- 1Istituto per lo Studio Degli Impatti Antropici e Sostenibilità Ambientale di Capo Granitola, Consiglio Nazionale delle Ricerche (IAS-CNR), Campobello di Mazara, Italy
- 2Istituto di Scienze Marine, Consiglio Nazionale delle Ricerche (ISMAR-CNR), Naples, Italy
- 3Dipartimento di Scienze e Tecnologie Biologiche Chimiche e Farmaceutiche (STEBICEF), Università degli Studi di Palermo, Palermo, Italy
The Gulf of Milazzo (north-eastern Sicily) has been recognized as Italian Site of National Interest (SNI; areas characterized by high level of contamination with potential effects on human health) in 2005 because of its high level of pollution. In this study we measured the concentration of polycyclic aromatic hydrocarbons (PAHs), organochlorine pesticides (OCPs), and polyBrominated diphenyl ether (PBDE) in seawater and sediments sampled from the Gulf of Milazzo in order to assess (i) the environmental status of contamination, and (ii) cancer and non-cancer human health risk potentially due to dermal absorption from contaminated seawater and/or ingestion of contaminated fish. Particularly, POPs content in pelagic and demersal fish of different size classes (small, medium, and large) were estimated, starting from the measured seawater and sediments concentrations, using the KABAM model. In particular, Monte Carlo simulation techniques were applied to address uncertainty in assessment of the risk and to provide quantitative estimates of probability of exposition. Ingestion of contaminated pelagic and demersal fish was the dominant pathway of exposition with high probability of significant cancer risk (Ingestion Cancer Risk >10–4) and significant non-cancer risk (Hazard Index >1). No human health risks emerged to be associated to dermal adsorption from contaminated seawater. Benzo(a)pyrene show the highest Ingestion Cancer Risk with respect to the other PAHs, while the highest Hazard Index for non-cancerogenic molecules was estimated for the PBDE47 congener.
Introduction
Persistent organic pollutants (POPs) are organic compounds with a worldwide occurrence in the marine environment because of their resistance to environmental degradation. The high lipophilicity makes them capable to bioaccumulate in large quantities in tissues of marine organisms. The polycyclic aromatics hydrocarbons (PAHs) are a mixture of aromatic compounds of great environmental concern released both by natural sources (pyrolysis, incomplete combustion of organic matter) and anthropogenic activities (industrial processes, combustion of wood and fossil fuels, motor vehicles, incinerators, oil plants and oil spills). Several researches indicate that high environmental PAHs concentrations are related to increasing incidence of cancer and mutagenic events for exposed organisms (Ramesh et al., 2012). As a consequence, several high molecular weight PAHs have been classified as recognized (class 1), probable (class 2A), or possible (class 2B) human carcinogens (International Agency for Research on Cancer [IARC], 1987). On the other side, the intensifications of agricultural activities has led to increased concentration in the environment of pesticides, a group of POPs belonging to organochlorines compounds (OC). Organochlorines are commonly classified as endocrine disrupting chemicals (EDCs) and are reported to increase the risk of hormone-related cancers by interfering with the endocrine metabolism (Wolff et al., 1993; Sohail et al., 2004). Moreover, epidemiological studies demonstrated a positive correlation between high levels of OCs and incidence of hypertension, cardiovascular diseases and other health-related issues in humans (Subramaniam and Solomon, 2006; Jayaraj et al., 2016). Finally, Polybrominated diphenyl ethers (PBDEs) are a group of emerging ubiquitous POPs used as flame-retardants during the production of textiles, paints, furnitures, electronic circuit boards and plastics. Toxicological studies on laboratory animals demonstrated that PBDEs exposure is positively correlated with thyroid homeostasis disruption, neurotoxic effects, reproductive disorders and cancer (Linares et al., 2015). As recommended by the Marine Strategy Framework Directive (MSFD) (2008/105/CE; Descriptor D8), monitoring studies are needed to assess the environmental risk due to traditional and emerging contaminants and their possible adverse effects on wildlife and human health. The Gulf of Milazzo, located in northern Sicily (southern Italy), has been recognized as Site of National Interest (SNI) in 2005 because of its high level of pollution due to the intensive industrial activities started in the 1950. High arsenic concentrations were recorded in seawater (La Pera et al., 2008) while high concentrations of zinc, lead, cobalt, and copper were recorded in sediments (Pepe et al., 2010). In this paper, we report POPs concentration in seawater and sediments of the Gulf of Milazzo in order to assess the human health risk due to the presence of these contaminants. In particular, we evaluate human cancer and non-cancer risk due to POPs in seawater through two ways of exposure: direct contact by skin during summer activities and by ingestion of contaminated fish. Monte Carlo simulation techniques were run to verify the probability distribution of risk indices, useful for a better assessment of pathways of exposition.
Materials and Methods
Sampling Strategy
Seawater stations (n = 16) were sampled in the Gulf of Milazzo, during two oceanographic cruises: Cisas I Milazzo (July–August, 2017) and Cisas II Milazzo (April, 2018) aboard R/V Luigi Sanzo, at three different depths of the water column (surface, intermediate, and bottom) with 10 L “Niskin” bottles. Sediment samples (n = 8) were collected using a box-corer. The sampling stations are shown in Figure 1. Analyses on sediment samples were carried out on samples collected from the superficial 5 cm depth. Seawater samples were preserved in dark glass bottles, previously cleaned with acetone and rinsed with Milli-Q. All the samples were stored at −20°C, until the analysis. During each survey, hydrological parameters (temperature, salinity, dissolved oxygen, fluorescence, and pH) were measured along the water column (more details are reported in the Supplementary Material). The hydrology study showed evidence of a stratified system with a mixed layer depth (MLD) of about 15 m and an evident thermocline, at about 20 m of depth (Supplementary Material). We measured the contaminants only in surface waters.
Chemical Materials
All reagents used, methanol (CH3OH), methylene chloride (CH2Cl2), n-hexane (n-C6H14), ethyl acetate (C4H8O2), purchased by VWR were pure and pesticides free. The solid phase disk C18 was Empore disk C18 of 50 mm. The PAHs mix standard containing naphthalene (Nap), acenaphthylene (Acn), acenaphthene (Ace), fluorene (Flo), phenanthrene (Phe), anthracene (Ant), fluoranthene (Flu), pyrene (Pyr), benzo[a]anthracene (BaA), chrysene (Chr), benzo(b)fluoranthene (BbF), benzo(k)fluoranthene (BkF), benzo(a)pyrene (BaPy), indeno(1,2,3-cd)pyrene (InP), dibenzo(a,h)anthracene (DahA), and Benzo(g,h,i)perylene (BghiP), with a concentration of 100 μg/ml per each congeners, get by Dr. Ehrenstorfer GmbH (PAH-MIX9), was used to calibrate the instrument. The internal standard was a mix of four deuterated PAHs (PAH Mix 25), and a mix of three more deuterated PAHs (PAH Mix77 get by Dr. Ehrenstorfer GmbH) was used to check the recovery. A mix of PBDE (28, 47, 99, 100, 153, 154, and 209) was prepared ad hoc by LabStandard (Italy). Two mixes of OCPs, one containing Alachlor, Aldrin, Dieldrin, Endrin, Isodrin, DDE, DDD, DDT (Σ isomers), Endosulfan (Σ isomers), with a concentration of 100 mg/l each one; and the other containing Hexachlorobenzene, Hexachlorobutadiene, Hexachlorocyclohexane (Σ isomers), Pentachlorobenzene with a concentration of 100 mg/l each-one, were prepared ad hoc by LabStandard (Italy).
Chemical Analysis
Seawater Analysis
USEPA Method 525.1, EPA 8270D e EPA 8081 was run to extract and analyze POPs from seawater. Particularly, the extraction of POPs from seawater was carried out, according to US EPA Method 525.1, using a solid phase disk bakerbond speedisk C18 of 50 mm and an apparatus of six extractors coupled with a vacuum system. 1 L of seawater sample was poured onto the disk previously cleaned with 10 ml of CH2Cl2, conditioned with 20 ml of CH3OH and 20 ml of distilled water (with 0.5% of CH3OH). The elution was run by (i) drying the disk, venting air for at least 10 min with a vacuum pump; (ii) pouring 10 ml of C4H8O2 (ethyl acetate) onto the disk (this step was necessary to eliminate any remaining water from the disk and to increase the recovery of the contaminants); (iii) pouring 10 ml of a mix 50:50 of C4H8O2/CH2Cl2 and at last 20 ml of CH2Cl2. All eluted solvents were collected in the same test tube and dried using a multivapor (got by BÜCHI). Finally, 1 ml of n-Hexane (containing internals standard) was spilled inside the test tube and recovered for GC/MS. Analysis was carried out using a GC/MS Triple Quadrupole (by Thermo Fisher GC Trace 1310 coupled with a TSQ8000 mass spectrometry and Triplus RSH autosampler). The GC was equipped with a DB-5 ms capillary column (30 m × 0.25 mm, 0.25 μm) and with a PTV injector set in large volume mode. PAHs, OCPs and PBDE were determined in SRM Mode as reported in the Thermo Scientific application note 52389.
