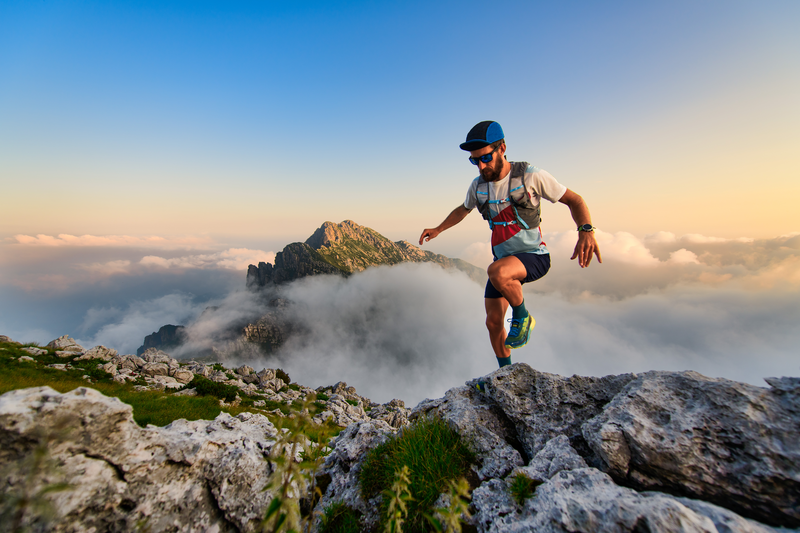
94% of researchers rate our articles as excellent or good
Learn more about the work of our research integrity team to safeguard the quality of each article we publish.
Find out more
ORIGINAL RESEARCH article
Front. Ecol. Evol. , 04 March 2019
Sec. Conservation and Restoration Ecology
Volume 7 - 2019 | https://doi.org/10.3389/fevo.2019.00037
This article is part of the Research Topic Synergistic Effects of Pervasive Stressors on Ecosystems and Biodiversity View all 7 articles
A major task for researchers in the twenty-first century is to predict how climate-mediated stressors such as wildfires may affect biodiversity under climate change. Previous model predictions typically did not address non-stationarity in climate-fire relationships across time and space. In this study, we applied spatially-explicit non-stationary area burned projection models to evaluate recent and future climate-driven trends in area burned across the ranges of three spotted owl subspecies in the western United States. We also used high-severity fire probability models to evaluate the risk of high-severity fire in recent times. Results suggest that the proportion of area burned will increase within the range of all three subspecies under climate change, but the extent of that increase will vary both among subspecies and among ecoregions within subspecies. Similarly, the current risk of high-severity wildfire varies both among subspecies and among regions within subspecies. The Mexican spotted owl is expected to have a 13-fold increase in area burned within its range by the 2080s. The combination of increased climate-driven fire extent and risk of high-severity fire suggests a potential for large-scale future loss or modification of spotted owl habitat. We recommend conducting further studies to understand the interaction and synergistic effects of climate change and wildfire on the spotted owl, especially in regions that are understudied such as Mexico.
Climate change is one of the most pressing threats to biodiversity in the twenty-first century (Thomas et al., 2004). Many species, especially those with low tolerance for rising temperature, directly suffer from global warming trends and are vulnerable to extinction. Climate change also affects species by altering ecosystem processes such as fire disturbance regimes (Flannigan et al., 2009). Together, climate change and wildfire can synergize to negatively affect biodiversity. In the western United States, the overall area burned as well as burn severity are predicted to increase under projected climate change (McKenzie et al., 2004; Littell et al., 2009, 2018; Westerling et al., 2011; Dennison et al., 2014; Abatzoglou and Williams, 2016), which may lead to an increasing rate of habitat loss and fragmentation across broad regions for many species. Understanding wildfire risks under current and future climate conditions is critical for effective management and conservation of species and habitats, especially for those species and habitats that are most sensitive to environmental changes.
One such species is the spotted owl (Strix occidentalis), which occurs in the western United States and Mexico (Gutiérrez et al., 1995). All three recognized subspecies of spotted owls (northern spotted owl [S. o. caurina], California spotted owl [S. o. occidentalis], and Mexican spotted owl [S. o. lucida]; Gutiérrez et al., 1995) have declined over the last century (Forsman et al., 1996, 2011; Seamans et al., 1999; Franklin et al., 2004; Anthony et al., 2006; Blakesley et al., 2010; Conner et al., 2013; Dugger et al., 2016). Conserving and managing the spotted owl is challenging as they are simultaneously threatened by multiple environmental stressors, such as climate change, high-severity wildfire, logging activities, and barred owl invasion (Wan et al., 2018a). In addition to the independent effect of each stressor, some stressors can interact to synergistically threaten the spotted owl. For example, because spotted owls are fairly heat intolerant (Ganey et al., 1993; Weathers et al., 2001), projected warmer climate alone can increase energetic requirements, and predicted warmer and drier climate conditions increased the modeled extinction rate for the Mexican spotted owl (Peery et al., 2012). But climate change is also linked with wildfire regimes, and this link may increase impacts of climate change. The spotted owl typically nests within mature, late-seral forests (Gutiérrez et al., 1995). Many of these forests, as well as the matrix of forests surrounding nesting habitat, have become increasingly vulnerable to wildfires, partly due to warmer and drier climate (Westerling et al., 2006; Miller et al., 2009; Westerling, 2016; Holden et al., 2018). Not all wildfires are detrimental to spotted owl habitat, but fire severity is also increasing within the range of the spotted owl (Miller et al., 2009), and high-severity fire may have negative impact on spotted owl habitat (Jones et al., 2016; Ganey et al., 2017). Therefore, climate change and wildfire can jointly accelerate the rate of loss and fragmentation of spotted owl habitat. Furthermore, post-fire salvage logging activities may occur in spotted owl habitat and can further degrade post-fire habitat quality (Hanson et al., 2018).
Each spotted owl subspecies has its own unique geographic range, apart from a small area of overlap between the ranges of the northern and California spotted owls (Barrowclough et al., 2011). Because of their geographic separation, each subspecies faces different combinations of stressors operating under different ecological conditions. Subsequently, the degree of influence from each stressor is likely to differ among subspecies due to regional variation in environmental conditions and differences in the ecology of the subspecies. In particular, regional differences in climate and fuel availability can influence interactions between changing climate and wildfire, leading to differential wildfire risks and impacts among spotted owl subspecies. These differences remain largely understudied (Wan et al., 2018a).
In this paper, we explore the vulnerability of landscapes within the ranges of the three spotted owl subspecies to interactions between climate change and wildfire risk, including area burned and high-severity fire. To accomplish this, we quantitatively compared fire risk among the ranges of the three subspecies by applying two sets of recent climate-fire models: (1) non-stationary area burned projection models (Littell et al., 2018) and (2) high-severity fire probability models (Parks et al., 2018). Both models were developed specifically for the western United States and considered spatially-explicit environmental factors such as fuel availability and climate. We hypothesized that habitats within the ranges of three owl subspecies have been and will be differentially affected by wildfire and climate change. Specifically, we hypothesized the following: (1) Percent area burned in recent history is greater within the range of the northern and the California spotted owls because while the Mexican spotted owl typically occurs in forested habitats, it also occurs in rocky canyonlands that have lower fuel loading and geological constraints on fire spread and in areas with lower human population density, which is a major ignition source of large fires. (2) Percent area burned will increase within the ranges of all three spotted owl subspecies under climate change, but the California and Mexican spotted owl ranges will experience greater increase because future conditions in the Southwest will be more susceptible to wildfires compared with the Northwest. (3) Risk of high-severity fire is currently higher within the range of the northern and the California spotted owls than within the range of the Mexican spotted owl for the same reason in our first hypothesis.
