- 1Princeton Environmental Institute and Department of Ecology and Evolutionary Biology, Princeton University, Princeton, NJ, United States
- 2Center for Systems Integration and Sustainability, Department of Fisheries and Wildlife, Michigan State University, East Lansing, MI, United States
- 3School for Environment and Sustainability, University of Michigan, Ann Arbor, MI, United States
Stream salmonid fisheries are ecologically and socioeconomically important at local to global scales throughout the world. Although these fisheries are interacting systems of biota, habitats, and humans, systematic social-ecological integration across space and time is scarce. However, theoretical and methodological advancements in the study of coupled human and natural systems (CHANS) offer new insights for stream salmonid research, management, and policymaking. The metacoupling framework is a novel tool for studying and managing social-ecological linkages that occur within stream salmonid fisheries as well as between adjacent and distant fisheries (i.e., metacouplings). For instance, coldwater streams containing brook charr (Salvelinus fontinalis) and brown trout (Salmo trutta) in Michigan, United States, encompass metacoupled movements of water, information, fish, people, and money throughout CHANS that provide drinking water, recreational fisheries, and employment. However, groundwater withdrawal is altering stream hydrology and causing public controversy over how hydrological changes affect salmonid populations and thermal habitats. Using this complex social-ecological scenario as a case study, we describe the utility of the metacoupling framework for fisheries systems analysis and demonstrate how this approach promotes metacoupled governance– management of relationships among metacoupled systems rather than specific physical places alone– to better sustain stream salmonid fisheries locally, regionally, and globally. Overall, stream salmonid science and management can be enhanced by using the metacoupling framework to synthesize social and ecological information, characterize cross-scalar tradeoffs and feedbacks, understand stakeholder diversity, and ultimately develop metacoupling-informed policies that promote socially and ecologically desirable outcomes.
Introduction
Fisheries are ecologically, socioeconomically, culturally, and nutritionally important resources at local to global scales. For instance, fish are predators of– and prey for– numerous aquatic organisms, transfer energy among trophic levels, recycle nutrients within and between aquatic and terrestrial ecosystems, and serve as indicators of environmental impairment (Fausch et al., 1990; McIntyre et al., 2007; Frisch et al., 2014). In addition, fish support commercial, recreational, and subsistence fisheries and associated economies while playing important roles in religion, art, folklore, mythology, and other aspects of human culture across the world (Moyle and Moyle, 1991; Taylor et al., 2007; Liebich et al., 2018). Moreover, fish are vital for food security in many of the world’s low-income food-deficit countries. Fish is the primary protein source for one in five people throughout the world (>1 billion), with 3.2 billion people depending on fish for at least 20% of their animal protein intake (Food and Agriculture Organization [FAO], 2018).
As such, it is clear that fish contribute much to the integrity and sustainability of aquatic ecosystems and human systems alike. Fisheries are generally defined by three major components (i.e., biota, habitats, humans) and their associated structures, functions, and interactions (Hubert and Quist, 2010). Each of these components has individually received ample research attention extending back to the dawn of fisheries science (Baranoff, 1918; Ricker, 1954; Vannote et al., 1980; Dietz et al., 2003; Hilborn et al., 2004). However, research on the linkages and feedbacks among fisheries biota, habitats, and humans– indeed, the very identity of fisheries as coupled human and natural systems (CHANS) – is comparatively scarce, representing a hindrance to socially, ecologically robust fisheries management that achieves objectives set for fish and human stakeholders alike (Carlson et al., 2017b, 2018). To date, fisheries CHANS research has yielded insights for understanding social-ecological couplings at relatively large scales (e.g., national, global; Wilson, 2006; Pinsky and Fogarty, 2012; Österblom and Folke, 2015; Tapia-Lewin et al., 2017). Studies such as these lay a foundation for more comprehensive fisheries CHANS research that explicitly evaluates social-ecological linkages at local to global scales. Such multi-scalar fisheries research has been uncommon to date and resulted in relatively limited understanding of– and few management programs that leverage– the magnitude, causes, and effects of fisheries interactions locally, regionally, and globally. Recently, researchers have classified these local to global interactions as metacouplings (i.e., socioeconomic and environmental interactions within individual CHANS, as well as between adjacent and distant CHANS) and developed a metacoupling framework for evaluating and ultimately managing social-ecological systems locally, regionally, and globally (Liu, 2017, 2018; Liu et al., 2018).
Since publication of the first metacoupling study approximately two years ago by Liu (2017), the metacoupling framework has not been widely applied to fisheries. This is unfortunate as the metacoupling framework provides aquatic resource professionals with a propitious tool for investigating when, where, why, and how fisheries function as CHANS and understanding the causes, effects, and management/policy relevance of fisheries’ social-ecological interactions, locally and globally. For instance, invasive species, climate change, watershed fragmentation, and habitat degradation (via land-use change, groundwater withdrawal, etc.) are anthropogenic stressors that increasingly affect fish production, recruitment, and survival locally and throughout the world, often in negative ways (Roni et al., 2008; Myers et al., 2017; Carlson et al., 2019a). As such, the metacoupling framework provides a systematic, adaptive approach for studying these coupled human-natural stressors and associated fisheries effects (i.e., metacouplings) in terms of their flows (e.g., fish, money, information), human and organizational actors, drivers, and consequences. In turn, diverse social-ecological information provided by the metacoupling framework (e.g., cross-scalar tradeoffs, feedbacks, surprises; Liu, 2017) is a leverage point for sustainable fisheries management and governance that improves social and ecological outcomes at local to global scales (Carlson et al., 2017b, 2018).
Salmonid fisheries are ideal systems for metacoupling research due to their global distribution coupled with their regional and local importance (e.g., ecological, socioeconomic, recreational, commercial, cultural, nutritional) throughout the world (Lynch et al., 2002; Quinn, 2005; Prosek, 2013). Stream salmonid fisheries in the Midwestern United States provide billions of dollars in annual economic benefits to local communities and have a long, rich history of supporting human recreation and nutrition (Weithman and Haas, 1982; Gartner et al., 2002; Schroeder, 2013; Anderson, 2016; Carlson et al., 2016; Cooke et al., 2017; Carlson and Zorn, 2018). In the State of Michigan, United States, stream-dwelling brook charr (Salvelinus fontinalis) and brown trout (Salmo trutta) have enormous socioeconomic and recreational significance. For instance, brown trout are the primary target of anglers in many Michigan rivers, making the species a fisheries management priority for the Michigan Department of Natural Resources (DNR) Fisheries Division, which considers brown trout an “important naturalized species” rather than a harmful non-native species (Michigan Department of Natural Resources [MDNR], 2015). Brook charr and brown trout support multimillion-dollar sport fisheries in Michigan and represent a crucial component of statewide fisheries that generate US$2.5 billion in retail sales and $4.2 billion in overall economic effect (U.S. Fish and Wildlife Service [UWFWS], 2013; Zorn, 2018). These species help support 38,000 angling-related jobs in Michigan and enrich the angling experiences of 586,000 riverine anglers spread across 8,159,000 angling-days every year (Southwick Associates, 2012; Zorn, 2018). Moreover, naturally-reproduced brook charr and brown trout in Michigan streams (n = 2.7 million annually, 3.5 times the number stocked) provide an economic benefit of $5.6 million every year (Wills et al., 2006; Zorn et al., 2018).
Given their unquestionable socioeconomic and societal value and important ecological roles as predators and prey, brook charr and brown trout are ideal species for integrative social-ecological research using the metacoupling framework. However, salmonid research to date has generally not accounted for the social-ecological interactions involved in brook charr and brown trout fisheries at both intra- and inter-stream scales. As such, the purpose of this study was to evaluate how Michigan stream salmonid fisheries are socioeconomically and ecologically connected within and between adjacent and distant streams using the metacoupling framework, with the goal of developing an approach for leveraging multi-scalar social-ecological information to advance the sustainable management of salmonid fisheries in Michigan and beyond. Our analysis focused on Chippewa Creek and Twin Creek, two coldwater, groundwater-dominated streams containing brook charr and brown trout whose watersheds share a common boundary in Osceola County, MI, United States (Figure 1). These rivers are impacted by land-use change and groundwater withdrawal resulting from human development, timber production, and a large-capacity (0.016 m3/s, 360,000 gallons per day) groundwater well owned by Nestlé Water North America (hereafter Nestlé) that lies on the streams’ shared watershed boundary (Figure 1; Waco and Taylor, 2010). Our objectives were to characterize the social-ecological structure (e.g., systems, causes, effects) of stream salmonid metacouplings– particularly those related to groundwater withdrawal, land-use change, and stream temperature–in Twin and Chippewa creeks and demonstrate how the metacoupling framework provides the information depth and breadth necessary for ecologically and socioeconomically informed groundwater governance and salmonid management programs. Given the ubiquity of CHANS in aquatic and terrestrial environments throughout the world, we hope to lay a foundation for future metacoupling research within and beyond stream salmonid fisheries so that other areas of conservation science and practice can adopt, and benefit from, a social-ecological and local-regional-global (i.e., metacoupled) perspective.
