- 1Département de Biologie, Institut de Biologie Intégrative et des Systèmes, Université Laval, Québec, QC, Canada
- 2Production apicole, Centre de Recherche en Sciences Animales, de Deschambault, Québec, QC, Canada
- 3UFR Sciences du vivant - Université Paris Diderot, Paris, France
- 4Lallemand SAS, Blagnac, France
Honey bees (Apis mellifera) are facing multiple stressors affecting their lifespan, health and productivity. Among them, Nosema ceranae is an intracellular microsporidian parasite, which plays a major impact on honey bees colonies. However, both efficiency and innocuity of current treatment against N. ceranae are being questioned, thus raising the urgent need to develop alternative prophylactic and curative strategies. Endogenous microbial communities (i.e., host microbiota) are known to play a major role in disease prevention, and more recently both bacterial and yeast strains issued from gut microbiota were observed to improve hosts resistance against intracellular parasites both in mammals and insect models. The use of probiotics in honey bee nutrition is therefore promising to treat or prevent diseases. Therefore, further investigations are needed to properly select microorganisms with probiotic properties. In an in vivo experimental infection by N. ceranae, the probiotic effect of two honeybee gut bacterial strains (Parasaccharibacter apium (PC1 sp.) and Bacillus sp. (PC2 sp.)), and two broad spectra probiotics (Bactocell® and Levucell SB®) has been measured. Both curative and prophylactic administrations were tested: honey bees infected with N. ceranae and non-infected. For the four probiotic candidates, significant increases of survival probabilities (20–30%) were measured after two weeks of treatment with the administration of 103 CFU/mL in sugar syrup, both in curative and prophylactic treatments. The present study shows that endogenous bacterial strains were at least as much efficient and safe than broad spectra probiotics in increasing survival in the context of experimental infection with N. ceranae. Therefore, taking advantage of beneficial host microbiota properties is a promising avenue to develop efficient and sustainable curative strategies against opportunistic diseases in honey bee colonies.
Introduction
The European honey bee Apis mellifera is the most important pollinator present in the agricultural (Alberoni et al., 2016) environmental and economic sectors (Klein et al., 2017). Over the last few decades, major mortalities in honey bee colonies have been reported globally (Fairbrother et al., 2014; Vanegas, 2017). With the intensive exploitation of honey bees for the pollination of agricultural crops, bee colonies mortality is more accentuated in some area as Europe and US than in others part of the world. Although the stock of honey colonies is increasing, it fails to meet our actual needs (Aizen and Harder, 2009; Smith et al., 2014). Numerous studies agree that synergetic interactions between multiple abiotics (Doublet et al., 2014; Alburaki et al., 2015; Kakumanu et al., 2016; Poquet et al., 2016; Li et al., 2017; López et al., 2017) and biotics stressors (Goulson et al., 2015; Dussaubat et al., 2016) are involved as major causes of bee colonies decline (see also reviews by Fairbrother et al., 2014; Alberoni et al., 2016; Sánchez-Bayo et al., 2016; Klein et al., 2017). Synergies between many of these factors have a strong negative impact on immune defense (Nazzi et al., 2012; Di Prisco et al., 2013), metabolism (Koch and Schmid-Hempel, 2011; Dussaubat et al., 2013; Bordier et al., 2017), and on bees cognitive mechanisms (Klein et al., 2017).
Now, honey bee gastrointestinal microorganisms (i.e., gut microbiota) are known to play a critical role in regulating specific functions associated with metabolism and immune response (Evans and Lopez, 2004; Alberoni et al., 2016; Hyrsl et al., 2017). Microbial symbionts participate in various processes such as digestion of food (Koch and Schmid-Hempel, 2011), detoxification of harmful molecules, supply of essential nutrients, participation in the host's defense system (Lemaitre and Hoffmann, 2007; Hooper et al., 2012), and protection against pathogens and parasites (Evans and Lopez, 2004; Flint et al., 2012; Engel and Moran, 2013; Alberoni et al., 2016; Chaplinska et al., 2016). Although our understanding of mechanisms underlying interactions between the intestinal microbiota and parasites is still partial, it is widely acknowledged that the composition of the intestinal microbiota is a key factor in controlling infection dynamics (Berrilli et al., 2012). To this respect, members of the gut microbiota, including parasites, can be either beneficial, neutral or harmful to their host (Engel and Moran, 2013). Furthermore, according to the host physiological state, some strains can shift from opportunistic to infectious when the host is stressed (Boutin et al., 2013), and potentially favor parasite infections. Nosema ceranae is an obligate intracellular microsporidian parasite, which is the causative agent of one of the most prevalent honey bee disease, called nosemosis (Higes et al., 2008). Honey bee's infection occurs by proliferation of N. ceranae spores in the midgut after the incorporation of infected food (Ptaszynska et al., 2014) and spore accumulation is often associated with mortalities (Higes et al., 2007, 2008, 2009; Martín-Hernández et al., 2007).
Nosemosis disease is involved in physiological and behavioral perturbations in the honey bee colony (Leoncini et al., 2004; Goblirsch et al., 2013) like the disruption of the pheromone production, the ethyl oleate (EO). Ethyl oleate (EO) is involved in the behavioral maturation, in the transition from hive work to foraging activities (Leoncini et al., 2004; Dussaubat et al., 2010, 2013) and suppression of the cellular immune response (Antúnez et al., 2009). Nosemosis is also correlated with a modification of the natural feeding behavior of honey bees, making them less cooperative to participate in trophallaxis process (Naug and Gibbs, 2009). Nosema ceranae infection mainly impacts colony robustness (Cox-Foster et al., 2007; Antúnez et al., 2009): infected honey bees are more susceptible to other pathogens (Mayack and Naug, 2009).
Furthermore, spore accumulation is suspected to cause gut microbiota dysbiosis and to impair the defense system of the host (Alberoni et al., 2016). Dussaubat et al. (2012) showed that N. ceranae triggers inhibition of gene expression involved in the regeneration of the gut epithelial tissue (e.g., Wnt signaling pathway), which in turn could affect the ecological niche of beneficial host microbes. Gut epithelial tissue damages are symptoms of intestinal diseases, including diarrhea. Overall, intracellular intestinal protozoan parasites impact can be extremely damaging, especially in individuals who have weakened immune system (Vitetta et al., 2016). Given that modern agroenvironments exert a strong negative impact on immune defense, (Alaux et al., 2010; Di Prisco et al., 2013), it is not surprising that nosemosis prevalence is rapidly increasing (Higes et al., 2008).
Current treatment against nosemosis homologated in many countries (for example USA and Canada) is the antibiotics Fumagillin-B® and Fumidil-B® (Van Den Heever et al., 2015). Unfortunately, Nosema spp. strains are showing various levels of antibiotic-resistance (Huang et al., 2013) and several studies have measured reduced Fumagillin-B® efficacy on both N. apis and N. ceranae infections (Pajuelo et al., 2008; Williams et al., 2011). For this reason, Fumagillin-B® was recently banned in the European Union (Gisder and Genersch, 2015) like in France in 2002 (Fernandez and Coineau, 2007). Furthermore, antibiotics showed to disrupt host microbiota equilibrium by killing endogenous bacteria (Aguilera et al., 2013; Li et al., 2017). Moreover, antibiotics were observed to increase the susceptibility to Nosema infection, thus inducing a negative impact on the honey bee lifespan (Li et al., 2017).
Therefore, there is a need to develop effective and sustainable alternative strategies to maintain or restore intestinal flora homeostasis. Alternatively, beneficial microorganisms (i.e., probiotics) were successfully used as therapeutic tools in animal production (Hamdi et al., 2011; Alberoni et al., 2016). Oral administration of probiotics has showed a protective effect on the intestinal microbiota of the host (Gismondo et al., 1999). In murine models, both bacterial and yeast probiotic strains have also proven their effectiveness against intracellular parasites and showed positive improvement of the health of host (Alak et al., 1997, 1999; Benyacoub et al., 2005; Humen et al., 2005; Shukla et al., 2008; Dinleyici et al., 2011). Concerning honey bees, endogenous gut bacteria belonging to Lactobacilliaceae, Bifidobacteriaceae, and Acetobacteraceae families have been found to play an antagonistic role against N. ceranae, either in caged bee trial or in field conditions, by reducing the spore load (Sabaté et al., 2012; Audisio et al., 2015; Baffoni et al., 2016; Corby-Harris et al., 2016).
Our research goal focused in comparing the probiotic potential of two endogenous strains [Parasaccharibacter apium (PC1 sp.), Bacillus sp. (PC2 sp.)] and two commercial probiotics strains (Bactocell® and Levucell SB®, Lallemand Inc.) as an alternative treatment against N. ceranae infections in honey bees. These four probiotic candidates were targeted either for their proven or putative probiotic properties. The endogenous strains [P. apium (PC1 sp.) and Bacillus sp. (PC2 sp.)] are respectively closely and distantly related to strains that have been successfully used to inhibit honeybee disease (Sabaté et al., 2009; Yoshiyama and Kimura, 2009; Corby-Harris et al., 2016) and to enhance colony performance (Sabaté et al., 2012). Parasaccharibacter apium (PC1 sp.) belongs to the Acetobacteraceae family, which is particularly abundant in honey crop, hypopharyngeal glands, royal jelly, and larval gut through nurse worker bees feeding behavior (Corby-Harris et al., 2016). Overall, commensal Acetobacteraceae affect tissue development in insects such as in Anopheles mosquitoes (Chouaia et al., 2012; Mitraka et al., 2013) and control maturation of gut immunity, such as in Drosophila melanogaster (Ryu et al., 2008). Then, Bacillus sp. (PC2 sp.) belongs to the phylum Firmicutes and more specifically to the Bacillus genus. Bacillus species are dominant in the honeybee stomach (Wang et al., 2015). This genus can survive at high temperatures and pH and is usually harmless, with the exception of B. anthracis and B. cereus (Sabaté et al., 2012). Administration of Bacillus strains helped the development of bee colonies by enhancing the brood and also honey yield (Sabaté et al., 2012). Concerning the commercial strains, Bactocell® (Pediococcus acidilactici), is a Lactobacillaceae that is used for a wide range of host species: pigs, chickens, shrimps, and salmonids (EFSA, 2012). Bactocell® was demonstrated to improve the laying performance of hens (Denev et al., 2013), the survival rate, and the growth of the shrimp L. Stylirostris (Castex, 2009). Lactobacillacea strains are used as probiotics formulations in animals (Nikoskelainen et al., 2003; Bovera et al., 2012; Piccolo et al., 2015; Billiet et al., 2017) and in honey bees (Evans and Lopez, 2004). Interestingly, a decrease of Lactobacillaceae sp. was correlated with unhealthy honey bees (Cox-Foster et al., 2007; Olofsson and Vásquez, 2008), thus highlighting the important role of this bacterial family in honey bee health. Levucell®SB (Saccharomyces cerevisiae boulardii) is used for monogastrics (swine and chicken) to improve growth performance, gut histology (Le Bon et al., 2010), decrease carriage of foodborne pathogens (Mountzouris et al., 2015) and improve immunity response (Collier et al., 2011). Moreover, other studies showed positive impact of commercial probiotic on bee host (Kazimierczak-Baryczko and Szymas, 2006; Ptaszynska et al., 2016). Consequently, we decided to test the impact on honey bee health of two commercial strains, Bactocell® and Levucell®SB that showed beneficial effects on other animals.