All three subspecies of spotted owls are most common in coniferous or conifer-hardwood forest types (Gutiérrez et al., 1995), but both the California and Mexican subspecies also occur in oak (Quercus spp.)-dominated forest and woodland types (Ganey et al., 1992; Gutiérrez et al., 1992) and the Mexican subspecies occurs in rocky canyonlands (Rinkevich and Gutiérrez, 1996; Willey and van Riper III, 2007; Bowden et al., 2015). Forest types occupied by spotted owls range from mesic forests which generally experienced high-severity fire at infrequent intervals to drier forest types that typically experienced frequent (generally 5–25 years), low-moderate intensity fires (Agee, 1993; Covington and Moore, 2004). Fire regimes thus vary widely both within and among subspecies, and wetter and drier forest types often intermingle in a complex fashion within areas occupied by owls.
Nesting habitat in most forest types occupied by spotted owls occurs primarily in older stands featuring large trees and characterized by moderate to high levels of canopy cover and uneven-aged multilayered stand structures (Forsman et al., 1984; Blakesley et al., 1992; Gutiérrez et al., 1992; Seamans and Gutiérrez, 1995; LaHaye et al., 1997; Hershey et al., 1998; May et al., 2004; Ganey et al., 2013). These areas often feature relatively continuous canopies and high fuel loads, making them susceptible to high-severity wildfires when climatic conditions favor such fire (Birch et al., 2015).
Littell et al. (2018) modeled recent and future area burned across the entire western United States. Their models accounted for the non-stationarity of climate-fire relationships and attributed variability in area burned to climatic drivers using Bailey's ecosection (Bailey, 1995) level time series fire and climate data. We used this model because it considers non-stationary climate-fire relationships and covers all spotted owl habitats in the western United States. We used area burned projections from their models to compare recent and expected future fire risks across the three spotted owl subspecies ranges. Recent annual area burned data were estimated based on annual area burned on federal lands from 1980 to 2006. For future projections, Littell et al. (2018) included 2 climatological periods, 2030–2059 (2040s) and 2070–2099 (2080s) by 5 different climate forcings (composite, ECHAM5, MIROC3.2, PCM1, and HadGEM1) based on different bracketing of general circulation models (GCMs) under the SRES A1B (balanced) emission scenario (Nakicenović et al., 2000). We applied their recent annual area burned results as well as projections of annual area burned for both future climatological periods but restricted our analysis to the composite climate forcing scenario for future projections. This scenario represented an ensemble of 10 GCMs (ECHAM5, BCCR, HadCM, MIROC3.2, HadGEM1, ECHO-G, PCM1, CNRM-CM3, CSIRO3.5, MIROC3.2.HI; Littell et al., 2011). Ecosections analyzed varied in size, with larger ecosections having larger potential burned area than smaller ecosections. Therefore, in order to compare area burned projections across all ecosections at a common scale, we calculated the percent area burned for each ecosection (i.e., the burned area as a proportion to the total ecosection area). We classified the percent area burned of each ecosection into seven groups: (1) <0.1%, (2) 0.1–0.5%, (3) 0.5–1%, (4) 1–3%, (5) 3–5%, (6) 5–10%, and (7)>10%. This classification was performed on the recent scenario and the 2040s and 2080s future scenarios. Then, we extracted the ecosection portion that intersected with each subspecies range using a spotted owl range polygon shapefile obtained from BirdLife International NatureServe (2015) and summarized the seven classified groups as percentages of the total area of each subspecies range. This allowed us to evaluate the fire risks that each spotted owl subspecies faced recently, and how those risks change in the future under climate change.
We used predictions from models developed by Parks et al. (2018) to evaluate high-severity fire risks within each spotted owl subspecies range. We used this model because it is the only spatially-explicit model that predicts high-severity fire probability across a large portion of spotted owl habitats in western United States. These models predict probability of high-severity fire across western United States forests as a function of four categories of environmental factors, including fuel load, topography, climate, and fire weather. It has a mean independent cross-validated AUC statistic of 0.72 (ranged 0.66–0.81 among ecoregions). Predictions were modeled for 19 terrestrial ecoregions (Olson and Dinerstein, 2002), including Okanagan, Columbia Plateau, East Cascades, West Cascades, Klamath, Sierra Nevada, California North Coast, California Central Coast, California South Coast, Canadian Rockies, Northern Great Plains, Middle Rockies, Utah-Wyoming Rockies, Great Basin, Southern Rockies, Utah High Plateaus, Colorado Plateau, Arizona-New Mexico Mountains, and Apache Highlands. The resulting predictions were compiled into high-resolution (30 × 30 m) georeferenced raster layers available for download at the Fire Research and Management Exchange System (FRAMES; www.frames.gov/NextGen-FireSeverity). However, raster layers in ecoregions with model performance of AUC <0.70 are unavailable for download, including Okanagan, Columbia Plateau, East Cascades, Klamath, Sierra Nevada, and Northern Great Plains ecoregions (Figure 1).
Figure 1. Availability of high-severity fire probability models by ecoregions (Parks et al., 2018) and the three spotted owl subspecies ranges (BirdLife International NatureServe, 2015).
To compare risks of high-severity fire among the ranges of the three spotted owl subspecies, we first downloaded and mosaiced all available raster layers produced by the high-severity fire probability model (Parks et al., 2018). This gave us a continuous surface showing the probability of high-severity fire in western United States forests across ecoregions described above. Then, we extracted from this high-severity fire probability surface the values of all pixels that intersected with each spotted owl subspecies range using the spotted owl range polygon shapefile described above. We performed a kernel density estimation of those extracted values. Pixels without data were excluded from the analysis. This analysis allowed us to visualize and quantitatively compare the distribution of high-severity fire probabilities within the range of each spotted owl subspecies, with the caveat that spatial inference for this analysis was limited to areas where models were available (Figure 1).
Overall, annual percent area burned during the recent period was <0.2% across all three spotted owl subspecies ranges and was projected to increase in the 2040s and 2080s (Figure 2). The Mexican spotted owl range was projected to have the greatest increase in annual percent area burned compared to the other subspecies ranges, reaching as high as 0.7 and 2.7% in the 2040s and 2080s, respectively. In the northern spotted owl range, the annual percent area burned was projected to increase to 0.4 and 2.1% in the 2040s and 2080s, respectively. The California spotted owl range was projected to have the least increase, having annual percent area burned of 0.2 and 0.5% in the 2040s and 2080s respectively (Figure 2).
Figure 2. Trends in total percent of the range of the spotted owl burned annually by subspecies and time period. NSO, Northern spotted owl; CSO, California spotted owl; MSO, Mexican spotted owl.
Spatially, most ecoregions that intersected with the spotted owl ranges were projected to increase in percent area burned, with the exception of the Sierra Nevada region, where annual percent area burned was projected to be between 0.1 and 0.5% during both recent times and the 2040s, and then decrease to <0.1% in 2080s (Figure 3). In the 2040s, the annual percent area burned was projected to be as high as 3–5% within some parts of the spotted owl range (e.g., southern Utah; Figure 3). By the 2080s, the projected annual percent area burned within spotted owl range could be >10% in some regions (e.g., eastern Cascades and southern Utah; Figure 3).
Figure 3. Increasing trends in percent area burned across the western United States by Bailey's ecosections (Bailey, 1995) based on predictions from non-stationary climate-fire models by Littell et al. (2018). Ranges of the northern spotted owl (NSO), California spotted owl (CSO), and Mexican spotted owl (MSO) are indicated by black, red, and blue polygons respectively. Area in gray indicates data were unavailable.
Among the three subspecies, the California spotted owl had the highest percent area burned during the recent period, with almost half of its range having 0.1–0.5% area burned annually (Figures 3, 4). The northern and Mexican spotted owls had a similar percent area burned within their ranges, with about 25% of their ranges recording 0.1–0.5% annual area burned. The rest of the ranges of all three subspecies had <0.1% annual area burned during the recent period. This trend was projected to reverse in the 2040s, when the Mexican spotted owl had the highest percent area burned within its range, and the California spotted owl had the lowest overall percent area burned within its range (Figure 2). In the 2040s, about 40% of the Mexican spotted owl range was projected to have >0.5% area burned, with some regions having up to 3–5% area burned annually (Figures 3, 4). Similarly, the northern spotted owl had about 40% of its range projected to have an annual rate of >0.5% area burned and up to 1–3% area burned within about one quarter of its range during the 2040s. In the California spotted owl range, the percent area burned in the 2040s was projected to remain similar to the recent trend. In the 2080s, the percent area burned was expected to further increase within most regions within the ranges of the northern and Mexican spotted owls, with about half of those regions having 1–3% area burned and some regions having >10% of their area burned annually (Figure 4). Within the range of the California spotted owl, some regions were projected to have a lower percent area burned (e.g., Sierra Nevada) while other regions would have an increased percent area burned of up to 3–5% annually (Figures 3, 4).