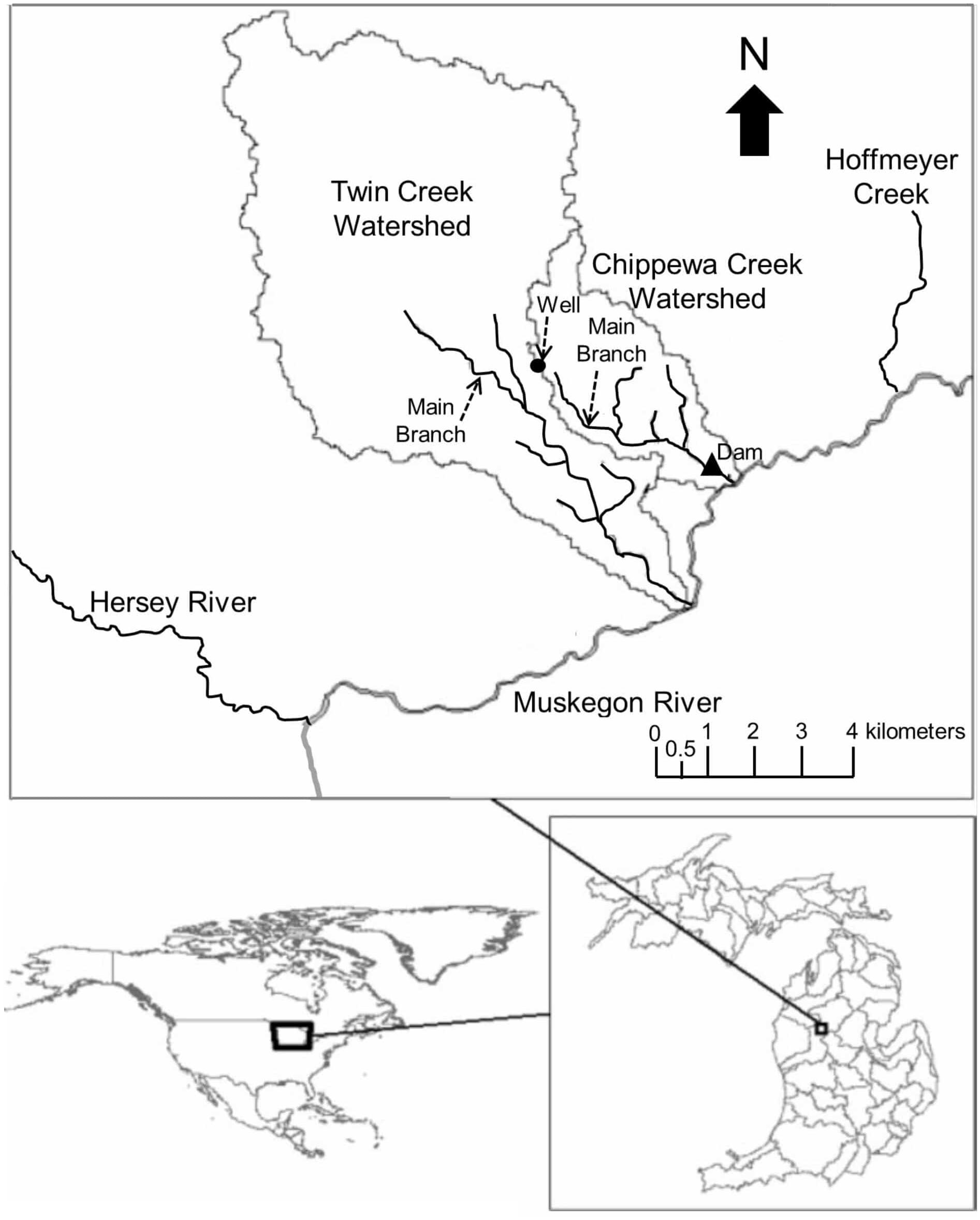
Figure 1. Map of the study area, including the Twin and Chippewa creek watersheds, Hoffmeyer Creek, and the Hersey River in Osceola County, MI, United States. The black circle denotes a large-capacity (0.0040 m3/s, 100,000 gallons per day) groundwater well owned by Nestlé Water North America. The black triangle shows a dam in the lower portion of Chippewa Creek that prevents upstream fish movement.
Materials and Methods
Metacoupling Framework
The metacoupling framework builds on concepts such as globalization (socioeconomic interactions between human systems over distances), teleconnection (environmental interactions between natural systems over distances), and telecoupling (socioeconomic and environmental interactions between CHANS over distances; Dreher et al., 2008; Liu et al., 2013). The metacoupling framework makes conceptual and empirical advancements over the above paradigms because it simultaneously considers socioeconomic and environmental interactions (unlike globalization and teleconnection) at local, regional, and global scales (unlike telecoupling; Liu, 2017). In other words, the metacoupling framework is designed to assess human-nature interactions at three distinct spatial levels: local (within individual CHANS such as Twin Creek), regional (between adjacent CHANS such as Twin and Chippewa creeks), and supraregional (between non-adjacent CHANS such as Twin and Hoffmeyer creeks). Together, these local interactions (intracouplings), regional interactions (pericouplings), and supraregional interactions (telecouplings) constitute metacouplings (Figure 2).
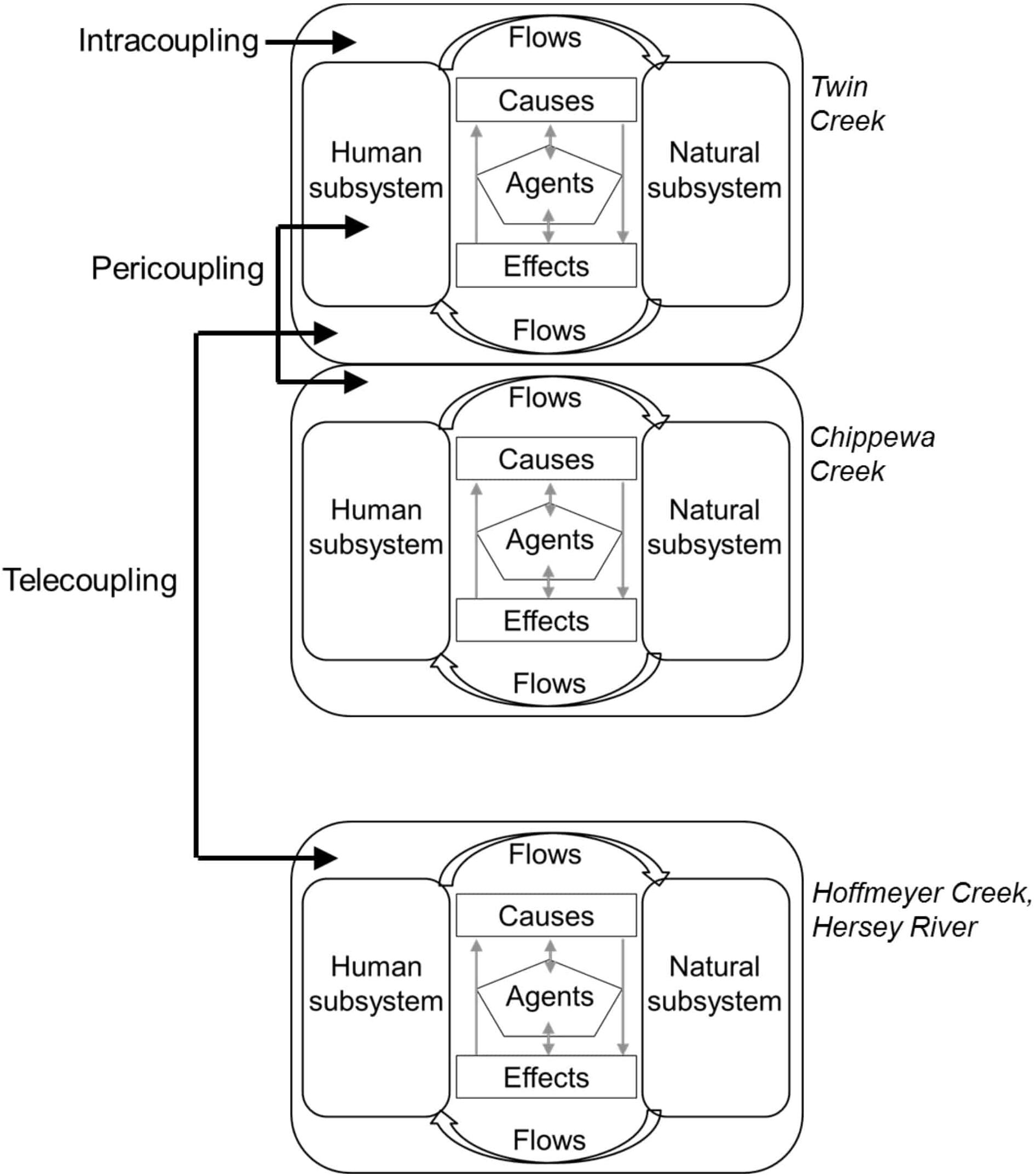
Figure 2. Diagram illustrating metacoupling:social-ecological interactions within a particular or local fishery (intracoupling) as well as between adjacent fisheries (pericoupling) and between distant, non-adjacent fisheries (telecoupling). Different coupling types are illustrated using rivers from the study area (see Figure 1). White and black arrows depict flows, defined as movements of water, information, fish, people, and money within and between fisheries. See Table 1 for details on metacoupling systems, flows, agents, causes, and effects.
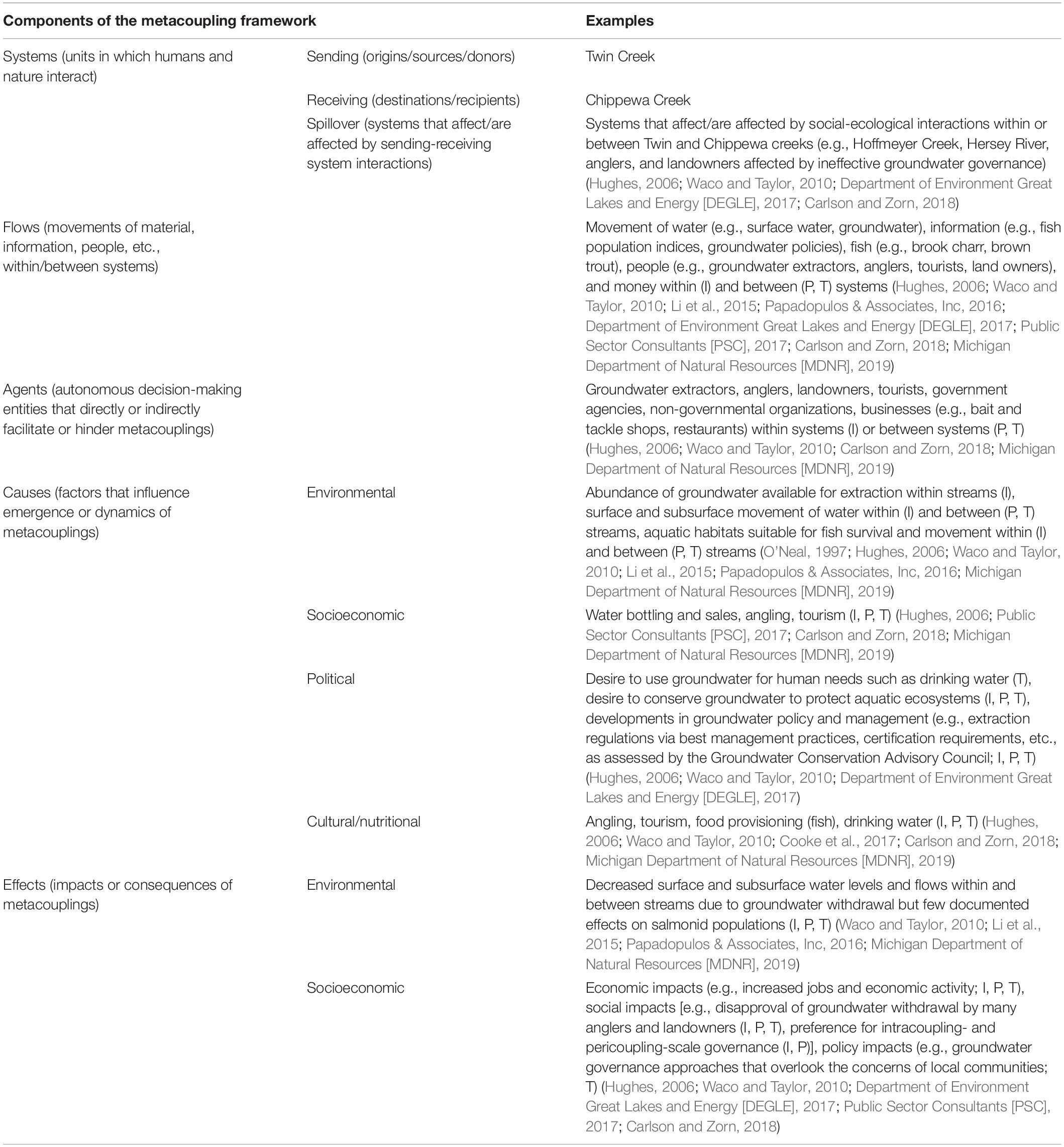
Table 1. Summary of systems, flows, agents, causes, and effects associated with metacouplings involving Michigan stream salmonid fisheries. Intracoupling, pericoupling, and telecoupling processes are denoted by I, P, and T, respectively.
