- 1Department of Animal Ecology and Physiology, Institute for Water and Wetland Research, Radboud University, Nijmegen, Netherlands
- 2Rijn en IJssel Regional Water Authority, Doetinchem, Netherlands
- 3Dutch Bryological and Lichenological Society, St. Anna Parochie, Netherlands
Whether fish mediate plant dispersal in temperate freshwaters is largely unknown. A prerequisite for successful dispersal is ingestion and surviving the journey in the intestinal tract. This study asks whether plant propagules are being ingested under field conditions and what factors shape dispersal potential, focusing on differences across plant species and propagule form (seed or fragment), seasonal differences and plant and fish traits that facilitate dispersal. We focused on three common fish species reported to differ in foraging strategy. Fish were caught monthly over a 1-year period in a Dutch lowland stream. Before they were returned to the stream, fish were kept in water for 26 h and their feces were collected, resulting in 150 fecal samples. Excreted animal remains and plant propagules were identified and enumerated. Plant propagules were tested for viability. In total, 88,579 vegetative fragments of vascular plants, 316 of mosses and 14 of charophytes were identified. Viability was low (<<1%) except for mosses (53.5%). Roach (Rutilis rutilis) and Rudd (Scardinius erythrophthalmus) displayed a preference for filamentous algae and certain plant species (i.e., Elodea nuttallii and Lemna sp.), likely because they were more palatable. Of the 1,787 generative propagules of vascular plants that were identified, 120 germinated (6.7%), representing 15 species. Betula pendula, Juncus effusus, and Poa trivialis were most abundant. Tench (Tinca tinca) egested most seeds, despite being the least herbivorous species. Particularly, germination was high for seeds that were light (<1.07 mg) and that floated for a long time. Our results show that fish do ingest plant propagules under field conditions and that fish can contribute to vegetative dispersal of vascular plants and several aquatic and riparian moss species. Ingestion of propagules is affected by water temperature and season, their availability in the propagule bank, and their palatability. Both seed traits (related to buoyancy, size and hardness) and fish traits (related to size and identity) were important. Despite substantial dietary overlap, the three fish species displayed subtle differences in their diet, and together can act as vectors for the dispersal of a range of plant and moss species of freshwater systems.
Introduction
Streams are important vectors for plant propagules facilitating dispersal between habitats, communities, and populations (Honnay et al., 2010; Nilsson et al., 2010; Fraaije et al., 2017). Many plant species have evolved seeds or vegetative propagules that are well equipped to “go with the flow” and thus achieve long-distance dispersal via hydrochory (Boedeltje et al., 2003, 2004; Sarneel, 2012; Favre-Bac et al., 2017). However, hydrochory in flowing waters is only in one direction, and going against the flow requires different vectors such as ducks or fish (Wubs et al., 2016). Fish may be particularly important vectors for upstream dispersal (Horn et al., 2011).
Knowledge of ichthyochory, i.e., seed dispersal by fish, is largely derived from Neotropical studies (Kubitzki and Ziburski, 1994; Galetti et al., 2008; Anderson et al., 2011; Correa et al., 2015, 2016), but a few studies also addressed the role of fish-mediated plant dispersal in temperate freshwater systems (Chick et al., 2003; VonBank et al., 2018). Experiments under controlled conditions have shown that seeds of several plant species from temperate regions are capable of germination following ingestion and egestion by fish (Pollux et al., 2006a, 2007; Sumoski and Orth, 2012; Boedeltje et al., 2015, 2016). However, to ensure seed ingestion in these experiments, fish were offered pellets of fish food in which plant seeds were enclosed. It is therefore not clear whether native fish of temperate fresh waters actually ingest seeds under natural conditions (but see Chick et al., 2003), and which factors influence seed consumption. Horn et al. (2011) have suggested that temperate fish ingest seeds unintentionally when foraging in the sediment. Seed availability in the sediment seed bank might therefore be a determining factor for seed ingestion. In addition, the availability of macro-invertebrates as an alternative food source may also play a role (Garvey and Chipps, 2012). The availability and need for macro-invertebrates or plants as food sources may vary throughout the year, with the possible consequence that fish-mediated dispersal may be determined by seasonal diet shifts of the fish as well as dietary differences across fish species.
Plant propagule uptake, egestion and dispersal may also depend on plant traits such as seed size (Pollux et al., 2007), seed hardness and seed shape (Boedeltje et al., 2015). However, the abundance of plant species in the local vegetation might also be important, as was demonstrated for hydrochorous plant dispersal (Boedeltje et al., 2003, 2019).
This study addressed the potential for plant dispersal in a temperate lowland stream by three native fish species: Roach (Rutilis rutilis), Rudd (Scardinius erythrophthalmus) and Tench (Tinca tinca). Tench is reported to primarily feed on benthos (Perrow et al., 1996). Roach and Rudd additionally forage throughout the water column, with Rudd being best adapted to feeding on prey at the water surface (Kennedy and Fitzmaurice, 1974; García-Berthou and Moreno-Amich, 2000). Their feeding behavior may vary in relation to temperature and according to the seasonal and spatial availability of food (e.g., Brabrand, 1985; Jamet, 1994; Michel and Oberdorff, 1995; Guinan et al., 2015). Roach and especially Rudd are considered to be the most herbivorous native fish in the European region (Prejs, 1984; Dorenbosch and Bakker, 2012), but Tench may also occasionally consume vegetation (Michel and Oberdorff, 1995), making them all potential plant dispersers.
Over a 1-year period, in this study we quantified each month the amount and viability of generative and vegetative plant parts as well as macro-invertebrate remains in the feces of the three fish species in a vegetated lowland stream. We first related the diet of the three fish species to season and temperature and the abundance of plant species in the vegetation and in the propagule bank. We next addressed the question whether these factors, in addition to plant specific traits, affected the probability of being successfully dispersed (i.e., viably egested) by the fish.
Materials and Methods
Study Area
The study was conducted in the Groenlose Slinge (52°116′ N, 6°494′ E), a channelized lowland stream in the eastern part of the Netherlands (Figure S1). The stream is a tributary of the small river Berkel, which in turn flows into the river IJssel, one of the lower reaches of the river Rhine. Thanks to fish passages (Figure S1), fish can move freely from the IJssel to the upstream areas of the tributaries and vice versa. The Groenlose Slinge is c. 25 km long and its slope at the research stretch is ~0.4 m/km. Its width ranges from 1 m in the upper course up to 10 m downstream. At the research stretch, discharge varies from 16 m3.s−1 at yearly peak discharges to 0.8 m3.s−1 at base flow, with a corresponding flow velocity of ~0.04 m.s−1. Fish were caught in a downstream stretch of 1.9 km length. The east bank of this stretch is steep with a narrow zone of helophytes bordering the water, whereas the west bank consists of 3 m wide, shallow zone (Figure S1). This is overgrown with helophytes of which Glyceria maxima is the most abundant plant species (Table S4). The higher parts of the west bank are forested (Figure S1) with Alnus glutinosa and willows (Salix spec.) as the dominant species. The eutrophic water is densely vegetated with submerged vascular plants, filamentous algae, mosses, and charophytes. Duckweeds (Lemna spec.) and floating-leaved species such as Nuphar lutea frequently occur (Table S4). To ensure unimpeded water discharge, the floating, submerged, and emergent vegetation is cut in mid-June and September. The riparian helophyte vegetation is not mown.
Measuring Plant Abundance in the Vegetation
In July 2017, when vegetation was at its peak of development, the presence of vascular plant species, filamentous algae, and charophytes was investigated. The 1.9 km research stretch was divided into sections of 100 m, each comprising the aquatic and bank zone. An aquatic plot included the entire stream width; a bank plot covered the 1–4 m wide helophyte zone of the bank, assuming that diaspores of species from this area could potentially reach the water. In addition, riparian species outside this helophyte zone were included if their height was such that seeds were likely to be able to reach the water. Species were recorded within the plots whilst wading through the water. The abundance was estimated using a scale of nine classes: 1 = 1–4, 2 = 5–20, 3 = 20–100, 4 = >100 individual(s) or tiller(s) in a plot and covering <5%; 5 = covering 5–12.5%, 6 = 12.5–25%, 7 = 25–50%, 8 = 50–75%, 9 = 75–100%. As mosses occurred on solid substrates (mostly stones) only, moss diversity was recorded separately in July 2018. Moss abundance was established in 8 sampling plots of 0.25 m2 (0.5 × 0.5 m), from 20 cm below to 30 cm above mean water level using the scale mentioned above. Nomenclature of vascular plants and mosses is, respectively according to van der Meijden (1996) and Siebel and During (2006).
Sampling the Propagule Bank
Propagule bank samples were taken in March 2017, assuming that natural stratification of seeds had occurred during the previous winter. In eight 4 m2-plots, evenly distributed over the research stretch, a sample was taken from the surface sediment, comprising eight cores each, using a transparent PVC-tube (Ø 6 cm). Four cores were taken in the shallow bank zone and four in the bordering aquatic zone. Sampling was restricted to the upper layer (5 cm) of the sediment, assuming that only this (organic) material might be foraged by fish. The cores of a plot were pooled and mixed, transferred to the laboratory and processed immediately.