Sediment Analysis
USEPA Method 3545, EPA 8270D, EPA 8081 was used to extract and analyze POPs from sediments. Synthetically, 2 g of dry, sieved and homogenized sediment, spiked with surrogate standards, was extracted by Accelerated Solvent Extraction (ASE 200, DIONEX, Thermo Scientific) using a hexane/acetone (80:20 v/v) mixture. The clean up was run using SPE Silica Gel and SPE Florisil to PAH and OCPs, respectively. The final extracts were analyzed by Gas Chromatography (GC-MS ISQ; Thermo Finnigan) with Mass Spectrometric detection in Selective Ion Monitoring (SIM) mode for PAH with a limit of detection estimated as 1 μg/kg for each PAH and by Gas Chromatography with mass spectrometric Ion-Trap with tandem mode (GC-Ion Trap Polaris; Thermo Finnigan) in MSN mode for OCPs with a limit of detection estimated as 0,25 μg/kg.
Total Organic Carbon in Sediments
Total organic carbon was determined by a Thermo Electron Flash EA 1112 coupled to a Thermo Electron Delta V Advantage mass spectrometer. Analysis was carried out after elimination of all of the carbonate present in the samples (ca. 10–15 mg of bulk sediment samples were de-carbonated using HCl 1 M in silver cups for 24 h at ambient temperature and then dried in an oven at 60°C). An internal standard (urea with C = 20%, N = 46%) was run every six samples. The detection limit for TOC measurements was established at 0.05%, considering chromatogram peak >500 mV and correspondingly determining the sample weight.
Quality Control
Laboratory quality control procedures for PAHs, OCPs and PBDEs both in seawater and sediments included analyses of blanks, spiked samples and reference materials for sediments. The recovery measured on artificial seawater spiked with a final concentration of 100 ng/l per each contaminant was between 75 and 115%. A mix of deuterated PAH with a final concentration of 10 ng/l was added before the extraction to monitor the recovery percentage (PAH deuterated MIX) in each seawater sample. The reproducibility measured by three consecutive extractions was less than 20%.
The reference material used for quality control, on sediment analyses, was SRM 1941b-NIST.
The recoveries for each analyte of PAHs ranged between 94 and 107%, the reproducibility was about 10% for all substances, and the accuracy was estimated greater than 10% for each single analysis. The SRM 1941b-NIST.reference material was also used to measure the accuracy of total organic carbon percentage (TOC%) in sediment. The accuracy and reproducibility were about 5 and 0,4%, respectively.
Human Health Risk Assessment
The human health risk for resident population was assessed hypothesizing two routes of contaminant exposure:
(1) Dermal adsorption during summer activities (e.g., swimming).
(2) Ingestion of contaminated fishes.
Dermal Cancer and Hazard Risk
Dermal Hazard Risk (DHR) and Dermal Cancer Risk (DCR) were assessed according to US EPA protocol, Risk Assessment Guidance for Superfund: part E (RAGS, E), following the equations 1–5:
where FA is the fraction of the adsorbed water, Kp is dermal permeability coefficient calculated per each compound (cm/h) as function of Kow and molecular weight, Cw is pollutant concentration in surface water (ng/l), τ event is lag time per event (h/event), τ_event is the duration of the event (h/event), EV is the frequency of the event (events/day), ED is the exposure duration (years), EF is the frequency of exposure (days/year), SA is the skin surface area (cm2), BW is the body weight (kg), AT is the average lifespan (years, AT = ED for not cancer risk), SFo is Cancer Slope Factor oral (mg/kg/day), RfDo is oral Reference Dose (mg/kg/day), and ABSGI is the fraction of contaminant absorbed in the gastro-intestinal tract. The values of these parameters are shown in Table 1. The combined DCR associated to all congeners of PAH was calculated as Benzo(a)pyreneTEQ (Eq. 6)
where TEFi is Toxicity Effect Factor and Ci is the concentration of each congener in seawater (ng/l). The combined DCR associated to all POPs was calculated as the sum of each carcinogenic compound that can injury the same target organ.
Contaminant Concentrations in Fish Tissues
Contaminant concentrations in fish were simulated using the model KABAM (Kow based Aquatic Bioaccumulation Model) following Arnot and Gobas (2004), who parameterized a bioaccumulation model in aquatic ecosystems using the octanol-water partition coefficient (Kow) to estimate uptake and elimination constants through respiration and diet of organisms in different trophic levels. We used the model to estimate the concentration of contaminants in edible tissues of pelagic and demersal fish of 3 size classes, small fish (SF, 10–100 g), medium fish (MF, 100–1,000 g), and large fish (LF, −1,000 g), starting from the contaminant concentrations in seawater column and in pore waters, respectively. Contaminant concentrations in seawater and sediments were calculated as the average of concentrations measured in all samples during the two surveys. We used values corresponding at half of detection limit for not quantifiable measures. We used the sediment/liquid partition coefficients (Kd = Koc⋅ foc = Csediment/Cpore water) to estimate contaminant concentrations in pore waters starting from concentrations measured in sediment samples. Time to steady-state (Tss) for each compound was calculated using Kow as follows:
Contaminant concentrations in fish were normalized at 2-year life for each compound with Tss greater than 730 days (2 years). Furthermore, we set the KABAM model (ecosystem input paragraph) according to the diet and habitat of the fish.
Ingestion Cancer and Hazard Risk
The Average Daily potential Dose (ADD) was calculated as follows:
where Cfish is the estimated concentration of contaminant in fish tissue, IR is the Ingestion Rate (gr/days); EF is the Exposure Frequency (days/years); ED is the Exposure Duration (years); BW is the Body Weight (kg) and AT is the average lifespan (years, AT = ED for not cancer risk). The values of these parameters are shown in Table 1. The Ingestion Cancer Risk (ICR) was calculated multiplying the ADD by the Cancer Slope Factor oral (CSF) of each contaminant:
The combined ICR associated to all POPs was calculated as the sum of each compound that can injury the same organ. The Hazard Quotient risk (HQ) regarding non-cancerogenic health issues was calculated dividing the ADD by the Reference Dose oral (RfDo) of each contaminant:
Statistical Analysis
Monte Carlo simulation techniques with 5,000 iterations were applied to estimate variability and uncertainty in risk assessment. The repeated sampling was based on probability distributions of contaminant concentrations in water and fish. The procedure was performed by R 3.6.1 (R Core Team, 2019). Differences of contaminant contribution for the ingestion cancer risk were tested by a non-parametric test (Kruskal–Wallis test) and by pairwise comparison. The level of significance was set at p < 0.05.