Figure 4. Recent and projected percent of range burned by spotted owl subspecies and by 7% area burned classes. NSO, Northern spotted owl; CSO, California spotted owl; MSO, Mexican spotted owl.
Probability of high-severity fire varied greatly across the three subspecies ranges (Figure 5). Within areas where models were available (Figure 1), the kernel density distribution of the northern spotted owl range skewed more to the right side of the plot than distributions for the other subspecies, suggesting a generally higher likelihood of high-severity fire within the range of the northern spotted owl (Figure 6). The distribution for the California spotted owl range was similar in shape to the distribution for the northern spotted owl range distribution, but with lower probability of high-severity fire (Figure 6). The distribution for the Mexican spotted owl range showed probability of high-severity fire <0.2 within much of the range and very few areas with a probability >0.4, suggesting lower high-severity fire risk within the range of the Mexican spotted owl compared to the other two subspecies ranges (Figure 6).
Figure 5. Probability of high-severity fire across the western United States forests predicted by the Parks et al. (2018) models, which modeled the effects of fuel, topography, climate, and fire weather on high-severity fire probability. Ranges of the northern spotted owl (NSO), California spotted owl (CSO), and Mexican spotted owl (MSO) are indicated by black, red, and blue polygons, respectively. Area in gray indicates data were unavailable either due to area being non-forest, low model performance, or no available model (see Figure 1).
Figure 6. Kernel density estimation of high-severity fire probability distribution within the ranges of three spotted owl subspecies. NSO, Northern spotted owl; CSO, California spotted owl; MSO, Mexican spotted owl.
Within the range of the northern spotted owl range, high-severity fire risks were highest in the West Cascades (also the highest risk across all three subspecies ranges) and lower along the northern California coast (Figure 5). Within the California spotted owl range, high-severity fire risks ranged from mild to high along the California south coast, with San Bernardino National Forest having the highest risk. Unfortunately, there was no data on risk of high-severity fire for the Klamath and Sierra Nevada ecoregions, where large populations of northern spotted owl and California spotted owl occur, respectively. Within the range of the Mexican spotted owl, probability of high-severity fire was highest in forests in Colorado and northern New Mexico. While high-severity fire risk was generally low in Utah and Arizona, there were pockets of habitats that had high probabilities of high-severity fire in central and southern Arizona (Figure 5).
This study used some of the most sophisticated fire and climate-fire models available to evaluate trends in area burned and current risk of high-severity fire within the range of the spotted owl. Results supported some, but not all, of our a priori hypotheses. Contrary to our first hypothesis, percent area burned was relatively similar across the ranges of the three spotted owl subspecies during the recent period, with the California spotted owl range having the highest and the northern spotted owl range the lowest percent area burned (Figures 3, 4). The spatial patterns in percent area burned among and within the ranges of the spotted owl may be largely due to regional differences in historical fire regimes, but may also be related to variation in fire suppression efforts and human ignitions, as well as the spatial variance of the distribution and density of human population. We correctly predicted that percent area burned would increase within all three subspecies' ranges over time under climate change. We also correctly predicted that this increase would be greatest within the range of the Mexican spotted owl. Percent area burned was projected to increase dramatically within the ranges of the Mexican spotted owl and the northern spotted owl, increasing by 13- and 10-fold, respectively, by 2080s (Figure 2). Contrary to our prediction, the increase within the California spotted owl range was the lowest among all, but the overall projected increase was still substantial and ~3 times larger than current levels (Figure 2). While it is possible that a 3-fold increase in area burned may be within the prehistoric (pre-1800) range of variability for the California spotted owl (Stephens et al., 2007), the projected 13- and 10-fold increases undoubtedly raise concerns for the other two spotted owl subspecies. To our surprise, projected percent area burned in the 2080s only increased from the 2040s in a small portion of the California spotted owl range, and either decreased or remained similar in most other parts of the range (Figures 3, 4). Nonetheless, the projected increase in the smaller part was so large that it outweighed the changes among other parts and led to the overall percent area burned being increased. The contrasting patterns among ecosections support the idea that wildfire and climate change effects on species habitats are non-stationary both spatially and temporally (McKenzie and Littell, 2017).
Historical fire regimes within the range of the spotted owl are variable and the subject of considerable debate. Most studies agree that drier forest types within the range of the spotted owl were historically characterized by a frequent, low-severity fire regime, with moderate- to high-severity fires common only at higher elevations and/or in wetter forest types that burned less frequently (Cooper, 1961; Agee, 1993; Weaver, 2014). Since European settlement, human activities such as timber harvesting, livestock grazing, urban development, and fire suppression and exclusion have altered the distribution and types of vegetation and fuels on the landscape, leading to substantial changes in forest structures (Skinner, 1995; Fulé et al., 1997; Hessburg and Agee, 2003). As a result, many areas within these drier forest types are now dominated by dense stands with connected canopies and understories of small trees, which are prone to stand-replacing wildfires (Fulé et al., 2004; Hessburg et al., 2005). In contrast, however, some authors argue that high-severity fire historically was more common than previously thought, and that fire risks have neither increased nor deviated from historical reference conditions (Hanson et al., 2009; Williams and Baker, 2012; Odion et al., 2014).
Regardless of these different viewpoints, fires have impacted all three spotted owl subspecies in recent years. Within the range of the northern spotted owl, Davis et al. (2015) estimated that 191,900 ha of nesting and roosting habitat on federal lands were lost to wildfires between 1994 and 2013. This area represented four times the amount of habitat considered lost from timber harvest (47,000 ha) and comprised 5.2% of the total habitat originally protected in 1994 (3,678,500 ha) under the Northwest Forest Plan (Davis et al., 2015). Ager et al. (2012) simulated fire behavior in the Deschutes National Forest of Oregon and predicted that 60 to 71% of burnable area in northern spotted owl habitats would experience active crown fire activity. Our results for area burned indicate that fire activity will increase in the future within the range of this subspecies.
In the Sierra Nevada, the U.S. Forest Service estimated that the area burned by wildfires has risen from 17,400 ha per year during the period from 1910 to 1980 to 25,500 ha per year during the period from 1995 to 2004 (U.S. Department of Agriculture, 2004). They also predicted that about 485,000 ha or ~10% of the 4.5 million ha of total forest area will be modified by wildfires over the next 20 years, affecting a large portion of California spotted owl habitat. Our results also suggest the overall increase in future area burned within the range of the California spotted owl (Figure 2), but the increase might eventually slow in the Sierra Nevada.
Wildfires also have severely impacted Mexican spotted owl habitat. Since the beginning of this millennium, over 527,000 ha in Arizona and New Mexico were burned by merely three fires, the 2002 Rodeo-Chediski Fire (189,700 ha), the 2011 Wallow Fire (217,700 ha), and the 2012 Whitewater-Baldy Complex Fire (120,500 ha). These (and other) fires collectively burned significant portions of occupied and potential Mexican spotted owl habitat. Our results suggest that area burned will increase within the range of this subspecies, driven by changing climate.
Across regions where models of high-severity fire risk were available, our results indicate that such risk is currently highest within areas where the northern spotted owl occurs, followed by the California spotted owl, and is lowest within areas where the Mexican spotted owl occurs (Figures 5, 6). However, the strength of inference here is limited because model results were not available for several important ecoregions covering major portions of the range of the spotted owl (Figure 1). Data on the probability of high-severity fire were not available for the Sierra Nevada region, but Stephens et al. (2016) estimated that 85,046 ha of potential spotted owl nesting habitat in this region was burned between 2000 and 2014 by wildfires that resulted in ≥50 % basal area mortality and reduced canopy cover to <25 %. They predicted (Stephens et al., 2016: Figure 5) that the cumulative amount of potential nesting habitat burned at ≥50 % tree basal area mortality would exceed the amount of existing nesting habitat within 75 years.
