- 1Laboratoire de Botanique, Département de Biologie et Ecologie Végétales, Faculté des Sciences, Université d'Antananarivo, Antananarivo, Madagascar
- 2Kew Madagascar Conservation Centre, Antananarivo, Madagascar
- 3Comparative Plant and Fungal Biology, Royal Botanic Gardens, London, United Kingdom
- 4Laboratoire Evolution and Diversité Biologique, CNRS/ENSFEA/IRD/Université Toulouse III, Université Paul Sabatier, Toulouse, France
- 5Conservation Science, Royal Botanic Gardens, London, United Kingdom
- 6Biodiversity Informatics and Spatial Analysis, Royal Botanic Gardens, London, United Kingdom
- 7School of GeoSciences, The University of Edinburgh, Edinburgh, United Kingdom
- 8Centre for African Ecology, School of Animal and Plant Sciences, University of Witwatersrand, Johannesburg, South Africa
- 9Royal Botanic Garden Edinburgh, Edinburgh, United Kingdom
Grassland, woodland, and forest are three key vegetation types that co-occur across the central highlands of Madagascar, where the woodland has historically been considered as degraded forest. Here, we use grass functional traits to inform our understanding of the biogeography of Malagasy vegetation and the differentiation of vegetation types in the region. We sampled grass community composition at 56 sites across the central highlands of Madagascar spanning grassland, woodland, and forest. We selected seven functional traits known to correlate with different aspects of life history collated via GrassBase (habit, culm type, physiology, leaf consistency, plant height, leaf width, and leaf length) for the 71 constituent species. Via analyses of the beta diversity, rank abundance, functional dispersion, functional group richness, and community phylogenetic structure of grassland communities, we differentiate these vegetation types using plant functional traits. Grassland and woodland are highly similar in grass species composition and dominated by the same species (Loudetia simplex, Trachypogon spicatus, and Schizachyrium sanguineum). In contrast, forest grass species composition significantly differs from both grassland and woodland. Consistent with these species composition patterns, the vegetation types can be distinguished based on physiology, culm type, and leaf consistency. Forests harbor primarily C3 grasses, which are almost invariably laterally spreading with herbaceous leaves. In contrast, both grassland and woodland species are predominantly tall, caespitose C4 grasses with coriaceous leaves. Forest grasses are phylogenetically clustered and less diverse than the grassland and woodland communities. Further, we sampled bark thickness of the common woody species occurring in the woodland and forest of the region and found that the relative bark thickness of the woodland tree species was significantly greater than that of forest species from the same genus. We found that the functional traits and architecture of grasses diverge strongly between forest and the grassland-woodland mosaic. We conclude that the woodlands, primarily dominated by Uapaca bojeri Baill., are a form of savanna and not forest as has been previously suggested.
Introduction
Forest and savanna are vegetation types that can co-occur within landscapes and found in areas with similar climate and soils (Bond, 2005; Bond and Parr, 2010; Charles-Dominique et al., 2015). While savanna and forest can appear superficially similar in terms of the density and number of trees, the functional traits of the trees of each vegetation type differ (Ratnam et al., 2011; Griffith et al., 2017). Savanna and tropical grasslands are open canopy environments with variable tree cover and a continuous grassy ground layer (Bond et al., 2003a; Bond, 2008; Lehmann et al., 2009; Bond and Parr, 2010; Edwards et al., 2010). Defining the functional nature of a vegetation type is often linked to a broader issue of vegetation classifications based on structural associations and even climatic definitions (Schimper and Fisher, 1903; Whittaker, 1975; Scholes and Hall, 1996; Woodward et al., 2004), and often almost entirely based on the woody components of vegetation. The Food and Agriculture Organization of the United Nations (FAO) defines forest as land spanning more than 0.5 ha with more than 10% tree canopy cover and trees higher than 5 m (or having the potential to reach a height of 5 m; FAO, 2006). Alternatively, the United Framework Convention on Climate Change (UNFCCC) defines thresholds of 0.5–1.0 ha forested land, 10–30% tree canopy cover, and 2–5 m tree height. While savannas are defined as mixed tree–grass systems with a discontinuous tree canopy in a continuous grass layer (Scholes and Archer, 1997; House et al., 2003), the notion of the limits of tropical grassy vegetation types as being defined by dominance of C4 grasses in the ground layer is more recent (Bond, 2008). House and Hall (2001) described savannas as a continuum between tropical forests and grasslands and use the term to include all plant communities from treeless grasslands to closed canopy woodlands. In other literature, grasslands encompass non-woody and woody grasslands (savannas, woodlands, shrublands, and tundra) together (White et al., 2000) because they are defined as terrestrial ecosystems dominated by herbaceous and shrub vegetation, maintained by fire, grazing, drought, and/or freezing temperatures.
Persistent confusion around the delineation of vegetation types often centers on the limited ecological information in structural definitions (Ratnam et al., 2011; Parr et al., 2014; Lehmann and Parr, 2016). While structural definitions offer precision, they are inadequate when the functional responses of forest and savanna plant species to processes such as fire, herbivory, and drought differ (Lehmann et al., 2011). Several studies have shown functional trait differences between trees of forests and savannas in South America (Hoffmann et al., 2003, 2005, 2012), Australia (Lawes et al., 2013) and Africa (Charles-Dominique et al., 2015), all demonstrating that savanna trees have morphological and physiological traits which make them adapted to the frequent fires that characterize savannas in high rainfall regions (Ratnam et al., 2011; Charles-Dominique et al., 2015). Hoffmann et al. (2003, 2012) showed that bark thickness is a key trait to attain fire-resistance threshold and savanna species have thicker bark than forest species, thereby reducing the risk of stem death during fire.
However, the composition and traits of the grassy ground layer are often neglected yet responsible for the physiognomic structure of vegetation (Bond, 2008), promoting either fire, or herbivory due to grass productivity and architecture (Knapp et al., 1998; Sage and Monson, 1998; Archibald and Hempson, 2016). On the other hand, C4 grasses are considered absent from forest understorey, which can be dominated by herbaceous life forms (Ratnam et al., 2011). However, some C4 grasses are known from forest understories [e.g., Setaria palmifolia (J. Koenig) Stapf, Microstegium nudum (Trin.) A. Camus, and Digitaria radicosa (J. Presl) Miq. (Clayton, 1970)]. There are numerous areas of the world where C3 grasses either dominate or co-dominate with C4 grasses and these are still considered savanna [e.g., Neotropical savannas (Cabido et al., 1997; Bremond et al., 2012), and tropical savannas in Africa and Australia (Lattanzi, 2010)]. Overall, the focus of studies examining the functional traits of vegetation in distinguishing forest and savanna has been woody rather than grass species (Hoffmann et al., 2003, 2005, 2012; Ratnam et al., 2011; Lawes et al., 2013; Charles-Dominique et al., 2015). While photosynthetic pathway is no doubt of high relevance, this focus also belies important functional variation among grasses of the same photosynthetic pathway (Forrestel et al., 2015; Simpson et al., 2016).
Across Madagascar, a mosaic of grassland covers 65 % of the island and in the central region, it is interspersed with subhumid forest, along with extensive areas of woodland (Moat and Smith, 2007). In this region, woodlands are dominated almost solely by Uapaca bojeri Baill. (commonly called Tapia) in association with a few other woody species that belong to families endemic to Madagascar, especially Sarcolaenaceae (Leptolaena pauciflora Baker, Sarcolaena oblongifolia F. Gérard, Schizolaena microphylla H. Perrier) and Asteropeiaceae (Asteropeia densiflora Baker, A. labatii G.E. Schatz, Lowry & A.-E. Wolf). Malagasy grasslands and savannas have long been considered as anthropogenically derived, the result of forest degradation via clearing, and altered fire and grazing regimes over the last 2,000 years (Perrier de la Bâthie, 1921; Humbert, 1927; Koechlin et al., 1974; Lowry et al., 1997). As such, woodlands of the central highlands have been historically and persistently classified as degraded forests (Koechlin et al., 1974; White, 1983; Faramalala, 1995) and managed as such through suppression of fire (Kull, 2002). Recent studies however support early origins of these extensive grasslands with their numerous endemic grass, forb, and animal species that evolved locally millions of years before human colonization (Bond et al., 2008; Vorontsova et al., 2016; Hackel et al., 2018), which occurred only a few 1,000 years before present (Pierron et al., 2017). Grassland, woodland and forest co-occur in the central highlands of Madagascar with changes in vegetation types occurring over small scales, often just meters where there are no discernible differences in climate and soils. These mosaics offer an opportunity to study the functional and compositional characteristics of the ground layer among these vegetation types to identify the disturbance regimes each is adapted to. Within Poaceae are a broad suite of architectural and morphological functional traits commonly assessed in woody plants, but little explored in grasses. In this study, we examine the composition and functional traits of grass communities among these three vegetation types to quantify differences in community composition and grass functional traits. This allows us to test whether any differences map to vegetation types, in a region where woodlands have long been considered degraded forests but where limits between woodland and grassland appear idiosyncratic. We then assess the phylogenetic structure of each vegetation type as an indicator of its evolutionary history. Finally, as an independent test of vegetation type differences, i.e., savanna vs. forest, we compare the relative bark thickness of woody species, including closely related species of Uapaca that characterize woodland and forest, to determine whether functional traits known to differ between forest and savanna can corroborate findings of the grass communities. We hypothesize that grassland and woodland harbor a high phylogenetic diversity of grass species which have evolved for a long period before human arrival. We predict a functional similarity between grassland and woodland grass community, where light availability and fire regimes are similar. In contrast, forest vegetation, characterized by a low-light environment in the ground layer, will select for a different grasses community with another set of functional traits.
