- Department of Biological Sciences, Lehman College-City University of New York, Bronx, NY, United States
Emotions are often associated with the evolution of monogamy. For example, fear of cuckoldry has been recently proposed as the driving force for human monogamy. We used prairie voles (Microtus ochrogaster) as a model of human behavior to study how stress reactivity is shaped by socio-ecological disruptions experienced as neonates and as subadults. We hypothesized that social disruptions would have a greater impact on the vole's stress levels than ecological disruptions, and that females would be more susceptible to the experience than males. At 6 days postpartum, the housing conditions were manipulated to have offspring raised by: (1) both parents under a protective cover (NoDisrupt); (2) Both parents uncovered (EcoDisrupt); (3) Mother alone covered (SocDisrupt); (4) Mother alone uncovered (SocEcoDisrupt). To experience disruptions as subadults, offspring were weaned then housed either alone (Isolate) or with a same-sex sibling (Social). As adults, each offspring was placed in an open-field arena and tested over 3 consecutive days to measure its behavior in response to an empty space (Day1), a same-sex vole in a container (Day2), and an empty container (Day3). The brain of a subgroup of subjects was processed for Golgi staining to assess the impact of disruptions on hippocampal dendritic morphology in adulthood. Males that experienced social disruption in early life displayed lower stress levels on Day2 of testing than males and females in other groups. This effect was only evident in males that did not experience social disruptions as subadults. Socio-ecological disruptions at postpartum had an unanticipated impact on the hippocampus of the voles. The apical dendrites of the CA3 neurons in male and female voles that experienced either social or ecological disruptions in early life and remained socially isolated as subadults were longer than those in undisturbed voles. Our results suggest that social disruptions experienced in early life modulate the male's stress-related behaviors and may thus influence his monogamous tendencies. Exposure to disruptions may also impact the memory circuits of the brain that monogamous animals use to make mating decisions.
Introduction
The evolutionary pressure that led humans and other mammals to form monogamous relationships is an enigma (Wittenberger and Tilson, 1980; Henrich et al., 2012). Given the low cost of reproduction for male mammals, they should maximize their fitness by pursuing as many females as possible (Clutton-Brock and Parker, 1992; Kappeler, 2013), but not all of them do (Lukas and Clutton-Brock, 2012). Males of a few species across a broad range of taxa display pair-living and copulate with their partner rather than with novel females (Lukas and Clutton-Brock, 2013). Although some of these males occasionally come in contact with other females and engage in extra-pair copulations (Solomon and Jacquot, 2002; Cohas and Allaine, 2009; Streatfeild et al., 2011), most share a home range with one female for an extended period of time and some even participate in parental care (Reichard, 2003). Studies from a variety of species that have attempted to explain why some males forgo their mating opportunities to invest in their family have produced contradictory results (Dobson et al., 2010). While the conflicting data continues to puzzle the evolutionary biologists (Kappeler, 2013), the research has provided neurobiologists with multiple avenues to explore the underlying physiological mechanisms that may maintain social monogamy within a population.
The neurobiology of social monogamy is studied from multiple angles. Some have focused on the biology of attachment in monogamous species as a rewarding emotion that keeps sexual partners in close proximity of each other and fosters paternal care (Lim et al., 2004; Young and Wang, 2004; Young, 2009; Young et al., 2011; Burkett and Young, 2012; Johnson and Young, 2015). This line of research, which has been immensely fruitful in discovering the neurochemistry of selective partner preference and social bonding, has been based on the theory that the evolution of monogamy was driven by the need for males to invest in paternal care, thus making bonding an adaptive behavior (Geary, 2000; Fraley et al., 2005; Young et al., 2011). However, others have viewed the problem of monogamy as a male tactic to maximize his fitness in environments where access to mates is limited and where he could be cuckolded into caring for another male's progeny (Phelps and Ophir, 2009; Ophir, 2017). This approach that is focused on cognition is more in line with the theory that the pressure driving the evolution of monogamy was mate guarding or resource scarcity instead of paternal investment (Schacht and Bell, 2016). Accordingly, individuals choose a mating strategy to increase their fitness and lower their chances of being cuckolded by processing social and spatial information within a given space. That information enables them to identify and remember the location of potential mates and their competitors. Additionally, a unified model of emotions and cognition controlled by the so called “social decision-making network” has been proposed to show how animals could evaluate their social environment when deciding whether to remain attached to a single mating partner, mate outside the relationship or seek many partners (Ophir, 2017). A key brain region in this model is the hippocampus, which along with the retrosplenial cortex, anterior thalamus, and septal nuclei is part of a network for forming spatial and social memories, and is also a main accessory area of the mesolimbic reward circuitry (Ikemoto, 2010; O'Connell and Hofmann, 2011; Ophir, 2017).
If social monogamy is a cost-benefit assessment evolved to enhance fitness, then it is likely to be entangled not only with the positive emotion of attachment, but also with stress that can affect decisions making. In real life, when individuals are challenged with physical threats or face uncertainty, neither humans nor other animals weigh all alternatives to choose the best strategy and their cognitive abilities can be affected by their stress responses (Giora, 1987; Lima, 1998; Porcelli and Delgado, 2017; Summers et al., 2017). Although several studies have examined the role of stress in monogamy, most have focused on how stress influences or is impacted by partner preference and paternal care (DeVries et al., 1996; Bosch et al., 2009; Hostetler and Ryabinin, 2013; Hyer and Glasper, 2017; Kowalczyk et al., 2018). Our aim in the current study was to examine the development of stress reactivity in a socially monogamous rodent that displays alternative reproductive tactics in nature to understand how stress might shape an individual's decision-making processes associated with reproductive strategies.
To investigate the development of stress reactivity in a monogamous species, we looked at studies in other rodents. Research in rats has shown that the rearing environment in early life impacts the developing brain to shape the adult stress responses, cognition, and reproductive behavior (Liu et al., 2000; Cameron et al., 2008; Kundakovic and Champagne, 2015). However, the process is complex and depends on multiple factors including the offspring genotype, sex, and social interactions experienced later in life (Daskalakis et al., 2013). We used prairie voles as an animal model of social monogamy to examine the impact of early rearing environment and later social experience on stress reactivity of male vs. female offspring. Prairie voles are one of the rare mammalian species that display socially monogamous relationships and biparental care in captivity and in nature (Getz et al., 1981; Oliveras and Novak, 1986; Terleph et al., 2004). Like humans, prairie voles are designated as a socially monogamous species because the adult male and female pairs show a preference to live together but do not mate exclusively (Carter et al., 1995; Strassmann, 2003; Solomon et al., 2004; Streatfeild et al., 2011). Laboratory studies have shown that when male prairie voles are given the opportunity to access multiple mates, they show a preference to mate with only one of them (Blocker and Ophir, 2016). Although pair-bonded males are attentive to the sensory stimuli of other sexually-receptive females (Parker et al., 2011; Rodriguez et al., 2013), when given a choice, they prefer to remain in contact with their mate rather than a novel female (Blocker and Ophir, 2016). This suggests that males may be predisposed to forgo a desire to seek other mates once they form a pair bond.
Nevertheless, observations of prairie voles under natural and semi-natural settings have shown that the behavior of both males and females is dynamic and can shift from exclusive mating with a single partner to occasional mating with a novel conspecific (Solomon and Jacquot, 2002; Ophir et al., 2008; McGuire and Getz, 2010). For example, wild male prairie voles are reported to switch their mating tactics from being residents that share a home range with a single female to being wanderers that visit multiple nests (Solomon and Jacquot, 2002; Ophir et al., 2008). Given that the reproductive advantage of monogamy vs. polygamy for prairie voles is equivocal (Mabry et al., 2011; Okhovat et al., 2015), it is unclear why some male voles choose a pair-living tactic while others do not and why some males switch their tactic. An insight into this conundrum might be found by examining the stress responses of voles when they encounter conspecifics and must decide to form a monogamous bond or to copulate outside the relationship. It is possible that stress reactivity of prairie voles affects their decision-making processes when exploring their mating options in unpredictable environments.
