- 1Limnological Institute, University of Konstanz, Konstanz, Germany
- 2Theoretical Aquatic Ecology and Ecophysiology, Institute for Biochemistry and Biology, University of Potsdam, Potsdam, Germany
Sex-specific differences in nutritional requirements may crucially influence the performances of the sexes, which may have implications for sexual reproduction and thus is of great ecological and evolutionary interest. In the freshwater model species Daphnia magna, essential lipid requirements have been extensively studied. Dietary deficiencies in sterols and polyunsaturated fatty acids (PUFA) have been shown to constrain somatic growth and parthenogenetic reproduction of female Daphnia. In contrast, nutrient requirements of male Daphnia have not been studied yet. Supplementation experiments were conducted to investigate differences in sterol (cholesterol) and PUFA (eicosapentaenoic acid, EPA) requirements between female and male D. magna. Thresholds for sterol-limited juvenile growth were higher in females than in males, suggesting that females are more susceptible to dietary sterol deficiencies than males. Sex-specific differences in maximum somatic growth rates were evident primarily in the presence of dietary EPA; females could not exploit their generally higher growth potential in the absence of dietary PUFA. However, the thresholds for EPA-limited growth did not differ between sexes, suggesting that both sexes have similar dietary EPA requirements during juvenile growth. During a life history experiment, the gain in body dry mass was higher in females than in males, irrespective of food treatment. In both sexes, the gain in body dry mass increased significantly upon EPA supplementation, indicating that both sexes benefited from dietary EPA supply also later in life. However, the positive effects of EPA supplementation were most pronounced for female reproduction-related traits (i.e., clutch sizes, egg dry masses, and total dry mass investment in reproduction). The high maternal investment in reproduction resulted in a depletion of nutrients in female somata. In contrast, the comparatively low paternal investment in reproduction allowed for the accumulation of nutrients in male somata. We conclude that males are generally less susceptible to dietary nutrient deficiencies than females, because they can rely more on internal body stores. Our data suggest that the performances of the sexes are differentially influenced by lipid-mediated food quality, which may have consequences for sexual reproduction and thus the production of resting eggs and the maintenance of Daphnia populations.
Introduction
The sexes of gonochorous animal species are subject to different reproduction-related selection pressures. Divergent selection provides the basis for more or less distinct sexual dimorphisms in morphological, physiological or behavioral traits. Adaptive divergence can involve sex-specific differences in the molecular metabolic machinery, nutritional requirements, and feeding behavior (Lee et al., 2008; Maklakov et al., 2008; Hoffman et al., 2014; Camus et al., 2017). In some insect species, females have been shown to require higher dietary protein concentrations than males, while males seem to rely more on an adequate dietary supply with carbohydrates (Maklakov et al., 2008; Reddiex et al., 2013; Jensen et al., 2015; Camus et al., 2017). Sex-specific differences in macronutrient requirements have been attributed to the different reproductive roles of the sexes. The maternal investment in reproduction is generally much larger than the paternal investment, primarily because females invest heavily in the biochemical composition of eggs, which can make up a large fraction of the female's body mass and thus are metabolically expensive (Trivers, 1972). Eggs contain high amounts of energy storage molecules and building material, especially lipids and lipoproteins (yolk), which are required for embryonic development. In insects, high dietary protein concentrations translate into a higher number of viable eggs and thus increased female fitness (Terashima and Bownes, 2004; Bowman and Tatar, 2016). Female fitness has been proposed to be strongly limited by the rate at which resources can be converted into egg biomass (Andersson, 1994). In contrast, male investment in reproduction is commonly considered to be negligible, because sperm and seminal fluids are inexpensive to produce and lack any nutritive component (Trivers, 1972). However, recent evidence suggests that macronutrient balance and caloric restriction can exert significant paternal effects (Bonduriansky and Runagall-McNaull, 2016; Polak et al., 2017). In cockroaches, the dietary protein to carbohydrate ratio has been reported to affect sperm number but not sperm viability (Bunning et al., 2015). A diet rich in carbohydrates has been suggested to aid males in pursuing and courting females, resulting in a higher number of mating events and thus increased male fitness (South et al., 2011; Morimoto and Wigby, 2016). In general, however, it appears that females are more susceptible to inadequate nutrition, both in terms of quantity and quality, due to their higher reproductive investment.
Besides macronutrients, many animals rely on an adequate dietary supply with essential biochemicals, such as sterols and polyunsaturated fatty acids (PUFA). Dietary deficiencies in these essential lipids crucially impair growth and reproduction of zooplankton (Ahlgren et al., 1990; Müller-Navarra, 1995; Becker and Boersma, 2003; Martin-Creuzburg et al., 2009; Wacker and Martin-Creuzburg, 2012) and thus are assumed to play import roles in aquatic food web processes (Müller-Navarra et al., 2000; Wacker and von Elert, 2001; von Elert et al., 2003; Hartwich et al., 2012).
Sterol auxotrophy is widespread among invertebrates and it is generally accepted that all arthropods require a dietary source of sterols to cover their physiological demands (Goad, 1981; Behmer and Nes, 2003; Martin-Creuzburg et al., 2014). Sterols are indispensable structural components of eukaryotic cell membranes and are involved in the regulation of various membrane properties, such as permeability and fluidity (Haines, 2001; Ohvo-Rekilä et al., 2002). Cholesterol, the predominant body sterol of animals, serves as precursor for steroid hormones, such as the molt-inducing ecdysteroids in arthropods. In addition, ecdysteroids are involved in the regulation of arthropod reproduction and embryogenesis (Subramoniam, 2000; Brown et al., 2009; Mykles, 2011). Moreover, cholesterol itself has been shown to modify signaling proteins involved in the patterning of embryonic structures during development (Porter et al., 1996). Sex-specific differences in the functional role of sterols in invertebrate physiology are poorly understood.
Like sterols, PUFA are important structural components of cell membranes and are involved in the modulation of a variety of vital membrane properties (Valentine and Valentine, 2004).
In addition, C20 PUFA, such as arachidonic acid (ARA, 20:4n-6) and eicosapentaenoic acid (EPA, 20:5n-3), serve as precursors for eicosanoids, which play important roles in reproduction, ion transport, and immunity in vertebrates and invertebrates (Stanley, 2000). In a number of insect species, eicosanoids have been shown to induce egg-laying behavior and to influence ovarian protein uptake (Stanley, 2006). In invertebrates, sex-specific differences in eicosanoid-mediated physiological processes remain largely unclear. Mammalian spermatozoa are characterized by a high proportion of long-chain PUFA, mostly docosahexaenoic acid (DHA, 22:6n-3), which are believed to play crucial roles in fertilization (Esmaeili et al., 2015). In guppies, dietary restriction in n-3 PUFA has been linked to a depression in sperm competitiveness (Rahman et al., 2014). To the best of our knowledge, lipid profiles of invertebrate spermatozoa have not been studied yet, and male reproductive traits have not been linked to the dietary PUFA supply.
In the freshwater crustacean Daphnia, reproduction is characterized by an environmentally triggered alteration between asexual and sexual reproduction (cyclical parthenogenesis). Female and male offspring are produced asexually prior to sexual reproduction and thus are genetically identical (Nong et al., 2017), which allows minimizing confounding effects of genetic variation within the sexes in laboratory experiments. Sexual reproduction involves the production of resting eggs, which need to be fertilized before they are enclosed in a protective shell called an ephippium. Ephippia allow survival even during long periods of adverse environmental conditions and thus are essential to the maintenance of Daphnia populations (Decaestecker et al., 2009). The sexual dimorphism in Daphnia is primarily manifested in differences in body size, with adult females being much larger than males (Lürling and Beekman, 2006). Other morphological characteristics of male Daphnia include the longer first antennae as well as modifications at the ventral opening of the carapace.
Lipid profiles of female Daphnia have been extensively studied and related to changes in environmental conditions (Schlechtriem et al., 2006; Ravet et al., 2010; Hartwich et al., 2013). In contrast, lipid profiles of male Daphnia have not yet been studied. Sex-specific differences in lipid profiles have been found already in Drosophila (Parisi et al., 2011). The data revealed differences between sexes in saturation state in two lipid class fractions, the cholesterol ester and lysophosphatidylcholine fraction, with males containing higher amounts of saturated fatty acids and females containing higher amounts of mono- and polyunsaturated fatty acids (Parisi et al., 2011). The fatty acid composition of female Daphnia strongly reflects that of its food (Brett et al., 2006). Female Daphnia rapidly take up, accumulate and retain especially the physiologically important C20 PUFA (Taipale et al., 2011). In contrast, dietary phytosterols are mostly converted into cholesterol, the predominant body sterols in Daphnia (Martin-Creuzburg et al., 2014). Female Daphnia allocate substantial amounts of dietary PUFA into asexually and sexually produced eggs (Abrusán et al., 2007; Wacker and Martin-Creuzburg, 2007; Schlotz et al., 2013; Putman et al., 2015). Cholesterol is also allocated into the eggs but seems to be retained to a higher degree in somatic tissues (Wacker and Martin-Creuzburg, 2007).
