- 1Department of Biological and Environmental Sciences, University of Gothenburg, Gothenburg, Sweden
- 2IVL Swedish Environmental Research Institute, Stockholm, Sweden
- 3Swedish Chemicals Agency (KemI), Sundbyberg, Sweden
The role of microplastics as chemical vectors delivering environmental contaminants into biota has been proposed, but their environmental relevance remains an issue of a debate. In this paper we compared the propensity and relative importance of synthetic polymer microparticles [glassy polystyrene (PS) and rubbery polyethylene (PE)] and silica glass particles (SG) to act as vectors for hydrophobic organic chemicals (HOCs) into fish after ingestion. Particles were spiked with three HOCs [17α-ethinylestradiol, chlorpyrifos and benzo(α)pyrene], which differ in hydrophobicity and induce well-known biomarker responses. Three-spined stickleback were exposed to 8 different diets: control diets (1), diets with non-spiked particles (2–4), diets containing a mixture of particles spiked with 3 model contaminants (5–7) and, finally, diets loaded with only the chemical mixture (8), for 14 days. Chemical sorption onto the particles was quantified and chemical transfer into the fish was investigated via biomarkers (CYP1a, ERα, VTG, and AChE) in fish intestine, liver and brain and quantification of HOCs in fish muscle. Results demonstrated particle-mediated chemical transfer of moderately hydrophobic contaminants into fish. While PS and PE particles mediated higher chemical transfer and tissue accumulation of 17α-ethinylestradiol and chlorpyrifos than SG, the overall chemical transfer was found to be very low. The present work suggested that chemical sorption, desorption and subsequent transfer of chemicals in vivo depends on multiple interconnected factors, including physicochemical properties of particles and contaminants, as well as toxicokinetic and toxicodynamic interactions. The biomarker approach was, however, suboptimal for assessing chemical transfer when addressing particle-associated chemical mixtures.
Introduction
Microplastics (MPs) are widely known to sorb and concentrate hydrophobic organic chemicals (HOCs) from the ambient environment (Mato et al., 2001; Teuten et al., 2007; Rochman et al., 2013a; Prokic et al., 2019). Dozens of potentially toxic and persistent chemicals have been found associated with plastic debris, stimulating hypotheses indicating MPs as potential carriers, or vectors for hazardous environmental chemicals into aquatic organisms (Mato et al., 2001; Teuten et al., 2009; Hirai et al., 2011). While some field and laboratory studies demonstrate the capacity of MPs to facilitate chemical transfer into biota, the ecological importance of this phenomenon remains poorly understood and widely debated. Some experimental data suggest that certain polymers have the ability to sorb and accumulate HOCs to a greater extent than sediments and suspended organic particles (Mato et al., 2001; Teuten et al., 2007; Wang and Wang, 2018), however the comparative importance of chemical sorption to synthetic versus natural particulates, and subsequent transfer into organisms, remains relatively unknown.
Modeling studies strongly suggest that MPs play a negligible role for chemical influx into biota and that the magnitude of MP-mediated chemical exposure is low compared to other exposure pathways, such as uptake via food, other particulates or aqueous exposure (Gouin et al., 2011; Koelmans et al., 2016, 2013). The abundance of various mineral or organic particulates in the aquatic environment drastically exceeds the current reported levels of MPs (Ogonowski et al., 2017; Guzzetti et al., 2018; Alimba and Faggio, 2019). However, MPs concentrations in the aquatic environment are expected to increase in the future due to projected increase in plastics emission (Jambeck et al., 2015; Burns and Boxall, 2018; Everaert et al., 2018). The propensity of vector phenomenon remains an issue of discussion, especially while addressing the environmental relevance compared to other naturally occurring particulates (Koelmans et al., 2016; Ziccardi et al., 2016). Thus, it is important to improve our understanding about the role and relative importance of MP-mediated contaminant transfer, compared to naturally occurring particulates.
Also, knowledge regarding the potential for different polymers to first sorb and then transfer contaminants into aquatic animals is limited. Partitioning of hydrophobic environmental chemicals into plastic polymers is governed by both sorbent and sorbate properties (Lee et al., 2014). In polymers, the sorptive capacity of HOCs is highly dependent on the properties of the polymer matrix, such as segmental mobility and degree of cross-linking and crystallinity, porosity, as well as surface area and structure (Ngoc et al., 2010). Plastic polymers can be differentiated into glassy (adsorptive) or rubbery (absorptive) polymers, depending on their glass transition temperature (Hüffer and Hofmann, 2016; Hartmann et al., 2019). Rubbery polymers, such as polyethylene (PE), have amorphous regions where polymer segments are more mobile and flexible, allowing chemical sorption into the plastic (absorption), whereas in glassy polymers, such as polystyrene (PS), polymer chains are more condensed and cross-linked, thus favoring adsorption of HOCs onto their surfaces, or partitioning of chemicals into the nano-sized pores (Teuten et al., 2009). Also, the hydrophobicity of chemicals has been identified as one of the main properties governing chemical sorption onto polymer particles (Lee et al., 2014; Hüffer and Hofmann, 2016; Hartmann et al., 2017; Müller et al., 2018) and is important for not only for chemical sorption, but also for desorption, determining the influx of the chemicals into biota.
Ingestion of MPs has been suggested as an alternative pathway for chemical exposure and uptake of HOCs into aquatic organisms (Rochman et al., 2013b). As ingested particles can reside and, to some extent, accumulate in the gastrointestinal tract (Lu et al., 2016; Skjolding et al., 2017; Ding et al., 2018), the intestinal lumen represents an important interface between ingested particles and associated chemicals and the organism (Hartmann et al., 2017). Artificial intestinal fluids have been shown to augment chemical desorption from MPs (Bakir et al., 2014), suggesting that physiological conditions in the gastrointestinal tract can enhance chemical desorption, leading to subsequent intestinal absorption and distribution into an organism. However, the biological fate and processes governing chemical desorption of contaminants associated with MPs and other solid indigestible microparticles in an organism following ingestion remain largely unknown (Batel et al., 2016), and require further investigations.
The present work explored the propensity and relative importance of two types of synthetic MPs (glassy PS, rubbery PE) and one type of naturally occurring particles (silica glass) to act as vectors for pollutants into aquatic organisms. We quantified the chemical sorption and chemical transfer of three model HOCs into the fish. The chemicals used differed in hydrophobicity: a synthetic estrogen - 17α-ethinylestradiol (EE2; log Kow = 3.87), a chlorinated organophosphorous pesticide - chlorpyrifos (CPS; log Kow = 4.66) and a commonly used model PAH - benzo(α)pyrene (BαP; log Kow = 5.99). The spiked particles were used in a feeding experiment with three-spined stickleback and subsequent chemical transfer of model chemicals was investigated using well-established genetic and enzymatic biomarkers. We analyzed biological responses in different tissues of the fish to address local effects at the site of particle intake (intestine) and systemic chemical distribution (liver, brain). The accumulation of the chemical compounds was investigated in fish muscle.
Materials and Methods
Fish
Three-spined stickleback (Gasterosteus aculeatus) were chosen as a model species in this study for several reasons. The species are widely spread; they are present in both salt and fresh water systems throughout the Northern Hemisphere. They can be easily caught and kept under laboratory conditions, and due to high tolerance for handling, fish can successfully be used in experimental studies involving sublethal markers (Katsiadaki et al., 2006). Fish were caught in a shallow creek at the west coast of Sweden (Skaftö, Sweden) in April, 2017. The fish were housed in the Department of Biological and Environmental Sciences, University of Gothenburg, in artificial sea water (13 ± 1°C, 30%, pH = 7.9) under a constant photoperiod - light:dark (12:12 h). Fish were fed daily ad libitum with red mosquito larvae. Fish husbandry and feeding experiments were conducted in compliance with ethical practices from Swedish Board of Agriculture (Ethical permit number: 220-2013).
Microparticles and Model Chemicals
To assess the relative importance of MPs and naturally occurring particles to sorb and transfer HOCs, we used two types of synthetic polymer particles: glassy polystyrene (PS) and rubbery polyethylene (PE), and silica glass microparticles (SG) as a reference model of natural particles (Figure 1, and Supplementary Table S1). Commercial PE particles (reported size: 212–250 μm) were purchased from Cospheric (Santa Barbara, CA, United States) and PS particles (reported size: 250 μm) were purchased from Goodfellow Cambridge Ltd. (Huntington, United Kingdom). Silica lime glass particles (reported size: 250 μm) were also obtained from the commercial supplier (Cospheric, Santa Barbara, CA, United States).