By varying the social and ecological conditions of the natal nest, we attempted to identify the early-life factors that might contribute to the predisposition of male and female voles for responding to stress. We hypothesized that the anxiety level shown by adult voles in presence of a conspecific would differ based on their sex, whether they were exposed to either social or ecological disruptions in their early-life environment, and if they experienced additional disruptions by being socially isolated as subadults later in life. Given that the hippocampus can be reshaped by stress throughout life (McEwen and Magarinos, 2001) and is a key brain region within the so called “social decision-making network” (Ophir, 2017), we examined the dendritic morphology of its neurons to determine the impact of disruptions experienced in early life superimposed on disruptions experienced in later life. As voles are social animals that sometimes live in groups and are raised by both parents (McGuire and Getz, 1995), we predicted that social disruptions would increase the anxiety levels of adult offspring more than ecological disruptions. Based on previous research in prairie and mandarin voles showing that females are more sensitive than males to manipulations of their social environment such as paternal deprivation and social isolation (Ruscio et al., 2009; Yu et al., 2012), we also predicted that our female subjects would be more susceptible to the impact of postpartum disruptions than males. Given the adverse effects of social isolation in voles (Scotti et al., 2015), we expected the early-life disruptions to have a greater negative effect on the stress reactivity and neuronal development of voles that were isolated as subadults than the socially-housed voles that remained with their same-sex sibling.
Materials and Methods
Subjects
The subjects were the F2 generation of prairie-vole breeding pairs that are maintained in the animal facility at Lehman College. The breeding colony was formed from descendants of wild-caught voles that were originally captured in east-central Illinois, U.S.A. We minimize inbreeding in our colony by monitoring the relatedness of males and females that we select for breeding. All voles in our facility are housed in standard clear plastic cages (48 × 27 × 20 cm) and supplied with nesting material to simulate their natural habitat. Voles are underground burrowers that occupy a variety of habitats including ungrazed pastures, hay fields, and alfalfa fields (Cole and Batzli, 1979; Mankin and Getz, 1994). They prefer to live in areas of thick rather than sparse vegetation; they use vegetation as both food and cover (Lin and Batzli, 2001). They are more likely to disperse from areas of no vegetative cover (Lin et al., 2006). Given that voles are considered as pests, a way to control their population is to mow their vegetative cover (Carter and Getz, 1993; O'Brien, 1994). To create a protective and familiar environment for them in the lab, we first place a layer of ~5 cm of moistened peat moss at the bottom of their cage then fill the cage to the top with straw. Water and food, consisting of a mixture of sunflower seeds, rabbit chow, and cracked corn are available at libitum. The colony animals are kept in rooms with fluorescent lighting and at temperatures around 20–25°C. The light:dark of the room is set at 14:10 with lights on at 6:00 a.m. The offspring of our breeding colony serve as experimental subjects. They are weaned at 20 days then transferred to a separate room where they are housed with their same-sex siblings under a protective cover of straw until adulthood. All experimental and stimulus animals used in the study were at least 60-days-old, were sexually inexperienced at the beginning of the experiment, were raised by both parents. They were removed from their natal nest before their mother gave birth to another litter.
All applicable national and institutional guidelines for the care and use of animals were followed. Animal care and all procedures performed were in accordance with the ethical standards of Lehman College and were approved by the Lehman College Institutional Animal Care and Use Committee.
Housing Manipulations
We used a total of 64 adult males and 64 adult females that we separated from their same-sex siblings to create four sets of breeding pairs (N = 16 pairs for each of four conditions), whose offspring served as our experimental subjects. The animals selected for breeding were between 60 and 120 days old. They were unrelated and unfamiliar to each other. Each male was held in a wire mesh cylindrical container (10 × 7.7 cm) with some food. The container was capped and placed in the cage of a female for 24 h, so the partners would become accustomed to one another's sensory stimuli. The male was then released into the female's cage and the pair was left undisturbed, except for cage cleaning, until the birth of their first offspring. At 6 days postpartum, the cages of the breeding pairs were manipulated to expose offspring to early-life disruptions that they may experience in nature such as loss of their father, protective cover or both their father and their protective cover. A total of 16 family units were left undisturbed and served as control (NoDisrupt). The NoDisrupt offspring were reared by both parents under a protective cover of straw. In the 16 family units whose ecological condition was disrupted (EcoDisrupt) the protective cover was permanently removed leaving the mother and the father to raise the young with only the peat moss at the bottom of the cage and a handful of loose straws. In the 16 family units whose social condition was disrupted (SocDisrupt), the father was permanently removed leaving the mother to raise the young under the protective cover of straw. In the 16 family units whose social and ecological conditions were disrupted (SocEcoDisrupt), the father and the protective cover were permanently removed leaving the mother to raise the young alone with only the peat moss at the bottom of the cage and a handful of loose straws. All family units were then left undisturbed until the offspring were 20 days of age. To expose offspring to later-life disruptions, the subadults at weaning were sexed and housed either with a same sex-same sibling (Social) or housed alone (Isolate). The Social and Isolate groups were housed under a protective cover of straw until adulthood.
Anxiety Testing
Between 60 and 70 days of age, the Social and Isolate adult offspring from each family unit and of each sex were tested for their anxiety level in an open-field arena for 3 consecutive days. The open-field test is a common procedure where behavioral and physiological reactions such as immobility, leaving the preferred periphery of the arena to enter the center or defecation and urination are regarding as increased anxiety (Prut and Belzung, 2003). A day before testing, all subjects were placed alone in a new cage. The cage holding the Social or the Isolate vole was transferred to a brightly-lit room where an open-field testing box was placed directly under the fluorescent light. The square box (72 × 72 × 36 cm) was made of four white plywood sides and a Plexiglas bottom that was marked to divide the area into 12 equally-sized squares (18 × 18 cm each). An extra square was drawn in the center of the box at the intersection of the four middle squares. The subject's cage was left on a cart for 20 min for the animal to acclimate to the testing room. Each subject was then gently picked up by an experimenter and released at the same corner of the testing arena. A video camera attached to the ceiling above the open-field arena was turned on a minute before testing began. After releasing the animal, the experimenter left the room. Each subject was video-taped for 10 min and its behavior was later scored for the duration of time spent in the side squares, in the middle squares, in the center square, locomotion, autogrooming, freezing (remaining stationary), and rearing (standing on hind legs) by experimenters blind to the testing conditions. On day 1, the subject was tested in an arena that was empty. On day 2, the subject was tested in an arena containing an unfamiliar and unrelated stimulus animal of the same sex. The stimulus animal was held in a capped wire-mesh cylindrical container (10 × 7.7 cm) that was placed in the center square and attached to the Plexiglas floor with Velcro. On day 3, the subject was tested in an arena containing an empty capped wire-mesh cylindrical container that was similarly placed in the center. The open-field test was designed to determine the animal's tendency for overcoming its anxiety and risk moving from the arena's sides and explore its center. Previous studies in our lab have shown that prairie voles do not attend to or explore empty containers unless these containers have a stimulus animal within them (Parker et al., 2011). To ensure that our subjects would have an incentive to explore the arena, we tested them over 3 days with and without a social stimulus. On day 2 and 3 of the behavioral testing sessions, we also measured the time subjects spent investigating the container that had a stimulus conspecific or remained empty. However, because we found no differences in the time spent in the center of the arena and the time spent investigating the container, we are only reporting the time in the center. Following each testing session, the animal was returned to its cage and the number of fecal boli and urine puddles were counted. The arena was cleaned to remove the excreta, sprayed with 70% alcohol to remove all odors, and allowed to dry in between testing sessions.