Here, we studied sex-specific differences in essential lipid requirements in the freshwater model species Daphnia magna to improve our understanding of the effects of diet on sex-specific fitness traits. We reared female and male juvenile D. magna on dietary cholesterol and EPA gradients and compared thresholds for sterol- and PUFA-limited growth between the sexes. In addition, a life table experiment was conducted to assess the maternal dry mass and lipid investment in egg production on cholesterol and PUFA supplemented diets. In Daphnia, the paternal investment in reproduction is difficult to determine. Although there have been attempts to dissect testes and to quantify spermatozoa of male D. magna (Duneau et al., 2012; Gómez et al., 2016), it is quite difficult to obtain sufficient material for quantitative dry mass determination and subsequent biochemical analyses. A straightforward estimation of the paternal investment in reproduction-related tissues arises from comparing the total investment in male body tissues with the maternal investment in body tissues and eggs. To acquire lipid profiles of female and male somatic tissues, we dissected eggs from female brood chambers and analyzed the lipid profiles of eggs and female somata separately. We hypothesized that lipid profiles differ between sexes, especially between males and egg-bearing females. Considering that female Daphnia allocate substantial amounts of PUFA into their eggs but rather retain cholesterol in their somatic tissues (Wacker and Martin-Creuzburg, 2007), we hypothesized that female somata contain lower proportions of PUFA but higher proportions of cholesterol than their male counterparts, and that this translates into sex-specific differences in essential lipid requirements.
Material and Methods
Cultivation and Preparation of Organisms
Cohorts of a clone of Daphnia magna, originally isolated from a rock pool in Southern Finland (OER 3-3; Dieter Ebert, University of Basel), were raised in 1-L jars at 20°C in filtered lake water (0.2 μm pore-sized membrane filter) containing 2 mg C l−1 of the green alga Scenedesmus obliquus (Culture Collection of Algae, University of Göttingen, Germany, SAG 276-3a). S. obliquus was grown at 20°C in 5-L batch cultures in Cyano medium (Jüttner et al., 1983) and harvested in the late-exponential growth phase (illumination at 120 μmol quanta m−2 s−1). The animals were transferred into new jars containing freshly prepared food every other day. Male production was induced by enhancing the number of females per jar from 12 to 20 L−1 and by switching the day-night cycle from 16 h:8 h to 8 h:16 h (light:dark). During this time, daphnids were transferred to new jars 2 days after birth and then every 4 days until they released their third-clutch offspring. Experiments were conducted with third-clutch offspring born within 12 h. The sex of the offspring was determined by inspecting the length of the first antennae using a stereo-microscope. In neonatal Daphnia, the first antennae are already longer in males than in females. The sexes were gathered separately in beakers containing filtered lake water and 2 mg C L−1 of the cyanobacterium Synechococcus elongatus (juvenile growth experiments) or the green alga S. obliquus (life history experiment) from which they were randomly transferred to the experimental beakers (200 ml). S. elongatus (SAG 89.79) was used as food in the juvenile growth experiments because it is non-toxic and well-assimilated by Daphnia but lacks sterols and PUFA, resulting in a co-limitation of Daphnia by sterols and long-chain PUFA (Martin-Creuzburg et al., 2009; Sperfeld et al., 2012). S. elongatus was cultivated semi-continuously at a dilution rate of 0.25 d−1 in 5-L bottles containing Cyano medium (illumination at 80 μmol quanta m−2 s−1). S. obliquus was used as food in the life history experiment because it lacks long-chain PUFA (i.e., PUFA with more than 18 carbon atoms) but contains several phytosterols and is of moderate food quality for Daphnia (von Elert, 2002; Martin-Creuzburg and Merkel, 2016). The fecundity of Daphnia is generally higher on S. obliquus than on cholesterol- and EPA-supplemented S. elongatus; sufficient egg production was a prerequisite for the third experiment in which the lipid composition of eggs was analyzed. For the third experiment, S. obliquus was cultivated semi-continuously at a dilution rate of 0.25 d−1 in 5-L bottles containing Cyano medium (illumination at 120 μmol quanta m−2 s−1). Food suspensions were prepared by centrifugation and resuspension in fresh medium. Carbon concentrations of the food suspensions were estimated from photometric light extinctions using previously established carbon-extinction regressions. All experiments were carried out at 20°C and a 16:8 h light:dark cycle in glass beakers filled with 0.2 l of filtered lake water (< 0.2 μm) and saturating concentrations (2 mg C l−1) of S. elongatus or S. obliquus, respectively. Liposomes were prepared as described in Martin-Creuzburg et al. (2009).
Juvenile Growth Experiments
In a first experiment, female and male neonates were reared separately on a dietary sterol gradient which was established by adding increasing amounts of cholesterol-containing liposomes to the experimental beakers (200 ml). Seven dietary cholesterol concentrations were provided (0, 1.9, 3.8, 7.5, 15.0, 30.0, and 37.6 μg mg C−1), either in the absence or in the presence of saturating amounts of EPA (14 treatments in total). Simultaneous EPA supplementation was achieved by adding EPA-containing liposomes to the experimental beakers, corresponding to an EPA concentration of 27 μg mg C−1 (Figure S1).
In a second experiment, a dietary EPA gradient was established by adding increasing amounts of EPA-containing liposomes to the experimental beakers. Seven dietary EPA concentrations were provided (0, 0.5, 1.4, 2.5, 4.9, 10.2, and 20.3 μg mg C−1). In addition, to release the animals from sterol limitation, each beaker (including the control) was supplemented with cholesterol-containing liposomes, corresponding to a saturating dietary cholesterol concentration of 30 μg mg C−1 (Figure S1; Martin-Creuzburg et al., 2009).
To account for the increasing carbon and phosphorus (constituents of phospholipids) supply via cholesterol- or EPA-containing liposomes, unloaded (i.e., sterol- and EPA-free) control liposomes were added so that each beaker was provided in total with the same volume of liposome suspension. Each treatment consisted of six beakers (replicates), each containing five juvenile female or male D. magna. During the experiment, daphnids were transferred daily into new beakers with freshly prepared food suspensions. At day six, daphnids were removed from the beakers, rinsed with ultra-pure water, transferred into pre-weighed aluminum boats, and stored at −80°C until they were freeze-dried and weighed (Mettler Toledo XP2U; ±0.1 μg) for dry mass determination. Mass-specific juvenile somatic growth rates (g) were determined as the increase in dry mass from the beginning (M0) until the end (day six) of the experiment (Mt) with time (t) expressed as age in days:
Life History Experiment
In the third experiment, female and male neonates were reared separately in 200 ml beakers on the following three food treatments: S. obliquus supplemented with 50 μl of the control liposome suspension (henceforth referred to as “Scen”), S. obliquus supplemented with 30 μl of the EPA-containing liposome suspension (corresponding to a dietary EPA concentration of 16.2 μg mg C−1) and 20 μl of the control liposome suspension, and S. obliquus supplemented with 50 μl of the cholesterol-containing liposome suspension (corresponding to a dietary cholesterol concentration of 18.8 μg mg C−1). In total, all beakers were supplemented with 50 μl of liposome suspension. S. obliquus (2 mg C L−1) and the limiting nutrients EPA and cholesterol were provided at saturating concentrations (Figure S1). During the experiment, daphnids were transferred daily into new beakers with freshly prepared food suspensions. Initially, each food treatment consisted of 24 beakers each containing five female neonates and 24 beakers each containing five male neonates. At day four of the experiment and as soon as the female Daphnia deposited their first, second, and third clutch eggs, respectively, into their brood chambers, six beakers per treatment and sex were subsampled. The daphnids were removed from the beakers, rinsed with ultra-pure water and photographed for subsequent determination of body lengths using image processing software. Males were directly transferred into pre-weighed aluminum boats and stored at −80°C. Eggs from five egg-bearing females were collected under a stereomicroscope by gently flushing them out of the brood chamber with a lengthened glass Pasteur pipette (Wacker and Martin-Creuzburg, 2007; Schlotz et al., 2013). The eggs and the soma of the five egg-bearing females were rinsed again with ultra-pure water and then transferred separately into pre-weighed aluminum boats and stored at −80°C. At least 50 eggs were collected per sample; they were all in the first egg stage and did not show any morphological differentiation. All samples were freeze-dried, weighed for dry mass determination, and subsequently prepared for lipid extraction (as described below). Juvenile somatic growth rates (g) were determined from the increase in dry mass until day four of the experiment, as described above (Equation 1). Size-specific juvenile somatic growth rates were determined as the increase in body length from the beginning until day four of the experiment. Body length was defined as the distance from the mid-point of the compound eye to the base of the spina.
