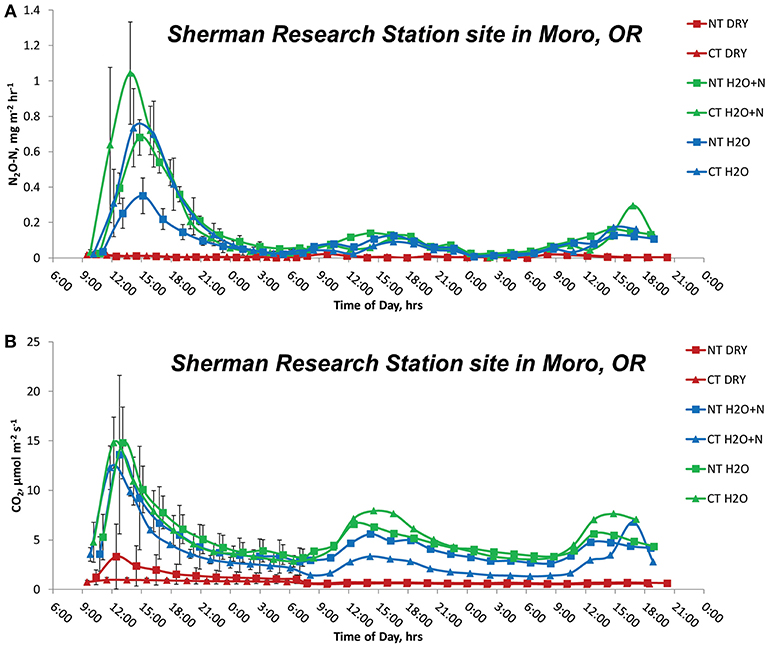
Figure 1. Emissions of N2O (A) and CO2 (B) during the first 3 days (0–72 h) of measurements with and without additions of 150 kg N ha−1 and water to no-tillage and conventional tillage management systems at Moro, OR site.
Soil sampling and analyses were conducted to aid assessment of factors that could affect N2O and CO2 emissions. Soil analytical methods and results are fully described and presented in Morrow et al. (2016). Briefly, soil was sampled at each site in CT and NT treatments at 0–10 cm depth in July 2013, just prior to the GHG chamber study, and analyzed for bulk density, pH in 1:1 DI water extract, total organic carbon (TOC), and total nitrogen via dry combustion, and 1M KCl extracted nitrate (-N) and ammonium (-N) (Table 1). Furthermore, soil C and N fractions were analyzed including non-hydrolyzable C and N, hydrolysable C and N, microbial biomass C and N, and water extractable C and N. Potential carbon mineralization was measured at 3, 10, 17, and 24 days, anaerobic N mineralization was determined at 28 days, and ion exchange membrane and were measured after 24 h (Morrow et al., 2016).
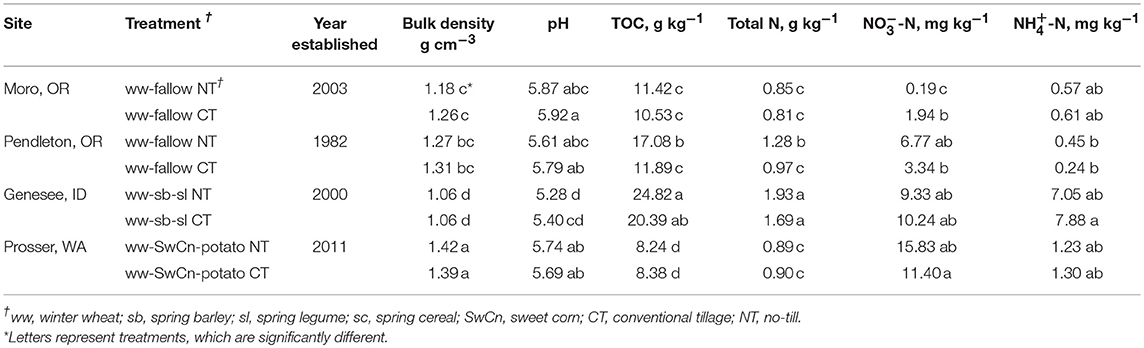
Table 1. Site crop rotations and soil properties (0–10 cm depth) at no-tillage and conventional tillage winter wheat plots across inland Pacific Northwest.
The change in concentration of the N2O mole fractions was determined via linear regression, and the R2 values were computed in Matlab R2011a (The Mathworks, Inc., Natick, MA). The first 30 s of N2O concentrations in the 8 min (480 s) measurement were eliminated from regression analysis, following the standard Li-Cor 8100A algorithm for calculation of the CO2 fluxes.
The GHG emissions and soil water and temperature data were analyzed for the effects of time, treatment and tillage system by the proc mixed procedure using Satterthwaite method in SAS (SAS 9.3, Cary, NC). Treatment effects at each time step were determined via the Differences of Least Squares Means analysis (P < 0.05 within proc mixed procedure). The effects of soil water and temperature on N2O and CO2 emissions were determined via stepwise multiple regression in proc reg procedure of SAS (SAS9.3, Cary, NC). Stepwise multiple regression was also used to determine which soil carbon and nitrogen fraction, potential C and N mineralization, and ion exchange membrane nitrogen variables best describe the cumulative N2O and CO2 flux over 22 h in prog reg (SAS 9.3, Cary, NC). Soil pH, TOC, TN, N, and -N were analyzed for treatment and tillage effects in SAS by proc mixed procedure with Tukey-Kramer difference of least squares means adjustment (SAS 9.3, Cary, NC).