The samples were treated according to the seedling emergence technique of Boedeltje et al. (2002). First, they were sieved (mesh width 200 μm) to remove fine soil material and dead organic parts. Potentially viable vegetative parts however, were kept in the samples. The remaining seeds and vegetative fragments of each sample were spread out in a thin layer (<5 mm) in one or more trays filled with a mixture of equal parts of sterilized sand and potting soil and set to germinate in a greenhouse under submerged conditions (water level 2 cm above soil surface) for 2 weeks and next under waterlogged conditions (water level 4 cm below soil surface) for 10 weeks (Figure S3). Air temperature in the greenhouse was at least 22°C between 06:00 and 21:00 h and 15°C between 21:00 and 06:00 h. A photoperiod between 06:00 and 21:00 h was maintained throughout the germination period. Seedlings and regenerated vegetative propagules were identified, counted, and removed from the trays.
Sampling and Housing the Fish
Between August 2016 to July 2017, the three fish species were captured monthly using a control box “TENCH 20” electro-fishing unit (Fishtronics.nl), connected to a standard gasoline generating set (230 V), mounted on a drift boat (Figure S1). The box was adjusted exactly so that the direct current of 3,000 W at 10 A in the water was strong enough to bring about a forced swimming movement to the anode-net, and causing minimum cramping of the fish. While actively electrofishing, a close proximity to the riparian vegetation was maintained, enabling fishing in both the aquatic and riparian vegetation. Both sides of the stream were sampled. Sampling continued until the end of the research section or until the minimum number of each species (50 individuals monthly) was reached. All fish were quickly put in an aerated tank on board and transported to the field station within half an hour after being caught. The field station was located 15 m away from the stream (Figure S1). For capture and subsequent release of fish, ethical approval was not required as per the local legislation.
In the field station, fish were sorted by species and size (estimated in “small”: <15 cm and “large”: ≥15 cm) so that conspecifics with similar size ended up in the same aerated 100 L tank. The (visually) estimated mean size of fish in each tank was recorded to be used as a covariate in statistical analysis. We choose not to measure fish more precisely as this might have caused too much stress and physical damage. To prevent propagules from being inadvertedly introduced into the tanks where fish were kept for defecation, the fish were rinsed in a bucket of tap water before being introduced to the tank. In addition, these tanks contained stream water that was filtered over a 200-μm sieve to prevent potential input of plant propagules from the stream. To prevent fish from leaping out, tanks were covered with fine-meshed nets (Figure S2). For each species we used five tanks, each containing small or large fishes. The actual number of fish per tank depended on the catch success and varied from 1 to 20 individuals (mean 10, median 10; Table S3). Fishes were then left undisturbed for 26 h, at which time they were transferred to the stream and released. The temperature in the field station was approximately equal to the ambient temperature and varied from 5°C in winter to 24°C in summer. No mortality was observed in response to electrofishing and housing the fish in aerated tanks.
Over the research year, 150 samples were taken, in which 1,467 fish individuals were caught: 41 samples (with 330 individuals) of Roach, 50 (461 individuals) of Rudd, and 59 (676 individuals) of Tench (Table 1). Roach and Tench could be caught every month, Rudd in 11 months (no catches in May).
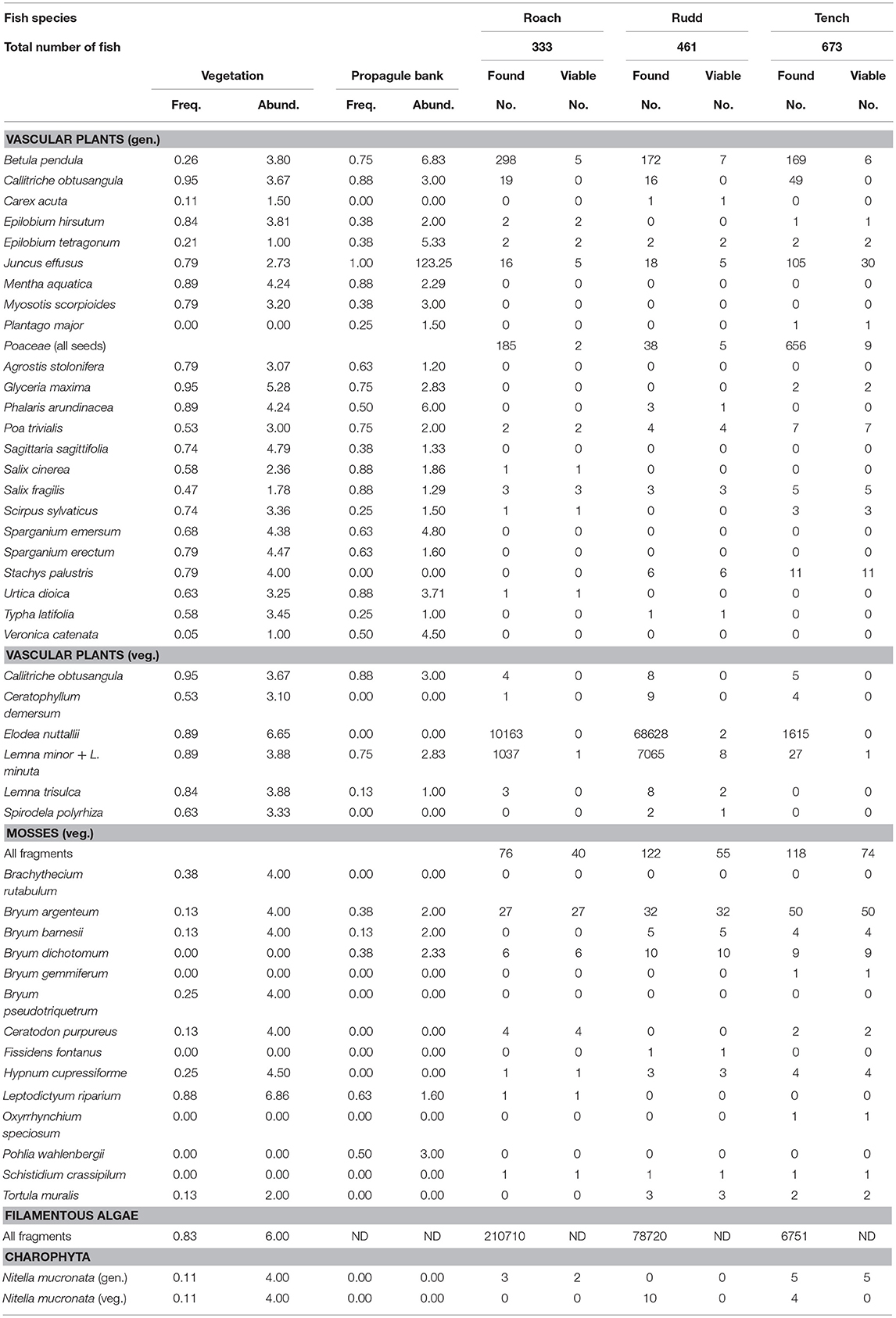
Table 1. Frequency and abundance of plant species in the vegetation and propagule bank, the number of generative (gen.) and vegetative (veg.) propagules found in the feces and the number of propagules found that proved to be viable (germinated or regenerated) for each of the three fish species.
Water temperature data were obtained from data collected at a gauge station, 500 m downstream from the study site. From these measurements, we selected the temperature data coinciding with the time we caught fish.
Sorting and Testing the Viability of Plant Parts
After the 26th hour of fish captivity, feces were collected by filtering the water of each tank over a 200-μm sieve (Figure S2), and next stored in tap water in 250 mL vials. By using a dissecting microscope, potentially viable seeds and vegetative plant parts were sorted out, identified and counted. The amount of vegetative filamentous algae and Characeae was determined by counting the number of fragments. When their quantities were large, the amount was estimated by counting fragments in 1/8 part of the microscope image field and then multiplying the number by 8. Since fragments differed in length, this is a proxy of their presence in the feces samples. Viability of algal fragments was not determined. Seeds were identified at least to the genus level using the Digital Seed Atlas of Cappers et al. (2006). Animal remains were transferred into alcohol (30%) and stored until identification. To test whether feces contained viable seeds and vegetative propagules, samples were next transferred to the greenhouse and handled according to the propagule bank protocol.
Seeds separated and identified were set to germinate in petri dishes (Ø 9 cm) on a double layer of Whatman No. 1 filter paper (Figure S2). The paper was kept water-saturated with tap water, as earlier experiments (Boedeltje et al., 2002, 2003) indicated that this was the optimal hydrological condition for both aquatic and riparian species. For 60 days, the dishes were placed in a climate-controlled room over 15-h light at 24°C and a night temperature of 15°C. Seedling emergence was assessed weekly.
After the germination and regeneration period, the non-germinated, but viable seeds in petri dishes and trays were placed water-saturated and waterlogged, respectively, in a dark room at 5°C for 10 weeks to promote loss of dormancy (Baskin and Baskin, 2014). After this cold treatment, petri dishes and trays were set to germinate again, as above.
Wet filter papers in petri dishes also were used as regeneration substrate for vegetative parts of bryophytes (Boedeltje et al., 2019; Figure S3). The closed dishes were exposed to natural day light conditions (without direct sunlight) in an unheated room (mean temperature 18°C) and watered with tap water when necessary. After 6 weeks, regeneration was recorded under a dissecting microscope and fragments were considered viable when they had produced rhizoids, shoots or green leaves (Figure S3). Hereafter, the term “viability” for a propagule is used for generative plant parts i.e., seeds or oospores that germinated or vegetative parts that grew into a new plant.