Lipid Analyses
For the analysis of fatty acids and sterols in the food suspensions, approximately 1 mg particulate organic carbon (POC) was filtered separately onto precombusted GF/F filters (Whatman; 25 mm). Filters and freeze-dried daphnids and eggs (along with the aluminum boats) were deposited in dichloromethane:methanol (2:1, v:v), vigorously sonicated and stored overnight at −20°C. On the next day, C23:0 methyl ester or 5α-cholestane was added as internal standards for fatty acid or sterol analysis, respectively. Total lipids were extracted using two washes with dichloromethane:methanol (2:1, v:v). The pooled cell-free lipid extracts were evaporated to dryness under N2-atmosphere and transesterified with 3 mol L−1 methanolic HCl (60°C, 15 min) for the analysis of fatty acids or saponified with 0.2 mol L−1 methanolic KOH (70°C, 1 h) for the analysis of sterols. Subsequently, fatty acid methyl esters (FAME) were extracted three times with 2 mL of iso-hexane; the neutral lipids were partitioned into iso-hexane:diethyl ether (9:1, v:v). The lipid-containing fraction was evaporated to dryness under nitrogen and resuspended in iso-hexane (10–20 μL). Fatty acids and sterols were analyzed and quantified by gas chromatography (GC) on a HP 6890 equipped with a flame ionization detector (FID) and a DB-225 (J&W Scientific, 30 m × 0.25 mm inner diameter [ID] × 0.25 μm film) capillary column to analyze FAME, or with a HP-5 (Agilent, 30 m × 0.25 mm ID × 0.25 μm film) capillary column to analyse sterols. Details of GC configurations are given elsewhere (Martin-Creuzburg et al., 2010; Martin-Creuzburg and Merkel, 2016). Fatty acids and sterols were quantified by comparison to internal standards (C23:0 methyl ester or 5α-cholestane) using multipoint calibration curves generated with lipid standards (Sigma). Fatty acids and sterols were identified by their retention times and their mass spectra, which were recorded with a gas chromatograph-mass spectrometer (GC-MS) (Agilent Technologies, 5975C inert MSD) equipped with a fused-silica capillary column (DB-5MS, Agilent; GC-MS configurations as for FID). Sterols were analyzed both in their free form and as their trimethylsilyl derivatives, which were prepared by incubating the iso-hexane sterol extracts with 10 μl of N,O-bis(trimethylsilyl)trifluoroacetamide (BSTFA) including 1% trimethylchlorosilane (TMCS) for 1 h at room temperature. Spectra were recorded between 50 and 600 Dalton in the electron impact (EI) ionization mode. The limit for quantitation of fatty acids and sterols was 10 ng. The absolute amount of each lipid was related to the dry mass of the animals or eggs. Lipids in the food suspensions were related to the particulate organic carbon content, which was determined using an elemental analyser (EuroEA3000, HEKAtech GmbH, Germany).
Data Analyses
Sterol- and EPA-limited growth responses and dietary thresholds were estimated based on saturation curve fitting (Sperfeld and Wacker, 2011) using a modified form of the Von Bertalanffy function (Von Bertalanffy, 1957) which describes somatic growth rates (g, d−1) in response to dietary nutrient concentrations (c, in μg mg C−1).
The parameters are: g0 = somatic growth rate (d−1) on unsupplemented S. elongatus, g∞ = asymptotic growth rate (d−1), b = exponential Bertalanffy growth coefficient in mg C (μg cholesterol or EPA)−1 with y = 4.
To estimate the uncertainty of each threshold depending on the variability of growth data we randomly sampled one out of six replicates for each of the original dietary sterol or EPA concentration levels and used Equation (2) to fit through the sampled growth rates using the non-linear least squares method of R 3.4.0. From the fitted curves we calculated the sterol and EPA concentrations at which maximum growth rates (g∞) were reduced by 25% and defined these concentrations as thresholds for sterol- or EPA limited growth, respectively. A growth rate reduction of 25% was used because the variability in threshold concentrations increases with increasing convergence to g∞ and this may compromise further evaluations (Sperfeld and Wacker, 2011). The value for g∞ cannot be used as estimation for growth saturation because saturation curves reach g∞ at infinity (Figure 1). Thus, we defined a saturation growth level clearly distinct to g∞ on the basis of the maximum growth improvement by dietary sterol or EPA supply (Figure 1). Using this conservative saturation growth level (reduction of g∞ by 25%) may result in a reduced sensitivity to detect growth limitations. However, this procedure clearly avoids biased threshold estimations due to neglecting some bootstrapped regression curves that do not intersect with the line of the defined saturation growth level, and allows for an unbiased comparison of threshold concentrations. By repeating this procedure 1,000 times, a parameter distribution around a median threshold was generated and used for further statistical comparison of thresholds among treatments.
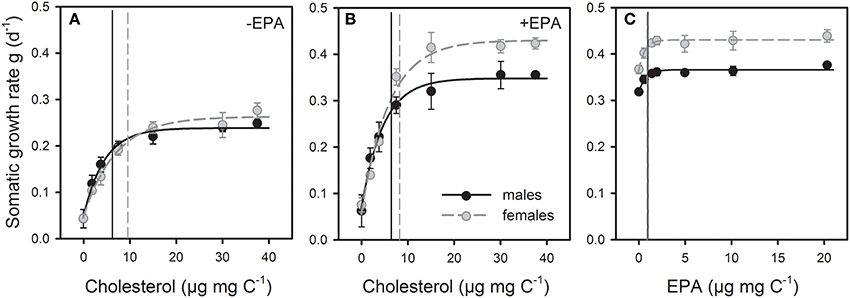
Figure 1. Juvenile somatic growth responses of female and male D. magna reared on a sterol- and PUFA-free food (Synechococcus elongatus) supplemented with increasing amounts of cholesterol, either in the absence (A) or presence of saturating amounts of EPA (B), or increasing amounts of EPA in the presence of saturating amounts of cholesterol (C). Vertical lines show dietary threshold concentrations defined as a growth rate reduction of g∞ by 25 %.
To statistically evaluate potential differences among thresholds, we randomly drew the same number of replicates as in the original experiment (n = 6) from the distribution of the 1,000 thresholds. This was done for each sex and treatment; the resulting replicates were compared using analysis of variance. Following the recommendation of Bolker (2008), this procedure was repeated 400 times. The proportion of significant tests, i.e., with P < 0.05 for each comparison, reflected the probability and power to detect significant differences among treatments. We searched for the critical P-value necessary to exceed an 80% proportion of significant tests (= 80% percentile) to hold for a statistical power of 0.8 (or a type two error of 0.2, respectively).
Mass- and size-specific juvenile somatic growth rates were analyzed using one-way analyses of variance (ANOVA). Data met the assumption of homogeneity of variance; treatment effects were analyzed using Tukey's HSD post-hoc tests. Clutch sizes, egg dry masses, and maternal dry mass investments were analyzed in a two-factorial ANOVA design, with clutch and food as factors and permitting their interaction. A two-factorial ANOVA design was also used to analyse differences in dry mass and lipid content between sexes and food treatments. Raw data were log-transformed, if necessary, to account for inhomogeneity of variances and non-normal distribution of residues. To account for multiple comparisons, rejection criteria were adjusted using the Holm-Bonferroni method. Treatment effects were analyzed using Tukey's HSD post-hoc tests.
A principal component analysis (PCA) was applied to compare the fatty acid profiles of egg-bearing females and males reared on the different food treatments and at the different sampling events. The PCA was calculated using proportions of total fatty acids. Proportions were used because we expected sex-specific changes in fatty acid profiles with age. The use of proportions allowed to explore relative changes in fatty acid profiles and to account for the general increase in the total fatty acid content with age in both sexes. For females, the sum of fatty acids detected in somatic tissue and eggs of the respective clutch was used. To avoid any bias, EPA was not considered in the PCA because it was detected only after supplementation in rather low concentrations.
All statistical calculations were carried out using the software package R (R Core Team, 2013).
Results
Juvenile Growth Experiments
Juvenile somatic growth rates (g) of female and male D. magna reared on unsupplemented S. elongatus were low, but increased considerably with increasing dietary cholesterol concentrations (Figure 1A). The sterol-limited growth responses of both sexes were even more pronounced in the presence of dietary EPA, resulting in significantly higher maximum growth rates than in the absence of dietary EPA (Figure 1B). Thresholds for sterol-limited growth (defined as a reduction of g∞ by 25%; Figure 1) were significantly higher in females than in males, but were not affected by additional EPA supplementation (Figure 2A, Table S1).
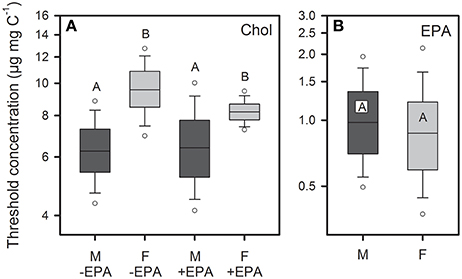
Figure 2. Dietary threshold concentrations (defined as 25% reduction of g∞) for sterol- and EPA-limited juvenile somatic growth of female (F) and male (M) D. magna. Juveniles were raised either on a dietary cholesterol gradient, in the absence and presence of saturating amount of dietary EPA (A), or on a dietary EPA gradient in presence of saturating amounts of cholesterol (B). Data are presented as medians, quartiles (box), 10 and 90% percentiles (whiskers), and 95% confidence intervals (circles). Data labeled with the same letters are not significantly different (A, sex: P < 0.01, EPA supplementation: P = 0.73, sex × EPA: P = 0.71; B, sex: P = 0.74).
Juvenile somatic growth rates of female and male D. magna reared on S. elongatus supplemented with increasing amounts of EPA and saturating amounts of cholesterol increased significantly with dietary EPA concentration (Figure 1C). The EPA-limited growth responses of females and males differed in their maximum growth rates, but did not reveal sex-specific differences in threshold concentrations for EPA-limited somatic growth (Figure 2B, Table S1).