Results and Discussion
Soil Water and Temperature Fluctuations
Additions of water, and water plus N to 1 × 1 m2 subplots resulted in similar moisture and temperature levels across tillage treatments at individual times at all sites (P > 0.05) (Table 2). Soil water content was higher in all treatments with water (H2O+N and H2O, P < 0.0001) than in treatments with no water additions (DRY, P < 0.0001), whereas soil temperatures were lower in treatments with water (H2O+N and H2O, P < 0.0001), as compared to treatments with no water additions (DRY, P < 0.0001). Therefore, treatments with water and treatments with no water were averaged across tillage treatments (Figures 2, 3). Here, after additions of water plus N and water only, soil water content spiked, and then gradually decreased over the course of 22 h (Figure 2). In contrast, soil temperature was generally higher in no water added treatments than in treatments with water additions at all sites (Figure 3).
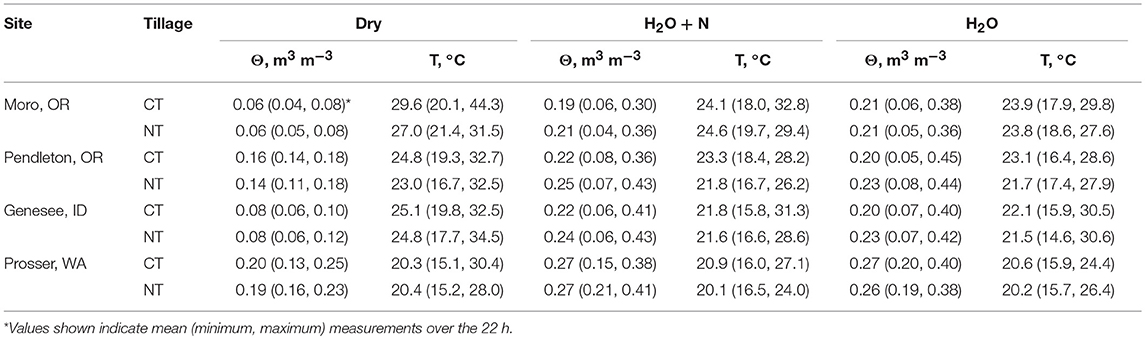
Table 2. Soil temperature and moisture (20-cm depth) at no-tillage and conventional tillage winter wheat sites across inland Pacific Northwest for treatments without water additions, with water plus N added, and with water only added.
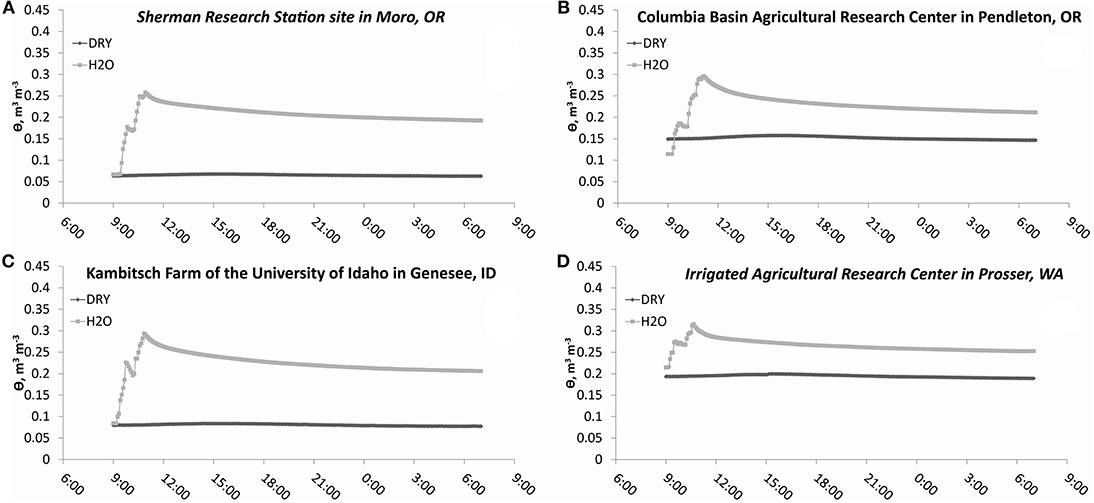
Figure 2. Soil water (20 cm depth) fluctuations in dry treatments and treatments with water additions during study over the course of 22 h. DRY is the average of CT DRY and NT DRY; H2O is the average of CT H2O+N, NT H2O+N, CT H2O, and NT H2O treatments. Water filled pore space reached 60% at the following moisture contents: (A) 0.27 m3 m−3, (B) 0.29 m3 m−3 (C) 0.24 m3 m−3 (D) 0.31 m3 m−3.
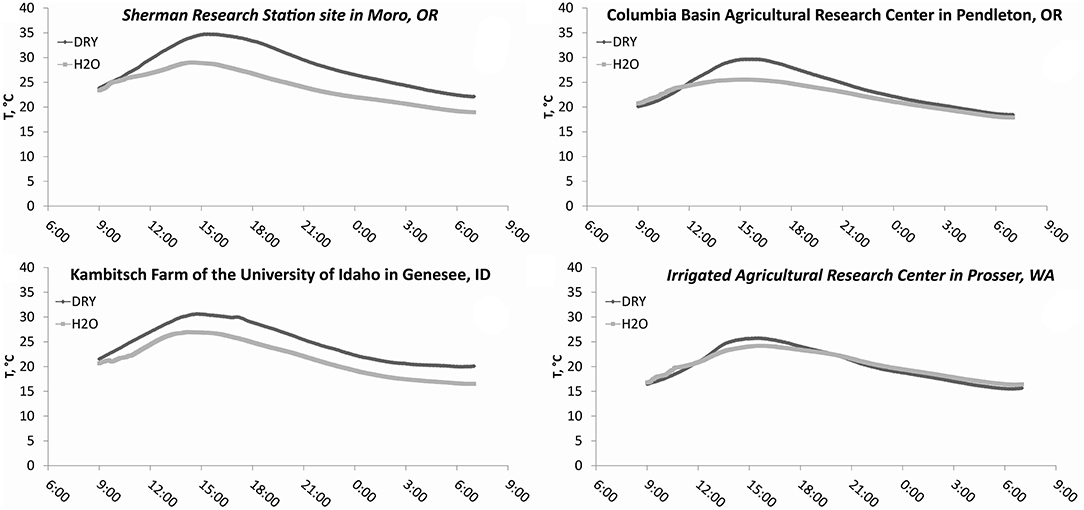
Figure 3. Soil temperature (20 cm depth) fluctuations in dry treatments and treatments with water additions during study over the course of 22 h. DRY is the average of CT DRY and NT DRY; H2O is the average of CT H2O+N, NT H2O+N, CT H2O, and NT H2O treatments.