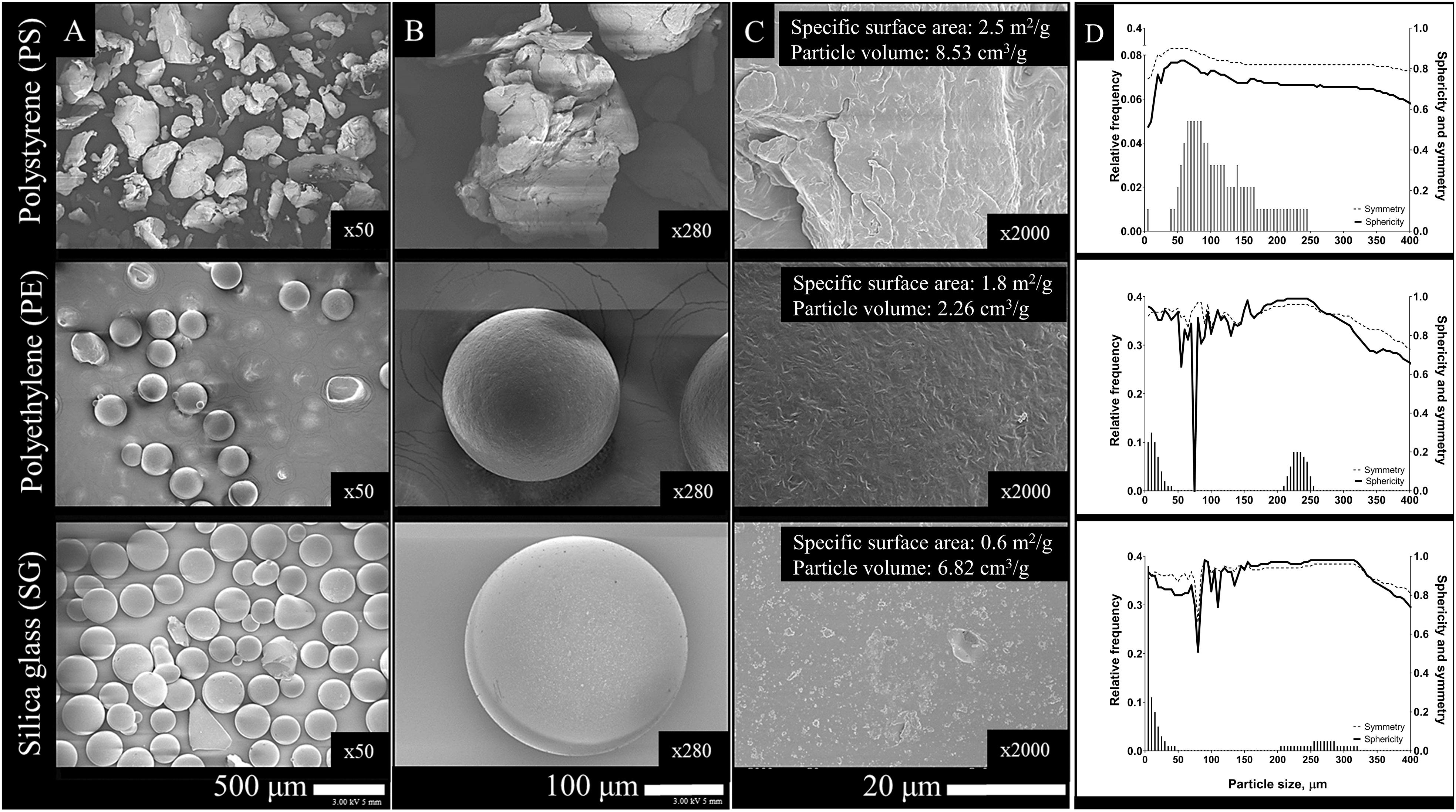
Figure 1. Morphometric characterization of experimental microparticles (PS, PE, and SG): particle morphology and surface topography (A–C) and particle size distribution, sphericity and symmetry (D). Specific N2-BET surface area and particle volume is complementarily provided (C).
The synthetic estrogen 17α-ethinylestradiol (EE2; log Kow = 3.87) acts as a ligand for estrogen receptor (ER), upregulating egg yolk protein vitellogenin (VTG). Chlorpyrifos is a chlorinated organophosphorous pesticide (CPS; log Kow = 4.66) and is known to act as an acetylcholine esterase (AChE) inhibitor (Supplementary Table S2). Benzo(α) pyrene (BαP; log Kow = 5.99) is a well-studied polycyclic aromatic hydrocarbon (PAH), and commonly used compound in MPs vector studies (Batel et al., 2016; Donovan et al., 2018; Pittura et al., 2018). BαP is a potent aryl hydrocarbon (AhR) receptor agonist, inducing cytochrome P450 (CYP1a) metabolism. Octanol-water partitioning coefficients for the model compounds were determined with EPI (Estimation Programs Interface) Suite KOWWIN Program (United States Environmental Protection Agency, United States, v. 1.67, 2000–2012) at pH = 7.
Particle Characterization
Particle morphology and surface topography were determined using scanning electron microscopy (SEM) (DSM 982 Gemini, Zeiss, DE) in a backscattered mode. Prior to imaging, particles (PS, PE, and SG) were spatter-coated with a gold layer (5 nm) (Turbomolecular-pumped coating system, Q150T ES, Quorum Technologies Ltd., United Kingdom). To determine particle size distribution and particle numbers (Figure 1), a single subsample of each type of commercial particle was analyzed with CAMSIZER (Retch Technology, DE) using Xarea model estimations, as described previously (Ašmonaite et al., 2018). Additionally, N2-BET surface areas and porosity of particles were estimated (3Flex Surface Characterization Analyzer, Micromeritics, United States) (Figure 1). Cumulative particle volume estimation was obtained using spherical approximation, applied on Camsizer data in each particle size category (Supplementary Table S1).
Chemical Spiking and Chemical Analysis
To ensure detection of chemicals in the tissues after the feeding experiments, the amount of chemicals to be used in the chemical spiking process was back-calculated from the chemical-analytical detection limits, amount of food ingested, body weight, with expected loss of 30% of each chemical (Smedes and Booij, 2012). Microparticles (PS, PE, and SG) were spiked with each model HOC to a nominal concentration of 1.2 mg chemical/g of particles in separate spiking experiments. Prior to spiking, each chemical was dissolved in a solvent: for BαP a mixture of acetonitrile (ACN):methanol (MeOH) (1:1) was used, and for CPS and EE2 solely MeOH was used. The final ratio between particles and solvent was adjusted to 1:1 (w:w) (Smedes and Booij, 2012). The spiking experiments were performed in glass tubes (100 mL) initially containing 6 mL solvent-particle suspension to which Milli-Q water was added to force the partitioning of chemicals into the particles. Water was added to the particle/chemical mixture-suspension daily with an increasing volume: 2; 4; 8; 16, and 20 mL to reach final water content of 90% after a period of 5 days. Tubes were kept on a shaking table throughout the entire procedure at room temperature. After the final addition of water, the particles were dried by filtering the solutions using a cartridge with a metal frit.
To investigate the final amounts of substances attached to the particles, liquid chromatography–tandem mass spectrometry (LC/MS-MS) was used for the EE2 and CPS, and High Performance Liquid Chromatography with Fluorescence Detection (HPLC-FLD) for BαP. A single aliquot of the particles was extracted using MeOH (for EE2 and CPS) or MeOH:ACN (1:1) (for BαP) followed by ultra-sonication for 40 min. The extractions were pooled and internal standards (IS) added: EE2-d4 (14 μg) for EE2, carbamazepine-carboxamide-13C,15N (100 ng) for CPS before analysis. No IS was used for BαP. For EE2 and CPS, a LC-20 Prominence high performance LC system (Shimadzu, Kyoto, Japan) coupled to an API 4000 Q TRAP triple quadropole MS (Applied Biosystems/MDS Sciex, Toronto, CA) was used. CPS was analyzed using positive electron spray ionization (ESI+) and EE2 was analyzed using negative electron spray ionization (ESI–). The LC column was a C18–XBridge (2.5 μm i.d., 3.0 × 50 mm, BEH Technology, USA) with a guard column. The mobile phases used for the CPS and EE2-analysis were: A: 1 mM ammonium fluoride (NH4F) in H2O and B: ACN:MeOH (1:1 v/v). The acquisition and processing of data were performed using Analyst software (v. 1.6.3, Applied Biosystems/MDS Sciex instruments, CA).
To compare sorption between different types of particles, the sorption efficiency was estimated for each model HOC (Eq. 1):
HOCs sorption was estimated primarily using mass units (Figure 2A). Complementary, mass-based sorption estimations were used to calculate sorption per particle surface area (Figure 2B) and particle volume (Figure 2C).