Brain Analysis
To determine how the brain is impacted by disturbances experienced early in life superimposed on those experienced later in life, we examined the dendritic morphology of neurons in the CA3 regions of the hippocampus of Isolate adult offspring that had experienced Social or Ecological disruptions in early life vs. the Social adult offspring that experienced no disruptions in early life. Following the behavioral tests, 16 brains from Isolate subjects (4 brains of each sex from the SocDisrupt and 4 brains of each sex from the EcoDisrupt groups) and 8 brains from Controls (4 brains of each sex from the NoDisrupt group that were socially housed after weaning) were placed in a Golgi-Cox solution. To stain the neurons, we followed the instructions in the SuperGolgi Kit by Bioenno LifeSciences (Bioenno Tech, LLC Santa Ana, California). After immersing the whole brain in Golgi solution then buffer, we sectioned it on a vibratome at 200 μm, mounted the sections, and completed the staining process on gel-coated slides. The slides were coded prior to image analysis with the Neurolucida morphometry software (MBF Bioscience). The images were acquired on a Zeiss Axiophot 2 microscope equipped with a motorized stage and a video camera, and the stained neurons were traced using a 40x/1.4 N.A., Plan-Apochromat oil immersion objective. An experimenter blind to the testing conditions measured the average length of the apical dendrites of selected neurons in the CA3 area identified on the rat atlas (Paxinos and Watson, 2013). Of the 24 brains that were processed, 3 did not stain clearly to be used in the analysis (1 male and 1 female brain from the EcoDisrupt group and 1 female brain from the SocDisrupt group). For the remaining animals, 3 adjacent neurons were selected from each hemisphere and analyzed for a total of 6 neurons per brain. The selected neuron had to satisfy two criteria: (1) reside within the pyramidal layer of the CA3 region of the designated hippocampus region; (2) be adjacent to each other.
Statistical Analysis
For all statistical analysis, we used the IBM SPSS Statistics software for Macintosh, Subscriptions. Prior to running the statistical analysis, we used the Shapiro-Wilk's test to determine if the data were normally distributed and used the Levene's test to check for homogeneity of variance. Some of the data did not pass those tests. The data that did not pass those tests were log, square-root or cube-root transformed. For the behavioral data, we used a three-way ANOVA with sex, group, and condition as between-subject variables to test for differences on each of the three testing days and used partial eta-squared (ηp2) as a measure of effect size. For the brain data, we used a two-way ANOVA with sex and conditions as between-subject variables. For significant P-values at <0.05, post-hoc analysis was conducted with the Holm-Bonferroni method. At weaning, a total of 102 offspring raised under four different conditions were assigned to the Social group and 93 were assigned to the Isolate groups (see Tables 1, 2 for sample sizes). We had missing data on Day 2 (a SocEcoDisrupt male) and on Day 3 (a NoDisrupt male). Also, the excreta of a EcoDisrupt female was not collected after the behavioral testing. The data for one NoDisrupt Isolate female were removed because they were outliers, and the data for one SocEcoDisrupt female could not be analyzed because the video-recordings were distorted. We had missing data on Day 2 (a NoDisrupt male and a SocDisrupt female) and on Day 3 (a SocDisrupt female and a SocEcoDisrupt male). Also, the excreta of one SocEcoDisrupt male was not collected after the behavioral testing. For differences in frequency of excreta by subjects within the testing arena, we first categorized the counts as High if there were more than 3 and as Low if there were <3 excrements then used Fisher's exact probability test to analyze the data for each testing day.
Results
Behavior in Open-Field Test
The three-way ANOVA results for the main effects are shown in Table 1 and for the interaction effects are shown in Table 2. There were no significant three-way interactions among sex, group, and conditions. However, for some of the behavioral measures on each testing day, we found significant main effects and two-way interactions.
On Day1, there were significant main and interaction effects for time spent on locomotion, autogrooming, freezing, and Rearing, but there were no differences in time spent on the Sides, Middle or Center of the arena. For locomotion and freezing, there was a significant group by condition effect. In the NoDisrupt condition, the Social subjects spent more time on locomotion (P = 0.004) and less time on freezing (P = 0.002) than the Isolate subjects. For autogrooming and rearing, there was a significant sex by group effect. Isolate females groomed themselves more (P = 0.0001) and spent less time on rearing (P = 0.004) than Isolate males.
On Day2, there were significant main effects for autogroom and time spent in the arena's center and middle. Females across groups and conditions groomed themselves more and spent less time in the center and middle sections of the arena than males. The NoDisrupt subjects had the highest mean for autogrooming that differed significantly with those in the SocEcoDisrupt condition (P = 0.04). For Freezing, there was a significant sex by condition effect. The SocDisrupt males spent less time freezing than the SocDisrupt females (P = 0.0001). For Rearing, there was a significant group by condition effect. In the SocDisrupt condition, the Isolate subjects spent more time on rearing than the Social subjects (P = 0.003). For time spent on the arena's sides, there was a significant sex by condition and a group by condition effect. Males spent much less time on the arena's sides than females in the SocDisrupt (P = 0.0001) and the SocEcoDisrupt (P = 0.001) conditions. Although males in the NoDisrupt group also showed a similar tendency (P = 0.05), those in the EcoDisrupt condition did not differ from females (Figure 1).
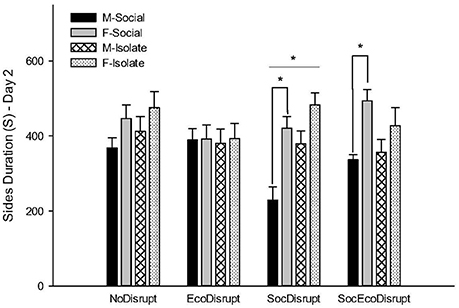
Figure 1. Time spent on the sides of the arena, close to the walls, by two groups of voles: adult males (M-Social) and females (F-Social) that were socially housed and adult males (M-Isolate) and females (F-Isolate) that were housed in isolation after weaning. The behavior shown was measured on Day 2 of testing when a same-sex conspecific was placed in the center of the field. The early-life conditions were NoDisrupt (both parents with a protective cover); EcoDisrupt (both parents without a protective cover); SocDisrupt (mother with a protective cover); SocEcoDisrupt (mother without a protective cover). The bars show means ± SE, *over the bars indicates significant sex differences at p < 0.05, and *over the bars indicates significant group differences at p < 0.05 for the SocDisrupt condition.
On Day3, there were significant sex differences in locomotion, freezing and rearing. Males were more active than females. They spent more time in locomotion and rearing and less time freezing than females. For Autogrooming, there was a significant sex by group effect. Isolate females spent more time grooming themselves than Isolate males (P = 0.0001). For time spent on the arena's sides and center, there were significant group by condition effect. In the EcoDisrupt group, the Isolate subjects spent more time in the center (P = 0.02) and less time in the arena's sides (P = 0.01) than the Social subjects.
The amount of excreta left in the arena on Day 2 of testing differed significantly in Isolate males (N = 50, P = 0.001, Fisher's exact test) and females (N = 41, P = 0.02, Fisher's exact test) but not in Social males (N = 52, P = 0.49) and females (N = 49, P = 0.40). Isolate males in the EcoDisrupt and SocDisrupt groups and Isolate females in the SocDisrupt groups urinated and defecated more in presence of a stimulus animal than voles on other days (Table 3).