Aquatic Macroinvertebrate Identification
Remains of invertebrates were identified at least to the family level and, if possible, to the genus level using the literature cited in Bijkerk (2010). Hard chitinous remains allowed identification of trichopterans, chironomids, coleopterans, and heteropterans (e.g., elytra, hemielytra, abdominal claws, frotoclypeus, labium, and pronotum). Molluscs were identified from their shell remains. Taxa with less sclerotized body parts or with softer exoskeletons (e.g., Malacostraca and Ephemeroptera) could still be identified, but could not be accurately enumerated. Cladocerans were also counted, but not further identified.
Data Analyses
To visualize patterns of taxa (hereafter “species”) abundance (plants and invertebrates) in the feces samples across the 12 months surveyed, principal coordinate analysis (PCoA) was applied using Canoco 5 (ter Braak and Smilauer, 2012). We focused on assessing differences in diet, and therefore centered and standardized by plant and animal species represented in the diet. Abundance of plant parts and invertebrates was calculated by dividing the number of items per taxon over the number of fish per tank. Redundancy analysis (RDA) was applied to determine the significance of the variables fish species, fish size, number of fish in a sample, and month of feces collection in explaining differences in the abundance of plant and animal species in the samples. To rank the explanatory variables, forward selection of the variables was performed (ter Braak and Smilauer, 2012). To meet the assumptions of PCoA and RDA, species abundances were log10 - transformed. We also used PCoA to visualize differences in plant species abundance between the vegetation and the propagule bank. We also summed the total number of plant parts found in the feces of each fish species and included these as additional samples to illustrate how the vegetable diet of the fish compared to the plant species abundances of the vegetation and the propagule bank.
To relate abundance of plant propagules and faunal remains in each of the fish species to time of year and temperature, we summed the abundance for each of six food categories: (1) aquatic invertebrate remains; (2) Bryophyta; (3) Characeae; (4) filamentous algae; (5) seeds of vascular plants, and (6) vegetative parts of vascular plants. This resulted in 6 observations for each of the 150 samples. Next, we ran a linear model with log(x+1)-transformed abundance as the dependent variable, and fish species, the number of fish in the tank and fish size as the independent factors. As water temperature and month covaried, we ran two separate models including either a 2nd order polynomial for temperature or the 3rd order polynomial for month. Polynomials were used to account for non-linearity and models with polynomials had much improved model fits (lower AIC values) compared to linear models. Next, we also included food category and the interaction between fish species, food category, and either temperature or month. The model summaries for each of the two models are given in Tables S1,S2.
To test whether the three fish species foraged in different microhabitats, we tested for difference in the habitat preference of egested invertebrates. Substrate preference of the prey groups is derived from information in Verdonschot (1990) on habitat and mode of locomotion and checked with information in Verberk et al. (2012). We first summed the abundance of invertebrates for each of three habitat preference categories: (1) sediment; (2) open water, and (3) plants. For taxa which fell into two habitat preference categories, their abundance was equally divided among both categories. Next, we ran three separate linear models, one for each category, with log(x+1)-transformed abundance as the dependent variable and fish species as the independent variable. In these comparisons a significance level of 0.05/3 = 0.0167 was used to account for multiple comparisons.
To unravel the relative impact of plant traits, fish-related traits and abiotic factors on the egestion of viable seeds we used a General Linear Mixed Model (GLMM) approach. As response variable we used the presence or absence of a plant species' seed in the feces. For each sample, i.e., each unique combination of fish species, month of sampling and replicate, a plant species was considered present if one or more viable seeds of that species were found. Note that for most samples there was either no viable seed or only one seed of a particular plant species. Therefore, rather than analyzing abundance, a response variable in terms of presence/absence was deemed more appropriate and this was analyzed using GLMM with a binomial distribution. Initially we also tried to model number of viable seeds with either negative binomial or poisson distribution, including zero-inflated versions, but all these models failed to converge. Only plant species with at least one viable seed in either combination of fish species, month and replicate were included in this analysis. Thirteen plant species met this criterium.
The plant species-specific traits included were seed mass and floating capacity (buoyancy), obtained from the D3 (Hintze et al., 2013) and LEDA trait base (Kleyer et al., 2008). In addition to these functional traits, we included species abundance in the standing vegetation (mean value of the abundance measure in each trajectory) and abundance in the sediment propagule bank (mean number of propagules in the nine samples) as explanatory variables. Fish-related “traits” were number, estimated size and species-identity of the fish in each sample. Water temperature was included as environmental parameter. A check on multicollinearity by visual inspection of plots and calculated correlation coefficients (<0.5 considered as acceptable) indicated no objections against this selection of variables. Prior to analyses, mean abundance in the propagule bank was log (x+1)-transformed. All continuous explanatory variables were standardized to zero mean and unit variance prior to analysis.
In our GLMM, we followed the approach of Jamil et al. (2013). In this approach, significance of trait-environment relationships is addressed while simultaneously including plant species and samples as random factors to avoid the problem of pseudo replication and heteroscedastic variance. In model selection, we followed the tiered forward selection procedure as recommended by Jamil et al. (2013). We started with a simple model that only included a fixed intercept and random intercepts for species and sample (i.e., a unique combination of replicate, fish species and sampling date); we subsequently added a new term, as a main term and in interaction with fish species. Interaction of size-class and number of fish with fish species could not be included since these were non-orthogonal. Before a new term was added, we removed the non-significant terms, but kept a non-significant main term in the model if its interaction was significant. Significance of an additional term was assessed by a likelihood ratio test and difference in Akaikes Information Criterium. Models were run and compared with the package lme4 (Bates et al., 2015) in the open source statistical software R (R Development Core Team, 2017).
Results
Dietary Composition of Fish
Based on the fecal samples, fish consumed filamentous algae, vegetative parts of vascular plants, mosses and Characeae, generative parts of vascular plants and Characeae, and invertebrates (Figure 1, Table S3, Figure S2). Filamentous algae were found in large numbers. Fragments of vascular plants and mosses comprised 7 and 11 species, respectively; leaves and stems of Elodea nuttallii were most abundant (Figure S2). Seeds were found from 16 vascular plant species (Table 1). In total, 108 taxa of invertebrates were detected of which planktonic crustaceans (Daphnia and Cyclops species), larvae of midges (Chironomidae), and caddisflies (Trichoptera), amphipod crustaceans (Gammarus sp.) and Mollusca occurred in high numbers (Table S3).
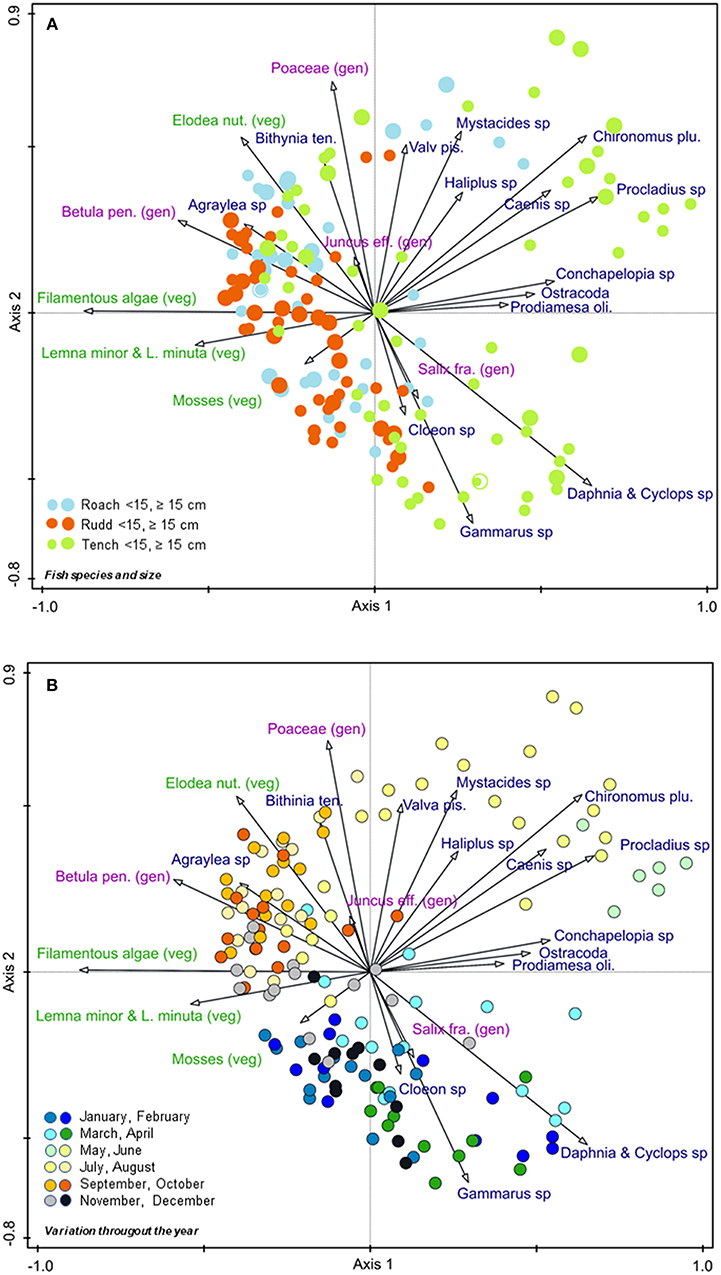
Figure 1. PCoA-diagrams for the first two axes, showing the relative positions of samples and taxa. In (A) samples are classified by fish species and fish size, in (B) by month. Eigenvalues and explained variation of axis 1 and 2 are 0.178 and 17.8%, and 0.155 and 15.5%, respectively. For full names of taxa, see Table S1. Gen, generative propagules (pink); veg, vegetative propagules (green), items in blue are invertebrates.
