- 1Institute for the Study of Anthropic Impact and Sustainability in the Marine Environment, National Research Council, Genova, Italy
- 2Costa Edutainment S.p.A., Acquario di Genova, Genova, Italy
Microplastics (MPs) can be ingested by marine organisms directly or indirectly through trophic transfer from contaminated prey. In the marine ecosystem, zooplankton are an important link between phytoplankton and higher trophic levels in the marine food web. Among them, copepods and gelatinous species have been recently reported to ingest MPs, but no potential MP transfer has been verified yet. In this study, a simplified two-level trophic chain – formed by nauplii of the Tigriopus fulvus copepod as prey, and the ephyrae stage of Aurelia sp. as predator – was selected to investigate MP trophic transfer. The experimental setup consisted in feeding ephyrae with nauplii previously exposed to fluorescent 1–5 μm polyethylene MPs and evaluating two ecotoxicological end-points: jellyfish immobility and pulsation frequency. After 24 h, the jellyfish ingested nauplii contaminated with MPs; however, neither immobility nor behavior was affected by MP transfer. These findings show that MPs can be transported at different trophic levels, but more research is needed to identify their potential effects on the marine food web.
Introduction
Microplastics (MPs, <5 mm, Arthur et al., 2009) are bioavailable to marine organisms, through direct or indirect ingestion by trophic transfer from contaminated prey (Lusher, 2015; Nelms et al., 2018). Marine organisms can ingest unknown quantities of MPs using different feeding strategies since MPs occupy the same size range as plankton (Cole et al., 2013; Farrell and Nelson, 2013).
MP uptake is primarily due to their particle size in relation to natural prey and particle density, which determines their position in the water column and the possibility of encountering the organisms (Desforges et al., 2015). MP ingestion and accumulation have been successfully demonstrated in several marine species belonging to different trophic levels, from zooplankton to fish and marine mammals (Boerger et al., 2010; Avery-Gomm et al., 2012; Goldstein and Goodwin, 2013; Frias et al., 2014; Desforges et al., 2015; Bellas et al., 2016). Many zooplankton species feed on phytoplankton and pass this energy upward through the food web. Moreover, they play a key role in ecosystem functioning, including nutrient, and carbon cycling and the production of sinking fecal pellets (Botterell et al., 2019).
Marine zooplankton are most susceptible to MPs, since their ingestion has been recorded in more than 30 species from 28 taxonomic orders (Zheng et al., 2020). Among them, crustaceans have been widely investigated and MP ingestion has been reported in several copepod species (i.e., Tigriopus fulvus, Acartia clausi, Centropages typicus, Calanus helgolandicus, Temora longicornis, Neocalanus cristatus; Cole et al., 2013, 2015; Beiras et al., 2018; Botterell et al., 2019; Coppock et al., 2019), accounting for a high proportion of the total zooplankton carbon biomass. In these organisms, MP ingestion affects their fecundity and reduces their feeding capacity, being able to discriminate natural algal prey from MPs (Cole et al., 2015; Botterell et al., 2019; Coppock et al., 2019). Recently, also gelatinous zooplankton (i.e., jellyfish, tunicates, salps), feeding on crustaceans and fish larvae and a key food source for pelagic top predators (Purcell et al., 2007), have been reported to internalize MPs with further impairment of swimming, lower feeding rate, growth, and oxygen consumption (Macali et al., 2018; Paffenhöfer and Köster, 2018; Wieczorek et al., 2019; Brandon et al., 2020; Costa et al., 2020).
Among gelatinous zooplankton, jellyfish (i.e., Aurelia sp., Pelagia noctiluca) are very vulnerable to plastic pollution: marine litter may be transferred through jellyfish to pelagic predators that may confuse floating plastics for prey (Macali et al., 2018). While many studies are available for both crustaceans and gelatinous zooplankton (Beiras et al., 2018; Macali et al., 2018) on MP ingestion and its effects on individual zooplankton species either under laboratory conditions or in the field, very little is known about MP transfer from lower to higher trophic levels. The latter may cause potential cascading effects in marine food webs (Frias et al., 2014; Setälä et al., 2014; Botterell et al., 2019; Wieczorek et al., 2019), since zooplankton play a key role in the link between primary producers and higher trophic organization (Sun et al., 2017).
To date, MP transfer among zooplankton has only been reported from polychaete larvae and copepods to mysid shrimps (Setälä et al., 2014). In general, MP potential to enter the marine food chain has been reported from adult mussels to crabs (Farrell and Nelson, 2013), from plankton to fur seals (Eriksson and Burton, 2003) and benthic filter feeders (Van Colen et al., 2020), from crustaceans to fish (Batel et al., 2016), from beach hoppers to ray-finned fish (Tosetto et al., 2017), and in marine top predators (i.e., from Atlantic mackerel to gray seals, Nelms et al., 2018).