The main objective of the present study was to compare the survival of young caged bees fed with two endogenous honey bee gut bacteria (PC2 and PC1) and two commercial probiotics (Bactocell®, Levucell SB®, two trademarks of Lallemand Inc.), experimentally infected by N. ceranae. This work is one of the very first studies comparing commercial probiotics, which usually have a broad host range, with honey bee endogenous strains, to assess whether beneficial members of the commensal gut microbial community perform better both in prophylactic and curative conditions. The motivation of this study is that probiotic curating strategies based on broad range commercial probiotic strains such as acid bacteria commercial strains such as L. rhamnosus and Lactobacillus sp. were not able to prevent nosemosis, and disrupted the honeybee immune system (Andrearczyk et al., 2014; Ptaszynska et al., 2016). Moreover, Andrearczyk et al. (2014) observed an increase of Nosema spp. infections in young honeybees fed with commercial Lactobacillus sp. and Saccharomyces cerevisiae probiotic strains. Finally, some cases of commercial probiotic induced microbiota-host perturbations were documented (Doron and Snydman, 2015; Durchschein et al., 2016). Taken together, this suggests that some broad range probiotics are not necessary suitable for the honey bees nutrition (Andrearczyk et al., 2014).
The present study shows that both endogenous bacterial strains were at least as much efficient and safe as broad spectra probiotics in significantly increasing survival in the context of experimental infection with N. ceranae.
Materials and Methods
Isolation and Culture of Honeybee Gut Bacteria
Sixty healthy adult worker honey bees were collected from five different colonies at the Centre de Recherche en Sciences Animales de Deschambault (CRSAD, Deschambault, Qc, Canada) and transported alive to the Institute for Integrative and Systems Biology (IBIS) in Quebec city, Canada. Upon arrival, whole bee guts were removed from the abdomen. Bee guts were then macerated, vortexed and placed into a sterile 10 mL Snap-cap tube (Sarstedt Inc. No. 62.554.002 PP*) filled with 5 mL of PBS 1X (Phosphate buffered saline). Gut bacterial community was prepared for culture by using several dilutions (10−1 to 10−4) from the starting volume of PBS 1X. Then, 100 μL of these different dilutions were transferred onto petri dishes of Tryptic Soy Broth agar (TSB) (Difco Inc.) and on De Man-Rogosa-Sharpe agar (MRS) (Difco Inc.), both incubated at 37°C for 48 h under aerobic conditions. After 48 h, individual colonies were picked and transferred to new agar plates to obtain pure strains. Each strain was transferred in a 2 mL cryotube for long-term storage by adding sterile glycerol to a final concentration of 15%; frozen samples were stored at −80°C for further molecular characterization and culture.
DNA Extraction and PCR Amplification of Endogenous Probiotic Strains 16S rRNA Gene
For DNA extraction, isolated strains were sampled from their specific media. Then, DNA was extracted using the Dneasy Blood and Tissues kit (Qiagen Inc., 2016) as indicated in the manufacturer's protocol with the following modifications: cultured strains were suspended in 200 μL of PBS 1X followed by a thermal shock at 95°C for 5 min. Next protocol steps were completed as indicated in the manufacturer's protocol (Pretreatment for Gram-Positive Bacteria In DNeasy® Blood & Tissue Handbook. 2006). For the PCR amplification, 16S rRNA gene was targeted using the universal bacterial primers (Sigma-Aldrich,Genosys) F-Tot (5′-GCAGGCCTAACACATGCAAGTC) and 1389R (5′-AGGCCCGGGAACGTATTCAC). The PCR was conducted in a total volume of 50 μL: H2O 18 μL; TaKaRa Taq 25 μL, F-tot (10 μM) 25 μL; 1389R (10 μM) 25 μL; DNA 2 μL. After initial denaturation at 95°C for 2 min, amplification was performed using 30 cycles of 1 min at 95°C, 1 min at 55°C and 1 min 30 at 72°C followed by a final extension at 72°C for 5 min. Then, amplification products were run on 2.0% agarose gels and sent to the “Plate-forme d'Analyses Génomiques” of Laval University for a Sanger DNA sequencing. Taxonomic identification of 16S rRNA gene sequences was completed using the BLAST sequence analysis tool of NCBI databases.
Survival of Endogenous and Commercial Probiotic Strains in Sugar Syrup
Endogenous bacterial strains used in this research were obtained from the glycerol stock (storage at −80°C) and streaked on Tryptic Soy Broth agar (TSB) or De Man-Rogosa-Sharpe agar (MRS) plates. Plates were incubated at 37°C for 48 h under aerobic conditions. After 48 h, 5–6 colony-forming unit (CFU) were inoculated in 10 mL of sugar syrup (1:1, w:v). In parallel, commercial probiotics were also inoculated in 10 mL of sugar syrup (1:1, w:v). Subsequently, 1 mL cultures of each solution (syrup sugar + CFU) were plated on MRS and TSA agar medium, depending on the bacterial strain, at T = 0, 48 h, 72 h, and 1, 2, 3 weeks in triplicate. All plates were incubated at 37°C for 48 h under aerobic conditions. Survival of two endogenous and two commercial probiotics in sugar syrup was measured by counting CFU after 2 days of incubation on their respective culture media.
Honey Bee Cages, Experimental Conditions, and Nosema spp. Infection
Experiments were conducted at the Centre de Recherche en Sciences Animales de Deschambault (CRSAD, Deschambault, 46°40′26.85″N, 71°54′54.39″W), Québec, Canada. The effect of endogenous and commercial probiotics against Nosema spp. infection was investigated in vivo on adult honeybees, maintained in cages under laboratory conditions. All bees used in this study originated from four European honeybee colonies (A. mellifera L.) headed by sister queens in September 2015. Accordingly to the methodology described by Williams et al. (2013), five combs of capped brood with dark eyes and gray skin pupae were transferred in a “nursery colony” that consisted of a Langstroth hive body with a frame of honey and pollen for food supply. To obtain newly emerging bees, the nursery colony was placed in an incubator (model 3040, Forma Scientific Inc., Ohio, U.S.A.) adjusted at 35°C and 55% relative humidity for 6 days. Young bees of 4–6 days old were placed in a 4 frame hive body before being randomly distributed to cages. Each cage consisted of a 14 oz. single-use plastic ventilated cup (Evans et al., 2009) and an inverted sterile syringe (20 mL, BD, Franklin Lakes, New Jersey, U.S.A.) containing sucrose syrup. Bees rapidly learned to take the syrup from the bottom opening of the syringe. Twenty bees were randomly distributed in each cage and all cages were kept in an environmentally controlled room (30°C ± 1°C and 50% ± 5% relative humidity) in total darkness for the duration of the experiment (27 days). Cages were randomly distributed in each experimental group (Table 1) and treatments begun on day 0. Each experimental group was composed of 20 cages. Five cages per experimental group were sampled each time. There are four treatment groups, one per probiotic strain: Bactocell®, Levucell SB®, PC1, and PC2. For each treatment group there are two conditions, curative vs. prophylactic administration, and two controls: with and without Nosema, therefore totalitizing 10 experimental groups.
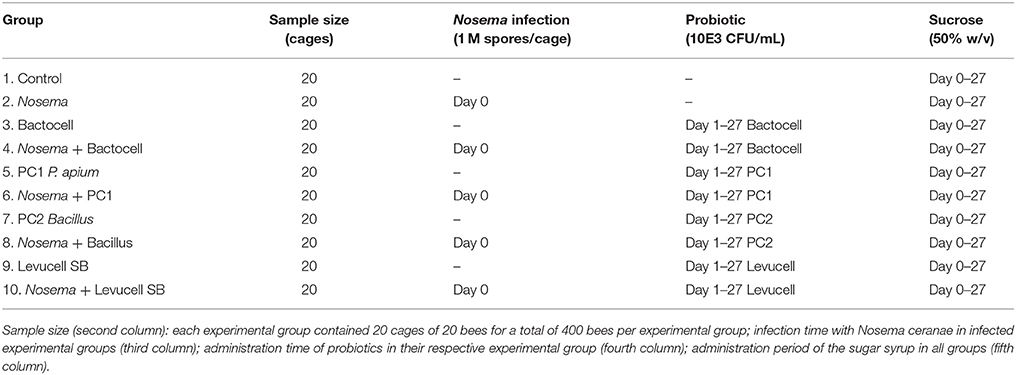
Table 1. Experimental groups (20 bees in each cage for a total of 400 bees in each experimental group).
To induce Nosemosis, N. ceranae spores were obtained directly before experiments from naturally infected honey bee ventriculus as described by Fries et al. (2013). To insure homogenous experimental infection of Nosema across experimental group, Nosema spore concentration in sugar syrup was checked three times before administration. To ensure that all bees had low Nosema infection level, we used newly emerged bees (4–6 days old) from healthy colonies, which were checked by PCR for Nosema prevalence. One micro liter of a sucrose syrup (1:1, w:v) containing 1 000 000 Nosema ssp. spores were administrated orally to Nosema group cages on day 0, with the inverted syringe. Following spore administration, endogenous or commercial probiotics were fed according to protocol with a dose of 103 CFU per mL. Commercial probiotics Bactocell® and Levucell® were supplied by Lallemand Inc. (Lallemand, 2016, Montréal, Québec, Canada) while endogenous probiotic candidates were isolated from healthy adult honeybee guts (as described in previous section). Each probiotic strain was mixed thoroughly in sucrose syrup (1:1, w:v). Sucrose solution was replenished weekly with a freshly made solution in all cages. Every 2 days, the number of dead bees was recorded in each cage to evaluate mortality rate in each group. On day 0, 50 bees were sampled to assess N. ceranae infection. Then, once a week until day 27, five cages per group were randomly sampled, and bee samples were stored at −80°C for further genomic analyses and evaluation of N. ceranae presence in honeybee gut.
Molecular Detection of Nosema
Total DNA for each sample (a pool of 5 bees per cage, 5 cages per experimental group) was extracted from intestinal honeybee using a rapid salt-extraction as described in (Aljanabi and Martinez, 1997) with some modifications. Intestinal tissues were removed from the bees stored at −80°C and placed into a 1.5 mL microtube. We added 440 μL of sterile salt homogenizing buffer (0.4 M NaCl 10 mM Tris–HCl pH 8.0 and 2 mM EDTA pH 8.0), 40 μL of 20% SDS and 8 μL of 20 mg/mL proteinase K (400 μg/mL final concentration) in the 1.5 mL microtube and vortexed for few seconds. Next, the samples were incubated at 60°C for at least 1 h and vortexed every 20 min. Then, 300 μL of 6 M NaCl was added to each sample followed by a centrifugation for 20 min at 13,300 rpm at 4°C. The supernatant was transferred in a new 1.5 mL microtube. After this step, 600 μL of cold isopropanol was added and incubated at −20°C for 30 min. Centrifugation was repeated before collecting the supernatant. This step was repeated twice, and the pellet was dried overnight. The dried pellet was suspended in 100 μL of sterile water and stored at 4°C. Then, N. ceranae was detected by PCR amplification. The Nosema gene marker was targeted using the primers (Sigma-Aldrich, Genosys), L203 (5′-CAGTTATGGGAAGTAATATTATATTG) and R253 (5′-TTGATTTGCCCTCCAATTAATCAC). The PCR was conducted in a total volume of 50 μL: Non- acetylated BSA (1 mg/mL) (20 μL; dNTPs (2.5 mM) (4 μL); Forward primer, L203 (3 μM) 2.5 μL; Reverse primer, R253 (3 μM) 2.5 μL; MgCl2 (25 mM) (2.5 μL); reaction buffer (10X) (Feldan); Taq (Feldan (10 U/μL) 25 μL, H2O 12.2 μL and DNA 1 μL. After initial denaturation at 94°C for 2 min, amplification was performed using 29 cycles of 45 s at 94°C, 45s at 56°C and 45s at 72°C followed by a final extension at 72°C for 5 min. Then, amplification products were run on 2.0% agarose gels for the detection of the specific 250 pb DNA fragments, together with a 100 pb DNA Ladder.
