- 1Laboratoire Chrono-Environnement, UMR CNRS 6249, Université Bourgogne Franche-Comté, Besançon, France
- 2Laboratoire Chrono-Environnement, UMR CNRS 6249, Université Bourgogne Franche-Comté, Montbéliard, France
- 3Faculté des Sciences et Technologies, Université de Lorraine, Nancy, France
The increasing production of wastes that are landfilled might contribute to sources of potentially toxic elements; this is the case of residual red gypsum tailings, a by-product of titanium dioxide extraction. Revegetation of such a site is essential, and Mn phytoextraction may render the operations economically profitable. This study aimed to apply phytomanagement techniques for increasing the plant development, tailings revegetation and an optimal Mn phytoextraction using silver birch, the most abundant plant species on this site. To enhance the nutrient availability from the tailings, amendments that reduce the pH, i.e., pine bark chips, Miscanthus straw, white peat, and ericaceous compost, were mixed with residual red gypsum and birches were allowed to grow for 3 months. The pine bark chips and ericaceous compost led to a maximum decrease in pH, allowing the accumulation of up to 1400 mg Mn kg–1 dry matter in the leaves silver birch leaves. However, some nutrient competition was found in the pine bark treatment, which halved biomass production as compared to control. Further amendment addition may be needed to take advantage of the pine bark capabilities as a soil conditioner and Mn solubilizing treatment in residual red gypsum.
Introduction
The global population surpassed 7 billion inhabitants by January 2016, increasing concerns about food security, climate change, public health, resource extraction, and waste recycling (Abdulkadyrova et al., 2016). Waste generation and its management is currently of major importance (European Commission [EC], 2015). Total waste production includes waste produced in manufacturing, mineral extraction, and households, with some of these main waste sources in Europe reaching up to 2500 million tons (Eurostat, 2016) and becoming landfill waste. The number of potentially contaminated sites in Europe is as high as 3 million (EEA, 2007), and this number could eventually increase with the increase in the population and industry. Industrial landfills may contain mine slags and tailings that generally contain potentially toxic elements (PTEs) that affect organisms differently (Kurt-Karakus, 2012). The urbanization near landfill areas may increase population exposure to PTEs by dust inhalation, ingestion of home-grown vegetables (Assad et al., 2019), and dermal contact with contaminated substrates (Jiang et al., 2019). Additionally, tree development in mining soils with high rhizosphere pH, i.e., from the bauxite extraction or coal mining industries, may be affected by a nutrient deficiency, rather than by soil metal(loid)s in excess (Zhang and Zwiazek, 2016).
Management and remediation of industrial residues usually imply engineering technologies, although these are often disregarded due to the unappealing cost-effectiveness of operations and further environmental impact (Ciadamidaro et al., 2019). Alternatively, phytomanagement could be employed, through the combination of chemical, biological or the use of organic amendments, for the reclamation of contaminated sites or extraction of certain PTEs (Robinson et al., 2009). A previous study (Hueso-González et al., 2017) used straw and pine chips for mulching and mixing with the soils to improve water retention and therefore increase plant survival rate in dry environments. These organic residues were selected due to their low additional cost for reforestation and for the services they offered to the ecosystem (e.g., improvement of aggregate stability, aeration, and hydraulic conductivity) (Hueso-González et al., 2018). Peat mineral mix was also used as a soil cover for tailings and mine overburden to improve the availability of micronutrients for Pinus contorta and Picea glauca (Manimel Wadu and Chang, 2017). In a field experiment carried out in a gold mining tailings in Southeastern Manitoba (Canada), papermill sludge and woodchips were used to improve substrate aggregation and organic content. The use of these substrates in low quantities (<5.6 t ha–1) improved directly the physico-chemical soil conditions and consequently the biological activity without altering the pH (Young et al., 2015).
Previous studies on the residual red gypsum (RRG) of the Ochsenfeld site, in Eastern France, showed that the harshness and nutrient deficiencies of the substrate somehow restrained plant colonization (Zappelini et al., 2018). The absence of a vegetation cover on such mine tailings may represent a health concern for the villagers and settlements surrounding the site. Managing the Ochsenfeld site by following the Green Chemistry principles would allow this RRG site to be returned to a productive area, since RRG has abundant presence of some metals of commercial interest (Zapata-Carbonell et al., 2019), where the raw material can be exploited for secondary uses. In this regard, the production of commercial glazes for ceramic production (Kamarudin and Zakaria, 2007) and the production of cement as a binder (Hughes et al., 2011; Gázquez et al., 2014) are some of the main uses for RRG. Moreover, given recent chemical studies, RRG might also be used as raw material for the synthesis of other compounds, such as catalysts (Grison et al., 2015; Deng et al., 2016).
Silver birch (Betula pendula), which is naturally occurring and dominates the vegetation cover at the RRG Ochsenfeld site (Zapata-Carbonell et al., 2019), showed tolerance and Mn accumulation rates that are close to those found in other Betula species (Ciadamidaro et al., 2019). This colonist would be a suitable species for the phytomanagement of Mn-contaminated sites. As it is well known, the chemical speciation and solubility of nutrients and trace elements is mainly driven by the pH, and thus, we hypothesized that a reduction in pH of the RRG, currently at 7.8, would render the nutrients more readily available, which will improve the plant development and hence revegetation. This work aims to demonstrate the hypothesis that amending the RRG with commercial organic amendments known for lowering soil pH, i.e., ericaceous compost, white peat, pine bark chips, and Miscanthus straw, will: (1) increase growth of the silver birch by allowing the revegetation of this particular contaminated site and (2) enhance the amount of Mn taken up by the tree roots and, hence, its accumulation in harvestable plant parts for possible valorization.
Materials and Methods
Nature of Substrate and Origins
The substrate used was RRG, the product of the neutralization of the sulfuric TiO2 extraction effluent, and the landfill is located at the Ochsenfeld site (47.79686 N, 7.132775 E), near Thann in Eastern France. The RRG has been in situ for over a decade and contains abundant concentrations of Ca, S, Fe, Mn, and Mg (approximately 200000, 110000, 650000, 5000, and 3500 mg kg–1 DW, respectively) (Assad et al., 2017; Zapata-Carbonell et al., 2019). We also found other essential elements such as Co, Cu, K, Na, P, S, Si, and Zn, or other PTEs such as Cr, Cd, or Ni. However, for most of the PTEs, we did not register higher accumulation of these elements in the edible parts of crops or in poplar leaves grown on red gypsum compared with the control soil in a previous experiment (Assad et al., 2017), except for Cr for which we indeed found higher amounts in poplar leaves.
Tree Production
Betula pendula seeds were collected from the Thann site in 2017 and used in all subsequent experiments. Seeds were sown on peat (Brill Typical, Germany), and seedlings were grown for 3 months in a culture chamber with a 16 h, 23°C and 8 h, 21°C day/night regime, with an average moisture of 70% and a daylight intensity of approximately 600 μmol photons photosynthetically actives m–2 s–1.
Experimental Setup
The RRG was collected at the Ochsenfeld site on a fully characterized area (Zapata-Carbonell et al., 2019) in February 2017. The amendments selected for lowering the pH were ericaceous compost (Ec) (Sorexto, France), white peat (Wp) (Hawita Baltic, Latvia), pine bark chips (Pc) (Eden Garden, France) and Miscanthus straw (Ms). Mixtures of substrates 1:1 (v/v) were homogenized and set up in 0.5 L pots (n = 12) using RRG, some 320 g DW of substrate, and each of the above amendments were applied in their crushed form. We selected the amendment doses, following recommendations found in previous studies where incorporation of feedstocks in similar proportions into a mine soil (Tandy et al., 2009) or a contaminated sediment (Mattei et al., 2017) were found to be efficient in promoting plant growth. The four treatments were as follows (1) ericaceous compost + red gypsum (Ec), (2) white peat + red gypsum (Wp), (3) pine bark + red gypsum (Pc), and (4) Miscanthus straw + red gypsum (Ms). Untreated RRG was used as a control treatment (Unt). Pots were placed on one plate per treatment to avoid leakage exchange among the treatments. One seedling of silver birch was planted per pot immediately after mixing the amendments in the pots and kept in the culture chamber mentioned above. Pots were watered from the bottom every 4 days in order to maintain a 23% of humidity as found in situ. The leaves and roots were harvested after 3 months, washed and rinsed with distilled water to avoid contamination, and then oven-dried at 70°C and weighed.