Our current knowledge is insufficient to understand MP potential for bioaccumulation and biomagnification within the marine food chain, due to a lack of robust data describing MP transfer from prey to predator. Many questions remain when considering ecosystem-level dynamics, such as MP trophic transfer in the marine environment (Au et al., 2017). Since marine zooplankton are an important link between primary producing phytoplankton and higher trophic levels in food webs (Turner, 2004), more research is needed to clarify MP transfer among them. To fill this gap, a simplified two-level trophic chain was set up, consisting of a zooplankton species such as the nauplii of T. fulvus copepod as prey and the ephyrae stage of Aurelia sp. jellyfish as predator, to investigate MP trophic transfer. These species were selected since they are quite abundant and easy to culture in the laboratory and are promising models to evaluate MP pollution. To achieve this goal, nauplii were previously exposed to polyethylene MPs. Once contaminated, they were fed to ephyrae jellyfish. Finally, the ecotoxicological effect of MP trophic transfer in ephyrae jellyfish was investigated by evaluating two end-points (Immobility and Pulsation Frequency), recently proposed for this model organism (Faimali et al., 2014; Costa et al., 2015, 2020; Giussani et al., 2015).
Methods
Predators and Prey
Tigriopus fulvus nauplii and Aurelia sp. ephyrae were used as preys and predators, respectively. T. fulvus nauplii, a harpacticoid copepod widely distributed in the Mediterranean, originated from synchronized cultures (24 h) obtained from ovigerous adult females at CNR-IAS laboratory, following UNICHIM protocol.
Ephyrae of Aurelia sp. were released by strobilation from polyp cultures in the laboratories of the “Acquario di Genova, Costa Edutainment S.p.A.,” and transported to CNR-IAS, according to Costa et al. (2020).
Microplastics
Fluorescent green 1–5 μm polyethylene MPs (1.3 g/cm3 density, 414 nm excitation/515 nm emission) μm polyethylene MPs (CPMS-0.96, 0.99 g/cm3 density) were purchased from Sigma-Aldrich (Germany). Stock solutions of MPs (10 mg/L) were prepared in 0.22 μm of Filtered Natural Sea Water (FNSW).
Artificial Food Chain
A simplified two-level trophic chain was set up by feeding ephyrae jellyfish with nauplii of T. fulvus previously exposed for 6 h to different concentrations (0–1–10 mg/L) of fluorescent 1–4 μm polyethylene MP, according to Beiras et al. (2018). Briefly, 2 mL of nauplii (25 organisms per mL) was transferred by using a Pasteur pipette into small glass vials containing 2 mL of control FNSW, or serial dilutions (1–10 mg/L) of MPs. Vials were then placed in 50 mL centrifuge tubes (5 vials/tube), fixed on a rotatory wheel (2 rpm speed) and kept at 20°C. After 6 h, the nauplii were transferred from glass vials into a multiwell plate, to verify MP ingestion under an epi-fluorescence microscope (Olympus). Each treatment, including controls, was prepared in triplicates.
Then, nauplii were offered to ephyrae in a semi-dynamic condition according to Costa et al. (2020) for 24 h. More in detail, 10 ephyrae collected immediately after strobilation were incubated in a glass beaker filled with 100 mL of FNSW, with constant aeration for oxygen supply, in order to ensure current balance to the organisms (Widmer, 2008). For each tested MP concentration, each batch of 10 ephyrae was fed with previously contaminated 50 T. fulvus nauplii. The control group of ephyrae was fed with untreated (MP-free) nauplii. The prey (nauplii)/predator (ephyrae) ratio was previously defined in order to avoid an excess of food in the beaker considering that Aurelia sp. ephyrae can capture only live preys (Suchman and Sullivan, 2000; Bamstedt et al., 2001). Indeed, the non-consumed nauplii setting on the beaker bottom could have caused overfishing conditions. For each MP dilution, 3 replicates were prepared. Constant temperature was maintained by keeping the glass beaker in a thermostatic room at 20°C ± 0.5 for 24 h.
MP Transfer
In order to analyze only the MP transfer from copepods to ephyrae, after 24 h, the ephyrae were recovered and washed three times with fresh FNSW to remove any microspheres which, if released in the water by nauplii, could bind to the gelatinous body. The organisms were anesthetized with the addition of a few menthol crystals, fixed in 4% paraformaldehyde solution in phosphate-buffered saline (PBS, pH 7.4), and mounted in glycerol–PBS (1:1) to be visualized by immunofluorescence under an epi-fluorescence microscope (Olympus) according to Williams and Van Syoc (2007) and Costa et al. (2020).
Ecotoxicological Responses
Before anaesthetization, each ephyra was transferred from the glass beaker into a single Petri dish (containing clean FNSW) in order to evaluate immobility (acute end-point) and pulsation frequency (subacute response). This was done using an automatic recording system coupled with a specially designed video graphics analyzer: Swimming Behavioral Recorder (SBR; Faimali et al., 2014; Costa et al., 2020). Completely motionless ephyrae were counted as immobile organisms, and the immobility percentage (I%) was calculated for each dilution and compared to controls. Pulsation frequency was calculated by recording the number of pulsations made by each ephyra in 1 min.
Statistical Analysis
Significant differences between treated samples and controls were evaluated using one-way analysis of variance (ANOVA) followed by Tukey test. When data failed to meet the assumption of normality, the non-parametric Kruskal–Wallis test and Mann–Whitney test were used. Data were considered significantly different when p < 0.05. SPSS statistical software (Statistical Package for the Social Sciences, Version 20) was used for data analysis.