Molecular Detection of Endogenous Probiotic Candidates
Parasaccharibacter apium sp. (PC1) Detection
Total RNA for each sample (a pool of 5 bees per cage, 5 cages per experimental group) was extracted from intestinal honeybee using TRIzol® Reagent protocol from Invitrogen (Chomczynski, 1993) as described in Boutin et al. (2015). Complementary DNA (cDNA) was synthesized using qScript™ cDNA SuperMix (Quanta Biosciences). A total RNA of 0.5 μg was mixed with 4 μL qScript™ cDNA SuperMix and a variable volume of nuclease free water to obtain 20 μL as total volume, following the manufacturer's instructions (Quanta BioSciences, Inc. 2013). Then, standard PCR was conducted on Sigma (Biometra® T1 plus thermocycler, Montreal Biotech Inc.) PCR was carried out in a total volume of 12.5 μL: TaKaRa 6.25 μL; Primer M3_325_Fw (5′-GAAGCCGGCATCGTGGCCTG) (Sigma-Aldrich, Life Science) 1.25 μL; Primer M3_325_Rv (5′-ATGTACACGGCATCTGTCCA) (Sigma-Aldrich, Life Science)) 1.25 μL; cDNA template 1.875 μL and H2O 1.875 μL. After initial denaturation at 94°C for 1 min, amplification was performed using 41 cycles of 30 s at 94°C, 30 s at 60°C and 45 s at 72°C followed by a final extension at 72°C for 10 min.
Bacillus sp. (PC2) Detection
Detection was realized on genomic DNA, using extraction protocol as described in Aljanabi and Martinez (1997) followed by two successive DNA purifications and a standard PCR conducted on Sigma (Biometra® T1 plus thermocycler, Montreal Biotech Inc.) PCR was carried out in a total volume of 12.5 μL: TaKaRa 6.25 μL; Primer B3_339_Fw (5′-AAAAACTCGGTGGCGTAATG) (Sigma-Aldrich, Life Science) 1.25 μL; Primer B3_339_Rv (5′-TCAACACCTTTTAAGGGTGC) (Sigma-Aldrich, Life Science)) 1.25 μL; DNA template 1.875 μL and H2O 1.875 μL. After initial denaturation at 94°C for 1 min, amplification was performed using 26 cycles of 30 s at 94°C, 30 s at 60°C and 45 s at 72°C followed by a final extension at 72°C for 10 min. Then, amplification products were run on 2.0% agarose gels for both endogenous probiotic.
Quantification of Nosema ceranae Spore Loads in Honeybee Gut
For each experimental group, five bees from one cage were randomly sampled and pooled together to detect N. ceranae occurrence using an adapted protocol derived from Fries et al. (2013). In summary, we proceed as follow: All materials were cleaned with 70% ethanol. The five dissected abdomens were grinded into 5 mL of distilled water within a mortar using a pestle. Then, large debris were removed and mortar contents were poured into a 10 mL flacon tube and stored overnight at 4°C. Next, spore counting was performed using a hemocytometer grid. The hemocytometer grids were sterilized with 70% ethanol and a KimWipes™, then the preparation was homogenized manually by vortexing. Afterwards, a glass coverslip was put over the hemocytometer grid. Then, 20 μL of each pooled sample were placed into the hemocytometer. The hemocytometer was placed on an optical microscope equipped with a 400x magnification lens for observation. All the analyses were performed at day 14 (bees age = 18–20 days) of the experience. For the spore loads count, pools of 5 bees per cage were analyzed. Spore counting was performed in duplicate to reduce variability (Figure 1). Estimation of the number of spores per bee gut were calculated with the following formula: [(Raw spore count from 5 blocks) * 50.000)] = number of spores per bee.
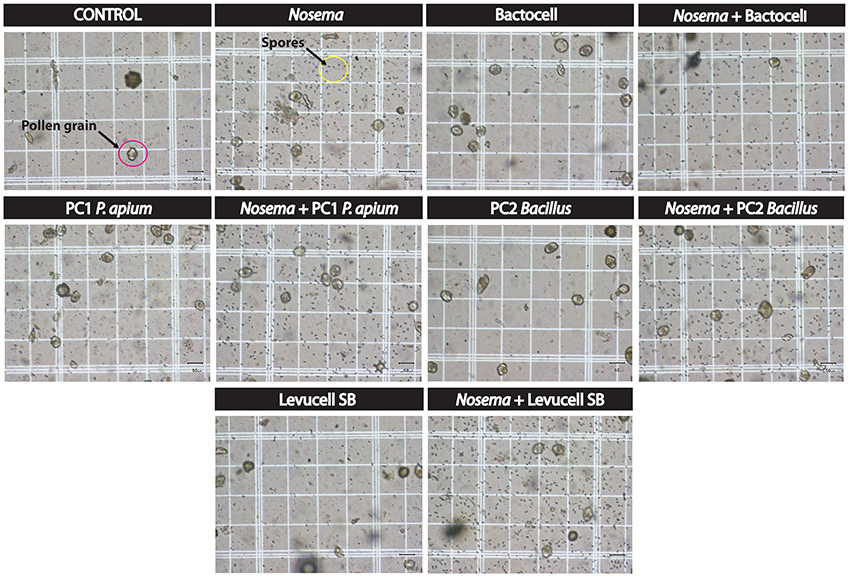
Figure 1. Estimation of the number of spore per bee in each experimental group: pictures were taken from an hematocytometer placed on an optical microscope equipped with a 400x magnification lens for observation. Five bees per experimental group were grinded together. For spore counting, we used 5 blocks (four blocks localized in the grid corner and the one in the 5 × 5 grid centrum). Each block represents 4 × 4 grids (16 squares). Pictures illustrated in this figure represent one of the smaller 4 × 4 grids (16 squares) from the 5 × 5 grids that are used for spore counting.
Statistical Analysis
Survival analysis of the in vivo experiment was performed with the Kaplan-Meier Method using R language (R Development Core Team, 2008). Kaplan-Meier analysis illustrates survival probabilities of bees between groups during the 27 days experiment by taking into account the censored data that represent sampling of cages during the in vivo experiment. Data mortality registered for each cage was formatted using script from our group and survival individuals were analyzed using “Survival” R package. Survival curves of multiple groups were obtained with the Kaplan-Meier (Kaplan and Meier, 1958) formula (fitted Cox model). Kaplan-Meier estimate generally assumes independence among the individual death events. In order to determine over time and for specific time if there is a statistically significant difference between control (non-infected group) vs. experimental group (curative and prophylactic groups) and within experimental groups we performed a Cox's proportional hazards regression using the coxph function in R. The hazard function or death rate is the instantaneous probability of death for individuals still alive. The Cox regression model estimates the hazard ratio of dying when comparing treatment vs. control (non-infected group). The P-values from the Cox model summary indicate the significance of differences between compared groups (Package survival, R).
Nosema prevalence was assessed by quantifying the number of N. ceranae spores per bee and per cup independently for each experimental group at day 14. The significance of differences between our collected data was evaluated by using a Variance test (test ANOVA) in R (Tables 2, 3).
A single factor analysis of variance (ANOVA) was performed to test whether significant differences in terms of survival differential occurred between prophylactic (i.e., probiotic vs. control) and curative (i.e., Nosema + probiotic vs. Nosema control) use of the four probiotic strains (Table 7).
Results
Survival of Endogenous and Commercial Probiotics in Sugar Syrup
We showed that the amount of CFUs for both endogenous and commercial probiotic candidates were stable after 3 weeks in the sugar syrup (1:1) (sugar:water), thus confirming that the syrup formulation was suitable for the administration of the four probiotics candidates. After 3 weeks, a ten-fold decrease of CFU counts was recorded for the four probiotic candidate strains. To be conservative, the syrup formulation (1:1) (syrup sugar + CFUs) used in this study was renewed weekly.
Nosema ceranae Spore Titers in the Experimental Groups
Nosema ceranae was unambiguously identified with the amplification of a taxonomic marker (See methods). At T0 (prior to Nosema infection), diagnostic PCR was negative. N. ceranae spores were recorded neither in control (non-infected group) (group 1) nor in probiotic controls (group 3, 5, and 9) at day 14. A minimal occurrence of spore was however observed in the Bacillus, probiotic candidate (group 7). Then, strong occurrences of N. ceranae spores were recorded in all of the infected groups (2, 4, 6, 8, and 10; See Tables 2, 3; Figure 2).
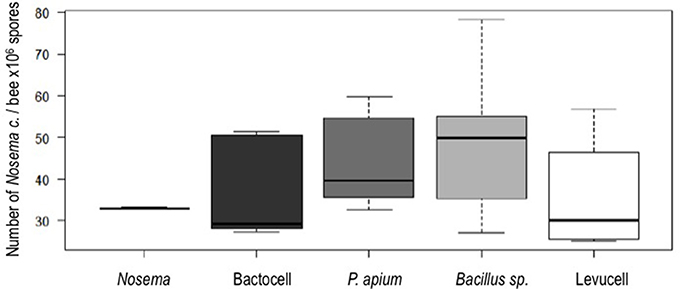
Figure 2. Spore counts per bee in the experimental groups infected with Nosema ceranae. Fifteen bees sampled at day 14 were analyzed (5 bees per experimental group, one cage per experimental group).
Honey Bee Survival
During this 27 days experiment, cumulative mortalities were recorded every 2 days and survival probabilities for each group were estimated by comparison of the Kaplan-Meier curves obtained and illustrated in Figure 3.
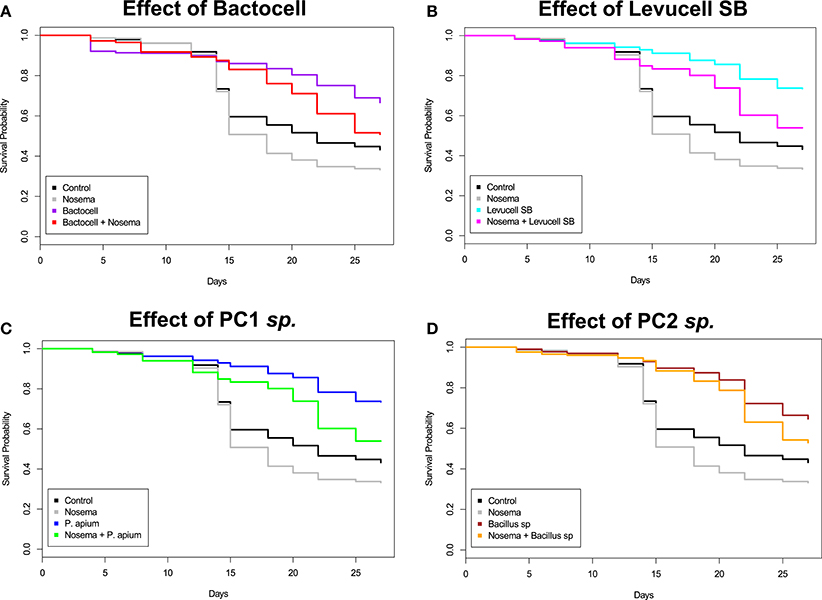
Figure 3. Kaplan-Meier Survival curves distribution of bees in each experimental group during the 27 days cage bee experiment. The y-axis represents the Kaplan-Meier estimates of the survival probabilities. The x-axis represents the experimental days. (A) Effect of Bactocell® administration (prophylactic: Bactocell® group and curative: Nosema + Bactocell) on honeybee survival. (B) Effect of Levucell® administration (prophylactic: Levucell group and curative: Nosema + Levucell) on honeybee survival. (C) Effect of PC1 sp. (Parasaccharibacter apium probiotic candidate) administration (prophylactic: PC1 sp. group and curative: Nosema + PC1 sp.) on honeybee survival (D) Effect of PC2 sp. (Bacillus probiotic candidate) administration (prophylactic: PC2 sp. group and curative: Nosema + PC2 sp.) on honeybee survival.