Metacouplings– and the intracouplings, pericouplings, and telecouplings they encompass– have five common elements: systems, flows, agents, causes, and effects (Liu et al., 2013; Liu, 2017; Figure 2). Systems (i.e., CHANS) are defined according to their position relative to flows (movements of organisms, money, information, people, etc.). Sending systems are those in which flows originate, receiving systems are those to which flows move, and spillover systems are those that affect– or are affected by– interactions between sending and receiving systems (Liu et al., 2013; Liu, 2017). For example, when two stream salmonid fisheries (A and B) are connected by flows of fish, money, information, and people, and agricultural expansion leads to deforestation within stream A, effects of land-use change (e.g., reduced fish growth, survival, habitat quality/quantity) may cascade to stream B or “spill over” to affect streams C, D, etc. (spillover systems) that are not directly involved in sending-receiving system interactions. Agents are the individuals, organizations, governments, and other entities (including fishes, climate, etc.) involved in human-nature couplings. All couplings are driven by causes (e.g., ecological, economic, social, political) and produce effects (social-ecological outcomes and consequences; Figure 2).
By systematically assessing these five metacoupling elements, researchers can address important questions about the structure and function of social-ecological interactions in fisheries. For instance, how are individual stream salmonid fisheries connected to adjacent and distant fisheries via social-ecological linkages (e.g., fish movement, human movement, information transfer, monetary exchange)? What are the implications of metacouplings for stream salmonid management and governance? Regarding groundwater withdrawal in particular, how can metacoupling analysis holistically link potential changes in stream hydrology and salmonid thermal habitats to human values and attitudes about groundwater extraction and thereby inform water and fisheries management decisions?
Metacoupling research is broadly categorized as empirical (i.e., analysis of author-collected data; Liu et al., 2015; Sun et al., 2017) or synthetic, involving integration of prior research on metacoupling systems, flows, agents, causes, and effects that were studied without formal application of the metacoupling framework (Hulina et al., 2017; Carlson et al., 2018). The present study is both empirical and synthetic, as we collected much of the social and ecological data on which this manuscript is based (Hughes, 2006; Waco and Taylor, 2010; Carlson and Zorn, 2018) and, over time, integrated these data using the metacoupling framework. Such is the nature of metacoupling research, wherein multiple studies build on each other and eventually facilitate social-ecological synthesis (Liu, 2017). We recognize that Michigan stream salmonid fisheries encompass a metacoupled system involving social-ecological phenomena (e.g., watershed fragmentation, invasive species, stocking; Cooper et al., 2016; Zorn, 2018; Smith et al., 2019) beyond those studied herein. However, we focused on groundwater withdrawal, land-use change, and stream temperature due to the unique social-ecological importance of these issues in Twin and Chippewa creeks. Although these topics have been researched extensively in our study area and associated data are abundant (Hughes, 2006; Waco and Taylor, 2010; Department of Environment Great Lakes and Energy [DEGLE], 2017; Public Sector Consultants [PSC], 2017), previous research has been monothematic– it has emphasized either social or ecological phenomena rather than social-ecological interactions. As such, there is a need to investigate social-ecological dynamics of groundwater withdrawal, land-use change, and stream temperature using the metacoupling framework. These reasons for studying certain metacoupled issues before others (i.e., importance for the study area, available data) are common to all metacoupling analyses, which are inherently iterative due to the infeasibility of investigating all metacoupling components simultaneously given limitations in time, money, personnel, etc. (Liu, 2017). Instead, metacoupling framework operationalization– a multi-phase process of goal-setting, system selection, literature review, coupling delineation, and research communication (Liu, 2017) – demands subdividing metacoupling investigations into smaller interconnected projects and eventually synthesizing them, as is done herein for Hughes (2006), Waco and Taylor (2010), Carlson and Zorn (2018), and related studies. The present study is the first investigation of fisheries metacouplings related to groundwater withdrawal, land-use change, and stream temperature in our study area, laying a foundation for future metacoupling analyses of other social-ecological phenomena.
Study Systems and Data
Brook charr are native to Michigan’s Upper Peninsula and coastal streams in the northernmost portion of the Lower Peninsula (Zorn et al., 2018). Brook charr were first introduced to streams in southwestern Michigan in 1879, whereas brown trout were first introduced to Michigan in 1884. Both species inhabit Twin and Chippewa creeks, which are relatively small streams flowing 10 and 5 km, respectively, before reaching their confluence with the Muskegon River (Figure 1). Despite their size, Twin and Chippewa creeks are classified as Designated Trout Streams by the Michigan DNR in recognition of their cold, groundwater-dominated thermal conditions and forested riparian zones and watersheds that create high-quality physicochemical habitats for salmonids and aquatic macroinvertebrates (Waco and Taylor, 2010; Wesener, 2010; Michigan Department of Natural Resources [MDNR], 2019). Both streams have simple fish communities primarily composed of brook charr, brown trout, mottled sculpin Cottus bairdii, creek chub Semotilus atromaculatus, and central mudminnow Umbra limi (Michigan Department of Natural Resources [MDNR], 2019). These fish communities are novel (i.e., historically created by salmonid stocking) yet stable and self-sustaining, as neither Twin nor Chippewa Creek has been stocked with brown trout or brook charr since the 1930s, 1940s, and 1950s (Michigan Department of Natural Resources [MDNR], 2019). In this study, the ecological impacts of groundwater withdrawal and land-use change are considered relative to these novel fish communities. However, it is recognized that brown trout (and, in some cases, brook charr) introductions can have profound effects on aquatic ecosystems, including population fragmentation and local extinction of native fishes and aquatic invertebrates, interspecific competition for food and resting spaces, and food web restructuring (Fausch and White, 1981; Townsend, 1996; Spens et al., 2007; Budy et al., 2013). Although ecological concerns about salmonid introductions receive comparatively little attention in Michigan, where brown trout are managed as an “important naturalized species” and brook charr are native throughout most of their range in the state (Michigan Department of Natural Resources [MDNR], 2019), future research on stream salmonid metacouplings in other parts of the world should consider undesirable ecological effects of these species.
Overall, we focused on Twin and Chippewa creeks because they are high-quality salmonid streams, groundwater is being withdrawn by an industrial-scale water bottling facility in their watersheds, and these systems are vulnerable to land-use change resulting from human development and timber production (Waco and Taylor, 2010). In addition, groundwater withdrawal has been studied in Twin and Chippewa creeks for two decades (Malcolm Pirnie Inc. [MPI], 2000; Li et al., 2015; Advanced Ecological Management [AEM], 2016; Papadopulos & Associates, Inc, 2016; U. S. Geological Survey. [USGS], 2020a, b), associated data are available and warrant analysis, and this issue has great metacoupling relevance amid ongoing public controversy regarding the social-ecological effects of groundwater extraction on salmonid populations and human communities (Hughes, 2006; Waco and Taylor, 2010; Department of Environment Great Lakes and Energy [DEGLE], 2017). These complex social-ecological conditions are best addressed using a framework such as metacoupling that synthesizes social and ecological information across spatial scales (Liu, 2017).
We projected effects of various potential groundwater withdrawal regimes on baseflow and stream temperatures in Twin and Chippewa creeks using Interactive Groundwater (IGW), a computer-based hydrology tool for quantifying and simulating groundwater flow based on geology, elevation, topography, and surface water features within streams and their watersheds (Li and Liu, 2006). IGW models were first calibrated to assess how various levels of groundwater withdrawal– 0.0044 m3/s [70 gallons per minute (gpm)], 0.0095 m3/s (150 gpm), 0.0252 m3/s (400 gpm), 0.0442 m3/s (700 gpm), 0.0631 m3/s (1,000 gpm), and 0.1262 m3/s (2,000 gpm/) – would affect stream baseflow in Twin and Chippewa creeks (Waco and Taylor, 2010). At present, the continuous rate of groundwater withdrawal in these streams is 0.016 m3/s (250 gpm); an increase to 0.025 m3/s (400 gpm) was approved by the Michigan Department of Environment, Great Lakes, and Energy (DEGLE) in April 2018 and is in the process of being implemented. Groundwater extraction models were then combined with an assessment of how four hypothetical land-use changes (i.e., forest to pasture, grassland to urban, agriculture to grassland, forest to shrub land) would affect groundwater recharge rates (Waco and Taylor, 2010). Using outputs from the above models, a U.S. Geological Survey (USGS) stream temperature model (SSTEMP; Bartholow, 2002) was used to predict how summer stream temperature (July, August) and discharge would change as a result of changes in baseflow caused by groundwater withdrawal and land-use change. Full methodological details are available in Waco and Taylor (2010). Results from Waco and Taylor (2010) were integrated with findings from other hydrological research in Twin and Chippewa creeks (Malcolm Pirnie Inc. [MPI], 2000; Li et al., 2015; Advanced Ecological Management [AEM], 2016; Papadopulos & Associates, Inc, 2016) to understand the effects of groundwater withdrawal on stream temperature, discharge, water levels, and salmonid thermal habitats. This included an assessment of ongoing daily (15min interval) measurements of discharge in Twin and Chippewa creeks (U. S. Geological Survey. [USGS], 2020a, b) that were initiated by the USGS in late November/early December 2018 to provide an independent third-party review of groundwater withdrawal’s hydrological effects. Expanding hydrological and ecological results from Waco and Taylor (2010) to a full-fledged metacoupling investigation examining social-ecological couplings required information on economic contributions and human perceptions of groundwater withdrawal and land-use change within and beyond Twin and Chippewa creeks. With its rich groundwater and surface water resources, the State of Michigan is home to numerous water- and fisheries-related governmental agencies (e.g., DNR; DEGLE) and non-governmental organizations (NGOs; e.g., Muskegon River Watershed Council, Michigan Citizens for Water Conservation, Michigan Trout Unlimited) that advocate for aquatic resource conservation. Combined with the abundance and social-ecological importance of stream salmonid fisheries in Michigan (Carlson and Zorn, 2018; Zorn, 2018; Zorn et al., 2018), the diversity of groundwater stakeholders operating at local, regional, and supraregional levels– scales corresponding with those of the metacoupling framework (i.e., intracouplings, pericouplings, telecouplings) – renders Michigan an ideal study area for aquatic metacoupling research. Groundwater withdrawal increased in Michigan in the mid-2000s due to expansion of water bottling facilities, prompting the need for salmonid thermal habitat research (e.g., Waco and Taylor, 2010) and creation of a Groundwater Conservation Advisory Council (hereafter Groundwater Council). Composed of representatives from diverse sectors (e.g., agriculture, utilities, business/manufacturing, local government, NGO, general public), the Groundwater Council was tasked with studying the sustainability of Michigan’s groundwater use and determining how the state could best regulate groundwater extraction via best management practices, certification requirements, and related approaches.