N2O and CO2 Emissions at Individual Sites
Higher N2O peaks occurred for H2O+N treatments than H2O treatments in both dryland and irrigated scenarios (Figure 4). Both H2O+N and H2O treatments had higher N2O and CO2 emissions than the no water treatments (Figures 4, 5). The average cumulative emissions of N2O and CO2 over the 22 h are also shown in Table 3 to aid the interpretation of the Figures 3, 4. These results are consistent with the studies on fertilizer and water additions to non-irrigated soil (Ruser et al., 2006; Sainju et al., 2014; Perego et al., 2016). The exponential increase in N2O emissions during the first 4–8 h following water and N additions was likely due to achieving the WFPS and sufficient soil N to promote nitrification and denitrification processes (Barton et al., 1999; Shcherbak et al., 2014).
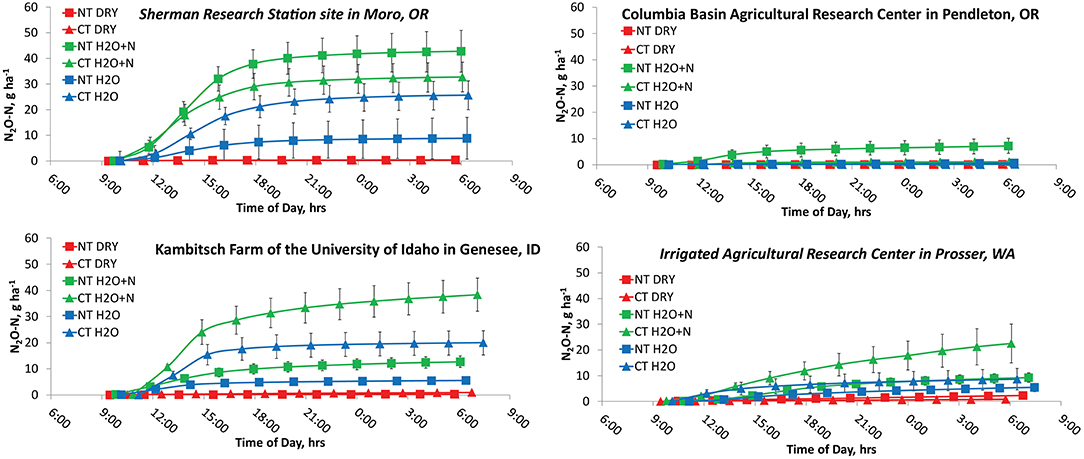
Figure 4. Cumulative emissions of N2O during the first day (0–22 h) of measurements with and without additions of 150 kg N ha−1 and water to no-tillage and conventional tillage management systems.
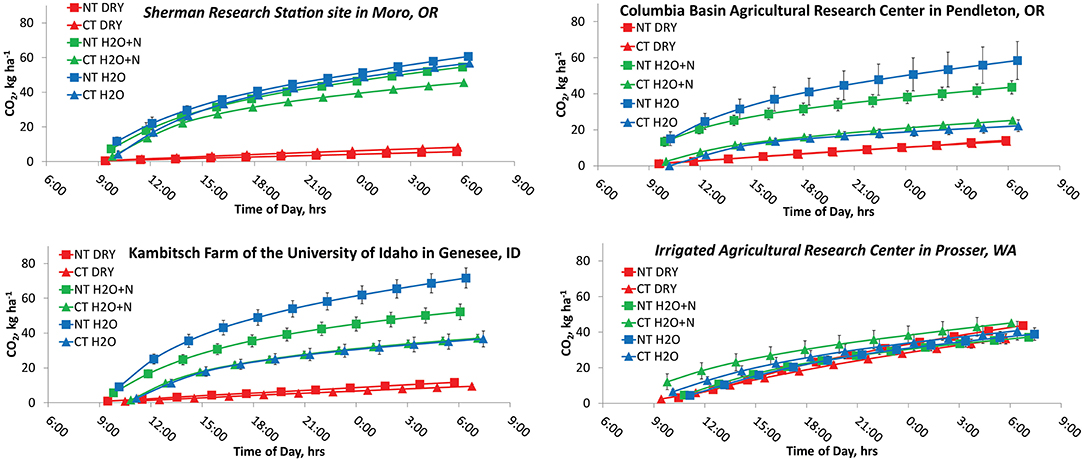
Figure 5. Cumulative emissions of CO2 during the first day (0–22 h) of measurements with and without additions of 150 kg N ha−1 and water to no-tillage and conventional tillage management systems.