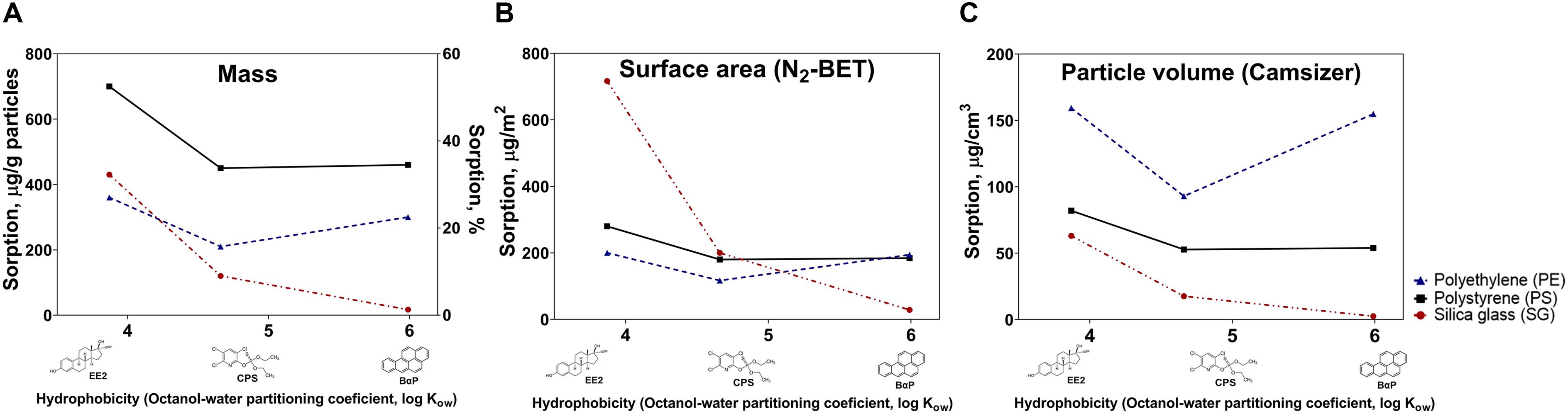
Figure 2. Sorption of model compounds (EE2, CPS, and BαP) on experimental microparticles (PE, PE, and SG) measured per mass of particles (A; μg/g and %) and sorption estimates normalized for specific N2-BET surface area (B; μg/m2) and particle volume (C; μg/cm3).
Feeding Experiment
In the study, a non-equilibrium experimental design was used, where uncontaminated fish were fed with contaminated diet (Koelmans et al., 2016). Eight different types of experimental feeds were prepared for feeding trials: (1) negative control, i.e., fish food without added particles or chemicals; (2–4) feeds each containing one type of virgin particles (5% of daily food intake; PS, PE, SG) without added chemicals; (5–7) feeds each containing one type particle spiked with EE2, CPS, and BαP (subsequently labeled PSMix, PEMix, SGMix); (8) feed loaded with a mixture of the three chemicals but without added particles (Mix). For each exposure treatment, fish (N = 8 × 2; 1.45 ± 0.32 g) were randomly allocated into in two (replicate) glass aquaria (19 L, 35 × 21 × 26). Experiments were conducted in well-aerated artificial seawater (35 PSU) under semi-static conditions with manual water exchange every second day. During the experiment, fish were fed daily (6% of their body weight) for a period of 14 days. The fish consumed all food rapidly (in less than 1 minute), and no particle dissolution into the water was observed prior to ingestion. Maximum daily exposure doses to model HOCs (associated with spiked particles) were estimated (Eq. 2):
Preparation of Fish Feed
Red mosquito larvae were used as basis for experimental feeds (crude protein 3%, crude fat 3%, ash 0.8%, fiber 0.9%) (Imazo AB, Vara, SE). For feed preparation, thawed red mosquito larvae (40 mL) were homogenized in batches using a high performance dispersing instrument Ultra-Turrax® (Labasco, Janke and Kunkel KG, IKA Werk, DE) (50 Hz, u/min 20 000, 30 s), then centrifuged at 700 rpm for 1 min and re-homogenized for 30 s. To eliminate non-grinded material, the homogenate was sieved with a fine-threaded net (Ø 1 mm). Gelatin sheets were soaked in cold Milli-Q water for 10 min, the excess water was drained, and the gelatinous solute was microwaved (20 s) and allowed to cool. To prepare the experimental diets, particles spiked with each of the three model compounds were mixed in equal parts by weight, and added into food preparation. Particles were added to the liquid gelatin and were thoroughly mixed to obtain a homogenous particle-gelatin mixture, prior adding it to red mosquito larvae homogenate. For preparation of the chemical mixture feed, model chemicals (EE2, CPS, and BαP) were dissolved in solvents: MeOH (EE2 and CPS) and DMSO (BαP). Solvents were allowed to evaporate prior mixing with melted gelatinous solute, which then was added into red mosquito larvae mixture. Estimated daily intake of each model compound was 0.5 μg/fish/day. Thereafter, the prepared experimental feed mixtures were loaded into sterile syringes (10 mL; Plastipak, Becton Dickinson, ESP), which were refrigerated at 4°C until the mass reached jelly consistency. Feeds were individually shaped and portioned into worm-shaped pellets (Ø 2 mm), frozen on dry ice in foil packages in individual portions, and stored at −20°C until use. With this food preparation methodology we mimic dietary exposure via contaminated prey. Each portion constituted the daily food intake in a single exposure aquarium (N = 8 fish; 6% fish body weight). Selected exposure levels of PS and PE MPs, as well as concentrations of model HOCs, were not intended to represent exposure scenarios that fish are likely to encounter in the natural environment. Chemical analysis was additionally performed to confirm the presence of model HOCs in experimental fish feed.
Sampling
After the exposure, fish from each treatment were sacrificed, weighed and measured. No physical changes were observed on fish during the feeding experiment. The intestinal tracts, livers and brains were immediately dissected and preserved frozen in liquid nitrogen until use in biomarker analysis (N = 6). Ingested particles were observed in the dissected gut at the final sampling time (also confirming the ingestion of particles during the experiment). The remaining carcasses was stored in – 80°C until chemical analysis.
Biomarker Approach
Gene Expression Using Quantitative Real-Time PCR (qPCR)
Gene expression of CYP1a, ERα, VTG, and AChE were analyzed in liver and intestines using quantitative real-time PCR (qPCR) (Supplementary Table S3). RNA extraction was performed using the RNeasy® Plus Mini kit (Qiagen, Hilden, DE) according to the manufacturer’s instructions. Liver and gut samples (10 mg) were taken directly from liquid nitrogen and added to RLT buffer plus β-mercaptoethanol. Samples were homogenized at 25 HZ for 2 × 3 min using the TissueLyser II (Qiagen, Hilden, DE). Quality and quantity of extracted RNA was measured with a Nanodrop 2000 Spectrophotometer (Thermo scientific, United States) and automatic electrophoresis RNA ScreenTape System 2200 (Agilent Technologies, Inc., Waldbronn, DE). Synthesis of cDNA was performed using an iScript cDNA synthesis kit (Bio-Rad Laboratories, Inc.), using 1000 ng of mRNA. The following thermal protocol for the synthesis was used: (1) 5 min at 25°C, (2) 30 min at 42°C, and (3) 5 min at 85°C. Each qPCR reaction was performed with a final reaction volume of 5 ng/μL containing 20 ng cDNA. The final primer concentrations for CYP1a and ERα were 500 nM, 700 nM for VTG and 300 nM for AChE. The genes used as references for normalization was RPL13 and HPRT (intestinal tissue), and HPRT and β-tubulin (hepatic tissue).
Enzymatic Assays
Ethoxyresorufin-O-deethylase (EROD) fluorometric assay was used to measure the enzymatic activity of cytochrome P450 1A (CYP1a) in the S9 of the liver tissue, according to the established procedure (Förlin et al., 1984). Acetylcholine esterase (AChE) activity was measured in fish brain using an established methodology (Ellman et al., 1961). Enzymatic measurements were normalized with protein content, assessed with the Lowry method, using bovine serum albumin (BSA) as the protein standard (Lowry et al., 1951).
Quantification of Model Compounds in Fish Muscle
To quantify the chemicals in the fish muscle, samples from individual fish (N = 6; 1.06 ± 0.28 g) were homogenized in polypropylene tubes followed by the addition of an internal standard mixture (ethinylestradiol-d4, carbamazepine-(carboxamide-13C,15N) and benzo[a]pyrene-d12, 100 ng of respective compound). Chemicals were extracted twice with 6 mL of ACN, followed by spinning for 1 min, ultra-sonication for 20 min and centrifugation at 2500 × g for 5 min. Thereafter, the supernatants were transferred to a new tube. A third extraction was performed by the addition of 6 mL methyl tert-butyl ether (MTBE), spinning for 1 min, ultra-sonication for 20 min and centrifugation at 2500 × g for 5 min. The supernatants were combined and concentrated with N2 and gentle heating to a volume of 6 mL. To remove the lipids, a dispersive solid phase extraction kit from Agilent (QuEChERS Enhanced Matrix Removal – Lipid) was used and each tube was spun for 1 min and then centrifuged at 2500 × g for 5 min. The supernatant was collected and concentrated with N2 and gentle heat to a final volume of 1 mL ACN. The extracts were left overnight at -20°C and filtrated (with polypropylene micro-filters) before analysis. To quantify transfer of the model compounds CPS and EE2 into fish muscle, LC/MS-MS in multiple ion monitoring (MRM) mode was used following the methodology described in section “Chemical Spiking and Chemical Analysis.” For BαP quantitative analysis was carried out using a GC/MS-MS Agilent 7000 (Agilent Technologies, Santa Clara, California, United States) in Electron Impact mode. The GC/MS column used was a DB5-MS 30 m + 10 m guard column. The GC/MS was operated with electron energy of 70 eV and the ion source was set to 230°C. Both quadrupoles were set to 150°C and a pulsed splitless injection was used. The acquisition and processing of data were performed using MassHunter (version B.08.00, 2016). BαP levels were below detection limit, thus were not considered further in the analysis. To estimate transfer of model HOCs (EE2 and CPS) from particles (PSmix, PEmix, SGmix) into the fish, the following equation was used (Eq. 3):
Due to the small size of this fish species (and limited organ weight), we performed chemical analyses in fish muscle, and used other target organs in biomarker analyses.