Materials and Methods
Study Sites
The grass flora was sampled at 56 sites distributed across the central ecoregion of Madagascar, which measures 192,141 km2 (Humbert, 1955) (Figure 1 and Supplementary Table 1). From North to South, Ibity, Ambatofinandrahana, Itremo, Ambalavao, Andringitra, Isalo, and Ihorombe were the study locations. Rajeriarison and Faramalala (1999) categorized the climate of these regions as subhumid with a mean annual rainfall ranging from 1,000 to 1,500 mm and 5–7 months of dry season. The elevation ranges from 800 to 1,800 meters and soils are primarily ferrallitic, on sandstone and basement gneiss (Moat and Smith, 2007). Fire regime in the sites is every one to 3 years (Alvarado et al., 2018). In our study, we are going to use “grassland” for treeless vegetation, consisting of continuous C4 grass layer, and “woodland” for woody grasslands. Forest is defined as closed-canopy vegetation, with no C4 grass layer. We selected sites considered as undisturbed by the absence of invasive species and overgrazing. Each site was in an area of uniform vegetation that was a minimum of 60 m wide. We sampled 24 sites of grassland, 20 sites of tapia (Uapaca bojeri) dominated woodland with herbaceous layer and 12 sites of gallery forest.
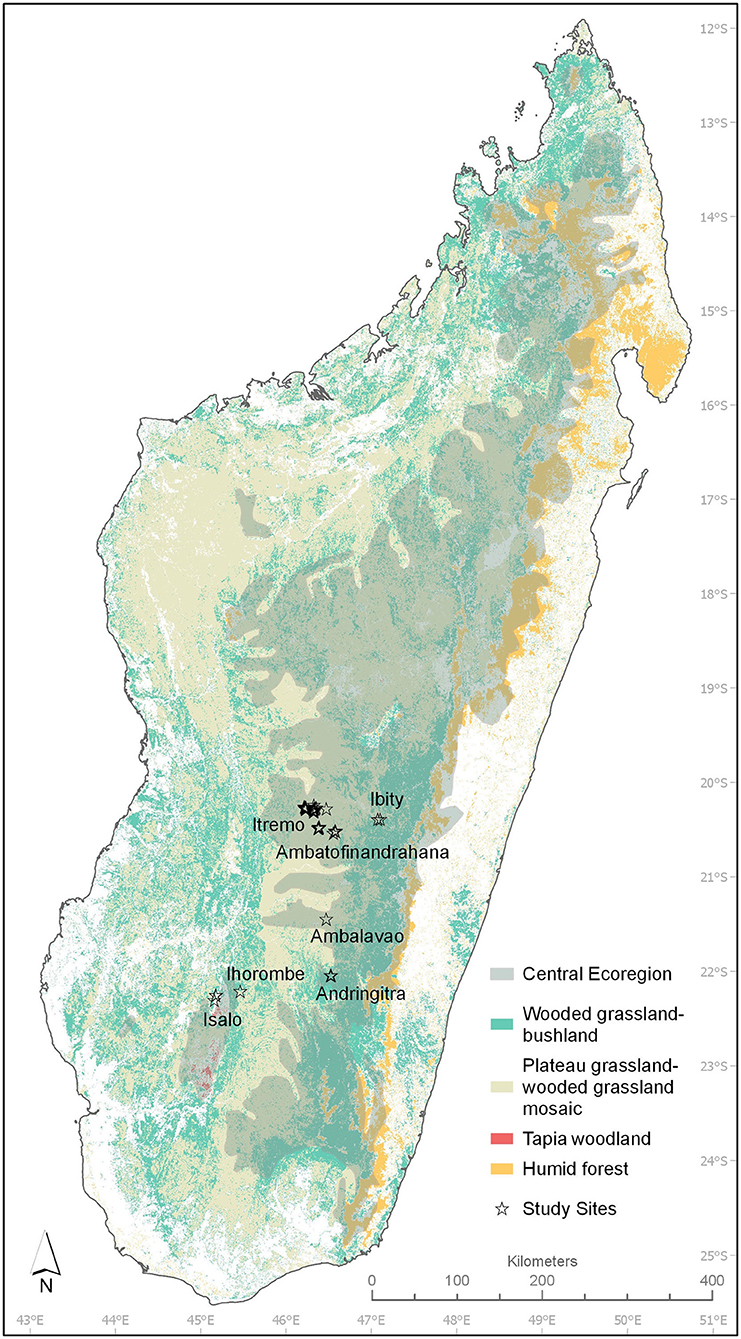
Figure 1. Map of the central ecoregion as per Humbert (1955); grassland (“plateau grassland—wooded grassland mosaic and wooded grassland—bushland,” tapia woodland and humid forest distribution as per Moat and Smith (2007) and the study sites location.
Data Collection
Grass Species Composition
Based on the sampling method described by Vorontsova et al. (2016), we collected data on grass species composition at 56 sites; data from 17 sites from Vorontsova et al. (2016) that met the above criteria were also included. The method focuses on grass species composition and frequency and consists of four transects. First, all grass species within a center circle plot of one meter diameter were documented. Then, from the center point, four 25-m transects, each following a random direction (based on a compass bearing), were laid out. Along each transect, the same circular plot of one-meter diameter was sampled at five-meter intervals, representing grass species composition over 16.5 m2 for a single site (see Supplementary Table 1).
Grass Functional Traits
Across these 56 sites, 71 grass species were recorded, and for each species, seven functional traits were collated: (1) habit (annual, perennial); (2) culm type (prostrate, decumbent, ascendant, erect); (3) leaf consistency (herbaceous, coriaceous); (4) culm height; (5) leaf width; (6) leaf length, and (7) photosynthetic pathway. These traits were derived from GrassBase, a global database of 11,369 standardized grass species descriptions compiled from taxonomic literature (Clayton et al., 2006). These data were supplemented from literature (Bosser, 1969; Van Oudtshoorn, 2002) and by data gathering from multiple herbarium specimens held at the herbarium of the Parc Botanique et Zoologique de Tsimbazaza and the Royal Botanic Gardens, Kew, UK. Photosynthetic pathway data were obtained from the checklist of Osborne et al. (2014); for species with an undetermined pathway, it was inferred from their position in known C3 or C4 lineages (Hackel et al., 2018).
The choice of these seven traits was based on their role related to light acquisition and flammability. The annual or perennial habit of the species was recorded. In GrassBase, perennials are described as possessing one or more of the following: rhizome or rootstock; a compact mass of dead basal sheaths remaining from previous year's growth; dormant buds in the basal leaf axils for the following year's growth. Annual grass species are commonly considered more abundant than perennials in disturbed habitats, even those with frequent fire (Grime, 1977; Pitelka, 1977). Culm type was categorized into four types: prostrate (lying flat), decumbent (lying along the ground, but with culm apex upright), ascendant (growing upward after an initial horizontal segment) and erect (fully upright) (Beentje, 2010). A prostrate culm type is expected to aid light interception in shaded environments by minimizing self-shading within the plant via lateral spreading (Falster and Westoby, 2003). Erect life forms tend to be species with intravaginal buds [buds between leaf sheaths, not breaking through them to form stolons and rhizomes as in extravaginal buds (Brejda et al., 1989)], that can increase protection of buds from fire, but also confine the architecture of a grass by preventing lateral spreading as by definition these grass species cannot root at the node (Linder et al., 2018), and are therefore generally associated with environments characterized by frequent fire and high light. Depending on the toughness of the leaf blade, we recorded leaf consistency which was categorized into two groups: coriaceous [leathery, tough (Beentje, 2010)], or herbaceous [with the texture of herb, soft and pliable (Beentje, 2010)] leaves.
Data Analysis
Environmental Occurrence of Grassland, Woodland, and Forest
The central highlands were delimited following Humbert's (1955) ecoregion map classifying it as “Central ecoregion.” The vegetation map developed by Moat and Smith (2007) was used to define the limits of our three vegetation types. Vegetation units classified as “plateau grassland—wooded grassland mosaic and wooded grassland—bushland,” “tapia forest” and “humid forest” in the vegetation map correspond, respectively to our studied vegetation types grassland, woodland, and forest. Any other vegetation units in the vegetation map were omitted from our analysis. The distribution of the three vegetation types across climatic variables (Bio_1: annual mean temperature and Bio_12: annual precipitation) obtained from Worldclim Global Climate Data version 2 (Fick and Hijmans, 2017), within the central ecoregion was plotted (see Supplementary Figures 1, 2). The variables were downloaded at 30 s spatial resolution. From these, we have generated datasets per climatic variable for our studied ecoregion. Data for each environmental variable were ordered from lowest to highest and binned in the relevant 1°C and 50-mm intervals, respectively. Due to the very high density of points within each bin, we randomly subsampled our data into 1,000 points, using 1,000 iterations, without replacement. For the subsampled data within each interval, the probability of the presence of each of the three vegetation types was calculated as the mean of all points within the temperature and precipitation interval and the standard errors were calculated for each proportion. The probability was then plotted against Bio_1 and Bio_12 to compare the distribution of grassland, woodland and forest within the region. We fitted our 56 sampled sites within the temperature and precipitation gradient as rugs on each x-axis of the probability plots. For each temperature and precipitation interval, the area of land covered by each vegetation was plotted. From the raw climate data, the area of each vegetation type was obtained by counting the number of points within each interval as each point corresponds to one kilometer square of land.
Grass Species and Trait Composition
We examined the composition of grass communities from three distinct vegetation types as defined by their woody component (or its absence) and its traits: grassland, woodland and forest. All statistical analyses were performed in R 3.0.2 (R Core Team, 2013). We used permutational multivariate analysis of variance (PERMANOVA) using the Bray–Curtis dissimilarity (see Supplementary Table 2) and accounted for spatial autocorrelation by including latitude and longitude as predictor variables to assess differences in plant community composition between the three vegetation types, with the R package “vegan” (Oksanen et al., 2016). Grass species and tribes were ranked by frequency per vegetation type with the “BiodiversityR” package (Kindt and Coe, 2005).