Effect of Nosema ceranae Infection on Honeybee Survival
Significant differences of survival probabilities between all groups were found from the second week of the experiment (day 8 to day 14) to the last day (day 27). Table 4 shows the Cox model comparisons of survival probabilities between control group (non-infected group) and all others groups. On day 25, all groups receiving probiotic candidates exhibited significantly higher survival rates compared to the control group (non-infected group; Table 4). Cox Proportional Hazard model comparisons showed that honey bees infected with N. ceranae had a significantly lower survival probability than bees from the control group (non-infected group) on day 18 (13.5% less), 25 (10.6% less), and 27 (9,5% less), evidencing the negative effect of N. ceranae infection on honey bee survival.
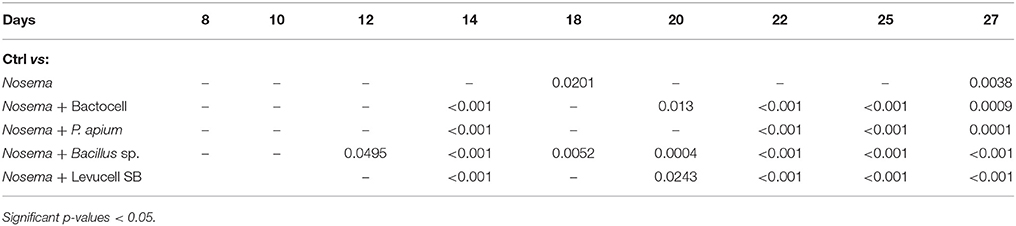
Table 4. Cox Proportional Hazard model (Cox-model) comparisons between survival of the control group (ctrl) and Nosema group and experimental groups with curative administration of tested probiotics during the 27 days experiment.
Prophylactic Administration of Commercial Probiotics (Bactocell® and Levucell SB®)
Overall, honey bees receiving a prophylactic administration of Bactocell® and Levucell SB® had a significantly higher survival probability than the non-infected control group (Cox-model, Figures 3A,B). From day 14 to day 27, survival probabilities of bees receiving a prophylactic dose of the commercial probiotic Bactocell® were significantly higher than those of control group bees receiving sucrose syrup. At day 18, bees have a significant higher survival probability (Kaplan-Meier curves, + 30%) than bees from the control group (54.2% ± 2.49 and 83.0% ± 1.89, respectively for control and Bactocell® group). Similarly, honey bees fed with the commercial probiotic Levucell SB® also showed a significantly higher survival probability when compared to the control group at day 8, 14, and from day 20 to 27 (Figure 3B, Table 5). On day 22, survival probability of bees receiving a prophylactic dose of the commercial probiotic Levucell SB® was 75.4% ± 2.15 compared to 45.5% ± 2.49) which is a significant increase of 29.9% of survival rate.

Table 5. Cox Proportional Hazard model (Cox-model) comparisons between survival of the control group (ctrl) and probiotic controls groups during the 27 days experiment.
Prophylactic Administration of Endogenous Probiotics
Overall, honey bees receiving a prophylactic administration of endogenous probiotics PC1 and PC2 had a significantly higher survival probabilities than bees of the non-infected control group, from the third week of the experiment (Table 5) to the last day (day 27). On day 22, honey bees receiving a prophylactic dose of PC1 had a significant increase of survival (Cox model, + 30%), when compared to survival of the control group (75.5% ± 2.09 compared to 45.5% ± 2.49). Prophylactic administration of the PC2 candidate also correlated with significantly higher survival probabilities on day 14 and 20 to 27 when compared to the control group. The highest survival difference was recorded at day 20: 83.0% ± 1.88 of bee receiving a prophylactic dose of the PC2 candidate survived, while 50.5% ± 2.5 of bees of the non-infected control group survived.
Curative Administration of Commercial Probiotics
Bees receiving a curative administration of Bactocell® after N. ceranae infection (test group 4) showed significant higher survival rates than control group (non-infected group) and Nosema infected control group bees from day 14 to the end of the in vivo experiment (Tables 4, 6, Figure 3A). On day 22, Kaplan-Meier curves indicate a survival rate of 34.2% ± 2.37 for Nosema control group and 60.5% ± 2.43 for the Nosema + Bactocell® test group (i.e., group receiving a curative dose of Bactocell®), representing a survival increase of 26.3%. Identically, Nosema + Levucell SB® test group showed significant higher survival rates than in Nosema control group. On day 14, survival rates were 71.0% ± 2.27 for the Nosema control group and 87.0% ± 1.68 for the Nosema + Levucell SB® test group, representing a survival increase of 16%. On day 22, bees from the Nosema + Levucell SB® test group had 75.4% ± 2.15 survival rate compared to 34.2% ± 2.37 for Nosema infected group, which is 41.2% higher.

Table 6. Cox Proportional Hazard model (Cox-model) comparisons between survival of the artificial Nosema infection groups (Nosema ctrl) and experimental groups with curative administration of tested probiotics during the 27 days experiment.
Curative Administration of Endogenous Probiotics
The survival probabilities of bees receiving a curative administration of the endogenous probiotic candidate PC1 after an artificial infection of N. ceranae (Nosema + PC1 test group, group 6) showed significant higher survival probabilities compared to Nosema control group bees (group 2) from day 14 to day 27 (Table 6, Figure 3D). Survival rates were significantly higher in the group 6 compared to group 2 from day 14 until the end of the experiment (32.7% ± 2.35 and 53.5% ± 2.49). Finally, infected bees receiving the endogenous probiotic candidate PC2 (Nosema + PC2 test group, group 8) showed a significant increase of survival from day 14 to day 27 (Table 6, Figure 3C), compared to Nosema control group. At day 27, a significant survival increase of 19.9% (52.6% ± 2.57% vs. 32.7% ± 2.35) was observed for the Nosema + PC2 test group.
Survival Differential Between Curative Groups and Prophylactic Groups
Significant differences regarding survival differential between prophylactic groups (i.e., probiotic vs. control) and curative groups (i.e., Nosema + probiotic vs. Nosema control) were detected from day 15 onwards. Survival differential between curative and prophylactic groups was significant for Levucell SB® at T15, T18, T20, T22, T25. For PC2, survival differential between curative and prophylactic groups was significant at T15, T18, T25. Finally, for Bactocell®, survival differential between curative and prophylactic groups was only significant at T25.
Discussion
The results clearly showed that both prophylactic and curative administration of all the four probiotics candidates, either with broad host range and endogenous, significantly increased honey bees survival rates from 20 to 40% when compared to the non-infected control group. For a prophylactic administration, highest survival rates were obtained for (in decreasing order): PC1, Bactocell®, Levucell SB®, and PC2. For the curative administration, highest survival rates were obtained for (in decreasing order): Levucell SB®, PC1, PC2 and Bactocell®. However, there was no significant difference in terms of survival improvement between commercial and endogenous probiotic candidates.
Regarding the patterns of survival rates, a drastic decrease was observed at day 14 in both control and Nosema control. From day 14 to the end of the trial (day 27), the Nosema control group survival was always lower than the control without Nosema, thus confirming that Nosema infection exerted a negative effect on survival. Furthermore, this drastic decrease did not occurred in the groups fed with probiotics, either with or without Nosema infection. This result strongly suggests that probiotics were very efficient to improve bee survival both in curative and prophylactic mode of action. Regarding the cause itself of this drastic survival decrease at day 14 in both control and Nosema control, we observed a similar pattern of sudden survival decrease in a preliminary trial (data not shown), although to a lower extent. We would hypothesize that maintaining honey-bees in cages fed without protein intake (i.e., pure 1:1 sugar syrup) triggered microbiota dysbiosis and in turn gut inflammation. In the probiotic fed groups, it is very likely that both anti-microbial effects and positive immune response stimulations, which were demonstrated for both Bactocell® and Levucell SB®, in alternative host organisms (Qamar et al., 2001; Standen et al., 2013), mitigated gut dysbiosis, thus increasing survival rate. Then, from day 15 onwards, we observed significant differences regarding survival differential between curative groups (i.e., Nosema + probiotic vs. Nosema control) and prophylactic groups (i.e., probiotic vs. control; Table 7). Basically, survival differential between curative and prophylactic groups varied from one experimental group to another, and from one sampling time to another. This result suggest that under curative use, at least three out of the four tested probiotic strains had a non-additive effect on survival relative to prophylactic use. Therefore, although it may suggest these three probiotic strains exert a specific effect on Nosema activity, further experiments are needed to univocally assess whether there is a direct functional interaction with the parasite.
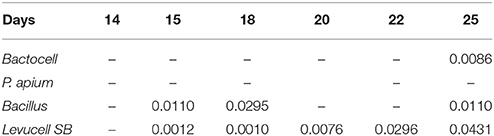
Table 7. ANOVA single factor analysis of variance was performed to test whether significant differences in terms of survival occurred between prophylactic and curative administration of probiotics (significant p-values < 0.05).
Finally, despite of the significant increase of survival rate in curative groups, no significant reduction of Nosema spores was observed in any of the four probiotic groups. At first sight, this result may be surprising as significant decreases of N. ceranae spore loads were recorded in previous studies: Baffoni et al. (2016) combined several strains of microorganisms isolated from the honeybee gut and found that both Bifidobacteriaceae and Lactobacilliaceae strains were correlated with a significant decrease of N. ceranae spore loads. Maggi et al. (2013) also reported a 52% reduction of N. ceranae spores per bee following application of the bacteria L. Johnsonii metabolites in caged bees. Finally, Corby-Harris et al. (2016) documented a significant reduction of Nosema spores following the administration of the endogenous strain P. apium. However, two key parameters from these previous studies are different from those of the present work, and could explain, at least partly, contrasted results in terms of spore reduction. First, relatively low titers (ranging from 1.103 to 1.104 spores per microliter of sugar syrup) of N. ceranae spores were administrated to caged honey bees to induce nosemosis (Maggi et al., 2013; Baffoni et al., 2016; Corby-Harris et al., 2016). Here, administrated N. ceranae spore titer was 100 to 1,000 times higher (1.106 spores per microliter of sugar syrup). Second, probiotic titers administrated in the present study were 100 to 1,000 times lower to previous studies (Baffoni et al., 2016; Corby-Harris et al., 2016). Interestingly, Corby-Harris et al. (2016) administrated a closely related strain of the endogenous symbiont P. apium at 2.106 CFU per mL, which is two thousand times higher than what was administrated in the present work. Despite of this, N. ceranae titers remained high enough, that the statistically significant reduction of spores recorded in those previous studies was not biologically relevant (Alberoni et al., 2016) to clearly reflect an antagonistic effect on nosemosis, as reduction of spore load did not exceeded 40%, when compared to infected control group. Indeed, workers from infected colonies exhibited mean nosema counts ranging from 0.04 to 1.87.106 spores per bee (Traver and Fell, 2011). In the caged bee trial of Corby-Harris et al. (2016) however, the probiotic fed group housed a mean of 951.106 spores per bee. Also, no survival improvement was recorded in all experimental groups (Corby-Harris et al., 2016). This is not surprising when considering our results as significant differences in survival were observed after 14 days, whereas the duration of the challenge with Nosema spores in Corby-Harris et al. (2016) was 10 days. A peak of bee mortality at day 14 post infection was previously observed (Dussaubat et al., 2012) thus corroborating our results regarding N. ceranae infection dynamics.