Results
MP Transfer
Copepod nauplii ingested 1 and 10 mg/L MPs after 6 h of exposure. Particles were shown to have accumulated in the gut (data not shown). Jellyfish fed with contaminated copepods for 24 h showed to have ingested them, thus indicating that MP trophic transfer had occurred (Figure 1): both control (Figure 1A) and treated (Figures 1B,C) organisms ingested a variable number of nauplii (from 1 to 4). In detail, control ephyrae that ingested untreated copepods are indicated in Figure 1A. Details of the ephyra mouth, manubrium, and gastric filaments are seen in Figure 1B, where two copepods were found that had internalized MPs in an aggregate form. Figure 1C highlights one copepod, containing aggregates of fluorescent MPs, close to the ephyra’s gastric filament.
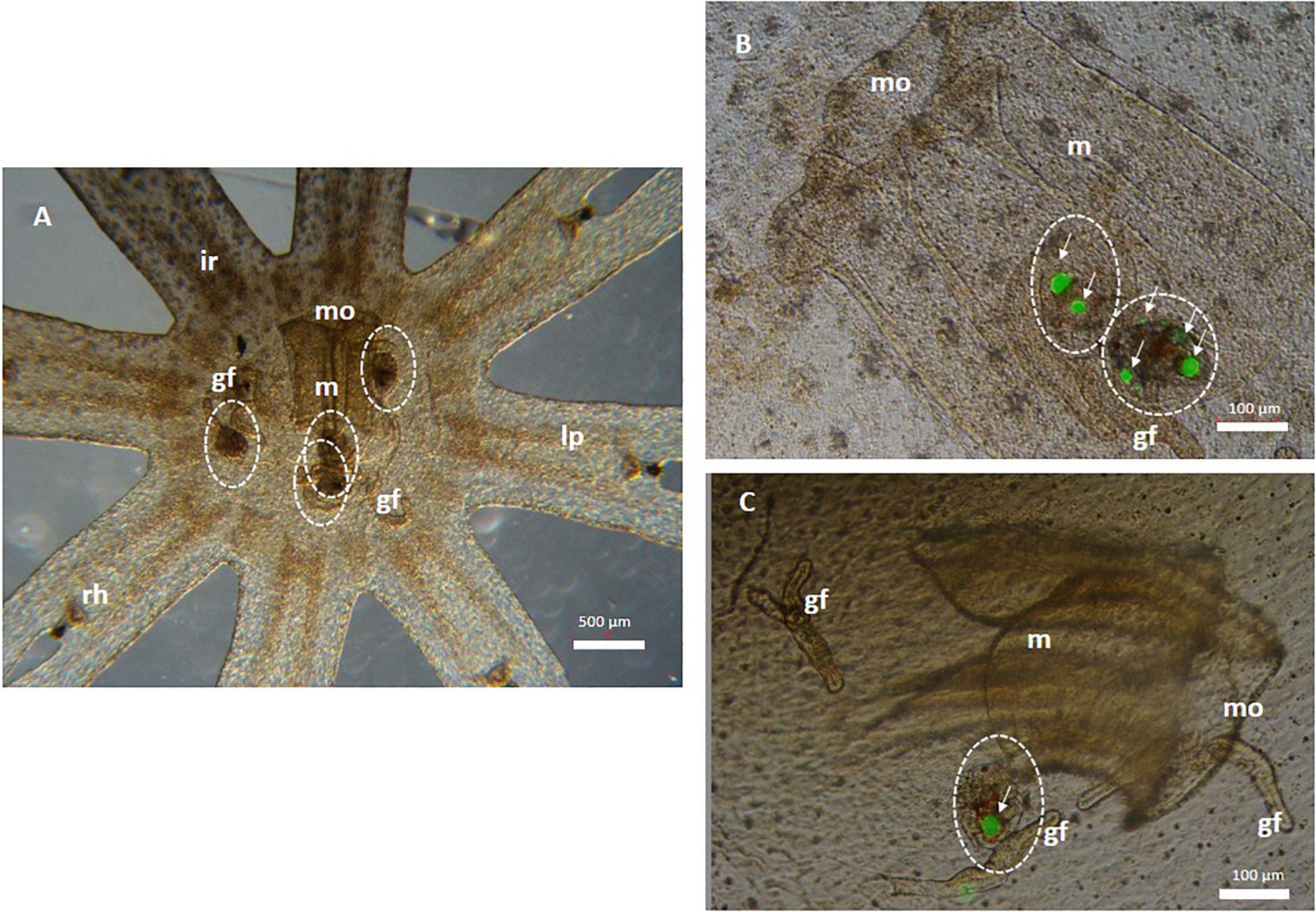
Figure 1. Microplastic (MP) trophic transfer from T. fulvus nauplii (prey) to Aurelia sp. Ephyrae (predator) after a 24 h exposure. (A) Control Aurelia sp. ephyrae that ingested uncontaminated copepods (white dotted circles). (B,C) Copepods (white dotted circle) containing fluorescent polyethylene MPs (white arrows) are clustered in the manubrium of the mouth and near gastric filaments. mo, mouth; m, manubrium; ir, inter-radial; lp, lappets; rh, rhopalia; gf, gastric filaments; white dotted circle, copepod; white arrows, MPs (or aggregate).