Dendritic Morphology
For the length of the apical dendrites in the CA3 area of the hippocampus (Figure 2a), we found a significant effect of condition [F(2, 15) = 13.7, P = 0.001, ηp2 = 0.6]. The apical dendritic length in the pyramidal neurons was longer for the SocDisrupt (P = 0.001) and for the EcoDisrupt (0.02) than for the Control subjects (Figures 2b–d). There were no significant sex [F(1, 15) = 0.1, P = 0.341, ηp2 = 0.07] or interactions effects [F(2, 15) = 1.4, P = 0.29, ηp2 = 0.15].
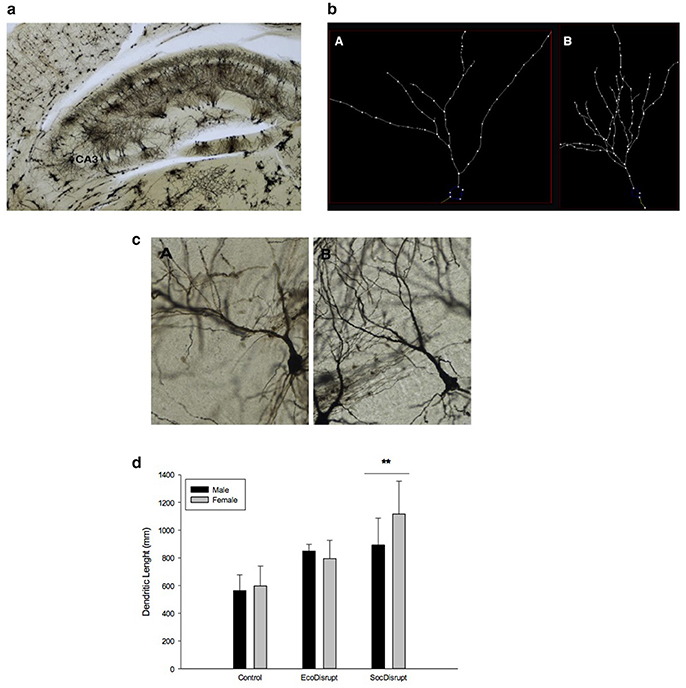
Figure 2. (a) Photomicrograph showing CA3 area of the hippocampus of a prairie vole prepared with Golgi Stain. (b) Reconstructed CA3 neuron in the hippocampus showing the apical dendrites in a representative animal from (A) Control and (B) SocDisrupt group. (c) Photomicrograph of the CA3 neuron in the hippocampus showing the apical dendrites in a representative animal from (A) Control and (B) SocDisrupt group. (d) Group differences in apical dendritic length of neurons in the CA3 region of the hippocampus. The conditions were Control (adult offspring raised by both parents under a protective cover and housed with a same-sex partner after weaning), EcoDisrupt (adult offspring raised by both parents without a protective cover and housed alone after weaning); SocDisrupt (adult offspring raised by mother alone with a protective cover and housed alone after weaning). The bars show means ± SE and **over the bars indicate significant group differences at p < 0.01.
Discussion
We disrupted the social and ecological rearing environment of prairie-vole offspring during the first week of life when rodent brains are rapidly growing and are susceptible to modifications by experience (Bandeira et al., 2009; Kundakovic and Champagne, 2015). The results were contrary to our predictions. Exposure to disruptions early in life had an anxiolytic effect, but the impact on males was greater than on females. Social disruption created by removal of the father, even in the absence of protective cover, was more effective in reducing anxiety than other conditions. The effect was noticeable in males on Day 2 of testing when a same-sex stimulus animal was placed in the center of the arena. Male offspring having experienced the loss of their father were more likely to risk moving from the arena's sides to the center for investigating a social object compared to males and females in other groups. Confronting the subjects with an empty container that was previously housed with a stimulus animal on Day 3 reduced the Social males' tendency to move away from the sides and investigate the inanimate object in the arena's center while increasing the Isolate voles' propensity to move to the center. Exposing the male and female voles to social isolation in early life increased the subjects' anxiety-related behaviors in adulthood, but the experience had a greater impact on females than on males. Social isolation enhanced the emotionality of both sexes in response to the social stimulus as evidenced by their increased defecation and urination on Day 2 and reduced the anxiolytic effect of social disruption in males. Although the Isolated voles showed sex differences in their behavior and appeared to be more anxious than social voles, examination of their dendritic morphology indicated that both social and ecological disruptions induced growth rather than retraction of dendrites in the hippocampus.
Our results are contrary to some of the previous research in monogamous rodents. In mandarin voles, removal of the father immediately after birth or separation of young from their littermates increased adulthood anxiety levels measured in an empty open-field testing arena (Jia et al., 2009). Exposure of mandarin voles to paternal loss as neonates also lowered their sociality, particularly in females. As adults, the subjects were less parental, less likely to investigate novel conspecifics, and the females were less likely to show partner preference (Jia et al., 2011; Yu et al., 2012; Cao et al., 2014). In California mice, removal of the father 3 days postpartum had no effect on the anxiety of the adult offspring tested in an empty open-field arena, but the subjects showed reduced social interactions and increased aggression (Bambico et al., 2015). In prairie voles, removal of the father a day after birth had no effect on the anxiety levels of adult offspring tested on the elevated plus maze but increased their social affiliation toward a conspecific (Tabbaa et al., 2017). However, with removal of the father 2 days before birth, the adult offspring displayed delayed partner preference and were less parental. They showed indications of reduced anxiety in an empty open-field testing arena and on an elevated plus maze (Ahern and Young, 2009; Ahern et al., 2011). Collectively, these studies suggest that social disruption created by the father's removal has a negative impact on sociality but a variable effect on stress reactivity. The stress results are understandable in light of a vast literature indicating that the outcome of early-life disruptions in mammals depends on multiple factors including its timing, severity, and testing procedure (Macrì et al., 2011; Chen and Baram, 2016). For example, there is evidence that moderate disruptions of the rearing environment produce offspring that are hyposensitive, whereas severe disruptions produce offspring that are hypersensitive to threats as adults (Macri and Wurbel, 2007; Coutellier et al., 2008). Furthermore, exposure to stress-induced changes during postnatal days 3–4 can result in hypersensitivity, whereas the same changes introduced on postnatal days 7–8 can result in hyposensitivity to stress later in life (Van Oers et al., 1998). By removing the father or the cover at postnatal day 6, we think we created a moderately stressful environment that prairie-vole family units may experience in nature as they occupy surface nests or because their protective cover might be reduced by mowing. When confronted with a conflicting situation as adults, only SocDisrupt males that were socially-housed had sufficiently reduced stress reactivity for overcoming their anxiety of open spaces to approach and investigate a stranger.
Multiple hypotheses may explain why we found the SocDisrupt males to show reduced anxiety following the loss of their father. It is possible that their mother altered her maternal behavior when we removed her partner on postpartum day 6. Many studies have found the mother to be the source of modifications in stress reactivity of offspring when disruptions to the maternal environment are introduced at critical points during development (Curley and Champagne, 2016). Laboratory studies in uniparental species such as rats and mice have shown that when the mother is confronted with foraging demands, removal of nesting materials or predator threats, she alters her nest attendance and active maternal care thus changing the activity of her offspring hypothalamic-pituitary-adrenal (HPA) axis in a sex-specific manner (Ivy et al., 2008; Coutellier et al., 2009; Mashoodh et al., 2009). Hence, it is possible that the behavior of prairie-vole mothers in our study altered her adult sons' stress responses. Although we did not record the mother's behavior following the father's removal, we think this is an unlikely scenario because previous studies under laboratory or semi-natural conditions in prairie and mandarin voles have shown that the mother does not compensate when the father is removed from the nest (McGuire et al., 2007; Jia et al., 2009; Ahern et al., 2011). However, because voles are biparental, it is possible that reduction in total overall care that is usually provided by both parents affected the sons' behavior later in life (Ahern and Young, 2009).