Redundancy analysis revealed that month (5%, F = 7.7, P = 0.008), fish species (4.5%, F = 7.1, P = 0.008), and fish size (1.4%, F = 2.9, P = 0.048) were the most significant variables to explain variation in dominance of egested species across our 150 fecal samples.
Vegetative plant parts were mainly eaten from July to October, seeds of grasses (Poaceae) in summer, larvae of Chironomidae in May and June, and Gammarus sp. and planktonic crustaceans in early spring (Figure 1B).
Numbers of egested items for the different food categories showed seasonal differences that were distinct for the three fish species (Figure 2A, Table S1). Invertebrates were egested most in spring and early summer, especially by Tench. In summer and autumn, vegetable food categories were eaten most, especially filamentous algae, but also plant seeds. Toward winter, consumption and egestion was generally lower. When plotting the same data against temperature (Figure 2B), fish generally started egesting more food items with increasing water temperature, although this varied both with fish species and species-group being consumed (Tables S1, S2). Rudd and Roach were clearly the two most herbivorous species, with Rudd becoming more herbivorous with increasing water temperature, egesting many vegetative parts of vascular plants. For Tench and Rudd, a higher consumption of invertebrates was accompanied by an increase of seed uptake, but no such relationship was apparent for Roach (Figure 2). Characeae appeared to be low in abundance, irrespective of water temperature or fish species, whereas Bryophyta were egested less with increasing water temperature.
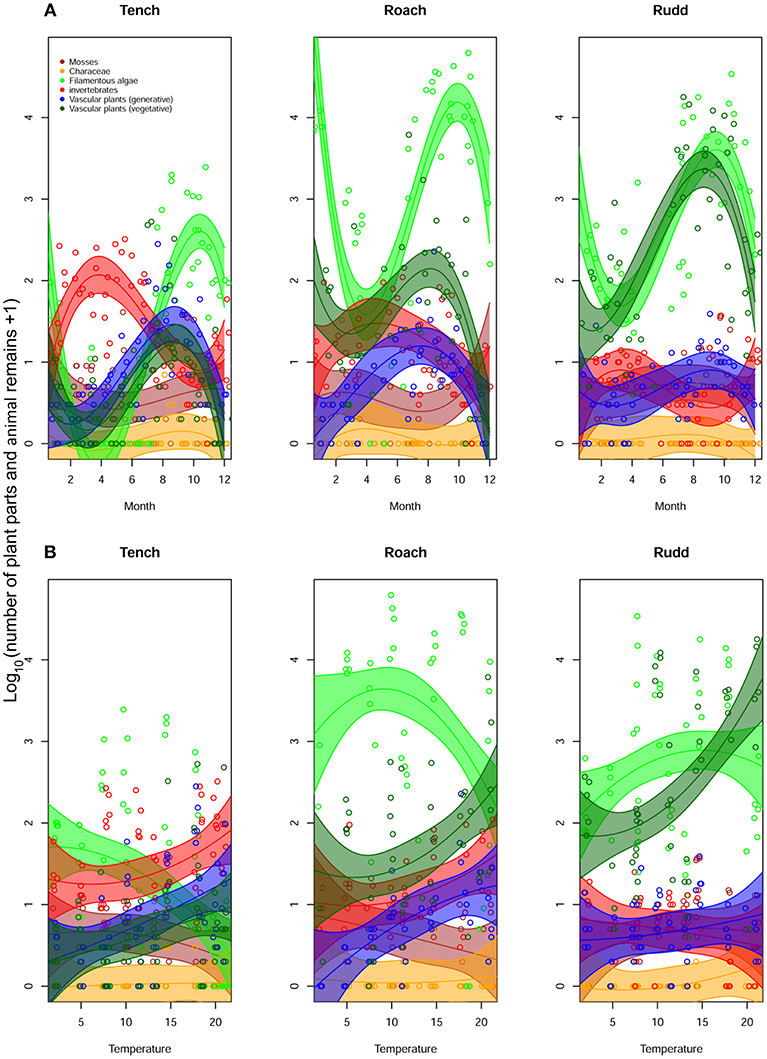
Figure 2. Variation in the number of plant parts and animal remains of taxonomic groups throughout the year (A), and in relation to water temperature (B) for each of the three fish species.
Relation Between Vegetable Items and Their Abundance in the Vegetation, Propagule Bank and Fish Feces
Plant species that were egested by fish, originated both from the standing vegetation and from the sediment propagule bank. The vegetation comprised 111 vascular plant species, 1 Charophyte and 12 mosses; the propagule bank comprised propagules from 37 vascular plants and 5 mosses (Table S4).
In total, 22 vascular plant species, 1 Charophyte and 12 mosses were retrieved and identified from the fish feces (Table 1, Table S3). All vascular plant species and the charophyte Nitella mucronata found were present in the vegetation, whereas four moss species (Bryum gemmiferum, Fissidens fontanus, Oxyrrhynchium speciosum, and Schistidium crassipilum) were found in the feces, but were not detected in the vegetation (Table 1).
Plant species composition and abundances differed between the vegetation and the propagule bank (Figure 3) and this difference was captured by the 2nd ordination axis. The three fish species were also differentiated along this 2nd ordination axis, and Tench showed the highest similarity between egested generative propagules (i.e., seeds of vascular plants) and species abundances in the benthic propagule bank, whereas Rudd showed the highest similarity between egested vegetative plant parts and species abundances in the vegetation (Table 3). The main difference (i.e., the 1st ordination axis) reflected that only a small subset of the plant, algae and moss species that were egested by fish had propagules present in feces in large numbers (Figure 3).
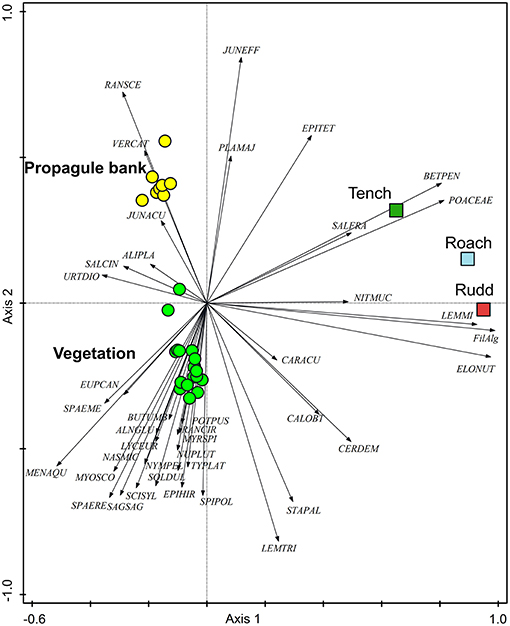
Figure 3. PCoA-diagram for the first two axes, showing the relative positions of vegetation (green) and propagule bank (yellow) samples, of the main plant species (arrows) and of the samples with total number of plant parts found in feces of each fish species (squares). Eigenvalues and explained variation of axis 1 and 2 are 0.357 and 35.7%, and 19.5 and 19.5%, respectively. Abbreviations of species names: first three letters of the genus name, followed by the first three letters of the species name (for full names see Table S2).
Leaves of E. nuttallii and threads of filamentous algae growing in the water column dominated the fecal samples, especially for Roach and Rudd (Figure 3). Other common species growing in the water column such as Callitriche obtusangula, Ceratophyllum demersum, and Sagittaria sagittifolia, were absent or occurred less frequently in the feces (Table 1, Figure 3). Free floating duckweeds (Lemna minor and L. minuta) were egested most by Rudd and Roach (Figure 3). Other common floating plants such as Lemna trisulca and Spirodela polyrhiza were found only infrequently (Table 1, Table S3).
In the sediment layer, the propagule bank is dominated by seeds of Juncus effusus, Betula pendula, several grasses (Phalaris arundinacea and G. maxima), Urtica dioica and Sparganium emersum. Seeds of Veronica catenata were also frequently present (Figure 3, Table S4, Figure S3). Reflecting their high abundance in the propagule bank, seeds of J. effusus, B. pendula, and grasses were most frequently retrieved from the feces of the fish (Table 1, Table S3). Significantly more seeds from J. effusus and grasses were retrieved from the feces of Tench than those of Rudd (Figure 3; ANOVA, P < 0.05).
Viable Plant Parts Found
In total, 88,579 vegetative fragments of vascular plants, 316 fragments of mosses and 14 fragments of Charophytes were identified of which <<1, 53.5, and 0% remained viable, respectively (Table 1). Viability of filamentous algae was not established. Thirteen vascular plant fragments, representing 4 species (E. nuttallii- Figure S5, Lemna minor, L. trisulca, S. polyrhiza) regenerated from the feces of Rudd, whereas in Roach and Tench only a single vegetative propagule appeared viable. For mosses, 52.6% fragments, representing 6 species, regenerated from feces of Roach, 45.1% fragments (7 species) from feces of Rudd, and 62.7% (9 species) from feces of Tench (Figure S3). Bryum argenteum was the most common moss species and constituted 85% of the viable moss fragments in fish feces (Table 1).
In total, 1,787 generative propagules of vascular plants were identified of which 6.7% germinated, representing 15 species (Table 1). For Roach, these numbers were 528 (identified), 4.2% (germinated), and 9 (species), respectively; for Rudd: 257 (identified), 11.7% (germinated) and 9 (species); for Tench: 1003 (identified), 6.8% (germinated), 10 (species). Seeds of J. effusus germinated in highest numbers. From the Charophyte N. mucronata viable oospores were found in feces of Roach and Rudd (Table 1). We detected seasonal patterns in egestion of grasses, J. effusus and B. pendula, but not of Bryophyta (Figure 4).