Recognizing that Michigan’s groundwater withdrawal issue involves metacoupling dynamics (e.g., extraction’s effects on local/regional/state economies, human opinions and attitudes, stream salmonids and thermal habitats), we conducted semi-structured interviews of 30 Groundwater Council members and their colleagues in 2005 to measure human values, attitudes, and policy preferences regarding groundwater use and sustainability (Hughes, 2006). The interview methodology and questions were scrutinized and approved by the Michigan State University Institutional Review Board (IRB #05-292) to ensure that the research approach was appropriate for a human-subjects investigation. Interviewees were groundwater policymakers and/or researchers that spanned a wide range of ages, political identities, and education levels (Supplementary Table S1), providing a robust human dimensions dataset that was essential for management-relevant metacoupling research (Liu, 2017). As described fully in Hughes (2006), interviews typically lasted for 1hand involved fixed and open-ended questions regarding social-ecological effects of groundwater withdrawal and groundwater’s economic contributions in Michigan. Interview information of particular relevance for metacoupling analysis included Groundwater Council members’ perceptions of how spatial scale influences groundwater-related issues in Michigan (e.g., water quality, extraction policy effectiveness, information dissemination) and their opinions regarding the scales (i.e., stream, watershed, state, Great Lakes region) at which groundwater withdrawal decisions should be made. These data were compared with hydrological information using the metacoupling framework to identify couplings (intracouplings, pericouplings, telecouplings) that need to be created and enhanced for socially and ecologically informed groundwater management.
We also evaluated economic contributions of groundwater withdrawal in terms of jobs and economic activity supported at the intracoupling, pericoupling, and telecoupling scales using data from a report published by Public Sector Consultants (Public Sector Consultants [PSC], 2017). The intracoupling (county) scale included the Osceola and Mecosta counties, the pericoupling (regional) scale included five counties across the Muskegon River watershed (Kent, Mecosta, Montcalm, Newaygo, Osceola), and the telecoupling (statewide) scale encompassed all of Michigan. Input-output analysis and IMPLAN (IMpact analysis for PLANning) software were used to estimate economic contributions of Nestlé’s groundwater withdrawal operations, facility expansion, and capital expenditures in 2017. Economic contributions were classified as direct (annual employment and spending), indirect (annual employment and spending generated via purchase of goods and services), and induced (annual economic contributions from household spending of people directly or indirectly employed by Nestlé’s economic activity; Public Sector Consultants [PSC], 2017). Full methodological details are available in Public Sector Consultants [PSC] (2017).
In addition, we worked with the Michigan DNR to design and conduct an Inland Trout Angler Survey (ITAS) in 2015 to assess opinions and practices of Michigan’s stream salmonid anglers with respect to salmonid regulations and management priorities in inland waterbodies (i.e., streams, inland lakes; Carlson and Zorn, 2018). The survey was developed to provide information on these relatively unstudied characteristics of Michigan salmonid anglers– the last analogous study occurred in 1981 (Fenske, 1983) – for incorporation into the DNR’s first statewide management plan for inland populations of brook charr, brown trout, rainbow trout Oncorhynchus mykiss, lake trout Salvelinus namaycush, and splake Salvelinus fontinalis x S.namaycush. Like other stream salmonid angler surveys (Responsive Management, 2008; Schroeder, 2013; Petchenik, 2014), the ITAS included questions (n = 57) about stream salmonid anglers’ preferences for fishing methods, angling regulations, salmonid species, and waterbodies (including Michigan counties of primary fishing activity). The survey also asked anglers about their opinions regarding the DNR Fisheries Division’s salmonid management activities and identified their residences, particularly counties and zip codes (Carlson and Zorn, 2018). SurveyMonkey software was used to deliver the ITAS to Michigan’s 83,000 salmonid anglers in March 2015. Full survey details are available in Carlson and Zorn (2018).
We analyzed ITAS data to generate an overall picture of fishing practices and fisheries management opinions of Michigan stream salmonid anglers while also identifying differences between key segments of the state’s salmonid angling population to provide a basis for understanding fisheries metacouplings. In particular, the ITAS was used to obtain information about the metacoupling components– flows (e.g., information, people, money), agents (e.g., anglers, fisheries agencies), causes, and effects– that link Twin and Chippewa creeks and other rivers in our study area (Figure 1). For instance, intracoupling, pericoupling, and telecoupling flows of anglers were measured by subdividing ITAS respondents (n = 4,161) by Michigan county of primary fishing, county of residence, and zip code of residence. We emphasized the location of Twin and Chippewa creeks in Osceola County and, more specifically, zip code 49631 (where these streams are primary trout fishing destinations). The number of stream salmonid anglers who lived and fished in Osceola County (i.e., intracoupling flow) was compared with the number of anglers who lived outside but fished in the county (i.e., pericoupling/telecoupling flow) to delineate angler-flow metacouplings at the county scale. The same logic was applied to zip code 49631 to approximate intracoupling, pericoupling, and telecoupling angler flows at the stream scale (Twin and Chippewa creeks). In addition, ITAS data were partitioned into “members” and “non-members” of stream salmonid angling groups (i.e., Michigan Trout Unlimited, Anglers of the Au Sable, Federation of Fly Fishers), enabling assessment of attitudinal and behavioral differences (e.g., stream selection factors, preferred fishing regulations and tackle, salmonid management opinions) between these different “agents” of angling. Overall, findings from our groundwater, land-use, and stream temperature modeling (Waco and Taylor, 2010), Groundwater Council interviews (Hughes, 2006), and the ITAS (Carlson and Zorn, 2018) were integrated with other information sources (e.g., Li et al., 2015; Department of Environment Great Lakes and Energy [DEGLE], 2017; Public Sector Consultants [PSC], 2017; U. S. Geological Survey. [USGS], 2020a, b) using the metacoupling framework to identify ecologically and socially balanced strategies for groundwater governance and salmonid management. These balanced, metacoupled strategies were defined as those that holistically integrate environmental and human information across spatial scales and account for potential social-ecological trade-offs in groundwater and fisheries decision-making.
Results and Discussion
Intracouplings
Intracouplings are social-ecological linkages that occur within, in this case, individual stream salmonid fisheries (Figure 2). Examples of intracouplings affecting salmonid fisheries include groundwater extraction (via changes in water volume, temperature, etc.), land-use change (via changes in water quality, physiochemical habitats, etc.), angling and fish stocking (via changes in fish abundance, size, etc.), and watershed fragmentation by dams and roads (via changes in water depth, discharge, habitat connectivity, etc.; Waco and Taylor, 2010). An intracoupled system such as the Twin Creek salmonid fishery consists of human subsystems (e.g., local economy, communities of anglers, and tourists) and natural subsystems (e.g., salmonid populations, habitats, local weather) as well as flows, agents, causes, and effects that link the human and natural subsystems (Table 1 and Figure 2).
Flows of water, fish, people, and money connect human and natural subsystems in Twin and Chippewa creeks. These flows are promoted or inhibited by agents such as groundwater extractors, Groundwater Council members, anglers, governmental agencies, and NGOs (Table 1). For instance, a 2006 decision by the Michigan DEGLE (then the Department of Environmental Quality) allowed Nestlé to construct a large-capacity groundwater well near Twin and Chippewa creeks (Waco and Taylor, 2010). In the context of groundwater withdrawal, movement of water from aquifers (sending systems) to the Nestlé water bottling facility (receiving system) is an intracoupling flow. In this case, causes (reasons why intracouplings occur) are water bottling, resultant drinking water sales and revenue for Nestlé, and water’s abundance in this groundwater-rich region of Michigan (Table 1). Effects of groundwater withdrawal include county-level economic contributions, including 284 jobs and $24.2 million in total economic activity in 2017 alone (Table 2; Public Sector Consultants [PSC], 2017), and social effects such as disapproval of groundwater stakeholders (e.g., anglers, landowners) who oppose groundwater extraction because they believe it decreases stream water levels, salmonid abundance, and angling quality (Hughes, 2006; Waco and Taylor, 2010; Department of Environment Great Lakes and Energy [DEGLE], 2017; Public Sector Consultants [PSC], 2017).
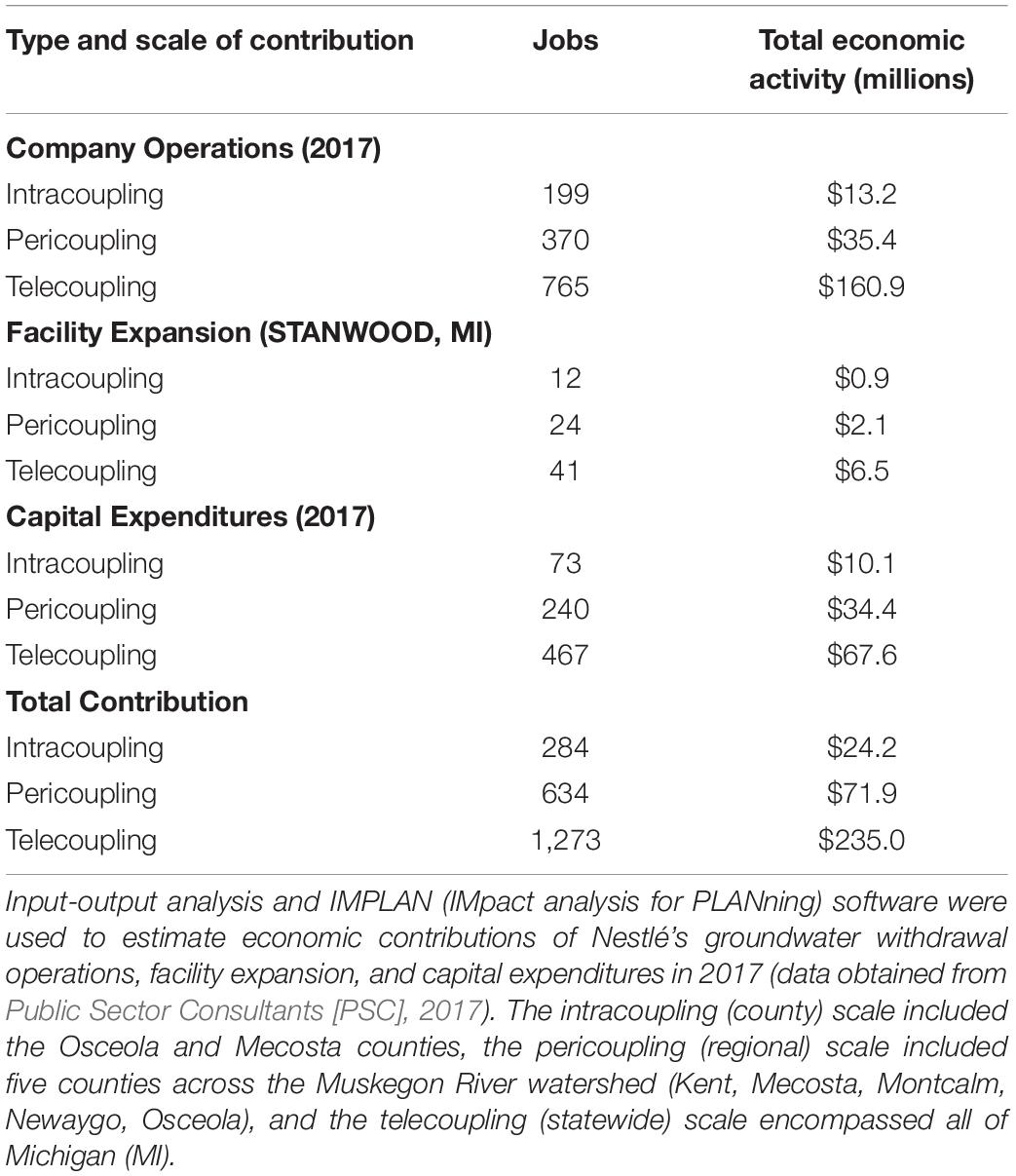
Table 2. Economic contributions of groundwater withdrawal (jobs, total economic activity) at the intracoupling, pericoupling, and telecoupling scales.