Alternatively, the sons themselves may have sensed and reacted to the changes in their social environment as we abruptly removed their father. Their father's absence may have caused a shift in how the HPA axis and the sympathetic nervous system balance the subjects' risk-taking tendencies in adulthood (Del Giudice et al., 2011). That change may have mobilized the voles in our study to move from the safety zones to the center of the arena where a social stimulus was placed. It is also plausible that the father's removal in our study altered the activity of the mother's HPA-axis, and that change was transferred to her young through lactation. Prairie voles form a pair bond shortly after mating, and separation of the partners increases their levels of plasma corticosterone (Bosch et al., 2009; McNeal et al., 2013). Rodent studies have shown that maternal corticosterone can be transferred to young via milk to affect regulation of HPA axis and behavior of the offspring (Casolini et al., 1997; Catalani et al., 2011). Unlike uniparental species in which only the mother creates the early social environment of the young, in biparental species such as prairie voles, the father also contributes to that social environment and his absence can have either a direct or an indirect effect on the offspring brain and behavior (McGuire et al., 2007; Ahern et al., 2011).
Disruptions in life can affect offspring not only in early phases of their lives but also during adolescence (Weintraub et al., 2010). There is evidence that exposure of subadults to social disruptions can alter their behavior so they can adapt to their environment (Sachser et al., 2011). To determine how later experience affects prairie vole offspring that were exposed to social or ecological disturbances, we isolated half of them and examined their behavior and hippocampal dendritic morphology in adulthood. Our behavioral results were consistent with past research showing that chronic isolation activates the subjects' sympathetic drive and enhances their anxiety and depression-like behaviors (Grippo et al., 2007; Ruscio et al., 2009). Our subjects showed signs of increased emotionality in presence of a social stimulus within the open-field arena and the male's mobilization to the center for investigating a conspecific was diminished following isolation.
However, we found changes in the length of hippocampal neurons that were contrary to our expectations. Previous research in a number of species have shown that hippocampal CA1-CA3 neurons are particularly susceptible to stress and atrophy in response to chronic stress including social isolation (McEwen, 1999; McEwen and Magarinos, 2001; Silva-Gómez et al., 2003). In our subjects, both males and females that had experienced either social or ecological disruptions as neonates and social isolation as juveniles displayed increases in the dendritic length of their CA3 neurons. However, our data are consistent with a recent study in California mice showing that social isolation enhances cell survival and proliferation in the hippocampus (Ruscio et al., 2015). Chronic social isolation in prairie voles also increase estrogen receptors in the medial amygdala (MEA) and the bed nucleus of stria terminalis (BST) of males, decrease corticotropin-releasing hormone (CRH) receptor type 1 but increase CRH receptor type 2 in the hippocampus (Pournajafi-Nazarloo et al., 2009; Perry et al., 2016). Given that CRH-R1 and CRH-R2 have been found to differentially modulate dendritic growth of hippocampal neurons (Sheng et al., 2012), it is possible that these receptors along with estrogen receptors may mediate alterations of the hippocampal neuronal structure in response to moderate environmental disruptions experienced during offspring development of monogamous species. Combined, the increased dendritic length of the hippocampal neurons and the anxiolytic effects of paternal absence on sons that we observed in prairie voles suggest that moderate stress during development may be adaptive. The experience might prepare offspring to counter stressful challenges such as the perils of exposure to predators or the risk of leaving the social group as ecological variables change and affect their decision to opt for a resident vs. a wanderer strategy (Solomon, 2003; McGuire and Getz, 2010).
The hippocampus has recently been proposed to be part of a socio-spatial memory circuit that is entangled with the pair-bonding network where neuropeptides such as vasopressin and oxytocin act to influence prairie vole's decision for remaining with a single partner, moving away to mate outside the relationship or adopting a non-monogamous mating tactic (Ophir, 2017). We have shown that disruptions of the neonatal environment at critical periods during development alters the anxiety profile of individuals to affect their risk-taking tendencies and enhance hippocampal neuronal growth. These changes in behavior and brain of voles in response to stress experienced during development may modify how they integrate socio-spatial information about the position of their mates and competitors in space and thus the decision to remain monogamous.
Author Contributions
OA-N designed and conducted the behavioral study. NR assisted with conducting the behavioral experiments. JD conducted the brain staining and analysis. SC, FA, and MC analyzed the behavioral data, MB oversaw the study and wrote the manuscript.
Conflict of Interest Statement
The authors declare that the research was conducted in the absence of any commercial or financial relationships that could be construed as a potential conflict of interest.
Acknowledgments
The study was funded by PSC-CUNY 67673-00 45 awarded to MB. The authors gratefully acknowledge Dr. Dara Dickstein at the Fishberg Department of Neuroscience, Icahn School of Medicine at Mount Sinai for her technical assistance in analyzing brain images.
References
Ahern, T. H., Hammock, E. A., and Young, L. J. (2011). Parental division of labor, coordination, and the effects of family structure on parenting in monogamous prairie voles (Microtus ochrogaster). Dev. Psychobiol. 53, 118–131. doi: 10.1002/dev.20498
Ahern, T. H., and Young, L. J. (2009). The impact of early life family structure on adult social attachment, alloparental behavior, and the neuropeptide systems regulating affiliative behaviors in the monogamous prairie vole (Microtus ochrogaster). Behav. Neurosci. 3, 1–19. doi: 10.3389/neuro.08.017.2009
Bambico, F. R., Lacoste, B., Hattan, P. R., and Gobbi, G. (2015). Father absence in the monogamous california mouse impairs social behavior and modifies dopamine and glutamate synapses in the medial prefrontal cortex. Cereb. Cortex 25, 1163–1175. doi: 10.1093/cercor/bht310
Bandeira, F., Lent, R., and Herculano-Houzel, S. (2009). Changing numbers of neuronal and non-neuronal cells underlie postnatal brain growth in the rat. Proc. Natl. Acad. Sci. U.S.A. 106, 14108–14113. doi: 10.1073/pnas.0804650106
Blocker, T. D., and Ophir, A. G. (2016). A preference to bond? Male prairie voles form pair bonds even in the presence of multiple receptive females. Anim. Behav. 122, 89–97. doi: 10.1016/j.anbehav.2016.10.007