Results and Discussion
Occurrence of Contaminants in the Study Area
The concentrations of PAHs, OCPs, and PBDEs in seawater and superficial sediments of the Gulf of Milazzo are shown in Table 2. Higher concentrations of low molecular weight PAHs (sum of congeners with 2 and 3 rings) were found in the water column because of their greater solubility, while high molecular weight PAHs (sum of congeners 4, 5 and 6 rings) were more abundant in sediments. Alachlor is the most concentrated OCPs in seawater (average 2.84 ng/l) followed by DDE (average 1.06 ng/l), while PBDE47 is the most concentrated PBDEs (3.58 ng/l). Contaminant concentrations in seawater samples are lower than the maximum admissible concentrations (MAC) set by the Italian Regulations n.172/2015 (application of the European Directive 2013/39/EU, 2008/105/CE, and 2000/60/CE) except for Benzo(ghi)Perylene and ΣPBDE that exceeds the threshold limits in 13 and 5 sampling stations, respectively. Depending on PAHs contamination, seawater could be classified as: micro-polluted, ΣPAHs 10–50 ng/l; light-polluted, ΣPAHs 50–250 ng/l; moderately polluted, ΣPAHs 250–1,000 ng/l and heavily polluted, ΣPAHs >1,000 ng/l according to Chen (2008). The concentrations of ΣPAHs in seawater ranged from 1.6 to 70.3 ng/l, showing that the site could be classified as micro-polluted/light-polluted by PAHs. Particularly, light-polluted seawaters were found in the stations MZ13 (ΣPAHs = 59.2 ng/l) and MZ43 (ΣPAHs = 70.3 ng/l). PAHs concentrations in sediments (26.94–862.0 μg/kg), are much higher than organochlorine pesticides (2.50–3.72 μg/kg) and PBDE concentrations (0.93–3.72 μg/kg). Hexachlorobenzene (HCB) is the most concentrated organochlorine pesticide in sediments followed by DDD and DDT, while the same concentrations were found for PBDE congeners. Contaminant concentrations in superficial sediments are lower than the maximum admissible concentrations (MAC) set by the European Directive 2013/39/EU, except for Fluoranthene concentrations in 2 sampling stations (MZ13 and MZ33), Benzo(b)fluoranthene in 1 sampling station (MZ13), Benzo(k)fluoranthene in 1 sampling station (MZ13) and Benzo(a)pyrene in 1 sampling station (MZ13). Total PAH concentrations, sediments may be classified according to Soclo et al. (2000) as highly contaminated with PAHs > 500 μg/kg, moderately contaminated with PAHs > 250, and slightly contaminated with PAHs < 250 μg/kg. Our data demonstrate that the superficial sediment samples of the Gulf of Milazzo cover the entire range of contamination. Particularly, high PAHs contamination was found in the stations MZ13 (ΣPAHs = 861 μg/kg) and MZ33 (ΣPAHs = 544 μg/kg). Relatively low values of organic chlorinated pesticides (OCPs) concentrations were found in seawaters and superficial sediment samples suggesting a reduced level of this class of contaminant contribution from agricultural activities in the study area. A comparison of PAHs concentrations in sediments from other industrialized marine sites is shown in Table 3. No sufficient data on OCP and PBDE concentrations in the Mediterranean Sea are available in literature for robust and statistically significant comparisons. This work offers one of the first complete datasets of these classes of contaminants in seawater and sediments from highly contaminated industrial areas.
PAHs Source Identification
The identification of the PAHs source was assessed calculating Fluo/Fluo + Pyr and Ant/Ant + Phe ratios per each sample. Fluo/Fluo + Pyr <0.40 show a petroleum contamination, between 0.40 than 0.50 a liquid fossil fuel (vehicle and crude oil) combustion and >0.50 are characteristic of grass, wood, or coal combustion; Ant/Ant + Pyr <0.1 petroleum and >0.1 pyrogenic sources (Yunker et al., 2002; He et al., 2014; Soliman et al., 2014; Ya et al., 2014). Fluo/Fluo + Pyr and Ant/Ant + Pyr show mean value of 0.41(±0.14) and 0.28(±0.14) in seawater and 0.54(±0.07) and 0.21(±0.2) in sediment. These results suggest both petroleum contamination and pyrogenic source due to refinery activities/marine traffic and thermopower plant.
Health Risk Assessment
Cancer Risk Assessment
The Dermal Cancer Risk (DCR) due to dermal absorption of all contaminants in surface seawater (ΣDCR) for each sampling station ranged from 4.8⋅ 10–7 to 6.0⋅ 10–6. These values are shown in the distribution map (Figure 2), using inverse distance weighting (IDW) and setting a buffer with a fixed distance of 1 km from sampling station, in order to identify the most dangerous area. The average values of DCR for each contaminant ranged from 10–13 to 10–7 (Table 4). The cancer risk due to fish ingestion ranged from 10–13 to 5.0⋅ 10–4 (Table 4). We estimated the combined cancer risk for different organs supposing additive carcinogenic effects of contaminants with the same target. The information about the target organs of each contaminant was checked using IRIS database. The combined DCR per target organs ranged from 7.0⋅ 10–9 for breast cancer to 9.4⋅ 10–7 for lung cancer, while the combined ICR per target organs ranged from 1.7⋅ 10–7 for breast cancer to 5⋅ 10–4 for gastro-intestinal cancer (Table 5). The target organ with the highest potential to develop a carcinogenic event is the gastro-intestinal tract (followed by liver, skin, lung, and breast) because of the higher ICR associated with BaPy (2.7⋅ 10–4, Table 4). The risks here analyzed are compared with the acceptable risk levels proposed by the international agencies. The US EPA assumed acceptable risk level in a range from 10–6 to 10–4 (Environmental Protection Agency [EPA], 1991). No remediation measures are required when the cancer risk falls within this range. The US EPA assumes the unconditionally acceptable risk level below 10–6 (Environmental Protection Agency [EPA], 2004) while a risk greater than 10–4 requires protective measures to mitigate the risk. All the DCRs here found were under the USEPA acceptable range (10–6–10–4). We recorded ICRs above the upper bound of the USEPA acceptable range (10–4) due to the ingestion of demersal medium and large fishes and pelagic large fishes (Tables 4, 5), suggesting a significant risk to develop cancer for the resident population. The probabilistic distributions of Dermal Cancer Risk (DCR) and Ingestion Cancer Risks (ICR) for small (SF), medium (MF), large (LF) pelagic, and demersal fish were obtained from Monte Carlo simulations (Figures in Supplementary Material). The descriptive statistical values (including 5th percentile, 25th percentile, median, 75th percentile and 95th percentile) are reported in Table 7. Integration of variability can lead to a more realistic evaluation of risk estimation, that could be affected by data scarcity, parameter variability and model limitations (Chen et al., 2015; Qu et al., 2015). According to the guideline established by United States Environmental Protection Agency [US EPA] (1991), the unconditionally acceptable risk level is below 10–6 and the acceptable risk levels range from 10–6 to 10–4. All the simulated statistical values of DCR and ICR of PBDE of pelagic and demersal fish were lower than 10–6. On the contrary, the density distributions of ICR due to PAHs exposure from ingestion of pelagic and demersal fish estimated the unacceptable risk level (10–4) in correspondence with the 93th percentile for medium pelagic fishes, the 80th percentile for large pelagic fishes, the 85th percentile for small demersal fishes, the 65th percentile for medium demersal fishes, and 38th percentile for large demersal fishes. This means that the probability to exceed the unacceptable threshold is 7, 20, 15, 35, and 62%, respectively. About 5% of ICR due to exposure to OCPs for large pelagic fish, 8% for medium demersal fish and 22% for large demersal fish is above the unacceptable risk level (10–4).
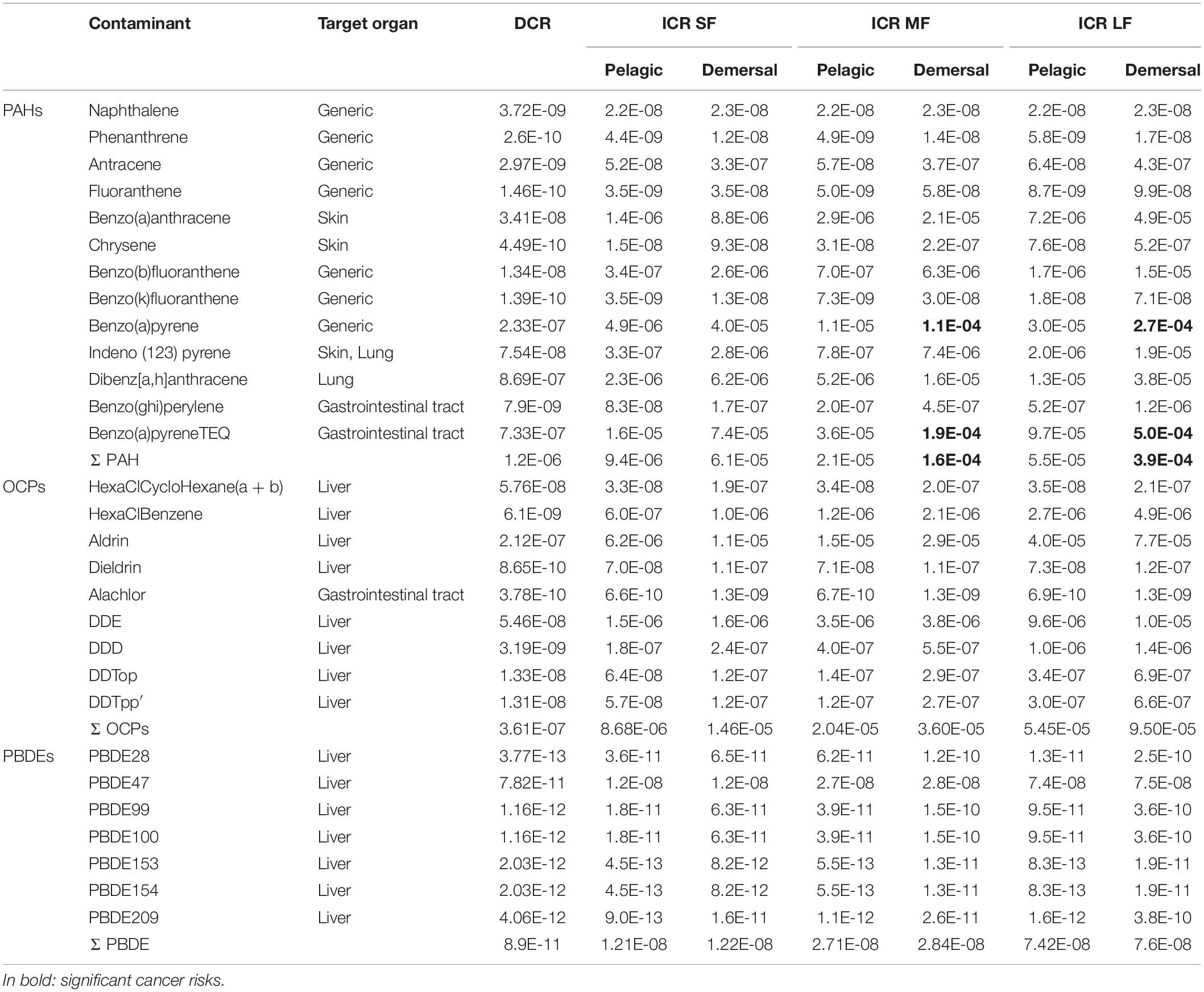
Table 4. Mean Ingestion Cancer Risk (ICR) for small (SF), medium (MF), large (LF) pelagic, and demersal fish and mean Dermal Cancer Risk (DCR).