However, scientific evidence to date suggests that groundwater withdrawal and land-use change have relatively minor effects on stream salmonid thermal habitats in Twin and Chippewa creeks. For instance, groundwater extraction regimes ranging from 0 to 0.1262 m3/s (2,000 gpm) were projected to increase summer stream temperatures in Twin and Chippewa creeks by ≤ 0.91°C (Supplementary Tables S2, S3), and 0.10°C was the largest stream temperature increase predicted from land-use change, particularly a grassland-to-urban transition (Supplementary Table S4; Waco and Taylor, 2010). In Twin Creek, an observed decrease in baseflow of 0.0449 m3/s (712 gpm) caused surface water levels to decrease by 9.8 cm between 2006 and 2015 (Papadopulos & Associates, Inc, 2016). In Chippewa Creek, a baseflow decline of 0.0110 m3/s (173 gpm) reduced surface water levels by 3.7 cm. Research suggests that these hydrological changes have not altered salmonid thermal habitat quality, which is considered excellent in both streams and characterized by water temperatures and depths that are cold and deep enough to withstand 0.91°C warming and a 9.8 cm water level decline (Waco and Taylor, 2010; Wesener, 2010; Li et al., 2015; Papadopulos & Associates, Inc, 2016).
Moreover, in the 14 months since the USGS began monitoring Twin and Chippewa creeks (U. S. Geological Survey. [USGS], 2020a, b), discharge has remained relatively consistent beyond expected daily and seasonal fluctuations (Supplementary Figures S1, S2), suggesting minimal impacts of recent groundwater extraction on stream hydrology and salmonid thermal habitats. Intracoupled flows of fish (e.g., movement within Twin and Chippewa creeks) have been largely unaffected by groundwater withdrawal and land-use change (Waco, 2009; Waco and Taylor, 2010; Li et al., 2015; Papadopulos & Associates, Inc, 2016; Michigan Department of Natural Resources [MDNR], 2019), yet human subsystems in Twin and Chippewa creeks are marked by controversy over groundwater withdrawal. In particular, local anglers and landowners claim to have observed local reductions in stream water levels, salmonid abundance, and angling quality due to groundwater withdrawal since 2006, and they feel victimized by a groundwater governance process that has overlooked their ecological and recreational concerns about groundwater extraction (Hughes, 2006; Department of Environment Great Lakes and Energy [DEGLE], 2017). The personal experiences of these stakeholders (e.g., catching fewer fish, feeling unrepresented in groundwater governance), coupled with claims by governance organizations (as yet unsupported by data) that groundwater withdrawal has not affected salmonid populations, have made anglers and landowners a spillover system (Table 1) whose frustration is growing will likely expand if groundwater withdrawal rates increase, as proposed by Nestlé. Such spillover effects have motivated anglers and landowners to travel to public meetings throughout the region, including those held at Ferris State University (Big Rapids, Michigan) in April 2017 and 2018, to voice their concerns over groundwater withdrawal (Department of Environment Great Lakes and Energy [DEGLE], 2017).
The Twin and Chippewa creek salmonid fisheries also feature intracouplings related to fish, people, and money. Not only do salmonids move naturally within the streams, anglers fish in the rivers and spend money at local businesses (e.g., bait and tackle shops, restaurants) in pursuit of recreational experiences and fish for consumption (Table 1; Carlson and Zorn, 2018; Zorn et al., 2018; Michigan Department of Natural Resources [MDNR], 2019). Effects of these intracouplings include fish harvest as well as fish consumption and human nutrition (fish is an excellent source of protein, micronutrients, and essential fatty acids; Cooke et al., 2017; Bennett et al., 2018; Carlson and Zorn, 2018). In addition, angling is a source of psychological well-being, community cohesion, and revenue generation in local economies (Knuth, 2002; Zorn, 2018; Carlson et al., 2019b).
Pericouplings
Whereas intracouplings are social-ecological linkages within individual stream salmonid fisheries, pericouplings are social-ecological linkages between adjacent fisheries systems (Figure 2). Although Twin and Chippewa creeks each have intracoupled flows of water, information, fish, people, and money, the adjacency of these streams permits between-system, pericoupled flows (Figure 2). For instance, water moves between the streams via surface, hyporheic, and groundwater flows; salmonids and other fishes move naturally between the streams; and people (e.g., anglers, landowners, tourists) move between the watersheds, often causing additional flows of money and information (Table 1; O’Neal, 1997; Li et al., 2015; Advanced Ecological Management [AEM], 2016; Papadopulos & Associates, Inc, 2016; Carlson and Zorn, 2018; Michigan Department of Natural Resources [MDNR], 2019). In addition, groundwater withdrawal generates pericoupled economic contributions, including 634 jobs and $71.9 million in total economic activity in the five-county region surrounding Twin and Chippewa creeks (Table 2; Public Sector Consultants [PSC], 2017). Not only do these pericouplings affect water and fish distribution as well as corporate and consumer spending at a multi-river scale (O’Neal, 1997; Public Sector Consultants [PSC], 2017; Carlson and Zorn, 2018; Zorn, 2018; Zorn et al., 2018), they influence stakeholder perceptions of groundwater governance. For instance, the majority of Groundwater Council members (77%, n = 20; Supplementary Table S5) stated that groundwater withdrawal decisions should account for individual streams and their connections across aquifers and watersheds (i.e., intracouplings and pericouplings), linkages whereby groundwater extraction can affect hydrology of non-target streams (Hughes, 2006). Such intracoupling- and pericoupling-scale governance corresponds with scales at which groundwater modeling and aquifer recharge occur in the study area (Waco and Taylor, 2010; Li et al., 2015; Papadopulos & Associates, Inc, 2016), suggesting a metacoupling “win-win” scenario wherein governance preferences and hydrological phenomena are spatially aligned. However, this is not always the case. For example, in selecting groundwater-related problems in Michigan, a plurality of Groundwater Council members (36%, n = 11) chose water quality. This suggests a metacoupling “mismatch,” as a socially-identified problem (water quality) cannot be fully addressed using socially-preferred solutions (intracoupling- and pericoupling-scale policies) because water quality impairment is driven by metacoupled ecological stressors such as point-source and non-point-source pollution, climate change, and land-use change that transcend intracouplings and pericouplings (Waco and Taylor, 2010; Carlson et al., 2017a, c). In Twin and Chippewa creeks, additional data are needed to evaluate how water quality is affected by groundwater withdrawal and other metacoupled ecological stressors, allowing for development of water quality management approaches that account for intracouplings, pericouplings, and telecouplings.
In some cases, pericouplings between stream fisheries are disrupted by stressors such as groundwater withdrawal and watershed fragmentation. For instance, groundwater withdrawal reduces surface and subsurface water levels and flows between Twin and Chippewa creeks, but studies indicate minimal effects on salmonid populations (Waco and Taylor, 2010; Li et al., 2015; Advanced Ecological Management [AEM], 2016; Papadopulos & Associates, Inc, 2016). In addition, pericoupled fish movements from Twin Creek to Chippewa Creek are impeded by an impoundment near the latter’s confluence with the Muskegon River (Figure 1), even though downstream movements from Chippewa to Twin creek are unimpeded (O’Neal, 1997; Waco, 2009; Waco and Taylor, 2010; Michigan Department of Natural Resources [MDNR], 2019). Ultimately, groundwater withdrawal’s real and perceived effects have ignited public controversy in the absence of community-engaged governance, which seeks to incorporate the social-ecological concerns of all stakeholders and thereby build trust and shared understanding (Hughes, 2006; Ansell and Gash, 2008). Without such governance, communities of anglers and landowners are spillover systems without adequate representation in the policy arena.
Telecouplings
Telecouplings, unlike intracouplings and pericouplings, are social-ecological linkages between distant, non-adjacent fisheries systems (Figure 2). For instance, the arrival of the Nestlé corporation in the Chippewa Creek and Twin Creek watersheds was caused by an information telecoupling involving a policy decision at Nestlé headquarters (in Stamford, Connecticut), with subsequent Michigan DEGLE approval, to bottle water and generate revenue in a groundwater-rich region of Michigan. Likewise, for 67 years, organizations such as the Michigan DNR, Michigan DEGLE, and Advanced Ecological Management have practiced aquatic resource management in Twin (and more recently Chippewa) Creek by collecting hydrological and biological data (e.g., water temperature, discharge, fish, and macroinvertebrate abundance) and distributing these data via information telecouplings throughout the region and the state (Michigan Department of Natural Resources [MDNR], 2019). Telecouplings also affect stakeholder perceptions of groundwater governance, as evidenced by Groundwater Council members’ general lack of support for telecoupled groundwater withdrawal policies– those scaled to the state and Great Lakes basin levels, favored by 23% of interviewees (n = 6) – compared to intracoupling- and pericoupling-scale policies (Supplementary Table S5).