Monitoring and Sampling
The initial and final element concentrations (Al, Ca, Cr, Fe, K, Mn, Na, P, and S) were determined in substrates and leaf samples. Substrate samples were taken monthly in the shape of thin cores (5–10 mm in diameter) along the edges of the pots oven-dried at 40°C, ground and sieved through a 2 mm sieve to analyze and monitor changes in the pH and CaCl2 extractable fractions of the elements cited above. Similarly, the physiological response was assessed by measuring the relative chlorophyll content index (CCI) through a CCM-200 plus chlorophyll meter (Opti-Sciences, Hudson, NY, United States) using the third most developed leaf each time this parameter was measured in every plant for consistency.
Elemental Analysis
The substrate pH was determined using a Hach HQ40d pH meter (Colorado, United States) after 1:2.5 (w/v) parts of substrate in 1 M KCl had been shaken for 1 h (Hesse, 1971). Analysis of element concentrations in substrate extracts was performed using induced coupled plasma atomic emission spectrometry (ICP-AES, Thermo Fisher Scientific, Inc., Pittsburg, PA, United States). To determine the CaCl2 extractable fraction, 2.5 g of substrate were mixed with 25 mL of 0.01 M CaCl2 solution and incubated at room temperature for 3 h under agitation (140 rpm). This solution was finally filter through a Whatman 2 filter paper (Houba et al., 2000).
Initial total concentrations in the substrates and the ionome in the birch leaves were determined in wet-digested samples using either 0.5 g of substrate + 2 mL HNO3 + 5 mL HCl or 0.125 g of leaf DM + 1.75 mL HNO3 + 0.5 mL H2O2, respectively, in a digestion block (DigiPREP, SCP Sciences, Courtaboeuf, France) prior to the ICP-AES analysis (Assad et al., 2017). The calculated recovery percentages of the studied elements ranged from 89 to 156% for the substrates, and 60 to 101% for the leaves. The reference materials used were loamy clay soil (CRM052, LGC Promochem, Molsheim, France) and oriental basma tobacco leaves (INCT-OBTL-5, LGC Promochem, Molsheim, France) for the substrate and leaves, respectively.
For a better understanding of the soil-plant interaction and nutrient exchanges, bioconcentration factor (BCF), which integrates both soil and plant concentrations was computed for each element (Chopin and Alloway, 2007; Kabata-Pendias and Pendias, 2011). The bioconcentration factors were calculated with the foliar element concentration over the substrate element concentration, as explained by Takarina and Pin (2017). The phytoextracted Mn was calculated taking into account the Mn concentration accumulated in leaves (mg kg–1 DM) times the biomass produced (kg DM).
Statistical Analysis
Data obtained was processed with R (Ver. 3.4.2) packages FactoMineR (Lê et al., 2008), factoextra (Kassambara and Mundt, 2017), and agricolae (de Mendiburu, 2014), and the graphs were made with ggplot2 (Wickam, 2017). All tests were considered significant when p < 0.05. Analysis included two-way analysis of variance (ANOVA), with the Tukey-Kramer test for differences in the means used for group classification. For the CaCl2 extractable concentrations of Mn and Cr, data were log-transformed relative to the ANOVA postulates. Principal component analysis (PCA) were performed using the FactoMineR package to explain the distribution of the elements determined in the leaves and in the substrates among the treatments. Ellipses were drawn at the 95% confidence interval of the barycenter of each treatment. The results reported in the tables are presented in means ± standard deviation (SD).
Results
Amendment Influence on the Substrate Properties
Compared to the untreated substrate, the amended substrates displayed changes in physico-chemical parameters. First, changes in pH were found at the initial time (T0), with a decrease of up to one unit for the Pc soil. Few to no significant differences were determined for Wp, Ms and Ec soils. The differences remained similar during the experiment, with the highest pH observed for the Unt soil, followed by the Wp, Ec, and Ms soils, and the lowest pH being in the Pc soil. Throughout the experiment, the amendments tended to initially decrease the pH which was increased over time, including the Unt soil (Figure 1).
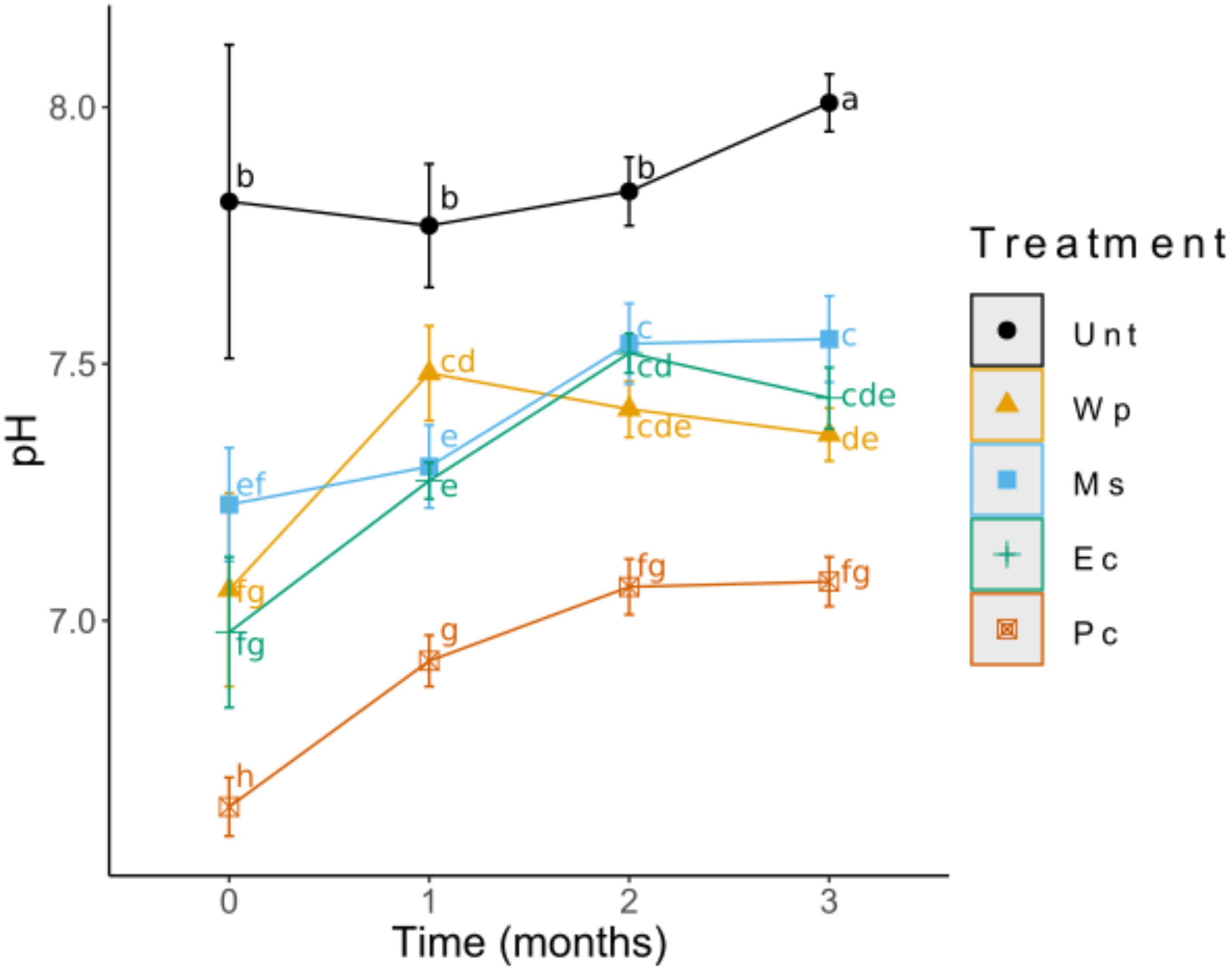
Figure 1. Changes in soil pH for each treatment during a 3-month growth period. Values shown (means ± SD) represent the results of 12 replicates.
A PCA was performed using only the soil total concentrations of elements (Supplementary Figure S1). This analysis shows the distribution of the elements among the treatments. The first component explained 69.4% of the variance, as most of the arrows, representing the elements determined by ICP-AES, were oriented in this axis toward the Unt soil (Al, Ca, Cr, Fe, Mg, Mn, and S) and on the opposite side toward Ec and Pc soils (P and K). On the other hand, the second component explained 13.3% of the variance, the variable that had more influence on this component was Na. Further changes noticed in the amended soils included changes in the solubility (CaCl2 extractable fraction) of some elements (Supplementary Table S1), notably Mn and Cr (Figure 2). The incorporation of ericaceous compost, white peat and pine bark chips decreased significantly the CaCl2 extractable Cr concentration and likely root exposure from T0 as compared to the Unt soil (Figure 2A). The use of Miscanthus straw induced a more progressive change in Cr extractability; however, equally different from the Unt soil. In contrast, Mn solubility significantly increased in the Pc, Ec, and Wp soils. Additionally, the highest Mn solubility occurred in the Pc soil at T0, reaching up to 16 mg Mn kg–1 soil DW (Figure 2B).