More importantly, the probiotic feed additive administration protocol used by Corby-Harris et al. (2016) was quite different from the present study. Corby-Harris et al. (2016) administrated P. apium probiotic strain (2.106 CFUs) during 7 weeks into the pollen patty on colony overwintering, and thus, prior to the cage bee trial. In our experiment, probiotics strains (1.103 CFUs per mL) were administrated via sugar syrup during the cage trial. Then, in the caged bee trial, young honeybees had ad libitum access to unsterilized pollen patty. Such protocol could have further improved overall survival, by providing bees with both proteins and other bacterial strains. Furthermore, Corby-Harris et al. (2016) used young spring bees from the overwintering hives for their cages experience, whereas we used winter bees. Taken together, the higher load of Nosema spores combined with lower concentration of probiotic candidates and the lack of protein supply in the present work, likely explain the absence of spore load decrease. However, all the four probiotic candidates significantly improved bee survival by 20–40%. Although it is yet not possible to state whether probiotic effects could directly or indirectly interfere with Nosema functional activity, it is reasonable to hypothesize that probiotic effect allows honey bee to better tolerate high loads of Nosema spores. Functional interaction of N. ceranae with honey bee was essentially investigated by focusing on enzyme regulation involved in detoxification process, immune response, and the metabolism activity. At the gene-expression level, honey bee midgut responded to N. ceranae infection by increasing oxidative stress pathway with the concurrent synthesis of antioxidant, defense, and protective molecules (Dussaubat et al., 2012; Di Pasquale et al., 2013). At the proteomic level, differential abundance of proteins associated to metabolism, response to oxidative stress, and apoptosis were measured between sensitive and resistant honey bees (Kurze et al., 2016). Other studies focused on other genetic mechanisms such as microRNA, which were suspected to regulate host honey bee metabolism related genes in response to N. ceranae infection (Huang et al., 2015, 2016). Overall, honey bee health is a complex system resulting from a combination of functional interacting networks between the bee host, the symbiotic gut microorganisms (Anderson et al., 2011) and the pathogens (Schwarz et al., 2015). To this respect, symbiotic gut microorganisms play a central role in modulating energy reserves and immune system development, which are key actors for host defenses against parasites (Habtewold et al., 2017; Knutie et al., 2017). Immune resistance mechanisms can either prevent parasites and pathogens from invading or eliminate them after invasion. Contrastingly to resistance mechanisms, immune tolerance mechanisms primarily involve both a lowered responsiveness to a given immune stimulus, and the initiation of tissue repair processes to protect the host from damage caused by the pathogen (Habtewold et al., 2017). Honey bee tolerance regarding high N. ceranae spores was documented in Huang et al. (2012), where a lower mortality rates were correlated to an up-regulated immune response in tolerant honey bee colonies day 6 post infection, when compared to control groups. Therefore, a balance between immune resistance and tolerance mechanisms ensures protection from parasites and/or pathogens and, at the same time, limits the tissue inflammation that may arise as a result of excessive immune effector production. As it is now suspected that host microbiota is fully involved in both resistance and tolerance mechanisms (Vitetta et al., 2016), it is worth reviewing known prophylactic and curative effects of both commercial probiotic strains tested in the present study. Commercial strains are administrated to a wide panel of host organisms as food additives to increase zootechnical performances by improving both metabolism and immune response (Denev et al., 2013; Schoster et al., 2013; Hou et al., 2015). To this respect, Bactocell® (P. acidilactici) was demonstrated to exert a protective action by stimulating the intestinal mucosal cells activity inducing a positive response of the immune system of the tilapia (Oreochromis niloticus; Standen et al., 2013). Overall, administration of lactic acid bacteria (LAB) acts as an inhibitor of bacterial infection propagation by activating the host immune system (Servin, 2004). Interestingly, it was observed in mammals that administration of LAB has the potential to alter gut microbial diversity, affect intestinal barrier function, and modulate innate immune response (e.g., via NF-κB pathway regulation) by increasing mucosal barrier function, thus leading to a decrease in translocation of bacteria and a subsequent decrease in the ability of pathogenic bacteria to attach to the gut mucosa (Reiff and Kelly, 2010). As insects and mammals share the same NF-κB mediated induction of innate immunity (Hoffmann et al., 1999), one of the putative mechanism underlying survival improvement would be that Bactocell® (P. acidilactici) may exert an effect on the honey bee immune system itself rather than directly interfere with Nosema activity. Then, other mechanism favoring honey bee survival may be involved. Lactic Acid bacteria (LAB) produce active antimicrobial molecules such as bacteriocins, antibiotics, hydrogen peroxide, to name a few. Those excreted molecules can control growth and/or survival of surrounding microorganisms, including viruses, bacteria, yeast, fungi, and protozoan parasites (Cleusix et al., 2007). Also, by lowering the local intestinal pH with lactic acid, LAB are able to inhibit growth of acid-sensitive organisms (Wohlgemuth et al., 2010). Saccharomyces cerevisiae boulardii strains, to which belong Levucell SB®, are nonpathogenic yeast which have proven experimental effectiveness in preventing and curating diseases with a predominant inflammatory component, thus indicating this probiotic might interfere with inflammatory induced cellular signaling pathways (Palma et al., 2015). Saccharomyces cerevisiae boulardii strains have a broad anti-pathogenic action, due to its outer surface molecular profile, which attaches to pathogenic bacteria such as Escherichia coli and Salmonella. Furthermore, S. cerevisiae boulardii is able to effectively compete with and displace parasitic yeast strains such as Candida (Tomičić et al., 2016). S. cerevisiae boulardii also produces anti-fungal substances such as capric, caprylic, and caproic acids, which were proven to inhibit pathogenic yeast growth in its close environment. Finally, S. cerevisiae boulardii promotes the secretion of immune molecules (e.g., IgA), which decrease the action of pro-inflammatory cytokines pathways generated in response to pathogenic bacteria, toxins and antigens (Qamar et al., 2001). Although little is known about the efficacy of S. cerevisiae boulardii against protozoan parasites, three studies documented a significant effect of this probiotic against amoebiasis (Dinleyici et al., 2009), giardiasis (Besirbellioglu et al., 2006), and infection with Blastocystis hominis (Dinleyici et al., 2011). Finally, Lee et al. (2007) documented the combination of P. acidilactici and S. cerevisiae boulardii on coccidiose. However, the molecular mechanism remains to be characterized.
Overall, experimental studies on both bacterial and yeast probiotic action against parasites involving the secretion of an active principle that can inhibit parasite development was only documented for Cryptosporidium, Giardia, and Eimeria, even though the molecular nature of the active component is still to identify (Travers et al., 2011).
Conclusion
This study confirms the potential of endogenous strains isolated from the honey bee as efficient probiotic candidates to improve honeybee survival. Then, we can conclude that the mortality of honey bees was not directly related to N. ceranae spore loads. Furthermore, as the experiment duration was longer enough to cover up to two parasite life cycles (6 days), it strongly suggests that all of the four probiotic strains did not exert direct antagonism effect on the parasite development. Our results, combined with the previous documented probiotic effects of strains related to our four candidates, suggest that mechanism of action is more related to tolerance, rather than resistance against the parasite, potentially by improving the immune system and tissue repair processes to protect the host from damage caused by the parasite. Also, our results, combined with the other experimental infection trials with N. ceranae suggest that both Nosema inoculated titers and probiotic strains concentrations have to be taken into account to properly interpret results, as different mechanisms of tolerance or resistance are potentially involved. Finally, these encouraging preliminary results for the four probiotic candidates were obtained in vivo with minimal nutritional inputs (e.g., no proteins); therefore in situ validation is necessary to fully assess the potential of these probiotic candidates as sustainable preventive and curative tools against nosemosis.
Extended studies are definitely needed to understand the mechanisms underlying the functional interaction between the honey bee, its microbiota, the probiotic strain and the parasite. The metatranscriptomic approach, which consists in our case to measure the gene expression level of all organisms inhabiting the honey bee gut microbiome, including host epithelial cells, is straightforward to better understand the molecular mechanisms underlying the improvement of honey bee survival.
Author Contributions
PG and ND wrote the grant, conceived the experiments, supervized all the experiments, and wrote the manuscript; SE performed data analyses, bacterial cultures, endogenous strains molecular identification, and wrote the manuscript; AR performed the caged bee trials and wrote the manuscript; AL performed Nosema spore counts and statistical analyses; BC supervized statistical analyses; SB performed bioinformatic analyses to identify endogenous probiotic strains and designed primers for PCR detection; VD and MC provided their expertise in probiotic development along the project and co-write the manuscript; P-LM was involved in every steps of molecular biology protocols (bee dissection, DNA extraction, PCR, sequencing) and probiotic cultures.
Funding
This work was funded with the Agri-Innovation programme from Agriculture and Agri-Food Canada. Grant number: 14-AP-247.
Conflict of Interest Statement
The authors declare that the research was conducted in the absence of any commercial or financial relationships that could be construed as a potential conflict of interest.
Acknowledgments
Authors are grateful to Agriculture and Agri-Food Canada (AgriInnovation program PAI-P199) and Lallemand Inc. for funding this study, as well as the Centre de recherche en sciences animales de Deschambault, Laval University and the Institute for Integrative and Systems Biology (IBIS). Authors would also like to thank Amélie Bégin, Pierre Mermoz for laboratory assistance.
References
Aguilera, M., Vergara, P., and Martínez, V. (2013). Stress and antibiotics alter luminal and wall-adhered microbiota and enhance the local expression of visceral sensory-related systems in mice. Neurogastroenterol. Motil. 25, e515–e529. doi: 10.1111/nmo.12154
Aizen, M. A., and Harder, L. D. (2009). Geographic variation in the growth of domesticated honey bee stocks: disease or economics? Commun. Integr. Biol. 2, 464–466. doi: 10.4161/cib.2.6.9258
Alak, J. I., Wolf, B. W., Mdurvwa, E. G., Pimentel-Smith, G. E., and Adeyemo, O. (1997). Effect of Lactobacillus reuteri on intestinal resistance to Cryptosporidium parvum infection in a murine model of acquired immunodeficiency syndrome. J. Infect. Dis. 175, 218–221 doi: 10.1093/infdis/175.1.218
Alak, J. I., Wolf, B. W., Mdurvwa, E. G., Pimentel-Smith, G. E., Kolavala, S., Abdelrahman, H., et al. (1999). Supplementation with Lactobacillus reuteri or L. acidophilus reduced intestinal shedding of cryptosporidium parvum oocysts in immunodeficient C57BL/6 mice. Cell. Mol. Biol. 45, 855–863.