Statistical Data Analysis
Prior to statistical analysis, data were tested for the normality (Shapiro-Wilk test) and for the homogeneity of variance (Levene’s test). Data that did not comply with these assumptions was log-transformed. Data from replicate tanks were pooled for subsequent analyses, as no tank-specific effects were observed (T-tests). One way analysis of variance (ANOVA) was used to compare biomarker responses across exposure groups and Dunnett’s post hoc test was used to assess differences between the control and exposure treatments, and Bonferroni post hoc test was used to obtain multi-comparisons between all the groups. A factorial analysis using a two-way ANOVA was used to assess particle-specific effects, and test whether particles (PS, PE, and SG) induced different effects than their chemically spiked counterparts (PSmix, PEmix, and SGmix) (Supplementary Table S4). Also, a principal component analysis (PCA) was performed to visualize particle-chemical interactions (Supplementary Figure S1). For analyses, SPSS Statistics (v.25, IBM Corporation, United States, 2017) and open-access software R (v. 3.3.2) and R studio (v. 1.1463©, 2009–2018 RStudio, Inc.).
Results
Sorption of HOCs Onto Microparticles
The sorption efficacy of each model HOC on three types of microparticles (PS, PE, and SG) was primarily estimated using mass-based units (Figure 2A), which are commonly used in MP spiking studies (Hüffer and Hofmann, 2016; Batel et al., 2018). Additionally, surface area and particle volume estimations were performed (Figures 2B,C). Mass-based results showed that synthetic plastic polymer particles (PS and PE) absorbed more of CPS and BαP compared to inorganic SG (Figure 2A). In regards to chemical sorption of HOCs on different types of synthetic polymers, we found that the glassy PS particles sorbed more chemicals than rubbery PE particles per mass unit (Figure 2A). The similar pattern was observed when sorption was normalized to particle surface area (Figure 2B), but not to particle volume (Figure 2C). No clear relationship was observed between the chemical sorption and compound’s hydrophobicity (Figures 2A–C). On contrary to what was expected, the sorption of EE2, which had the lowest degree of hydrophobicity, in many instances, exceeded that of CPS and BαP.
Chemical Transfer of HOCs Into Fish
Biomarker Responses
Changes in the expression of target genes in fish intestine (mRNA of CYP1a, ER, VTG and AChE) were observed (Figure 3A and Supplementary Figure S1), suggesting the chemical release of model HOCs from test particles and subsequent activation of intestinal metabolism. The upregulation of CYP1a expression in the intestinal tissue (p < 0.05) suggested release of aryl hydrocarbon receptor (AhR) agonist, i.e., BαP, from all spiked particles. The observed increase of ER and VTG mRNA levels (p < 0.05 and p > 0.05, respectively) suggested desorption and uptake of EE2 into intestinal lumen and tissue, whereas marginally elevated AChE mRNA levels (p > 0.05) indicated potential dissociation of CPS.
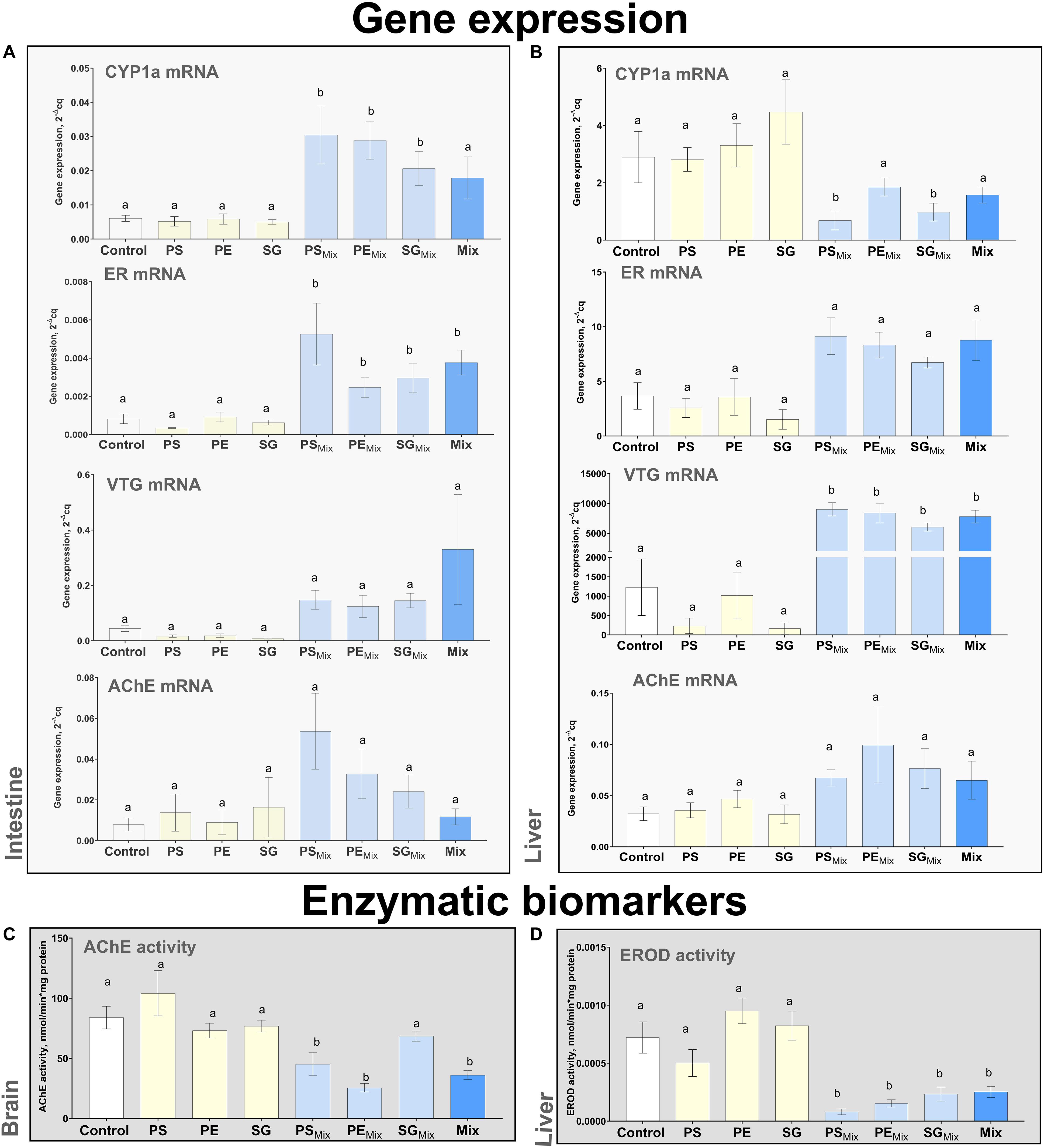
Figure 3. Biomarker responses: gene expression (A,B) and enzymatic activities (C,D) in different organs: intestine (A), liver (B,D) and brain (C). Data expressed as means ± SE, N = 6. Different letters indicate statistical differences between the control and exposure treatments (one-way ANOVA, post hoc Dunnett test, p < 0.05).
Similarly, changes in hepatic gene biomarker responses indicated uptake of EE2 and CPS in fish liver (Figures 3B,D). An increase in gene expression of ER and VTG (p > 0.05 and p < 0.05, respectively) were observed in fish from PSmix, PEmix, and SGmix treatments. A trend towards upregulation of AChE was observed, but these changes were not statistically significant (p > 0.05). Cytochrome P450 metabolism was not induced in the liver, as indicated in the down-regulation of CYP1a mRNA (p < 0.05 for PSmix, SGmix, and p > 0.05 for PEmix) and suppression of EROD activity was observed for all treatments containing BαP (p < 0.05) (Figures 3B,D).
Regarding chemical transfer in brain, AChE inhibition has been indicated, suggesting transfer of the CPS - AChE inhibitor, into this region (Figure 3C, p < 0.05 for PSmix, PEmix, p > 0.05 for SGmix).
Chemical Quantification in Fish Muscle
Quantification of chemical burden in fish muscle revealed that two of model compounds (EE2 and CPS; log Kow = 3.87 and 4.66, respectively) were taken up and further systematically distributed in an organism (Table 1), confirming the accumulation of model HOCs beyond the gastrointestinal tract. It was not possible to detect BαP in the muscle in all treatments containing this chemical. Overall, results indicated that microparticle-mediated transfer of chemicals with low to moderate hydrophobicity (EE2 and CPS) is quite low (Table 1). When the accumulation potential for HOCs was compared across different particle types, it can be seen that synthetic MPs (PS and PE) mediated greater chemical transfer into the fish than SG.