In addition, understanding telecouplings is important for groundwater governance and policy development because they can eclipse smaller-scale couplings with negative effects. For instance, agents such as the Michigan DEGLE promote groundwater regulation and conservation (i.e., causes) by developing statewide and regional policies for groundwater withdrawal. By and large, these telecoupling- and pericoupling-scale policies do not contain local, intracoupling-level information about how groundwater extraction will affect specific streams or communities of anglers, landowners, and other groundwater stakeholders. Moreover, groundwater policies are disseminated throughout the State of Michigan via information telecouplings (e.g., online/printed reports and news articles; Department of Environment Great Lakes and Energy [DEGLE], 2017; Table 1) rather than localized intracouplings that target stakeholders who live or fish near water bottling facilities and wells. The predominance of telecoupling-scale policies and information exchange influences stakeholder perceptions of groundwater governance. For instance, Groundwater Council members stated that groundwater-related problems in Michigan include ineffective state-level groundwater withdrawal policies (30%, n = 9 respondents), lack of publically-available local information on withdrawal (27%, n = 8), and public misconceptions of withdrawal’s local effects (7%, n = 2; Hughes, 2006). They believed that these problems could be best addressed by making policies and public outreach efforts more locally relevant (i.e., by enhancing information intracouplings). However, as exemplified in Twin and Chippewa creeks, flows of groundwater-related information within and between decision-making organizations (e.g., DEGLE, Nestlé) have not incorporated the concerns of local communities, making them spillover systems that are largely excluded from groundwater governance processes (Hughes, 2006; Department of Environment Great Lakes and Energy [DEGLE], 2017). Overall, emphasis on policy and information telecouplings at the expense of intracoupling-informed groundwater governance has caused public outcry and exacerbated a controversy over groundwater extraction that would have been less acrimonious if intracoupling-level concerns of local communities had been addressed.
Telecouplings also link coldwater streams and anglers. For instance, Twin Creek is connected with Hoffmeyer Creek, the Hersey River, and other salmonid streams within and outside Osceola County by telecoupled flows of anglers (Table 1). In a statewide survey of Michigan stream salmonid anglers (Carlson and Zorn, 2018), 53 of 4,161 respondents (1.3%) stated that Osceola County was their primary fishing county (Supplementary Table S6). In comparison, only 19 of these respondents (0.5% overall) lived in Osceola County, suggesting that the county had 1.6 times as many non-local (pericoupled/telecoupled) anglers as local (intracoupled) anglers in 2015. Extrapolating these percentages to the 83,000 stream salmonid anglers in Michigan (Carlson and Zorn, 2018), Osceola County had an estimated 1,079 stream salmonid anglers, 415 of whom were intracoupled and 664 of whom were pericoupled/telecoupled (Supplementary Table S6). Hence, more anglers moved into (and out of) Osceola County to fish for stream salmonids than those who fished and lived within it. Similarly, in the code containing Twin and Chippewa creeks, an estimated 100 stream salmonid anglers were intracoupled and 160 anglers were pericoupled/telecoupled, suggesting that angler movement pericouplings and telecouplings, in addition to intracouplings, play an important role in determining who fishes for stream salmonids in these streams (Carlson and Zorn, 2018).
Moreover, groundwater withdrawal causes telecouplings that affect humans in positive and negative ways. For instance, groundwater extraction has increased telecoupled flows of bottled water from Twin and Chippewa creeks to areas throughout Michigan and the United States, but groundwater withdrawal (originally caused by telecoupled information transfer between Connecticut and Michigan) has decreased surface and subsurface water levels and flows in these streams (Malcolm Pirnie Inc. [MPI], 2000; Waco and Taylor, 2010; Papadopulos & Associates, Inc, 2016; Public Sector Consultants [PSC], 2017). Groundwater withdrawal provides telecoupled economic contributions, including 1,273 jobs and $235.0 million in total economic activity throughout Michigan (Table 2; Public Sector Consultants [PSC], 2017), but communities of anglers and landowners are spillover systems, and their ecological and recreational concerns about groundwater extraction have not been adequately addressed by groundwater governance organizations (Hughes, 2006). In fact, public citizens aired their grievances about groundwater withdrawal in Twin and Chippewa creeks over a 205day comment period in which the Michigan DEGLE received 340,000 petition signatures (to stop increased groundwater extraction) and 50,000 statements of concern (e.g., environmental damage, resource rights infringement, water quality impairment), a rate of one comment every 6minfor nearly 7months (Department of Environment Great Lakes and Energy [DEGLE], 2017). Arising from stakeholders locally, regionally, and statewide, these comments generated metacoupled information flow that culminated in strong public opposition to groundwater withdrawal in Twin and Chippewa creeks. In addition, there are concerns that groundwater withdrawal will elicit telecouplings that affect other fish species that use, and move among, rivers and streams in the Muskegon River watershed. These fishes include burbot (Lota lota), northern pike (Esox lucius), smallmouth bass (Micropterus dolomieu), yellow perch (Perca flavescens), walleye (Sander vitreus), and at least 32 other species native to Michigan (O’Neal, 1997) whose habitats, movements, and ecological and socioeconomic services (e.g., predation, nutrient cycling, angling) could be affected by continuing groundwater withdrawal (Waco and Taylor, 2010; Department of Environment Great Lakes and Energy [DEGLE], 2017) – an important subject for future research.
Insights and Applications of the Metacoupling Framework
By providing an approach for systematic social-ecological integration across space and time, the metacoupling framework advances conventional research methods that focus only on socioeconomic or ecological dynamics operating in specific places. For instance, although uniform statewide regulation of groundwater withdrawal in Michigan is efficient in some ways, investigating social-ecological linkages demonstrates a stakeholder preference for intracoupling- and pericoupling-scale governance that actively engages human communities and considers groundwater dynamics in individual streams and watersheds (Hughes, 2006). Without such insights from the metacoupling framework, important groundwater stakeholders and their concerns have been overlooked, leading to negative social outcomes and ultimately governance that is socially and ecologically imbalanced, as demonstrated in Twin and Chippewa creeks. However, use of the metacoupling framework can impart social-ecological balance to groundwater governance across spatial scales and lead to positive outcomes, including improved relationships among the many stakeholders involved in aquatic resource management (Hughes, 2006).
In addition, by enabling holistic analysis of riverine ecosystems, social systems, and their interconnections, the metacoupling framework promotes aquatic resource management and policy approaches that address multiple social-ecological objectives and potential tradeoffs. For example, Waco and Taylor (2010) predicted that a riparian forest-to-shrub transition in Twin and Chippewa creeks would decrease water temperatures by 0.09°C and thereby help maintain cold thermal habitats needed by brook charr and brown trout (Raleigh, 1982; Raleigh et al., 1986; Lyons et al., 2009). However, metacoupling analysis indicated that this land-use change would also reduce angling (and associated revenue generation for local economies) because streams’ forested aesthetic beauty is highly valued by salmonid anglers (Carlson and Zorn, 2018). In fact, anglers ranked aesthetic beauty the most important among 16 decision factors for stream fishing, including the chance to catch brook charr, brown trout, rainbow trout, trophy trout, and large numbers of salmonids (Carlson and Zorn, 2018). Hence, it is important that salmonid managers account for the aesthetic value of forested riparian zones and watersheds alongside other important considerations (e.g., thermal habitat quality, anglers’ harvest and tackle preferences) to design socially and ecologically balanced, metacoupled strategies for salmonid management within and beyond Twin and Chippewa creeks. After all, what is ecologically beneficial for fisheries (e.g., thermally favorable land-use changes) can be socially and economically detrimental, and vice versa. By using a metacoupling perspective, fisheries and aquatic resource professionals can identify– and potentially reconcile– social-ecological complexities and tradeoffs in ways that promote holistic, sustainable groundwater governance and salmonid management.
Using the metacoupling framework also helps fisheries professionals differentiate between the many types of stakeholders they serve. For example, in our survey of Michigan stream salmonid anglers, members and non-members of stream salmonid angling groups (i.e., Michigan Trout Unlimited, Anglers of the Au Sable, Federation of Fly Fishers) often held disparate attitudes and opinions regarding stream salmonid fishing and fisheries management. In deciding whether or not to fish a particular stream, angling group members prioritized wild salmonids and trophy-sized salmonids to a greater degree than non-members, who believed that stocked salmonids and the number of salmonids caught were most important (Carlson and Zorn, 2018). Members were less harvest-oriented than non-members, and they attended fisheries-related public meetings more frequently, reflecting their comparatively high degree of personal involvement in Michigan stream salmonid management. By using the metacoupling framework to tease apart these important attitudinal and behavioral differences between fisheries stakeholder groups, fisheries professionals can design management strategies (e.g., catch limits, stocking rates, public outreach approaches) that are appropriately scaled to the diverse angler populations they serve, leading to improved social outcomes in fisheries management.
Similarly, the efficacy of stakeholder communication and engagement activities is regulated by metacouplings. For instance, according to our survey, the most popular resources that Michigan stream salmonid anglers use to plan fishing trips are the DNR Fishing guide (59% of anglers) and DNR online maps (42%; Carlson and Zorn, 2018). In addition, 63% of salmonid anglers use smart phones during fishing trips to access fishing-related information from the internet, which may be an intracoupled, pericoupled, or telecoupled process depending on the proximity between where anglers fish and where their information source is located. Hence, fisheries professionals can best communicate with anglers (regarding fish harvest regulations, groundwater withdrawal, etc.) by understanding the metacoupled nature of information exchange and designing communication mechanisms that cater to anglers’ preferences for printed and electronic resources (e.g., websites, smart phone apps) as opposed to using other media (e.g., DNR phone line, map books, bait shop contacts). Overall, the metacoupling framework helps fisheries professionals understand and manage the complexities of fisheries ecosystems and human systems, providing a knowledge base for enhancing the social-ecological resilience of fisheries that have traditionally been viewed through socially or ecologically focused (rather than integrated) lenses (Baranoff, 1918; Ricker, 1954; Vannote et al., 1980; Dietz et al., 2003; Hilborn et al., 2004).
Conclusion
In conclusion, the metacoupling framework helps advance stream salmonid research and management in numerous ways. For instance, the metacoupling framework operationalizes the study of salmonid fisheries as CHANS, offering an organized method for assessing the causes and effects of social-ecological linkages across local to global scales and thereby advancing conventional research approaches that are location-specific and either social or ecological. In addition, the metacoupling framework provides novel insights about stream salmonid fisheries (e.g., cross-scalar tradeoffs, feedbacks, surprises). In turn, these insights represent leverage points for ecologically, socioeconomically informed fisheries management, including metacoupled governance of relationships among sending, receiving, and spillover systems rather than specific issues and physical places alone. The metacoupling framework also has substantial flexibility because its systematic structure can be widely applied to disparate fisheries to provide social-ecological insights that advance fisheries science and practice. Future research directions include better quantifying metacoupled flows of water, information, fish, people, and money within and beyond Twin and Chippewa creeks and mathematically modeling metacouplings– particularly interactions among intracouplings, pericouplings, and telecouplings– via time series approaches, agent-based models, network analyses, and related methods (Liu, 2017; Carlson et al., 2018; Dou et al., 2019). In addition, future metacoupling research is needed on topics not directly studied herein, but which are important for salmonid and broader fisheries management within and beyond our study area (e.g., watershed fragmentation, invasive species, stocking, pollution, fish community interactions, biodiversity conservation; Cooper et al., 2016; Zorn et al., 2018; Smith et al., 2019). Overall, the ecological dynamics, socioeconomic benefits, and public fascination associated with stream salmonids are best understood and managed by conceptualizing salmonid fisheries as metacoupled CHANS.
Data Availability Statement
All datasets generated for this study are included in the article/Supplementary Material.