Ecotoxicological Responses
Ingestion of MP-contaminated preys did not cause any, either acute or subacute, ecotoxicological effects. Immobility percentage in ephyrae fed with T. fulvus nauplii contaminated with 1 mg/L and 10 mg/L polyethylene MPs was not significantly affected (0%). Further, the subacute end-point (pulsation frequency) was not significantly affected (p > 0.05) in ephyrae fed with T. fulvus nauplii contaminated with both polyethylene MPs dilutions. Actually, after the ingestion of 10 mg/L MP-contaminated copepods (Figure 2), only a very slight decrease in the number of pulsations (vs. controls) was observed.
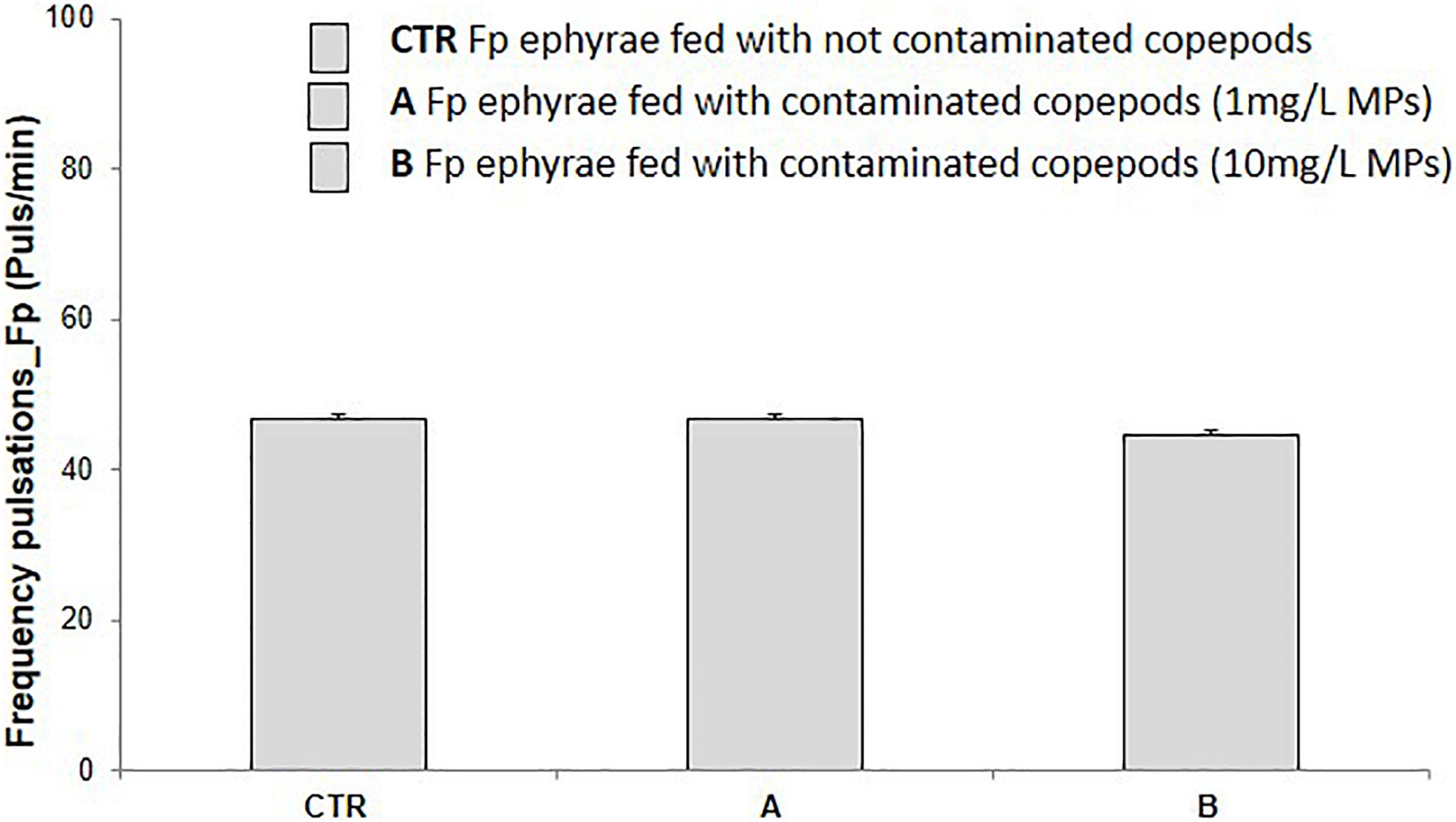
Figure 2. Pulsation frequency (pulse/min) recorded for Aurelia sp. ephyrae after feeding uncontaminated T. fulvus nauplii (CTR) and nauplii contaminated with 1 mg/L (A) and 10 mg/L (B) of polyethylene MPs. (M ± SE, n = 3). No significant difference was observed between treatments and controls in any of the tests (A,B).