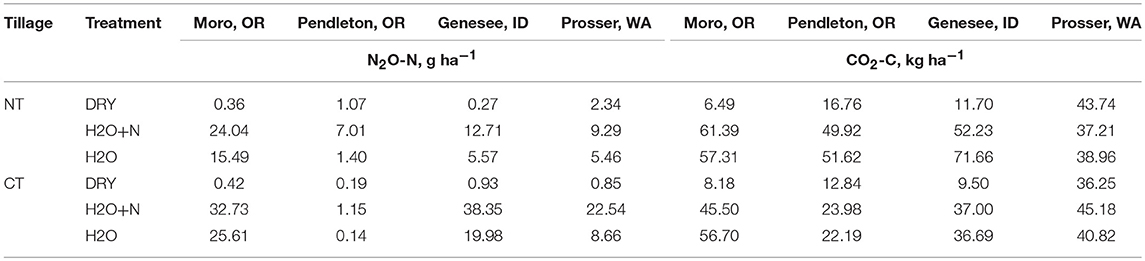
Table 3. Summary table for cumulative emissions of N2O and CO2 during the first day (0–22 h) of measurements with and without additions of 150 kg N ha−1 and water to no-tillage and conventional tillage management systems.
The Sherman Research Station site in Moro, OR had significant effects of treatment (P < 0.0001), time (P = 0.0131), and interactions of treatment by tillage (P = 0.0148) on cumulative N2O emissions (Figure 4A). The N2O emissions in the NT peaked at 15:00, and NT H2O + N produced 42.5 ± 15.0 g N2O-N ha−1 more than the NT DRY treatment, and 33.9 ± 15.0 g N2O-N ha−1 more than the NT H2O treatment over 22 h. The CT treatment N2O emissions also peaked at 15:00. The CT H2O+N and CT H2O treatments resulted in N2O production higher than the CT DRY treatment by 32.3 ± 15.0 g N2O-N ha−1 and 25.2 ± 15.0 g N2O-N ha−1, respectively. The CT H2O + N treatment produced 23.9 ± 15.0 g N2O-N ha−1 more over 22 h than the NT H2O, at the comparatively low probability (P = 0.115). The CO2 emissions produced highest peaks at 11:00 and showed treatment by tillage (P = 0.0328) and treatment by time (P < 0.0001) interactions. Emissions in the NT were higher from H2O and H2O + N than the DRY treatment by 50.8-54.9 ± 6.7 kg CO2-C ha−1 over 22 h, and in CT by 37.3–48.5 ± 6.7 kg CO2-C ha−1.
Similar N2O fluxes from the CT and NT treatments at the Moro, OR site despite increased levels of N in CT (Table 1) suggest, that denitrification was not likely the major driving source for N2O emissions at the levels of WFPS established in the experiment (Figures 2A, 4A). Production of N2O as a byproduct of nitrification process is a recognized source of N2O emissions in unsaturated soils (Wrage et al., 2001; Kool et al., 2011); therefore, differences in organic N mineralization and nitrification following the boost in microbial activity from water additions was a likely source of treatment effects observed at this site.
Columbia Basin Agricultural Research Center in Pendleton, OR showed a treatment by tillage interaction (P < 0.0001) for N2O and CO2 emissions. The levels of N2O in the NT peaked at 15:00 and were 5.9 ± 1.0 g N2O-N ha−1 higher in the NT H2O+N than the NT DRY treatment (Figure 4B). The NT H2O+N treatment produced 5.9 ± 1.2 g N2O-N ha−1 more over 22 h than the CT H2O + N (P < 0.0001). The CO2 emissions peaked at 11:00 and were higher by 32.5–33.1 ± 7.0 kg CO2-C ha−1 over 22 h in the NT H2O and NT H2O-N treatments compared to NT DRY (Figure 5B). The CO2 emissions were also higher by 9.3–11.1 ± 9.8 kg CO2-C ha−1 over 22 h in the CT H2O and CT H2O + N treatments compared to DRY.
The N2O emissions produced from the NT treatments were higher than CT, possibly due to higher levels of soil organic matter in the NT plots, indicated by the total organic C and the total N, which were higher in NT than CT by 5.20 ± 1.38 kg C m−3 (P = 0.0165) and 0.31 ± 0.09 kg N m−3 (P = 0.0270), respectively. The CO2 emissions were similar in CT and NT, which was consistent with previous research (Chavez et al., 2009).
Kambitsch Farm of the University of Idaho in Genesee, ID showed a treatment by tillage interaction (P < 0.0001) on N2O, and a treatment by tillage (P < 0.0001) and treatment by time (P < 0.0001) interaction on CO2 emissions. The levels of N2O peaked at 15:00 in the NT and at 13:00 in the CT. In the NT, H2O+N produced 12.4 ± 5.6 g N2O-N ha−1 more over 22 h than the DRY treatment (Figure 4C). In CT, H2O and H2O + N produced 19.0–37 ± 5.6 g N2O-N ha−1 more over 22 h than the DRY. The H2O + N CT treatment produced 7.2 ± 5.6 g N2O-N ha−1 more over 22 h than the H2O + N NT, at a comparatively low probability (P = 0.196). The levels of CO2 peaked at 21:00 in the DRY and at 11:00 in the H2O+N and H2O treatments in both CT and NT. Both H2O + N and H2O were higher than DRY after 22 h in CT and NT. Interestingly, unlike other sites, at Genessee, ID the NT the H2O treatment emitted more CO2 than H2O-N by 19.4 ± 5.7 kg CO2-C ha−1 over 22 h (Figure 5C).
Slightly higher N2O rates from the CT treatments measured at this 12 year research site are consistent with the existing studies (Six et al., 2004; Rochette, 2008). Decrease in CO2 emissions in the H2O + N treatment compared to the H2O is likely due to the effects of in the added fertilizer, which was previously reported to suppress heterotrophic and autotrophic respiration by 20% at the application rate of 150 kg N ha−1 (Gagnon et al., 2016).
Irrigated Agricultural Research Center in Prosser, WA showed increase in N2O emissions in CT from the H2O + N compared to DRY at 17:00 only. This was likely due to the lack of contrasting WFPS in treatments as the site was irrigated on the 3 day interval prior to the study, and despite no irrigation for 3 days prior to our GHG experiment. Previous research indicated N2O and CO2 increase primarily occurs in the dryland sites when the period of drought is followed by water additions or natural rainfall event (Barton et al., 1999; Barton and Schipper, 2001). The H2O + N CT treatment produced 21.4 ± 12.7 g N2O-N ha−1 more over 22 h than the H2O + N NT (Figure 4D), at a comparatively low probability (P = 0.0938).