Ethics Statement
The studies involving human participants were reviewed and approved by Michigan State University (MSU) Institutional Review Board. The participants provided their written informed consent to participate in this study.
Author Contributions
AC conceived the research idea, analyzed the angler survey research, and wrote the majority of the manuscript. WT helped conceive the research idea and provided text in addition to thoughtful comments and revisions. SH performed surveys of groundwater stakeholders on which this manuscript is based and provided thoughtful comments and revisions.
Funding
Funding was provided by the University Distinguished Fellowship [Michigan State University (MSU)], the MSU Graduate School, the MSU Department of Fisheries and Wildlife, the Michigan Department of Natural Resources, the Robert C. Ball and Betty A. Ball Fisheries and Wildlife Fellowship, the Red Cedar Fly Fishers Graduate Fellowship, the Fly Fishers International Conservation Scholarship, the Schrems Fellowship awarded by the West Michigan Chapter of Trout Unlimited, and the George L. Disborough Grant awarded by the Kalamazoo Chapter of Trout Unlimited. Funding in support of this research was also provided by Princeton University’s Dean For Research, Princeton Environmental Institute, Andlinger Center for Energy and the Environment, and the Office of the Provost. Special thanks to D. I. Rubenstein and S. A. Levin.
Conflict of Interest
The authors declare that the research was conducted in the absence of any commercial or financial relationships that could be construed as a potential conflict of interest.
Acknowledgments
We thank T. Hein, R. Schinegger, G. Weigelhofer, D. Infante, and J. Schoelynck for coordinating the Challenges and Innovative Solutions in River Sciences section of Frontiers in Environmental Science. We thank S. Li, H. Abbas, B. Northcott, D. Infante, C. Andrews, R. Goodwin, N. Weil, and Malcolm Pirnie, Inc. for research assistance on the groundwater modeling component of this study. AC thanks T. Zorn, D. Hayes, E. Walter, J. Dexter, D. Infante, J. Mistak, G. Whelan, S. Winterstein, T. Cwalinski, N. Godby, B. Gunderman, P. Schneeberger, M. Tonello, S. Knoche, J. Simoes, and F. Lupi for guidance and support in research leading to this manuscript. SH thanks T. Dietz and J. Rose for research assistance on the groundwater stakeholder surveys necessary for this study.
Supplementary Material
The Supplementary Material for this article can be found online at: https://www.frontiersin.org/articles/10.3389/fenvs.2020.00027/full#supplementary-material
References
Advanced Ecological Management [AEM] (2016). White Pine Springs: Evaluation of Fish, Macroinvertcbrates, and Aquatic Habitat Resulting from an Increase in Groundwater Withdrawal. Reed City, MI: Advanced Ecological Management.
Anderson, D. (2016). Economic Impact of Recreational Trout Angling in the Driftless Area. La Crosse, WI: University of Wisconsin—La Crosse.
Ansell, C., and Gash, A. (2008). Collaborative governance in theory and practice. J. Publ. Adm. Res. Theor.18, 543–571. doi: 10.1093/jopart/mum03
Baranoff, F. I. (1918). On the question of the biological basis of fisheries. Nauchnge Issledovaniya Ikhtiologicheskii Instituta Izvestiya1, 81–128.
Bartholow, J. M. (2002). SSTEMP for Windows: The Stream Segment Temperature Model (Version 2). U.S. Geological Survey Computer Model and Documentation. Available online at: https://www.sciencebase.gov/catalog/item/4f4e4b16e4b07f02db6a5271 (accessed October 23, 2019).
Bennett, A., Patil, P., Kleisner, K., Rader, D., Virdin, J., and Basurto, X. (2018). Contribution of Fisheries to Food and Nutrition Security: Current Knowledge, Policy, and Research. Durham, NC: Duke University, Nicholas Institute for Environmental Policy Solutions. Report 18-02.
Budy, P., Thiede, G. P., Lobón-Cerviá, J., Fernandez, G., McHugh, P., McIntosh, A., et al. (2013). Limitation and facilitation of one of the world’s most invasive fish: an intercontinental comparison. Ecology94, 356–367. doi: 10.1890/12-0628.1
Carlson, A. K., French, W. E., Vondracek, B., Ferrington, L. C.Jr., Mazack, J. E., et al. (2016). Brown trout growth as related to landscape and local factors in Minnesota streams. J. Freshw. Ecol.31, 421–429. doi: 10.1080/02705060.2016.1160449
Carlson, A. K., Taylor, W. W., Kinnison, M. T., Sullivan, S. M. P., Weber, M. J., Melstrom, R. T., et al. (2019a). Threats to freshwater fisheries in the United States: perspectives and investments of state fisheries administrators and agricultural experiment station directors. Fisheries44, 276–287. doi: 10.1002/fsh.10238
Carlson, A. K., Taylor, W. W., and Liu, J. (2019b). Using the telecoupling framework to improve Great Lakes fisheries sustainability. Aquat. Ecosyst. Health.22, 342–354. doi: 10.1080/14634988.2019.1668660
Carlson, A. K., Taylor, W. W., Hartikainen, K. M., Infante, D. M., and Beard, T. D.Jr., et al. (2017a). Comparing stream-specific to generalized temperature models to guide salmonid management in a changing climate. Rev. Fish. Biol. Fisher.27, 443–462. doi: 10.1007/s11160-017-9467-0
Carlson, A. K., Taylor, W. W., Liu, J., and Orlic, I. (2017b). The telecoupling framework: an integrative tool for enhancing fisheries management. Fisheries42, 395–397. doi: 10.1080/03632415.2017.1342491
Carlson, A. K., Taylor, W. W., Schlee, K. M., Zorn, T. G., and Infante, D. M. (2017c). Projected impacts of climate change on stream salmonids with implications for resilience-based management. Ecol. Freshw. Fish.26, 190–204. doi: 10.1111/eff.12267
Carlson, A. K., Taylor, W. W., Liu, J., and Orlic, I. (2018). Peruvian anchoveta as a telecoupled fisheries system. Ecol. Soc.23:35. doi: 10.5751/ES-09923-230135
Carlson, A. K., and Zorn, T. G. (2018). Values, Opinions, and Behavior of Inland Trout Anglers in Michigan. Lansing, MI: Michigan Department of Natural Resources. Fisheries Report 29.
Cooke, S. J., Twardek, W. M., Lennox, R. J., Zolderdo, A. J., Bower, S. D., Gutowsky, L. F. G., et al. (2017). The nexus of fun and nutrition: recreational fishing is also about food. Fish. Fish.19, 201–224. doi: 10.1111/faf.12246
Cooper, A. R., Infante, D. M., Wehrly, K. M., Wang, L., and Brenden, T. O. (2016). Identifying indicators and quantifying large-scale effects of dams on fishes. Ecol. Indic.61, 646–657. doi: 10.1016/j.ecolind.2015.10.016
Department of Environment Great Lakes and Energy [DEGLE] (2017). Nestlé Permit Overview. Lansing, MI: Department of Environment, Great Lakes, and Energy.
Dietz, T., Ostrom, E., and Stern, P. C. (2003). The struggle to govern the commons. Science302, 1907–1912. doi: 10.1126/science.1091015
Dou, Y., Millington, J. A., Bicudo, Da Silva, R. F., McCord, P., Viña, A., et al. (2019). Land-use changes across distant places: design of a telecoupled agent-based model. J. Land. Use. Sci.14, 191–209. doi: 10.1080/1747423X.2019.1687769
Dreher, A., Gaston, N., and Martens, P. (2008). Measuring Globalisation: Gauging its Consequences. New York, NY: Springer, doi: 10.1007/978-0-387-74069-0
Food and Agriculture Organization [FAO] (2018). The State of World Fisheries and Aquaculture: Meeting the Sustainable Development Goals. Rome: Food and Agriculture Organization of the United Nations.
Fausch, K. D., Lyons, J., Karr, J. R., and Angermeier, P. L. (1990). Fish communities as indicators of environmental degradation. Am. Fish. Soc. Symp.8, 123–144.
Fausch, K. D., and White, R. J. (1981). Competition between brook trout (Salvelinus fontinalis) and brown trout (Salmo trutta) for positions in a Michigan stream. Can. J. Fish. Aquat. Sci.38, 1220–1227. doi: 10.1139/f81-164
Fenske, J. L. (1983). Attitudes and Attributes of Anglers Who Fish for Trout in Michigan. Ann Arbor, MI: Michigan Department of Natural Resources. Fisheries Research Report 1916.
Frisch, A. J., Ireland, M., and Baker, R. (2014). Trophic ecology of large predatory reef fishes: energy pathways, trophic level, and implications for fisheries in a changing climate. Mar. Biol.161, 61–73. doi: 10.1007/s00227-013-2315-4
Gartner, W. C., Love, L. L., Erkkila, D., and Fulton, D. C. (2002). Economic Impact and Social Benefits Study of Coldwater Angling in Minnesota. St. Paul, MN: University of Minnesota Extension Service.
Hilborn, R., Stokes, K., Maguire, J.-J., Smith, T., Botsford, L. W., Mangel, M., et al. (2004). When can marine reserves improve fisheries management?Ocean Coast. Manage.47, 197–205. doi: 10.1016/j.ocecoaman.2004.04.001
Hubert, W. A., and Quist, M. C. (2010). Inland Fisheries Management in North America, 3rd Edn. Bethesda, MA: American Fisheries Society.
Hughes, S. M. (2006). The Changing Value of Water: the Conundrum for Future Water Managers. Master’s thesis. Lansing, MI: Michigan State University.
Hulina, J., Bocetti, C., Campa, H.III, Hull, V., Yang, W., and Liu, J. (2017). Telecoupling framework for research on migratory species in the Anthropocene. Elementa: Sci. Anthrop.5:5. doi: 10.1525/elementa.184
Knuth, B. A. (2002). “The many faces of salmon: implications of stakeholder diversity in the Great Lakes,” in Sustaining North American salmon: Perspectives Across Regions and Disciplines, eds K. D. Lynch, M. L. Jones, and W. W. Taylor (Bethesda, MD: American Fisheries Society), 181–192.