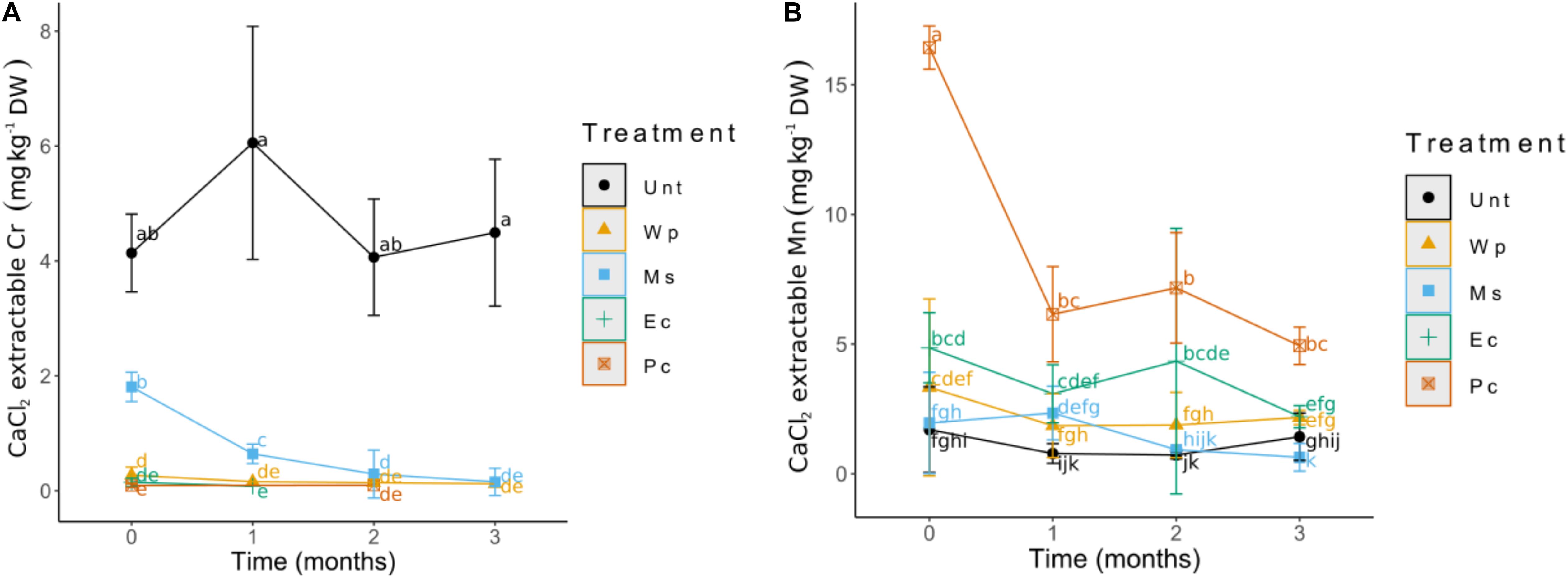
Figure 2. Changes in the CaCl2 extractable soil fractions of Cr (A) and Mn (B). Values shown represent (means ± SD) the results of 12 replicates.
Birch Growth
The plant answers somewhat differed across the treatments. At harvest, the mortality rate for plants growing in the Ec and Ms treatments was one individual in each treatment (8.3%), whereas no plants died in the Unt and Wp treatments. Finally, for Pc, the mortality rate was 25% (3 individuals).
The amendment effects on the biomass production at harvest are shown in Figure 3. Unexpectedly, the root biomass production was similar for the Unt, Wp, and Ec treatments, whereas the Pc plants significantly displayed a lower root biomass than the Unt plants (p < 0.01). The root biomass of the Ms plants did not differ from the other ones. As for the roots, leaf biomass production was similar for the Unt, Wp, and Ec plants. In contrast, the leaf biomass of the Pc and Ms plants were lower than that of the Unt plants.
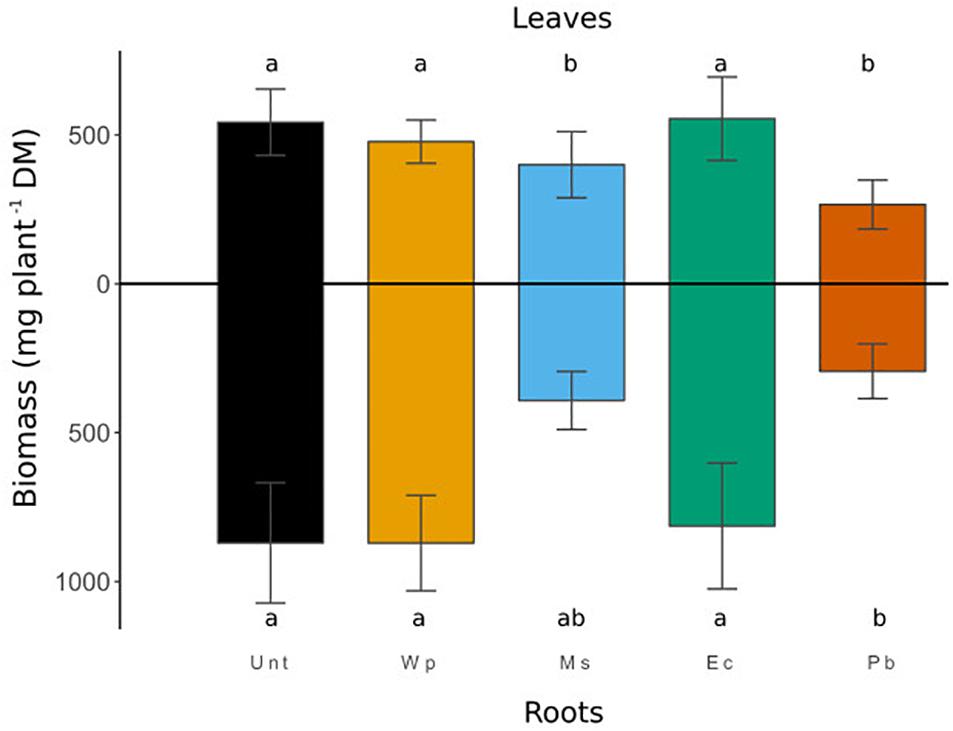
Figure 3. Root and leaf DM yields of birch plants (mg/plant) harvested after a 3-month growth period. Values shown represent (means ± SD) the results of 12 replicates. Identical letters indicate that no significant differences (p < 0.05) occurred in the birch biomass across the various treatments.
The CCI showed no significant differences across the treatments after 1 month; however, after 3 month, the Unt, Wp, and Ms birches showed similar chlorophyll values, whereas the Pc and Ec plants had significant lower chlorophyll values (Figure 4).
