- 1Department of Biology, San Diego State University, San Diego, CA, United States
- 2School of Biological Sciences, Queen's University of Belfast, Belfast, United Kingdom
- 3Centre for Marine Bioproducts Development, College of Medicine and Public Health, Flinders University, Adelaide, SA, Australia
- 4Department of Chemical Engineering, Instituto Superior de Engenharia Do Porto, Porto, Portugal
- 5Department of Energy, Environment, and Climate, School of Environment, Resources and Development, Asian Institute of Technology, Pathumthani, Thailand
- 6Department of Civil and Environmental Engineering, University of Michigan, Ann Arbor, MI, United States
- 7Department of Engineering Technology, College of Technology, University of Houston, Houston, TX, United States
Editorial on the Research Topic
Methane: A Bioresource for Fuel and Biomolecules
Methane (CH4), a highly reduced C1 compound, is one of the long-lived atmospheric gases with high global warming potential i.e., 28–36 times that of CO2 over 100 years. The atmospheric levels of CH4 reached ~1863 part per billions (ppb) in 2014, and annual increase of atmospheric CH4 level thereafter measured as ~10 ppb. The CH4 is projected to drive the rise in global temperature of ~4–6°C by 2050, and thus it is currently considered as the main target for global climate stabilization and mitigation (COP-21, 2015). Capturing anthropogenic CH4 to produce value products is highly feasible, but the great challenge is that to tap, concentrate, purify, store, transport, and utilize the CH4 from different point emission sources is presently not economically viable.
In this special issue, a conceptual model of “Methane-Biorefinery Hub” is proposed as a sustainable development to mitigate the CH4 emissions from the most significant anthropogenic sources. The idea of “Methane-Biorefinery Hub” is deeply rooted in natural CH4 production and consumption processes (Figure 1). While in general, with a few exceptions, the natural CH4 cycle is balanced, the anthropogenic disturbances have typically led to increase the CH4 emissions. Thus, better understanding of mechanisms that control CH4 cycle in nature can be used to engineer better systems in human-built environments. For example, Holmes et al.. established that the bacteria (donors) and archaea (acceptors) communicate through nano-wires or electron transfer molecules i.e., the electrons transfer through e-pili (i.e., direct interspecies electron transfer), while protons diffusion (direct interspecies hydrogen transfer) is regulated by the partial pressure of the bioreactor system. Mimicking natural processes in laboratory/pilot-scale bioreactors that are designed to optimize specific operational conditions to regulate such communication may lead to successful implementation of technology to effectively utilize the waste materials e.g., municipal solid waste, food waste, industrial organics and wastewaters, or low-grade coals with/without the aid of external carbon/electron sources to produce CH4 as bio-energy, as shown by Yang et al. As pointed out by Wojcieszak et al., well-balanced microbial consortium are crucial for efficient biogas production, and inoculum sampled from typical methanogenic environments can be gradually adapted to industrial installations to allow effective biogas production. Addition of microbial supplements, metals/nutrients, organic sources (Zaldívar Carrillo et al.), electron conductive materials or electrodes, and altering the head-space gas composition (and partial pressure) are proposed as options for facilitating electron transfer and microbial interactions, while it may also change the equilibrium between dissolved vs. gas phase CH4 concentrations within the system.
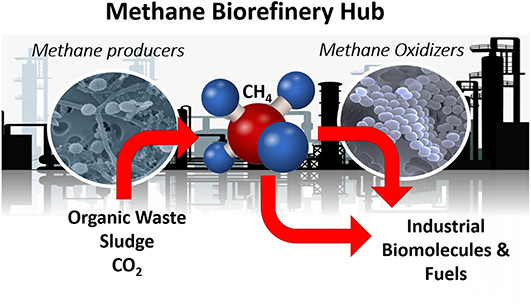
Figure 1. Methane biorefinery for value chemical and biofuels. Microbial images (SEM) courtesy of Dr. Dennis Kunkel.
Another unique approach for recovering dissolved CH4 using degassing membrane contactors was proposed by Velasco et al.. While still at the stage of infancy, this unique technology highlights limitless opportunities for innovative approaches in CH4 capturing. If not recovered effectively, the dissolved CH4 and manipulating conditions may be expected to fuel the anaerobic methane oxidizers (ANME), and also sulfate reducing bacteria (SRB), to thrive and compete with methanogens for electrons/protons that may lead to low CH4 yield. The ANME possess a reverse methanogenesis process, i.e., utilizing CH4 (and CO2) as carbon sources to produce acetate or other products. On the other hand SRB compete with methanogens for similar substrates or electron donors. So, there may not be a competition between SRB and ANME for substrate, but it required investigation. On the other hand, the industrial applications of ANME are challenging, and still limited by the number of unresolved biochemical questions. However, an example of how a solid understanding of enzyme kinetics and energy transfer between the microbial communities can be used to manipulate the operating conditions to either facilitate or eliminate methane production was presented by Grisewood et al.. Alternatively, establishment of co-cultures of methanogens and ANME to produce value chemicals from anaerobic digestion processes was proposed and validated using a newly developed mathematical model by Nazem-Bokaee and Maranas, which, however, still relies on the electron coupling theory. Once fully understood, the ANME-based approach could be a viable option for reducing CH4 emissions from natural settings, while aerobic oxidation is recommended for industrial-scale organic digestion facilities.