Li, B.-D., Zhang, X.-H., Xu, C.-Y., Zhang, H., and Song, J.-X. (2015). Water balance between surface water and groundwater in the withdrawal process: a case study of the Osceola watershed. Hydrol. Res.46, 943–953. doi: 10.2166/nh.2015.137
Li, S., and Liu, Q. (2006). Interactive Ground Water (IGW). Environ. Modell. Softw.21, 417–418. doi: 10.1016/j.envsoft.2005.05.010
Liebich, K. B., Kocik, J. F., and Taylor, W. W. (2018). Reclaiming a space for diadromous fish in the public psyche and sense of place. Fisheries43, 231–240. doi: 10.1002/fsh.10063
Liu, J. (2017). Integration across a metacoupled world. Ecol. Soc.22, 29. doi: 10.5751/ES-09830-220429
Liu, J. (2018). An integrated framework for achieving sustainable development goals around the world. Ecol. Econ. Soc.1, 11–17. doi: 10.5751/ES-07082-1904-49
Liu, J., Hull, V., Luo, J., Yang, W., Liu, W., Viña, A., et al. (2015). Multiple telecouplings and their complex interrelationships. Ecol. Soc.20:44. doi: 10.5751/ES-07868-200344
Liu, J., Viña, A., Yang, W., Li, S., Xu, W., and Zheng, H. (2018). China’s environment on a metacoupled planet. Annu. Rev. Env. Resour.43, 1–34. doi: 10.1146/annurev-environ-102017-030040
Liu, J. G., Hull, V., Batistella, M., DeFries, R., Dietz, T., Fu, F., et al. (2013). Framing sustainability in a telecoupled world. Ecol. Soc.18, 26. doi: 10.5751/ES-05873-180226
Lynch, K. D., Jones, M. L., and Taylor, W. W. (2002). Sustaining North American Salmon: Perspectives Across Regions and Disciplines. Bethesda, MA: American Fisheries Society.
Lyons, J., Zorn, T., Stewart, J., Seelbach, P., Wehrly, K., and Wang, L. (2009). Defining and characterizing coolwater streams and their fish assemblages in Michigan and Wisconsin, USA. N. Am. J. Fish. Manage.29, 1130–1151. doi: 10.1577/M08-118.1
Malcolm Pirnie Inc. [MPI] (2000). Hydrogeologic Assessment Technical Memorandum, Volume I of III Report, Spring Hill Camp Study Area, Osceola County, Michigan. East Lansing, MI: Malcolm Pirnie Inc.
McIntyre, P. B., Jones, L. E., Flecker, A. S., and Vanni, M. J. (2007). Fish extinctions alter nutrient recycling in tropical freshwaters. Proc. Natl. Acad. Sci. U.S.A.104, 4461–4466. doi: 10.1073/pnas.0608148104
Michigan Department of Natural Resources [MDNR] (2015). Cheboygan River Assessment. Lansing, MI: Michigan Department of Natural Resources.
Michigan Department of Natural Resources [MDNR] (2019). Chippewa and Twin Creek Survey Report, Osceola County, Michigan. Lansing, MI: Michigan Department of Natural Resources.
Moyle, P. B., and Moyle, M. A. (1991). Introduction to fish imagery in art. Environ. Biol. Fish.31, 5–23. doi: 10.1007/BF00002153
Myers, B. J. E., Lynch, A. J., Bunnell, D. B., Chu, C., Falke, J. A., Kovach, R. P., et al. (2017). Global synthesis of the documented and projected effects of climate change on inland fishes. Rev. Fish Biol. Fisher.27, 339–361. doi: 10.1007/s11160-017-9476-z
O’Neal, R. P. (1997). Muskegon River Watershed Assessment. Ann Arbor, MI: Michigan Department of Natural Resources.
Österblom, H., and Folke, C. (2015). Globalization, marine regime shifts and the Soviet Union. Philos. T. Roy. Soc. B370, 20130278. doi: 10.1098/rstb.2013.0278
Papadopulos & Associates, Inc (2016). Evaluation of Groundwater and Surface Water Conditions in the Vicinity of Well PW-101, Osceola County, Michigan. Bethesda, MA: S. S. Papadopulos & Associates.
Petchenik, J. (2014). Trout Fishing in Wisconsin: Angler Behavior, Program Assessment And Regulation And Season Preferences. Madison, WI: Wisconsin Department of Natural Resources, Bureau of Science Services.
Pinsky, M. L., and Fogarty, M. (2012). Lagged social-ecological responses to climate and range shifts in fisheries. Clim. Change115, 883–891. doi: 10.1007/s10584-012-0599-x
Public Sector Consultants [PSC] (2017). Economic Contribution of NWNA to Michigan: Statewide, Regional, and Local Contribution. Lansing, MI: Public Sector Consultants.
Quinn, T. P. (2005). The Behavior and Ecology of Pacific Salmon and Trout. Seattle, WA: TheUniversity of Washington Press.
Raleigh, R. F. (1982). Habitat Suitability Index Models: Brook Trout. Washington, D.C: U.S. Fish and Wildlife Service. Biological Report Number 82.
Raleigh, R. F., Zuckerman, L. D., and Nelson, P. C. (1986). Habitat Suitability Index Models and Instream Flow Suitability Curves: Brown Trout. Washington, D.C: U.S. Fish and Wildlife Service. Biological Report Number 82.
Responsive Management (2008). Pennsylvania Trout Fishing Survey. Conducted for the Pennsylvania Fish and Boat Commission. Harrisonburg, VA: Responsive Management.
Ricker, W. E. (1954). Stock and recruitment. J. Fish. Res. Board. Can.11, 559–623. doi: 10.1139/f54-039
Roni, P., Hanson, K., and Beechie, T. (2008). Global review of the physical and biological effectiveness of stream habitat rehabilitation techniques. N. Am. J. Fish. Manag.28, 856–890. doi: 10.1577/M06-169.1
Smith, K. R., Roth, B. M., Jones, M. L., Hayes, D. B., Herbst, S. J., and Popoff, N. (2019). Changes in the distribution of Michigan crayfishes and the influence of invasive rusty crayfish (Faxonius rusticus) on native crayfish substrate associations. Biol. Invasions.21, 637–656. doi: 10.1007/s10530-018-1852-7
Southwick Associates (2012). Sportfishing in America: An Economic Force for Conservation. Alexandria, VA: American Sportfishing Association.
Spens, J., Alanärä, A., and Eriksson, L.-O. (2007). Nonnative brook trout (Salvelinus fontinalis) and the demise of native brown trout (Salmo trutta) in northern boreal lakes: stealthy, long-term patterns?Can. J. Fish. Aquat. Sci.64, 654–664. doi: 10.1139/f07-040
Sun, J., Tong, Y., and Liu, J. (2017). Telecoupled land-use changes in distant countries. J. Integr. Agr.16, 368–376. doi: 10.1016/S2095-3119(16)61528-9
Tapia-Lewin, S., Vergara, K., De La Barra, C., Godoy, N., Castilla, J. C., and Gelcich, S. (2017). Distal impacts of aquarium trade: exploring the emerging sandhopper (Orchestoidea tuberculata) artisanal shore gathering fishery in Chile. Ambio46, 706–716. doi: 10.1007/s13280-017-0906-x
Taylor, W. W., Leonard, N. J., Kratzer, J. F., Goddard, C., and Stewart, P. (2007). “Globalization: implications for fish, fisheries, and their management,” in Globalization: Effects on Fisheries Resources, eds W. W. Taylor, M. S. Schechter, and L. G. Wolfson (New York, NY: Cambridge University Press), 21–46. doi: 10.1017/CBO9780511542183
Townsend, C. R. (1996). Invasion biology and ecological impacts of brown trout Salmo trutta in New Zealand. Biol. Conserv.78, 13–22. doi: 10.1016/0006-3207(96)00014-6
U. S. Geological Survey. [USGS] (2020a). Data from: USGS 04121494 Chippewa Creek at 90th Avenue near Evart. Evart MI: USGS.
U. S. Geological Survey. [USGS] (2020b). Data from: USGS 04121507 Twin Creek at Eight Mile Road near Evart. Evart, MI: USGS.
U.S. Fish and Wildlife Service [UWFWS] (2013). 2011 National Survey of Fishing, Hunting, And Wildlife-Associated Recreation—Michigan. Washington, D.C: U.S. Department of the Interior.
Vannote, R. L., Minshall, G. W., Cummins, K. W., Sedell, J. R., and Cushing, C. E. (1980). The river continuum concept. Can. J. Fish. Aquat. Sci.37, 130–137. doi: 10.1139/f80-017
Waco, K. E. (2009). The Influence of Groundwater Withdrawal on Available Brook Charr (Salvelinus fontinalis) Thermal Habitat in Twin and Chippewa creeks, Osceola County, Michigan. Master’s thesis, Michigan State University, East Lansing, MI.
Waco, K. E., and Taylor, W. W. (2010). The influence of groundwater withdrawal and land use changes on brook charr (Salvelinus fontinalis) thermal habitat in two coldwater tributaries in Michigan. U.S.A. Hydrobiol.650, 101–116. doi: 10.1007/s10750-010-0204-0
Weithman, A. S., and Haas, M. A. (1982). Socioeconomic value of the trout fishery in Lake Taneycomo, Missouri. T. Am. Fish. Soc.111, 223–230. doi: 10.1577/1548-86591982111¡223:SVOTTF¡2.0.CO;2
Wesener, M. (2010). Biological Assessment of the Middle Muskegon River Watershed, Montcalm, Mecosta, Osceola, and Clare Counties, Michigan, July-September 2006. Lansing, MI: Michigan Department of Natural Resources and Environment, Water Bureau. MDEQ Report No. MI/DNRE/WB-10/013.
Wills, T. C., Zorn, T. G., and Nuhfer, A. J. (2006). “Stream status and trends program sampling protocols,” in Manual of Fisheries Survey Methods II: with Periodic Updates, ed. J. C. Schneider (Ann Arbor, MI: Michigan Department of Natural Resources), 1–49.
Wilson, J. A. (2006). Matching social and ecological systems in complex ocean fisheries. Ecol. Soc.11:9. doi: 10.5751/ES-01628-110109
Zorn, T. G. (2018). “Ecology and management of stream-resident brown trout in Michigan,” (USA),” in Brown Trout: Biology, Ecology and Management (First Edition), eds J.Lobón-Cerviá and N. Sanz (Hoboken, NJ: John Wiley & Sons Ltd), 667–696. doi: 10.1002/9781119268352.ch27
Keywords: brook charr, brown trout, metacoupling framework, coupled human and natural systems, salmonid management
Citation: Carlson AK, Taylor WW and Hughes SM (2020) The Metacoupling Framework Informs Stream Salmonid Management and Governance. Front. Environ. Sci. 8:27. doi: 10.3389/fenvs.2020.00027
Received: 30 November 2019; Accepted: 25 February 2020;
Published: 11 March 2020.
Edited by:
Rafaela Schinegger, University of Natural Resources and Life Sciences Vienna, AustriaReviewed by:
Maria Alp, National Research Institute of Science and Technology for Environment and Agriculture (IRSTEA), FranceKentaro Morita, Japan Fisheries Research and Education Agency (FRA), Japan
Douglas Joseph Dieterman, Minnesota Department of Natural Resources, United States
Copyright © 2020 Carlson, Taylor and Hughes. This is an open-access article distributed under the terms of the Creative Commons Attribution License (CC BY). The use, distribution or reproduction in other forums is permitted, provided the original author(s) and the copyright owner(s) are credited and that the original publication in this journal is cited, in accordance with accepted academic practice. No use, distribution or reproduction is permitted which does not comply with these terms.
*Correspondence: Andrew K. Carlson, andrewkc@princeton.edu; andrewcarlson422@gmail.com
†Present address: Andrew K. Carlson, Princeton Environmental Institute, Princeton University, Princeton, NJ, United States