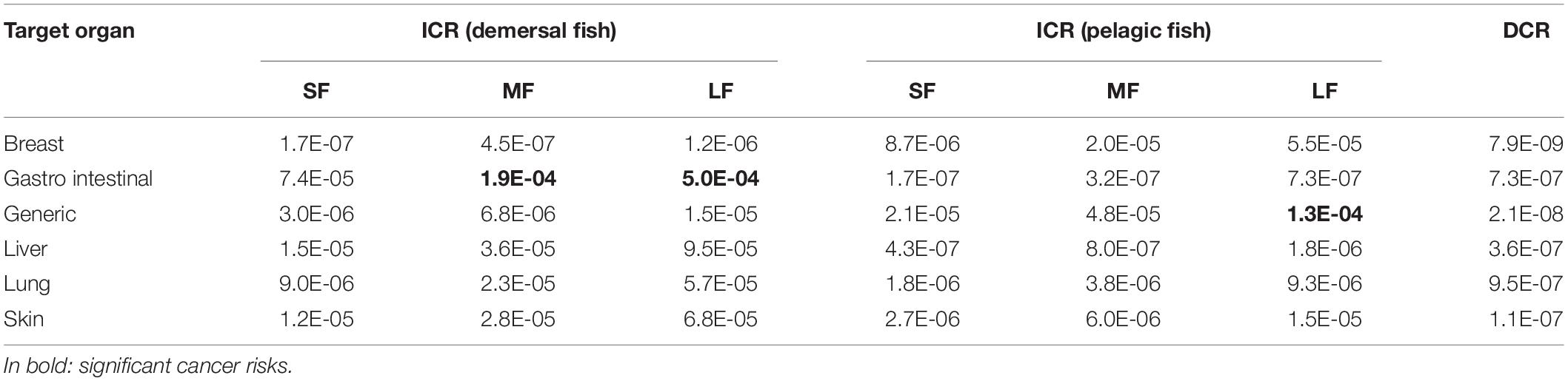
Table 5. Combined Ingestion Cancer Risk for small (SF), medium (MF), large (LF) demersal-pelagic fishes, and Combined Dermal Cancer Risk calculated for the target organ of each carcinogenic contaminant.
Non-cancer Risk Assessment
Hazard Quotients (HQ) were applied for the evaluation of non-carcinogenic health risk through ingestion and dermal adsorption of single contaminant. It is well known that POPs exert hemato-, cardio-, renal-, neuro-, immuno-, reproductive toxic effects in laboratory animals and humans (Ramesh et al., 2004; WHO, 2010). Hazard Index (HI) were applied to evaluate the non-cancerogenic health risk through ingestion and dermal adsorption of the sum of contaminants found in the study area. The hazard quotients calculated for the dermal adsorption (DHQ) and the ingestion of contaminated fishes (IHQ) and hazard index (HI) for the sum of all POPs are given in Table 6. We found HQ values less than 1 for all the measured POPs due to ingestion and dermal adsorption, which indicated little or no potential adverse effects on local residents’ health through ingestion and/or dermal absorption of a single contaminant. The exception is represented by the HQ of PBDE47 due to the ingestion of pelagic and demersal large fishes (IHQ = 2.47 and 2.49, respectively; Table 6). According to Table 6, the HIs due to the ingestion of pelagic and demersal medium-large fishes are above the unity, indicating potential adverse effects on local resident’s health due to the ingestion of a mix of pollutants from contaminated commercial fishes. Thus, it can be concluded that the mix of pollutants can cause harmful non-carcinogenic health effects in the resident population of Milazzo. The HQs and HI due to dermal absorption of all contaminants are negligible. The HI values are shown in a distribution map (Figure 3) using inverse distance weighting (IDW). The probabilistic distributions of Dermal Hazard Risk (DHR) and the Ingestion Hazard Risks (IHR) for small (SF), medium (MF), large (LF) pelagic and demersal fishes were obtained from Monte Carlo simulations (Figures in Supplementary Material). The descriptive statistical values (5th percentile, 25th percentile, median, 75th percentile and 95th percentile) are reported in Table 8. According to the guideline established by United States Environmental Protection Agency [US EPA], 1991, the acceptable risk levels is below the unit. All the simulated statistical values of DHR and IHR were lower than 1, except for IHR from OCPs exposition of medium and large pelagic fish and of all demersal fish and PBDEs exposition of all fishes. Specifically, the estimated probability to exceed the unacceptable threshold (1) is 5% for IHR due to exposure to OCPs from ingestion of medium pelagic fishes, 32% from large pelagic fishes, 25% from small demersal fishes, 84% from medium demersal fishes and 55% from large demersal fishes. Moreover, the exposition to PBDEs determinate a percentage of 9% of IHR higher than the unit for small pelagic fishes, 25% for medium pelagic fishes, 71% for large pelagic fishes, 8% of small demersal fishes, 25% for medium demersal fishes and 70% for large demersal fishes.