Bosch, O. J., Nair, H. P., Ahern, T. H., Neumann, I. D., and Young, L. J. (2009). The CRF system mediates increased passive stress-coping behavior following the loss of a bonded partner in a monogamous rodent. Neuropsychopharmacology 34, 1406–1415. doi: 10.1038/npp.2008.154
Burkett, J. P., and Young, L. J. (2012). The behavioral, anatomical and pharmacological parallels between social attachments, love and addiction. Psychopharmacology 224, 1–26. doi: 10.1007/s00213-012-2794-x
Cameron, N. M., Fish, E. W., and Meaney, M. J. (2008). Maternal influences on the sexual behavior and reproductive success of the female rat. Horm. Behav. 54, 178–184. doi: 10.1016/j.yhbeh.2008.02.013
Cao, Y., Wu, R., Tai, F., Zhang, X., Yu, P., An, X., et al. (2014). Neonatal paternal deprivation impairs social recognition and alters levels of oxytocin and estrogen receptor α mRNA expression in the MeA and NAcc, and serum oxytocin in mandarin voles. Horm. Behav. 65, 57–65. doi: 10.1016/j.yhbeh.2013.11.005
Carter, C. S., DeVries, A. C., and Getz, L. L. (1995). Physiological substrates of mammalian monogamy: the prairie vole model. Neurosci. Biobehav. Rev. 19, 303–314. doi: 10.1016/0149-7634(94)00070-H
Carter, C. S., and Getz, L. L. (1993). Monogamy and the prairie vole. Sci. Am. 268, 100–106. doi: 10.1038/scientificamerican0693-100
Casolini, P., Cigliana, G., Alemà, G. S., Ruggieri, V., Angelucci, L., and Catalani, A. (1997). Effect of increased maternal corticosterone during lactation on hippocampal corticosteroid receptors, stress response and learning in offspring in the early stages of life. Neuroscience 79, 1005–1012. doi: 10.1016/S0306-4522(96)00668-9
Catalani, A., Alemà, G. S., Cinque, C., Zuena, A. R., and Casolini, P. (2011). Maternal corticosterone effects on hypothalamus-pituitary-adrenal axis regulation and behavior of the offspring in rodents. Neurosci. Biobehav. Rev. 35, 1502–1517. doi: 10.1016/j.neubiorev.2010.10.017
Chen, Y., and Baram, T. Z. (2016). Toward understanding how early-life stress reprograms cognitive and emotional brain networks. Neuropsychopharmacology 41, 197–206. doi: 10.1038/npp.2015.181
Clutton-Brock, T. H., and Parker, G. A. (1992). Potential reproductive rates and the operation of sexual selection. Q. Rev. Biol. 67, 437–456. doi: 10.1086/417793
Cohas, A., and Allainé, D. (2009). Social structure influences extra-pair paternity in socially monogamous mammals. Biol. Lett. 5, 313–316. doi: 10.1098/rsbl.2008.0760
Cole, F. R., and Batzli, G. O. (1979). Nutrition and population dynamics of the prairie vole, Microtus ochrogaster, in central illinois. J. Anim. Ecol. 48, 455–470. doi: 10.2307/4172
Coutellier, L., Friedrich, A. C., Failing, K., Marashi, V., and Würbel, H. (2009). Effects of foraging demand on maternal behaviour and adult offspring anxiety and stress response in C57BL/6 mice. Behav. Brain Res. 196, 192–199. doi: 10.1016/j.bbr.2008.08.042
Coutellier, L., Friedrich, A. C., Failing, K., and Würbel, H. (2008). Variations in the postnatal maternal environment in mice: effects on maternal behaviour and behavioural and endocrine responses in the adult offspring. Physiol. Behav. 93, 395–407. doi: 10.1016/j.physbeh.2007.09.008
Curley, J. P., and Champagne, F. A. (2016). Influence of maternal care on the developing brain: mechanisms, temporal dynamics and sensitive periods. Front. Neuroendocrinol. 40, 52–66. doi: 10.1016/j.yfrne.2015.11.001
Daskalakis, N. P., Bagot, R. C., Parker, K. J., Vinkers, C. H., and de Kloet, E. R. (2013). The three-hit concept of vulnerability and resilience: toward understanding adaptation to early-life adversity outcome. Psychoneuroendocrinology 38, 1858–1873. doi: 10.1016/j.psyneuen.2013.06.008
Del Giudice, M., Ellis, B. J., and Shirtcliff, E. A. (2011). The Adaptive Calibration Model of stress responsivity. Neurosci. Biobehav. Rev. 35, 1562–1592. doi: 10.1016/j.neubiorev.2010.11.007
DeVries, A. C., DeVries, M. B., Taymans, S. E., and Carter, C. S. (1996). The effects of stress on social preferences are sexually dimorphic in prairie voles. Proc. Natl. Acad. Sci. U.S.A. 93, 11980–11984. doi: 10.1073/pnas.93.21.11980
Dobson, F. S., Way, B. M., and Baudoin, C. (2010). Spatial dynamics and the evolution of social monogamy in mammals. Behav. Ecol. 21, 747–752. doi: 10.1093/beheco/arq048
Fraley, R. C., Brumbaugh, C. C., and Marks, M. J. (2005). The evolution and function of adult attachment: a comparative and phylogenetic analysis. J. Pers. Soc. Psychol. 89, 731–746. doi: 10.1037/0022-3514.89.5.751
Geary, D. C. (2000). Evolution and proximate expression of human paternal investment. Psychol. Bull. 126, 55–77. doi: 10.1037/0033-2909.126.1.55
Getz, L. L., Carter, C. S., and Gavish, L. (1981). The mating system of the prairie voles, Microtus ochrogaster: field and laboratory evidence for pair-bonding. Behav. Ecol. Sociobiol. 8, 189–194. doi: 10.1007/BF00299829
Keinan, G. (1987). Decision making under stress: scanning of alternatives under controllable and uncontrollable threats. J. Personal. Soc. Psy. 52, 639–644. doi: 10.1037/0022-3514.52.3.639
Grippo, A. J., Lamb, D. G., Carter, C. S., and Porges, S. W. (2007). Social isolation disrupts autonomic regulation of the heart and influences negative affective behaviors. Biol. Psychiatry 62, 1162–1170. doi: 10.1016/j.biopsych.2007.04.011
Henrich, J., Boyd, R., and Richerson, P. J. (2012). The puzzle of monogamous marriage. Philos. Trans. R. Soc. B Biol. Sci. 367, 657–669. doi: 10.1098/rstb.2011.0290
Hostetler, C. M., and Ryabinin, A. E. (2013). The CRF system and social behavior: a review. Front. Neurosci. 7:92. doi: 10.3389/fnins.2013.00092
Hyer, M. M., and Glasper, E. R. (2017). Separation increases passive stress-coping behaviors during forced swim and alters hippocampal dendritic morphology in California mice. PLoS ONE 12:e0175713. doi: 10.1371/journal.pone.0175713
Ikemoto, S. (2010). Brain reward circuitry beyond the mesolimbic dopamine system: a neurobiological theory. Neurosci. Biobehav. Rev. 35, 129–150. doi: 10.1016/j.neubiorev.2010.02.001
Ivy, A. S., Brunson, K. L., Sandman, C., and Baram, T. Z. (2008). Dysfunctional nurturing behavior in rat dams with limited access to nesting material: a clinically relevant model for early-life stress. Neuroscience 154, 1132–1142. doi: 10.1016/j.neuroscience.2008.04.019
Jia, R., Tai, F., An, S., Zhangb, X., Broders, H., et al. (2009). Effects of neonatal paternal deprivation or early deprivation on anxiety and social behaviors of the adults in mandarin voles. Behav. Process. 82, 271–278. doi: 10.1016/j.beproc.2009.07.006
Jia, R., Tai, F., An, S., and Zhang, X. (2011). Neonatal paternal deprivation or early deprivation reduces adult parental behavior and central estrogen receptor α expression in mandarin voles (Microtus mandarinus). Behav. Brain Res. 224, 279–289. doi: 10.1016/j.bbr.2011.05.042
Johnson, Z. V., and Young, L. J. (2015). Neurobiological mechanisms of social attachment and pair bonding. Curr. Opin. Behav. Sci. 3, 38–44. doi: 10.1016/j.cobeha.2015.01.009
Kappeler, P. M. (2013). Why male mammals are monogamous. Science 341, 469–470. doi: 10.1126/science.1242001
Kowalczyk, A. S., Davila, R. F., and Trainor, B. C. (2018). Effects of social defeat on paternal behavior and pair bonding behavior in male California mice (Permoyscus californicus). Horm. Behav. 98, 88–95.doi: 10.1016/j.yhbeh.2017.12.010