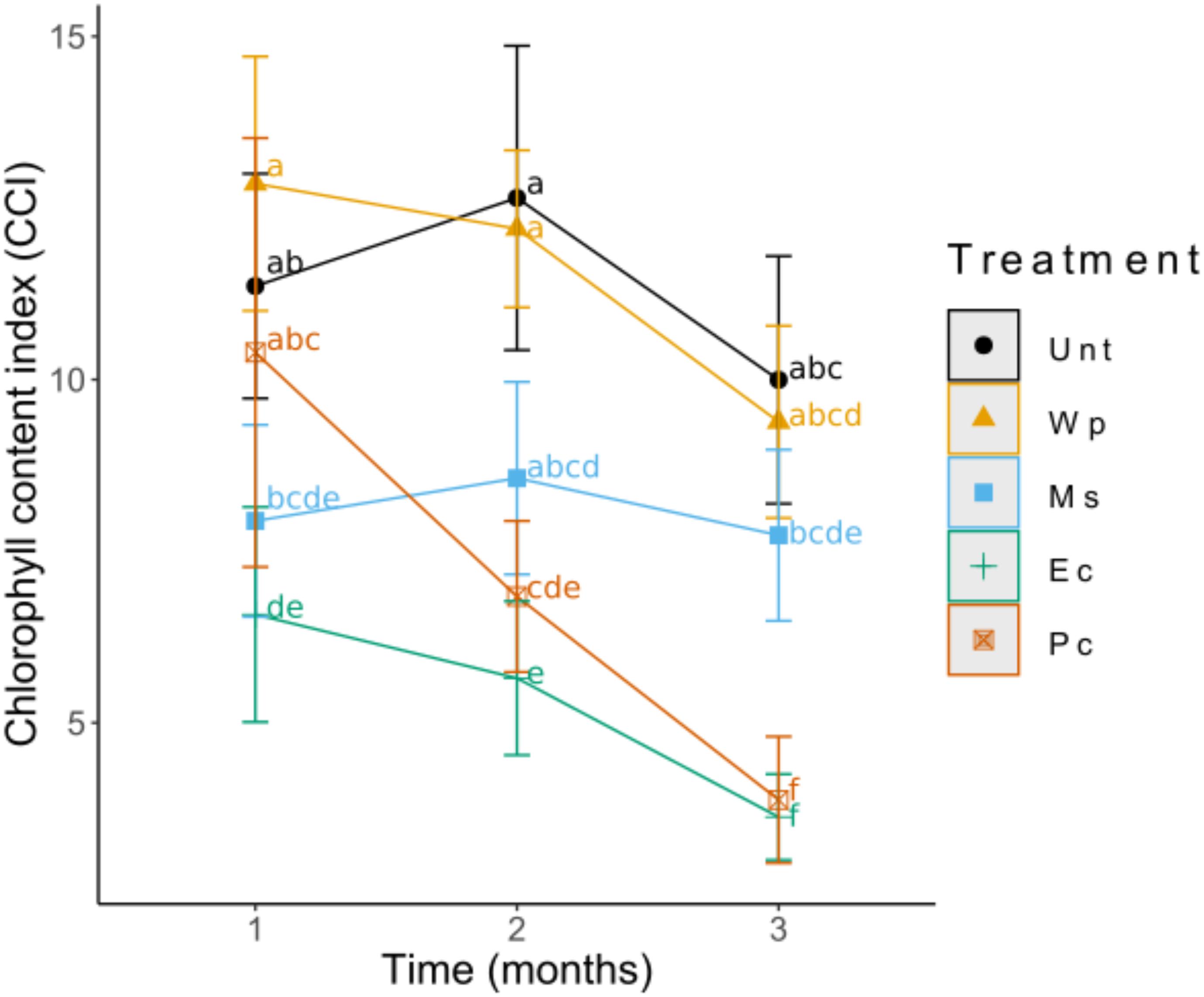
Figure 4. Changes in the chlorophyll index of birch leaves during the 3-month growth period. Analyses were performed using the 3rd most developed leaf of each treatment and measured by a CCM-200 plus chlorophyll meter. Values shown represent (means ± SD) the results of 12 replicates.
Foliar Ionome of Birch Plants
The elements determined in the leaves and the substrates are summarized in Table 1. The PCA based on the foliar ionomes and soil concentrations explained 43% of the variance and 12% in its first and second component, respectively (Figure 5). The barycenter of untreated RRG, Ec, and Pc were aligned with the first component, where total concentrations of S, Mg, Cr, Ca, Al, Mn, and Fe in soils, Cr and Mg in leaves were more abundant in the Unt direction and Mn, P in leaves and total concentrations of K and P in soil were most abundant in Ec and Pc. The second component was mostly influenced by Fe, Al, Na in leaves and on the opposite end K in leaves. Again, some leaf samples of Pc and Ms were characterized by high concentrations of the former group of elements, whereas some samples of Wp were characterized by the latter group.
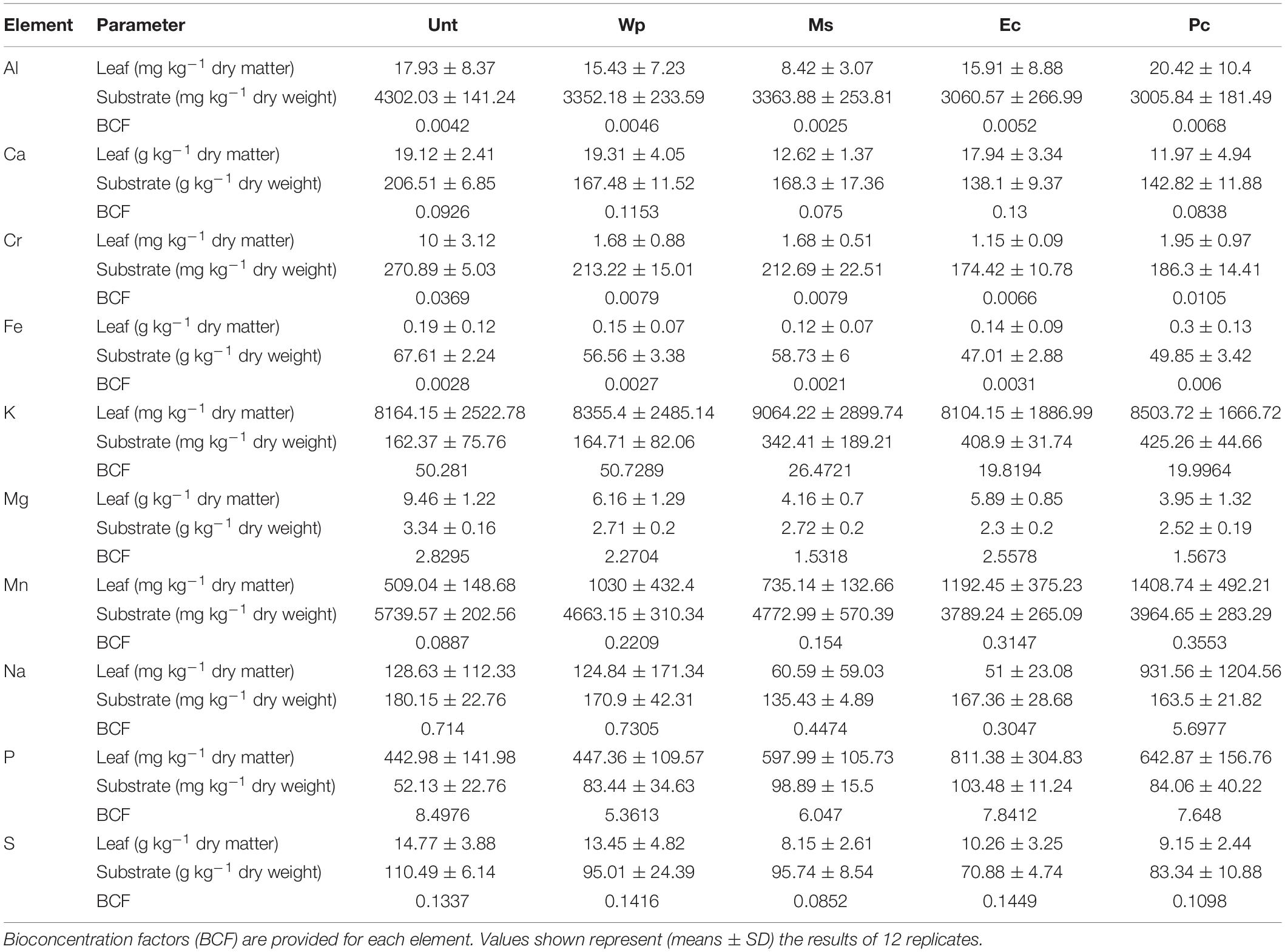
Table 1. Concentrations of elements in the RRG substrates and in leaves of birch seedlings under the five RRG treatments after a 3-month growth period.