At least some areas within the ranges of all three subspecies showed high probability of high-severity fire. This may be a function of the types of forests typically used for nesting. All three subspecies tend to nest in forests with high canopy cover and fuel loads, and when these types of forests burn, they frequently burn at high severity (Reilly et al., 2017). The high risk of high-severity fire in such habitat when it burns, coupled with the trend toward increasing area burned, suggests that loss of nesting habitat to high-severity fire is likely to increase in the future. This is particularly evident in some ecoregions, such as the West Cascades and California South Coast regions (Figure 5).
In contrast, vulnerability of Mexican spotted owls to habitat loss due to high-severity wildfire may be mitigated in some regions by their ability to nest in topographically driven refuges in rocky canyons, which are less prone to wildfires.
The different fire regimes across the three spotted owl subspecies ranges may play a role in the adaption and response of each subspecies to climate-mediated wildfires. Responses of each subspecies to wildfires are, however, highly nuanced because the intensity, extent, and spatial pattern of any given burn greatly influence the subspecies responses. Pre- and post-fire weather may also play a role in determining how the spotted owl copes with fire.
Studies that examined the response of spotted owls to fire showed mixed results. For example, Bond et al. (2002) combined data from all three spotted owl subspecies and analyzed the short-term (1-year) post-fire effects on the survival, site fidelity, and reproductive success of spotted owls within four demography study areas. Minimum post-fire survival was similar to historic annual adult survival rates from these study areas, and 16 of 18 (89%) surviving owls remained in the same territory 1 year after fire, similar to historic site fidelity rates for these study areas. Reproductive rates were similar to or slightly higher than historic reproductive rates of owls in these study areas, suggesting that fire had little immediate or short-term impact on the three subspecies within these areas. However, the northern spotted owl data used by Bond et al. (2002) were from the southernmost end of the subspecies distribution. Further north, in southwest Oregon, the northern spotted owl responded more negatively to fire, showing decreased occupancy and survival and increased extinction probability 3–5 years after fire (Clark et al., 2011, 2013). This implies that the increasing future area burned may have some negative impacts on the northern spotted owl in at least some regions.
Some studies suggest that the California spotted owl might benefit from fires because it evolved in a fire-adapted forest ecosystem (Bond, 2016; Lee, 2018). For example, Bond et al. (2010) reported that 3 of 5 studied California spotted owl individuals used burned forests for roosting; and 29 of 45 (64%) and 13 of 43 (30%) roost sites were enclosed within burn perimeters during the breeding and non-breeding seasons, respectively. Bond et al. (2013) estimated the home range size of radio-marked California spotted owls 4 years after fire and found it to be similar to the home range size of California spotted owls in unburned forests in the Sierra Nevada. Bond et al. (2009) investigated burn severity effects on the California spotted owl's nesting, roosting, and foraging. The California spotted owl selected low-severity burned area for nesting and roosting and used higher severities and avoided unburned forests for foraging. In addition, occupancy rates of the California spotted owl were found to be relatively insensitive to fire or fire severity in some studies (Roberts et al., 2011; Lee et al., 2012; Lee and Bond, 2015a,b). Roberts et al. (2011) detected similar occupancy rate and density of the California spotted owl between recently mixed-severity burned (<15 years) and unburned forests in Yosemite National Park. Lee et al. (2012) also found no negative effect of fire or fire severities on the occupancy rate of the California spotted owl for up to 7 years after fire in the Sierra Nevada. Lee and Bond (2015a) studied the occupancy rate of the California spotted owl 1 year after the Rim Fire (104,000 ha), the largest wildfire in the Sierra Nevada mountain range, and found that site occupancy remained high even in areas burned by high-severity fires. Fire size and post-fire salvage logging play an important role in determining the effects of fire on the California spotted owl. Lee et al. (2013) and Lee and Bond (2015b) found little to no difference in the occupancy rate of the California spotted owl between burned and unburned sites in the San Bernardino and San Jacinto Mountains of southern California when the high-severity burned area within an owl territory is small (<50 ha), but extinction probability increased and colonization probability decreased as a function of the amount of severely burned areas when the high-severity burned area within a territory exceeds the 50 ha threshold, and post-fire salvage logging increases the extinction probability of the California spotted owl. In contrast to the above studies, two studies on the California spotted owl showed negative response to fire. Gutiérrez and Pritchard (1990) detected no California spotted owl within a recently burned area but reported a higher density of California spotted owl in habitats neighboring the burned area, suggesting that fire might have displaced the California spotted owl from the burned area into the unburned area. Jones et al. (2016) also demonstrated that a high-severity megafire (39,545 ha) increased the annual probability of owl site extirpation by 7-fold (12% pre-fire vs. 88% post-fire). In the year following this fire, their study population underwent the largest decline observed over 23 years of study. Increasing future area burned likely increases the exposure of the California spotted owl to megafires, and thus potential negative effects in some cases.
Relatively few studies have examined the relationship between the Mexican spotted owl and fire. Jenness et al. (2004) conducted owl surveys in 31 unburned and 33 burned sites (1–4 years after fire) within Mexican spotted owl territories in Arizona and New Mexico. They found no effect of fire or fire severity on the occupancy and reproduction of the Mexican spotted owl but noted that they had low power to detect such effects. Ganey et al. (2014) documented the use of burned areas by the Mexican spotted owl during winter, and prey abundance and biomass were higher in those burned wintering areas than in nest core areas during the same time period. Since food resources are scarce during winter, Mexican spotted owls might be selectively foraging in burned areas where prey abundance is greater. Based on these Mexican spotted owl studies and results from pooled data with the other two subspecies (Bond et al., 2002), impacts of fire on the Mexican spotted owl ranged from none to slightly positive.
Based on the above findings, the California spotted owl and the Mexican spotted owl appear to be more resilient to the projected increasing fire activity shown in this study, whereas the northern spotted owl might be more negatively affected. However, this should not be deemed conclusive because of the generally low statistical power due to small sample size in the above studies, as well as the low number of studies available for drawing conclusions. These studies all evaluated only short-term effects, and none evaluated the effects of cumulative habitat loss due to numerous large wildfires or wildfire trends under projected climate change (Ganey et al., 2017). Given cumulative loss of nesting habitat over time and the apparent increasing trends in area burned and fire severity, The Mexican Spotted Owl Recovery Plan (U.S. Fish and Wildlife Service, 2012) concluded that habitat loss to wildfire was the biggest threat facing Mexican spotted owls. The projected increase in annual area burned among many ecosections within the range of the Mexican spotted owl generally supports this conclusion.
There are still many knowledge gaps pertaining to fire effects on the spotted owl. For example, we have little information about fire effects on the subspecies in Mexico, within which a large portion of the subspecies' range lies. Further, a recent simulation study suggests that habitat fragmentation can potentially lead to reduced gene flow and genetic diversity of the spotted owl (Wan et al., 2018b), but empirical studies on this topic are lacking. Therefore, empirical genetic studies are critically needed to ascertain the effects of forest fragmentation by fire on the genetics of the spotted owl.
Wildfires and climate change can also indirectly affect the spotted owl by affecting its prey. Fires generally have positive effects on the abundance of prey species that prefer open-canopy, such as deer mouse (Peromyscus maniculatus) and woodrat (Neotoma spp.), which are key prey species for the California spotted owl and the Mexican spotted owl (Converse et al., 2006; Amacher et al., 2008; Roberts et al., 2015). A warming climate that opens forests may also create more habitats for these prey species (Hornsby and Matocq, 2012). Therefore, fires might be simultaneously reducing nesting habitat quality and creating higher quality foraging habitats for the spotted owl, especially for the California and the Mexican spotted owl subspecies.