Life History Experiment
Mass-specific juvenile somatic growth rates of D. magna reared on the green alga S. obliquus differed significantly among food treatments but not between sexes and did not reveal a significant interaction between sex and food [two-factorial ANOVA; sex: F(1, 30) = 0.05, P = 0.83; food: F(2, 30) = 82.2, P < 0.001; sex × food: F(2, 30) = 1.04, P = 0.36]. Mass-specific growth rates increased significantly upon EPA supplementation but were not affected by cholesterol supplementation (Tukey's HSD, P < 0.05; Figure 3). In contrast, size-specific somatic growth rates differed significantly also between sexes [two-factorial ANOVA; sex: F(1, 30) = 25.1, P < 0.001; food: F(2, 30) = 23.3, P < 0.001; sex × food: F(2, 30) = 2.85, P = 0.07; Figure S2], which was due to the fact that females achieved significantly higher size-specific growth rates on the EPA-supplemented diet (Tukey's HSD, P < 0.05; Figure S2).
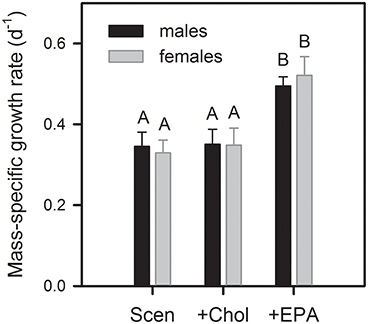
Figure 3. Mass-specific juvenile somatic growth rates of female and male D. magna reared on S. obliquus and S. obliquus supplemented with cholesterol or EPA. Growth rates were calculated from the increase in body dry mass up to day four of the experiment. Data represent means of n = 6 ± standard deviation (SD). Bars labeled with the same letters are not significantly different (Tukey's HSD, P < 0.05, following ANOVA).
Starting with a similar dry mass at birth [ANOVA, F(1, 4) = 3.42, P = 0.14], females gained significantly more body dry mass than males until the end of the experiment. This is indicated by the slopes of the dry mass-time regressions (Figure 4), which were always significantly higher for female than for male growth responses, irrespective of food treatment (non-overlapping 95% confidence intervals; Figures 4D–F). Differences in slopes were most pronounced between males and egg-bearing females but were evident also when considering somata of males and females only. The dry mass-time regressions also revealed a significantly higher dry mass investment in egg production upon EPA supplementation (Figures 4D,F). Cholesterol supplementation did not affect the gain in body dry mass or the investment in egg production (Figures 4D,E). The dry mass investment of females in egg production in each of the first three reproduction cycles roughly matched the total body dry mass of males sampled at the same time/age (Figures 4A–C). Females and males also did not differ in body size at birth [ANOVA, F(1, 18) = 0.79, P = 0.39]. However, females were almost twice as large as males at the end of the experiment in all food treatments (Figure S3).
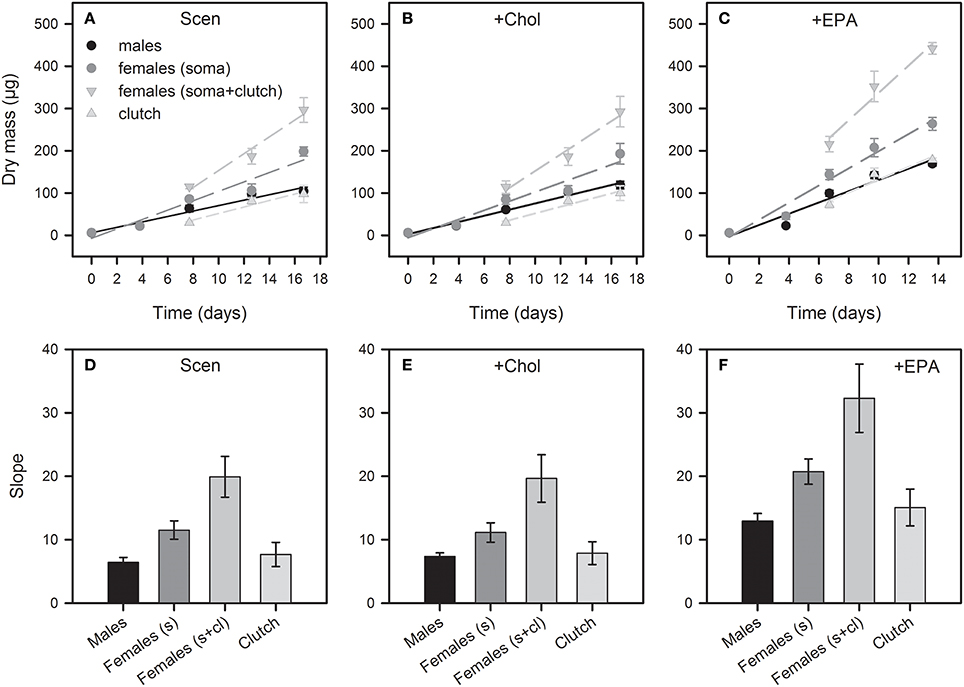
Figure 4. Dry mass increase of female and male D. magna reared on S. obliquus (A) and S. obliquus supplemented with cholesterol (B) or EPA (C). Females and males were subsampled at birth, at day four of the experiment, and when females deposited the first-, second-, and third-clutch eggs in their brood chambers. For dry mass determination and subsequent lipid analyses, eggs were removed from the brood chambers and female somata and eggs were weighed and analyzed separately. Clutch data represent the total female dry mass investment in egg production in the first, second and third reproduction cycle, respectively. The total dry mass of females was calculated by adding up soma and clutch dry mass (soma+clutch). Data in (A–C) represent means of n = 6 ± SD. The slopes of the linear regressions were used to assess sex-specific differences in dry mass increase (D–F). Error bars in (D–F) show 95% confidence intervals (CI). Non-overlapping CIs indicate significant differences.
Clutch size, egg dry mass, and total maternal dry mass investment significantly increased with each reproduction cycle and also differed significantly among food treatments (Figure 5, Table S2). At each of the first three reproduction cycles, clutch size, egg dry mass, and total maternal dry mass investment was significantly higher on the EPA-supplemented diet (Tukey's HSD, P < 0.05). Cholesterol supplementation did not affect female reproduction-related traits (Figure 5).
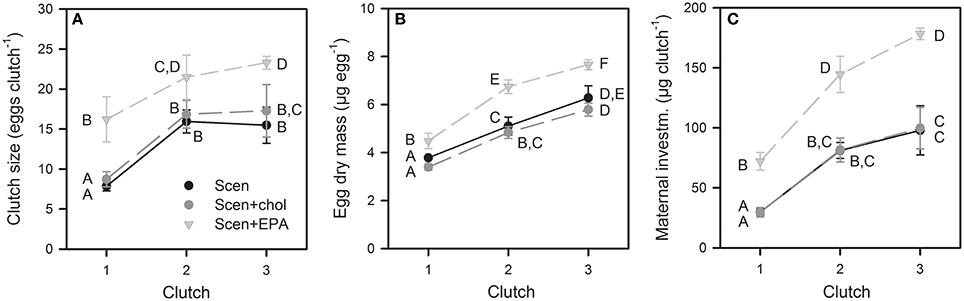
Figure 5. Clutch size (A), egg dry mass (B), and total maternal dry mass investment (C) in the first three reproduction cycles of D. magna reared on S. obliquus and S. obliquus supplemented with cholesterol or EPA. Data represent means of n = 6 ± SD. Data points labeled with the same letters are not significantly different (Tukey's HSD, P < 0.05, following ANOVA; Table S2).
The dry mass and lipid content of male and female somata (without eggs) at the end of the experiment, when females deposited their third clutch eggs in their brood chambers, differed significantly between sexes and among food treatments (Figure 6, Table S3). While female somata were characterized by a higher dry mass on all food treatments and higher cholesterol content on the unsupplemented and the cholesterol-supplemented food, male somata contained higher amounts of total fatty acids, linoleic acid (LIN; 18:2n-6), α-linolenic acid (ALA: 18:3n-3), and EPA (Figure 6). This finding was even more evident when the data were related to the body dry mass (Figure S4). Male somata contained much higher concentrations of LIN and ALA than female somata, while the concentrations of EPA and cholesterol did not differ significantly between sexes. The concentrations of LIN and ALA were significantly higher in eggs than in female somata, while EPA and cholesterol concentrations did not differ between eggs and female somata (Figure S4, Table S4).