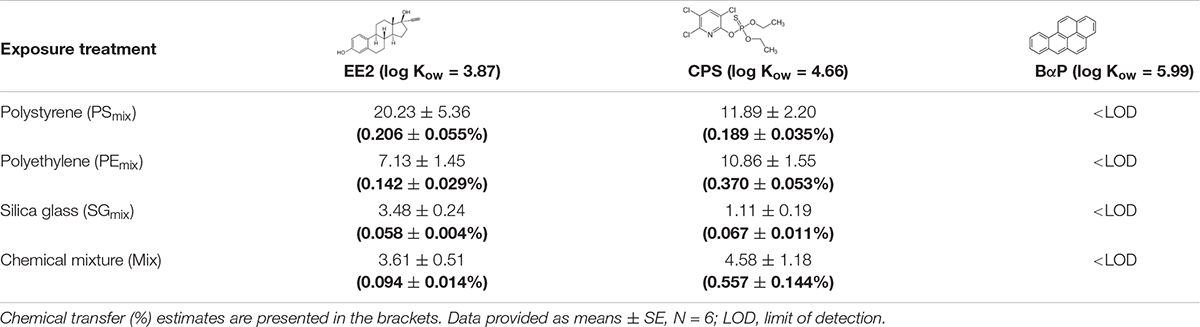
Table 1. Accumulation of model HOCs compounds in fish muscle, expressed by average amount chemical (ng) per g fish in the end of the experiment ± SE.
Discussion
To address the ongoing discussions concerning the propensity and relevance of MPs to act as vectors for environmental contaminants, we examined the sorption of three common hydrophobic environmental pollutants (EE2, CPS, BαP) to two types of synthetic MPs and one type of naturally occurring particles, and followed their subsequent transfer into the fish upon ingestion.
Sorption of HOCs Onto Microparticles
In the first part of the study, we performed artificial chemical spiking with selected HOCs and investigated the capacity of test chemicals to sorb onto test microparticles (PS, PE, and SG). The findings suggested that synthetic particles (PS, PE) sorb more of two of the model compounds: CPS and BαP than SG (Figure 2A). Herein, we used silica glass particles as a proxy for naturally occurring particles, a comparison which is crucial but often overlooked in experimental MP studies (Ogonowski et al., 2017). When comparing chemical sorption of HOCs on natural occurring inorganic particulates, it has been observed that sorption onto MPs exceeds sorption to natural sediments (Teuten et al., 2007). Our findings agree with such observation (with an exception with EE2, Figures 2A,B). While naturally occurring organic particulates have been demonstrated as having higher affinity to HOCs than synthetic particles (Ghosh et al., 2000; Beckingham and Ghosh, 2017), the sorption to natural inorganic (non-porous) solids is generally smaller, and likely limited to chemical adsorption onto the surface. While our results suggested that PS and PE MPs were prone to higher sorption of two test HOCs (CPS and BαP) than SG particles, we can argue that sorption of HOC on microparticles depends on complex interplay between the properties of sorbate and sorbent, as well as on general laboratory spiking conditions (e.g., duration, availability of sorbate, solvent choice, etc.). In this study it was difficult to elucidate a clear relationship between chemical sorption and hydrophobicity of HOCs. It is generally accepted that sorption affinity to sorbent increases with increasing hydrophobicity of the compound (Müller et al., 2001; Smedes et al., 2009). The highest mass-based sorption onto all types of particles was observed for EE2, which had the lowest log Kow among the selected model compounds. Chemicals with lower hydrophobicity are known to reach chemical equilibrium faster than more hydrophobic compounds (Endo et al., 2013), thus the observed trend may indirectly indicate faster sorption kinetics onto different particles during the artificial spiking procedure. Also, the lower sorption of more hydrophobic substance onto particles could be explained by the loss of compound during the spiking procedure (e.g., due to attachment to the vessel). On the other hand, EE2 have hydroxyl functional group that can interact with the surface of the substrate, thereby increasing or decreasing the sorption (Magner et al., 2009).
In regards to chemical sorption of HOCs on synthetic polymer particles, glassy PS revealed higher chemical association than rubbery PE (Figure 2A), which somewhat contradicted with aforementioned glassy-rubbery state theory. Glassy polymers possess dual sorption mechanisms: partitioning or dissolution of HOCs in the amorphous polymer regions and adsorption (hole-filling) into internal polymer nanovoids (Teuten et al., 2009). Although PS is considered as a glassy polymer, higher sorption capacity than predicted by its glass transition temperature has been previously reported (Alimi et al., 2018). The presence of the benzene ring in the polymeric backbone of PS monomer was suggested to distend the distance between adjacent polymer chains, facilitating diffusion into the polymer (Pascall et al., 2005; Velzeboer et al., 2014). While adsorption is the dominant sorption mechanism for PS MPs, the larger surface area of the PS MPs (Figure 1C) could have facilitated a faster interaction and greater sorption of HOCs compared to the PE MPs. Particle characterization revealed high numbers of the smallest size particles (<200 μm) in PS MPs (Figure 1D). Such fine particulate fractions are known as considerably more effective sorbents due to higher abundance of binding sites on larger surface area (Ngoc et al., 2010). On the other hand, when the sorption was normalized for particle volume, a property facilitating absorption, it could be seen that PE sorbed more HOCs compared to PS (Figure 2C). Structurally, glassy amorphous polymers (PS) are dense with little void spacing, whereas rubbery polymers (PE) can encapsulate larger volumes between the molecules allowing greater diffusion of contaminants into the polymer (Bakir et al., 2012; Hartmann et al., 2017; Alimi et al., 2018). Having larger (free) volume available for chemical absorption, PE may be viewed as a more prominent sorbent for HOCs than PS. However, it is important to note that for smaller polymer particles having large surface-to-volume ratio, adsorption of HOCs onto surface may be more important mechanism than absorption into the polymer. It is also important to consider that the methodology used here, with a relatively short sorption time, might favor surface adsorption (as opposed diffusion into the polymer phase), in a potentially non-equilibrium situation (Koelmans et al., 2016; Mohamed Nor and Koelmans, 2019). Importantly, in this experiment particles were neither weathered nor exposed to biofilms, which are, of course, an important factors that can affect the binding affinity to chemicals under environmentally realistic conditions (Velez et al., 2018). Also, herein, artificial spiking was used to load particles for feeding experiment, and did not intend to describe sorption kinetics, which notably could be very valuable for better understanding HOCs and microparticle interactions. While there are no established guidelines for MPs spiking experiments in vector studies, the characterization and presentation of test materials remain of high importance for results’ interpretation. It is important to consider not only the mass, but also particle morphology (size, shape, surface area), and/or other properties that relate to sorptive capacity of the materials (crystallinity, surface-to-volume ratio, surface polarity).
Chemical Transfer Into Fish
The second part of the study addressed the chemical transfer from spiked microparticles into the fish following ingestion. By measuring the changes in metabolic pathways, specific to the model compounds, we could infer the release of particle-associated HOCs into fish intestine and subsequent chemical distribution to peripheral organs. It is known that intestinal surfactants can enhance desorption of hydrophobic compounds from MPs (Bakir et al., 2014), liberating and making them bioavailable for metabolism, and/or for intestinal absorption. While we could document uptake and transfer of EE2 and CPS, the transfer of BαP into fish was not detected. This could be explained that BαP released from particles may not have been transferred across intestine and was largely contained (and/or metabolized) in the intestine or/and rapid metabolism of the compound took place in the hepatic tissue (Table 1 and Figure 3). While number of studies suggest rapid transformation of BαP in liver (thus limited transfer of the mother substance in to the muscle) (Lemaire et al., 1990; Van Veld et al., 1997; Oris et al., 2004), some studies indicate metabolism and storage of BαP metabolites in intestinal epithelia. For instance, it has been shown that BαP administrated via dietary exposures associate with lipids, and its hydrolysis products can be found absorbed by enterocytes, and can be stored in the lipid droplets (Vetter et al., 1985). Due to extensive biotransformation, or storage, occurring in the gut, dietary exposure to BαP can increase CYP1a in intestinal mucosal epithelia and vasculature (Van Veld et al., 1997). The levels of active compounds, such as BαP can subsequently be reduced, thus limiting its translocation to other tissues. Some studies suggest that the efficiency of gastrointestinal absorption of hydrophobic chemicals (log Kow > 5.5) is thought to decrease (Gouin et al., 2011). The uptake of lipophilic compounds (log Kow > 6.3) following dietary intake is considerably less efficient and is limited due to slow diffusion in unstirred water layer adjacent to brush border membrane of enterocytes (Kelly et al., 2004). It has been predicted that the bioaccumulation of HOCs, associated with MPs, having log Kow higher than 5 could lead to the reduction of body burden concentrations, also known as the “cleaning” effect (Gouin et al., 2011). The desorption of HOCs, chemical uptake and potential organismal distribution can be influenced by a number of factors, such as chemical loading, hydrophobicity of ab/ad-sorbed chemicals, intrinsic particle characteristics (surface area, porosity, etc.), desorption kinetics and physiological conditions and metabolism in the intestine (Teuten et al., 2009; Bakir et al., 2014; Wardrop et al., 2016; Sleight et al., 2017). In addition to this, the importance of gut retention time is becoming increasingly recognized in studies investigating MP-mediated chemical transfer (Mohamed Nor and Koelmans, 2019). In stickleback, due to short retention time (<48 h) of MPs in the gastrointestinal tract (Bour et al., 2020), the desorption of HOCs from ingested plastics could be rate-limited (Mohamed Nor and Koelmans, 2019), limiting the uptake of particle-bound chemicals into the fish. The metabolization of a compound could also play an important role in mediating not only the influx of HOCs from ingested plastic into organism, but also its bioaccumulation potential (Diepens and Koelmans, 2018). For example, metabolization of PAHs is thought to diminish fugacity in the lipids, leading to attenuated transfer into an organism (Diepens and Koelmans, 2018). The metabolic capacity of various HOCs in fish intestine is not well known, and generally is excluded from consideration in MP-vector studies, but can be crucial for chemical release kinetics and organismal distribution. While the results, regarding BαP transfer, remain inconclusive, EE2 and CPS-based results suggest that gastrointestinal tract is an important physiological compartment, where the chemical desorption and metabolism of particle-bound chemicals take place, and that microparticles act as carriers for certain HOCs into intestine and further into an organism.