Site Comparisons
Analysis of N2O emissions from all sites showed the site by tillage interaction (P < 0.0001, n = 3), as well as treatment by tillage by time by site interaction (P < 0.0001, n = 244), suggesting existence of strong effects of tillage (NT and CT) and site on N2O emissions over the 22-h period across the agro-ecological classes. These effects are compounded by water additions and N additions to soil.
The data on the 22-h greenhouse gas emissions following fertilization and water additions indicated NT tended to produce higher levels of N2O than CT in winter wheat rotation with fallow, and CT treatments tended to produce higher levels of N2O than NT in winter wheat rotations without fallow during that time. Emissions of N2O from the plots with no water or N added were negligible. In dryland sites, all water and N and water only treatments had higher CO2 emissions than treatments without water added. Emissions of CO2 generally increased in NT in the H2O treatments compared to H2O + N during the 22-h period (Figures 5B,C).
To describe the effects of soil water and temperature on N2O emissions, we fitted the soil water and temperature data across sites into a model via stepwise multiple regression (Table 4). The measurable effect of soil rewetting on N2O emissions shows the importance of those events on total N2O budget in the context of intensification of the water cycle with climate change (Ruser et al., 2006; Betts et al., 2007; Rochette, 2008); (Mangalassery et al., 2015).
The cumulative N2O and CO2 flux over the 22 h period was also regressed on the C and N soil properties, and returned the following models:
where HN, mg g−1–acid-hydrolysable N, NH4-N, mg g−1–ion exchange membrane NH4-N.
where MBC, mg g−1–microbial biomass C, Cmin24, mg g−1–potentially mineralized C at 24 days.
The N2O flux increased with reduction of acid-hydrolyzable N and increase of NH4-N in soil, which suggests that N2O production in the short-term water additions events is mostly produced via nitrification process. That was consistent with previous research indicating nitrifier denitrification as a major source of N2O in semi-saturated conditions (Wrage et al., 2001; Kool et al., 2011). Nitrifier denitrification was also demonstrated to be the major pathway of N2O production in agricultural soils by δ15N isotope technique in recent research (Snider et al., 2017). The CO2 flux was negatively affected by pH, and increased with decrease in microbial biomass and increase in potential C mineralization. As C mineralization is commonly associated with narrow C/N ratios (Haney et al., 2012), and decrease in pH with increased levels of N, the factors affecting CO2 production levels were consistent with previous research (Wilson and Al-Kaisi, 2008; Saggar et al., 2013).
The Pendleton, OR site resulted in increased N2O emissions from the NT H2O + N, while in the rest of the treatments N2O emissions were negligible. This was complemented by higher levels of organic matter in the NT plots, indicated by the total organic C and total N (Table 1). The study conducted at the same plots by Morrow et al. (2016) also reported increased rates of potential N mineralization over 24 days (PNM) in the NT than CT, which were 53.9 mg N kg−1 and 18.7 mg N kg−1, respectively. The same study found increased levels of permanganate oxidizable C (POXC) at the NT treatment at Pendleton, OR site. The rest of the sites did not show differences in PNM (Morrow et al., 2016). Pendleton, OR was also the only site to show increased cumulative C mineralization at day 1 (Cmin0−1d) in the NT compared to CT, which was 55 g CO2-C kg−1 and 38 g CO2-C kg−1, respectively (Morrow et al., 2016).
High rates of both N2O and CO2 emissions were observed at the Genesee, ID site (Figures 4C, 5C). This site also had the highest levels of total organic C and total N, as well as the lowest soil bulk density (Table 1). Though the magnitude of N2O emissions at the Genesee, ID site was larger, the pattern of emissions was consistent with the N2O emissions in Prosser, WA and partially in Moro, OR, where NT had reduced or similar N2O emissions compared to CT. Therefore, the only factors that make Genesee, ID, Moro, OR and Prosser, WA sites different from Pendleton, OR are soil C and N characteristics, PNM and Cmin0−1d, which are indicators of increased N availability in contrasting treatments.
The more detailed study on soil C and N characteristics at the sites of interest reported in Morrow et al. (2016) show significant increases of SOC and POXC with NT compared to CT (Table 5). Differences in crop rotation and associated soil tillage intensity rating (STIR) (USDA-NRCS, 2006), which are incorporated in the site effect in data analysis on the N2O and CO2 emissions, could also contribute to the variability of N2O and CO2 emissions in the current study.
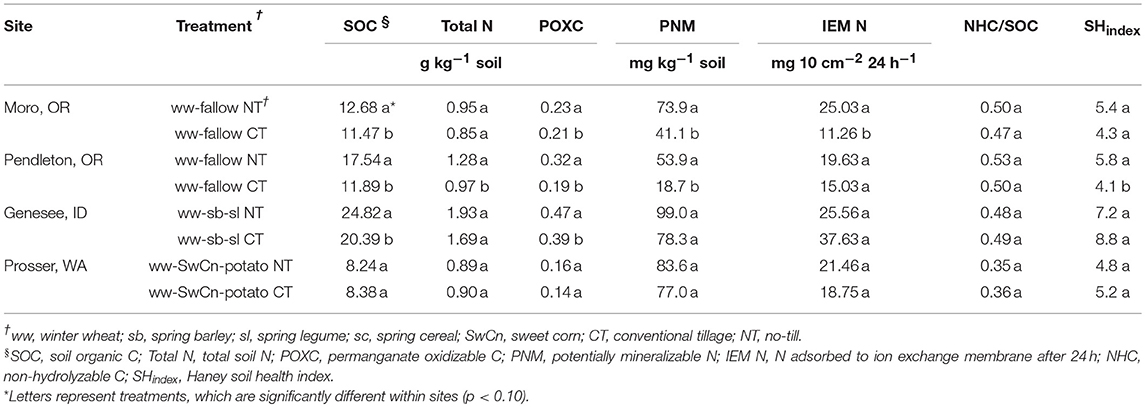
Table 5. Soil health metrics (0–10 cm depth) at no-tillage and conventional tillage winter wheat study sites across inland Pacific Northwest (Morrow et al., 2016).