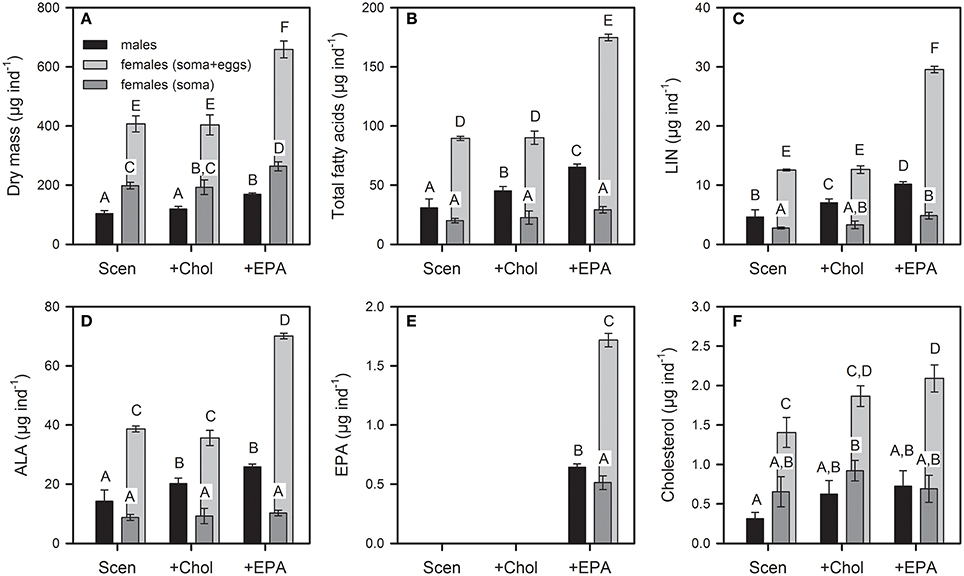
Figure 6. Dry mass (A), total fatty acid (B), linoleic acid (LIN; C), α-linolenic acid (ALA; D), eicosapentaenoic acid (EPA; E), and cholesterol (F) content (μg individual−1) of male and female somata of D. magna reared on S. obliquus (Scen) and S. obliquus supplemented with cholesterol or EPA until females deposited their third-clutch eggs into their brood chambers. Female somata and eggs produced in the first three reproduction cycles were analyzed separately to assess biomass and lipid allocation into female reproduction. Light gray bars (soma + eggs) represent the dry mass and lipid content of female somata at the end of the experiment figured up with the cumulative dry mass and lipid content of eggs produced within the first three reproduction cycles. Dry mass data represent means of n = 6 ± SD; lipid data represent means of n = 3 ± SD. Data points labeled with the same letters are not significantly different (Tukey's HSD, P < 0.05, following ANOVA; Table S3).
To assess the total biomass and lipid acquisition in females during the first three reproduction cycles, the cumulative dry mass and lipid investment in egg production was determined and figured up with the dry mass and lipid content of female somata at the end of the experiment (light gray bars in Figure 6). The dry mass of somatic tissues was 1.6–1.9-times higher in females (soma without eggs) than in males and the total dry mass acquisition, considering eggs produced in the first three reproduction cycles, was 3.4–3.9-times higher in females (soma + eggs) than in males, depending on the food treatment. The cumulative female dry mass investment in eggs of the first three reproduction cycles was 1.8–2.3-times higher than the total body dry mass of one male at the end of the experiment. The total PUFA content (primarily represented by LIN and ALA) of somatic tissues was 1.6–2.4-times higher in males than in females (soma without eggs; Figure 6) while the total PUFA acquisition was 1.8–2.8-times higher in females (soma + eggs) than in males. The cumulative PUFA allocation by one female in eggs of the first three reproduction cycles was 1.3–2.3-times higher than the total body PUFA content of one male at the end of the experiment. Similar allocation patterns were observed for single PUFA. EPA could be detected in somatic tissues and eggs only after supplementation (Figure 6E). The EPA content of somatic tissues was 1.3-times higher in males than in females while the total PUFA acquisition was 2.7-times higher in females (soma + eggs) than in males (Figure 6E). The cumulative EPA allocation by one female in eggs of the first three reproduction cycles was 1.9-times higher than the total body EPA content of one male at the end of the experiment. Cholesterol was also substantially allocated into the eggs but seemed to be more retained in female somata than fatty acids (Figure 6F).
The PCA of the fatty acid profiles of egg-bearing females and males projected most of the variance of the multivariate fatty acid data set on the first three principal component axes (PC1-3; Figure 7). PC1 explained 39.7% of variation, primarily separated the different sampling events, and revealed an increase in the proportion of ALA with age in both sexes. PC2 explained 21.4% of variation and revealed slightly higher proportions of 16:0 and 18:1n-9 in females than in males. PC3 explained 11.9% of variation and revealed a lower proportion of LIN in females than in males, with the exception of the EPA treatment. EPA supplementation resulted in a higher proportion of LIN in females but not in males. The increase in the proportion of LIN upon EPA supplementation was most pronounced in the third reproduction cycle, where the proportion of LIN was similar to the one found in males.
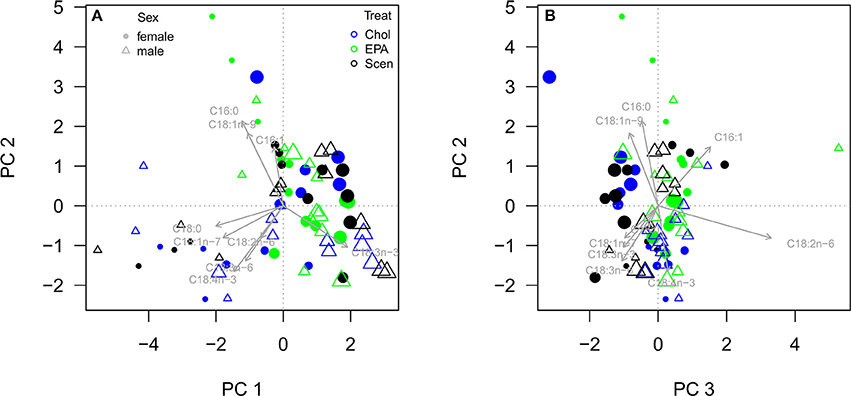
Figure 7. Principal component analysis (PCA) of the fatty acid profiles of egg-bearing female (filled circles) and male (triangles) D. magna reared on S. obliquus (Scen) supplemented with cholesterol- (Chol) or EPA-containing liposomes (EPA). PC1, PC2 (A), and PC3 (B) explained 39.7, 21.4, and 11.9% of variation, respectively. The PCA was calculated using fatty acid proportions of total fatty acids without considering EPA, which was detected only after supplementation in low concentrations. Female data represent fatty acids detected in somatic tissue and eggs of the respective clutch. The four consecutive sampling events are exemplified by increasing symbol sizes, i.e., the first sampling at day four is represented by the smallest symbols and the last sampling at the third reproduction cycle is represented by the largest symbols.
Discussion
We show here that dietary sterol requirements of female and male D. magna differ during juvenile somatic growth, as indicated by sex-specific differences in dietary threshold concentrations for sterol-limited growth. The thresholds for sterol-limited growth were significantly higher in females than in males, suggesting that females are more susceptible to dietary sterol deficiencies than males. The growth response obtained by supplementing increasing amounts of cholesterol suggests that 9.6 μg mg C−1 of cholesterol is required to release female juveniles from sterol limitation, which corroborates previous supplementation experiments (Martin-Creuzburg et al., 2009, 2014; Sperfeld and Wacker, 2009). In the presence of dietary EPA, the threshold for sterol-limited growth was somewhat lower (8.2 μg mg C−1) than in the absence of dietary EPA. Although this effect was not statistically significant it nevertheless supports previous findings showing lower thresholds for sterol-limited growth in the presence of dietary PUFA (Martin-Creuzburg et al., 2010; Sperfeld and Wacker, 2011). The threshold for sterol-limited growth of male juveniles was not affected by additional EPA supplementation (6.2 and 6.4 μg mg C−1 with and without EPA, respectively). Sex-specific differences in maximum somatic growth rates were evident primarily in the presence of dietary EPA, i.e., the generally higher growth potential of females did not come into play in the absence of dietary PUFA.
At saturating dietary sterol concentrations, juvenile somatic growth rates of both sexes increased with increasing dietary EPA concentrations. The maximum growth response upon EPA supplementation was significantly higher in females than in males, but the thresholds for EPA-limited growth did not differ between sexes. The obtained thresholds are comparable to previously published thresholds for EPA-limited juvenile growth of female D. magna (Sperfeld and Wacker, 2011). Thus, females achieve higher maximum somatic growth rates than males when released from dietary EPA limitation, due to their generally higher growth potential, but the sexes do not differ in their susceptibility to dietary EPA limitation during juvenile somatic growth.
The results discussed above were obtained by rearing Daphnia on a sterol- and PUFA-deficient diet, the cyanobacterium S. elongatus. Daphnia feeding on S. elongatus are simultaneously limited by sterols and PUFA (Martin-Creuzburg et al., 2009; Sperfeld et al., 2012), which makes it possible to study the sterol- and PUFA-requirements of Daphnia by supplementing single sterols and/or PUFA. Although simultaneous supplementation of S. elongatus with both resources, i.e., sterols and PUFA, results in rather high somatic and population growth rates (Martin-Creuzburg et al., 2009, 2010), fecundity is typically still lower than on eukaryotic algal diets. Thus, to study sex-specific differences in lipid requirements and allocation in mature animals, we used the green alga Scenedesmus obliquus as the experimental diet. S. obliquus is of moderate food quality for Daphnia, primarily because of a deficiency in long-chain (≥C20) PUFA (von Elert, 2002) and possibly because of its high content of Δ7-sterols, which may be less efficiently used by Daphnia than the more common Δ5-sterols (Martin-Creuzburg et al., 2014; Martin-Creuzburg and Merkel, 2016). The use of S. obliquus resulted in higher egg production, which was a precondition for subsequent lipid analyses, but still allowed for testing lipid-mediated food quality effects.