Discussion
In this study, MP trophic transfer has been successfully demonstrated between copepod nauplii and ephyra jellyfish. Polyethylene MPs were ingested indirectly as a result of trophic transfer, whereby contaminated prey was consumed by predators. The potential of MPs to be transferred between trophic levels, from copepods to macrozooplankton (mysid shrimps), following ingestion, has been already observed by Setälä et al. (2014). An indirect MP transfer has also been demonstrated from mussels to crabs (Farrell and Nelson, 2013), from Atlantic mackerels to gray seals (Nelms et al., 2018), from plankton to fur seals (Eriksson and Burton, 2003), and from crustacean larvae to fish (Batel et al., 2016) by either laboratory experiments or field observations. Taken together, these studies suggest that trophic transfer is an indirect major pathway of MP ingestion for any species whose feeding ecology involves the consumption of whole prey (Nelms et al., 2018). Zooplankton may incorporate several elements or toxic compounds, including persistent organic pollutants (POPs), either directly, by absorption from water, or indirectly, by ingestion of contaminated algae and other zooplankton (Nizzetto et al., 2012; Bettinetti and Manca, 2014). POPs and other hydrophobic organics can adsorb to plastics and MPs in the environment, being bioavailable to aquatic organisms (Au et al., 2017). Among zooplankton, copepods are a potential carrier of organic hydrocarbon compounds (i.e., dioxins), by dietary uptake and bioaccumulation of contaminated phytoplankton (Wallberg et al., 2001; Zhang et al., 2011).
Our results demonstrate that copepods can also transfer MPs to the higher trophic chain levels, since MP-contaminated nauplii were ingested in ephyrae gelatinous tissues. Jellyfish have a strong top-down control and influence on plankton communities (West et al., 2009). In addition, as a group of gelatinous plankton, they are considered part of marine ecosystems’ microbial – crustacean – fish – carnivorous macrozooplankton pathway (Richardson et al., 2009; Epstein et al., 2016; Boero et al., 2019). Jellyfish feed on fish eggs and larvae as well as on crustaceans. Therefore, they may transfer contaminants from other zooplankton prey to predators. Jellyfish can accumulate several kinds of contaminants in their gelatinous tissues, such as heavy metals (i.e., cadmium, chromium, copper; Richardson et al., 2009; Templeman and Kingsford, 2010, 2015; Klein et al., 2015), polycyclic aromatic hydrocarbons (PAHs; Almeda et al., 2013), and MPs (Macali et al., 2018; Costa et al., 2020). However, transfer of these contaminants to their natural predators has been suggested only for some contaminants. In this regard, Caurant et al. (1999) linked jellyfish cadmium accumulation and transfer to leatherback turtles, while Almeda et al. (2013) proposed that crude oil PAHs accumulated in jellyfish may be potentially transferred up the food web, thus affecting apex predators. Since in our study MP transfer occurred in a simplified two-level trophic chain, formed by copepods and jellyfish, further investigations are needed to verify any subsequent bottom-up implications for higher trophic levels, considering that jellyfish may play a role as a carrier of plastics along the food web. Although MP transfer occurred from zooplankton to jellyfish larvae (as reported in “Predators and Prey”), it did not affect ephyrae immobility and behavior. A longer feeding exposure timeframe (>24 h) may be required in order to observe any ecotoxicological effect from MP trophic transfer from prey to predator. It has been reported that direct MP exposure in jellyfish ephyrae temporarily affects both their survival and behavior due to mechanical disturbance caused by MPs triggering a loss in radial symmetry (Costa et al., 2020). Conversely, no measurable response could be detected after indirect MP exposure through ingestion of MP-contaminated copepods. The short feeding time (24 h) used in this study might have not been enough to determine any measurable effects in jellyfish ephyrae. However, studies on freshwater food web (crustaceans and zebrafish) have demonstrated that even indirect MP transfer through chronic exposure (up to 2 weeks) poses no serious harm along the trophic chain, since no signs of stress or disease were observed (Batel et al., 2016).
Jellyfish are involved in MP transfer through zooplankton; however, MP transfer toward high trophic levels still needs further investigations. Kakani et al. (2017) and Brandon et al. (2020) demonstrated that filter feeders and gelatinous zooplankton – namely, larvaceans and salps – can ingest large MP amounts from near surface water and move MPs to the deep sea, by sinking fecal pellet aggregates. Therefore, they are biological carriers for MP transport in the marine ecosystem through the water column, from sea surface to the seabed. Likewise, gelatinous zooplankton such as jellyfish may contribute to MP transfer from zooplankton to pelagic top predators. This study for the first time reports an indirect MP trophic transfer in a simplified food chain formed by copepod nauplii and jellyfish ephyrae. While no ecotoxicological responses were observed in jellyfish fed with MP-contaminated preys, this study shows that MPs are indirectly ingested in predators: further studies are needed to detect any potential MP transfer effects on the marine food web.
Data Availability Statement
The raw data supporting the conclusions of this article will be made available by the authors, without undue reservation, to any qualified researcher.
Author Contributions
EC and CG conceived the study and wrote the manuscript. EC and SL collected the jellyfish. VP, EC, and CG performed the experiments and analyzed the results. VP, SL, MF, and FG edited and reviewed the text. All authors approved the final version of the manuscript.
