- 1Farming Systems Ecology, Wageningen University & Research, Wageningen, Netherlands
- 2Agroecology and Sustainable Intensification of Annual Crops (AIDA), University of Montpellier, French Agricultural Research Centre for International Development (CIRAD), Montpellier, France
- 3International Maize and Wheat Improvement Center (CIMMYT), Sustainable Intensification Program, Nairobi, Kenya
- 4Agroecology, Environment and Systems Group, Instituto de Investigaciones Forestales y Agropecuarias de Bariloche (IFAB), INTA-CONICET, San Carlos de Bariloche, Río Negro, Argentina
- 5Groningen Institute of Evolutionary Life Sciences, Groningen University, Groningen, Netherlands
Sustainable food production requires approaches that reconcile agricultural production with the conservation and sustainable use of natural resources, biodiversity and associated ecosystem services. While the contribution of agriculture to the provision of individual ecosystem services has received considerable scientific attention, little is known about the extent to which tropical landscapes can meet societal expectations related to food production and environmental sustainability simultaneously. We assessed how the spatial configuration of pedo-morphology and land uses influences the provision of three soil-based ecosystem services in eastern Amazonia: carbon storage (CS), habitat for biodiversity (HB), and agricultural commodity production (CP). We use the Functional Land Management framework to assess the supply and demand of these ecosystem services in a spatially explicit manner to identify areas of (mis)matches and trade-offs in the municipality of Paragominas, Brazil. The supply of ecosystem services was informed by a literature review for the various combinations of pedo-morphological characteristics and land uses in the region. The demand for ecosystem services was mapped based on federal and state policy targets. Mapping the supply and demand of CS indicated that half of the carbon in the region is stored in remnants of undisturbed forest which cover only a third of the municipality. Demand for HB in terms of forested area is met but it does not guarantee safeguarding biodiversity. Roughly a third of the territory shows scarce quality of HB even when compliant with legislation. Concerning CP, we identified areas where both supply and the demand to increase production are relative high due to road access and lower intensification costs. The demand for agricultural production can eventually incentivize the expansion of agriculture on fertile soils, which could compromise environmental targets. Our results suggest that the simultaneous delivery of multiple ecosystem services may require land-use pathways that combine land sparing and sharing approaches. Our analysis can inform integrated land-use planning initiatives where, historically, the supply and demand for CP have been the single dominant driver for the current landscape configuration.
Introduction
With the growing human population in many parts of the world there is a challenge to reconcile food production with the conservation of biodiversity and associated ecosystem services. This requires that the production of higher volumes of food is accompanied by profound changes in the way food is produced, accessed and distributed (Holt-Giménez et al., 2012). The Amazon basin is a crucial region to tackle this challenge due to its unrivaled biodiversity, its regional and global regulating function of the carbon and hydrological cycle (Barthem et al., 2004; UNEP et al., 2009; Townsend et al., 2011), and its massive potentially available area for cropland expansion (Eitelberg et al., 2015). More than half of the Amazon rain forest is in Brazil, and of this portion, one fifth of the forestland has been cleared since the 1970s (WWF, 2019). The major cause of this loss is land-use changes driven by the development of subsistence agriculture, large-scale monocultures, and livestock production (Barthem et al., 2004).
Between 2004 and 2012, public and private forest conservation measures triggered a sharp decline of deforestation rates in the Brazilian Amazon (Nepstad et al., 2014). Nevertheless, these have resurged since then, casting doubts on the long-term effectiveness of control and command measures to protect biodiversity (Fearnside, 2017; Schielein and Börner, 2018). In forest frontiers of eastern Amazonia land-use change from primary forest to agricultural land takes different forms depending on road accessibility, population density, governance and cultural background of settlers (Schielein and Börner, 2018). Therefore, in addition to restrictions on deforestation, agricultural intensification, and diversification is recognized as a critical step to conciliate biodiversity conservation and agricultural production (Macedo et al., 2012; Barretto et al., 2013). In light of this, it is not clear which spatial land-use configurations can reconcile ecosystem services at the landscape scale. Since land-use change often threatens natural resources and the continued delivery of ecosystem services, there is an urgent need for developing land-use planning that better accounts for the societal demands for ecosystem services, and the biotic and abiotic characteristics of the land to support these ecosystem services (Tittonell, 2014; Schulte et al., 2015).