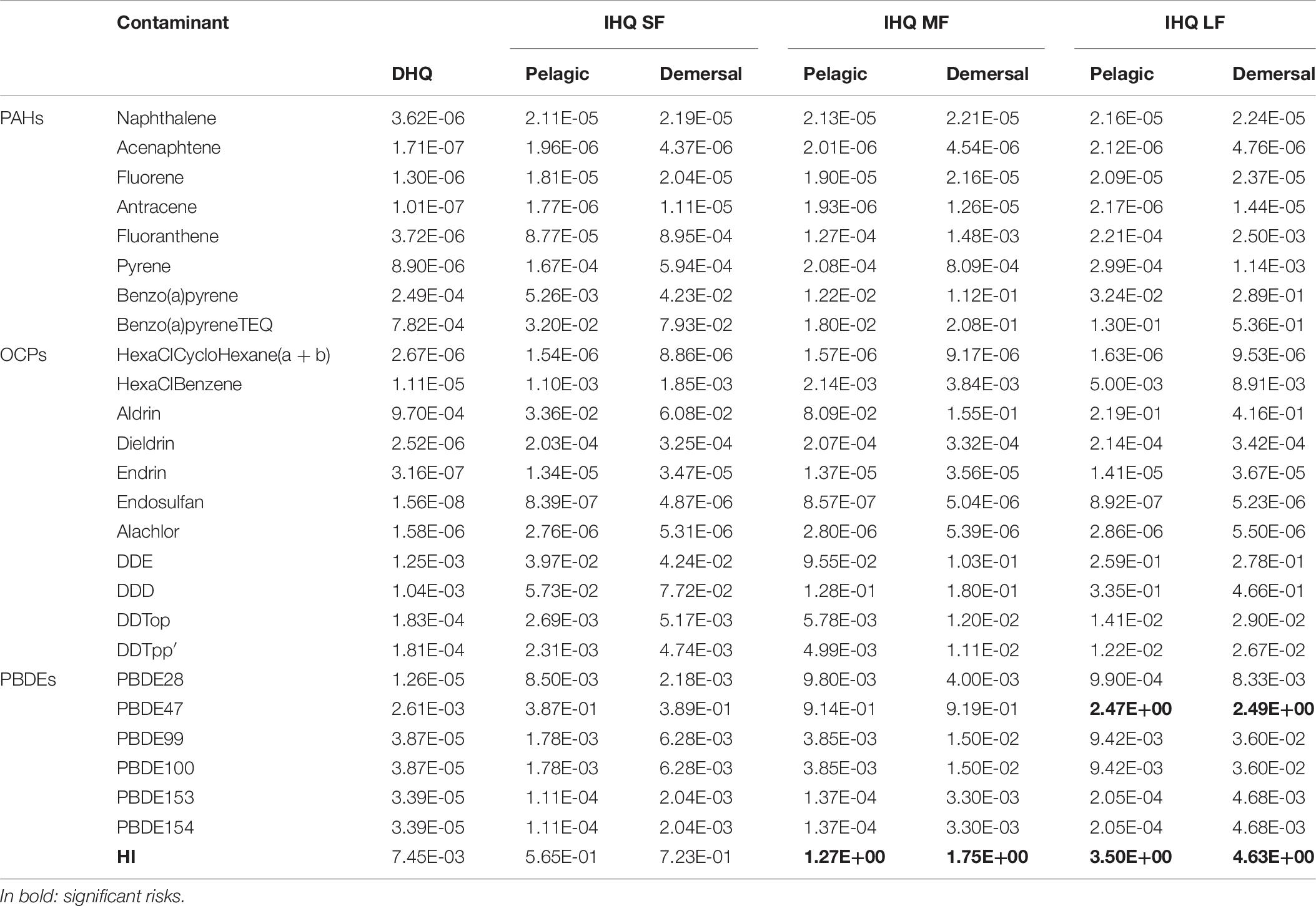
Table 6. Ingestion Hazard Quotient (IHQ) for small (SF), medium (MF), large (LF) pelagic and demersal fishes, Dermal Hazard Quotient (DHQ) and Hazard Index (HI) as the sum of the HQs.
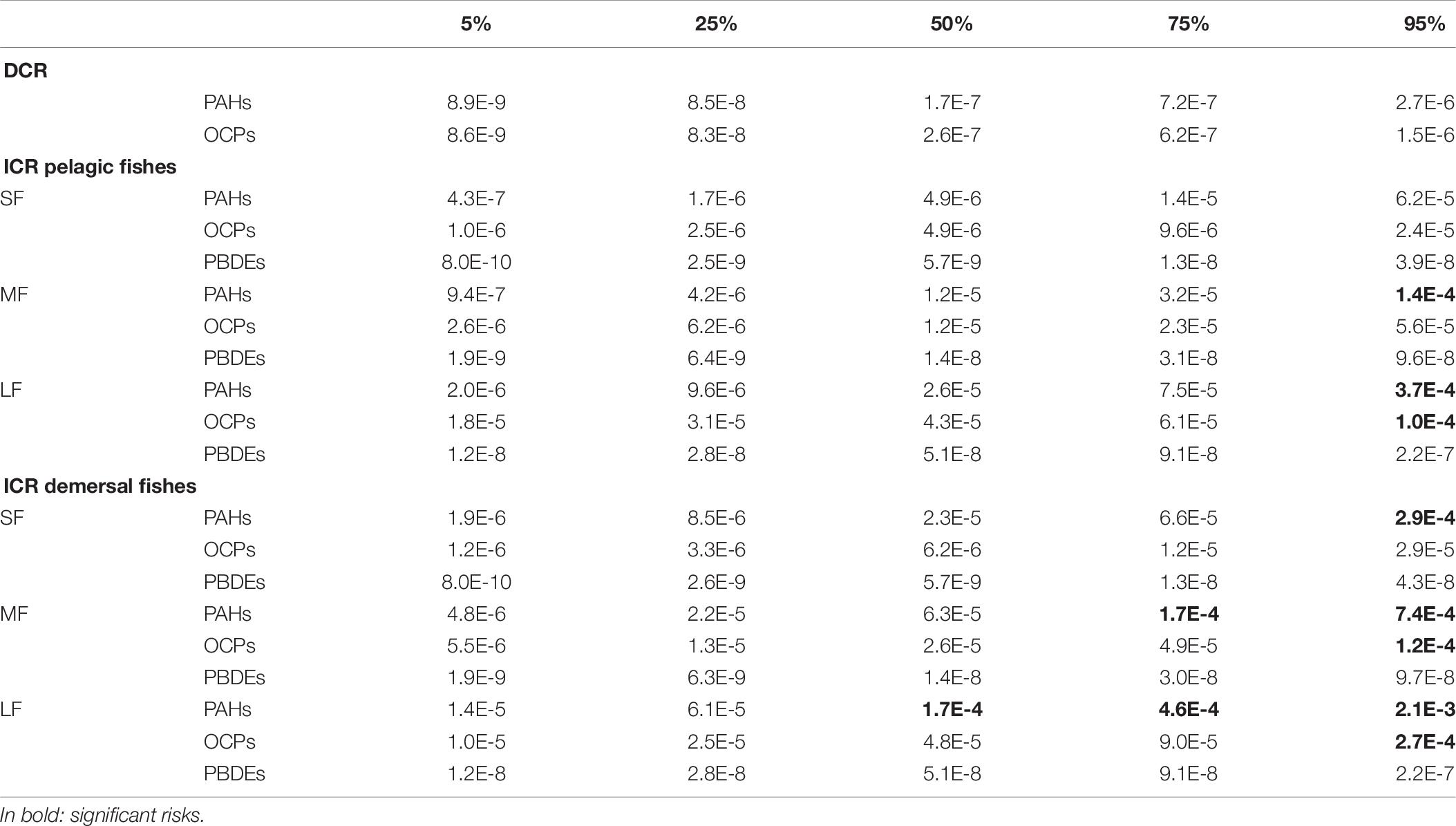
Table 7. Monte Carlo simulation, probabilistic distribution of DCR and ICR, statistical values (percentiles).
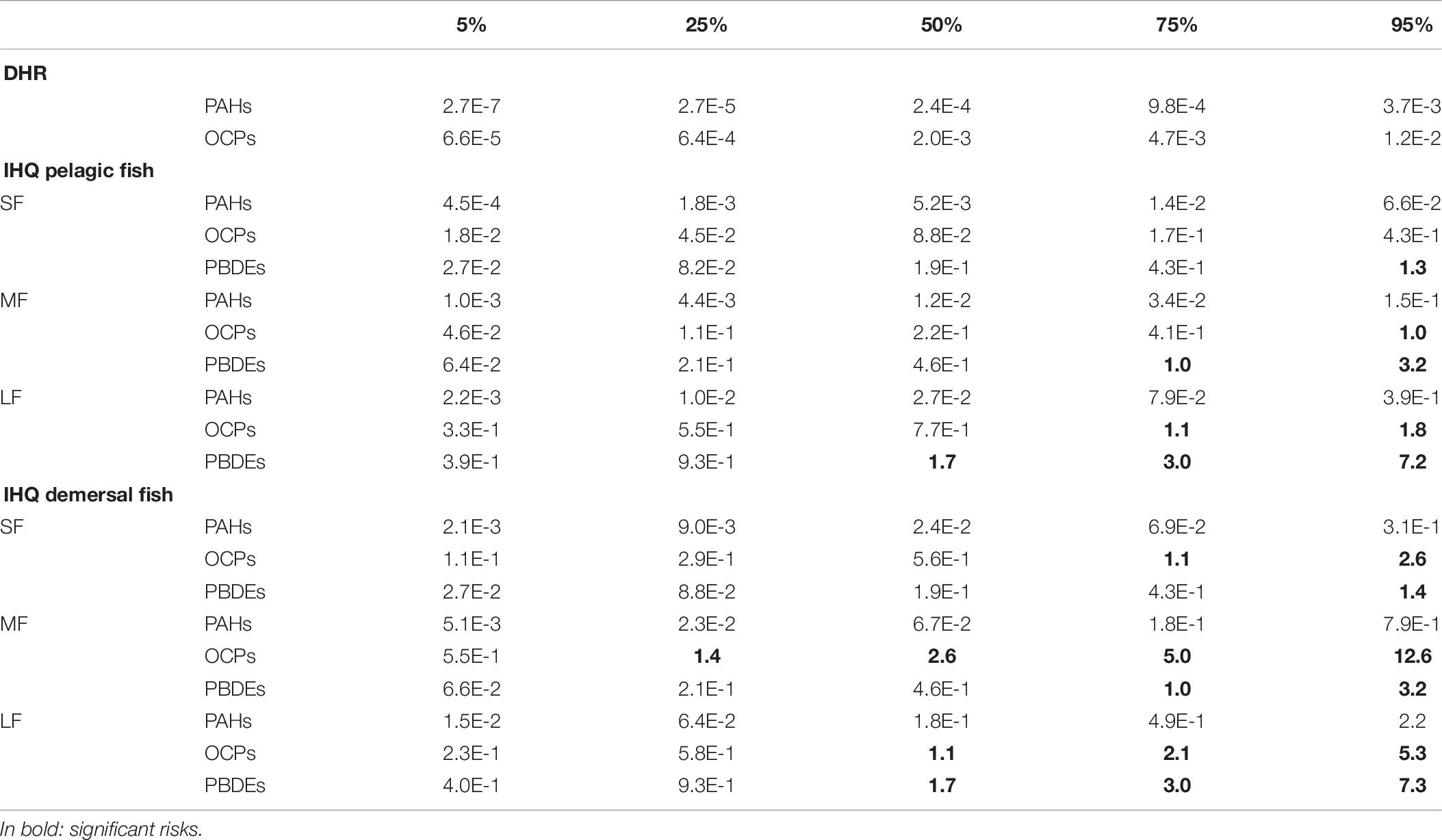
Table 8. Monte Carlo simulation, probabilistic distribution of DHR and IHR, statistical values (percentiles).