Kundakovic, M., and Champagne, F. A. (2015). Early-life experience, Epigenetics, and the developing brain. Neuropsychopharmacology 40, 141–153. doi: 10.1038/npp.2014.140
Lim, M. M., Wang, Z., Olazábal, D. E., Ren, X., Terwilliger, E. F., and Young, L. J. (2004). Enhanced partner preference in a promiscuous species by manipulating the expression of a single gene. Nature 429, 754–757. doi: 10.1038/nature02539
Lima, S. L. (1998). Stress and decision making under the risk of predation: recent developments from behavioral, reproductive, and ecological perspectives. Adv. Stud. Behav. 27, 215–290. doi: 10.1016/S0065-3454(08)60366-6
Lin, Y. K., Keane, B., Isenhour, A., and Solomon, N. G. (2006). Effects of patch quality on dispersal and social organization of prairie voles: an experimental approach. J. Mammal. 87, 446–453. doi: 10.1644/05-MAMM-A-201R1.1
Lin, Y. T. K., and Batzli, G. O. (2001). The influence of habitat quality on dispersal, demography, and population dynamics of voles. Ecol. Monogr. 71, 245–275. doi: 10.1890/0012-9615(2001)071[0245:TIOHQO]2.0.CO;2
Liu, D., Diorio, J., Day, J. C., Francis, D. D., and Meaney, M. J. (2000). Maternal care, hippocampal synaptogenesis and cognitive development in rats. Nat. Neurosci. 3, 799–806. doi: 10.1038/77702
Lukas, D., and Clutton-Brock, T. (2012). Life histories and the evolution of cooperative breeding in mammals. Proc. R. Soc. B Biol. Sci. U.S.A. 279, 4065–4070. doi: 10.1098/rspb.2012.1433
Lukas, D., and Clutton-Brock, T. H. (2013). The evolution of social monogamy in mammals. Science 341, 526–530. doi: 10.1126/science.1238677
Mabry, K. E., Streatfeild, C. A., Keane, B., and Solomon, N. G. (2011). Avpr1a length polymorphism is not associated with either social or genetic monogamy in free-living prairie voles. Anim. Behav. 81, 11–18. doi: 10.1016/j.anbehav.2010.09.021
Macrì, S., Zoratto, F., and Laviola, G. (2011). Early-stress regulates resilience, vulnerability and experimental validity in laboratory rodents through mother-offspring hormonal transfer. Neurosci. Biobehav. Rev. 35, 1534–1543. doi: 10.1016/j.neubiorev.2010.12.014
Macri, S., and Wurbel, H. (2007). Effects of variation in postnatal maternal environment on maternal behaviour and fear and stress responses in rats. Anim. Behav. 73, 171–184. doi: 10.1016/j.anbehav.2006.03.029
Mankin, P. C., and Getz, L. L. (1994). Burrow morphology as related to social organization of microtus ochrogaster. J. Mammal. 75, 492–499. doi: 10.2307/1382573
Mashoodh, R., Sinal, C. J., and Perrot-Sinal, T. S. (2009). Predation threat exerts specific effects on rat maternal behaviour and anxiety-related behaviour of male and female offspring. Physiol. Behav. 96, 693–702. doi: 10.1016/j.physbeh.2009.01.001
McEwen, B. S. (1999). Stress and hippocampal plasticity. Annu. Rev. Neurosci. 22, 105–122. doi: 10.1146/annurev.neuro.22.1.105
McEwen, B. S., and Magarinos, A. M. (2001). Stress and hippocampal plasticity: implications for the pathophysiology of affective disorders. Hum. Psychopharmacol. 16, S7–S19. doi: 10.1002/hup.266
McGuire, B., and Getz, L. L. (1995). Communal nesting in prairie voles (Microtus ochrogaster): an evaluation of costs and benefits based on patterns of dispersal and settlement. Can. J. Zool. 73, 383–391. doi: 10.1139/z95-042
McGuire, B., and Getz, L. L. (2010). Alternative male reproductive tactics in a natural population of prairie voles Microtus ochrogaster. Acta Theriol. 55, 261–270. doi: 10.4098/j.at.0001-7051.077.2009
McGuire, B., Parker, E., and Bemis, W. E. (2007). Sex differences, effects of male presence and coordination of nest visits in prairie voles (Microtus ochrogaster) during the immediate postnatal period. Am. Midl. Nat. 157, 187–201. doi: 10.1674/0003-0031(2007)157[187:SDEOMP]2.0.CO;2
McNeal, N., Scotti, M. A., Wardwell, J., Chandler, D. L., Bates, S. L., Larocca, M., et al. (2013). Disruption of social bonds induces behavioral and physiological dysregulation in male and female prairie voles. Auton. Neurosci. 180, 9–16. doi: 10.1016/j.autneu.2013.10.001
O'Brien, J. M. (1994). “Voles,” in Prevention and Control of Wildlife Damage, eds S. E. Hygnstrom, R. M. Timm, and G. E. Larson (Lincoln: University of Nebraska), B-177–B-181.
O'Connell, L. A., and Hofmann, H. A. (2011). The vertebrate mesolimbic reward system and social behavior network: a comparative synthesis. J. Comp. Neurol. 519, 3599–3639. doi: 10.1002/cne.22735
Okhovat, M., Berrio, A., Wallace, G., Ophir, A. G., and Phelps, S. M. (2015). Sexual fidelity trade-offs promote regulatory variation in the prairie vole brain. Science 350, 1371–1374. doi: 10.1126/science.aac5791
Oliveras, D., and Novak, M. (1986). A comparison of paternal behaviour in the meadow vole Microtus pennsylvanicus, the pine vole M. pinetorum and the prairie vole M. ochrogaster. Anim. Behav. 34, 519–526. doi: 10.1016/S0003-3472(86)80120-8
Ophir, A. G. (2017). Navigating monogamy: nonapeptide sensitivity in a memory neural circuit may shape social behavior and mating decisions. Front. Neurosci. 11:397. doi: 10.3389/fnins.2017.00397
Ophir, A. G., Phelps, S. M., Sorin, A. B., and Wolff, J. O. (2008). Social but not genetic monogamy is associated with greater breeding success in prairie voles. Anim. Behav. 75, 1143–1154. doi: 10.1016/j.anbehav.2007.09.022
Parker, J. T., Rodriguez, N., Lawal, B., Delevan, C. J., and Bamshad, M. (2011). Mating increases male's interest in other females: a cognitive study in socially monogamous prairie voles (Microtus ochrogaster). Behav. Process. 88, 127–134. doi: 10.1016/j.beproc.2011.08.010
Paxinos, G., and Watson, C. (2013). The Rat Brain In Stereotaxic Coordinates. 7th Edn. New York, NY: Academic Press.
Perry, A. N., Carter, C. S., and Cushing, B. S. (2016). Chronic social isolation enhances reproduction in the monogamous prairie vole (Microtus ochrogaster). Psychoneuroendocrinology 68, 20–28. doi: 10.1016/j.psyneuen.2016.02.016
Phelps, S. M., and Ophir, A. G. (2009). “Monogamous brains and alternative tactics: Neuronal V1aR, space use and sexual infidelity among male prairie voles,” in Cognitive Ecology II, eds R. Dukas, and J. Ratcliffe (Chicago, IL: University of Chicago Press), 156–176. doi: 10.7208/chicago/9780226169378.003.0009
Porcelli, A. J., and Delgado, M. R. (2017). Stress and decision making: effects on valuation, learning, and risk-taking. Curr. Opin. Behav. Sci. 14, 33–39. doi: 10.1016/j.cobeha.2016.11.015
Pournajafi-Nazarloo, H., Partoo, L., Sanzenbacher, L., Paredes, J., Hashimoto, K., Azizi, F., et al. (2009). Stress differentially modulates mRNA expression for corticotrophin-releasing hormone receptors in hypothalamus, hippocampus and pituitary of prairie voles. Neuropeptides 43, 113–123. doi: 10.1016/j.npep.2008.12.002
Prut, L., and Belzung, C. (2003). The open field as a paradigm to measure the effects of drugs on anxiety-like behaviors: a review. Eur. J. Pharmacol. 463, 3–33. doi: 10.1016/S0014-2999(03)01272-X
Reichard, U. H. (2003). “Monogamy: past and present,” in Monogamy, Mating Strategies and Partnerships in Birds, Humans and other Mammals, eds U. H. Reichard, and C. Boesch (Cambridge: Cambridge University Press), 3–25.