Funding
This work was supported by the CLAIM (Cleaning Litter by developing and Applying Innovative Methods in European Seas) project which receives funding from the European Union’s Horizon 2020 Research and Innovation Program under Grant Agreement No. 774586.
Conflict of Interest
SL is employed by company Costa Edutainment S.p.A., Acquario di Genova.
The remaining authors declare that the research was conducted in the absence of any commercial or financial relationships that could be construed as a potential conflict of interest.
Acknowledgments
This research was conducted in the frame of the JPI-Oceans EPHEMARE Project and EU-H2020 CLAIM project. We are particularly thankful to the tropical laboratory of the Acquario of Genoa for polyps and ephyrae jellyfish collection, and for supporting during the experiment.
References
Almeda, R. Z., Wambaugh, C., Chai, Z., Wang, Z., and Liu, Z. (2013). Effects of crude oil exposure on bioaccumulation of polycyclic aromatic hydrocarbons and survival of adult and larval stages of 8 gelatinous zooplankton. PLoS One 8:74476. doi: 10.1371/journal.pone.0074476
Arthur, C., Bamford, H., and Baker, J. (2009). International Research Workshop on The Occurrence, Effects and Fate of Microplastic Marine Debris. NOAA Technical 443 Memorandum NOS-OR&R-30. Silver Spring, MD: NOAA.
Au, S. Y., Lee, C. M., Weinstein, J. E., van den Hurk, P., and Klaine, S. J. (2017). Trophic transfer of microplastics in aquatic ecosystems: identifying critical research needs. Integr. Environ. Assess. Manag. 13, 505–509. doi: 10.1002/ieam.1907
Avery-Gomm, S., O’Hara, P. D., Kleine, L., Bowes, V., Wilson, L. K., and Barry, K. L. (2012). Northern fulmars as biological monitors of trends of plastic pollution in the eastern North Pacific. Mar. Pollut. Bull. 64, 1776–1781. doi: 10.1016/j.marpolbul.2012.04.017
Bamstedt, U., Ishii, H., and Martinussen, M. B. (2001). Significance of food type for growt of ephyrae Aurelia aurita (Scyphozoa). Mar. Biol. 139, 641–650. doi: 10.1007/s002270100623
Batel, A., Linti, F., Scherer, M., Erdinger, L., and Braunbeck, T. (2016). The transfer of benzo[a] pyrene from microplastics to Artemia nauplii and further to zebrafish via a trophic food web experiment – CYP1a induction and visual tracking of persistent organic pollutants. Environ. Toxicol. Chem. 35, 1656–1666. doi: 10.1002/etc.3361
Beiras, R., Bellas, J., Cachot, J., Cormier, B., Cousin, X., Engwall, M., et al. (2018). Ingestion and contact with polyethylene microparticles does not cause toxicity on marine zooplankton. J. Haz. Mater. 360, 452–460. doi: 10.1016/j.jhazmat.2018.07.101
Bellas, J., Martínez-Armental, J., Martínez-Cámara, A., Besada, V., and Martínez-Gómez, C. (2016). Ingestion of microplastics by demersal fish from the Spanish Atlantic and Mediterranean coasts. Mar. Pollut. Bull. 109, 55–60. doi: 10.1016/j.marpolbul.2016.06.026
Bettinetti, R., and Manca, M. (2014). “Understanding the role of zooplankton in transfer of pollutants through trophic food webs,” in Zooplankton: Species Diversity, Distribution and Seasonal Dynamics, ed. G. Kehayias (Hauppauge, NY: Nova Publisher).
Boerger, C. M., Gwendolyn, L. L., Moore, S. L., and Moore, C. J. (2010). Plastic ingestion by planktivorous fishes in the North Pacific Central Gyre. Mar. Pollut. Bull. 60, 2275–2278. doi: 10.1016/j.marpolbul.2010.08.007
Boero, F., Leo, F., Fraschetti, S., and Ingrosso, G. (2019). The cells of ecosystem functioning: towards a holistic vision of marine space. Adv. Mar. Biol. 82, 129–153. doi: 10.1016/bs.amb.2019.03.001
Botterell, L. R., Beaumont, N., Dorrington, T., Steinke, M., Thompson, R. C., and Lindeque, P. K. L. (2019). Bioavailability and effects of microplastics on marine zooplankton: a review. Environ. Pollut. 245, 98–110. doi: 10.1016/j.envpol.2018.10.065
Brandon, J. A., Freibott, A., and Sala, L. M. (2020). Patterns of suspended and salp-ingested microplastic debris in the North Pacific investigated with epifluorescence microscopy. Limnol. Oceanogr. Lett. 5, 46–53. doi: 10.1002/lol2.10127
Caurant, F., Bustamante, P., Bordes, M., and Miramand, P. (1999). Bioaccumulation of cadmium, copper and zinc in some tissues of three species of marine turtles stranded along the French Atlantic coasts. Mar. Pollut. Bull. 38, 1085–1091. doi: 10.1016/s0025-326x(99)00109-5
Cole, M., Lindeque, P., Fileman, E., Halsband, C., and Galloway, T. S. (2015). The impact of polystyrene microplastics on feeding, function and fecundity in the marine copepod Calanus helgolandicus. Environ. Sci. Technol. 49, 1130–1137. doi: 10.1021/es504525u
Cole, M., Lindeque, P., Fileman, E., Halsband, C., Goodhead, R., Moger, J., et al. (2013). Microplastic ingestion by zooplankton. Environ. Sci. Technol. 47, 6646–6655. doi: 10.1021/es400663f
Coppock, R. L., Galloway, T. S., Cole, M., Fileman, E. S., Queirós, A. M., and Lindeque, P. K. (2019). Microplastics alter feeding selectivity and faecal density in the copepod, Calanus helgolandicus. Sci. Total Environ. 687, 780–789.