The aerobic CH4 oxidization process is easily coupled with digesters, while the process can yield a number of value products such as polymers, organic acids, single cell protein (SCP), compatible solutes, short/long chain fatty acids, omega fatty acids, vitamins, methanol, formate, etc. Methanotrophs are classified into Group I and Group II based on their physiology. Group I methanotroph e.g., Methylococcus capsulatus (Bath) is reported to produce SCP, while Group-II methanotrophs (e.g., Methylosinus trichosporium OB3b) are capable of accumulating biopolymers/SFA/LFA from CH4. An example of coupled production is highlighted by the work of Henard et al.. Metabolic modeling can further impower application of natural CH4 consuming bacteria, as exemplified by Lieven et al.. While it is widely acknowledged that the natural capacities of microbial systems (as axenic or mixed cultures) in CH4-consumption and accumulation of value products are governed by a number of factors e.g., carbon, nutrients, and metals, we are only now applying the knowledge for improving their industrial potential. Further, an example of how nitrogen starvation activates polyhydroxyalkanoate accumulation and alters the fatty acid compositions in biomass is provided by Tays et al.. The metal-switch impacts on the key enzyme activities, kinetics, the internal electron pool, and the carbon flux e.g., are described in the work by Akberdin et al..
Nevertheless, the industrial applications and innovations remain to be challenged by long-standing fundamental questions regarding CH4 biocatalysts; including: (a) source of electron donors for CH4 activation as well as electron acceptors for process intensification; (b) regulation of contaminations or development of efficient strategies for controlling natural communities or synthetic co-cultures; (c) improved genetic traceability of methanotrophs in a mixed consortium and (d) coupling of CH4-conversion potential with efficient nitrogen fixation and denitrification. We would like to acknowledge significant progress in developing systems biology toolbox for manipulating methanotrophic bacteria, as well as new advances in overcoming technological bottlenecks related to CH4 mass-transfer limitation. Yet, some challenges still remain. New developments highlight additional need for further research, specifically in areas of O2-capturing, cell immobilization, CH4 and CO2 conversion by coculture of methanotrophs and algae/methylotrophs, coupling CH4 conversion and electrocatalysis. Algae and methanotrophs exchange nutrients during co-culturing are beneficial, while methylotrophs helps to alleviate any methanol toxicity.
The papers published in this special issue confirm that CH4 emission/production is related to the microbiomes of the system, which are easy to be manipulated through biological/chemical augmentation methods. By coupling CH4 production with either ANME or methanotrophs, emissions could be significantly reduced and high-value products could be recovered through an integrated “Methane-Biorefinery” approach. Interestingly, in the work by Burton et al. it was concluded that the aerobic methanotrophs, methylotrophs and methanogenic archaea found to have common lineage i.e., use tetrahydromethanopterin (H4MPT) and/or tetrahydrofolate (H4F) as coenzymes in one-carbon (C1) transfer pathways that have been overlooked in the past and the relationships need to be well-studied for harnessing the benefits under “Microbiome” theory.
Author Contributions
OP and MK written this editorial. NC, KH, CV, and DK edited the final text. All authors approved the final version.
Conflict of Interest
The authors declare that the research was conducted in the absence of any commercial or financial relationships that could be construed as a potential conflict of interest.
Keywords: methane, methanotrophs, electron transfer, bioreactor, value addition and sustainability
Citation: Kalyuzhnaya MG, Kumaresan D, Heimann K, Caetano NS, Visvanathan C and Parthiba Karthikeyan O (2020) Editorial: Methane: A Bioresource for Fuel and Biomolecules. Front. Environ. Sci. 8:9. doi: 10.3389/fenvs.2020.00009
Received: 18 October 2019; Accepted: 13 January 2020;
Published: 05 February 2020.
Edited by:
Hyung-Sool Lee, University of Waterloo, CanadaReviewed by:
Seung Gu Shin, Pohang University of Science and Technology, South KoreaWei-Min Wu, Stanford University, United States
Copyright © 2020 Kalyuzhnaya, Kumaresan, Heimann, Caetano, Visvanathan and Parthiba Karthikeyan. This is an open-access article distributed under the terms of the Creative Commons Attribution License (CC BY). The use, distribution or reproduction in other forums is permitted, provided the original author(s) and the copyright owner(s) are credited and that the original publication in this journal is cited, in accordance with accepted academic practice. No use, distribution or reproduction is permitted which does not comply with these terms.
*Correspondence: Obulisamy Parthiba Karthikeyan, opkens@gmail.com; pobulisa@umich.edu