Mass-specific juvenile somatic growth rates of female and male D. magna reared on S. obliquus increased significantly upon EPA supplementation, while cholesterol supplementation had no effect. Our data suggest that both sexes benefit equally from dietary EPA supplementation during juvenile somatic growth. Conversely, the deficiency in Δ5-sterols in S. obliquus did not impair juvenile somatic growth rates. In contrast, differences between sexes were observed for size-specific somatic growth rates, which only in females increased significantly upon EPA supplementation. Thus, the dietary EPA supply may differently affect the allocation of resources toward size- and mass-specific somatic growth in female and male Daphnia.
To assess the maternal investment in reproduction, we analyzed female somata and eggs separately in each of the first three reproduction cycles. Males were subsampled at the same time the females deposited the first-, second-, and third-clutch egg in their brood chambers. The gain in body dry mass during the experiment was significantly higher in females than in males, irrespective of food treatment, as indicated by the higher slopes of the dry mass-time regressions (Figures 4D–F). Differences in slopes were most pronounced between males and egg-bearing females but were evident also between male and female somata (without eggs). Thus, despite the substantial investment in egg production during the first three reproduction cycles, the gain in somatic dry mass (without eggs) was significantly higher in females than in males. In each of the first three reproduction cycles, the dry mass investment of females in egg production roughly matched the total body dry mass of males sampled at the same time (Figures 4A–C), i.e., each female invested the dry mass equivalent of one age-matched male in each reproduction cycle. The dry mass-time regressions also revealed a significant increase in dry mass investment in egg production upon EPA supplementation (Figures 4D–F), which supports previous findings showing that the dietary EPA supply strongly improves parthenogenetic reproduction (Becker and Boersma, 2005; Martin-Creuzburg et al., 2009). In each of the first three reproduction cycles, clutch sizes, egg dry masses, and total maternal dry mass investments were significantly higher on the EPA-supplemented diet. Larger eggs may result in larger body size at first reproduction and thus may increase offspring fecundity (Guisande and Gliwicz, 1992; Lampert, 1993). It has been shown already that the dietary EPA supply is associated with strong maternal effects, which are most likely mediated by the allocation of dietary EPA into the eggs (Schlotz et al., 2013; Sperfeld and Wacker, 2015). However, our data suggest that the positive effects of EPA supplementation on female reproduction are multifaceted, involving traits not directly related to EPA allocation, resulting in higher egg dry mass. It should be noted, however, that the gain in body dry mass increased significantly upon EPA supplementation in both sexes, indicating that both sexes benefited from dietary EPA supply (Figure 8). Cholesterol supplementation did not affect the gain in body dry mass in both sexes or the investment in egg production, suggesting that both sexes were sufficiently supplied with sterols while growing on the green alga S. obliquus.
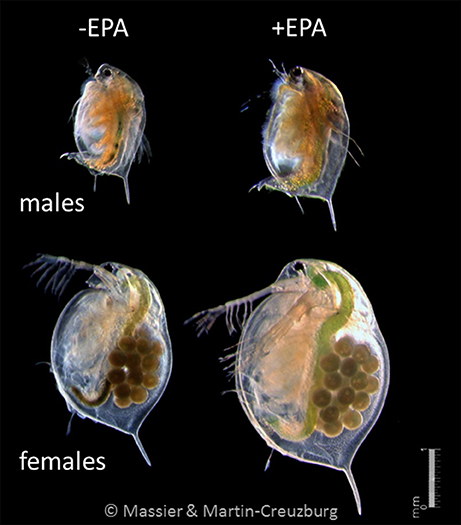
Figure 8. Female and male D. magna reared on S. obliquus and S. obliquus supplemented with EPA. The photographs were taken at the end of the life history experiment when females deposited their third clutch eggs in their brood chambers (day 16.7 without EPA, day 13.6 with EPA). Both sexes benefited from dietary EPA supplementation but the effects of EPA enrichment were most pronounced regarding female reproduction.
With our experimental set-up it was not possible to separate the effects of EPA supplementation on the development of somatic and reproductive tissues, because we did not dissect ovaries and testes prior to analysis. Although there have been attempts to dissect testes and to quantify spermatozoa of male D. magna (Duneau et al., 2012; Gómez et al., 2016), it appears impossible to obtain sufficient material for quantitative dry mass determination and lipid analysis. Moreover, even if it would be possible to quantitatively dissect ovaries and testes it would be difficult to preserve the remaining body tissues for separate analyses. Thus, to assess sex-specific differences in nutrient acquisition, we analyzed the dry mass and lipid content of female (somata and eggs separately) and male D. magna in each of the first three female reproduction cycles.
At the end of the experiment, when females deposited their third clutch of eggs into their brood chambers, the dry mass and lipid content of female and male somata differed significantly between sexes and among food treatments. According to their generally higher growth potential, female somata (without eggs) were characterized by a higher dry mass, irrespective of food treatment. Nevertheless, male somata contained higher amounts of total fatty acids, LIN, ALA, and EPA than female somata on all supplemented diets. These findings were even more evident when the data were related to the body dry mass (Figure S4). The concentrations of LIN and ALA were much higher in male than in female somata; LIN and ALA were also more concentrated in eggs than in female somata. In contrast, the concentrations of EPA and cholesterol were comparable in all tissues. We have shown previously that female Daphnia allocate substantial amounts of PUFA into the eggs, while cholesterol is retained more in somatic tissues (Wacker and Martin-Creuzburg, 2007). The lower content of total fatty acids and PUFA in female than male somata most likely resulted from the females' substantial allocation of nutrients into the eggs produced during the first three reproduction cycles. Especially LIN and ALA, which were sufficiently provided through the green algal diet, were allocated in high amounts into the eggs. EPA was available in much lower dietary concentrations than LIN and ALA, potentially resulting in a higher retention of EPA in female somata and thus similar EPA concentrations in female and male somata. Overall, however, the dry mass and lipid acquisition until the end of the experiment was significantly higher in females (incl. eggs of 1.-3. clutch) than in males. Thus, the high maternal investment in reproduction leads to a depletion of nutrients in female somata, while the comparatively low paternal investment in reproduction allows for the accumulation of nutrients in male somata. This suggests that males are less susceptible to dietary deficiencies than females, because they can rely more on internal body stores. When nutrients are scarce, females may retain higher amounts of nutrients in somatic tissues, potentially at the expense of a lower reproductive output.
In Daphnia, sex-specific differences in lipid profiles have not been studied yet. We applied a principal component analysis (PCA) to compare fatty acid profiles of egg-bearing females (somata with eggs) and males reared on the different food treatments. The PCA was calculated using proportions of total fatty acids, which made it possible to compare the relative changes in fatty acid profiles between sexes, among food treatments and with age. The PCA revealed that the fatty acid profiles of egg-bearing females and males primarily changed with age; both sexes revealed a temporal increase in the relative proportion of ALA. The proportion of LIN was lower in females than in males, although the proportion in females approached the one in males after EPA supplementation. EPA supplementation resulted in higher egg production and in the allocation of EPA into the eggs, which was accompanied by a generally higher allocation of dietary n-3 and n-6 PUFA (primarily ALA and LIN) into the eggs. The increased acquisition of dietary LIN in response to EPA supplementation may represent a mechanism by which egg-bearing females balance the allocation of n-3 and n-6 PUFA into their eggs. This finding, however, should be subjected to further investigations. Overall, fatty acid profiles changed primarily with age while the effects of food and sex were less distinct. In Drosophila, differences between sexes have been found in the fatty acid saturation state but only within two lipid class fractions, the cholesterol ester and lysophosphatidylcholine fraction, with females containing higher amounts of mono- and polyunsaturated fatty acids (Parisi et al., 2011). Our data suggest that, in Daphnia, sex-specific differences in fatty acid profiles are qualitatively rather low and primarily manifested in quantitative differences that are mediated by sex-specific allocation patterns.
Conclusion
The sex-specific differences in dietary threshold concentrations for sterol-limited juvenile growth suggest that females are more susceptible to dietary sterol deficiencies than males, presumably due to their generally higher growth potential. Sex-specific differences in maximum somatic growth rates were evident primarily in the presence of dietary EPA; females did not exploit their higher growth potential in the absence of dietary PUFA. However, the thresholds for EPA-limited growth did not differ between sexes, suggesting that both sexes have similar dietary EPA requirements during juvenile growth. The gain in body dry mass during the life history experiment was significantly higher in females (with and without eggs) than in males, irrespective of food treatment. In both sexes, the gain in body dry mass increased significantly upon EPA supplementation, indicating both sexes benefited from dietary EPA supply also later in life. EPA supplementation resulted in an increased maternal investment in reproduction, primarily reflected in larger clutch sizes and egg dry masses. The increased maternal investment in reproduction was accompanied by a depletion of nutrients in female somata, while the comparatively low paternal investment in reproduction resulted in the accumulation of nutrients (including PUFA) in male somata. This suggests that males are generally less susceptible to dietary nutrient deficiencies than females, because they can rely more on internal body stores. We propose that the performances of the sexes are differentially influenced by food quality, which may have consequences for sexual reproduction and, consequently, the formation of resting eggs and the maintenance of Daphnia populations. Studying sex-specific differences in nutritional traits may help to understand the patterns of sexual reproduction in Daphnia populations and thus is of great ecological and evolutionary interest.