To measure species functional diversity in each vegetation type, we computed the functional dispersion index (FDis) which estimates the dispersion of species in a trait space, based on principal coordinate analysis (PCoA) and calculated on a Gower dissimilarity matrix (Gower, 1971; Laliberté and Legendre, 2010) with the “FD” package (Laliberté et al., 2014). We compared the three vegetation types in terms of FDis with an analysis of variance (ANOVA) while accounting for spatial autocorrelation. A functional group richness (FGR) was used to group grass species based on functional traits, using the same package. The resulting dendrogram was plotted against the functional traits. The number of clusters was identified visually on the resulting dendrogram.
Community phylogenetic structure was assessed per site to test how closely related the average pair of grass individuals were in each vegetation type. We used a Bayesian time-calibrated phylogenetic tree of the 71 species sampled (Supplementary Figure 3) extracted from a larger analysis of Malagasy grass species (Hackel et al., 2018). Hackel et al. (2018) did not include Andropogon trichozygus Baker, Hyparrhenia variabilis Stapf and Ischaemum polystachyum J. Presl, which were replaced with the closest available species [Andropogon huillensis Rendle, Hyparrhenia schimperi (Hochst. ex A. Rich.) and Ischaemum koleostachys (Steud.) Hack., respectively]. The species richness (Whittaker, 1972) within each vegetation type was calculated. Phylogenetic relatedness of species within grassland, woodland, and forest was measured via phylogenetic diversity (PD) index (Faith, 1992). The differences in species richness and phylogenetic diversity between vegetation types were assessed with a generalized linear model (GLM) with a Poisson distribution and log link function. The net relatedness index (NRI) and nearest taxon index (NTI) (Webb, 2000; Webb et al., 2002) for each site was calculated with the “picante” package (Kembel et al., 2010). The observed phylogenetic relatedness was compared to the expected pattern using the functions ses.mpd and ses.mntd with the “richness” null model, not taking into account species abundances in each plot, and using 999 randomizations.
Tree Bark Thickness
The bark thickness and stem circumference of 39 individuals of Uapaca bojeri in woodlands, and 27 samples from 16 species in forest (listed in Supplementary Table 3) were measured at Isalo National Park and on wood samples from humid forest in the East of Madagascar, available at the forestry department of Antananarivo University, respectively. The tree species from Eastern humid forest are mostly dominant species in our study region (KMCC, 2012). A t-test was used to compare bark thickness between Uapaca bojeri dominated woodland and forest species, and as bark thickness varies with tree size (Werner and Murphy, 2001; Lawes et al., 2011), measures of bark thickness were transformed into relative bark thickness (the ratio of individual bark thickness to stem diameter; Lawes et al., 2013) prior to analysis.
Results
Distribution of Grassland, Woodland, and Forest Across Temperature and Precipitation
Across the central ecoregion mean annual temperature ranges from 11 to 27°C and mean annual rainfall ranges from 764 to 2,660 mm per year. Here, grassland has a greater than 50% probability of occurrence where mean temperatures range from 16 to 22°C, and a 90% of probability of occurrence where temperature ranges from 11 to 12°C and 22 to 27°C. Grassland has a >50% probability of occurrence where rainfall ranges from 764 to 864 mm and from 1,160 to 1,760 mm, and a 90% or greater probability of occurrence where rainfall ranges from 864 to 1,160 mm. The probability of forest occurrence is >50% where temperature ranges from 13 to 15°C and mean rainfall ranges from 1,810 to 2,010 mm and a 90% greater probability of occurrence where rainfall is >2,010 mm.
In total, the sampled woodland covers 4,816 km2 which represents only 0.95% of the central highlands total land area (Supplementary Figures 1B, 2B). The probability of occurrence of woodland is < 5% across the rainfall and temperature gradient, corresponding to the limited land area occupied by this vegetation type. However, the greatest probability of occurrence is at 23 and 24°C. Our studied sites are primarily located in areas with a medium annual mean temperature (20–23°C). Woodland is only found in very low precipitation areas with a highest probability of occurrence of 25% where rainfall ranges from 760 to 800 mm.
Grass Diversity and Composition
A total of 71 grass species from 40 genera were recorded and identified using the Poaceae checklist of Itremo Protected Area (Nanjarisoa et al., 2017) and specimens at K, P, and TAN herbaria. We found between 2 and 19 species per plot, with a mean of 8 species. Species richness was higher in woodland and grassland than in forest, with a significant difference between the three habitats (GLM with Poisson distribution, P < 0.001). Although there is some spatial autocorrelation, the type of vegetation has a significant effect on species composition according to PERMANOVA (F = 14.42, P = 0.001). The vegetation type and the spatial location explain 33 and 9% of the variance, respectively. However, when separate pairwise comparisons are made, grassland and woodland do not differ significantly in composition (F = 1.82, P = 0.06). There are highly significant differences between both the composition of grassland and forest (F = 19.43, P = 0.001) and woodland and forest (F = 23.95, P = 0.001).
The same three species that are most common in grassland are also the dominant species in woodland: Loudetia simplex (Nees) C.E. Hubb., Trachypogon spicatus (L. f.) Kuntze and Schizachyrium sanguineum (Retz.) Alston (Figures 2A,B). All three are absent from forest, which is most commonly characterized by Oplismenus compositus (L.) P. Beauv., O. hirtellus (L.) P. Beauv., O. flavicomus Mez, and Arundinella nepalensis Trin (Figure 2C).
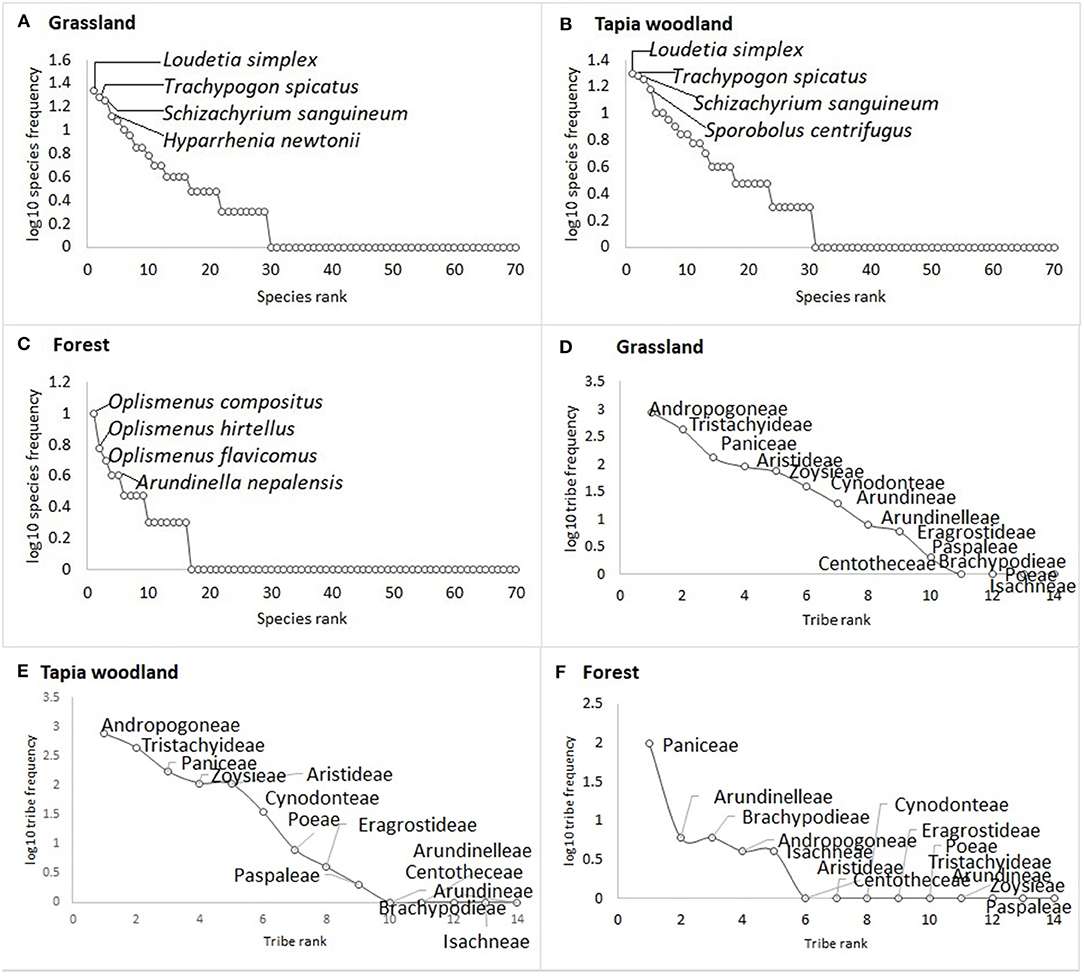
Figure 2. Species rank frequency (A–C) and tribe rank frequency (D–F) for the 71 grass species sampled within grassland, tapia woodland and forest. The same three species, belonging to the same tribes are the most frequent in grassland and tapia woodland. Forest habitat is dominated by Oplismenus species, in the Paniceae tribe.
Rank-frequency curves per grass tribe also support differences in species composition of grassland and woodland in comparison with forest (Figures 2D–F). The two Poaceae tribes Andropogoneae and Tristachyideae dominate grassland and woodland, whereas forest species mostly belong to the tribe Paniceae.
Grass Traits
Beyond the similarities in taxonomic composition, grassland and woodland grass species were also characterized by similar trait communities (Table 1). This is evidenced by the functional group richness classification (Figure 3) which identified two distinct groups based on the seven functional traits. The two supported clusters demonstrate a close match with vegetation type.