Contribution of Different Pathways
It is noteworthy that food ingestion is the main pathway of contaminants exposure for humans when compared with other routes such as inhalation, dermal contact and drinking water (Lioy et al., 1988; Butler et al., 1993). Studies conducted on human exposure to BaPy revealed that the range and magnitude of dietary exposures (2–500 ng/day) were larger for inhalation and dermal contact (Lioy et al., 1988). Diet makes a substantial contribution (more than 70%) to the exposure to PAHs (Beckman et al., 1998; Phillips, 1999). We found significantly higher ICRs with respect to DCRs that could be affecting the resident population, confirming these assumptions. As expected, higher ICRs were recorded for large pelagic/demersal fish due to biomagnification process that enhances the bioaccumulation factor of contaminants in the large fishes that live on the top of the food chains. The affinity of POPs for organic materials in sediments are high enough to cause accumulation in this matrix with long-lasting consequences for environmental health. Thus, sediment-dwelling, filtering organisms and demersal fishes are most susceptible to POPs contamination. Our results reported higher ICRs for demersal fishes with respect to pelagic fishes due to their feeding and breathing in the pore water in contact with contaminated sediments.
Contribution of Different Contaminants
Statistically significant differences (KW test, p = 0,002) between contaminant contribution for the ICRs were evidenced. Particularly, higher PAHs contribution was recorded concerning OCPs and PBDEs because of their higher concentrations in seawater and sediments of the study area. BaPy has the highest ICR with respect to the other PAH congeners that exceed the upper bound of EPA acceptable range (2.7⋅ 10–4 for the ingestion of large demersal fishes, Table 3). BaPy is the most known and studied member of PAHs because of its highest carcinogenic potential (Howard and Fazio, 1980). A lot of epidemiological studies confirm the strong relationship between the ingestion of highly contaminated food with BaPy and risk for gastro-intestinal cancer, particularly stomach, esophagus (Ward et al., 1997) and colorectal cancer (Schiffman and Felton, 1990; Muscat and Wynder, 1994; Sinha et al., 1999). BaPy concentration higher than the MAC set by the Directive 2013/39/EU here found in sediment of the sampling station MZ13 located near the industrial pole (where both thermopower plant and refinery plant are present), suggest a significant anthropogenic input of this contaminant in the study area with a relevant health risk for local populations. Although a not statistically significant level, the higher mean contribution of OCPs was found for non-cancerogenic health issues with respect to PAHs and PBDEs contributions (Table 5). OCPs, such as Endosulphan and Lindane (γ-hexachlorocyclohexane), are well known to exert neurotoxic effects inhibiting the calcium ion influx and Ca- and Mg-ATPase and causing release of neurotransmitters (Mathew, 2012). Moreover, epidemiological studies have shown that exposure to OCPs is strongly associated with type 2 diabetes (Lee et al., 2006) and Parkinson’s disease (Steenland et al., 2014). As shown in Table 5, the HI associated to OCPs and due to the ingestion of large demersal fishes is higher than 1 suggest that local population could experience these pathologies after a long-time exposure of OCPs. As shown in Table 2, all the OCPs concentrations in seawater and sediment samples are lower than the MAC set by the Directive 2013/39/EU. This study evidence that the threshold limits imposed by legislation could not be sufficiently protective against a long-time exposure of a mix of highly toxic contaminants. Although the OCPs has the highest mean contribution, the highest HI was reported for PBDE47 (HI = 2,5; Table 5). PBDE-47 is an emerging contaminant diffuse worldwide in the marine environment at constant increasing concentrations (Hites et al., 2004; Schecter et al., 2007) although its use has been banned in many countries. In spite of these concern, limited information on the PBDE47 toxicity is available. Exposure of neonatal mice to PBDE47 is reported to exert neurodevelopment toxicity causing behavioral alterations, learning and memory deficits and dysfunctions in the cholinergic system in adult stage (Eriksson et al., 2001, 2002; Branchi et al., 2003). PBDE47 exceed the MAC set by the Directive 2013/39/EU in seawater column of 5 sampling stations, suggesting relevant contamination of this pollutant in the study area.
Uncertainty
As reported in the Basic Information about the Integrated Risk Information System (IRIS), Cancer Oral slop factor (CSFo), and Reference Dose (RfDo) are parameters estimated with uncertainty due to limitations of the data used and could represent an uncertainty factor for risk assessment. Fixed exposure factors, commonly used in risk assessment, may not be adequately accurate in reproducing reality because of their variability due to different life stages and/or different environmental condition. Finally, the cancer risks per target organs here calculated are probably underestimated for the lack of information about carcinogenic action of every single PAH on human. For example, we established a specific carcinogenic action of BaPy in the gastro-intestinal tract based on IRIS database, excluding other target organs for the carcinogenic action of this compound for lack of evidence in humans. However, several studies with experimental animals suggest that oral, intratracheal and subcutaneous injection of BaPy led to carcinogenic events in multiple sites (IARC, 2010). Furthermore, BaPy has been proven to induce breast tumors in animal through genotoxic activities implied in p53 mutations (Morris and Seifter, 1992). This information suggests that the carcinogenic action of BaPy could affect other organs and not exclusively the gastro-intestinal tract. Moreover, uncertainty is not considered in the models applied to mixtures of compounds thus possibly affecting their mobility, partitions and health impacts.
Conclusion
The study investigated, for the first time, persistent and emerging pollutants in seawater and sediments of the Gulf of Milazzo. The concentration of BghiP, PBDE in seawater samples and BbF, BkF, BaPy in sediment samples higher than MAC show a low quality of this marine environment.
The human health risk was calculated through cancer and non-cancer risk indices. These indices provide only point estimates giving little information about uncertainty and variability surrounding the risk impact. Therefore, Monte Carlo simulation was necessary to provide complete information on the likelihood of the various risk levels. The cancer risk assessment shows higher risks for resident population through the ingestion of large demersal fish, especially gastro-intestinal cancer caused by BaPy. Negligible cancer risks were associated with dermal adsorption of contaminants from seawater. The non-cancer risk assessment (HI) shows significant risks for resident population to develop health issues due to the ingestion of large demersal fish contaminated by mixture of the analyzed pollutants. OCPs and especially PBDE47 represent the major contribution for non-cancer risk. The present study might provide, assuming permanent environmental conditions, useful information on human exposure to POPs in Milazzo bay and will be useful for strategies focused on risks mitigation. Particular attention should be done to reduce BaPy emission from refinery plant, thermopower plant and maritime transport. The use of other less toxic flame retardants than PBDE47 for the production of plastic and fiber materials is also recommended.
Data Availability Statement
All datasets generated for this study are included in the article/Supplementary Material.
Author Contributions
FD’A led the conception and design of the manuscript. AB led the conception and writing of the manuscript. MD led the sampling strategy. EQ carried out the statistical analysis and developed the risk map. SG carried out the chemical analysis on sediment samples. FP carried out the interpretation of oceanographic data. NS carried out the total organic carbon analysis on sediment samples. GB led the oceanographic survey. GA and VD contributed to carry out the chemical analysis on seawater samples and to review the manuscript. All authors contributed to the article and approved the submitted version.