Costa, E., Gambardella, C., Piazza, V., Greco, G., Lavorano, S., Beltrandi, M., et al. (2015). Effect of neurotoxic compounds on ephyrae of Aurelia aurita jellyfish. Hydrobiologia 759, 75–84. doi: 10.1007/s10750-015-2284-3
Costa, E., Gambardella, C., Piazza, V., Vassalli, M., Sbrana, F., Lavorano, S., et al. (2020). Microplastics ingestion in the ephyra stage of Aurelia sp. triggers acute and behavioral responses. Ecotox. Environ. Saf. 189:109983. doi: 10.1016/j.ecoenv.2019.109983
Desforges, J.-P. W., Galbraith, M., and Ross, P. S. (2015). Ingestion of microplastics by zooplankton in the Northeast Pacific Ocean. Arch. Environ. Contam. Toxicol. 69, 320–330. doi: 10.1007/s00244-015-0172-5
Epstein, H. E., Templeman, M. A., and Kingsford, M. J. (2016). Fine-scale detection of pollutants by a benthic marine jellyfish. Mar. Pollut. Bull. 107, 340–346. doi: 10.1016/j.marpolbul.2016.03.027
Eriksson, C., and Burton, H. (2003). Origins and biological accumulation of small plastic particles in fur seals from Macquarie Island. AMBIO J. Hum. Environ. Stud. 32, 380–384. doi: 10.1579/0044-7447-32.6.380
Faimali, M., Garaventa, F., Piazza, V., Costa, E., Greco, G., Mazzola, V., et al. (2014). Ephyra Jellyfish as a new model for ecotoxicological bioassays. Mar. Environ. Res. 93, 93–101. doi: 10.1016/j.marenvres.2013.07.004
Farrell, P., and Nelson, K. (2013). Trophic level transfer of microplastic: Mytilus edulis (L.) to Carcinus maenas (L.). Environ. Pollut. 177, 1–3. doi: 10.1016/j.envpol.2013.01.046
Frias, J., Otero, V., and Sobral, P. (2014). Evidence of microplastics in samples of zooplankton from Portuguese coastal waters. Mar. Environ. Res. 95, 89–95. doi: 10.1016/j.marenvres.2014.01.001
Giussani, V., Sbrana, F., Asnaghi, V., Vassalli, M., Faimali, M., Casabianca, S., et al. (2015). Active role of the mucilage in the toxicity mechanism of the harmful benthic dinoflagellate Ostreopsis cf. ovata. Harmful Algae 44, 46–53. doi: 10.1016/j.hal.2015.02.006
Goldstein, M. C., and Goodwin, D. S. (2013). Gooseneck barnacles (Lepas spp.) ingest microplastic debris in the North Pacific Subtropical Gyre. PeerJ 1:e184. doi: 10.7717/peerj.184
Kakani, K., Choy, C. A., Sherlock, R. E., Sherman, A. D., and Robinson, H. B. (2017). From the surface to the seafloor: how giant larvaceans transport microplastics into the deep sea. Sci. Adv. 3:1700715.
Klein, G. S., Pitt, K. A., and Carroll, R. (2015). Reduced salinity increases susceptibility of zooxanthellate jellyfish to herbicide toxicity during a simulated rainfall event. Environ. Pollut. 209, 79–86. doi: 10.1016/j.envpol.2015.11.012
Lusher, A. (2015). “Microplastics in the marine environment: distribution, interactions and effects,” in Marine Anthropogenic Litter, eds M. Bergmann, L. Gutow, and M. Klages (Cham: Springer).
Macali, A., Semenov, A., Venuti, V., Crupi, V., D’Amico, F., Rossi, B., et al. (2018). Episodic records of jellyfish ingestion of plastic items reveal a novel pathway for trophic transference of marine litter. Sci. Rep. 8:6105.
Nelms, S. E., Galloway, T. S., Godley, B. J., Jarvis, D. S., and Lindeque, P. K. (2018). Investigating microplastic trophic transfer in marine top predators. Environ. Pollut. 238, 999–1007. doi: 10.1016/j.envpol.2018.02.016
Nizzetto, L., Gioia, R., Li, J., Borgå, K., Pomati, F., Bettinetti, R., et al. (2012). Biological pump control of the fate and distribution of hydrophobic organic pollutants in water and plankton. Environ. Sc. Tech. 46, 3204–3211. doi: 10.1021/es204176q
Paffenhöfer, G., and Köster, M. (2018). The Effects of Microplastics on Dolioletta gegenbauri (Tunicata, Thaliacea). Arch. Environ. Contam. Toxicol. 78, 94–105. doi: 10.1007/s00244-019-00676-z
Purcell, J. E., Uye, S., and Lo, W. (2007). Anthropogenic causes of jellyfish blooms and their direct consequences for humans: a review. Mar. Ecol. Prog. Ser. 350, 153–174. doi: 10.3354/meps07093
Richardson, A. J., Bakun, A., Hays, G. C., and Gibbons, M. J. (2009). The jellyfish joyride: causes consequences and management responses to a more gelatinous future. Trends Ecol. Evol. 24:6.