Datasets are Available on Request
The raw data supporting the conclusions of this manuscript will be made available by the authors, without undue reservation, to any qualified researcher.
Author Contributions
DM-C and TM designed the experiments. TM conducted the experiments and performed the lipid analyses. All authors analyzed the data. DM-C wrote the first draft of the manuscript; all authors contributed substantially to subsequent versions of the manuscript.
Funding
DM-C was supported financially by the University of Konstanz; AW was supported by the German Research Foundation (DFG, WA 2445/8-2).
Conflict of Interest Statement
The authors declare that the research was conducted in the absence of any commercial or financial relationships that could be construed as a potential conflict of interest.
Acknowledgments
We thank P. Merkel for excellent technical assistance.
Supplementary Material
The Supplementary Material for this article can be found online at: https://www.frontiersin.org/articles/10.3389/fevo.2018.00089/full#supplementary-material
References
Abrusán, G., Fink, P., and Lampert, W. (2007). Biochemical limitation of resting egg production in Daphnia. Limnol. Oceanogr. 52, 1724–1728. doi: 10.4319/lo.2007.52.4.1724
Ahlgren, G., Lundstedt, L., Brett, M., and Forsberg, C. (1990). Lipid composition and food quality of some freshwater microalgae. J. Plankton Res. 12, 809–818. doi: 10.1093/plankt/12.4.809
Becker, C., and Boersma, M. (2003). Resource quality effects on life histories of Daphnia. Limnol. Oceanogr. 48, 700–706. doi: 10.4319/lo.2003.48.2.0700
Becker, C., and Boersma, M. (2005). Differential effects of phosphorus and fatty acids on Daphnia magna growth and reproduction. Limnol. Oceanogr. 50, 388–397. doi: 10.4319/lo.2005.50.1.0388
Behmer, S. T., and Nes, W. D. (2003). Insect sterol nutrition and physiology: a global overview. Adv. Insect Physiol. 31, 1–72. doi: 10.1016/S0065-2806(03)31001-X
Bonduriansky, R. A., and Runagall-McNaull, Crean, A. J. (2016). The nutritional geometry of parental effects: maternal and paternal macronutrient consumption and offspring phenotype in a neriid fly. Funct. Ecol. 30, 1675–1686. doi: 10.1111/1365-2435.12643
Bowman, E., and Tatar, M. (2016). Reproduction regulates Drosophila nutrient intake through independent effects of egg production and sex peptide: implications for aging. Nutr. Healthy Aging 4, 55–61. doi: 10.3233/NHA-1613
Brett, M. T., Muller-Navarra, D. C., Ballantyne, A. P., Ravet, J. L., and Goldman, C. R. (2006). Daphnia fatty acid composition reflects that of their diet. Limnol. Oceanogr. 51, 2428–2437. doi: 10.4319/lo.2006.51.5.2428
Brown, M. R., Sieglaff, D. H., and Rees, H. H. (2009). Gonadal ecdysteroidogenesis in arthropoda: occurrence and regulation. Annu. Rev. Entomol. 54, 105–125. doi: 10.1146/annurev.ento.53.103106.093334
Bunning, H., Rapkin, J., Belcher, L., Archer, C. R., Jensen, K., and Hunt, J. (2015). Protein and carbohydrate intake influence sperm number and fertility in male cockroaches, but not sperm viability. Proc. Biol. Sci. 282:20142144. doi: 10.1098/rspb.2014.2144
Camus, M. F., Fowler, K., Piper, M. W. D., and Reuter, M. (2017). Sex and genotype effects on nutrient-dependent fitness landscapes in Drosophila melanogaster. Proc. Biol. Sci. 284:20172237. doi: 10.1098/rspb.2017.2237
Decaestecker, E., De Meester, L., and Mergeay, J. (2009). “Cyclical parthenogenesis in Daphnia: sexual versus asexual reproduction,” in Lost Sex: The Evolutionary Biology of Parthenogenesis, eds I. Schön, K. Martens, and P. van Dijk (Dordrecht: Springer), 295–316.
Duneau, D., Luijckx, P., Ruder, L. F., and Ebert, D. (2012). Sex-specific effects of a parasite evolving in a female-biased host population. BMC Biol. 10:104. doi: 10.1186/1741-7007-10-104
Esmaeili, V., Shahverdi, A. H., Moghadasian, M. H., and Alizadeh, A. R. (2015). Dietary fatty acids affect semen quality: a review. Andrology 3, 450–461. doi: 10.1111/andr.12024
Goad, L. J. (1981). Sterol biosynthesis and metabolism in marine invertebrates. Pure Appl. Chem. 53, 837–852. doi: 10.1351/pac198153040837
Gómez, R., Van Damme, K., Gosálvez, J., Morán, E. S., and Colbourne, J. K. (2016). Male meiosis in Crustacea: synapsis, recombination, epigenetics and fertility in Daphnia magna. Chromosoma 125, 769–787. doi: 10.1007/s00412-015-0558-1
Guisande, C., and Gliwicz, Z. M. (1992). Egg size and clutch size in two Daphnia species grown at different food levels. J. Plankton Res. 14, 997–1007. doi: 10.1093/plankt/14.7.997
Haines, T. H. (2001). Do sterols reduce proton and sodium leaks through lipid bilayers? Prog. Lipid Res. 40, 299–324. doi: 10.1016/S0163-7827(01)00009-1
Hartwich, M., Martin-Creuzburg, D., Rothhaupt, K. O., and Wacker, A. (2012). Oligotrophication of a large, deep lake alters food quantity and quality constraints at the primary producer-consumer interface. Oikos 121, 1702–1712. doi: 10.1111/j.1600-0706.2011.20461.x
Hartwich, M., Martin-Creuzburg, D., and Wacker, A. (2013). Seasonal changes in the accumulation of polyunsaturated fatty acids in zooplankton. J. Plankton Res. 35, 121–134. doi: 10.1093/plankt/fbs078
Hoffman, J. M., Soltow, Q. A., Li, S., Sidik, A., Jones, D. P., and Promislow, D. E. (2014). Effects of age, sex, and genotype on high-sensitivity metabolomic profiles in the fruit fly, Drosophila melanogaster. Aging Cell 13, 596–604. doi: 10.1111/acel.12215
Jensen, K., McClure, C., Priest, N. K., and Hunt, J. (2015). Sex-specific effects of protein and carbohydrate intake on reproduction but not lifespan in Drosophila melanogaster. Aging Cell 14, 605–615. doi: 10.1111/acel.12333
Jüttner, F., Leonhardt, J., and Mohren, S. (1983). Environmental factors affecting the formation of mesityloxide, dimethylallylic alcohol and other volatile compounds excreted by Anabaena cylindrica. J. Gen. Microbiol. 129, 407–412. doi: 10.1099/00221287-129-2-407
Lampert, W. (1993). Phenotypic plasticity of the size at first reproduction in Daphnia: the importance of maternal size. Ecology 74, 1455–1466. doi: 10.2307/1940074
Lee, K. P., Simpson, S. J., Clissold, F. J., Brooks, R., Ballard, J. W. O., Raubenheimer, D., et al. (2008). Lifespan and reproduction in Drosophila: new insights from nutritional geometry. Proc. Natl. Acad. Sci. U.S.A 105, 2498–2503. doi: 10.1073/pnas.0710787105
Lürling, M., and Beekman, W. (2006). Growth of Daphnia magna males and females fed with the cyanobacterium Microcystis aeruginosa and the green alga Scenedesmus obliquus in different proportions. Acta Hydrochim. Hydrobiol. 34, 375–382. doi: 10.1002/aheh.200500634
Maklakov, A. A., Simpson, S. J., Zajitschek, F., Hall, M. D., Dessmann, J., et al. (2008). Sex-specific fitness effects of nutrient intake on reproduction and lifespan. Curr. Biol. 18, 1062–1066. doi: 10.1016/j.cub.2008.06.059
Martin-Creuzburg, D., and Merkel, P. (2016). Sterols of freshwater microalgae: potential implications for zooplankton nutrition. J. Plankton Res. 38, 865–877. doi: 10.1093/plankt/fbw034
Martin-Creuzburg, D., Oexle, S., and Wacker, A. (2014). Thresholds for sterol-limited growth of Daphnia magna: a comparative approach using 10 different sterols. J. Chem. Ecol. 40, 1039–1050. doi: 10.1007/s10886-014-0486-1
Martin-Creuzburg, D., Sperfeld, E., and Wacker, A. (2009). Colimitation of a freshwater herbivore by sterols and polyunsaturated fatty acids. Proc. R. Soc. B 276, 1805–1814. doi: 10.1098/rspb.2008.1540
Martin-Creuzburg, D., Wacker, A., and Basen, T. (2010). Interactions between limiting nutrients: consequences for somatic and population growth of Daphnia magna. Limnol. Oceanogr. 55, 2597–2607. doi: 10.4319/lo.2010.55.6.2597
Morimoto, J., and Wigby, S. (2016). Differential effects of male nutrient balance on pre- and post-copulatory traits, and consequences for female reproduction in Drosophila melanogaster. Sci. Rep. 6:27673. doi: 10.1038/srep27673
Müller-Navarra, D. C. (1995). Evidence that a highly unsaturated fatty acid limits Daphnia growth in nature. Arch. Hydrobiol. 132, 297–307.