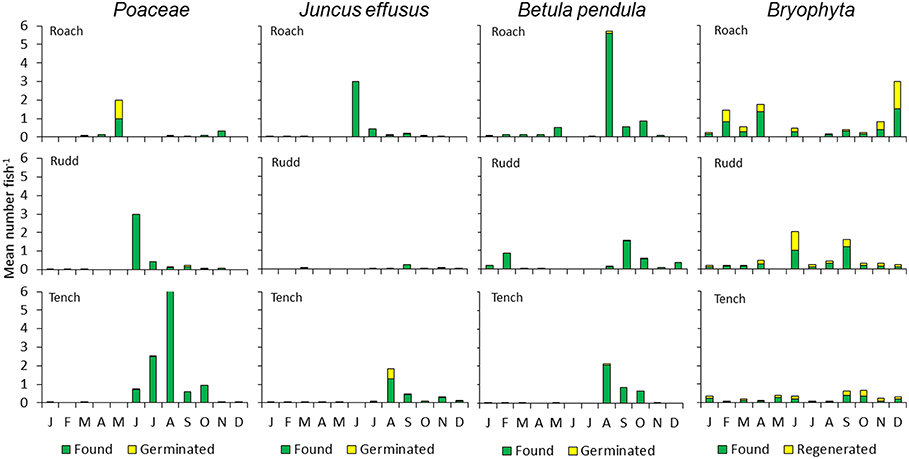
Figure 4. Monthly mean number of seeds and bryophyte fragments per fish for Roach, Rudd, and Tench. The proportions of germinated seeds or regenerated fragments are indicated for seeds or fragments of grasses (Poaceae), Juncus effusus, Betula pendula and Bryophyta. J–D (January–December, months during the research year).
Factors Related to Egestion of Viable Seeds
Plant species were more likely to be dispersed, or at least egested as viable seeds, if fish were bigger and water temperature was higher (Table 2). The temperature effect was similar for all three species, i.e., we found no significant fish-water temperature interaction. Plant specific factors that increased the probability of fish-mediated dispersal were abundance in the seed bank and buoyancy of seeds. The beneficial effect of seed buoyancy was fish species-dependent (Table 2). As indicated by the coefficients of the interaction terms, seeds that float for a longer time had a higher change of being dispersed in case of Rudd, but buoyancy seemed to have no such beneficial effect with either Roach or Tench as seed vectors. However, mean probability of being dispersed was rather low, with modeled estimates never exceeding a 10% probability (Table 2).
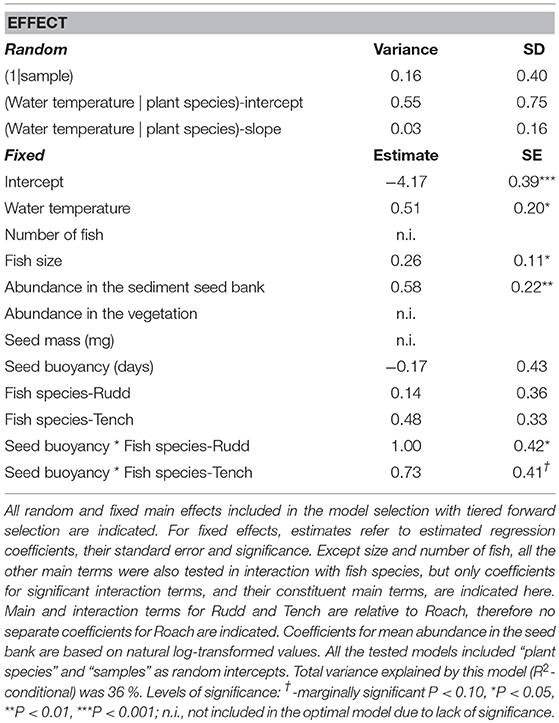
Table 2. Coefficients and their significance of the binomial GLMM-model best explaining the presence of plant species (as a viable seed) in feces of Rudd, Roach, and Tench.
Compared to the regional species pool, i.e., all the different plant species present in either the water or shoreline vegetation (Table S4), ichthyochory seemed to be prevalent for a subset only, comprising especially plant species with seeds that have both a relatively high buoyancy and a relatively small size (Figure 5). As indicated by high similarity in trait distribution between the benthic seed bank and fish feces (Figure 5), traits that facilitate incorporation in the seed bank may also facilitate dispersal by the fish.
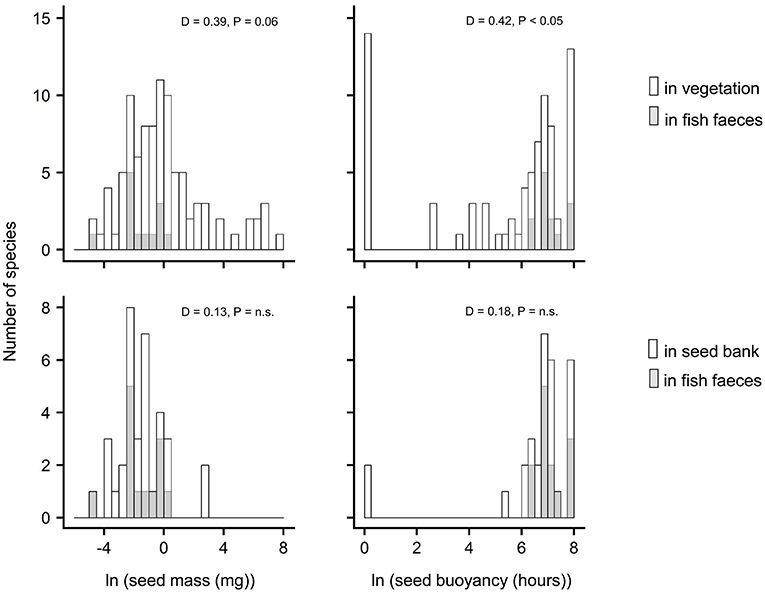
Figure 5. Frequency distribution of seed mass and seed buoyancy for number of vascular plant species in fish feces, vegetation, and the seed bank. Distributions were compared with Kolmogorov's two sample test; outcomes are indicated by the D-values and their significance in each panel. n.s. = not significant.
Discussion
Successful dispersal of plants requires that seeds are ingested and survive their journey in the intestinal tract before being egested. In our previous work (Boedeltje et al., 2015), we addressed how seeds differ in their ability to survive the process of ingestion, mastication and digestion, showing that survival depended both on traits of the plant seed (e.g., related to size and seed hardness) and traits of the fish (e.g., related to mechanical or chemical digestion). Since most studies employ pellets of fish food in which plant seeds were enclosed, an open question is whether fish actually ingest seeds under natural conditions in temperate waters. Here, we have demonstrated that a range of generative and vegetative propagules are being consumed under natural conditions by temperate freshwater fish (Table 1). The quantity and type of propagules consumed differs seasonally and may partly depend on their availability in the field and the temperature of the stream water. Moreover, there were differences across the three fish species with respect to the strata in which they foraged (water layer, sediment or vegetation) and with respect to their preference for either plants or invertebrates (Figures 1, 2). A caveat of the current method is that diet is inferred from egested plant and animal remains. The food items digested beyond recognition were, therefore, missed in our counts, especially in the case of prey without bones or chitin (Garvey and Chipps, 2012). While flushing of the stomach could have given a more complete view of what food items are being ingested by the fish, we opted for fecal collection as this provides unambiguous evidence for the ability of plant propagules to survive ingestion and digestion.
For Rudd and to a lesser extent for Roach and Tench, vegetative and generative plant parts were mainly retrieved from July to October, whereas in winter and spring the feces were dominated by animal remains. This indicates that fish shifted their feeding strategy from being mostly carnivorous to being mostly herbivorous in a seasonal basis, corroborating previous observations in both Roach (Brabrand, 1985) and Rudd (Guinan et al., 2015). Even Tench, the fish which was least herbivorous, consumed more food items of plant origin during the warm summer and autumn months. Increasing water temperature and more abundant growth of aquatic plants (Figure 2) are the likely mechanisms underlying this transition to herbivory (cf. Guinan et al., 2015). As Cyprinidae, including Rudd, Roach, and Tench, lack organs for the fermentation of cellulose by microorganisms, herbivory may only be energetically beneficial at elevated water temperatures when cellulolytic activity is higher in aquatic ecosystems (Niederholzer and Hofer, 1979; Behrens and Lafferty, 2007; Guinan et al., 2015). Plants also differ from each other in stoichiometry, having different carbon to nitrogen ratios. Stoichiometric considerations would predict that the higher energetic requirements in warmer water allows for use of food sources that are low in metabolizable nitrogen content relative to carbon-rich compounds like fat or carbohydrates which can be metabolized to generate energy (see Klaassen and Nolet, 2008). It would therefore be interesting to consider plant stoichiometry in future dispersal studies.
Our results indicate that all three fish species may contribute to plant dispersal, but may differ in their dispersal propensity across plant species. Differences in the consumption of either vegetative or generative propagules could be partly related to differences in foraging strategy. We could broadly confirm the different foraging strategies reported in the literature: Tench foraged most on benthos, consuming more animals that preferred the sediment (Figure 2, Figure S6) and showing a greater propensity to eat seeds present in the propagule bank (Figure 3, Tables 1, 3), although the propagule bank was sampled in March when Tench fed most on animal prey. Roach and Rudd foraged most in the water column, consuming mainly vegetative plant parts and Rudd appeared to forage more on the water surface, eating more floating seeds (Table 2) and free-floating duckweeds. Still, there appears to be substantial overlap in diet across fish species, especially when all seasons are considered, suggesting that they are rather opportunistic feeders and therefore all three of them can contribute to dispersal of a broad range of plant species.