Alaux, C., Ducloz, F., Crauser, D., and Le Conte, Y. (2010). Diet effects on honeybee immunocompetence. Biol. Lett. 6, 562–565 doi: 10.1098/rsbl.2009.0986
Alberoni, D., Gaggia, F., Baffoni, L., and Di Gioia, D. (2016). Beneficial microorganisms for honey bees: problems and progresses. Appl. Microbiol. Biotechnol. 100, 9469–9482. doi: 10.1007/s00253-016-7870-4
Alburaki, M., Boutin, S., Mercier, P.-L., Loublier, Y., Chagnon, M., and Derome, N. (2015). Neonicotinoid-coated zea mays seeds indirectly affect honeybee performance and pathogen susceptibility in field trials. PLoS ONE 10:e0125790. doi: 10.1371/journal.pone.0125790
Aljanabi, S. M., and Martinez, I. (1997). Universal and rapid salt-extraction of high quality genomic DNA for PCR-based techniques. Nucleic Acids Res. 25, 4692–4693. doi: 10.1093/nar/25.22.4692
Anderson, K. E., Sheehan, T. H., Eckholm, B. J., Mott, B. M., and DeGrandi-Hoffman, G. (2011). An emerging paradigm of colony health: microbial balance of the honey bee and hive (Apis mellifera). Insectes Sociaux 58, 431–444. doi: 10.1007/s00040-011-0194-6
Andrearczyk, S., Kadhim, M. J., and Knaga, S. (2014). Influence of a probiotic on the mortality, sugar syrup ingestion and infection of honeybees with Nosema spp. under laboratory assessment. Medycyna Weterynaryjna 70, 762–765.
Antúnez, K., Martín-Hernández, R., Prieto, L., Meana, A., Zunino, P., and Higes, M. (2009). Immune suppression in the honey bee (Apis mellifera) following infection by Nosema ceranae (Microsporidia). Environ. Microbiol., 11, 2284–2290. doi: 10.1111/j.1462-2920.2009.01953.x
Audisio, M. C., Sabaté, D. C., and Benítez-Ahrendts, M. R. (2015). Effect of Lactobacillus johnsonii CRL1647 on different parameters of honeybee colonies and bacterial populations of the bee gut. Benef. Microbes 6, 687–695. doi: 10.3920/BM2014.0155
Baffoni, L., Gaggìa, F., Alberoni, D., Cabbri, R., Nanetti, A., Biavati, B., et al. (2016). Effect of dietary supplementation of Bifidobacterium and Lactobacillus strains in Apis mellifera L. against Nosema ceranae. Beneficial Microbes. 7, 45–51. doi: 10.3920/BM2015.0085
Benyacoub, J., Perez, P. F., Rochat, F., Saudan, K. Y., Reuteler, G., Antille, N., et al. (2005). Enterococcus faecium SF68 enhances the immune response to Giardia intestinalis in mice. J. Nutr. 135, 1171–1176. doi: 10.1093/jn/135.5.1171
Berrilli, F., Di Cave, D., Cavallero, S., and D'Amelio, S. (2012). Interactions between parasites and microbial communities in the human gut. Front. Cell. Infect. Microbiol. 2:141. doi: 10.3389/fcimb.2012.00141
Besirbellioglu, B. A., Ulcay, A., Can, M., Erdem, H., Tanyuksel, M., Avci, I. Y., et al. (2006). Saccharomyces boulardii and infection due to Giardia lamblia. Scand. J. Infect. Dis. 38, 479–481. doi: 10.1080/00365540600561769
Billiet, A., Meeus, I., Cnockaert, M., Vandamme, P., Van Oystaeyen, A., Wäckers, F., et al. (2017). Effect of oral administration of lactic acid bacteria on colony performance and gut microbiota in indoor-reared bumblebees (Bombus terrestris). Apidologie 48, 41–50. doi: 10.1007/s13592-016-0447-5
Bordier, C., Suchail, S., Pioz, M., Devaud, J. M., Collet, C., Charreton, M., et al. (2017). Stress response in honeybees is associated with changes in task-related physiology and energetic metabolism. J. Insect Physiol. 98, 47–54. doi: 10.1016/j.jinsphys.2016.11.013
Boutin, S., Audet, C., and Derome, N. (2013). Probiotic treatment by indigenous bacteria decreases mortality without disturbing the natural microbiota of Salvelinus fontinalis. Can. J. Microbiol. 59, 662–670. doi: 10.1139/cjm-2013-0443
Boutin, S., Alburaki, M., Mercier, P.-L., Giovenazzo, P., and Derome, N. (2015). Differential gene expression between hygienic and non-hygienic honeybee (Apis mellifera L.) hives. BMC Genomics 16:500. doi: 10.1186/s12864-015-1714-y
Bovera, F., Iannaccone, F., Mastellone, V., Nizza, S., Lestingi, A., De Martino, L., et al. (2012). Effect of spray application of Lactobacillus plantarum on in vivo performance, caecal fermentations and haematological traits of suckling rabbits. Ital. J. Anim. Sci. 11:e27. doi: 10.4081/ijas.2012.e27
Castex, M. (2009). Evaluation of Probiotic Bacteria Pediococcus Acidilactici MA18/5M on Penaeid Shrimp Litopenaeus stylirostris in New Caledonia. Doctoral dissertation, AgroParisTech.
Chaplinska, M., Gerritsma, S., Dini-Andreote, F., Salles, J. F., and Wertheim, B. (2016). Bacterial communities differ among Drosophila melanogaster populations and affect host resistance against parasitoids. PLoS ONE 11:e0167726. doi: 10.1371/journal.pone.0167726
Chomczynski, P. (1993). A reagent for the single-step simultaneous isolation of RNA, DNA and proteins from cell and tissue samples. Biotechniques 15, 532–534.
Chouaia, B., Rossi, P., Epis, S., Mosca, M., Ricci, I., Damiani, C., et al. (2012). Delayed larval development in Anopheles mosquitoes deprived of Asaia bacterial symbionts. BMC Microbiol. 12:S2. doi: 10.1186/1471-2180-12-S1-S2
Cleusix, V., Lacroix, C., Vollenweider, S., and Le Blay, G. (2007). Glycerol induces reuterin production and decreases Escherichia coli population in an in vitro model of colonic fermentation with immobilized human feces. FEMS Microbiol. Ecol. 63, 56–64. doi: 10.1111/j.1574-6941.2007.00412.x
Collier, C. T., Carroll, J. A., Ballou, M. A., Starkey, J. D., and Sparks, J. C. (2011). Oral administration of Saccharomyces cerevisiae boulardii reduces mortality associated with immune and cortisol responses to Escherichia coli endotoxin in pigs. J. Anim. Sci. 89, 52–58 doi: 10.2527/jas.2010-2944
Corby-Harris, V., Snyder, L., Meador, C. A. D., Naldo, R., Mott, B., and Anderson, K. E. (2016). Parasaccharibacter apium, gen. nov., sp. nov., improves honey bee (Hymenoptera: Apidae) resistance to Nosema. J. Econ. Entomol. 109, 537–543. doi: 10.1093/jee/tow012
Cox-Foster, D. L., Conlan, S., Holmes, E. C., Palacios, G., Evans, D. J., Moran, N. A., et al. (2007). A metagenomic survey of microbes in honey bee colony collapse disorder. Science 5848, 283–287. doi: 10.1126/science.1146498
Denev, S., Chevaux, E., and Demey, V. (2013). “Efficacité du probiotique Pediococcus pediococcus acidilactici sur les performances zootechniques de poules pondeuses,” in Conference Paper. JRA JRFG, 943–946. Available online at: https://www.researchgate.net/publication/235984461_EFFICACITE_DU_PROBIOTIQUE_PEDIOCOCCUS_ACIDILACTICI_SUR_LES_PERFORMANCES_ZOOTECHNIQUES_DE_POULES_PONDEUSESQ (Accessed November 16, 2017).
Di Pasquale, G., Salignon, M., Le Conte, Y., Belzunces, L. P., Decourtye, A., Kretzschmar, A., et al. (2013). Influence of pollen nutrition on honey bee health: do pollen quality and diversity matter? PLoS ONE 8:e72016. doi: 10.1371/journal.pone.0072016
Di Prisco, G., Cavaliere, V., Annoscia, D., Varricchio, P., Caprio, E., Nazzi, F., et al. (2013). Neonicotinoid clothianidin adversely affects insect immunity and promotes replication of a viral pathogen in honey bees. Proc. Natl. Acad. Sci. U.S.A. 110, 18466–18471. doi: 10.1073/pnas.1314923110
Dinleyici, E. C., Eren, M., Dogan, N., Reyhanioglu, S., Yargic, Z. A., and Vandenplas, Y. (2011). Clinical efficacy of Saccharomyces boulardii or metronidazole in symptomatic children with Blastocystis hominis infection. Parasitol. Res. 108, 541–545. doi: 10.1007/s00436-010-2095-4
Dinleyici, E. C., Eren, M., Yargic, Z. A., Dogan, N., and Vandenplas, Y. (2009). Clinical efficacy of Saccharomyces boulardii and metronidazole compared to metronidazole alone in children with acute bloody diarrhea caused by amebiasis: a prospective, randomized, open label study. Am. J. Trop. Med. Hyg. 80, 953–955. doi: 10.4269/ajtmh.2009.80.953
Doron, S., and Snydman, D. R. (2015). Risk and safety of probiotics. Clin. Infect. Dis. 60(Suppl. 2), S129–S134. doi: 10.1093/cid/civ085
Doublet, V., Labarussias, M., De Miranda, J. R., Moritz, R. F. A., and Paxton, R. J. (2014). Bees under stress: sublethal doses of a neonicotinoid pesticide and pathogens interact to elevate honey bee mortality across the life cycle. Environ. Microbiol. 17, 969–983 doi: 10.1111/1462-2920.12426
Durchschein, F., Petritsch, W., and Hammer, H. F. (2016). Diet therapy for inflammatory bowel diseases: the established and the new. World J. Gastroenterol. 22:2179. doi: 10.3748/wjg.v22.i7.2179
Dussaubat, C., Brunet, J. L., Higes, M., Colbourne, J. K., Lopez, J., Choi, J. H., et al. (2012). Gut pathology and responses to the microsporidium Nosema ceranae in the honey bee Apis mellifera. PLoS ONE 7:e37017. doi: 10.1371/journal.pone.0037017
Dussaubat, C., Maisonnasse, A., Alaux, C., Tchamitchan, S., Brunet, J.-L., Plettner, E., et al. (2010). Nosema spp. Infection alters pheromone production in honey bees (Apis mellifera). J. Chem. Ecol. 36, 522–525. doi: 10.1007/s10886-010-9786-2
Dussaubat, C., Maisonnasse, A., Crauser, D., Beslay, D., Costagliola, G., Soubeyrand, S., et al. (2013). Flight behavior and pheromone changes associated to Nosema ceranae infection of honey bee workers (Apis mellifera) in field conditions. J. Invertebr. Pathol. 113, 42–51. doi: 10.1016/j.jip.2013.01.002
Dussaubat, C., Maisonnasse, A., Crauser, D., Tchamitchian, S., Bonnet, M., Cousin, M., et al. (2016). Combined neonicotinoid pesticide and parasite stress alter honeybee queens' physiology and survival. Sci. Rep. 6:31430. doi: 10.1038/srep31430
EFSA (2012). Scientific opinion on the efficacy of Bactocell (Pediococcus acidilactici) when used as a feed additive for fish. EFSA J. 10:2886. doi: 10.2903/j.efsa.2012.2886
Engel, P., and Moran, N. A. (2013). The gut microbiota of insects - diversity in structure and function. FEMS Microbiol. Rev. 37, 699–735. doi: 10.1111/1574-6976.12025
Evans, J. D., and Lopez, D. L. (2004). Bacterial probiotics induce an immune response in the honey bee (Hymenoptera: Apidae). J. Econ. Entomol. 97, 752–756. doi: 10.1093/jee/97.3.752
Evans, J., Chen, Y. P., Di Prisco, G. D. P., Pettis, J., and Williams, V. (2009). Bee cups: single-use cages for honey bee experiments. J. Apic. Res. 48, 300–302. doi: 10.1080/00218839.2009.11101548
Fairbrother, A., Purdy, J., Anderson, T., and Fell, R. (2014). Risks of neonicotinoid insecticides to honeybees: risks of neonicotinoid insecticides to honeybees. Environ. Toxicol. Chem. 33, 719–731. doi: 10.1002/etc.2527
Fernandez, N., and Coineau, Y. (2007). Maladies, parasites et autres ennemis de l'abeille mellifère. Biarritz.