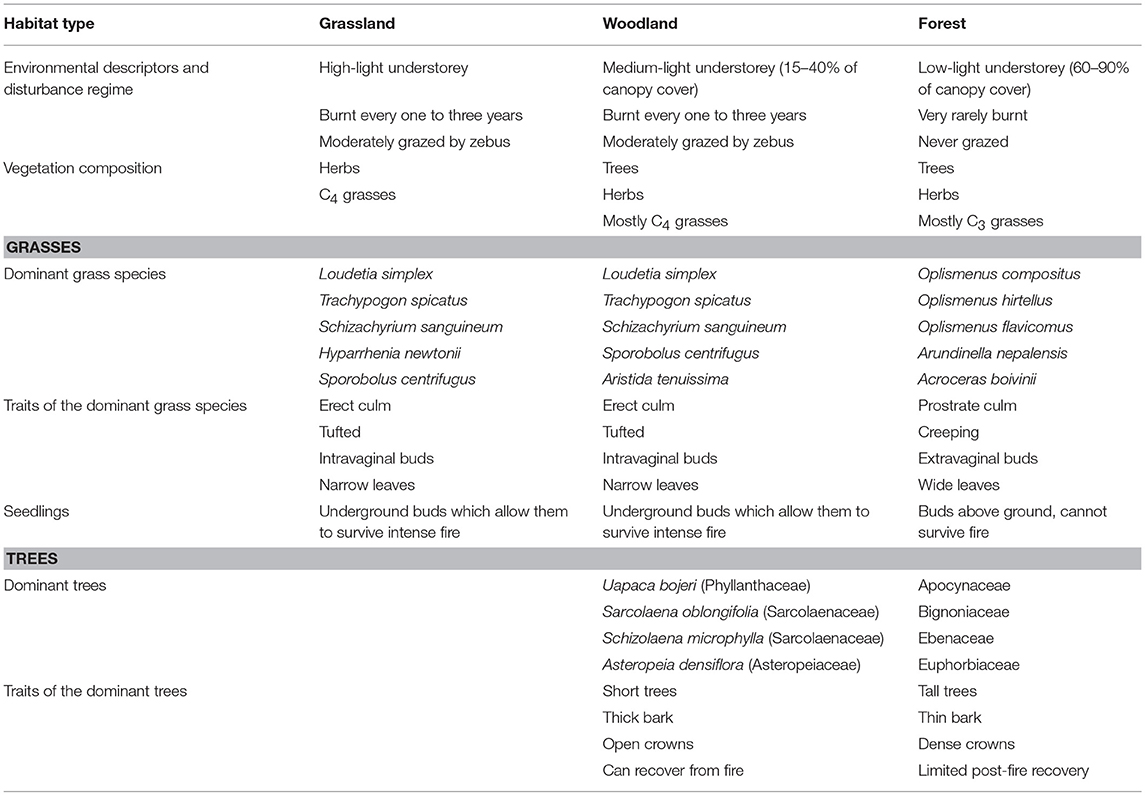
Table 1. Comparison of physical environments, species composition, and traits of dominant grass species and trees in grassland, woodland, and forests.
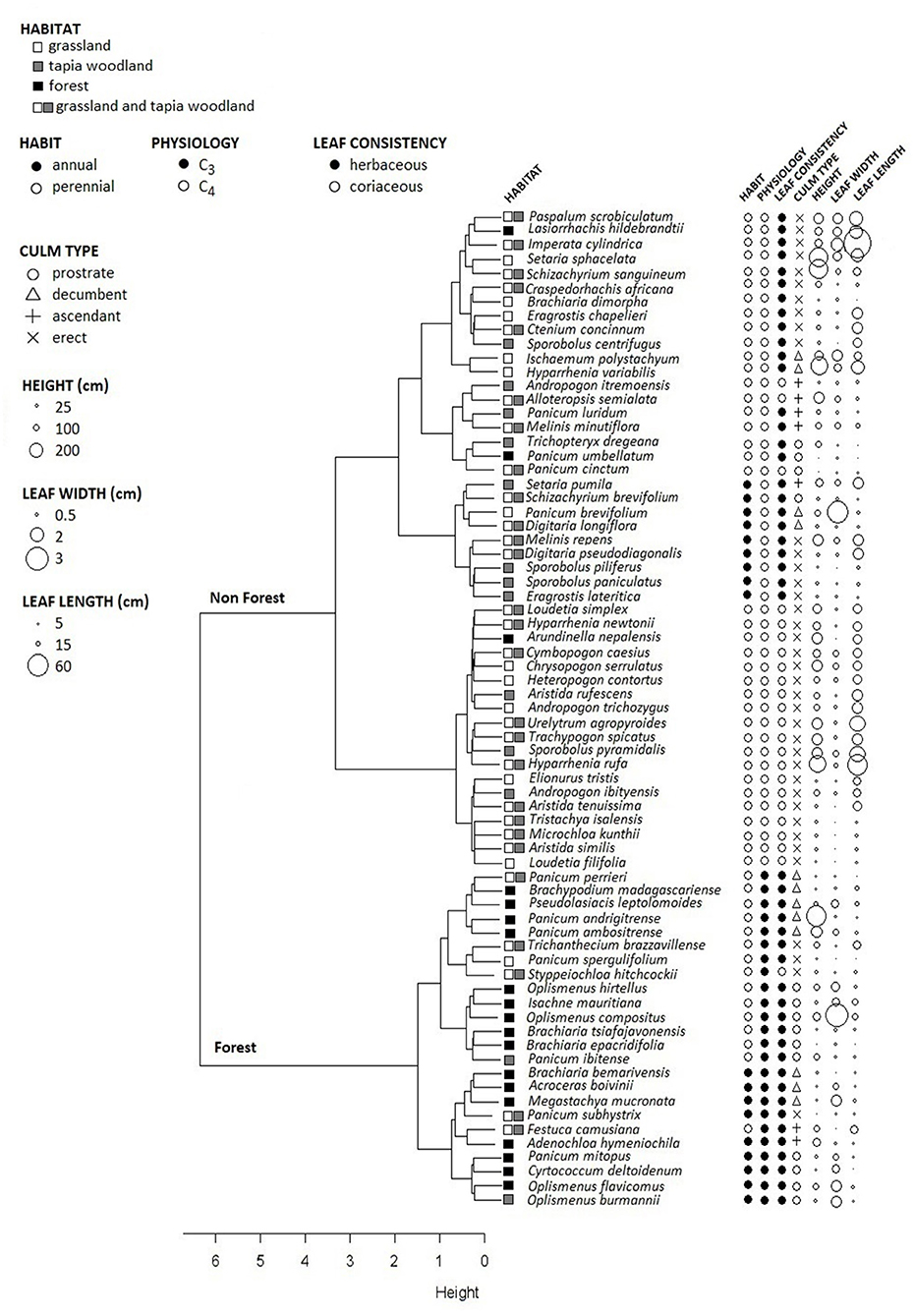
Figure 3. Functional group richness dendrogram, clustering the 71 grass species with their traits displayed. The dendrogram highlights two clusters grouping the species with similar traits from forest and non-forest habitat.
The first cluster of 44 species (called “Non Forest”) is primarily composed of species present in grassland and/or woodland (Figure 3). Within this group, 89% are C4, 86% are perennial, 91% are erect and 52.3% have coriaceous leaves. The median values of height, leaf width and leaf length of the species in this group are 100, 0.5, and 30 cm, respectively. The key trait that differentiated this “Non-Forest” from the “Forest” cluster was the architecture of culms (Figure 3). Grassland and woodland grass culms are primarily erect, leading to a tufted growth form. Their leaves are narrower but also longer and more coriaceous compared to those in the “forest” group which are wide, herbaceous and flaccid.
Of 19 grass species recorded in forest, 84% cluster in the “Forest” group by traits (Figure 3). The three forest grass species [Arundinella nepalensis, Lasiorrhachis hildebrandtii (Hack.) Stapf, and Panicum umbellatum (Trin.)] which do not cluster in this group are C4 and perennial. Species that also occur in grassland and woodland were assigned to the “forest” cluster (7 of 52 species): Panicum perrieri A. Camus, P. subhystrix A. Camus, P. ibitense A. Camus, P. spergulifolium A. Camus, Festuca camusiana St.-Yves, Styppeiochloa hitchcockii (A. Camus) Cope, and Trichanthecium brazzavillense (Franch.) Zuloaga & Morrone. These species are characterized by traits common amongst forest grasses as all species are C3, and all but one have herbaceous leaves that are shorter and wider. The Panicum species are often found in the sub-canopy of the grassland and woodland layer.
Species dispersion in functional trait space differed significantly between the three vegetation types according to ANOVA (P = 0.014). Grassland communities are significantly less diverse functionally compared to forest communities (P = 0.009) and no significant difference was found between grassland and woodland (P = 0.06). Functional diversity is similar between forest and woodland (P = 0.18).
Diversity and Phylogenetic Structure
Species richness of grass species is significantly different between the three vegetation types (GLM, P = 0.001). Species richness is the highest in woodland with a significant difference compared to grassland (GLM, P = 0.005) and forest (GLM, P < 0.001; Figure 4A). Phylogenetic diversity means are equal to 233.1, 272.3, and 140.1 for grassland, woodland, and forest, respectively. Woodland species are significantly more phylogenetically diverse compared to grassland (GLM, P < 0.001) and forest species (GLM, P < 0.001; Figure 4B). When compared to a null model, forest grass communities exhibit a pattern of phylogenetic clustering but there is a significant difference with grassland, only for NTI (ANOVA, P = 0.02). No significant difference is found between grassland and woodland (ANOVA, P = 0.145), or woodland and forest (ANOVA, P = 0.205) NTI. Woodland communities are only slightly clustered (NRI = 0.28; NTI = 0.20) and grassland communities are slightly over-dispersed phylogenetically (NRI = −0.01; NTI = −0.08; Figures 4C,D and Supplementary Figure 3).