In contrast, prey species that depend on interior forest, such as the northern flying squirrel (Glaucomys sabrinus), may be vulnerable to habitat loss from increasing fire severity and extent. A warming climate may lead to the reassembly of forest tree communities and reduce suitable habitats for such prey species (Weigl, 2007). Because the northern flying squirrel is a primary prey species for the northern spotted owl and makes up the majority (45–58.6%) of biomass consumed in many areas (Forsman et al., 1984, 2001, 2004; Hamer et al., 2001; Wiens et al., 2014), wildfire and climate change will likely have an impact on the northern spotted owl's diet (Meyer et al., 2007; Roberts et al., 2015). The northern flying squirrel is also part of the California spotted owl's diet, but its importance varies greatly, from only 3 to 39.3% of total biomass consumed depending on the region (Thrailkill and Bias, 1989; Smith et al., 1999). Therefore, the California spotted owl may also be affected, but perhaps not as severely as the northern spotted owl. The northern flying squirrel does not occur within the range of the Mexican spotted owl. It is also possible that post-fire increases in the abundance of prey species that prefer open-canopy habitats (e.g., deer mouse and woodrat) may compensate for the reduction in flying squirrels' availability, although there is little study on this topic (but see Roberts et al., 2015). Increases in fire extent will likely magnify any potential impacts described above, but how long the effects from fire on small mammal abundance persist is unknown.
Although this study uses some of the most sophisticated climate-fire models for understanding recent and future wildfire risks faced by the spotted owl, there are several important limitations. First, there are large and important regions within the range of each spotted owl subspecies where model results were unavailable. In particular, we do not have data for any of our models in Canada and Mexico. Although the spotted owl is virtually extinct in Canada, and the abundance of Mexican spotted owls in Mexico is unknown, much of the range of this subspecies occurs in Mexico, and trends in fire extent within that range remain unknown. We also do not have results from the high-severity fire models for the East Cascades within the range of the northern spotted owl, the Sierra Nevada within the range of the California spotted owl, or Mexico within the range of the Mexican spotted owl. These regions combined have large populations of spotted owl. Thus, the spatial inference of our results is restricted to those regions where data were available, and patterns elsewhere—including large portions of the range of the spotted owl—remain uncertain.
Second, the projected future climate-fire relationships in this study were based on fire regimes under recent climate and human influence. In the future, humans will probably play a bigger role in influencing the spatial variability of wildfire severity and extent as human populations continue to increase in many rural parts of the western United States. Specifically, fire ignitions by humans will likely increase with increasing human populations. There is uncertainty as to how fire suppression and fuel reduction treatment efforts may change in the future, and how effective these efforts may be in controlling wildfire severity and extent in the face of climate change. Land use change will also contribute to fire behavior changes. Projections in this study do not reflect these potential changes in human influences on wildfire patterns.
Third, because Littell et al. (2018) used a fire-rotation constraint to minimize overprojection of area burned due to static vegetation assumptions, their models represent conservative projections of future area burned. High-severity stand replacing fire is already decreasing forested area in the western United States. With warmer and drier conditions, this trend is expected to continue and will increase fire severity and the likelihood of replacement of forest with open vegetation. Therefore, the expected increase in area burned will likely be realized sooner than projected by the models. To more accurately predict fire response to climate change will require simulation of future vegetation trajectories, which was beyond the scope of this study.
Finally, the high-severity fire probability model developed by Parks et al. (2018) has a fair amount of model uncertainty in a few ecoregions where AUC scores were ~0.70, including California North Coast (AUC = 0.70), California South Coast (AUC = 0.72), West Cascades (AUC = 0.71), and Southern Rockies (AUC = 0.72), warranting further study to ascertain the trend and effects of high-severity fire among these regions.
A major task for researchers in the twenty-first century is to predict how climate-mediated stressors such as wildfires may affect biodiversity under climate change. Many model predictions on this topic were based on historical references, but a major weakness of this approach is that climate-fire relationships are unlikely to remain stationary (McKenzie and Littell, 2017; Turco et al., 2018). Our study assessed not only the recent history of wildfires within the range of the spotted owl, but also future trends in wildfire extent using models that considered non-stationarity in climate-fire relationships (Littell et al., 2018). Based on our findings, the proportion of area burned can be expected to increase within the range of all three subspecies, and previous work suggests that fire severity also may increase (Miller et al., 2009). Thus, fire impacts will continue to increase for all three spotted owl subspecies in the future, but such impacts will not remain stationary and the extent of the increase may vary both among subspecies and among regions within subspecies. We recommend more studies to be conducted to understand climate change and wildfire effects on the spotted owl across its range, especially in regions that are understudied. Particularly, most spotted owl studies have been conducted in the United States, and there is little information about the spotted owl and its relationship with climate change and fire in Mexico (Wan et al., 2018a).
Wildfire is a complex phenomenon, with the intensity, extent, and spatial pattern of any given fire interacting to create diverse post-fire landscapes. Landscape structure and composition, which differs widely among post-fire landscapes, influences how resident species respond to post-fire landscapes. Consequently, understanding how wildfire affects ecological relationships of species inhabiting these post-fire landscapes is difficult. The spotted owl is no exception here. Effects of wildfires on spotted owls are currently poorly understood and based primarily on short-term studies (Bond et al., 2002, 2009; Irwin et al., 2015; Lee and Bond, 2015a,b; Jones et al., 2016) that yielded conflicting results (Ganey et al., 2017). Long-term studies will be needed to understand the impact of predicted increasing fire activity on the spotted owl and its habitat. This range-wide study provides a synopsis of the variation in wildfires among ecoregions, but variation also exists within each ecoregion. Therefore, we recommend future studies to use a finer scale approach to ascertain fire and owl relationships, particularly across habitat types. Finally, because environmental stressors such as wildfires, climate change, and barred owl invasion can affect each other, future studies should focus on the interactive effects of these stressors on each subspecies (Wan et al., 2018a).
HW and SC conceptualized the study. HW carried out the analyses. HW and JG wrote the manuscript.
The authors declare that the research was conducted in the absence of any commercial or financial relationships that could be construed as a potential conflict of interest.
We thank Dr. Brenda C. McComb for inviting us to contribute our article to this special issue.
Abatzoglou, J. T., and Williams, A. P. (2016). Impact of anthropogenic climate change on wildfire across western US forests. Proc. Natl. Acad. Sci. U. S. A. 113, 11770–11775. doi: 10.1073/pnas.1607171113
Ager, A. A., Vaillant, N. M., Finney, M. A., and Preisler, H. K. (2012). Analyzing wildfire exposure and source-sink relationships on a fire prone forest landscape. For. Ecol. Manage. 267, 271–283. doi: 10.1016/j.foreco.2011.11.021
Amacher, A. J., Barrett, R. H., Moghaddas, J. J., and Stephens, S. L. (2008). Preliminary effects of fire and mechanical fuel treatments on the abundance of small mammals in the mixed-conifer forest of the Sierra Nevada. For. Ecol. Manage. 255, 3193–3202. doi: 10.1016/j.foreco.2007.10.059
Anthony, R. G., Forsman, E. D., Franklin, A. B., Anderson, D. R., Burnham, K. P., White, G. C., et al. (2006). Status and trends in demography of northern spotted owls, 1985-2003. Wildl. Monogr. 163, 1–48. doi: 10.2193/0084-0173(2006)163[1:SATIDO]2.0.CO;2
Bailey, R. G. (1995). Description of the Ecoregions of the United States (2nd ed.). Misc. Pub. No. 1391, Map scale 1:7,500,000. (Ogden, UT: USDA Forest Service).
Barrowclough, G. F., Gutiérrez, R. J., Groth, J. G., Lai, J. E., and Rock, D. F. (2011). The hybrid zone between northern and California spotted owls in the Cascade–Sierran suture zone. Condor 113, 581–589. doi: 10.1525/cond.2011.100203
Birch, D. S., Morgan, P., Kolden, C. A., Abatzoglou, J. Y., Dillon, G. K., Hudak, A. T., et al. (2015). Vegetation, topography and daily weather influenced burn severity in central Idaho and western Montana forests. Ecosphere 6:17. doi: 10.1890/ES14-00213.1
BirdLife International and NatureServe (2015). Bird Species Distribution Maps of the World. (Cambridge: BirdLife International; Arlington, VA: NatureServe).