Application of Biomarker Approach in Vector Studies
It is known that estrogenic compounds can interfere with AhR signaling and can suppress PAH-mediated CYP1a mRNA transcription and EROD activity (Förlin et al., 1984; Arukwe et al., 1997; Navas and Segner, 2001). As our results suggested, EE2 was taken up and reached hepatic tissue (Figure 3B and Table 1), however, an inhibitory effect on AhR signaling was suspected, as transcript levels of CYP1a enzyme and CYP1a catalytic activity in liver were significantly suppressed (Figures 3B,D). The above-mentioned example pointed towards the potential interference between model HOCs in biomarker responses. Effect-based biomarker approaches provide estimations of biologically active, or bioavailable fractions of the compound, but may be hindered by the specificity (or lack thereof) of the selected biological response. With that, we argue that solely effect-based approaches for vector studies may be insufficient to understand the causality and interactions between compounds, as chemicals can interact with different receptors, and metabolism can occur via the same pathways. While the biomarker approach was a valuable tool to determine bioavailability of desorbed model HOCs on enzyme and gene expression level, however, it also revealed its potential limitations in the context of mixture toxicology. BαP is commonly used in MPs vector studies (Batel et al., 2016; Donovan et al., 2018; Pittura et al., 2018; González-soto et al., 2019; Wang et al., 2019); however, herein it revealed some experimental implications for making conclusions regarding particle-mediated chemical transfer of this chemical into the fish. Therefore, it is important to address that toxicokinetic interactions between contaminants can occur, and potentially could impede the assessment not only of chemical transfer, but also of biological effects. As chemical sorption, desorption and subsequent biological uptake and distribution of chemicals in vivo depends on multiple interconnected factors including physiocochemical properties of particles and contaminants, and toxicokinetic and toxicodynamics, and these variables need to be carefully considered while designing vector studies with animals.
Comparative Importance of Chemical Transfer Mediated via Natural and Synthetic Particles
As results suggested, chemical transfer of moderately hydrophobic metabolizable chemicals of both glassy PS and rubbery PE MPs, as well as natural SG microparticles is likely, however, was low. SG are non-polymer mineral particles that generally very abundant, yet have low levels of sorption, thus it is not surprising that low-level chemical transfer was observed for these particles. However, given their wide occurrence, inorganic particles may also serve as an exposure pathway of these chemicals into biota, and this could be relevant for species foraging in benthic habitats. The relative importance of microparticle-mediated chemical exposure remains the subject of continued discussions and calls for more experimental studies to better understand particle-chemical interactions, especially in comparison to other solid particulates present in the environment. Whether MPs are of considerable importance for transfer of HOCs compared to other sources, e.g., prey, organic matter other colloidal particulates, or uptake directly from the water through the gills is still a matter of a debate (Bakir et al., 2012; Koelmans et al., 2016, 2015). We suggest that the low level chemical transfer demonstrated in this study, and low MPs encounter rates in the natural environment to date, should render MPs an insignificant exposure pathway for HOCs into fish.
Conclusion
This study explored the capacity of MPs and silica microparticles to sorb and transfer chemicals into fish and provided experimental insights and considerations for vector studies using fish. Results suggested that microparticle-mediated chemical transfer of moderately hydrophobic contaminants into fish is likely. The synthetic particles demonstrated higher chemical sorption (of CPS and BαP) than silica particles. PS and PE MPs mediated higher chemical transfer and accumulation of model HOCs (EE2 and CPS) into fish muscle compared to silica particles, but the overall dietary transfer was low. Considering the sheer abundance of these inorganic particles in the natural environment, compared to the relatively low levels of MPs, could still indicate that the amount of chemicals sorbed to inorganic particles would greatly exceed the amount of chemicals bound to MPs (Koelmans et al., 2016), reducing the relative importance of MPs as vectors under natural environmental conditions. We also concluded that while the biomarker approach was valuable tool to determine biological availability of contaminants in fish, however, it was determined to be suboptimal for assessing biological effects and chemical transfer when addressing chemical mixtures associated with microparticles.
Data Availability Statement
The raw data supporting the conclusions of this article will be made available by the authors, without undue reservation.
Ethics Statement
This animal study was reviewed and approved by the Swedish Board of Agriculture (Ethical permit number: 220–2013).
Author Contributions
BC, TB, JM, and EW designed the study. EW performed the artificial particle spiking, as well as chemical analysis (particles and fish tissue samples). MT and GA prepared the experimental diets. MT and BC carried out the feeding experiment. MT performed the biomarker measurements. GA performed the particle characterization, assisted in biomarker analysis, analyzed the data, and took the lead in writing the manuscript. All authors provided critical feedback and helped to shape the research, analysis, and manuscript.
Funding
This research was supported by Swedish Research Council FORMAS (project NICE; 2011-01733) and (2012-00625).
Conflict of Interest
The authors declare that the research was conducted in the absence of any commercial or financial relationships that could be construed as a potential conflict of interest.
Acknowledgments
We acknowledge the Centre for Cellular Imaging at the University of Gothenburg and the National Microscopy Infrastructure, NMI (VR-RFI 2016-00968) for providing assistance in microscopy. Also, authors are grateful for Christos Oikonomou, Uddeholm AB for opportunity to perform CAMSIZER measurements, and Georgios Giovanoulis at Swedish Environmental Research Institute for chemical analysis of the fish.