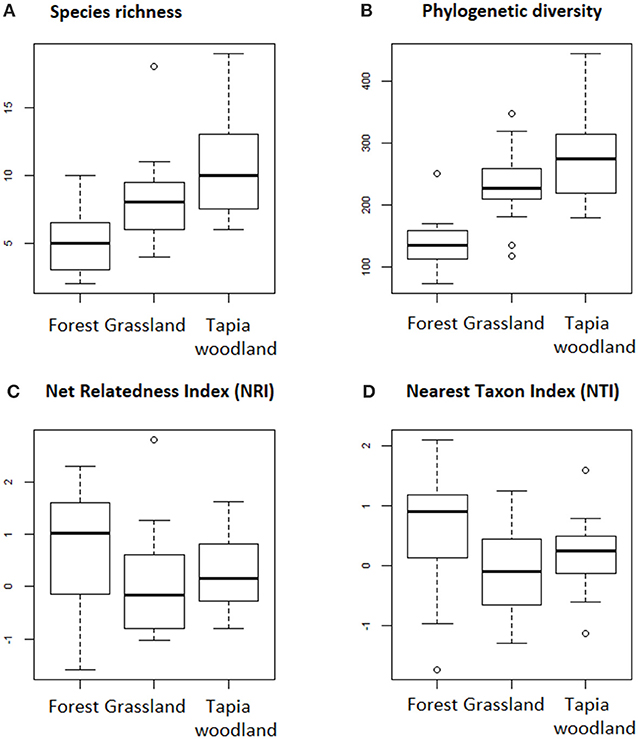
Figure 4. Boxplot of (A) species richness, (B) phylogenetic diversity, (C) Net Relatedness Index (NRI), and (D) Nearest Taxon Index (NTI) of grass species in forest, grassland and tapia woodland sites. The numbers of sites per vegetation type are respectively 12, 24, and 20. Species richness differed significantly between the three vegetation type (GLM, P = 0.001). There are significant differences between the phylogenetic diversity of the three habitats and between each pairs of habitat (GLM, P < 0.001 for each). There are no significant differences between the three vegetation types NRI (ANOVA, P = 0.09), grassland and tapia woodland NTI (ANOVA, P = 0.145) and tapia woodland and forest NTI (ANOVA, P = 0.205). The only significant difference is between grassland and forest NTI (ANOVA, P = 0.02).
Woodland and Forest Trees Traits
The three vegetation types also differ in terms of woody species composition and traits (Table 1). While there are no woody species in grassland, the woodland is dominated by Uapaca bojeri, associated with other woody species, especially Sarcolaena oblongifolia and Asteropeia densiflora. Tapia trees are shorter (8–12 m; Kull, 2002) than humid forest trees. Humid forest canopy height can reach 30–35 m at low to mid-elevations (Moat and Smith, 2007). Moreover, forest trees have wide and dense canopies, creating a low-light understorey. Woodland on the other hand is characterized by a high-light understorey as trees have open crowns which allow light to penetrate.
Uapaca bojeri has significantly thicker bark than the forest species (t = −16.5, P < 0.001; Figure 5). Among the forest species, five samples were from the same genus Uapaca (two U. ferruginea Leandri and three U. densifolia). The bark of U. bojeri (13.5%) is at least twice thicker that in forest Uapaca species (6.15% in U. ferruginea; see Supplementary Table 3).
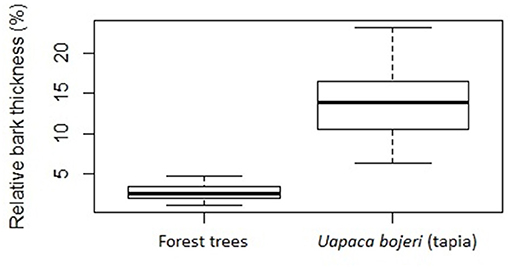
Figure 5. Boxplot of the relative bark thickness (bark thickness/tree diameter) for 39 individuals of Uapaca bojeri trees from tapia woodland and 27 individuals of dominant forest trees from 16 species. Differences are significant (t-test, t = −16.494, P < 0.001).
Discussion
The environmental envelopes of the three vegetation types show that grassland and woodland are most likely to co-occur, while forest has a distinct climatic envelope. Forest occurs at temperature ranging from 13 to 15°C and precipitation ranging from 1,810 to 2,660 mm, whereas grassland and woodland occur at temperature ranging from 18 to 25°C and precipitation ranging from 765 to 1,610 mm (Supplementary Figures 1, 2). An independent assessment based on herbarium records along environmental variables yielded an extremely similar environmental distribution of the Uapaca bojeri dominated woodland (Werkmeister, 2016). Werkmeister (2016) showed that annual temperature and mean annual precipitation are important factors in shaping the woodland's modern distribution, and the overall ranges of the woodland's environmental envelopes (temperature ranging from 15,1 to 25.1°C and precipitation ranging from 731 to 1,576 mm) coincide with our results of its occurrence probability. Our results on grassland and woodland distribution in Madagascar are similar to the distribution of savannas on the African continent where mean annual rainfall and rainfall seasonality are the key environmental correlates (Lehmann et al., 2011). The fire regime of the central highland grasslands has a return time of one to 3 years (Alvarado et al., 2018) which is in line with observations made for the African continent where the climate is similar (Lehmann et al., 2014). The locations sampled in this study span a precipitation gradient from 781 to 1,412 mm, similar to the rainfall of savannas in Africa, and corresponds to geographic regions where the three vegetation types can locally co-occur as a mosaic within the landscape. Further, in this study, we sampled 13% of the grass flora of Madagascar [71 out of 550 species (Vorontsova et al., 2016)] and these are taxonomically representative of the central highlands. Hence, the divergence we found between the diversity and functional traits of grass species in woodland and grassland in comparison to forest (Figures 2, 3) are representative of community composition and biogeographic patterns of these vegetation types across the region. Our analyses identified two distinct functional groups, that of grassland and woodland species vs. forest species defined by differentiation in architecture, physiology, and leaf traits, all of which are related to light interception, fire tolerance and flammability (Figure 3). The ground layer of Uapaca bojeri dominated woodland is characterized by grasses of the tribes Andropogoneae and Tristachyideae (Supplementary Figure 3), the same that characterize central Madagascar grasslands. Studies have shown that the tribe Andropogoneae exhibits a suite of functional traits that are advantageous in regularly burned grasslands (Everson et al., 1988; Morgan and Lunt, 1999; Bond et al., 2003b; Spasojevic et al., 2010; Forrestel et al., 2014; Simpson et al., 2016). The majority of grass species found in woodlands and grasslands are tall perennial C4 grasses, with coriaceous leaves, that burn frequently (Figure 3).
Grass species in forest and woodland showed a pattern of higher dispersion in the functional trait space than in grassland. This indicates a greater environmental heterogeneity in these vegetation types, where species exhibit a wide range of traits to adapt to their habitat (Anderson et al., 2006). Several studies have shown that high light intensity and high temperature environments favor C4 over C3 photosynthesis (Ehleringer et al., 1997; Osborne and Sack, 2012), and C4 grasses dominant in tropical savannas and grasslands (Sage and Monson, 1998; Bond, 2008). C4 grasses which dominate the woodland generally have coriaceous leaves, linked to traits that retard decomposition (Street et al., 1980) and increase flammability such as high leaf C:N ratios (D'Antonio and Vitousek, 1992; Mouillot and Field, 2005; Bond, 2008; Cardoso et al., 2008). These species are also tall grasses, promoting fire and making the vegetation highly flammable (Fensham et al., 2003; Vigilante et al., 2004; Archibald et al., 2005; Simpson et al., 2016) and are also strong light competitors (Olff and Ritchie, 1998). In contrast, grass species in the forest understory are essentially C3 members of the tribe Paniceae (Supplementary Figure 3). Forest understories are characterized by high moisture and low light conditions (Hoffmann et al., 2003, 2012), and forest grasses are largely annual, creeping, with wide leaves that enable them to optimize light interception (Milla and Reich, 2007) and minimize self-shading (Falster and Westoby, 2003). Leaf size has been demonstrated to be related to light acquisition. A significant positive relationship between leaf size and specific leaf area has been reported (Ackerly and Reich, 1999); and high specific leaf area has been shown to allow species to optimize light interception in shady conditions (Milla and Reich, 2007). A study from a woodland community in Australia has shown that small-leaved woody species were associated with more light in the understorey (Bragg and Westoby, 2002). In contrast, high-light environments of grassland and woodland are dominated by perennial tufted grasses with erect culms. With an erect architecture, these species can protect their meristematic tissue from fire damage with intravaginal buds protected within basal leaf sheaths or underground, and tillers tightly clustered (Linder et al., 2018). Thus, after fire, such species rapidly resprout (Ripley et al., 2015), and proliferate under the disturbance regime that characterizes grassland and woodlands, where humans have limited influence on the fire regimes (Alvarado et al., 2018).
Tapia woodland grass species have higher species richness and phylogenetic diversity compared to grassland and forest species (Figures 4A,B). This could be explained by the environmental gradient within the woodland promoted by heterogeneity in tree cover, enabling co-existence of a higher diversity of grass species (Silvertown, 2004) than in grassland or forest. There is an overall greater range of light environments within the woodland. Like grassland, woodland burns frequently, but the forests rarely burn (Ratnam et al., 2011; Charles-Dominique et al., 2015). Our analyses of community phylogenetic structure found evidence of evenness in grassland, slight clustering in woodland and clustering in forest. In forest, ground layer composition must be adapted to light availability (Charles-Dominique et al., 2015), potentially leading to phylogenetically conserved traits at local spatial scales. Here, most grass species found in forest belong to the “Madagascar shade clade” in the tribe Paniceae (Hackel et al., 2018) and share similar physiological and biological traits (creeping C3 species with herbaceous leaves). In contrast, it has been suggested that competitive exclusion plays a structuring role if co-occurring species are less closely related than expected by chance (Weiher and Keddy, 1995; Webb, 2000). Grassland and woodland grass species are evenly distributed across the phylogeny, and these communities are characterized by competition for resources and regular disturbance (Bond, 2008). No significant difference was found between the phylogenetic distance to the nearest taxon for each taxon in the sample (NTI) between grassland and woodland; and woodland and forest. The inconsistency in the differences of taxa distribution in each vegetation type relative to the whole species pool might be explained by the number of species in our sample. It is important to note that accounting for geographical scale and species abundance are important to draw strong conclusions from phylogenetic community structure (Vamosi et al., 2009). The differences in forest and woodland phylogenetic structure suggest the species characterizing these environments evolved independently and based on Vorontsova et al. (2016), it appears that both grassland and woodland communities have a long evolutionary history. Hackel et al. (2018) demonstrated that the “Madagascar shade clade” diversified in Madagascar and nearby islands since the Miocene and that endemic C4 grasses are the result of repeated dispersals to Madagascar mainly since the Late Miocene. It is likely that immigrating C4 grasses already featured traits similar to those we observed, but further study and comparison with their non-Malagasy relatives is needed.