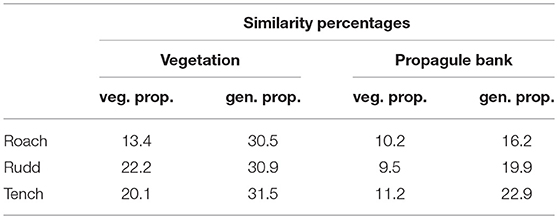
Table 3. Similarity percentages for egested vegetative and generative propagules and the species pool of the vegetation and propagule bank, respectively.
With regards to vegetative parts, E. nuttallii and filamentous algae were dominant in the feces of Roach and Rudd while other plant species such as C. demersum and L. trisulca were largely absent from their feces, despite being common in the vegetation. The absence of these latter plants from the feces could, in theory, be explained by being fully digested. However, it is more likely that certain plant species are more palatable and therefore preferentially consumed. As experiments with Rudd (Lake et al., 2002; Kapuscinski et al., 2014) and generalist snail herbivores (Grutters et al., 2017) have shown, the consumption rate of aquatic plants (e.g., Ceratophyllum demersum) with high chemical defense (e.g., phenolic content) and low nutritional quality is much lower than those of plant species with low defense and high nutritional quality (e.g., Nitella flexilis, Elodea canadensis, E. nuttallii). This implies that plant palatability increases the probability of being vegetatively dispersed, provided that propagules remain viable after gut passage. In our study, the survival of vegetative vascular plant parts after gut passage was extremely low (<<1%). We observed the regeneration (regrowth into viable parts) from two stem fragments of E. nuttallii (Figure S4), whereas regeneration from leaves of this species (which were by far the dominant organs in the samples) did not occur. Furthermore, only 11 duckweed plants with roots, stems and leaves, present in 5 samples with a total of 46 fish, were found to be viably egested. Although regeneration of egested vascular plant fragments was very low, certain palatable vascular plants were eaten frequently and could therefore be dispersed via ichthyochory.
In contrast to vascular plants, 53.5% of the vegetative bryophyte fragments regenerated. Nurminen et al. (2003) has previously shown bryophytes to be part of the diet of Rudd, but these authors did not establish viability of egested fragments. Our study thus presents the first evidence that ichthyochory may significantly contribute to vegetative dispersal of several aquatic and riparian moss species, confirming earlier assumptions by Glime (2017) and complementing dispersal by hydrochory (Boedeltje et al., 2019). Given the small size (0.2–0.4 mm) of egested moss fragments, and that they were found throughout the year, we hypothesize that bryophytes were ingested when fish were foraging in the soft sediment (including the propagule bank with moss fragments) or feeding on invertebrates living in moss cushions on submerged stones in the stream (cf. Glime, 2017).
Vegetative dispersal is also likely for filamentous algae. Although we did not test the viability of egested filamentous algae, it is likely that at least some algal threads remained viable. Filamentous algae retained their green color and relatively firm structure after gut passage, corroborating the assertion that they are not affected by mechanical or chemical processing in Cyprinidae (Sibbing, 1991). Vermeij et al. (2013) also demonstrated that red and green macroalgae remained viable after gut passage in herbivorous coral reef fishes.
Previous studies have highlighted bird mediated vegetative dispersal of aquatic ferns and vascular plants (Lovas-Kiss et al., 2018; Silva et al., 2018) and bryophytes (Wilkinson et al., 2017) complementing our findings that fish may also be vectors for vegetative dispersal of aquatic plant species. This suggests that endozoochory of plant fragments is a widespread but overlooked dispersal pathway.
With regard to generative propagules (i.e., seeds of vascular plants), we observed a clear peak in summer for egested seeds from grass species (mainly Poa trivialis and G. maxima). This peak can be related to the period of seed shedding and hydrochorous dispersal of P. trivialis and G. maxima (Boedeltje et al., 2004). Fish likely have actively foraged on the seeds of these grasses, as they are rich in nutrients (Hintze et al., 2013). Moreover, we found that buoyancy positively affected the probability of occurrence in the feces (Table 2), suggesting that fish may have preferentially foraged upon seeds floating at the water surface or in the water column, which seems most likely for Rudd. Tench likely consumed seeds of grasses and B. pendula after they were incorporated into the sediment propagule bank. This also matches with grass seeds being retrieved early in the season for Rudd, and later in the season for Tench (Figure 4).
In contrast to the active foraging on grass seeds, seeds of J. effusus where abundantly present in the sediment propagule bank and numerous small (0.5 mm) seeds of this species were retrieved from the feces of Tench throughout the year. The egested seeds germinated to a large extent, indicating that they were not crushed and digested. Cyprinid fish have pharyngeal teeth to crush and grind food but lack chemical digestion (Sibbing and Witte, 2005), making seed hardness an important trait to survive gut passage (Boedeltje et al., 2015). While seeds of grasses such as G. maxima are relatively soft and comparable with those of the soft-seeded Carex pseudocyperus (Boedeltje et al., 2015), they were likely crushed and digested, but the small and somewhat harder seeds of J. effusus could have slipped intact through the pharyngeal teeth. Germination percentages observed in this study for J. effusus were comparable to those observed after gut passage in common carp (Boedeltje et al., 2016).
The probability of finding a viable seed in the feces of fish was higher in large than in small fishes, indicating that the potential for effective seed dispersal might increase with body size. This may reflect ontogenetic differences in diet as small juvenile cyprinids feed mostly on invertebrates and switch to a more herbivorous diet as they grow (e.g., Nurminen et al., 2003). This result is in accordance with the findings of Galetti et al. (2008) and Costa-Pereira et al. (2017) for tropical fish. However, in temperate streams a larger dispersal potential of larger fish might apply only to small, relatively hard seeds. As bite force of Cyprinidae increases with age, dispersal of large, relatively soft seeds becomes increasingly unlikely in larger fish (Boedeltje et al., 2015).
Whether fish are effective vectors for long-distance plant dispersal depends also on the retention time in the digestive tract of the fish (Pollux et al., 2006a) and the distances it can travel during that time. Migration in fish may occur at a range of spatial and temporal scales, from diel migration among habitats to seasonal migrations on a landscape level (Lucas and Baras, 2001). All three species studied here have been reported to migrate seasonally between a tributary to the main river (Hohausová et al., 2003; Pollux et al., 2006b; Nunn et al., 2010). During winter, diurnal migration between a floodplain lake and a connected channel was observed for Roach (Heermann and Borcherding, 2006). Dispersal by fish may therefore enable upstream dispersal. This could explain why population genetic diversity, which is expected to increase downstream as a result of hydrochorous dispersal without upstream compensation, was not necessarily smaller upstream in a Belgium river (Honnay et al., 2010; Wubs et al., 2016). In addition, larger distances between stream networks may be mediated by secondary dispersal where fish and the plant seeds therein are consumed by piscivorous birds (van Leeuwen et al., 2017).
The first step to successful dispersal is ingestion by fish. Our results show ingestion is affected by water temperature and season, palatability of plant vegetative parts, fish species and fish size, and seed traits such as buoyancy, size, and seed hardness. Even the most carnivorous species included in our study (Tench) may contribute to plant dispersal as it forages preferentially on seeds in the sediment, and seeds are more likely than vegetative propagules to survive the journey in the intestinal tract of fish. Despite substantial dietary overlap, three different fish species can overlap in their roles as vectors for the dispersal of a range of moss species and vascular plants with palatable shoots and with small-sized, floating, hard seeds.
Ethics Statement
This study was carried out in accordance with the recommendations of the research organization Reptiles, Amphibians, and Fish Research of the Netherlands, RAVON, Nijmegen, The Netherlands.
Author Contributions
GB conceived and designed the research, collected and identified seeds and vegetative vascular plant parts, performed the germination and regeneration experiments, sampled the vegetation and propagule bank, and processed and partly analyzed and interpreted data. BK identified invertebrates and analyzed part of these data. MS and MdV collected fishes by electrofishing (Figure S1). PS identified the mosses. JL and WV analyzed and interpreted data. GB led writing the manuscript. BK, PS, JL, and WV contributed to drafts. All authors gave approval for submission.
Funding
The authors acknowledge the Prins Bernhard Cultuurfonds, section Meester Prikkebeenfonds, for supporting this research through a grant to GB.
Conflict of Interest Statement
The authors declare that the research was conducted in the absence of any commercial or financial relationships that could be construed as a potential conflict of interest.
Acknowledgments
We wish to thank Arthur de Bruin for his support during the initial phase of the research work and the tug of war club Heure for making a part of their clubhouse available as a field station for housing the fish. We thank André van Aalst for his expert help in housing the fish, Bastiaan van Zuidam for his help during sampling of the propagule bank, and the colleagues of the greenhouse of Radboud University for assistance. The suggestions for improvement, given by two reviewers and Andy Green, are highly appreciated.
Supplementary Material
The Supplementary Material for this article can be found online at: https://www.frontiersin.org/articles/10.3389/fevo.2019.00054/full#supplementary-material
References
Anderson, J. T., Nuttle, T., Saldaña Rojas, J. S., Pendergast, T. H., and Flecker, A. S. (2011). Extremely long-distance seed dispersal by an overfished Amazonian frugivore. Proc. R. Soc. Lond. B 278, 3329–3335. doi: 10.1098/rspb.2011.0155
Baskin, C. C., and Baskin, J. M. (2014). Seeds, Ecology, Biogeography, and Evolution of Dormancy and Germination. London: Academic Press.