Rodriguez, N. A., Legzim, K. M., Aliou, F., Al-Naimi, O. A. S., and Bamshad, M. (2013). Does mating prevent monogamous males from seeking other females? A study in prairie voles (Microtus ochrogaster). Behav. Process. 100, 185–191. doi: 10.1016/j.beproc.2013.10.002
Ruscio, M. G., Bradley King, S., and Haun, H. L. (2015). Social isolation increases cell proliferation in male and cell survival in female California mice (Peromyscus californicus). Physiol. Behav. 151, 570–576. doi: 10.1016/j.physbeh.2015.08.039
Ruscio, M. G., Sweeny, T. D., Gomez, A., Parker, K., and Carter, C. S. (2009). Social environment alters central distribution of estrogen receptor alpha in juvenile prairie voles. Physiol. Behav. 98, 296–301. doi: 10.1016/j.physbeh.2009.06.005
Sachser, N., Hennessy, M. B., and Kaiser, S. (2011). Adaptive modulation of behavioural profiles by social stress during early phases of life and adolescence. Neurosci. Biobehav. Rev. 35, 1518–1533. doi: 10.1016/j.neubiorev.2010.09.002
Schacht, R., and Bell, A. V. (2016). The evolution of monogamy in response to partner scarcity. Sci. Rep. 6:32472. doi: 10.1038/srep32472
Scotti, M. A., Carlton, E. D., Demas, G. E., and Grippo, A. J. (2015). Social isolation disrupts innate immune responses in both male and female prairie voles and enhances agonistic behavior in female prairie voles (Microtus ochrogaster). Horm. Behav. 70, 7–13. doi: 10.1016/j.yhbeh.2015.01.004
Sheng, H., Xu, Y., Chen, Y., Zhang, Y., Xu, X., He, C., et al. (2012). CRH-R1 and CRH-R2 differentially modulate dendritic outgrowth of hippocampal neurons. Endocrine 41, 458–464. doi: 10.1007/s12020-012-9603-5
Silva-Gómez, A. B., Rojas, D., Juárez, I., and Flores, G. (2003). Decreased dendritic spine density on prefrontal cortical and hippocampal pyramidal neurons in postweaning social isolation rats. Brain Res. 983, 128–136. doi: 10.1016/S0006-8993(03)03042-7
Solomon, N. G. (2003). A reexamination of factors influencing philopatry in rodents. J. Mammal. 84, 1182–1197. doi: 10.1644/BLe-013
Solomon, N. G., and Jacquot, J. J. (2002). Characteristics of resident and wandering prairie voles, Microtus ochrogaster. Can. J. Zool. 80, 951–955. doi: 10.1139/z02-053
Solomon, N. G., Keane, B., Knoch, L. R., and Hogan, P. J. (2004). Multiple paternity in socially monogamous prairie voles (Microtus ochrogaster). Can. J. Zool. 82, 1667–1671. doi: 10.1139/z04-142
Strassmann, B. I. (2003). “Social monogamy in a human society: marriage and reproductive success among the Dogon,” in Monogamy, Mating Strategies and Partnerships in Birds, Humans and other Mammals, eds U. H. Reichard, and C. Boesch (Cambridge: Cambridge University Press), 177–189.
Streatfeild, C. A., Mabry, K. E., Keane, B., Crist, T. O., and Solomon, N. G. (2011). Intraspecific variability in the social and genetic mating systems of prairie voles, Microtus ochrogaster. Anim. Behav. 82, 1387–1398. doi: 10.1016/j.anbehav.2011.09.023
Summers, T. R., Summers, T. L., Carpenter, R. E., Smith, J. P., Young, S. L., Meyerink, B., et al. (2017). Learning and CRF-induced indecision during escape and submission in rainbow trout during socially aggressive interactions in the stress-alternatives model. Front. Neurosci. 11:515. doi: 10.3389/fnins.2017.00515
Tabbaa, M., Lei, K., Liu, Y., and Wang, Z. (2017). Paternal deprivation affects social behaviors and neurochemical systems in the offspring of socially monogamous prairie voles. Neuroscience 343, 284–297. doi: 10.1016/j.neuroscience.2016.12.011
Terleph, T. A., Jean-Baptiste, N., and Bamshad, M. (2004). Mechanisms and time course for induction of paternal behavior in prairie voles (Microtus ochrogaster). J. Mammal. 85, 1124–1129. doi: 10.1644/BNS-108.1
Van Oers, H. J., De Kloet, E. R., and Levine, S. (1998). Early vs. late maternal deprivation differentially alters the endocrine and hypothalamic responses to stress. Dev. Brain Res. 111, 245–252. doi: 10.1016/S0165-3806(98)00143-6
Weintraub, A., Singaravelu, J., and Bhatnagar, S. (2010). Enduring and sex-specific effects of adolescent social isolation in rats on adult stress reactivity. Brain Res. 1343, 83–92. doi: 10.1016/j.brainres.2010.04.068
Wittenberger, J. F., and Tilson, R. L. (1980). The evolution of monogamy: hypotheses and evidence. Annu. Rev. Ecol. Syst. 11, 197–232. doi: 10.1146/annurev.es.11.110180.001213
Young, K. A., Gobrogge, K. L., Liu, Y., and Wang, Z. (2011). The neurobiology of pair bonding: insights from a socially monogamous rodent. Front. Neuroendocrionol. 32, 53–69. doi: 10.1016/j.yfrne.2010.07.006
Young, L. J., and Wang, Z. (2004). The neurobiology of pair bonding. Nat. Neurosci. 7, 1048–1054. doi: 10.1038/nn1327
Keywords: paternal deprivation, early-life environmental disruptions, socio-spatial memory circuit, social monogamy, adolescence, social isolation, HPA-axis
Citation: Al-Naimi OAS, Delvalle JR, Carryl SS, Rodriguez NA, Aliou F, Cambi M and Bamshad M (2018) Socio-Ecological Disruptions at Critical Periods During Development Alter Stress Responses and Hippocampal Dendritic Morphology of Prairie Voles: Implications for Social Monogamy. Front. Ecol. Evol. 6:84. doi: 10.3389/fevo.2018.00084
Received: 28 December 2017; Accepted: 29 May 2018;
Published: 19 June 2018.
Edited by:
Nancy G. Solomon, Miami University, United StatesReviewed by:
Karen Lisa Bales, University of California, Davis, United StatesAngela J. Grippo, Northern Illinois University, United States
Copyright © 2018 Al-Naimi, Delvalle, Carryl, Rodriguez, Aliou, Cambi and Bamshad. This is an open-access article distributed under the terms of the Creative Commons Attribution License (CC BY). The use, distribution or reproduction in other forums is permitted, provided the original author(s) and the copyright owner are credited and that the original publication in this journal is cited, in accordance with accepted academic practice. No use, distribution or reproduction is permitted which does not comply with these terms.
*Correspondence: Maryam Bamshad, bWFyeWFtLmJhbXNoYWQtYWxhdmlAbGVobWFuLmN1bnkuZWR1