Our assessment of the grass community composition and their characteristics in grassland, woodland and forest shows that the woodland formation dominated by Uapaca bojeri is more likely a type of savanna and has been previously wrongly attributed as forest (Perrier de la Bâthie, 1921; Humbert, 1949; Koechlin et al., 1974; Gade, 1996). Our results support previous studies which distinguished forest and savanna based on tree functional traits and diversity (Ratnam et al., 2011; Dantas et al., 2013; Charles-Dominique et al., 2015). Uapaca bojeri, the dominant tree species in woodland does not occur in forest as defined here and our comparative analysis of bark thickness reinforces this conclusion. Uapaca bojeri (tapia) has bark at least twice the thickness of nearby forest species. Bark thickness is often presented as a key mechanism for tree survival in frequently burnt ecosystems (Gignoux et al., 1997; Hoffmann et al., 2003; Lawes et al., 2013; Pausas, 2015) where thick bark reduces the probability of stem death and insulates buds buried in stems (Hoffmann et al., 2003; Pausas, 2015). In addition, Uapaca bojeri has other traits characteristic of pyrophytic species (Table 1), including fire retardant leaves and epicormic resprouting (Kuhnholtz-Lordat, 1938). Our results support Kull's (2002) assertion that Uapaca bojeri dominated woodlands are shaped and maintained by fire, suggesting this woodland is a savanna rather than degraded forest. However, comparison of multiple tree traits related to fire resistance, tolerance and recruitment is needed. Previous research has found differences in specific leaf area and leaf:stem allocation patterns in tree species across savanna and forest boundaries (Hoffmann and Franco, 2003) and such trait comparisons along with assessments of conditions of plant recruitment could help further elucidate the functional ecology of these woodlands linked to the functional ecology of the ground layer. These woodlands, with numerous endemic species in both the over- and under-story, are part of the cultural landscape of Madagascar and have therefore undergone changes linked to wood collection and human use (Kull, 2002). Developing effective land management and conservation strategies of the unique and endemic flora of Madagascar requires development of an understanding of the functional characteristics of the species and vegetation types. Our study provides the first assessment of grass species composition and functional traits as being distinct between forest and the mosaic of grassland and Uapaca bojeri dominated woodlands, suggesting that functional characteristics of the ground layer can help delimit functionally distinct ecosystems. Further, the grasses of both grassland and woodland are phylogenetically highly diverse, consistent with our hypothesis that the evolutionary history of these ecosystems is certainly far older that human settlement which occurred only 6,000 years ago on Madagascar such that these woodlands of the central highlands are an ancient feature of these landscapes.
Author Contributions
CS, MV, and CL designed research. CS, MV, JH, GB, JW, and CL collected data. CS and JH analyzed data. CS, MV, and CL wrote the paper. JH, GB, SC, JW and VJ contributed to the interpretation and the revision of the work.
Funding
CS was supported by KMCC and CERL by KMCC and University of Edinburgh. JH and GB received support from the University of Toulouse III through an Idex UNITI mobility grant (RECAC15EX110; UPS) and a DREIC project (VSR28AFRIQ; UPS). They are also supported by the French excellence project Labex TULIP (ANR-10-LABX-0041).
Conflict of Interest Statement
The authors declare that the research was conducted in the absence of any commercial or financial relationships that could be construed as a potential conflict of interest.
Acknowledgments
We thank T. Ramananantoandro and R. N. Razafinarivo from the forestry department of Antananarivo University for providing wood samples; British Ecological Society Travel Grant to Ecology Across Borders 2017 which enabled CLS to attend and present a poster at the conference; Madagascar National Parks (MNP), Direction générale des forêts (DGF) and Parc Botanique et Zoologique de Tsimbazaza (PBZT) for granting research permits, Dr. Tendro Tondrasoa (University of Aberdeen), Jan Kim (RBG Kew), and Tim Wilkinson (RBG Kew) for helping with data analysis, all Kew Madagascar Conservation Centre (KMCC) individual staff for support with permits and field trip, and all local community managers we worked with.
Supplementary Material
The Supplementary Material for this article can be found online at: https://www.frontiersin.org/articles/10.3389/fevo.2018.00184/full#supplementary-material
References
Ackerly, D. D., and Reich, P. B. (1999). Convergence and correlations among leaf size and function in seed plants: a comparative test using independent contrasts. Am. J. Bot. 86, 1272–1281. doi: 10.2307/2656775
Alvarado, S. T., Silva, T. S. F., and Archibald, S. (2018). Management impacts on fire occurrence: a comparison of fire regimes of African and South American tropical savannas in different protected areas. J. Environ. Man. 218, 79–87. doi: 10.1016/j.jenvman.2018.04.004
Anderson, M. J., Ellingsen, K. E., and McArdle, B. H. (2006). Multivariate dispersion as a measure of beta diversity. Ecol. Lett. 9, 683–693. doi: 10.1111/j.1461-0248.2006.00926.x
Archibald, S., Bond, W. J., Stock, W. D., and Fairbanks, D. H. K. (2005). Shaping the landscape: fire–grazer interactions in an African savanna. Ecol. Appl. 15, 96–109. doi: 10.1890/03-5210
Archibald, S., and Hempson, G. P. (2016). Competing consumers: contrasting the patterns and impacts of fire and mammalian herbivory in Africa. Philos. Trans. R. Soc. Lond. B Biol. Sci. 371:20150309. doi: 10.1098/rstb.2015.0309
Beentje, H. J. (2010). The Kew Plant Glossary: An Illustrated Dictionary of Plant Terms. London: Kew Publishing, Royal Botanic Gardens.
Bond, W. J. (2005). Large parts of the world are brown or black: a different view on the ‘Green World' hypothesis. J. Veg. Sci. 16, 261–266. doi: 10.1111/j.1654-1103.2005.tb02364.x
Bond, W. J. (2008). What limits trees in C4 grasslands and savannas? Annu. Rev. Ecol. Syst. 39, 641–659. doi: 10.1146/annurev.ecolsys.39.110707.173411
Bond, W. J., Midgley, G. F., and Woodward, F. I. (2003a). The importance of low atmospheric CO2 and fire in promoting the spread of grasslands and savannas. Glob. Change Biol. 9, 973–982. doi: 10.1046/j.1365-2486.2003.00577.x
Bond, W. J., Midgley, G. F., and Woodward, F. I. (2003b). What controls South African vegetation-climate or fire? S. Afr. J. Bot. 69, 79–91. doi: 10.1016/S0254-6299(15)30362-8
Bond, W. J., and Parr, C. L. (2010). Beyond the forest edge: ecology, diversity and conservation of the grassy biomes. Biol. Conserv. 143, 2395–2404. doi: 10.1016/j.biocon.2009.12.012
Bond, W. J., Silander, J. A., Ranaivonasy, J., and Ratsirarson, J. (2008). The antiquity of Madagascar's grasslands and the rise of C4 grassy biomes. J. Biogeogr. 35, 1743–1758. doi: 10.1111/j.1365-2699.2008.01923.x1743
Bosser, J. (1969). Graminées des Pâturages et des Cultures à Madagascar. Paris: Office de la Recherche Scientifique et Technique Outre-Mer.
Bragg, J. G., and Westoby, M. (2002). Leaf size and foraging for light in a sclerophyll woodland. Funct. Ecol. 16, 633–639. doi: 10.1046/j.1365-2435.2002.00661.x
Brejda, J. J., Moser, L. E., and Waller, S. S. (1989). “Rhizome and tiller development of three Nebraska Sandhills warm-season grasses,” Proceedings of the Eleventh North American Prairie Conference. 211–215.
Bremond, L., Boom, A., and Favier, C. (2012). Neotropical C3/C4 grass distributions–present, past and future. Glob. Change Biol. 18, 2324–2334. doi: 10.1111/j.1365-2486.2012.02690.x
Cabido, M., Ateca, N., Astegiano, M., and Anton, A. (1997). Distribution of C3 and C4 grasses along an altitudinal gradient in Central Argentina. J. Biogeogr. 24, 197–204. doi: 10.1046/j.1365-2699.1997.00085.x
Cardoso, M. F., Nobre, C. A., Lapola, D. M., Oyama, M. D., and Sampaio, G. (2008). Long-term potential for fires in estimates of the occurrence of savannas in the tropics. Glob. Ecol. Biogeogr. 17, 222–235. doi: 10.1111/j.1466-8238.2007.00356.x
Charles-Dominique, T., Staver, A. C., Midgley, G. F., and Bond, W. J. (2015). Functional differentiation of biomes in an African savanna/forest mosaic. S. Afr. J. Bot. 101, 82–90. doi: 10.1016/j.sajb.2015.05.005
Clayton, W. D. (1970). Flora of Tropical East Africa. Gramineae (Part 1). London: Crown Agents for Oversea Governments and Administrations.
Clayton, W. D., Vorontsova, M. S., Harman, K. T., and Williamson, H. (2006). GrassBase—The Online World Grass Flora. Available online at: http://www.kew.org/data/grasses-db.html (Accessed September 20, 2018).