Our findings showed that N2O emissions following short-term artificial or natural rainfall events are likely controlled by soil N availability coupled with the rate of organic matter mineralization (Robertson et al., 2000; Li et al., 2005; Gelfand et al., 2015). It is conceivable, that immediate application of N fertilizer alone does not determine susceptibility of tillage treatments to increase in N2O and CO2 emissions following a soil saturation event but they could be affected by tillage in combination with fertilizer-N use efficiency and the cropping system, i.e., crop rotation incorporated in the site factor in the current study (Van Groenigen et al., 2010). Our future research will continue to address the effects of short-term events on GHG emissions in conventional and no-tillage cropping systems, as well as elucidate the role of length of no-till treatment and the fertilizer application rate in annual N2O and CO2 emissions (Barton et al., 1999; Six et al., 2004).
Conclusions
Overall, the current study showed that emissions of CO2 increase during short-term events with increase in moisture and rise of temperature during daytime, and decrease under NH4NO3 application; however, the spike in N2O emissions is likely to eliminate the partial reduction of GHG emissions. Our results suggest that NT could reduce N2O emissions compared to CT during the short-term irrigation and N fertilization events; however, this effect was likely compounded by the site effect, which significantly includes crop rotation and the soil tillage intensity rating (STIR). The active fraction of soil organic matter plays a crucial role in soil's ability to reduce the N2O emissions. Our data shows that further studies are needed to investigate the effects of the early stages of no-till adoption as well as long-term no-till on spikes of N2O in dry conditions followed by short-term artificial or natural rainfall events.
Author Contributions
KK designed the study layouts at the sites, assembled the N2O and CO2 analyzer setups, conducted greenhouse gas emissions measurements, analyzed data and wrote the manuscript. DH as a principal PI on the USDA-NIFA project, obtained funds to supply instrumentation and workforce to conduct the study, located the long-term tillage, no-till sites within the irrigated and dryland management systems in inland Pacific Northwest, discussed the details of the study design, and data analysis with the corresponding author, edited the manuscript. CS as a PI on the USDA-NIFA project, obtained funds to supply instrumentation and workforce to conduct the study, discussed the treatments in order to obtain contrasting N2O and CO2 emissions effects during the study, provided suggestions for data analysis, and edited the manuscript. JM assisted in conducting greenhouse gas emissions measurements, collected and analyzed soil samples from respective treatments, discussed data analysis and edited the manuscript. IM designed the irrigated long-term sites, assisted in conducting the GHG measurements, discussed data analysis and edited the manuscript.
Conflict of Interest Statement
The authors declare that the research was conducted in the absence of any commercial or financial relationships that could be construed as a potential conflict of interest.
The handling editor and reviewer, EB, declared their involvement as co-editors in the Research Topic.
Acknowledgments
We are deeply grateful to Riley Devine and Joshua Gefrey for their invaluable assistance conducting the experiments across three states. We would like to express the special thanks to Robert Barry for equipment preparation.
References
Barton, L., McLay, C. D. A., Schipper, L. A., and Smith, C. T. (1999). Annual denitrification rates in agricultural and forest soils: a review. Aust. J. Soil Res. 37, 1073–1093. doi: 10.1071/SR99009
Barton, L., and Schipper, L. A. (2001). Regulation of nitrous oxide emissions from soils irrigated with dairy farm effluent. J. Environ. Qual. 30, 1881–1887. doi: 10.2134/jeq2001.1881
Bayer, C., Gomes, J., Zanatta, J. A., Vieira, F. C. B., and Dieckow, J. (2016). Mitigating greenhouse gas emissions from a subtropical Ultisol by using long-term no-tillage in combination with legume cover crops. Soil Tillage Res. 161, 86–94. doi: 10.1016/j.still.2016.03.011
Betts, R. A., Boucher, O., Collins, M., Cox, P. M., Falloon, P. D., Gedney, N., et al. (2007). Projected increase in continental runoff due to plant responses to increasing carbon dioxide. Nature 448, 1037–1041. doi: 10.1038/nature06045
BilandŽija, D., Zgorelec, Ž., and Kisić, I. (2016). Influence of tillage practices and crop type on soil CO2 emissions. Sustainability 8:90. doi: 10.3390/su8010090
Chavez, L. F., Amado, T. J. C., Bayer, C., La Scala, N., Escobar, L. F., Fiorin, J. E., et al. (2009). Carbon dioxide efflux in a rhodic hapludox as affected by tillage systems in Southern Brazil. Rev. Bras. Cienc. Solo 33, 325–334. doi: 10.1590/S0100-06832009000200010
Choudhary, M. A., Akramkhanov, A., and Saggar, S. (2002). Nitrous oxide emissions from a New Zealand cropped soil: tillage effects, spatial and seasonal variability. Agric. Ecosyst. Environ. 93, 33–43. doi: 10.1016/S0167-8809(02)00005-1
Forster, P. V., Ramaswamy, P., Artaxo, T., Berntsen, R., Betts, D. W., Fahey, J. Van Dorland, et al. (2007). “Changes in atmospheric constituents and in radiative forcing,” in Climate Change 2007. "The Physical Science Basis. Contribution of Working Group I to the Fourth Assessment Report of the Intergovernmental Panel on Climate Change, eds S. Solomon, D. Qin, M. Manning, Z. Chen, M. Marquis, K. B. Averyt, M. Tignor, and H. L. Miller (Cambridge; New York, NY: Cambridge University Press), 129–234.