Supplementary Material
The Supplementary Material for this article can be found online at: https://www.frontiersin.org/articles/10.3389/fenvs.2020.00087/full#supplementary-material
References
Alimba, C. G., and Faggio, C. (2019). Microplastics in the marine environment: current trends in environmental pollution and mechanisms of toxicological profile. Environ. Toxicol. Pharmacol. 68, 61–74. doi: 10.1016/j.etap.2019.03.001
Alimi, O. S., Farner Budarz, J., Hernandez, L. M., and Tufenkji, N. (2018). Microplastics and nanoplastics in aquatic environments: aggregation, deposition, and enhanced contaminant transport. Environ. Sci. Technol. 52, 1704–1724. doi: 10.1021/acs.est.7b05559
Arukwe, A., Förlin, L., and Goksøyr, A. (1997). Xenobiotic and steroid biotransformation enzymes in atlantic salmon (Salmo salar) liver treated with an estrogenic compound, 4-nonylphenol. Environ. Toxicol. Chem. 16:2576. doi: 10.1897/1551-5028(1997)016<2576:xasbei>2.3.co;2
Ašmonaite, G., Sundh, H., Asker, N., and Carney Almroth, B. (2018). Rainbow trout maintain intestinal transport and barrier functions following exposure to polystyrene microplastics. Environ. Sci. Technol. 52, 14392–14401. doi: 10.1021/acs.est.8b04848
Bakir, A., Rowland, S. J., and Thompson, R. C. (2012). Competitive sorption of persistent organic pollutants onto microplastics in the marine environment. Mar. Pollut. Bull. 64, 2782–2789. doi: 10.1016/j.marpolbul.2012.09.010
Bakir, A., Rowland, S. J., and Thompson, R. C. (2014). Enhanced desorption of persistent organic pollutants from microplastics under simulated physiological conditions. Environ. Pollut. 185, 16–23. doi: 10.1016/j.envpol.2013.10.007
Batel, A., Borchert, F., Reinwald, H., Erdinger, L., and Braunbeck, T. (2018). Microplastic accumulation patterns and transfer of benzo[a]pyrene to adult zebrafish (Danio rerio) gills and zebrafish embryos. Environ. Pollut. 235, 918–930. doi: 10.1016/j.envpol.2018.01.028
Batel, A., Linti, F., Scherer, M., Erdinger, L., and Braunbeck, T. (2016). Transfer of benzo[a]pyrene from microplastics to Artemia nauplii and further to zebrafish via a trophic food web experiment: CYP1A induction and visual tracking of persistent organic pollutants. Environ. Toxicol. Chem. 35, 1656–1666. doi: 10.1002/etc.3361
Beckingham, B., and Ghosh, U. (2017). Differential bioavailability of polychlorinated biphenyls associated with environmental particles: microplastic in comparison to wood, coal and biochar. Environ. Pollut. 220, 150–158. doi: 10.1016/j.envpol.2016.09.033
Bour, A., Hossain, S., Taylor, M., Sumner, M., and Carney Almroth, B. (2020). Synthetic microfiber and microbead exposure and retention time in model aquatic species, under different exposure scenarios. Front. Environ. Sci. doi: 10.3389/fenvs.2020.00083
Burns, E. E., and Boxall, A. B. A. (2018). Microplastics in the aquatic environment: evidence for or against adverse impacts and major knowledge gaps. Environ. Toxicol. Chem. 37, 2776–2796. doi: 10.1002/etc.4268
Diepens, N. J., and Koelmans, A. A. (2018). Accumulation of plastic debris and associated contaminants in aquatic food webs. Environ. Sci. Technol. 52, 8510–8520. doi: 10.1021/acs.est.8b02515
Ding, J., Zhang, S., Mamitiana, R., and Zou, H. (2018). Accumulation, tissue distribution and biochemical effects of polystyrene microplastics in the freshwater fish red tilapia (Oreochromis niloticus). Environ. Pollut. 238, 1–9. doi: 10.1016/j.envpol.2018.03.001
Donovan, S. O., Mestre, N. C., Abel, S., Fonseca, T. G., Carteny, C. C., Cormier, B., et al. (2018). Ecotoxicological effects of chemical contaminants adsorbed to microplastics in the clam Scrobicularia plana. Front. Mar. Sci. 5:143. doi: 10.3389/fmars.2018.00143
Ellman, G. L., Courtney, K. D., Andres, V., and Featherstone, R. M. (1961). A new and rapid colorimetric determination of acetylcholinesterase activity. Biochem. Pharmacol. 7, 88–95. doi: 10.1016/0006-2952(61)90145-9
Endo, S., Yuyama, M., and Takada, H. (2013). Desorption kinetics of hydrophobic organic contaminants from marine plastic pellets. Mar. Pollut. Bull. 74, 125–131. doi: 10.1016/j.marpolbul.2013.07.018
Everaert, G., Van Cauwenberghe, L., De Rijcke, M., Koelmans, A. A., Mees, J., Vandegehuchte, M., et al. (2018). Risk assessment of microplastics in the ocean: modelling approach and first conclusions. Environ. Pollut. 242, 1930–1938. doi: 10.1016/j.envpol.2018.07.069
Förlin, L., Andersson, T., Koivusaari, U., and Hansson, T. (1984). Influence of biological and environmental factors on hepatic steroid and xenobiotic metabolism in fish: interaction with PCB and β-naphthoflavone. Mar. Environ. Res. 14, 47–58. doi: 10.1016/0141-1136(84)90069-2
Ghosh, U., Gillette, J. S., Luthy, R. G., and Zare, R. N. (2000). Microscale location, characterization and association of polycyclic aromatic hydrocarbons on harbor sediment particles. Environ. Sci. Technol. 34, 1729–1736. doi: 10.1021/es991032t
González-soto, N., Hat, J., Katsumiti, A., Duroudier, N., María, J., Bilbao, E., et al. (2019). Impacts of dietary exposure to different sized polystyrene microplastics alone and with sorbed benzo [a] pyrene on biomarkers and whole organism responses in mussels Mytilus galloprovincialis. Sci. Total Environ. 684, 548–566. doi: 10.1016/j.scitotenv.2019.05.161
Gouin, T., Roche, N., Lohmann, R., and Hodges, G. (2011). A thermodynamic approach for assessing the environmental exposure of chemicals absorbed to microplastic. Environ. Sci. Technol. 45, 1466–1472. doi: 10.1021/es1032025
Guzzetti, E., Sureda, A., Tejada, S., and Faggio, C. (2018). Microplastic in marine organism: environmental and toxicological effects. Environ. Toxicol. Pharmacol. 64, 164–171. doi: 10.1016/j.etap.2018.10.009
Hartmann, N. B., Hüffer, T., Thompson, R. C., Hassellöv, M., Verschoor, A., Daugaard, A. E., et al. (2019). Are we speaking the same language? Recommendations for a definition and categorization framework for plastic debris. Environ. Sci. Technol. 53, 1039–1047. doi: 10.1021/acs.est.8b05297
Hartmann, N. B., Rist, S., Bodin, J., Jensen, L. H. S., Schmidt, S. N., Mayer, P., et al. (2017). Microplastics as vectors for environmental contaminants: exploring sorption, desorption, and transfer to biota. Integr. Environ. Assess. Manag. 13, 488–493. doi: 10.1002/ieam.1904
Hirai, H., Takada, H., Ogata, Y., Yamashita, R., Mizukawa, K., Saha, M., et al. (2011). Organic micropollutants in marine plastics debris from the open ocean and remote and urban beaches. Mar. Pollut. Bull. 62, 1683–1692. doi: 10.1016/j.marpolbul.2011.06.004
Hüffer, T., and Hofmann, T. (2016). Sorption of non-polar organic compounds by micro-sized plastic particles in aqueous solution. Environ. Pollut. 214, 194–201. doi: 10.1016/j.envpol.2016.04.018
Jambeck, J. R., Geyer, R., Wilcox, C., Siegler, T. R., Perryman, M., Andrady, A., et al. (2015). Plastic waste inputs from land into the ocean. Science 347, 768–771. doi: 10.1126/science.1260352
Katsiadaki, I., Morris, S., Squires, C., Hurst, M. R., James, J. D., and Scott, A. P. (2006). Three-spined stickleback (Gasterosteus aculeatus) as a sensitive in vivo test for detection of environmental antiandrogens. Environ. Health Perspect. 114, 115–121. doi: 10.1289/ehp.8063
Kelly, B. C., Gobas, F. A. P. C., and McLachlan, M. S. (2004). Intestinal absorption and biomagnification of organic contaminants in fish, wildlife, and humans. Environ. Toxicol. Chem. 23, 2324–2336. doi: 10.1897/03-545
Koelmans, A. A., Bakir, A., Burton, G. A., and Janssen, C. R. (2016). Microplastic as a vector for chemicals in the aquatic environment: critical review and model-supported reinterpretation of empirical studies. Environ. Sci. Technol. 50, 3315–3326. doi: 10.1021/acs.est.5b06069
Koelmans, A. A., Besseling, E., and Shim, W. J. (2015). “Nanoplastics in the aquatic environment,” in Marine Anthropogenic Litter, eds M. Bergmann, L. Gutow, and M. Klages (Cham: Springer), 325–340. doi: 10.1007/978-3-319-16510-3_12
Koelmans, A. A., Besseling, E., Wegner, A., and Foekema, E. M. (2013). Plastic as a carrier of POPs to aquatic organisms: a model analysis. Environ. Sci. Technol. 47, 7812–7820. doi: 10.1021/es401169n
Lee, H., Shim, W. J., and Kwon, J. H. (2014). Sorption capacity of plastic debris for hydrophobic organic chemicals. Sci. Total Environ. 47, 1545–1552. doi: 10.1016/j.scitotenv.2013.08.023
Lemaire, P., Mathieu, A., Carriere, S., and Drai, P. (1990). The uptake metabolism and biological half-life of benzo[a] pyrene in different tissues of sea bass, Dicentrarchus labrax. Ecotoxicol. Environ. Saf. 20, 223–233. doi: 10.1016/0147-6513(90)90001-l
Lowry, O. H., Rosebrough, N. J., Farr, A. L., and Randall, R. J. (1951). Protein measurement with Folin Phenol reagent. J. Biol. Chem. 193, 265–275. doi: 10.1016/0304-3894(92)87011-4
Lu, Y., Zhang, Y., Deng, Y., Jiang, W., Zhao, Y., Geng, J., et al. (2016). Uptake and accumulation of polystyrene microplastics in zebrafish (Danio rerio) and toxic effects in liver. Environ. Sci. Technol. 50, 4054–4060. doi: 10.1021/acs.est.6b00183
Magner, J. A., Alsberg, T. E., and Broman, D. (2009). Evaluation of Poly (Ethylene-Co-Vinyl Acetate-Co-Carbon Monoxide) and polydimethylsiloxane for equilibrium sampling of polar organic contaminants in water. Environ. Toxicol. Chem. 28, 1874–1880.