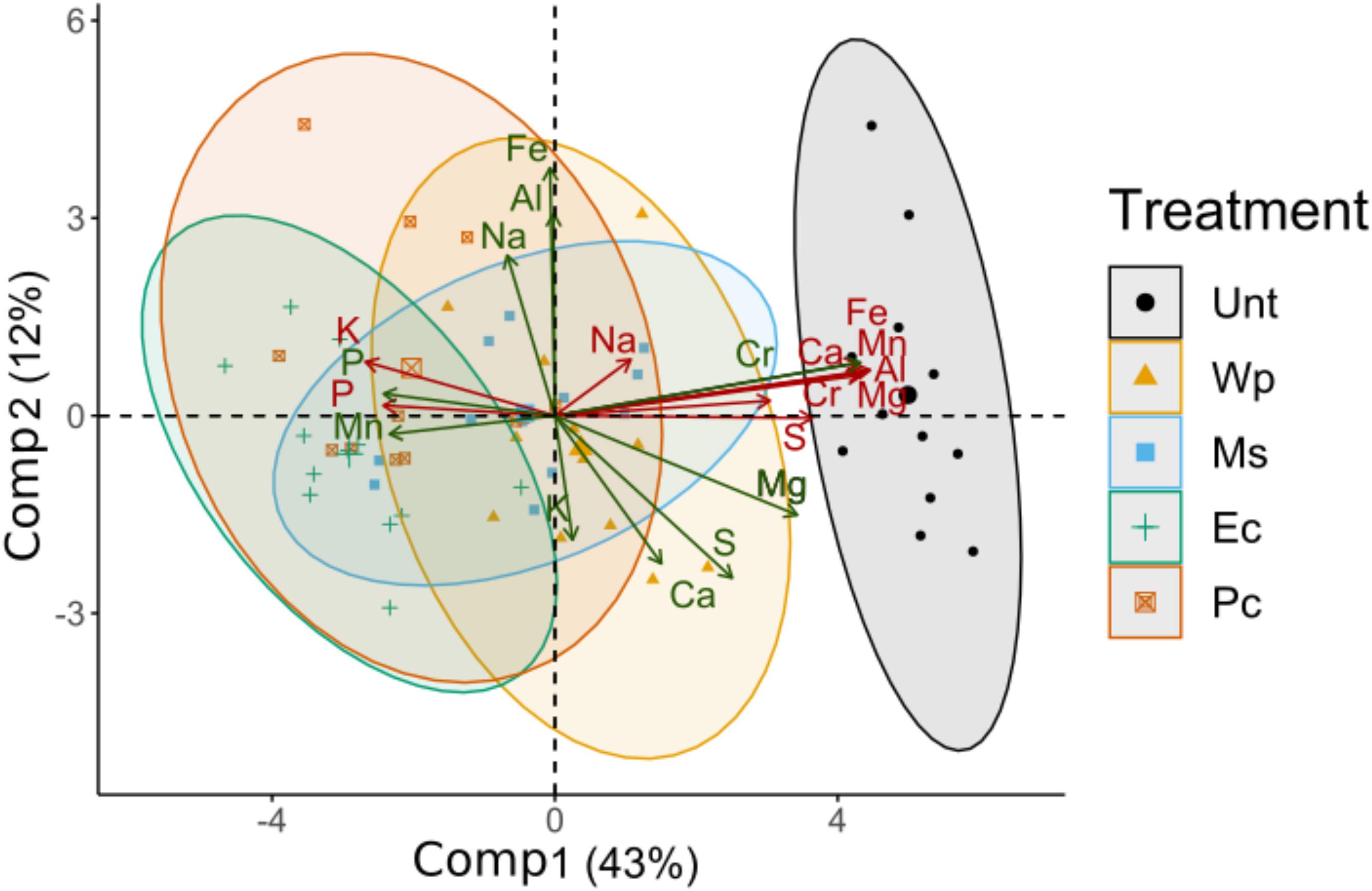
Figure 5. Principal component analysis (PCA) of the concentrations of elements determined in leaves (green) and the total concentrations of elements determined in the substrates at T0 (red). The largest symbols in the graphic represent the barycenter of each treatment. Ellipses represent the confidence intervals at 95%.
Some elements in foliar ionomes were influenced by the treatments. The foliar Cr concentration of plants from amended soils was 10-fold lower than that of the Unt plants. Foliar Mn concentration for the Wp and Ec plants exceeded that the Unt plants by two-fold, whereas in the Pc leaves, the Mn concentration was three-fold that of the Unt leaves. Foliar Mg concentrations for the Wp, Ms, Ec, and Pc plants were 0.3, 0.5, 0.5, and 0.6 times lower than that for the Unt plants, respectively. For foliar Na concentrations, Unt and Wp values were similar, Ms and Ec values were 0.5 times lower than the Unt ones whereas the Pc value increased 6 times as compared to the Unt one. Foliar P concentration for the Ec plants was two-fold higher than for the Unt ones.
The foliar Mn removal per plant (foliar Mn concentration x leaf biomass), and the analysis of variance indicated that Unt and Ms plants had similar foliar Mn removal, and the lowest values across the treatments. The highest foliar Mn removal was obtained in Ec. An intermediate group was formed by Pc and Wp (Figure 6).
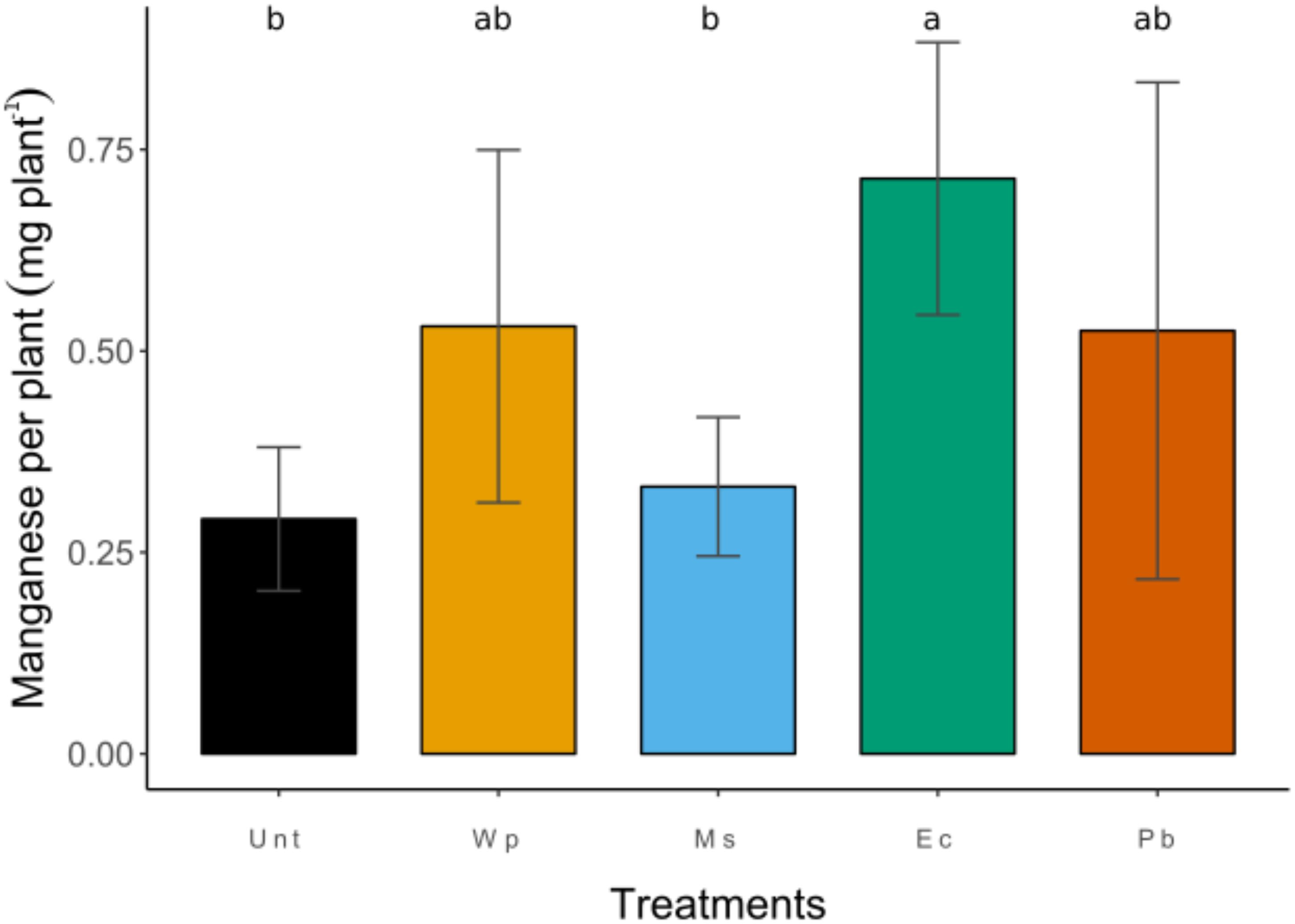
Figure 6. Amounts of Mn phytoextracted by birch biomass depending on soil treatments. Values shown represent (means ± SD) the results of 12 replicates. Identical letters indicate no significant differences (p < 0.05) across the treatments.