Dantas, V. de L., Batalha, M. A., and Pausas, J. G. (2013). Fire drives functional thresholds on the savanna-forest transition. Ecology 94, 2454–2463. doi: 10.1890/12-1629.1
D'Antonio, C. M., and Vitousek, P. M. (1992). Biological invasions by exotic grasses, the grass/ fire cycle, and global change. Annu. Rev. Ecol. Syst. 23, 63–87.
Edwards, E. J., Osborne, C. P., Strömberg, C. A. E., and Smith, S. A. C4 Grasses Consortium (2010). The origins of C4 grasslands: integrating evolutionary and ecosystem science. Science 328, 587–591. doi: 10.1126/science.1177216
Ehleringer, J. R., Cerling, T. E., and Helliker, B. R. (1997). C4 photosynthesis, atmospheric CO2, and climate. Oecologia 112, 285–299. doi: 10.1007/s004420050311
Everson, C. S., Everson, T. M., and Tainton, N. M. (1988). Effects of intensity and height of shading on the tiller initiation of 6 grass species from the highland sourveld of Natal. S. Afr. J. Bot. 54, 315–318. doi: 10.1016/S0254-6299(16)31297-2
Faith, D. P. (1992). Conservation evaluation and phylogenetic diversity. Biol. Conserv. 61, 1–10. doi: 10.1016/0006-3207(92)91201-3
Falster, D. S., and Westoby, M. (2003). Leaf size and angle vary widely across species: what consequences for light interception? New Phytol. 158, 509–525. doi: 10.1046/j.1469-8137.2003.00765.x
Faramalala, M. H. (1995). Formations Végétales et Domaine Forestier National de Madagascar. 1: 1,000,000 Color Map. Conservation International (CI), Direction des Eaux et Forêts.
Fensham, R. J., Fairfax, R. J., Butler, D. W., and Bowman, D. M. (2003). Effects of fire and drought in a tropical eucalypt savanna colonized by rain forest. J. Biogeogr. 30, 1405–1414. doi: 10.1046/j.1365-2699.2003.00934.x
Fick, S. E., and Hijmans, R. J. (2017). Worldclim 2: new 1-km spatial resolution climate surfaces for global land areas. Int. J. Climatol. 37, 4302–4315. doi: 10.1002/joc.5086
Forrestel, E. J., Donoghue, M. J., and Smith, M. D. (2014). Convergent phylogenetic and functional responses to altered fire regimes in mesic savanna grasslands of North America and South Africa. New Phytol. 203, 1000–1011. doi: 10.1111/nph.12846
Forrestel, E. J., Donoghue, M. J., and Smith, M. D. (2015). Functional differences between dominant grasses drive divergent responses to large herbivore loss in mesic savanna grasslands of North America and South Africa. J. Ecol. 103, 714–724. doi: 10.1111/1365-2745.12376
Gade, D. W. (1996). Deforestation and its effects in highland Madagascar. Mount. Res. Dev. 16, 101–116. doi: 10.2307/3674005
Gignoux, J., Clobert, J., and Menaut, J. C. (1997). Alternative fire resistance strategies in savanna trees. Oecologia 110, 576–583. doi: 10.1007/s004420050198
Gower, J. C. (1971). A general coefficient of similarity and some of its properties. Biometrics 27, 857–871. doi: 10.2307/2528823
Griffith, D. M., Lehmann, C. E. R., Strömberg, C. A., Parr, C. L., Pennington, R. T., Sankaran, M., et al. (2017). Comment on “The extent of forest in dryland biomes”. Science 358:eaao1309. doi: 10.1126/science.aao1309
Grime, J. P. (1977). Evidence for the existence of three primary strategies in plants and its relevance to ecological and evolutionary theory. Am. Nat. 111, 1169–1194. doi: 10.1086/283244
Hackel, J., Vorontsova, M. S., Nanjarisoa, O. P., Hall, R. C., Razanatsoa, J., Malakasi, P., et al. (2018). Grass diversification in Madagascar: in situ radiation of two large C3 shade clades and support for a Miocene to Pliocene origin of C4 grassy biomes. J. Biogeogr. 45, 750–761. doi: 10.1111/jbi.13147
Hoffmann, W. A., and Franco, A. C. (2003). Comparative growth analysis of tropical forest and savanna woody plants using phylogenetically independent contrasts. J. Ecol. 91, 475–484. doi: 10.1046/j.1365-2745.2003.00777.x
Hoffmann, W. A., Franco, A. C., Moreira, M. Z., and Haridasan, M. (2005). Specific leaf area explains differences in leaf traits between congeneric savanna and forest trees. Funct. Ecol. 19, 932–940. doi: 10.1111/j.1365-2435.2005.01045.x
Hoffmann, W. A., Geiger, E. L., Gotsch, S. G., Rossatto, D. R., Silva, L. C. R., Lau, O. N., et al. (2012). Ecological thresholds at the savanna–forest boundary: how plant traits, resources and fire govern the distribution of tropical biomes. Ecol. Lett. 15, 759–768. doi: 10.1111/j.1461-0248.2012.01789.x
Hoffmann, W. A., Orthen, B., and Kielse Vargas Do Nascimento, P. (2003). Comparative fire ecology of tropical savanna and forest trees. Funct. Ecol. 17, 720–726. doi: 10.1111/j.1365-2435.2003.00796.x
House, J. I., Archer, S., Breshears, D. D., Scholes, R. J., Coughenour, M. B., Dodd, M. B., et al. (2003). Conundrums in mixed woody-herbaceous plant systems. J. Biogeogr. 30, 1763–1777. doi: 10.1046/j.1365-2699.2003.00873.x
House, J. I., and Hall, D. O. (2001). “Productivity of tropical savannas and grasslands,” in Terrestrial Global Productivity, eds J. Roy, B. Saugier, and H. A. Mooney (New York, NY: Academic Press), 363–400.
Humbert, H. (1927). Destruction d'une flore insulaire par le feu: principaux aspects de la végétation à Madagascar. Mem. Acad. Malgache 5, 1–80.
Kembel, S. W., Cowan, P. D., Helmus, M. R., Cornwell, W. K., Morlon, H., Ackerly, D. D., et al. (2010). Picante: R tools for integrating phylogenies and ecology. Bioinformatics 26, 1463–1464. doi: 10.1093/bioinformatics/btq166
Kew Madagascar Conservation Centre (KMCC) (2012). Plan d'Aménagement et de Gestion de la Nouvelle Aire Protégée du Massif d'Itremo-Ambatofinandrahana, Region Amoron'I Mania, Madagascar. Antananarivo: KMCC.
Kindt, R., and Coe, R. (2005). Tree Diversity Analysis: A Manual and Software for Common Statistical Methods for Ecological and Biodiversity Studies. Nairobi: World Agroforestry Centre (ICRAF).
Knapp, A. K., Briggs, J. M., Hartnett, D. C., and Collins, S. L. (1998). Grassland Dynamics: Long-Term Ecological Research in Tallgrass Prairie. New York, NY: Oxford University Press.
Koechlin, J., Guillaumet, J. L., and Morat, P. (1974). Flore et Végétation de Madagascar. ed J. Cramer (Vaduz: Gantner Verlag), 701.
Kuhnholtz-Lordat, G. (1938). La Terre Incendiée: Essai D'agronomie Comparée. Nîmes: Editions de La Maison Carrée, Ateliers Brugier.
Kull, C. A. (2002). The “degraded” tapia woodlands of highland Madagascar: rural economy, fire ecology, and forest conservation. J. Cult. Geogr. 19, 95–128. doi: 10.1080/08873630209478290
Laliberté, E., and Legendre, P. (2010). A distance-based framework for measuring functional diversity from multiple traits. Ecology 91, 299–305. doi: 10.1890/08-2244.1
Laliberté, E., Legendre, P., and Shipley, B. (2014). FD: Measuring Functional Diversity from Multiple Traits, and Other Tools for Functional Ecology. R package version 1.0-12. (Accessed June 3, 2018).
Lattanzi, F. A. (2010). “C3/C4 grasslands and climate change,” in Proceedings of the 23rd General Meeting of the European Grassland Federation, Kiel, Germany, 29th August−2nd September 2010 (Duderstadt: Mecke Druck und Verlag), 3–13.
Lawes, M. J., Adie, H., Russell-Smith, J., Murphy, B., and Midgley, J. J. (2011). How do small savanna trees avoid stem mortality by fire? The roles of stem diameter, height and bark thickness. Ecosphere 2, 1–13. doi: 10.1890/ES10-00204.1
Lawes, M. J., Midgley, J. J., and Clarke, P. J. (2013). Costs and benefits of relative bark thickness in relation to fire damage: a savanna/forest contrast. J. Ecol. 101, 517–524. doi: 10.1111/1365-2745.12035
Lehmann, C. E., Anderson, T. M., Sankaran, M., Higgins, S. I., Archibald, S., Hoffmann, W. A., et al. (2014). Savanna vegetation-fire-climate relationships differ among continents. Science 343, 548–552. doi: 10.1126/science.1247355
Lehmann, C. E., Archibald, S. A., and Bond, W. J. (2011). Deciphering the distribution of the savanna biome. New Phytol. 191, 197–209. doi: 10.1111/j.1469-8137.2011.03689.x
Lehmann, C. E., and Parr, C. L. (2016). Tropical grassy biomes: linking ecology, human use and conservation. Phil. Trans. R. Soc. B Biol. Sci. 371:20160329. doi: 10.1098/rstb.2016.0329
Lehmann, C. E., Ratnam, J., and Hutley, L. B. (2009). Which of these continents is not like the other? Comparisons of tropical savanna systems: key questions and challenges. New Phytol. 181, 508–511. doi: 10.1111/j.1469-8137.2009.02734.x
Linder, H. P., Lehmann, C. E. R., Archibald, S. A., Osborne, C. P., and Richardson, D. M. (2018). Global grass (Poaceae) success underpinned by traits facilitating colonization, persistence and habitat transformation. Biol. Rev. 93, 1125–1144. doi: 10.1111/brv.12388
Lowry, P. P, Schatz, G. E., and Phillipson, P. B. (1997). The Classification of Natural and Anthropogenic Vegetation in Madagascar, in Natural Change and Human Impact in Madagascar. Washington, DC: Smithsonian Institution, 113–114.