Mato, Y., Isobe, T., Takada, H., Kanehiro, H., Ohtake, C., and Kaminuma, T. (2001). Plastic resin pellets as a transport medium for toxic chemicals in the marine environment. Environ. Sci. Technol. 35, 318–324. doi: 10.1021/es0010498
Mohamed Nor, N. H., and Koelmans, A. A. (2019). Transfer of PCBs from microplastics under simulated gut fluid conditions is biphasic and reversible. Environ. Sci. Technol. 53, 1874–1883. doi: 10.1021/acs.est.8b05143
Müller, A., Becker, R., Dorgerloh, U., Simon, F. G., and Braun, U. (2018). The effect of polymer aging on the uptake of fuel aromatics and ethers by microplastics. Environ. Pollut. 240, 639–646. doi: 10.1016/j.envpol.2018.04.127
Müller, J. F., Manomanii, K., Mortimer, M. R., and McLachlan, M. S. (2001). Partitioning of polycyclic aromatic hydrocarbons in the polyethylene/water system. Anal. Bioanal. Chem. 371, 816–822. doi: 10.1007/s002160101025
Navas, J. M., and Segner, H. (2001). Estrogen-mediated suppression of cytochrome P4501A (CYP1A) expression in rainbow trout hepatocytes: role of estrogen receptor. Chem. Biol. Interact. 138, 285–298. doi: 10.1016/S0009-2797(01)00280-0
Ngoc, C., Jin, D., Ra, S., and Schlenk, D. (2010). Sorption of estrogens onto different fractions of sediment and its effect on vitellogenin expression in Male Japanese medaka. Arch. Environ. Contam. Toxicol. 59, 147–156. doi: 10.1007/s00244-009-9429-1
Ogonowski, M., Gerdes, Z., and Gorokhova, E. (2017). What we know and what we think we know about microplastic effects – a critical perspective. Curr. Opin. Environ. Sci. Health 1, 41–46. doi: 10.1016/j.coesh.2017.09.001
Oris, D. W. T. A. U., Hen, P. I. N. G. C., and Ollino, C. A. A. P. (2004). Cytological changes in association with ethoxyresorufin-O-deethylase induction in fish upon dietary exposure to benzo[a]pyrene. Environ. Toxicol. Chem. 23, 1043–1050.
Pascall, M. A., Zabik, M. E., Zabik, M. J., and Hernandez, R. J. (2005). Uptake of polychlorinated biphenyls (PCBs) from an aqueous medium by polyethylene, polyvinyl chloride, and polystyrene films. J. Agric. Food Chem. 53, 164–169. doi: 10.1021/jf048978t
Pittura, L., Avio, C. G., Giuliani, M. E., Errico, G., and Keiter, S. H. (2018). Microplastics as vehicles of environmental PAHs to marine organisms?: combined chemical and physical hazards to the mediterranean mussels, Mytilus galloprovincialis. Front. Mar. Sci. 5:103. doi: 10.3389/fmars.2018.00103
Prokic, M. D., Gavri, J. P., and Faggio, C. (2019). Ecotoxicological effects of microplastics?: examination of biomarkers, current state and future perspectives. Trends Anal. Chem. 111, 37–46. doi: 10.1016/j.trac.2018.12.001
Rochman, C. M., Hoh, E., Hentschel, B. T., and Kaye, S. (2013a). Long-term field measurement of sorption of organic contaminants to five types of plastic pellets: implications for plastic marine debris. Environ. Sci. Technol. 47, 1646–1654. doi: 10.1021/es303700s
Rochman, C. M., Hoh, E., Kurobe, T., and Teh, S. J. (2013b). Ingested plastic transfers hazardous chemicals to fish and induces hepatic stress. Sci. Rep. 3, 1–7. doi: 10.1038/srep03263
Skjolding, L. M., Ašmonaitë, G., Jølck, R. I., Andresen, T. L., Selck, H., Baun, A., et al. (2017). An assessment of the importance of exposure routes to the uptake and internal localisation of fluorescent nanoparticles in zebrafish (Danio rerio), using light sheet microscopy. Nanotoxicology 11, 351–359. doi: 10.1080/17435390.2017.1306128
Sleight, V. A., Bakir, A., Thompson, R. C., and Henry, T. B. (2017). Assessment of microplastic-sorbed contaminant bioavailability through analysis of biomarker gene expression in larval zebrafish. Mar. Pollut. Bull. 116, 291–297. doi: 10.1016/j.marpolbul.2016.12.055
Smedes, F., and Booij, K. (2012). Guidelines for passive sampling of hydrophobic contaminants in water using silicone rubber samplers. ICES Techn. Mar. Environ. Sci. 52, 1–20. doi: 10.25607/OBP-236
Smedes, F., Geertsma, R. W., Van Der Zande, T., and Booij, K. (2009). Polymer-water partition coefficients of hydrophobic compounds for passive sampling: application of cosolvent models for validation. Environ. Sci. Technol. 43, 7047–7054. doi: 10.1021/es9009376
Teuten, E. L., Rowland, S. J., Galloway, T. S., and Thompson, R. C. (2007). Potential for plastics to transport hydrophobic contaminants. Environ. Sci. Technol. 41, 7759–7764. doi: 10.1021/es071737s
Teuten, E. L., Saquing, J. M., Knappe, D. R. U., Barlaz, M. A., Jonsson, S., Björn, A., et al. (2009). Transport and release of chemicals from plastics to the environment and to wildlife. Philos. Trans. R. Soc. B 364, 2027–2045. doi: 10.1098/rstb.2008.0284
Van Veld, P. A., Vogelbein, W. K., Cochran, M. K., Goksøyr, A., and Stegeman, J. J. (1997). Route-specific cellular expression of cytochrome P4501A (CYP1A) in fish (Fundulus heteroclitus) following exposure to aqueous and dietary benzo[a]pyrene. Toxicol. Appl. Pharmacol. 142, 348–359. doi: 10.1006/taap.1996.8037
Velez, J. F. M., Shashoua, Y., Syberg, K., and Khan, F. R. (2018). Considerations on the use of equilibrium models for the characterisation of HOC-microplastic interactions in vector studies. Chemosphere 210, 359–365. doi: 10.1016/j.chemosphere.2018.07.020
Velzeboer, I., Kwadijk, C. J. A. F., and Koelmans, A. A. (2014). Strong sorption of PCBs to nanoplastics, microplastics, carbon nanotubes, and fullerenes. Environ. Sci. Technol. 48, 4869–4876. doi: 10.1021/es405721v
Vetter, R. D., Carey, M. C., and Patton, J. S. (1985). Coassimilation of dietary fat and benzo (a) pyrene in the small intestine: an absorption model using the killifish. J. Lipid Res. 26, 428–434.
Wang, J., Cof, S., Sun, C., Schlenk, D., and Gan, J. (2019). Negligible effects of microplastics on animal fi tness and HOC bioaccumulation in earthworm Eisenia fetida in soil. Environ. Pollut. 249, 776–784. doi: 10.1016/j.envpol.2019.03.102
Wang, W., and Wang, J. (2018). Different partition of polycyclic aromatic hydrocarbon on environmental particulates in freshwater: microplastics in comparison to natural sediment. Ecotoxicol. Environ. Saf. 147, 648–655. doi: 10.1016/j.ecoenv.2017.09.029
Wardrop, P., Shimeta, J., Nugegoda, D., Morrison, P. D., Miranda, A., Tang, M., et al. (2016). Chemical pollutants sorbed to ingested microbeads from personal care products accumulate in fish. Environ. Sci. Technol. 50, 4037–4044. doi: 10.1021/acs.est.5b06280
Keywords: microplastics, vector effects, hydrophobic organic chemicals, fish, benzo(α)pyrene, EE2, chlorpyrifos, three-spined stickleback (Gasterosteus aculeatus)
Citation: Ašmonaitė G, Tivefälth M, Westberg E, Magnér J, Backhaus T and Carney Almroth B (2020) Microplastics as a Vector for Exposure to Hydrophobic Organic Chemicals in Fish: A Comparison of Two Polymers and Silica Particles Spiked With Three Model Compounds. Front. Environ. Sci. 8:87. doi: 10.3389/fenvs.2020.00087
Received: 27 March 2020; Accepted: 28 May 2020;
Published: 03 July 2020.
Edited by:
Cristina Panti, University of Siena, ItalyReviewed by:
Caterina Faggio, University of Messina, ItalyMonica Michelle Arienzo, Desert Research Institute (DRI), United States
Copyright © 2020 Ašmonaitė, Tivefälth, Westberg, Magnér, Backhaus and Carney Almroth. This is an open-access article distributed under the terms of the Creative Commons Attribution License (CC BY). The use, distribution or reproduction in other forums is permitted, provided the original author(s) and the copyright owner(s) are credited and that the original publication in this journal is cited, in accordance with accepted academic practice. No use, distribution or reproduction is permitted which does not comply with these terms.
*Correspondence: Bethanie Carney Almroth, bethanie.carney@bioenv.gu.se
†These authors have contributed equally to this work
‡Orcid: Giedrė Ašmonaitė, orcid.org/0000-0002-4227-0792