Discussion
The pH decrease occurring into the soils was expected, as all amendments were commercially known for their pH-decreasing properties, and on their own, the used amendments have a much lower pH themselves (Jackson et al., 2009). The progressive increase in soil pH found over the duration of the experiment was probably due to the presence of other salts in the RRG and the quality of the water used for irrigation, as reported by Al-Busaidi and Cookson (2003) in their alkaline substrates given the presence of sodium carbonates. In trials aiming at improving geranium and marigold growth on horticultural substrates, pine bark chips alone allowed to reach a pH of 4.5 (Jackson et al., 2009). Mixing peat with field soil in a strawberry-peat bioassay allowed for reaching a soil pH within the 4.5–4.8 range (de Tender et al., 2016). The pH values measured throughout the experiment would be acceptable for the plants in terms of tolerance since the pH for all treatments stabilized between 6.5 and 7.5.
Some changes occurred for the element solubility in the amended soils. The Mn solubility, for instance, may be affected by both pH and redox potential at a 6.5–7.5 pH, whereas at values of 5 or lower, the speciation will be mainly driven by pH (Gotoh and Patrick, 1972).
For Cr, all the amendments tested reduced significantly its solubility, a theoretically normal effect of acid pH (Takeno, 2005). This might be due to the binding properties of the dissolved organic matter and the presence of Fe contained in the added amendments, rending it undetectable (Fendorf, 1995).
In the case of Mn, oxidation states from II to VII are all possible, some bound to carbonates, silica and other forms of oxides and oxyhydroxides, although the soluble Mn2+ is the preferred form for plants (Alloway, 1995). In general, Mn speciation is regulated by the redox potential and pH, making Mn available to plants (II) under reducing conditions and acid pH or unavailable (>II) under oxidizing conditions and rather alkaline pH (Kabata-Pendias and Pendias, 2011). At pH values of 5.5 and higher, available Mn begins to decrease in the soil solution (van Winkle and Pullman, 2003). Furthermore, Mn may be absorbed either from the soil solution directly by the roots or can be solubilized after reduction in the rhizosphere (Reuter et al., 1988).
The determination of CCI is a commonly used parameter to assess, among essential physiological parameters, nitrogen uptake by plants (Lebourgeois et al., 2012). Chlorophyll measurements in seedlings from the Pc and Ms treatments showed a drop from the start. Since nitrogen is a macronutrient essential for plant growth, a lack of this element, reflected by the CCI, may be related in the low birch biomass produced compared to the other treatments. As reference, in uncontrolled conditions in the field, 4-month-old birch plants produced up to 17 g DM of leaves while using mineral fertilization and planted in a mixture of peat and sand (Kasurinen et al., 2012). At a similar age, the trees in our experiment growing in RRG barely reached 600 mg DM of leaves, demonstrating the presence of harsh conditions that the plants must endure. We have indeed evaluated that certain 5-year-old birches on the site measured less than 1 m in height. This also indicates the need for soil management to render nutrients more available to plants. The poor growth of plants in the Pc treatment could be related to the absence of N provided in the RRG (Zappelini et al., 2018), to the competition with microbes or by the possible but untested presence of allelopathic compounds that often characterize Pinus species (Prévosto and Ripert, 2008).
Moreover, the excess or lack of some elements in the leaves may involve health issues for plants. Mn is a micronutrient essential for various reactions in the cells (Millaleo et al., 2010); nevertheless, problems in plant development may occur with deficiency (10–20 mg kg–1 DM, Marschner, 2012) or excess (200–5300 mg kg–1 DM, Ducic and Polle, 2005). The tolerance to Mn in plants shall depend on adaptations and genotypes. Kitao et al. (2001) determined that, even when exposed to highly available concentrations, Betula platyphylla var. japonica reached up to 6300 mg Mn kg–1 DM in its leaves without showing signs of toxicity, thus our study only showing 1400 mg Mn kg–1 DM suggests that Mn toxicity levels were not reached. This may be further proved by measuring oxidative stress indicators such as some key reactive oxygen species.
In the same trend, the difference in foliar Mg concentration might indeed be a cause of the low leaf biomass production found in Pc, since Mg is a macronutrient present in the chlorophyll molecule and therefore related to plant growth (Marschner, 2012). In addition, some competition between the Mg, Mn, and Ca might exist given the involvement of these elements in some reactions because of their similar atomic radii; this could explain why a drop was reported in the birch leaf Mg when no differences were observed in the CaCl2 extractable Mg among the treatments (Supplementary Table S1). Chen et al. (2010) noted that the Mg deficiency in Betula alnoides seedlings produced an impaired root to shoot ratio, along with reductions in the stem width, height, and leaf area. The decrease in Mg leaf concentration directly affects the chlorophyll concentration (Farhat et al., 2016), which is seen in the CCI drop in Figure 4. Additionally, due to this Mn-Mg-Ca relation, a decline in leaf Ca was also observed in birch from the Ec and Pc treatments (Table 1; White and Broadley, 2003). A similar behavior was seen in Beta vulgaris (Hermans et al., 2005). Ericsson and Kähr (1995) also reported low values of Mg in B. pendula Roth., between 1400 and 1800 mg kg–1 DM, although their experiment used younger seedlings than the ones used in our study.
Chromium is a non-essential element for plants that can be detrimental for growth (Shanker et al., 2005. Concentrations in birches (Table 1) were within the 0.006–18 mg kg–1 DM range for common foliar concentrations (Shanker et al., 2005). However, all the organic treatments induced a strong decrease of Cr in birch leaves, as compared to the untreated RRG (Supplementary Figure S1). Our findings indicate that treating Cr-contaminated soils with organic amendments might be beneficial for both plant and human health (Kumpiene et al., 2008).
The foliar Na concentration of Pc reached up to six-fold the control concentration (some 930 mg kg–1 DM) nonetheless Modrzewska et al. (2016) reported foliar Na concentrations up to 264 mg kg–1 DM for B. pendula growing alongside roads with heavy traffic exposed to salt for winter. Leaves of Pc plants accumulated the highest amount of Mn, P, and Na, whereas they accumulated lower amounts of Cr, Mg, and Ca, confirming what was stated above. Differences on foliar Mn removal may be attributed to the variation in the nutrients supplied by the amendments; for instance, the ericaceous compost is undeniably more highly charged than Ms and even Pc with macronutrients (P, K, Mg, and Ca) and organic matter (Chevoleau et al., 2005 from Barbier, 2010). Even when the pH affected virtually only the solubility of Mn and Cr, the balance of Ca and Mg was affected, also inducing deficiencies, which are one of the most common problems in plant development (Zhang and Zwiazek, 2016). Despite the high Mn concentration in leaves induced by Pc, the foliar Mn removal was still lower than that of Ec because of the difference in biomass production. Finally, in the control treatment the BFC of Mn had a notably low factor (BCF = 0.08); however, when amendments were present values increased notoriously: 0.15 for Ms, 0.22 for Wp, 0.31 for Ec and 0.36 for Pc (Table 1). The BCF analysis indicated that even the use of a weak pH-modifying amendment such as Miscanthus straw increased the Mn bioconcentration factor by two-fold the BCF of RRG. Using ericaceous compost and pine bark amendments showed similar BCF values for Mn with around four-fold the values of RRG; however, the ericaceous compost allowed the highest Mn accumulation per plant, and this result should be considered when amendments are chosen.
Conclusion
The RRG tailings of the Ochsenfeld site is a potential micronutrient sink, i.e., Mn, Mg, and Ca. Such nutrients would be useful for the site revegetation but phytomanagement practices ought to be applied to increase the plant development and nutrient extraction. Additionally, the revalorization of such substrate as a phytoextracted raw material (i.e., for the production of ecocatalysts) would also possible, providing that a cost benefit analysis is achieved. Out of the four amendments the ericaceous compost allowed a decent plant development and biomass production, plus changes in substrate pH leading to a higher leaf Mn removal per plant. The use of pH-decreasing amendments resulted in Mn solubilization, notably when amending RRG with ericaceous compost and pine bark chips. In contrast, the addition of amendments reduced the Cr solubility preventing phytotoxicity issues in all treatments. Finally, the revegetation of the Ochsenfeld site is possible but the substrate may require a long-term source of macronutrients, e.g., nitrogen, for ensuring a relevant development of B. pendula.
Data Availability Statement
All datasets generated for this study are included in the article/Supplementary Material.
AUTHOR CONTRIBUTIONS
LC, FT-F, JP, and MC contributed to the conception and design of the experiment. JZ-C, LC, JP, and FT-F contributed to the acquisition of the data. JZ-C and JP contributed to the data analysis. JZ-C wrote the first draft of the manuscript. LC, JP, MC, and FT-F provided critical revision and suggestions to the first draft. All authors contributed to manuscript revision, read and approved the submitted version.