Gagnon, B., Ziadi, N., Rochette, P., Chantigny, M. H., Angers, D. A., Bertrand, N., et al. (2016). Soil-surface carbon dioxide emission following nitrogen fertilization in corn. Can. J. Soil Sci. 96, 219–232. doi: 10.1139/cjss-2015-0053
Gelfand, I., Cui, M., Tang, J., and Robertson, G. P. (2015). Short-term drought response of N2O and CO2 emissions from mesic agricultural soils in the US Midwest. Agric. Ecosyst. Environ. 212, 127–133. doi: 10.1016/j.agee.2015.07.005
Halvorson, A. D., Del Grosso, S. J., and Reule, C. A. (2008). Nitrogen, tillage, and crop rotation effects on nitrous oxide emissions from irrigated cropping systems. J. Environ. Qual. 37, 1337–1344. doi: 10.2134/jeq2007.0268
Haney, R. L., Franzluebbers, A. J., Jin, V. L., Johnson, M., Haney, E. B., White, M. J., Harmel, et al. (2012). Soil organic C:N vs. water-extractable organic C:N. Open J. Soil Sci. 2, 269–274. doi: 10.4236/ojss.2012.23032
IPCC (2007). “Chapter 2. Changes in atmospheric constituents and in radiative forcing,” in The Physical Science Basis, eds P. Forster, V. Ramaswamy, P. Artaxo, T. Berntsen, R. Betts, D. W. Fahey, J. Haywood, J. Lean, D. C. Lowe, G. Myhre, J. Nganga, R. Prinn, G. Raga, M. Schulz and R. Van Dorland (Cambridge; New York, NY: Cambridge University Press), 130–234.
Jarvis, P., Rey, A., Petsikos, C., Wingate, L., Rayment, M., Pereira, J., et al. (2011). Drying and wetting of Mediterranean soils stimulates decomposition and carbon dioxide emission: the “Birch effect”. Tree Physiol. 27, 929–940. doi: 10.1093/treephys/27.7.929
Kaur, H., Huggins, D. R., Rupp, R. A., Abatzoglou, J. T., Stöckle, C. O., and Reganold, J. P. (2017). Future Bioclimatic Drivers Increase Uncertainty of Dryland Agro-ecological Classes of the Inland Pacific Northwest, USA. Front Ecol. Evol. 5:74. doi: 10.3389/fevo.2017.00074
Kool, D. M., Dolfing, J., Wrage, N., and Van Groenigen, J. W. (2011). Nitrifier denitrification as a distinct and significant source of nitrous oxide from soil. Soil Biol. Biochem. 43, 174–178. doi: 10.1016/j.soilbio.2010.09.030
Kostyanovsky, K. I., Huggins, D. R., Stockle, C. O., Waldo, S., and Lamb, B. (2017). Developing a flow through chamber system for automated measurements of soil N2O and CO2 emissions. Measurement 113, 172–180. doi: 10.1016/j.measurement.2017.05.040
Kowalenko, C. G., Ivarson, K. C., and Cameron, D. R. (1978). Effect of moisture content temperature and nitrogen fertilization on carbon di oxide evolution from field soils. Soil Biol. Biochem. 10, 417–424. doi: 10.1016/0038-0717(78)90068-8
Leitner, S., Homyak, P. M., Blankinship, J. C., Eberwein, J., Jenerette, G. D., Zechmeister-Boltenstern, S., et al. (2017). Linking NO and N2O emission pulses with the mobilization of mineral and organic N upon rewetting dry soils. Soil Biol. Biochem. 115, 461–466. doi: 10.1016/j.soilbio.2017.09.005
Li, C. S., Frolking, S., and Butterbach-Bahl, K. (2005). Carbon sequestration in arable soils is likely to increase nitrous oxide emissions, offsetting reductions in climate radiative forcing. Clim. Change 72, 321–338. doi: 10.1007/s10584-005-6791-5
Mangalassery, S., Sjogersten, S., Sparkes, D. L., and Mooney, S. J. (2015). Examining the potential for climate change mitigation from zero tillage. J. Agric. Sci. 153, 1151–1173. doi: 10.1017/S0021859614001002
Morrow, J. G., Huggins, D. R., Carpenter-Boggs, L. A., and Reganold, J. P. (2016). Evaluating measures to assess soil health in long-term agroecosystem trials. Soil Sci. Soc. Am. J. 80, 450–462. doi: 10.2136/sssaj2015.08.0308
Mumford, M. T., Rowlings, D. W., Scheer, C., De Rosa, D., and Grace, P. R. (2019). Effect of irrigation scheduling on nitrous oxide emissions in intensively managed pastures. Agric. Ecosyst. Environ. 272, 126–134. doi: 10.1016/j.agee.2018.11.011
Nobre, A. D., Keller, M., Crill, P. M., and Harriss, R. C. (2001). Short-term nitrous oxide profile dynamics and emissions response to water, nitrogen and carbon additions in two tropical soils. Biol. Fertil. Soils 34, 363–373. doi: 10.1007/s003740100396
Perego, A., Wu, L., Gerosa, G., Finco, A., Chiazzese, M., and Amaducci, S. (2016). Field evaluation combined with modelling analysis to study fertilizer and tillage as factors affecting N2O emissions: a case study in the Po valley (Northern Italy). Agric. Ecosyst. Environ. 225, 72–85. doi: 10.1016/j.agee.2016.04.003
Robertson, G. P., Paul, E. A., and Harwood, R. R. (2000). Greenhouse gases in intensive agriculture: contributions of individual gases to the radiative forcing of the atmosphere. Science 289, 1922–1925. doi: 10.1126/science.289.5486.1922