Blakesley, J.A., Franklin, A.B., and Gutiérrez, R.J. (1992). Spotted owl roost and nest site selection in northwestern California. J. Wildl. Manag. 56, 388–392. doi: 10.2307/3808840
Blakesley, J. A., Seamans, M. E., Connor, M. M., Franklin, A. B., White, G. C., Gutiérrez, R. J., et al. (2010). Population dynamics of spotted owls in the Sierra Nevada, California. Wildl. Monogr. 174, 1–36. doi: 10.2193/2008-475
Bond, M. L. (2016). The heat is on: spotted owls and wildfire. Ref. Module Earth Syst. Environ. Sci. doi: 10.1016/B978-0-12-409548-9.10014-4
Bond, M. L., Gutiérrez, R. J., Franklin, A. B., LaHaye, W. S., May, C. A., and Seamans, M. E. (2002). Short-term effects of wildfires on spotted owl survival, site fidelity, mate fidelity, and reproductive success. Wildl. Soc. Bull. 30, 1022–1028.
Bond, M. L., Lee, D. E., and Siegel, R. B. (2010). Winter movements by California spotted owls in a burned landscape. West. Birds 41, 174–180.
Bond, M. L., Lee, D. E., Siegel, R. B., and Tingley, M. W. (2013). Diet and home-range size of California spotted owls in a burned forest. West. Birds 44, 114–126.
Bond, M. L., Lee, D. E., Siegel, R. B., and Ward, Jr, J. P. (2009). Habitat use and selection by California spotted owls in a postfire landscape. J. Wildl. Manag. 73, 1116–1124. doi: 10.2193/2008-248
Bowden, T. S., Ferguson, J. M., Ward, R. V., Taper, M. L., and Willey, D. W. (2015). Breeding season home range and habitat use of Mexican spotted owls (Strix occidentalis lucida) below the south rim of grand canyon national park. Wilson J. Ornithol. 127, 678–689. doi: 10.1676/15-004.1
Clark, D.A., Anthony, R.G., and Andrews, L.S. (2011). Survival rates of Northern spotted owls in post-fire landscapes of Southwest Oregon. J. Raptor Res. 45, 38–47. doi: 10.3356/JRR-10-42.1
Clark, D.A., Anthony, R. G., and Andrews, L. S. (2013). Relationship between wildfire, salvage logging, and occupancy of nesting territories by Northern spotted owls. J. Wildl. Manag. 77, 672–688. doi: 10.1002/jwmg.523
Conner, M. M., Keane, J. J., Gallagher, C. V., Jehle, G. J., Munton, T. E., Shaklee, P. A., et al. (2013). Realized population change for long-term monitoring: California spotted owl case study. J. Wildl. Manag. 77, 1449–1458. doi: 10.1002/jwmg.591
Converse, S. J., Block, W. M., and White, G. C. (2006). Small mammal population and habitat responses to forest thinning and prescribed fire. For. Ecol. Manage. 228, 263–273. doi: 10.1016/j.foreco.2006.03.006
Covington, W. W., and Moore, M. M. (2004). Postsettlement changes in natural fire regimes and forest structure: ecological restoration of old-growth ponderosa pine forests. J. Sustain. For. 2, 153–181. doi: 10.1300/J091v02n01_07
Davis, R.J., Hollen, B., Hobson, J., Gower, J. E., and Keenum, D. (2015). Northwest Forest Plan—the First 20 years (1994–2013): Status and Trends of Northern Spotted Owl Habitats. Portland, OR: USDA Forest Service.
Dennison, P. E., Brewer, S. C., Arnold, J. D., and Moritz, M. A. (2014). Large wildfire trends in the western United States, 1984–2011. Geophys. Res. Lett. 41, 2928–2933. doi: 10.1002/2014GL059576
Dugger, K. M., Forsman, E. D., Franklin, A. B., Davis, R. J., White, G. C., Schwarz, C. J., et al. (2016). The effects of habitat, climate, and barred owls on long-term demography of northern spotted owls. Condor 118, 57–116. doi: 10.1650/CONDOR-15-24.1
Flannigan, M. D., Krawchuk, M. A., de Groot, W. J., Wotton, B. M., and Gowman, L. M. (2009). Implications of changing climate for global wildland fire. Int. J. Wildl. Fire 18, 483–507. doi: 10.1071/WF08187
Forsman, E. D., Anthony, R. G., Dugger, K. M., Glenn, E. M., Franklin, A. B., White, G. C., et al. (2011). “Population demography of northern spotted owls: 1985–2008,” in Studies in Avian Biology, (Berkeley: University of California Press), 2–3.
Forsman, E. D., Anthony, R. G., Meslow, E. C., and Zabel, C. J. (2004). Diets and foraging behavior of northern spotted owls in Oregon. J. Raptor Res. 38, 214–230.
Forsman, E. D., DeStefano, S., Raphael, M. G., and Gutiérrez, R. J. (1996). Demography of the northern spotted owl. Stud. Avian Biol. 17, 1–122.
Forsman, E. D., Meslow, E. C., and Wight, H. M. (1984). Distribution and biology of the spotted owl in Oregon. Wildl. Monogr. 87, 3–64.
Forsman, E. D., Otto, I. A., Sovern, S. G., Taylor, M., Hays, D. W., Allen, H., et al. (2001). Spatial and temporal variation in diets of spotted owls in Washington. J. Raptor Res. 35, 141–150.
Franklin, A. B., Gutiérrez, R. J., Nichols, J. D., Seamans, M. E., White, G. C., Zimmerman, G. S., et al. (2004). Population dynamics of the California spotted owl (Strix occidentalis occidentalis): a meta-analysis. Ornithol. Monogr. 54, 1–54. doi: 10.2307/40166799
Fulé, P. Z., Covington, W. W., and Moore, M. M. (1997). Determining reference conditions for ecosystem management of southwestern ponderosa pine forests. Ecol. Appl. 7, 895–908. doi: 10.1890/1051-0761(1997)007[0895:DRCFEM]2.0.CO;2
Fulé, P. Z., Crouse, J. E., Cocke, A. E., Moore, M. M., and Covington, W. W. (2004). Changes in canopy fuels and potential fire behavior 1880-2040: Grand Canyon, Arizona. Ecol. Modell. 175, 231–248. doi: 10.1016/j.ecolmodel.2003.10.023
Ganey, J. L., Apprill, D. L., Rawlinson, T. A., Kyle, S. C., Jonnes, R. S., Ward, Jr., et al. (2013). Nesting habitat of Mexican spotted owls in the Sacramento Mountains, New Mexico. J. Wildl. Manag. 77, 1426–1435. doi: 10.1002/jwmg.599
Ganey, J. L., Balda, R. P., and King, R. M. (1993). Metabolic rate and evaporative water loss of Mexican spotted and great horned owls. Wilson Bulletin 105, 645–656.
Ganey, J. L., Duncan, R. B., and Block, W. M. (1992). “Use of oak and associated woodlands by Mexican spotted owls in Arizona,” in Ecology and Management of Oak and Associated Woodlands, General Technical Report RM-218, eds. P. F. Ffolliott, G. J. Gottfried, D. A. Bennett, V. M. Hernandez, C. A. Ortega-Rubio, and R. H. Hamre (Fort Collins, CO: USDA Forest Service), 125–128.
Ganey, J. L., Kyle, S. C., Rawlinson, T. A., Apprill, D. L., and Ward, Jr., J.P. (2014). Relative abundance of small mammals in nest core areas and burned wintering areas of Mexican spotted owls in the Sacramento Mountains, New Mexico. Wilson J. Ornithol. 126, 47–52. doi: 10.1676/13-117.1
Ganey, J. L., Wan, H. Y, Cushman, S. A., and Vojta, C. D. (2017). Conflicting perspectives on spotted owls, wildfire, and forest restoration. Fire Ecol. 13, 146–165. doi: 10.4996/fireecology.130318020
Gutiérrez, R. J., Franklin, A. B., and LaHaye, W. S. (1995). Spotted Owl (Strix occidentalis). (Washington, DC: The Academy of Natural Sciences Philadelphia, and The American Ornithologists Union). doi: 10.2173/bna.179
Gutiérrez, R. J., and Pritchard, J. (1990). Distribution, density, and age structure of spotted owls on two southern California habitat islands. Condor 92, 491–495. doi: 10.2307/1368246
Gutiérrez, R. J., Verner, J., McKelvey, K. S., Noon, B. R., Steger, G. N., Call, D. R., et al. (1992). “Habitat relations of the California spotted owl,” in The California Spotted Owl: A Technical Assessment of Its Current Status, General Technical Report PSW-GTR-133, eds. J. Verner, K. S. McKelvey, B. R. Noon, R. J. Gutiérrez, G. I. Gould, Jr., and T. W. Beck (Albany, CA: USDA Forest Service), 79–98.