Funding
This work was supported by the French National Research Agency (PHYTOCHEM ANR-13-CDII-0005-01) and the French Environment and Energy Management Agency (PROLIPHYT ADEME- 1172C0053). JZ-C received a Ph.D. grant from the Mexican National Council of Science and Technology (Beca – CONACYT 440492). This work was supported by the French government and by the Pays de Montbéliard Agglomération (13/070-203-2018).
Conflict of Interest
The authors declare that the research was conducted in the absence of any commercial or financial relationships that could be construed as a potential conflict of interest.
Acknowledgments
We acknowledge Dr. Nadia Morin-Crini and Caroline Amiot for the ICP-AES analyses. We thank Jean Michel Colin (CRISTAL Co., France) for providing us with access to the Thann site and RRG substrate. We acknowledge and thank Sophie Favre-Reguillon for her preliminary results during her internship at the Chrono-Environment Laboratory in 2016.
Supplementary Material
The Supplementary Material for this article can be found online at: https://www.frontiersin.org/articles/10.3389/fenvs.2020.00024/full#supplementary-material
References
Abdulkadyrova, M. A., Dikinov, A. H., Tajmashanov, H. E., Shidaev, L. A., and Shidaeva, E. A. (2016). Global food security problems in the modern world economy. Int. J. Environ. Sci. Educ. 11, 5320–5330.
Al-Busaidi, A. S., and Cookson, P. (2003). Salinity – pH relationships in calcareous soils. Agric. Mar. Sci. 8, 41–46. doi: 10.1016/j.scitotenv.2017.10.233
Alloway, B. J. (ed.) (1995). Heavy Metals in Soils, 2nd Edn, Dordrecht: Blackie Academic & Professional.
Assad, M., Chalot, M., Tatin-froux, F., Bert, V., and Parelle, J. (2019). Trace metal(oid) accumulation in edible crops and poblar cuttings grown on dredged sediment enriched soil. J. Environ. Qual. 47, 1496–1503. doi: 10.2134/jeq2018.03.0106
Assad, M., Tatin-Froux, F., Blaudez, D., Chalot, M., and Parelle, J. (2017). Accumulation of trace elements in edible crops and poplar grown on a titanium ore landfill. Environ. Sci. Pollut. Res. 24, 5019–5031. doi: 10.1007/s11356-016-8242-4
Barbier, C. (2010). Crevettes d’eau Douce en Aquariophilie: Exemple de Maintenance de la Neocardina Heteropoda Pour les Débutants. Thèse d’exercise, Médecine vétérinaire, Université de Toulouse, Toulouse.
Chen, L., Zeng, J., Xu, D. P., Zhao, Z. G., and Guo, J. J. (2010). Macronutrient deficiency symptoms in Betula alenoides Seedlings. J. Trop. For. Sci. 22, 403–413.
Chevoleau, P., Cusimano, E., Burnel, P., Negrini, M., Cavignaux, R., Grioche, A., et al. (2005). L’Aquarium D’eau Douce. Maurens: Animalia Editions.
Chopin, E. I. B. B., and Alloway, B. J. (2007). Distribution and mobility of trace elements in soils and vegetation around the mining and smelting areas of Tharsis, Ríotinto and Huelva, Iberian Pyrite Belt, SW Spain. Water. Air. Soil Pollut. 182, 245–261. doi: 10.1007/s11270-007-9336-x
Ciadamidaro, L., Parelle, J., Tatin-froux, F., Moyen, C., Durand, A., Zappelini, C., et al. (2019). Early screening of new accumulating versus non-accumulating tree species for the phytomanagement of marginal lands. Ecol. Eng. 130, 147–156. doi: 10.1016/j.ecoleng.2019.02.010
de Mendiburu, F. (2014). Agricolae: Statistical Procedures for Agricultural Research. R Packag. Version 1.
de Tender, C. A., Debode, J., Vandecasteele, B., D’Hose, T., Cremelie, P., Haegeman, A., et al. (2016). Biological, physicochemical and plant health responses in lettuce and strawberry in soil or peat amended with biochar. Appl. Soil Ecol. 107, 1–12. doi: 10.1016/j.apsoil.2016.05.001
Deng, D., Gopiraman, M., Kim, S. H., Chung, I., and Kim, I. S. (2016). Human hair: a suitable platform for catalytic nanoparticles. ACS Sustain. Chem. Eng. 4, 5409–5414. doi: 10.1021/acssuschemeng.6b01689
Ducic, T., and Polle, A. (2005). Transport and detoxification of manganese and cooper in plants. Braz. J. Plant Physiol. 17, 103–112. doi: 10.1590/s1677-04202005000100009
EEA (2007). Europe’s Environment — The Fourth Assessment — European Environment Agency. State Environ. Rep. No 1/2007. Available online at: https://www.eea.europa.eu/publications/state_of_environment_report_2007_1 (accessed September 19, 2017).
Ericsson, T. O. M., and Kähr, M. (1995). Growth and nutrition of birch seedlings at varied relative addition rates of magnesium. Tree Physiol. 15, 85–93. doi: 10.1093/treephys/15.2.85
European Commission [EC] (2015). The 2030 Agenda for Sustainable Development and the SDGs. Available online at: https://ec.europa.eu/environment/sustainable-development/SDGs/index_en.htm (accessed December 21, 2019).
Eurostat (2016). Statistical Office of the European Communities: Generation of Waste by Waste Category (Total Waste). Available online at: https://ec.europa.eu/eurostat/databrowser/view/ten00108/default/table?lang=en (accessed July 1, 2019).
Farhat, N., Elkhouni, A., Zorrig, W., Smaoui, A., Abdelly, C., and Rabhi, M. (2016). Effects of magnesium deficiency on photosynthesis and carbohydrate partitioning. Acta Physiol. Plant 38:145. doi: 10.1007/s11738-016-2165-z
Fendorf, S. E. (1995). Surface reactions of chromium in soils and waters. Geoderma 67, 55–71. doi: 10.1016/0016-7061(94)00062-f
Gázquez, M. J., Bolívar, J. P., Garcia-Tenorio, R., and Vaca, F. (2014). A review of the production cycle of titanium dioxide pigment. Mater. Sci. Appl. 05, 441–458. doi: 10.4236/msa.2014.57048
Gotoh, S., and Patrick, W. H. (1972). Transformation of manganese in a waterlogged soil as affected by redox potential and pH. Soil Sci. Am. Proc. 36, 738–742. doi: 10.2136/sssaj1972.03615995003600050018x
Grison, C., Escande, V., and Biton, J. (2015). Ecocatalysis: A New Integrated Approach to Scientific Ecology. Amsterdam: Elsevier.
Hermans, C., Bourgis, F., Faucher, M., Strasser, R. J., Delrot, S., and Verbruggen, N. (2005). Magnesium deficiency in sugar beets alters sugar partitioning and phloem loading in young mature leaves. Planta 220, 541–549. doi: 10.1007/s00425-004-1376-5
Houba, V. J. G., Temminghoff, E. J. M., Gaikhorst, G. A., and van Vark, W. (2000). Soil analysis procedures using 0.01 M calcium chloride as extraction reagent. Commun. Soil Sci. Plant Anal. 31, 1299–1396. doi: 10.1080/00103620009370514
Hueso-González, P., Martinez-Murillo, J. F., and Ruiz-Sinoga, J. D. (2018). El uso de bioresiduos en la restauracion de ecosistemas degradados: estabilidad estructural, infiltracion y cobertura vegetal. Papeles Geogr. 64, 63–79. doi: 10.6018/geografia/2018/320561
Hueso-González, P., Martinez-Murillo, J. F., and Sinoga, J. D. R. (2017). Beneficios de los acolchados de paja y poda como prácticas para la gestión forestal de montes mediterráneos. Cuad. Investig. Geogr. 43, 189–208.
Hughes, P. N., Glendinning, S., Manning, D. A. C., and White, M. L. (2011). Use of red gypsum in soil mixing engineering applications. Geotech. Eng. 164, 223–234. doi: 10.1680/geng.10.00061
Jackson, B. E., Wright, R. D., and Gruda, N. (2009). Container Medium pH in a pine tree substrate amended with peatmoss and dolomitic limestone affects plant growth. Hortscience 44, 1983–1987. doi: 10.21273/hortsci.44.7.1983
Jiang, F., Ren, B., Hursthouse, A., Deng, R., and Wang, Z. (2019). Distribution, source identification, and ecological-health risks of potentially toxic elements (PTEs) in soil of thallium mine area (southwestern Guizhou. China). Environ. Sci. Pollut. Res. 26, 16556–16567. doi: 10.1007/s11356-019-04997-3
Kabata-Pendias, A., and Pendias, H. (2011). Trace Elements in Soils and Plants, 4th Edn, Boca Raton: Taylor & Francis Group.