Bates, D., Maechler, M., Bolker, B., and Walker, S. (2015). lme4: Linear Mixed-Effects Models Using Eigen and S4. R Package Version 1.1-10.Available online at: https://cran.r-project.org/package=lme4
Behrens, M. D., and Lafferty, K. D. (2007). Temperature and diet effects on omnivorous fish performance: implications for the latitudinal diversity gradient in herbivorous fishes. Can. J. Fish. Aquat. Sci. 64, 867–873. doi: 10.1139/f07-063
Bijkerk, R. (2010). Handboek Hydrobiologie. Biologisch Onderzoek voor de Ecologische Beoordeling van Nederlandse zoete en Brakke Oppervlaktewateren. Rapport 2010-28, Stichting Toegepast Onderzoek Waterbeheer, Amersfoort.
Boedeltje, G., Bakker, J. P., Bekker, R. M., van Groenendael, J. M., and Soesbergen, M. (2003). Plant dispersal in a lowland stream in relation to occurrence and three specific life-history traits of the species in the species pool. J. Ecol. 91, 855–866. doi: 10.1046/j.1365-2745.2003.00820.x
Boedeltje, G., Bakker, J. P., ten Brinke, A., van Groenendael, J. M., and Soesbergen, M. (2004). Dispersal phenology of hydrochorous plants in relation to discharge, seed release time and buoyancy of seeds: the flood pulse concept supported. J. Ecol. 92, 786–796. doi: 10.1111/j.0022-0477.2004.00906.x
Boedeltje, G., Jongejans, E., Spanings, T., and Verberk, W. C. E. P. (2016). Effect of gut passage in fish on the germination speed of aquatic and riparian plants. Aquat. Bot. 132, 12–16. doi: 10.1016/j.aquabot.2016.03.004
Boedeltje, G., Sollman, P., and Lenssen, J. P. M. (2019). Floating ability, shoot length and abundance facilitate hydrochorous dispersal of moss and liverwort fragments. J. Veg. Sci. 2019, 1–12. doi: 10.1111/jvs.12695
Boedeltje, G., Spanings, T., Flik, G., Pollux, B. J. A., Sibbing, F. A., and Verberk, W. C. E. P. (2015). Effects of seed traits on the potential for seed dispersal by fish with contrasting modes of feeding. Freshw. Biol. 60, 944–959. doi: 10.1111/fwb.12550
Boedeltje, G., ter Heerdt, N. J., and Bakker, J. P. (2002). Applying the seedling-emergence method under waterlogged conditions to detect the seed bank of aquatic plants in submerged sediments. Aquat. Bot. 72, 121–128. doi: 10.1016/S0304-3770(01)00224-8
Brabrand, Å. (1985). Food of Roach (Rutilus rutilus) and ide (Leusiscus idus): significance of diet shift for interspecific competition in omnivorous fishes. Oecologia 66, 461–467. doi: 10.1007/BF00379334
Cappers, R. T. J., Bekker, R. M., and Lans, J. E. A. (2006). Digitale Zadenatlas van Nederland. Digital Seed Atlas of the Netherlands. Groningen: Barkhuis Publishing and Groningen University Library.
Chick, J. H., Cosgriff, R. J., and Gittinger, L. S. (2003). Fish as potential dispersal agents for floodplain plants: first evidence in North America. Can. J. Fish Aquat. Sci. 60, 1437–1439. doi: 10.1139/f03-155
Correa, S. B., Arujo, J. K., Penha, J., Nunes da Cunha, C., Bobier, K. E., and Anderson, J. T. (2016). Stability and generalization in seed dispersal networks: a case study of frugivorous fish in Neotropical wetlands. Proc. R. Soc. B 283:20161267. doi: 10.1098/rspb.2016.1267
Correa, S. B., Costa-Pereira, R., Fleming, T., Goulding, M., and Anderson, J. T. (2015). Neotropical fish–fruit interactions: eco-evolutionary dynamics and conservation. Biol. Rev. 90, 1263–1278. doi: 10.1111/brv.12153
Costa-Pereira, R., Correa, S. B., and Galetti, M. (2017). Fishing-down within populations harms seed dispersal mutualism. Biotropica 50, 319–325. doi: 10.1111/btp.12516
Dorenbosch, M., and Bakker, E. S. (2012). Effects of contrasting omnivorous fish on submerged macrophyte biomass in temperate lakes. Freshw. Biol. 57, 1360–1372. doi: 10.1111/j.1365-2427.2012.02790.x
Favre-Bac, L., Lamberti-Raverot, B., Puijalon, S., Ernoult, A., Burel, F., Guillard, L., et al. (2017). Plant dispersal traits determine hydrochorous species tolerance to connectivity loss at the landscape scale. J. Veg. Sci. 28, 605–615. doi: 10.1111/jvs.12518
Fraaije, R. G. A., Moinier, S., van Gogh, I., Timmers, R., van Deelen, J. J., Verhoeven, J. T. A., et al. (2017). Spatial patterns of water-dispersed seed deposition along stream riparian gradients. PLoS ONE 12:e0185247. doi: 10.1371/journal.pone.0185247
Galetti, M., Donatti, C. I., Pizo, M. A., and Giacomini, H. C. (2008). Big fish are the best: Seed dispersal of Bactris glaucescens by the Pacu Fish (Piaractus mesopotamicus) in the Pantanal, Brazil. Biotropica 40, 386–389. doi: 10.1111/j.1744-7429.2007.00378.x
García-Berthou, E., and Moreno-Amich, R. (2000). Rudd (Scardinius erythrophthalmus) introduced to the Iberian Peninsula: feeding ecology in Lake Banyoles. Hydrobiologia 436, 159–164. doi: 10.1023/A:1026587721375
Garvey, J. E., and Chipps, S. R. (2012). “Diets and energy flow”, in Fisheries Techniques, eds A. V. Zale, D. L. Parrish, and T. M. Sutton (Bethesda, MD: American Fisheries Society), 733–779.
Glime, J. M. (2017). “Chapter 13: Fish,” in Bryophyte Ecology, Volume 2, Bryological Interaction (Ebook sponsored by Michigan Technological University and the International Association of Bryologists. Available online at: http://digitalcommons.mtu.edu/bryophyte-ecology2/ (Accessed October 2, 2018).
Grutters, B. M. C., Roijendijk, Y. O. A., Verberk, W. C. E. P., and Bakker, E. S. (2017). Plant traits and plant biogeography control the biotic resistance provided by generalist herbivores. Funct. Ecol. 31, 1184–1192. doi: 10.1111/1365-2435.12835
Guinan, M. E., Kapuscinski, K. L., and Teece, M. A. (2015). Seasonal diet shifts and trophic position of an invasive cyprinid, the Rudd Scardinius erythrophthalmus (Linnaeus, 1758), in the upper Niagara River. Aquat. Invasions 10, 217–225. doi: 10.3391/ai.2015.10.2.10
Heermann, L., and Borcherding, J. (2006). Winter short-distance migration of juvenile fish between two floodplain water bodies of the Lower River Rhine. Ecol. Freshw. Fish 15, 161–168. doi: 10.1111/j.1600-0633.2006.00132.x
Hintze, C., Heydel, F., Hoppe, C., Cunze, S., König, A., and Tackenberg, O. (2013). D3: the dispersal and diaspore database – Baseline data and statistics on seed dispersal. Perspect. Plant Ecol. Evol. Syst. 15, 180–192. doi: 10.1016/j.ppees.2013.02.001
Hohausová, E., Copp, G. H., and Jankovský, P. (2003). Movement of fish between a river and its backwater: diel activity and relation to environmental gradients. Ecol. Freshw. Fish 12, 107–117. doi: 10.1034/j.1600-0633.2003.00014.x
Honnay, O., Jacquemyn, H., Nackaerts, K., Breyne, P., and Van Looy, K. (2010). Patterns of population genetic diversity in riparian and aquatic plant species along rivers. J. Biogeogr. 37, 1730–1739. doi: 10.1111/j.1365-2699.2010.02331.x
Horn, M. H., Correa, S. B., Parolin, P., Pollux, B. J. A., Anderson, J. T., Lucas, C., et al. (2011). Seed dispersal by fishes in tropical and temperate fresh waters: the growing evidence. Acta Oecol. 37, 561–577. doi: 10.1016/j.actao.2011.06.004
Jamet, J.-L. (1994). Feeding activity of adult Roach (Rutilus rutilus (L.)), perch (Perca fluviatilis L.) and ruffe (Gymnocephalus cernuus (L.)) in eutrophic Lake Aydat (France). Aquat. Sci. 56, 376–387. doi: 10.1007/BF00877183
Jamil, T., Ozinga, W. A., Kleyer, M., and Ter Braak, C. J. F. (2013). Selecting traits that explain species-environment relationships: a generalized linear mixed model approach. J. Veg. Sci. 24, 988–1000. doi: 10.1111/j.1654-1103.2012.12036.x
Kapuscinski, K. L., Farrell, J. M., Stehman, S. V., Boyer, G. L., Fernando, D. D., Teece, M. A., et al. (2014). Selective herbivory by an invasive cyprinid, the Rudd Scardinius erythrophthalmus. Freshw. Biol. 59, 2315–2327. doi: 10.1111/fwb.12433
Kennedy, M., and Fitzmaurice, P. (1974). Biology of the Rudd Scardinius erythrophthalmus (L) in Irish Waters. Proc. R. Irish Acad. Sect. B 74, 245–303.