Hamer, T. E., Hays, D. L., Senger, C. M., and Forsman, E. D. (2001). Diets of northern barred owls and northern spotted owls in an area of sympatry. J. Raptor Res. 35, 221–227.
Hanson, C. T., Bond, M. L., and Lee, D. E. (2018). Effects of post-fire logging on California spotted owl occupancy. Nat. Conserv. 24, 93–105. doi: 10.3897/natureconservation.24.20538
Hanson, C. T., Odion, D. C., DellaSala, D. A., and Baker, W. L. (2009). Overestimation of fire risk in the Northern Spotted Owl Recovery Plan. Conserv. Biol. 23, 1314–1319. doi: 10.1111/j.1523-1739.2009.01265.x
Hershey, K. T., Meslow, E. C., and Ramsey, F. L. (1998). Characteristics of forests at spotted owl nest sites in the Pacific Northwest. J. Wildl. Manag. 62, 1398–1410. doi: 10.2307/3802006
Hessburg, P. F., and Agee, J. K. (2003). An environmental narrative of Inland Northwest United States forests, 1800–2000. For. Ecol. Manage. 178, 23–59. doi: 10.1016/S0378-1127(03)00052-5
Hessburg, P. F., Agee, J. K., and Franklin, J. F. (2005). Dry forests wildland fires of the inland Northwest USA: Contrasting the landscape ecology of the presettlement and modern eras. For. Ecol. Manage. 211, 117–139. doi: 10.1016/j.foreco.2005.02.016
Holden, Z. A., Swanson, A., Luce, C. H., Jolly, W. M., Maneta, M., Oyler, J. W., et al. (2018). Decreasing fire season precipitation increased recent western US forest wildfire activity. Proc. Natl. Acad. Sci. U.S.A. 115, E8349–E8357. doi: 10.1073/pnas.1802316115
Hornsby, A. D., and Matocq, M. D. (2012). Differential regional response of the bushy-tailed woodrat (Neotoma cinerea) to late Quaternary climate change. J. Biogeogr. 39, 289–305. doi: 10.1111/j.1365-2699.2011.02616.x
Irwin, L. L., Rock, D. F., Rock, S. C., Loehle, C., and Van Deusen, P. (2015). Forest ecosystem restoration: Initial response of spotted owls to partial harvesting. For. Ecol. Manage. 354, 232–242. doi: 10.1016/j.foreco.2015.06.009
Jenness, J. S., Beier, P., and Ganey, J. L. (2004). Associations between forest fire and Mexican spotted owls. Forest Science 50, 765–772.
Jones, G. M., Gutiérrez, R. J., Tempel, D. J., Whitmore, S. A., Berigan, W. J., and Peery, M. Z. (2016). Megafires: an emerging threat to old-forest species. Front. Ecol. Environ. 14, 300–306. doi: 10.1002/fee.1298
LaHaye, W. S., Gutiérrez, R. J., and Call, D. R. (1997). Nest-site selection and reproductive success of California spotted owls. Wilson Bull. 109, 42–51.
Lee, D. E. (2018). Spotted owls and forest fire: a systematic review and meta-analysis of the evidence. Ecosphere 9:e02354. doi: 10.1002/ecs2.2354
Lee, D. E., and Bond, M. L. (2015a). Occupancy of California spotted owl sites following a large fire in the Sierra Nevada, California. Condor 117, 228–236. doi: 10.1650/CONDOR-14-155.1
Lee, D. E., and Bond, M. L. (2015b). Previous year's reproductive state affects Spotted Owl site occupancy and reproduction responses to natural and anthropogenic disturbances. Condor 117, 307–319. doi: 10.1650/CONDOR-14-197.1
Lee, D. E., Bond, M. L., Borchert, M. I., and Tanner, R. (2013). Influence of fire and salvage logging on site occupancy of spotted owls in the San Bernardino and San Jacinto Mountains of Southern California. J. Wildl. Manage. 77, 1327–1341. doi: 10.1002/jwmg.581
Lee, D. E., Bond, M. L., and Siegel, R. S. (2012). Dynamics of California spotted owl breeding-season site occupancy in burned forests. Condor 114, 792–802. doi: 10.1525/cond.2012.110147
Littell, J. S., McGuire Elsner, M., Mauger, G. S., Lutz, E. R., Hamlet, A. F., and Salathé, E. P. (2011). Regional Climate and Hydrologic Change in the Northern U.S. Rockies and Pacific Northwest: Internally Consistent Projections of Future Climate for Resource Management. Final Project Report, USFS JVA 09-JV-11015600-039 (Seattle, WA: Climate Impacts Group, University of Washington).
Littell, J. S., McKenzie, D., Peterson, D. L., and Westerling, A. L. (2009). Climate and wildfire area burned in western U.S. ecoprovinces, 1916–2003. Ecol. Appli. 19, 1003–1021. doi: 10.1890/07-1183.1
Littell, J. S., McKenzie, D., Wan, H. Y., and Cushman, S. A. (2018). Climate change and future wildfire in the western United States: an ecological approach to nonstationarity. Earth's Fut. 6, 1097–1111. doi: 10.1029/2018EF000878
May, C. A., Petersburg, M. L., and Gutiérrez, R. J. (2004). Mexican spotted owl nest- and roost-site habitat in northern Arizona. J. Wildl. Manag. 68, 1054–1064. doi: 10.2193/0022-541X(2004)068[1054:MSONAR]2.0.CO;2
McKenzie, D., Gedalof, Z., Peterson, D. L., and Mote, P. (2004). Climatic change, wildfire, and conservation. Conserv. Biol. 18, 890–902. doi: 10.1111/j.1523-1739.2004.00492.x
McKenzie, D., and Littell, J. S. (2017). Climate change and the eco-hydrology of fire: Will area burned increase in a warming western USA? Ecol. Appl. 27, 26–36. doi: 10.1002/eap.1420
Meyer, M. D., Kelt, D. A., and North, M. P. (2007). Microhabitat associations of northern flying squirrels in burned and thinned forest stands of the Sierra Nevada. Am. Midland Natur. 157, 202–211. doi: 10.1674/0003-0031(2007)157[202:MAONFS]2.0.CO;2
Miller, J. D., Safford, H. D., Crimmins, M., and Thode, A. E. (2009). Quantitative evidence for increasing forest fire severity in the Sierra Nevada and southern Cascade Mountains, California and Nevada, USA. Ecosystems 12, 16–32. doi: 10.1007/s10021-008-9201-9
Nakicenović, N., Alcamo, J., Grubler, A., Riahi, K., Roehrl, R. A., Rogner, H. H., et al. (2000). Special Report on Emissions Scenarios (SRES), A Special Report of Working Group III of the Intergovernmental Panel on Climate Change (Cambridge: Cambridge University Press), 4.