Setälä, O., Fleming-Lehtinen, V., and Lehtiniemi, M. (2014). Ingestion and transfer of microplastics in 445 the planktonic food web. Environ. Pollut. 185, 77–83. doi: 10.1016/j.envpol.2013.10.013
Suchman, C. L., and Sullivan, B. L. (2000). Effect of prey size on vulnerability of copepods to predation by the scyphomedusea Aurelia aurita and Cyanea sp. J. P. R. 22, 2289–2306. doi: 10.1093/plankt/22.12.2289
Sun, X., Li, Q., Zhu, M., Liang, J., Zheng, S., and Zhao, Y. (2017). Ingestion of microplastics by natural zooplankton groups in the northern South China Sea. Mar. Pollut. Bull. 115, 217–224. doi: 10.1016/j.marpolbul.2016.12.004
Templeman, M. A., and Kingsford, M. J. (2010). Trace element accumulation in Cassiopea s. (Scyphozoan) from urban marine environments in Australia. Mar. Environ. Res. 69, 63–72. doi: 10.1016/j.marenvres.2009.08.001
Templeman, M. A., and Kingsford, M. J. (2015). Predicting aqueous copper and zinc accumulation in the upside-down jellyfish Cassiopea maremetens through the use of biokinetic models. Environ. Monit. Assess. 187:416.
Tosetto, L., Williamson, J., and Brown, C. (2017). Trophic transfer of microplastics does not affect fish personality. Anim. Behav. 123, 159–167. doi: 10.1016/j.anbehav.2016.10.035
Turner, J. T. (2004). The importance of small planktonic copepods and their roles in pelagic marine food webs. Zool. Stud. 43, 255–266.
Van Colen, C., Vanhove, B., Diem, A., and Moens, T. (2020). Does microplastic ingestion by zooplankton affect predator-prey interactions? An experimental study on larviphagy. Environ. Pollut. 256:113479. doi: 10.1016/j.envpol.2019.113479
Wallberg, P., Jonsson, P. R., and Andersson, A. (2001). Trophic transfer and passive uptake of a polychlorinated biphenyl in experimental marine microbial communities. Environ. Toxicol. Chem. 20, 2158–2164. doi: 10.1002/etc.5620201004
West, L., Pitt, K., Welsh, D., Koop, K., and Rissik, D. (2009). Top-down and bottom-up influences of jellyfish on primary productivity and planktonic assemblages. Limnol. Oceanogr. 54, 20158–20271.
Widmer, C. L. (2008). How to Keep Jellyfish in Aquariums: An Introductory Guide for Maintaining Healthy Jellies. Tucson: Wheatmark.
Wieczorek, A., Croot, P., Lombard, L., Sheahan, J., and Doyle, T. (2019). Microplastic ingestion by gelatinous zooplankton may lower efficiency of the biological pump. Environ. Sci. Technol. 53, 5387–5395. doi: 10.1021/acs.est.8b07174
Williams, G. C., and Van Syoc, R. (2007). Methods pf preservations and anesthization of marine invertebrates. Preserv. Anesth. 4, 37–41. doi: 10.1525/9780520930438-010
Zhang, Q., Yang, L., and Wen-Xiong, W. (2011). Bioaccumulation and trophic transfer of dioxins in marine copepods and fish. Environ. Pollut. 159, 3390–3397. doi: 10.1016/j.envpol.2011.08.031
Keywords: behavior, ephyrae, immobility, ingestion, polyethylene, zooplankton
Citation: Costa E, Piazza V, Lavorano S, Faimali M, Garaventa F and Gambardella C (2020) Trophic Transfer of Microplastics From Copepods to Jellyfish in the Marine Environment. Front. Environ. Sci. 8:571732. doi: 10.3389/fenvs.2020.571732
Received: 11 June 2020; Accepted: 17 August 2020;
Published: 15 September 2020.
Edited by:
André Ricardo Araújo Lima, Center for Marine and Environmental Sciences (MARE), PortugalReviewed by:
Sarah Nelms, University of Exeter, United KingdomCindy Lee, Clemson University, United States
Copyright © 2020 Costa, Piazza, Lavorano, Faimali, Garaventa and Gambardella. This is an open-access article distributed under the terms of the Creative Commons Attribution License (CC BY). The use, distribution or reproduction in other forums is permitted, provided the original author(s) and the copyright owner(s) are credited and that the original publication in this journal is cited, in accordance with accepted academic practice. No use, distribution or reproduction is permitted which does not comply with these terms.
*Correspondence: Elisa Costa, elisa.costa@ias.cnr.it