Flint, H. J., Scott, K. P., Louis, P., and Duncan, S. H. (2012). The Role of the gut microbiota in nutrition and health. Nat. Rev. Gastroenterol. Hepatol 9, 577–589. doi: 10.1038/nrgastro.2012.156
Fries, I., Chauzat, M. P., Chen, Y. P. P., Doublet, V., Genersch, E., Gisder, S., et al. (2013). Standard methods for nosema research. J. Apic. Res. 52, 1–28. doi: 10.3896/IBRA.1.52.1.14
Gisder, S., and Genersch, E. (2015) Identification of candidate agents active against N. ceranae Infection in honey bees: establishment of a medium throughput screening assay based on N. ceranae infected cultured cells. PLoS ONE 10:e0117200. doi: 10.1371/journal.pone.0117200
Gismondo, M. R., Drago, L., and Lombardi, A. (1999). Review of probiotics available to modify gastrointestinal flora. Int. J. Antimicrob. Agents 12, 287–292. doi: 10.1016/S0924-8579(99)00050-3
Goblirsch, M., Huang, Z. Y., and Spivak, M. (2013). Physiological and behavioral changes in honey bees (Apis mellifera) induced by Nosema ceranae infection. PLoS ONE 8:e58165 doi: 10.1371/journal.pone.0058165
Goulson, D., Nicholls, E., Botías, C., and Rotheray, E. L. (2015). Bee declines driven by combined stress from parasites, pesticides, and lack of flowers. Science 347:1255957. doi: 10.1126/science.1255957
Habtewold, T., Groom, Z., and Christophides, G. K. (2017). Immune resistance and tolerance strategies in malaria vector and non-vector mosquitoes. Parasit. Vectors 10:186. doi: 10.1186/s13071-017-2109-5
Hamdi, C., Balloi, A., Essanaa, J., Crotti, E., Gonella, E., Raddadi, N., et al. (2011). Gut microbiome dysbiosis and honeybee health. J. Appl. Entomol. 135, 524–533. doi: 10.1111/j.1439-0418.2010.01609.x
Higes, M., García-Palencia, P., Martín-Hernández, R., and Meana, A. (2007). Experimental infection of Apis mellifera honeybees with Nosema ceranae (Microsporidia). J. Invertebr. Pathol. 94, 211–217. doi: 10.1016/j.jip.2006.11.001
Higes, M., Martín-Hernández, R., Botías, C., Bailón, E. G., González-Porto, A. V., Barrios, L., et al. (2008). How natural infection by nosema ceranae causes honeybee colony collapse. Environ. Microbiol. 10, 2659–69. doi: 10.1111/j.1462-2920.2008.01687.x
Higes, M., Martín-Hernández, R., Garrido-Bailón, E., González-Porto, A. V., García-Palencia, P., Meana, A., et al. (2009). Honeybee colony collapse due to Nosema ceranae in professional apiaries. Environ. Microbiol. Rep. 1, 110–113. doi: 10.1111/j.1758-2229.2009.00014.x
Hoffmann, J. A., Kafatos, F. C., Janeway, C. A., and Ezekowitz, R. A. B. (1999). Phylogenetic perspectives in innate immunity. Science, 284, 1313–1318 doi: 10.1126/science.284.5418.1313
Hooper, L. V., Littman, D. R., and Macpherson, A. J. (2012). Interactions between the microbiota and the immune system. Science, 336, 1268–1273. doi: 10.1126/science.1223490
Hou, C., Zeng, X., Yang, F., Liu, H., and Qiao, S. (2015). Study and use of the probiotic Lactobacillus reuteri in pigs: a review. J. Anim. Sci. Biotechnol. 6, 1–8. doi: 10.1186/s40104-015-0014-3
Huang, Q., Chen, Y. P., Wang, R. W., Cheng, S., and Evans, J. D. (2016). Host-parasite interactions and purifying selection in a microsporidian parasite of honey bees. PLoS ONE 11:e0147549 doi: 10.1371/journal.pone.0147549
Huang, Q., Chen, Y., Wang, R. W., Schwarz, R. S., and Evans, J. D. (2015). Honey bee microRNAs respond to infection by the microsporidian parasite Nosema ceranae. Sci.c Rep. 5:17494. doi: 10.1038/srep17494
Huang, Q., Kryger, P., Le Conte, Y., and Moritz, R. F. (2012). Survival and immune response of drones of a Nosemosis tolerant honey bee strain towards N. ceranae infections. J. Invertebr. Pathol. 109, 297–302. doi: 10.1016/j.jip.2012.01.004
Huang, W. F., Solter, L. F., Yau, P. M., and Imai, B. S. (2013). Nosema ceranae escapes fumagillin control in Honey Bees. PLoS Pathog. 9:1003185. doi: 10.1371/journal.ppat.1003185
Humen, M. A., De Antoni, G. L., Benyacoub, J., Costas, M. E., Cardozo, M. I., Kozubsky, L., et al. (2005). Infection and Immunity. 731265–9. doi: 10.1128/IAI.73.2.1265-1269.2005
Hyrsl, P., Dobes, P., Vojtek, L., Hroncova, Z., Tyl, J., and Killer, J. (2017). Plant alkaloid sanguinarine and novel potential probiotic strains Lactobacillus apis, Lactobacillus melliventris and Gilliamella apicola promote resistance of honey bees to nematobacterial infection. Bull. Insectol. 70, 31–38.
Kakumanu, M. L., Reeves, A. M., Anderson, T. D., Rodrigues, R. R., and Williams, M. A. (2016). Honey bee gut microbiome is altered by in-hive pesticide exposures. Front. Microbiol. 7:1255. doi: 10.3389/fmicb.2016.01255
Kaplan, E. L., and Meier, P. (1958). Nonparametric estimation from incomplete observations. J Am. Stat. Assoc. 53, 457–481. doi: 10.1080/01621459.1958.10501452
Kazimierczak-Baryczko, M., and Szymas, B. (2006). Improvement of the composition of pollen substitute for honey bee (Apis mellifera L.), through implementation of probiotic preparations. J. Agric. Sci. 15, 50–51
Klein, S., Cabirol, A., Devaud, J.-M., Barron, A. B., and Lihoreau, M. (2017). Why bees are so vulnerable to environmental stressors. Trends Ecol. Evol. 32, 268–278. doi: 10.1016/j.tree.2016.12.009
Knutie, S. A., Shea, L. A., Kuppro vol selaitis, M., Wilkinson, C. L., Kohl, K. D., and Rohr, J. R. (2017). Early-life diet affects host microbiota and later-life defenses against parasites in frogs. Integr. Comp. Biol. 57, 732–742. doi: 10.1093/icb/icx028
Koch, H., and Schmid-Hempel, P. (2011). Socially transmitted gut microbiota protect bumble bees against an intestinal parasite. Proc. Natl. Acad. Sci. U.S.A. 108, 19288–19292. doi: 10.1073/pnas.1110474108
Kurze, C., Dosselli, R., Grassl, J., Le Conte, Y., Kryger, P., Baer, B., et al. (2016). Differential proteomics reveals novel insights into Nosema-honey bee interactions. Insect Biochem. Mol. Biol. 79, 42–49. doi: 10.1016/j.ibmb.2016.10.005
Lallemand (2016). Lallemand Animal Nutrition. Available online at: http://lallemandanimalnutrition.com/fr/europe/nos-produits/details/bactocell/ and http://lallemandanimalnutrition.com/fr/europe/nos-produits/details/levucell-sb/ (Accessed 2015).