Funding
The CISAS project is a multidisciplinary project on environment/health relationships funded by the Italian Ministry of Education, Universities and Research (MIUR) and approved by the Interministerial Committee for Economic Planning (CIPE) – body of the Italian Government – with Resolution n. 105/2015 of 23 December 2015.
Conflict of Interest
The authors declare that the research was conducted in the absence of any commercial or financial relationships that could be construed as a potential conflict of interest.
Acknowledgments
The authors thank the project of the National Research Council of Italy (CNR) titled “International Center of Advanced Study in Environment, Ecosystem and Human Health (CISAS).”
Supplementary Material
The Supplementary Material for this article can be found online at: https://www.frontiersin.org/articles/10.3389/fenvs.2020.00117/full#supplementary-material
References
Alebic-Juretic, A. (2011). Polycyclic aromatic hydrocarbons in marine sediments from the Rijeka Bay area, Northern Adriatic, Croatia, 1998-2006. Mar. Poll. Bull. 62, 863–869. doi: 10.1016/j.marpolbul.2011.01.035
Branchi, I., Capone, F., Alleva, E., and Costa, L. G. (2003). Polybrominated diphenyl ethers: neurobehavioral effects following developmental exposure. Neurotoxicology 24, 449–462. doi: 10.1016/s0161-813x(03)00020-2
Antizar-Ladislao, B. (2009). Polycyclic aromatic hydrocarbons, polychlorinated biphenyls, phthalates and organotins in northern Atlantic Spain’s coastal marine sediments. J. Environ. Monit. 11, 85–91. doi: 10.1039/b808668k
Arnot, J. A., and Gobas, F. A. (2004). A food web bioaccumulation model for organic chemicals in aquatic ecosystems. Environ. Toxicol. Chem. 23, 2343–2355.
Asia, L., Mazouz, S., Guiliano, M., Doumenq, P., and Gilbert Mille, G. (2009). Occurrence and distribution of hydrocarbons in surface sediments from Marseille Bay (France). Mar. Pollut. Bull. 58, 443–451. doi: 10.1016/j.marpolbul.2008.11.022
Baumard, P., Budzinski, H., and Garrigues, P. (1998). PAHs in Arcachon Bay, France: origin and biomonitoring with caged organisms. Mar. Pollut. Bull. 36, 577–586. doi: 10.1016/s0025-326x(98)00014-9
Beckman, S. O., Thuvander, A., and Andersson, C. (1998). Preview of PAHs in Food: Potential Health Effects and Contents in Food, Report 8. Uppsala: Swedish National Food Administration.
Butler, J. P., Post, G. B., Lioy, P. J., Waldman, J. M., and Greenberg, A. (1993). Assessment of carcinogenic risk from personal exposure to benzo(a)pyrene in the total human environmental exposure study (THEES). J. Air Waste Manage. Assoc. 43, 970–977. doi: 10.1080/1073161x.1993.10467179
Chen, S., Chen, B., and Fath, B. D. (2015). Assessing the cumulative environmental impact of hydropower construction on river systems based on energy network model. Renew. Sustain. Energy Rev. 42, 78–92. doi: 10.1016/j.rser.2014.10.017
Chen, Y. (2008). The Spatial and Temporal Distribution, Source and Bioavailability of PAHs in Qiantang River. Hangzhou: College of Environmental and Resource Science, 15–16.
De Luca, G., Furesi, A., Leardi, R., Micera, G., Panzanelli, A., Piu, P., et al. (2004). Polycyclic aromatic hydrocarbons assessment in the sediments of the Porto Torres Harbor (Northern Sardinia. Italy). Mar. Chem. 86, 15–32. doi: 10.1016/j.marchem.2003.11.001
Environmental Protection Agency [EPA] (1991). Risk Assessment Guidance for Superfund. Vol. I. Human Health Evaluation Manual. Part B. Development of Risk-based Preliminary Remediation Goals. Interim. EPA/540R-92-003. Publication 9285.7-01B. Washington, DC: US Environmental Protection Agency.
Environmental Protection Agency [EPA] (2004). Risk Assessment Guidance for Superfund. Volume I, Human Health Evaluation Manual, Part E, Supplemental Guidance for Dermal Risk Assessment, EPA/540/R/99/005. Washington, DC: Environmental Protection Agency [EPA].
Eriksson, P., Jakobsson, E., and Fredriksson, A. (2001). Brominated flame retardants: a novel class of developmental neurotoxicants in our environment? Environ. Health Perspect. 109, 903–908. doi: 10.1289/ehp.01109903
Eriksson, P., Viberg, H., Jakobsson, E., Orn, U., and Fredriksson, A. (2002). A brominated flame retardant, 2,2’,4,4’,5-pentabromodiphenyl ether: uptake, retention, and induction of neurobehavioral alterations in mice during a critical phase of neonatal brain development. Toxicol. Sci. 67, 98–103. doi: 10.1093/toxsci/67.1.98
He, X., Pang, Y., Song, X., Chen, B., Feng, Z., and Ma, Y. (2014). Distribution, sources and ecological risk assessment of PAHs in surface sediments from Guan River Estuary, China. Mar. Pollut. Bull. 80, 52–58. doi: 10.1016/j.marpolbul.2014.01.051
Health Canada [HC] (2007a). Federal Contaminated Site Risk Assessment in Canada. Part I: Guidance on Human Health Preliminary Quantitative Risk Assessment. Version 2.0. Ottawa: Health Canada.
Health Canada [HC] (2007b). Federal Contaminated Site Risk Assessment in Canada. Part II: Health Canada Toxicological Reference Values (TRVs). Version 2.0. Ottawa: Health Canada.
Hites, R. A., Foran, J. A., Carpenter, D. O., Hamilton, M. S., Knuth, B. A., and Schwager, S. J. (2004). Global assessment of organic contaminants in farmed salmon. Science 303, 226–229. doi: 10.1126/science.1091447
Howard, J. W., and Fazio, T. (1980). Analytical methodology and reported findings of polycyclic aromatic hydrocarbons in foods. Ass. Offic. Anal. Chem. 63, 1077–1104. doi: 10.1093/jaoac/63.5.1077
IARC (2010). Some non-heterocyclic polycyclic aromatic hydrocarbons and some related exposures. IARC Monogr. Eval. Carcinog. Risks Hum. 92, 1–853.
ICRAM (2006). Progetto Preliminare di Bonifica Della Rada di Augusta Inclusa nel Sito di Bonifica di Interesse Nazionale di Priolo. Fase I e II. Elaborazione Definitiva # BoI-Pr-SI-PR-Rada di Augusta-03.22.
International Agency for Research on Cancer [IARC] (1987). Overall Evaluations of Carcinogenicity: An Updating of IARC Monographs. Lyon: International Agency for Research on Cancer, 1–42.
IRIS (2007). US EPA. Integrated Risk Information System. Online. IRIS Advanced Search. Available online at: https://cfpub.epa.gov/ncea/iris/search/index.cfm
Jayaraj, R., Megha, P., and Sreedev, P. (2016). Organochlorine pesticides, their toxic effects on living organisms and their fate in the environment. Interdiscip. Toxicol. 9, 90–100. doi: 10.1515/intox-2016-0012
Ju, T., Ge, W., Jiang, T., and Chai, C. (2016). Polybrominated diphenyl ethers in dissolved and suspended phases of seawater and in surface sediment from Jiaozhou Bay, North China. Sci. Total Environ. 55, 571–578. doi: 10.1016/j.scitotenv.2016.03.013
Khairy, M., Kolb, M., Mostafa, A., Fiky, A., and Bahadir, M. (2009). Risk assessment of polycyclic aromatic hydrocarbons in a Mediterranean semi-enclosed basin affected by human activities (Abu Qir Bay, Egypt). J. Hazard. Mater. 170, 389–397. doi: 10.1016/j.jhazmat.2009.04.084
La Pera, L., Di Bella, G., Rando, R., Lo Turco, V., and Dugo, G. (2008). Speciation of inorganic arsenic in coastal seawater from Ionian and Tyrrhenian Seas (Sicily, Italy) using derivative anodic stripping chronopotentiometry. Environ. Monit. Assess. 145, 119–126. doi: 10.1007/s10661-007-0021-8
Lee, J. Y., Kim, Y. P., Kang, C. H., Ghim, Y. S., and Kaneyasu, N. (2006). Temporal trend and long-range transport of particulate PAHs at Gosan in Northeast Asia between 2001 and 2004. J. Geophys. Res. 111:D11303. doi: 10.1029/2005JD006537
Linares, V., Bellés, M., and Domingo, J. L. (2015). Human exposure to PBDE and critical evaluation of health hazards. Arch. Toxicol. 89, 335–356. doi: 10.1007/s00204-015-1457-1
Lioy, P. L., Waldman, J. M., and Greenberg, A. (1988). The Total Human Environmental Exposure Study (THEES) to benzo(a)pyrene: comparison of the inhalation and food pathways. Arch. Environ. Health 43, 304–312. doi: 10.1080/00039896.1988.10545954
Liu, Y., Zheng, G. J., Yu, H., Martin, M., Richardson, B., Lam, M., et al. (2005). Polybrominated diphenyl ethers (PBDEs) in sediments and mussel tissues from Hong Kong marine waters. Mar. Pollut. Bull. 50, 1173–1184. doi: 10.1016/j.marpolbul.2005.04.025
Magi, E., Bianco, R., Ianni, C., and Di Carro, M. (2002). Distribution of polycyclic aromatic hydrocarbons in the sediments of the Adriatic Sea. Environ. Pollut. 119, 91–98. doi: 10.1016/s0269-7491(01)00321-9
Mathew, L. L. (2012). Organochloride Pesticide Toxicity. Drugs, Diseases and Procedures. Medscape References.