Milla, R., and Reich, P. B. (2007). The scaling of leaf area and mass: the cost of light interception increases with leaf size. Proc. R. Soc. B Biol. Sci. 274, 2109–2115. doi: 10.1098/rspb.2007.0417
Moat, J., and Smith, P. (2007). Atlas of the Vegetation of Madagascar. London: Royal Botanic Gardens.
Morgan, J. W., and Lunt, I. D. (1999). Effects of time-since-fire on the tussock dynamics of a dominant grass (Themeda triandra) in a temperate Australian grassland. Biol. Conserv. 88, 379–386. doi: 10.1016/S0006-3207(98)00112-8
Mouillot, F., and Field, C. B. (2005). Fire history and the global carbon budget: a 1 x 1 fire history reconstruction for the 20th century. Glob. Change Biol. 11, 398–420. doi: 10.1111/j.1365-2486.2005.00920.x
Nanjarisoa, O. P., Besnard, G., Ralimanana, H., Jeannoda, V., and Vorontsova, M. S. (2017). Grass survey of the Itremo Massif records endemic central highland grasses. Madagascar Conserv. Dev. 12, 34–40. doi: 10.4314/mcd.v12i1.6
Oksanen, J., Blanchet, F. G., Friendly, M., Kindt, R., Legendre, P., McGlinn, D., et al. (2016). vegan: Community Ecology Package. R Package Version 2.4-1. Available online at: https://CRAN.R-project.org/package=vegan
Olff, H., and Ritchie, M. E. (1998). Effects of herbivores on grassland plant diversity. Trends Ecol. Evol. 13, 261–265. doi: 10.1016/S0169-5347(98)01364-0
Osborne, C. P., and Sack, L. (2012). Evolution of C4 plants: a new hypothesis for an interaction of CO2 and water relations mediated by plant hydraulics. Philos. Trans. R. Soc. Lond. B Biol. Sci. 367, 583–600. doi: 10.1098/rstb.2011.0261
Osborne, C. P., Salomaa, A., Kluyver, T. A., Visser, V., Kellogg, E. A., Morrone, O., et al. (2014). A global database of C4 photosynthesis in grasses. New Phytol. 204, 441–446. doi: 10.1111/nph.12942
Parr, C. L., Lehmann, C. E. R., Bond, W. J., Hoffmann, W. A., and Andersen, A. N. (2014). Tropical grassy biomes: misunderstood, neglected, and under threat. Trends Ecol. Evol. 29, 205–213. doi: 10.1016/j.tree.2014.02.004
Pausas, J. G. (2015). Bark thickness and fire regime. Funct. Ecol. 29, 315–327. doi: 10.1111/1365-2435.12372
Pierron, D., Heiske, M., Razafindrazaka, H., Rakoto, I., Rabetokotany, N., Ravololomanga, B., et al. (2017). Genomic landscape of human diversity across Madagascar. Proc. Natl. Acad. Sci. U.S.A. 114, E6498–E6506. doi: 10.1073/pnas.1704906114
Pitelka, L. F. (1977). Energy allocation in annual and perennial lupines (Lupinus: Leguminosae). Ecology 58, 1055–1065. doi: 10.2307/1936925
R Core Team (2013). R: A Language and Environment for Statistical Computing. Vienna. Available online at: https://www.R-project.org/
Rajeriarison, C., and Faramalala, M. H. (1999). Nomenclature des Formations Végétales de Madagascar. Antananarivo: ANGAP.
Ratnam, J., Bond, W. J., Fensham, R. J., Hoffmann, W. A., Archibald, S., Lehmann, C. E., et al. (2011). When is a ‘forest' a savanna, and why does it matter? Glob. Ecol. Biogeogr. 20, 653–660. doi: 10.1111/j.1466-8238.2010.00634.x
Ripley, B., Visser, V., Christin, P. A., Archibald, S., Martin, T., and Osborne, C. (2015). Fire ecology of C3 and C4 grasses depends on evolutionary history and frequency of burning but not photosynthetic type. Ecology 96, 2679–2691. doi: 10.1890/14-1495.1
Schimper, A. F. W., and Fisher, W. R. (1903). Plant-Geography Upon a Physiological Basis. Vol. 2. Oxford: Clarendon Press.
Scholes, R. J., and Archer, S. R. (1997). Tree–grass interactions in savannas. Annu. Rev. Ecol. Evol. Syst. 28, 517–544. doi: 10.1146/annurev.ecolsys.28.1.517
Scholes, R. J., and Hall, D. O. (1996). The Carbon Budget of Tropical Savannas, Woodlands and Grasslands. SCOPE-Scientific Committee on Problems of the Environment International Council of Scientific Unions.
Silvertown, J. (2004). Plant coexistence and the niche. Trends Ecol. Evol. 19, 605–611. doi: 10.1016/j.tree.2004.09.003
Simpson, K. J., Ripley, B. S., Christin, P. A., Belcher, C. M., Lehmann, C. E. R., Thomas, G. H., et al. (2016). Determinants of flammability in savanna grass species. J. Ecol. 104, 138–148. doi: 10.1111/1365-2745.12503
Spasojevic, M. J., Aicher, R. J., Koch, G. R., Marquardt, E. S., Mirotchnick, N., Troxler, T. G., et al. (2010). Fire and grazing in a mesic tallgrass prairie: impacts on plant species and functional traits. Ecology 91, 1651–1659. doi: 10.1890/09-0431.1
Street, J. R., Henderlong, P. R., and Himes, F. L. (1980). “Influence of silica on chemical composition and decomposition of turfgrass tissue,” in Proceedings of the Third International Turfgrass Research Conference (Wisconsin: American Society of Agronomy, Crop Science Society of America, Soil Science Society of America), 329–336.
Vamosi, S. M., Heard, S. B., Vamosi, J. C., and Webb, C. O. (2009). Emerging patterns in the comparative analysis of phylogenetic community structure. Mol. Ecol. 18, 572–592. doi: 10.1111/j.1365-294X.2008.04001.x
Vigilante, T., Bowman, D. M., Fisher, R., Russell-Smith, J., and Yates, C. (2004). Contemporary landscape burning patterns in the far North Kimberley region of north-west Australia: human influences and environmental determinants. J. Biogeogr. 31, 1317–1333.doi: 10.1111/j.1365-2699.2004.01104.x
Vorontsova, M. S., Besnard, G., Forest, F., Malakasi, P., Moat, J., Clayton, W. D., et al. (2016). Madagascar's grasses and grasslands: anthropogenic or natural? Proc. R. Soc. B Biol. Sci. 283:20152. doi: 10.1098/rspb.2015.2262
Webb, C. O. (2000). Exploring the phylogenetic structure of eco-logical communities: an example for rainforest trees. Am. Nat. 156, 145–155. doi: 10.1086/303378
Webb, C. O., Ackerly, D. D., McPeek, M. A., and Donoghue, M. J. (2002). Phylogenies and community ecology. Annu. Rev. Ecol. Evol. Syst. 33, 475–505. doi: 10.1146/annurev.ecolsys.33.010802.150448
Weiher, E., and Keddy, P. (1995). Assembly rules, null models, and trait dispersion: new questions from old patterns. Oikos 74, 159–164. doi: 10.2307/3545686
Werkmeister, G. (2016). MaxEnt Modelling of the Distribution and Environmental Constraints of Endemic Tree Species Uapaca bojeri (tapia) in Madagascar. Master's thesis, Queen Mary University of London.
Werner, P. A., and Murphy, P. G. (2001). Size-specific biomass allocation and water content of above- and below-ground components of three Eucalyptus species in a northern Australian savanna. Aust. J. Bot. 49, 155–167. doi: 10.1071/BT99026
White, F. (1983). The Vegetation of Africa, Natural Resources Research n° 20. A Descriptive Memoir to Accompany UNESCO/AETFAT/UNSO Vegetation Map of Africa. Paris: UNESCO.
White, R., Murray, S., and Rohweder, M. (2000). Grassland Ecosystems. Washington, DC: World Resources Institute.
Whittaker, R. H. (1972). Evolution and measurement of species diversity. Taxon 21, 213–251. doi: 10.2307/1218190
Keywords: functional traits, species diversity, grassland, Poaceae, woodland, forest, savanna, Uapaca bojeri
Citation: Solofondranohatra CL, Vorontsova MS, Hackel J, Besnard G, Cable S, Williams J, Jeannoda V and Lehmann CER (2018) Grass Functional Traits Differentiate Forest and Savanna in the Madagascar Central Highlands. Front. Ecol. Evol. 6:184. doi: 10.3389/fevo.2018.00184
Received: 11 January 2018; Accepted: 23 October 2018;
Published: 15 November 2018.
Edited by:
Daniel M. Griffith, Oregon State University, United StatesReviewed by:
Zehao Shen, Peking University, ChinaDeusdedith M. Rugemalila, Wake Forest University, United States
Copyright © 2018 Solofondranohatra, Vorontsova, Hackel, Besnard, Cable, Williams, Jeannoda and Lehmann. This is an open-access article distributed under the terms of the Creative Commons Attribution License (CC BY). The use, distribution or reproduction in other forums is permitted, provided the original author(s) and the copyright owner(s) are credited and that the original publication in this journal is cited, in accordance with accepted academic practice. No use, distribution or reproduction is permitted which does not comply with these terms.
*Correspondence: Cédrique L. Solofondranohatra, lovacedrique@gmail.com