Le Bon, M., Davies, H. E., Glynn, C., Thompson, C., Madden, M., Wiseman, J., et al. (2010). Influence of probiotics on gut health in the weaned pig. Livest. Sci. 133, 179–181. doi: 10.1016/j.livsci.2010.06.058
Lee, S., Lillehoj, H. S., Park, D. W., Hong, Y. H., and Lin, J. J. (2007). Effects of Pediococcus and Saccharomyces-based probiotic (MitoMax®) on coccidiosis in broiler chickens. Comp. Immunol. Microbiol. Infect. Dis. 30, 261–268 doi: 10.1016/j.cimid.2007.02.002
Lemaitre, B., and Hoffmann, J. (2007). The host defense of Drosophila melanogaster. Annu. Rev. Immunol. 25, 697–743. doi: 10.1146/annurev.immunol.25.022106.141615
Leoncini, I., Le Conte, Y., Costagliola, G., Plettner, E., Toth, A. L., Wang, M., et al. (2004). Regulation of behavioral maturation by a primer pheromone produced by adult worker honey bees. 101, 17559–17564. doi: 10.1073/pnas.0407652101
Li, J. H., Evans, J. D., Li, W. F., Zhao, Y. Z., DeGrandi-Hoffman, G., Huang, S. K., et al. (2017) New evidence showing that the destruction of gut bacteria by antibiotic treatment could increase the honey bee's vulnerability to Nosema infection. PLoS ONE 12:e0187505. doi: 10.1371/journal.pone.0187505
López, J. H., Krainer, S., Engert, A., Schuehly, W., Riessberger-Gallé, U., and Crailsheim, K. (2017) Sublethal pesticide doses negatively affect survival the cellular responses in American foulbrood-infected honeybee larvae. Sci. Rep. 7:40853. doi: 10.1038/srep40853
Maggi, M., Negri, P., Plischuk, S., Szawarski, N., De Piano, F., De Feudis, L., et al. (2013). Effects of the organic acids produced by a lactic acid bacterium in Apis mellifera colony development, Nosema ceranae control and fumagillin efficiency. Vet. Microbiol. 167, 474–483. doi: 10.1016/j.vetmic.2013.07.030
Martín-Hernández, R., Meana, A., Prieto, L., Salvador, A. M., Garrido-Bailón, E., and Higes, M. (2007). Outcome of colonization of Apis mellifera by Nosema ceranae. Appl. Environ. Microbiol. 73, 6331–6338. doi: 10.1128/AEM.00270-07
Mayack, C., and Naug, D. (2009). Energetic stress in the honeybee Apis mellifera from Nosema ceranae infection. J. Invertebr. Pathol. 100, 185–88. doi: 10.1016/j.jip.2008.12.001
Mitraka, E., Stathopoulos, S., Siden-Kiamos, I., Christophides, G. K., and Louis, C. (2013). Asaia accelerates larval development of Anopheles gambiae. Pathog. Glob. Health 107, 305–311. doi: 10.1179/2047773213Y.0000000106
Mountzouris, K. C., Dalaka, E., Palamidi, I., Paraskeuas, V., Demey, V., Theodoropoulosk, G., et al. (2015). Evaluation of yeast dietary supplementation in broilers challenged or not with Salmonella on growth performance, cecal microbiota composition and Salmonella in ceca, cloacae and carcass skin. Poult. Sci. 94, 2445–2455. doi: 10.3382/ps/pev243
Naug, D., and Gibbs, A. (2009). Behavioral changes mediated by hunger in honeybees infected with Nosema ceranae. Apidologie 40, 595–599. doi: 10.1051/apido/2009039
Nazzi, F., Brown, S. P., Annoscia, D., Del Piccolo, F., Di Prisco, G., Varricchio, P., et al. (2012). Synergistic parasite-pathogen interactions mediated by host immunity can drive the collapse of honeybee colonies. PLoS Pathog. 8:e1002735. doi: 10.1371/journal.ppat.1002735
Nikoskelainen, S., Ouwehand, A. C., Bylund, G., Salminen, S., and Lilius, E. M. (2003). Immune enhancement in rainbow trout (Oncorhynchus mykiss) by potential probiotic bacteria (Lactobacillus rhamnosus). Fish Shellfish Immunol. 15, 443–452. doi: 10.1016/S1050-4648(03)00023-8
Olofsson, T. C., and Vásquez, A. (2008). Detection and identification of a novel lactic acid bacterial flora within the honey stomach of the honeybee Apis mellifera. Curr. Microbiol. 57, 356–363. doi: 10.1007/s00284-008-9202-0
Pajuelo, A. G., Torres, C., and Bermejo, F. J. O. (2008). Colony losses: a double blind trial on the influence of supplementary protein nutrition and preventative treatment with fumagillin against Nosema ceranae. J. Apic. Res Bee World 47, 84–86. doi: 10.1080/00218839.2008.11101429
Palma, M. L., Zamith-Miranda, D., Martins, F. S., Bozza, F. A., Nimrichter, L., Montero-Lomeli, M., et al. (2015). Probiotic Saccharomyces cerevisiae strains as biotherapeutic tools: is there room for improvement? Appl. Microbiol. Biotechnol. 99, 6563–6570. doi: 10.1007/s00253-015-6776-x
Piccolo, G., Bovera, F., Lombardi, P., Mastellone, V., Nizza, S., Di Meo, C., et al. (2015). Effect of Lactobacillus plantarum on growth performance and hematological traits of European sea bass (Dicentrarchus labrax). Aquac. Int. 23, 1025–1032. doi: 10.1007/s10499-014-9861-8
Poquet, Y., Vidau, C., and Alaux, C. (2016). Modulation of pesticide response in honeybees. Apidologie 47, 412–426. doi: 10.1007/s13592-016-0429-7
Ptaszynska, A. A., Borsuk, G., Mułenko, W., and Demetraki-Paleolog, J. (2014). Differentiation of Nosema apis and Nosema ceranae spores under Scanning Electron Microscopy (SEM). J. Apic. Res. 53, 537–544. doi: 10.3896/IBRA.1.53.5.02
Ptaszynska, A. A., Borsuk, G., Zdybicka-Barabas, A., Cytrynska, M., and Małek, W. (2016). Are commercial probiotics and prebiotics effective in the treatment and prevention of honeybee nosemosis C? Parasitol. Res. 115, 397–406. doi: 10.1007/s00436-015-4761-z
Qamar, A., Aboudola, S., Warny, M., Michetti, P., Pothoulakis, C., LaMont, J. T., et al. (2001). Saccharomyces boulardii stimulates intestinal immunoglobulin a immune response to clostridium difficile toxin a in mice. Infect. Immun. 69, 2762–2765. doi: 10.1128/IAI.69.4.2762-2765.2001
Qiagen Inc. (2016). DNeasy® Blood & Tissue Handbook. Available on: http://diagnostics1.com/MANUAL/General_Qiagen.pdf
R Development Core Team (2008). R: A Language and Environment for Statistical Computing. Vienna: R Foundation for Statistical Computing. Available online at: http://www.R-project.org
Reiff, C., and Kelly, D. (2010). Inflammatory bowel disease, gut bacteria and probiotic therapy. Int. J. Med. Microbiol. 300, 25–33. doi: 10.1016/j.ijmm.2009.08.004
Ryu, J. H., Kim, S. H., Lee, H. Y., Bai, J. Y., Nam, Y. D., Bae, J. W., et al. (2008). Innate immune homeostasis by the homeobox gene caudal and commensal-gut mutualism in Drosophila. Science 319, 777–782. doi: 10.1126/science.1149357
Sabaté, D. C., Carrillo, L., and Audisio, M. C. (2009). Inhibition of Paenibacillus larvae and Ascosphaera apis by Bacillus subtilis isolated from honeybee gut and honey samples. Res. Microbiol. 160, 193–199. doi: 10.1016/j.resmic.2009.03.002
Sabaté, D. C., Cruz, M. S., Benítez-Ahrendts, M. R., and Audisio, M. C. (2012). Beneficial effects of Bacillus subtilis subsp. subtilis Mori2, a honey-associated strain, on honeybee colony performance. Probiotics Antimicrob. Proteins 4, 39–46. doi: 10.1007/s12602-011-9089-0
Sánchez-Bayo, F., Goulson, D., Pennacchio, F., Nazzi, F., Goka, K., and Desneux, N. (2016). Are bee diseases linked to pesticides? a brief review. Environ. Int. 89–90, 7–11. doi: 10.1016/j.envint.2016.01.009
Schoster, A., Kokotovic, B., Permin, A., Pedersen, P. D., Bello, F. D., and Guardabassi, L. (2013). In vitro inhibition of Clostridium difficile and Clostridium perfringens by commercial probiotic strains. Anaerobe 20, 36–41. doi: 10.1016/j.anaerobe.2013.02.006
Schwarz, R. S., Huang, Q., and Evans, J. D. (2015). Hologenome theory and the honey bee pathosphere. Curr. Opin. Insect Sci. 10, 1–7. doi: 10.1016/j.cois.2015.04.006
Servin, A. L. (2004). Antagonistic activities of lactobacilli and bifidobaceria against microbial pathogens. FEMS Microbiol. Rev. 28, 405–440. doi: 10.1016/j.femsre.2004.01.003
Shukla, G., Devi, P., and Sehgal, R. (2008). Effect of Lactobacillus casei as a probiotic on modulation of giardiasis. Dig. Dis. Sci. 53, 2671–2679. doi: 10.1007/s10620-007-0197-3
Smith, K. M., Loh, E. H., Rostal, M. K., Zambrana-Torrelio, C. M., Mendiola, L., and Daszak, P. (2014). Pathogens, pests, and economics: drivers of honey bee colony declines and losses. Ecohealth 10, 434–445. doi: 10.1007/s10393-013-0870-2
Standen, B. T., Rawling, M. D., Davies, S. J., Castex, M., Foey, A., Gioacchini, G., et al. (2013). Probiotic Pediococcus acidilactici modulates both localised intestinal-and peripheral-immunity in tilapia (Oreochromis niloticus). Fish Shellfish Immunol. 35, 1097–1104. doi: 10.1016/j.fsi.2013.07.018
Tomičić, Z., Zupan, J., Matos, T., and Raspor, P. (2016). Probiotic yeast Saccharomyces boulardii (nom. nud.) modulates adhesive properties of Candida glabrata. Sabouraudia 54, 835–845. doi: 10.1093/mmy/myw026
Traver, B. E., and Fell, R. D. (2011). Prevalence and infection intensity of Nosema in honey bee (Apis mellifera L.) colonies in Virginia. J. Invertebr. Pathol. 107, 43–49. doi: 10.1016/j.jip.2011.02.003
Travers, M.-A., Florent, I., Kohl, L., and Grellier, P. (2011). Probiotics for the control of parasites: an overview. J. Parasitol. Res. 2011:11. doi: 10.1155/2011/610769
Van Den Heever, J. P., Thompson, T. S., Otto, S. J. G., Curtis, J. M., Ibrahim, A., and Pernal, S. F. (2015). Evaluation of Fumagilin-B® and other potential alternative chemotherapies against Nosema ceranae-infected honeybees (Apis mellifera) in cage trial assays. Apidologie 47, 617–630. doi: 10.1007/s13592-015-0409-3
Vanegas, M. (2017). The silent beehive: how the decline of honey bee populations shifted the environmental protection agency's pesticide policy towards pollinators. Ecol. Law Q. 44:311. doi: 10.15779/Z38FX73Z0H
Vitetta, L., Saltzman, E. T., Nikov, T., Ibrahim, I., and Hall, S. (2016). Modulating the gut micro-environment in the treatment of intestinal parasites. J. Clin. Med. 5:102. doi: 10.3390/jcm5110102
Wang, M., Zhao, W. Z., Xu, H., Wang, Z. W., and He, S. Y. (2015). Bacillus in the guts of honey bees (Apis mellifera; Hymenoptera: Apidae) mediates changes in amylase values. Eur. J. Entomol. 112, 619–624. doi: 10.14411/eje.2015.095
Williams, G. R., Alaux, C., Costa, C., Csáki, T., Doublet, V., Eisenhardt, D., et al. (2013). Standard methods for maintaining adult Apis mellifera in cages under in vitro laboratory conditions. J. Apic. Res. 52, 1–36. doi: 10.3896/IBRA.1.52.1.04
Williams, G. R., Shutler, D., Little, C. M., Burgher-MacLellan, K. L., and Rogers, R. E. L. (2011). The microsporidian Nosema ceranae, the antibiotic Fumagilin-B®, and western honey bee Apis mellifera colony strength. Apidologie 42, 15–22. doi: 10.1051/apido/2010030
Wohlgemuth, S., Loh, G., and Blaut, M. (2010). Recent developments and perspectives in the investigation of probiotic effects. Int. J. Med. Microbiol. 300, 3–10. doi: 10.1016/j.ijmm.2009.08.003
Keywords: honey bee, intracellular parasite, Nosema ceranae, endogenous probiotics, host-parasite interaction
Citation: El Khoury S, Rousseau A, Lecoeur A, Cheaib B, Bouslama S, Mercier P-L, Demey V, Castex M, Giovenazzo P and Derome N (2018) Deleterious Interaction Between Honeybees (Apis mellifera) and its Microsporidian Intracellular Parasite Nosema ceranae Was Mitigated by Administrating Either Endogenous or Allochthonous Gut Microbiota Strains. Front. Ecol. Evol. 6:58. doi: 10.3389/fevo.2018.00058
Received: 29 September 2017; Accepted: 20 April 2018;
Published: 23 May 2018.
Edited by:
David Georges Biron, Centre National de la Recherche Scientifique (CNRS), FranceReviewed by:
Cedric Alaux, INRA Centre Provence-Alpes-Côte d'Azur, FranceAndone Estonba, University of the Basque Country (UPV/EHU), Spain
Copyright © 2018 El Khoury, Rousseau, Lecoeur, Cheaib, Bouslama, Mercier, Demey, Castex, Giovenazzo and Derome. This is an open-access article distributed under the terms of the Creative Commons Attribution License (CC BY). The use, distribution or reproduction in other forums is permitted, provided the original author(s) and the copyright owner are credited and that the original publication in this journal is cited, in accordance with accepted academic practice. No use, distribution or reproduction is permitted which does not comply with these terms.
*Correspondence: Nicolas Derome, bmljb2xhcy5kZXJvbWVAYmlvLnVsYXZhbC5jYQ==
†These authors have contributed equally to this work.