Odion, D. C., Hanson, C.T., Arsenault, A., Baker, W. L., and DellaSala, D. A. (2014). Examining historical and current mixed-severity fire regimes in ponderosa pine and mixed-conifer forests of western North America. PLoS ONE 9:e87852. doi: 10.1371/journal.pone.0087852
Olson, D. M., and Dinerstein, E. (2002). The Global 200: priority ecoregions for global conservation. Ann. Missouri Botanical Garden 89, 199–224. doi: 10.2307/3298564
Parks, S. A., Holsinger, L. M., Panunto, M. H., Jolly, W. M., Dobrowski, S. Z., and Dillon, G. K. (2018). High-severity fire: evaluating its key drivers and mapping its probability across western US forests. Environ. Res. Lett. 13:044037. doi: 10.1088/1748-9326/aab791
Peery, M. Z., Gutiérrez, R. J., Kirby, R., Ledee, O. E., and Lahaye, W. (2012). Climate change and spotted owls: potential contrasting responses in the Southwestern United States. Glob. Chang. Biol. 18, 865–880. doi: 10.1111/j.1365-2486.2011.02564.x
Reilly, M. J., Dunn, C. J., Meigs, G. W., Spies, T. A., Kennedy, R. E., Bailey, J. D., et al. (2017). Contemporary patterns of fire extent and severity in forests of the Pacific Northwest, USA (1985–2010). Ecosphere 8:e01695. doi: 10.1002/ecs2.1695
Rinkevich, S. E., and Gutiérrez, R. J. (1996). Mexican spotted owl habitat characteristics in Zion National Park. J. Raptor Res. 30, 74–78.
Roberts, S. L., Kelt, D. A., van Wagtendonk, J. W., Miles, A. K., and Meyer, M. D. (2015). Effects of fire on small mammal communities in frequent-fire forests in California. J. Mammal. 96, 107–119. doi: 10.1093/jmammal/gyu011
Roberts, S. L., van Wagtendonk, J. W., Miles, A. K., and Kelt, D. A. (2011). Effects of fire on Spotted Owl site occupancy in a late successional forest. Biol. Conserv. 144, 610–619. doi: 10.1016/j.biocon.2010.11.002
Seamans, M. E., and Gutiérrez, R. J. (1995). Breeding habitat of the Mexican spotted owl in the Tularosa Mountains, New Mexico. Condor 97, 944–952. doi: 10.2307/1369533
Seamans, M. E., Gutiérrez, R. J., May, C. A., and Perry, M. Z. (1999). Demography of two Mexican spotted owl populations. Conserv. Biol. 13, 744–754. doi: 10.1046/j.1523-1739.1999.98302.x
Skinner, C. N. (1995). Change in spatial characteristics of forest openings in the Klamath Mountains of northwestern California, USA. Landsc. Ecol. 10, 219–228. doi: 10.1007/BF00129256
Smith, R. B., Peery, M. Z., Gutiérrez, R. J., and LaHaye, W. S. (1999). The relationship between spotted owl diet and reproductive success in the San Bernardino Mountains, California. Wilson Bull. 111, 22–29.
Stephens, S. L., Martin, R. E., and Clinton, N. E. (2007). Prehistoric fire area and emissions from California's forests, woodlands, shrublands, and grasslands. For. Ecol. Manage. 251, 205–216. doi: 10.1016/j.foreco.2007.06.005
Stephens, S. L., Miller, J. D., Collins, B. M., North, M. P., Keane, J. J., and Roberts, S. L. (2016). Wildfire impacts on California spotted owl nesting habitat in the Sierra Nevada. Ecosphere 7:e01478. doi: 10.1002/ecs2.1478
Thomas, C. D., Cameron, A., Green, R. E., Bakkenes, M., Beaumont, L. J., Collingham, Y. C., et al. (2004). Extinction risk from climate change. Nature 427, 145–148. doi: 10.1038/nature02121
Thrailkill, J., and Bias, M. A. (1989). Diets of Breeding and nonbreeding California spotted owls. J. Raptor Res. 23, 39–41.
Turco, M., Rosa-Cánovas, J. J., Bedia, J., Jerez, S., Montávez, J. P., Llasat, M. C., et al. (2018). Exacerbated fires in Mediterranean Europe due to anthropogenic warming projected with non-stationary climate-fire models. Nat. Commun. 9:3821. doi: 10.1038/s41467-018-06358-z
U.S. Department of Agriculture (2004). Sierra Nevada Forest Plan Amendment Final Supplemental Environmental Impact Statement. Vallejo, CA: USDA Forest Service.
U.S. Fish and Wildlife Service (2012). Final Recovery Plan for the Mexican Spotted Owl (Strix occidentalis lucida), First Revision. Albuquerque, NM: U.S. Fish and Wildlife Service.
Wan, H. Y., Cushman, S. A., and Ganey, J. L. (2018b). Habitat fragmentation reduces genetic diversity and connectivity of the Mexican spotted owl: a simulation study using empirical resistance models. Genes 9:403. doi: 10.3390/genes9080403
Wan, H. Y., Cushman, S. A., Ganey, J. L., and Vojta, C. D. (2018a). Managing emerging threats to spotted owls. J. Wildl. Manage. 82, 682–697. doi: 10.1002/jwmg.21423
Weathers, W. W., Hodum, P. J., and Blakesley, J. A. (2001). Thermal ecology and ecological energetics of California spotted owls. Condor 103, 678–690. doi: 10.1650/0010-5422(2001)103[0678:TEAEEO]2.0.CO;2
Weaver, H. (2014). Fire as an ecological and silvicultural factor in the ponderosa pine region of the pacific slope. Fire Ecol. 10, 3–13. doi: 10.1007/BF03400626
Weigl, P. D. (2007). The northern flying squirrel (Glaucomys sabrinus): a conservation challenge. J. Mammal. 88, 897–907. doi: 10.1644/06-MAMM-S-333RR.1
Westerling, A. L., Hidalgo, H. G., Cayan, D. R., and Swetnam, T. W. (2006). Warming and earlier spring increase western U.S. forest wildfire activity. Science 313, 940–943. doi: 10.1126/science.1128834
Westerling, A. L., Turner, M. G., Smithwick, E. A. H., Romme, W. H., and Ryan, M. G. (2011). Continued warming could transform Greater Yellowstone fire regimes by mid-21st century. Proc. Natl. Acad. Sci. U.S.A. 108, 13165–13170. doi: 10.1073/pnas.1110199108
Westerling, L. L. (2016). Increasing western US forest wildfire activity: sensitivity to changes in the timing of spring. Philoso. Transac. Royal Soc. 371:20150178. doi: 10.1098/rstb.2015.0178
Wiens, J. D., Anthony, R. G., and Forsman, E.D. (2014). Competitive interactions and resource partitioning between northern spotted owls and barred owls in Western Oregon. Wildl. Monogr. 185, 1–50. doi: 10.1002/wmon.1009
Willey, D. W., and van Riper III, C. (2007). Home range characteristics of Mexican spotted owls in the canyonlands of Utah. J. Raptor Res. 41, 10–15. doi: 10.3356/0892-1016(2007)41[10:HRCOMS]2.0.CO;2
Keywords: area burned, biodiversity, California spotted owl, fire severity, fragmentation, habitat loss, Mexican spotted owl, northern spotted owl
Citation: Wan HY, Cushman SA and Ganey JL (2019) Recent and Projected Future Wildfire Trends Across the Ranges of Three Spotted Owl Subspecies Under Climate Change. Front. Ecol. Evol. 7:37. doi: 10.3389/fevo.2019.00037
Received: 31 October 2018; Accepted: 31 January 2019;
Published: 04 March 2019.
Edited by:
Laurentiu Rozylowicz, University of Bucharest, RomaniaReviewed by:
Marco Turco, Barcelona Supercomputing Center, SpainCopyright © 2019 Wan, Cushman and Ganey. This is an open-access article distributed under the terms of the Creative Commons Attribution License (CC BY). The use, distribution or reproduction in other forums is permitted, provided the original author(s) and the copyright owner(s) are credited and that the original publication in this journal is cited, in accordance with accepted academic practice. No use, distribution or reproduction is permitted which does not comply with these terms.
*Correspondence: Ho Yi Wan, aG95aXdhbkBnbWFpbC5jb20=
Disclaimer: All claims expressed in this article are solely those of the authors and do not necessarily represent those of their affiliated organizations, or those of the publisher, the editors and the reviewers. Any product that may be evaluated in this article or claim that may be made by its manufacturer is not guaranteed or endorsed by the publisher.
Research integrity at Frontiers
Learn more about the work of our research integrity team to safeguard the quality of each article we publish.