Rochette, P. (2008). No-till only increases N2O emissions in poorly-aerated soils. Soil Tillage Res. 101, 97–100. doi: 10.1016/j.still.2008.07.011
Ruser, R., Flessa, H., Russow, R., Schmidt, G., Buegger, F., and Munch, J. C. (2006). Emission of N2O, N-2 and CO2 from soil fertilized with nitrate: Effect of compaction, soil moisture and rewetting. Soil Biol. Biochem. 38, 263–274. doi: 10.1016/j.soilbio.2005.05.005
Saggar, S., Jha, N., Deslippe, J., Bolan, N. S., Luo, J., Giltrap, D. L., et al. (2013). Denitrification and N2O:N-2 production in temperate grasslands: Processes, measurements, modelling and mitigating negative impacts. Sci. Total Environ. 465, 173–195. doi: 10.1016/j.scitotenv.2012.11.050
Sainju, U. M., Stevens, W. B., Caesar-TonThat, T., Liebig, M. A., and Wang, J. (2014). Net global warming potential and greenhouse gas intensity influenced by irrigation, tillage, crop rotation, and nitrogen fertilization. J. Environ. Qual. 43, 777–788. doi: 10.2134/jeq2013.10.0405
Shcherbak, I., Millar, N., and Robertson, G. P. (2014). Global metaanalysis of the nonlinear response of soil nitrous oxide (N2O) emissions to fertilizer nitrogen. Proc. Natl. Acad. Sci. U.S.A. 111, 9199–9204. doi: 10.1073/pnas.1322434111
Shrewsbury, L. H., Smith, J. L., Huggins, D. R., Carpenter-Boggs, L., and Reardon, C. L. (2016). Denitrifier abundance has a greater influence on denitrification rates at larger landscape scales but is a lesser driver than environmental variables. Soil Biol. Biochem. 103, 221–231. doi: 10.1016/j.soilbio.2016.08.016
Six, J., Feller, C., Denef, K., Ogle, S. M., Sa, J. C. D., and Albrecht, A. (2002). Soil organic matter, biota and aggregation in temperate and tropical soils - Effects of no-tillage. Agronomie 22, 755–775. doi: 10.1051/agro:2002043
Six, J., Ogle, S. M., Breidt, F. J., Conant, R. T., Mosier, A. R., and Paustian, K. (2004). The potential to mitigate global warming with no-tillage management is only realized when practised in the long term. Glob. Chang. Biol. 10, 155–160. doi: 10.1111/j.1529-8817.2003.00730.x
Smith, P., Martino, D., Cai, Z., Gwary, D., Janzen, H., Kumar, P., et al. (2008). Greenhouse gas mitigation in agriculture. Philos. Trans. R. Soc. B Biol. Sci. 363, 789–813. doi: 10.1098/rstb.2007.2184
Snider, D. M., Wagner-Riddle, C., and Spoelstra, J. (2017). Stable isotopes reveal rapid cycling of soil nitrogen after manure application. J. Environ. Qual. 46, 261–271. doi: 10.2134/jeq2016.07.0253
Stockle, C., Higgins, S., Kemanian, A., Nelson, R., Huggins, D., Marcos, J., et al. (2012). Carbon storage and nitrous oxide emissions of cropping systems in eastern Washington: a simulation study. J. Soil Water Conserv. 67, 365–377. doi: 10.2489/jswc.67.5.365
Trost, B., Prochnow, A., Drastig, K., Meyer-Aurich, A., Ellmer, F., and Baumecker, M. (2013). Irrigation, soil organic carbon and N2O emissions. A review. Agronomy Sust. Dev. 33, 733–749. doi: 10.1007/s13593-013-0134-0
USDA-NRCS (2006). The Soil Tillage Intensity Rating (STIR). TN Agronomy No. 50. USDA-NRCS, Boise, ID.
Van Groenigen, J. W., Velthof, G. L., Oenema, O., Van Groenigen, K. J., and Van Kessel, C. (2010). Towards an agronomic assessment of N2O emissions: a case study for arable crops. Eur. J. Soil Sci. 61, 903–913. doi: 10.1111/j.1365-2389.2009.01217.x
Wilson, H. M., and Al-Kaisi, M. M. (2008). Crop rotation and nitrogen fertilization effect on Soil CO2 emissions in central lowa. Appl. Soil Ecol. 39, 264–270. doi: 10.1016/j.apsoil.2007.12.013
Keywords: nitrous oxide, carbon dioxide, dryland cropping systems, irrigated crops, N fertilization
Citation: Kostyanovsky KI, Huggins DR, Stockle CO, Morrow JG and Madsen IJ (2019) Emissions of N2O and CO2 Following Short-Term Water and N Fertilization Events in Wheat-Based Cropping Systems. Front. Ecol. Evol. 7:63. doi: 10.3389/fevo.2019.00063
Received: 30 March 2017; Accepted: 19 February 2019;
Published: 24 April 2019.
Edited by:
Jodi Lynn Johnson-Maynard, University of Idaho, United StatesReviewed by:
Yong Liu, Hunan Academy of Agricultural Sciences (CAAS), ChinaErin Brooks, University of Idaho, United States
Copyright © 2019 Kostyanovsky, Huggins, Stockle, Morrow and Madsen. This is an open-access article distributed under the terms of the Creative Commons Attribution License (CC BY). The use, distribution or reproduction in other forums is permitted, provided the original author(s) and the copyright owner(s) are credited and that the original publication in this journal is cited, in accordance with accepted academic practice. No use, distribution or reproduction is permitted which does not comply with these terms.
*Correspondence: Kirill I. Kostyanovsky, kkostya@vt.edu