Müller-Navarra, D. C., Brett, M. T., Liston, A. M., and Goldman, C. R. (2000). A highly unsaturated fatty acid predicts carbon transfer between primary producers and consumers. Nature 403, 74–77. doi: 10.1038/47469
Mykles, D. L. (2011). Ecdysteroid metabolism in crustaceans. J. Steroid Biochem. Mol. Biol. 127, 196–203. doi: 10.1016/j.jsbmb.2010.09.001
Nong, Q. D., Ishak, N. S., Matsuura, T., Kato, Y., and Watanabe, H. (2017). Mapping the expression of the sex determining factor Doublesex1 in Daphnia magna using a knock-in reporter. Sci. Rep. 7:13521. doi: 10.1038/s41598-017-13730-4
Ohvo-Rekilä, H., Ramstedt, B., Leppimäki, P., and Slotte, J. (2002). Cholesterol interactions with phospholipids in membranes. Prog. Lipid Res. 41, 66–97. doi: 10.1016/S0163-7827(01)00020-0
Parisi, M., Li, R., and Oliver, B. (2011). Lipid profiles of female and male Drosophila. BMC Res. Notes 4:198. doi: 10.1186/1756-0500-4-198
Polak, M., Simmons, L. W., Benoit, J. B., Ruohonen, K., Simpson, S. J., and Solon-Biet, S. M. (2017). Nutritional geometry of paternal effects on embryo mortality. Proc.Biol. Sci. 284:20171492. doi: 10.1098/rspb.2017.1492
Porter, J. A., Young, K. E., and Beachy, P. A. (1996). Cholesterol modification of hedgehog signaling proteins in animal development. Science 274, 255–259. doi: 10.1126/science.274.5285.255
Putman, A., Martin-Creuzburg, D., Panis, B., and De Meester, L. (2015). A comparative analysis of the fatty acid composition of sexual and asexual eggs of Daphnia magna and its plasticity as a function of food quality. J. Plankton Res. 37, 752–763. doi: 10.1093/plankt/fbv043
Rahman, M. M., Gasparini, C., Turchini, G. M., and Evans, J. P. (2014). Experimental reduction in dietary omega-3 polyunsaturated fatty acids depresses sperm competitiveness. Biol. Lett. 10:20140623. doi: 10.1098/rsbl.2014.0623
Ravet, J. L., Brett, M., and Arhonditsis, G. (2010). The effects of seston lipids on zooplankton fatty acid composition in Lake Washington, Washington, USA. Ecology 91, 180–190. doi: 10.1890/08-2037.1
R Core Team (2013). R: A Language and Environment for Statistical Computing. Vienna: R Foundation for Statistical Computing. Version 3.02. Available online at: http://www.R-project.org/
Reddiex, A. J., Gosden, T. P., Bonduriansky, R., and Chenoweth, S. F. (2013). Sex-specific fitness consequences of nutrient intake and the evolvability of diet preferences. Am. Nat. 182, 91–102. doi: 10.1086/670649
Schlechtriem, C., Arts, M. T., and Zellmer, I. D. (2006). Effect of temperature on the fatty acid composition and temporal trajectories of fatty acids in fasting Daphnia pulex (crustacea, cladocera). Lipids 41, 397–400. doi: 10.1007/s11745-006-5111-9
Schlotz, N., Ebert, D., and Martin-Creuzburg, D. (2013). Dietary supply with polyunsaturated fatty acids and resulting maternal effects influence host - parasite interactions. BMC Ecol. 13:41. doi: 10.1186/1472-6785-13-41
South, S. H., House, C. M., Moore, A. J., Simpson, S. J., and Hunt, J. (2011). Male cockroaches prefer a high carbohydrate diet that makes them more attractive to females: implications for the study of condition dependence. Evolution 65, 1594–1606. doi: 10.1111/j.1558-5646.2011.01233.x
Sperfeld, E., Martin-Creuzburg, D., and Wacker, A. (2012). Multiple resource limitation theory applied to herbivorous consumers: Liebig's minimum rule vs. interactive co-limitation. Ecol. Lett. 15, 142–150. doi: 10.1111/j.1461-0248.2011.01719.x
Sperfeld, E., and Wacker, A. (2009). Effects of temperature and dietary sterol availability on growth and cholesterol allocation of the aquatic keystone species Daphnia. J. Exp. Biol. 212, 3051–3059. doi: 10.1242/jeb.031401
Sperfeld, E., and Wacker, A. (2011). Temperature- and cholesterol-induced changes in eicosapentaenoic acid limitation of Daphnia magna determined by a promising method to estimate growth saturation thresholds. Limnol. Oceanogr. 56, 1273–1284. doi: 10.4319/lo.2011.56.4.1273
Sperfeld, E., and Wacker, A. (2015). Maternal diet of Daphnia magna affects offspring growth responses to supplementation with particular polyunsaturated fatty acids. Hydrobiologia 755, 267–282. doi: 10.1007/s10750-015-2244-y
Stanley, D. (2006). Prostaglandins and other eicosanoids in insects: Biological significance. Ann. Rev. Entomol. 51, 25–44. doi: 10.1146/annurev.ento.51.110104.151021
Stanley, D. W. (2000). Eicosanoids in Invertebrate Signal Transduction Systems. Princeton, NJ: Princeton University Press.
Subramoniam, T. (2000). Crustacean ecdysteroids in reproduction and embryogenesis. Comp. Biochem. Physiol. C 125, 135–156. doi: 10.1016/S0742-8413(99)00098-5Get
Taipale, S. J., Kainz, M. J., and Brett, M. T. (2011). Diet-switching experiments show rapid accumulation and preferential retention of highly unsaturated fatty acids in Daphnia. Oikos 120, 1674–1682. doi: 10.1111/j.1600-0706.2011.19415.x
Terashima, J., and Bownes, M. (2004). Translating available food into the number of eggs laid by Drosophila melanogaster. Genetics 167, 1711–1719. doi: 10.1534/genetics.103.024323
Trivers, R. L. (1972). “Parental investment and sexual selection,” in Sexual Selection and the Descent of Man, ed B. Campbell (Chicago, IL: Transaction Publishers), 136–179.
Valentine, R. C., and Valentine, D. L. (2004). Omega-3 fatty acids in cellular membranes: a unified concept. Prog. Lipid Res. 43, 383–402. doi: 10.1016/j.plipres.2004.05.004
Von Bertalanffy, L. (1957). Quantitative laws in metabolism and growth. Q. Rev. Biol. 32, 217–231. doi: 10.1086/401873
von Elert, E. (2002). Determination of limiting polyunsaturated fatty acids in Daphnia galeata using a new method to enrich food algae with single fatty acids. Limnol. Oceanogr. 47, 1764–1773. doi: 10.4319/lo.2002.47.6.1764
von Elert, E., Martin-Creuzburg, D., and Le Coz, J. (2003). Absence of sterols constrains carbon transfer between cyanobacteria and a freshwater herbivore (Daphnia galeata). Proc. R. Soc. B 270, 1209–1214. doi: 10.1098/rspb.2003.2357
Wacker, A., and Martin-Creuzburg, D. (2007). Allocation of essential lipids in Daphnia magna during exposure to poor food quality. Funct. Ecol. 21, 738–747. doi: 10.1111/j.1365-2435.2007.01274.x
Wacker, A., and Martin-Creuzburg, D. (2012). Biochemical nutrient requirements of the rotifer Brachionus calyciflorus: co-limitation by sterols and amino acids. Funct. Ecol. 26, 1135–1143. doi: 10.1111/j.1365-2435.2012.02047.x
Keywords: allocation, cholesterol, eicosapentaenoic acid, food quality, male Daphnia, polyunsaturated fatty acids, sterols, lipid limitation thresholds
Citation: Martin-Creuzburg D, Massier T and Wacker A (2018) Sex-Specific Differences in Essential Lipid Requirements of Daphnia magna. Front. Ecol. Evol. 6:89. doi: 10.3389/fevo.2018.00089
Received: 25 February 2018; Accepted: 04 June 2018;
Published: 21 June 2018.
Edited by:
Dörthe Müller-Navarra, Universität Hamburg, GermanyReviewed by:
Serena Rasconi, Wasser Cluster Lunz, AustriaMichael T. Brett, University of Washington, United States
Copyright © 2018 Martin-Creuzburg, Massier and Wacker. This is an open-access article distributed under the terms of the Creative Commons Attribution License (CC BY). The use, distribution or reproduction in other forums is permitted, provided the original author(s) and the copyright owner are credited and that the original publication in this journal is cited, in accordance with accepted academic practice. No use, distribution or reproduction is permitted which does not comply with these terms.
*Correspondence: Dominik Martin-Creuzburg, dominik.martin-creuzburg@uni-konstanz.de