Klaassen, M., and Nolet, B. A. (2008). Stoichiometry of endothermy: shifting the quest from nitrogen to carbon. Ecol. Lett. 11, 785–792. doi: 10.1111/j.1461-0248.2008.01180.x
Kleyer, M., Bekker, R. M., Knevel, I. C., Bakker, J. P., Thompson, K., Sonnenschein, M., et al. (2008). The LEDA Traitbase: a database of life-history traits of the Northwest European flora. J. Ecol. 96, 1266–1274. doi: 10.1111/j.1365-2745.2008.01430.x
Kubitzki, K., and Ziburski, A. (1994). Seed dispersal in flood plain forests of Amazonia. Biotropica 26, 30–43. doi: 10.2307/2389108
Lake, M. D., Hicks, B. J., Wells, R. D. S., and Dugdale, T. M. (2002). Consumption of submerged aquatic macrophytes by rudd (Scardinius erythrophthalmus L.) in New Zealand. Hydrobiologia 470, 13–22. doi: 10.1023/A:1015689432289
Lovas-Kiss, A., Vizi, B., Vincze, O., Molnár, V. A., and Green, A. J. (2018). Endozoochory of aquatic ferns and angiosperms by mallards in Central Europe. J. Ecol. 106, 1714–1723. doi: 10.1111/1365-2745.12913
Lucas, M. C., and Baras, E. (2001). Migration of Freshwater Fishes. London: Blackwell Science. doi: 10.1002/9780470999653
Michel, P., and Oberdorff, T. (1995). Feeding habits of fourteen European freshwater fish species. Int. J. Ichthyol. 19, 5–46.
Niederholzer, R., and Hofer, R. (1979). The adaptation of digestive enzymes to temperature, season and diet in roach Rutilus rutilus L. and rudd Scardinius erythrophthalmus L. cellulase. J. Fish Biol. 15, 411–416. doi: 10.1111/j.1095-8649.1979.tb03624.x
Nilsson, C., Brown, R. L., Jansson, R., and Merritt, D. M. (2010). The role of hydrochory in structuring riparian and wetland vegetation. Biol. Rev. 85, 837–858. doi: 10.1111/j.1469-185X.2010.00129.x
Nunn, A. D., Copp, G. H., Vilizzi, L., and Carter, M. G. (2010). Seasonal and diel patterns in the migrations of fishes between a river and a floodplain tributary. Ecol. Freshw. Fish 19, 153–162. doi: 10.1111/j.1600-0633.2009.00399.x
Nurminen, L., Horppila, J., Lappalainen, J., and Malinen, T. (2003). Implications of rudd (Scardinius erythrophthalmus) herbivory on submerged macrophytes in a shallow eutrophic lake. Hydrobiologia 506, 511–518. doi: 10.1023/B:HYDR.0000008577.16934.a9
Perrow, M. R., Jowitt, A. J. D., and Johnsonf, S. R. (1996). Factors affecting the habitat of Tench in a shallow eutrophic lake. J. Fish Biol. 48, 859–870. doi: 10.1111/j.1095-8649.1996.tb01481.x
Pollux, B. J. A., De Jong, M. A., Steegh, A., Ouborg, N. J., van Groenendael, J. M., and Klaassen, M. (2006a). The effect of seed morphology on the potential dispersal of aquatic macrophytes by the common carp (Cyprinus carpio). Freshw. Biol. 51, 2063–2071. doi: 10.1111/j.1365-2427.2006.01637.x
Pollux, B. J. A., Korosi, A., Verberk, W. C. E. P., Pollux, P. M. J., and van der Velde, G. (2006b). Reproduction, growth, and migration of fishes in a regulated lowland tributary: potential recruitment to the river Meuse. Hydrobiologia 565, 105–120. doi: 10.1007/s10750-005-1908-4
Pollux, B. J. A., Ouborg, N. J., van Groenendael, J. M., and Klaassen, M. (2007). Consequences of intra-specific seed-size variation in Sparganium emersum for dispersal by fish. Funct. Ecol. 21, 1084–1091. doi: 10.1111/j.1365-2435.2007.01313.x
Prejs, A. (1984). Herbivory by freshwater fishes and its consequences. Environ. Biol. Fishes 10, 281–296. doi: 10.1007/BF00001481
R Development Core Team (2017). R: A Language and Environment for Statistical Computing. Vienna: R Foundation for Statistical Computing. Available online at: http://www.rproject.org/ (Accessed September 17, 2018).
Sarneel, J. (2012). The dispersal capacity of vegetative propagules of riparian fen species. Hydrobiologia 710, 219–225. doi: 10.1007/s10750-012-1022-3
Sibbing, F. A. (1991). “Food capture and oral processing,” in Cyprinid Fishes: Systematics, Biology and Expoitation, Fish and Fisheries Series 3, eds I. J. Winfield and J.S. Nelson (Dordrecht: Chapman and Hall; Springer), 377–408.
Sibbing, F. A., and Witte, F. (2005). “Adaptations to feeding in herbivorous fish (Cyprinidae and Cichlidae),” in Periphyton: Ecology, Exploitation and Management, eds M. E. Azim, M. C. J. Verdegem, A. A. van Dam, and M. C. M. Beveridge (Wallingford: CABI Publishing), 113–140.
Siebel, H., and During, H. (2006). Beknopte Mosflora van Nederland. Dutch Bryological and Lichenological Society; Royal Dutch Natural History Society, Utrecht.
Silva, G. G., Green, A. J., Weber, V., Hoffmann, P., Lovas-Kiss, A., Stenert, C., et al. (2018). Whole angiosperms Wolffia columbiana disperse by gut passage through wildfowl in South America. Biol. Lett. 14:20180703. doi: 10.1098/rsbl.2018.0703
Sumoski, S. E., and Orth, R. J. (2012). Biotic dispersal in eelgrass Zostera marina. Mar. Ecol. Prog. Ser. 471, 1–10. doi: 10.3354/meps10145
ter Braak and Smilauer (2012). Canoco 5, Software for Multivariate Data Exploration, Testing, and Summarization. Biometris, Plant Research International.
van Leeuwen, C. H. A., Lovas-Kiss, A., Ovegard, M., and Green, A.J (2017). Great cormorants reveal overlooked secondary dispersal of plants and invertebrates by piscivorous waterbirds. Biol. Lett. 13:20170406. doi: 10.1098/rsbl.2017.0406
Verberk, W. C. E. P., Verdonschot, P. F. M., van Haaren, T., and van Maanen, B. (2012). Milieu- en Habitatpreferenties van Nederlandse Zoetwater-Macrofauna. Eindhoven: Van de Garde-Jémé; WEW Themanummer.
Verdonschot, P. F. M. (1990). Ecological Characterization of Surface Waters in the Province of Overijssel, The Netherlands. Wageningen: Agricultural University.
Vermeij, M. J., van der Heijden, R. A., Olthuis, J. G., Marhaver, K. L., Smith, J. E., and Visser, P. M. (2013). Survival and dispersal of turf algae and macroalgae consumed by herbivorous coral reef fishes. Oecologia 171, 417–425. doi: 10.1007/s00442-012-2436-3
VonBank, J., DeBoer, J. A., Casper, A. F., and Hagy, H. M. (2018). Ichthyochory in a temperate river system by common carp (Cyprinus carpio). J. Freshw. Ecol. 33, 83–96. doi: 10.1080/02705060.2018.1423645
Wilkinson, D. M., Lovas-Kiss, A., Callaghan, D. A., and Green, A. J. (2017). Endozoochory of large bryophyte fragments by waterbirds. Crypt. Bryol. 38, 223–228. doi: 10.7872/cryb/v38.iss2.2017.223
Keywords: bryophyte, endozoochory, ichthyochory, seed dispersal, Rutilus rutilus, Scardinius erythrophthalmus, Tinca tinca, vegetative dispersal
Citation: Boedeltje G, Klutman B, Schaap M, Sollman P, de Vos M, Lenssen JPM and Verberk WCEP (2019) Plant Dispersal in a Temperate Stream by Fish Species With Contrasting Feeding Habits: The Role of Plant Traits, Fish Diet, Season, and Propagule Availability. Front. Ecol. Evol. 7:54. doi: 10.3389/fevo.2019.00054
Received: 30 October 2018; Accepted: 15 February 2019;
Published: 13 March 2019.
Edited by:
Andy J. Green, Estación Biológica de Doñana (EBD), SpainReviewed by:
Sandra Bibiana Correa, Mississippi State University, United StatesErik Kleyheeg, Max Planck Institute of Ornithology, Germany
Copyright © 2019 Boedeltje, Klutman, Schaap, Sollman, de Vos, Lenssen and Verberk. This is an open-access article distributed under the terms of the Creative Commons Attribution License (CC BY). The use, distribution or reproduction in other forums is permitted, provided the original author(s) and the copyright owner(s) are credited and that the original publication in this journal is cited, in accordance with accepted academic practice. No use, distribution or reproduction is permitted which does not comply with these terms.
*Correspondence: Ger Boedeltje, Zy5ib2VkZWx0amVAc2NpZW5jZS5ydS5ubA==
†Present Address: Michiel Schaap, Natuurmonumenten (Dutch Society for Nature Conservation), Vorden, Netherlands