Kamarudin, R. A., and Zakaria, M. S. (2007). The utilization of red gypsum waste for glazes. Malay. J. Anal. Sci. 11, 57–64.
Kassambara, A., and Mundt, F. (2017). Package Factoextra. Available online at: https://cran.r-project.org/web/packages/factoextra/factoextra.pdf (accessed July 1, 2019).
Kasurinen, A., Biasi, C., Holopainen, T., Rousi, M., Mäenpää, M., and Oksanen, E. (2012). Research paper: part of an invited issue on carbon allocation Interactive effects of elevated ozone and temperature on carbon allocation of silver birch (Betula pendula) genotypes in an open-air field exposure. Tree Physiol. 32, 737–751. doi: 10.1093/treephys/tps005
Kitao, M., Lei, T. T., Nakamura, T., and Koike, T. (2001). Manganese toxicity as indicated by visible foliar symptoms of Japanese white birch (Betula platyphylla var. japonica). Environ. Pollut. 111, 89–94. doi: 10.1016/S0269-7491(99)00332-2
Kumpiene, J., Lagerkvist, A., and Maurice, C. (2008). Stabilization of As, Cr, Cu, Pb and Zn in soil using amendments - A review. Waste Manag. 28, 215–225. doi: 10.1016/j.wasman.2006.12.012
Kurt-Karakus, P. B. (2012). Determination of heavy metals in indoor dust from Istanbul, Turkey: estimation of the health risk. Environ. Int. 50, 47–55. doi: 10.1016/j.envint.2012.09.011
Lê, S., Josse, J., and Husson, F. (2008). FactoMineR: an r package for multivariate analysis. J. Stat. Softw. 25, 1–18. doi: 10.1016/j.envint.2008.06.007
Lebourgeois, V., Bégué, A., Labbé, S., Houlès, M., and Martiné, J. F. (2012). A light-weight multi-spectral aerial imaging system for nitrogen crop monitoring. Precis. Agric. 13, 525–541. doi: 10.1007/s11119-012-9262-9
Manimel Wadu, M. C., and Chang, S. X. (2017). Micronutrient concentrations vary between peat-mineral mix and substrates in revegetated sites in the Alberta oil sands. Can. J. Soil Sci. 98, 181–192. doi: 10.1139/cjss-2016-0130
Mattei, P., Pastorelli, R., Rami, G., Mocali, S., Giagoni, L., Gonnelli, C., et al. (2017). Evaluation of dredged sediment co-composted with green waste as plant growing media assessed by eco-toxicological tests, plant growth and microbial community structure. J. Haz. Mat. 333, 144–153. doi: 10.1016/j.jhazmat.2017.03.026
Millaleo, R., Reyes-Diaz, M., Ivanov, A. G., Mora, M. L., and Alberdi, M. (2010). Manganese As essential and toxic element for plants: transport, accumulation and resistance mechanisms. J. Soil Sci. Plant Nutr. 10, 470–481. doi: 10.4067/S0718-95162010000200008
Modrzewska, B., Kosiorek, M., and Wyszkowski, M. (2016). Content of some nutrient in Scots pine silver birch and Norway maple in an urbanized environment. J. Elem. 21, 149–157. doi: 10.5601/jelem.2015.20.2.845
Prévosto, B., and Ripert, C. (2008). Regeneration of Pinus halepensis stands after partial cutting in southern France: Impacts of different ground vegetation, soil and logging slash treatments. Forest Ecol. Manag. 256, 2058–2064. doi: 10.1016/j.foreco.2008.07.027
Reuter, D. J., Alston, A. M., and Mcfarlane, J. D. (1988). “Occurrence and correction of manganese deficiency in plants,” in Manganese in Soils and Plants, eds R. D. Graham, R. J. Hannam, and N. C. Uren (Dordrecht: Springer), 205–224. doi: 10.1007/978-94-009-2817-6_15
Robinson, B. H., Bañuelos, G., Conesa, H. M., Evangelou, M. W. H., and Schulin, R. (2009). The Phytomanagement of Trace Elements in Soil. CRC Crit. Rev. Plant Sci. 28, 240–266. doi: 10.1080/07352680903035424
Shanker, A. K., Cervantes, C., Loza-Tavera, H., and Avudainayagam, S. (2005). Chromium toxicity in plants. Environ. Int. 31, 739–753. doi: 10.1016/j.envint.2005.02.003
Takarina, N. D., and Pin, T. G. (2017). Bioconcentration Factor (BCF) and translocation factor (TF) of heavy metals in mangrove trees of blanakan fish farm. Makara J. Sci. 21, 77–81. doi: 10.7454/mss.v21i2.7308
Takeno, N. (2005). Atlas of Eh-pH diagrams Intercomparison of thermodynamic databases. Geol. Surv. Jpn. 419:285.
Tandy, S., Healey, J. R., Nason, M. A., Williamson, J. C., and Jones, D. L. (2009). Remediation of metal polluted mine soil with compost: Co-composting versus incorporation. Environ. Pol. 157, 690–697. doi: 10.1016/j.envpol.2008.08.006
van Winkle, G. S., and Pullman, S. C. (2003). The combined impact of pH and activated carbon on the elemental composition of a liquid conifer embryogenic tissue initiation medium. Plant Cell Rep. 22, 303–311. doi: 10.1007/s00299-003-0686-6
White, P. J., and Broadley, M. R. (2003). Calcium in Plants. Ann. Bot. 92, 487–511. doi: 10.1093/aob/mcg164
Wickam, H. (2017). “ggplot2 - elegant graphics for data analysis,” in Journal of Statistical Software, ed. V. Gomez-Rubio (New York, NY: Springer-Verlag), 3–5. doi: 10.18637/jss.v077.b02
Young, I., Renault, S., and Markham, J. (2015). Low levels organic amendments improve fertility and plant cover on non-acid generating gold mine tailings. Ecol. Eng. 74, 250–257. doi: 10.1016/j.ecoleng.2014.10.026
Zapata-Carbonell, J., Bégeot, C., Carry, N., Choulet, F., Delhautal, P., Gillet, F., et al. (2019). Spontaneous ecological recovery of vegetation in a red gypsum land fill: Betula pendula dominates after 10 years of inactivity. Ecol. Eng. 132, 31–40. doi: 10.1016/j.ecoleng.2019.03.013
Zappelini, C., Alvarez-Lopez, V., Capelli, N., Guyeux, C., and Chalot, M. (2018). Streptomyces dominate the soil under betula trees that have naturally colonized a red gypsum landfill. Front. Microbiol. 9:1772. doi: 10.3389/fmicb.2018.01772
Keywords: Betula pendula, organic amendments, potentially toxic elements, phytomanagement, red gypsum
Citation: Zapata-Carbonell J, Ciadamidaro L, Parelle J, Chalot M and Tatin-Froux F (2020) Improving Silver Birch (Betula pendula) Growth and Mn Accumulation in Residual Red Gypsum Using Organic Amendments. Front. Environ. Sci. 8:24. doi: 10.3389/fenvs.2020.00024
Received: 25 September 2019; Accepted: 20 February 2020;
Published: 19 March 2020.
Edited by:
María Balseiro-Romero, University of Santiago de Compostela, SpainReviewed by:
Rafael Clemente, Center for Edaphology and Applied Biology of Segura (CSIC), SpainMichel Jean Mench, Institut National de la Recherche Agronomique, France
Copyright © 2020 Zapata-Carbonell, Ciadamidaro, Parelle, Chalot and Tatin-Froux. This is an open-access article distributed under the terms of the Creative Commons Attribution License (CC BY). The use, distribution or reproduction in other forums is permitted, provided the original author(s) and the copyright owner(s) are credited and that the original publication in this journal is cited, in accordance with accepted academic practice. No use, distribution or reproduction is permitted which does not comply with these terms.
*Correspondence: Michel Chalot, michel.chalot@univ-fcomte.fr
†Present address: Lisa Ciadamidaro, ECOSYS UMR 1402-INRA-AgroParisTech, Ecotoxicology Team, RD 10, Versailles, France