Moon, H.-B., Kannan, K., Lee, S.-J., and Choi, M. (2007). Polybrominated diphenyl ethers (PBDEs) in sediment and bivalves from Korean coastal waters. Chemosphere 66, 243–251. doi: 10.1016/j.chemosphere.2006.05.025
Morris, J. J., and Seifter, E. (1992). The role of aromatic Hydrocarbons in the genesis of breast cancer. Med. Hypothes. 38, 177–184. doi: 10.1016/0306-9877(92)90090-y
Muscat, J. E., and Wynder, E. L. (1994). The consumption of well-done red meat and the risk of colorectal cancer. Am. J. Publ. Health 84, 856–858. doi: 10.2105/ajph.84.5.856
Pepe, F., Scopelliti, G., Di Leonardo, R., and Ferruzza, G. (2010). Granulometry, mineralogy and trace elements of marine sediments from the Gulf of Milazzo (NE Sicily): evaluation of anthropogenic impact. Italian J. Geol. 129, 385–394.
Qiu, Y. W., Zhang, G.-Q., Liu, G., Guo, L.-L., Li, X.-D., and Wai, O. (2009). Polycyclic aromatic hydrocarbons (PAHs) in the water column and sediment core of Deep Bay, South China. Estuar. Coast. Shelf Sci. 83, 60–66. doi: 10.1016/j.ecss.2009.03.018
Qu, C., Li, B., Wu, H., Wang, S., and Giesy, J. P. (2015). Multi-pathway assessment of human health risk posed by polycyclic aromatic hydrocarbons. Environ. Geochem. Health 37, 587–601. doi: 10.1007/s10653-014-9675-7
R Core Team (2019). R: A language and Environment for Statistical Computing. Vienna: R Foundation for Statistical Computing. Available online at: https://www.R-project.org/
Ramesh, A., Hood, D., Guo, Z., and Loganathan, B. (2012). “Global environmental distribution and human health effects of polycyclic aromatic hydrocarbons,” in Global Contamination Trends of Persistent Organic Chemicals, 1st Edn, eds B. G. Loganathan and P. Kwan-Sing Lam (Boca Raton, FL: CRC Press).
Ramesh, A., Walker, S. A., Hood, D. B., Gullen, M. D., Schneider, K., and Weyand, E. H. (2004). Bioavailability and risk assessment of orally ingested polycyclic aromatic hydrocarbons. Int. J. Toxicol. 23, 301–333. doi: 10.1080/10915810490517063
Schecter, A., Johnson-Welch, S., Tung, K. C., Harris, T. R., Papke, O., and Rosen, R. (2007). Polybrominated diphenyl ether (PBDE) levels in livers of U.S. human fetuses and newborns. J. Toxicol. Environ. Health A 70, 1–6. doi: 10.1080/15287390600748369
Schiffman, M., and Felton, J. S. (1990). Fried foods and the risk of colon cancer. Am. J. Epidemiol. 131, 376–378. doi: 10.1093/oxfordjournals.aje.a115508
Sinha, R., Chow, W. H., Kulldorff, M., Denobile, J., Butler, J., Garcia-Closas, M., et al. (1999). Well-done, grilled red meat increases the risk of colorectal adenomas. Cancer Res. 59, 4320–4324.
Soclo, H. H., Garrigues, P. H., and Ewald, M. (2000). Origin of polycyclic aromatic hydrocarbons (PAHs) in coastal marine sediments: case studies in Cotonou (Benin) and Aquitaine (France) Areas. Mar. Pollut. Bull. 40, 387–396.
Sohail, E., Waseem, A., Chae, W. L., Jong, J. L., and Imitiaz, H. (2004). Endocrine disrupting pesticides: a leading cause of cancer among rural people in Pakistan. Exp. Oncol. 26, 98–105.
Soliman, Y. S., Al-Ansari, E., and Wade, T. (2014). Concentration, composition and sources of PAHs in the coastal sediments of the exclusive economic zone (EEZ) of Qatar, Arabian Gulf. Mar. Pollut. Bull. 85, 542–548.
Steenland, K., Mora, A. M., Barr, D. B., Juncos, J., Roman, N., and Wesseling, C. (2014). Organochlorine chemicals and neurodegeneration among elderly subjects in Costa Rica. Environ. Res. 134, 205–209.
Subramaniam, K., and Solomon, R. D. (2006). Organochlorine pesticides BHC and DDE in human blood in and around Madurai, India. Indian J. Clin. Biochem. 21, 169–172.
United States Environmental Protection Agency [US EPA] (1991). Risk Assessment Guidance for Superfund (RAGS), Vol. I: Human Health Evaluation Manual (HHEM), Part B, Development of Risk-Based Preliminary Remediation Goals, Publication 9285.7-01B, NTIS PB92-963333. Washington, DC: Office of Solid Waste and Emergency Response.
Ward, M. H., Sinha, R., Heineman, E. F., Rothman, N., Markin, R., Weisenburger, D. D., et al. (1997). Risk of adenocarcinoma of the stomach and esophagus with meat cooking method and doneness preference. Int. J. Cancer 71, 14–19.
WHO (2010). Some Non-Heterocyclic Polycyclic Aromatic Hydrocarbons and Some Related Exposures. IARC Monographs on the Evaluation of Carcinogenic Risks to Humans, Vol. 92. Lyon: International Agency for Research on Cancer.
Wolff, M. S., Toniolo, P. G., Lee, E. W., Rivera, M., and Dubin, N. (1993). Blood levels of organochlorine residues and risk of breast cancer. J. Natl. Cancer Inst. 21, 648–652.
Ya, M., Wang, X.-H., Wu, Y., Ye, C., and Li, Y.-Y. (2014). Enrichment and partitioning of polycyclic aromatic hydrocarbons in the sea surface microlayer and subsurface water along the coast of Xiamen Island, China. Mar. Pollut. Bull. 78, 110–117.
Keywords: human health risk, KABAM, polycyclic aromatic hydrocarbons, organochlorine pesticides, polybrominated diphenyl ether, bio-accumulation factor, risk assessment
Citation: D’Agostino F, Bellante A, Quinci E, Gherardi S, Placenti F, Sabatino N, Buffa G, Avellone G, Di Stefano V and Del Core M (2020) Persistent and Emerging Organic Pollutants in the Marine Coastal Environment of the Gulf of Milazzo (Southern Italy): Human Health Risk Assessment. Front. Environ. Sci. 8:117. doi: 10.3389/fenvs.2020.00117
Received: 16 October 2019; Accepted: 30 June 2020;
Published: 18 August 2020.
Edited by:
Ethel Eljarrat, Institute of Environmental Assessment and Water Research (CSIC), SpainReviewed by:
Cristina Silva Pereira, New University of Lisbon, PortugalNasri Ahmed, Carthage University, Tunisia
Copyright © 2020 D’Agostino, Bellante, Quinci, Gherardi, Placenti, Sabatino, Buffa, Avellone, Di Stefano and Del Core. This is an open-access article distributed under the terms of the Creative Commons Attribution License (CC BY). The use, distribution or reproduction in other forums is permitted, provided the original author(s) and the copyright owner(s) are credited and that the original publication in this journal is cited, in accordance with accepted academic practice. No use, distribution or reproduction is permitted which does not comply with these terms.
*Correspondence: Fabio D’Agostino, fabio.dagostino@cnr.it; Antonio Bellante, antonio.bellante@ias.cnr.it