We explore transition pathways in the municipality of Paragominas in northern state of Pará by taking a spatial approach and asking what spatial arrangements of land uses can enhance the provision of ecosystem services given a recent past of deforestation. Our study focuses at the landscape scale in order to draw an integrated assessment of the land in terms of the delivery of ecosystem services and societal demands thereof. We focus on three soil-related ecosystem services, carbon storage (CS), habitat for biodiversity (HB), and commodity production (CP) due to the tension between nature conservation and agricultural production (i.e., trade-offs between ecosystem services) that originated since the inception of the current landscape six decades ago (Schmink and Wood, 1992).
The objective of this study is to identify priority areas and targeting of interventions aimed at augmenting specific functions of land management, including carbon storage, the enhancement of biodiversity and the provision of agricultural commodities. We analyze the landscape at the lower jurisdictional level (i.e., municipality), in order to integrate multi-scale demands and local governance to manage potential trade-offs between conservation and development. Jurisdictional approach is a term recently coined to indicate a landscape governance that seeks common goals among government, community stakeholders and businesses (Pacheco et al., 2017). In the Amazonian context, this approach refers specifically to greening commodity chains and complying with zero-deforestation policies. By identifying areas where land-use changes would be most effective to meet societal demands while preserving the region's natural capital, our analysis can contribute to develop context-specific transition pathways in order to enhance landscape multifunctionality and resource-use efficiency in eastern Amazonia.
Methods
Study Site
Our study was conducted in the municipality of Paragominas in the north-eastern state of Pará, Brazil. The municipality is located at one of the oldest agricultural frontiers in the Brazilian Amazon currently transitioning toward land-use intensification as an array of policy measures have prompted the end of extensive deforestation. Such measures include the revision of the Forest Code in 2012 with a strong focus on protecting natural vegetation both in public and private lands, restricting credits to illegal deforesters, blacklisting municipalities deforesting illegally, implementing supply chains moratoria and command and control mechanisms (Piketty et al., 2015; Azevedo et al., 2017; Nunes et al., 2019).
Paragominas, nevertheless, has a history of intensive forest logging and livestock grazing that ignored key biophysical and environmental aspects for the spatial planning of these activities, such as land relief and soil characteristics (Poccard-Chapuis et al., 2014). This trajectory has shaped the landscape of the region (Rodrigues et al., 2014) into a disconnected mosaic of regrowth and degraded forest, old fields, and low-productivity pastures (Nepstad et al., 1991; IBGE, 2016). In turn, these land-use dynamics have led to soil degradation, disruption of nutrients cycling, biodiversity loss, and increased fire susceptibility (Uhl and Kauffman, 1990; Nepstad et al., 1999; Barlow et al., 2016).
Pedo-morphological conditions in the region follow a specific pattern of monoclinal sedimentary units with a lateritic pedo-genesis that results in geomorphological surfaces of different soil textures and erosion conditions (Laurent et al., 2017). In Paragominas this manifests as plateaus of 160–190 m.a.s.l., separated by valleys of up to 10–12 km wide (Laurent et al., 2017). As a result of the genesis of the bedrock and the local topography, plateaus in the Paragominas region are covered by the so-called Belterra clay (clayey Ferralsols with 70–80% kaolinite), upper valley slopes are covered by gravel soils, while the bottom of the valleys are covered by loamy sand Ferralsols (Rodrigues et al., 2003; Laurent et al., 2017). Soil texture, in turn, is one of the main determinants of soil fertility, erosion, compaction, cation exchange, and water holding capacity (Laurent et al., 2017). Therefore, our study site was first classified into pedo-morphological units that represent combinations of soil texture, slope, and proximity to water bodies (Figure 1). We limited our analysis to clayey plateaus and sandy valleys, which account for 77% of the territory in Paragominas. We assumed a consistent soil type-pedo-morphology relationship across the study area (Laurent et al., 2017). Regarding land use, we classified the region into managed areas (mechanized agriculture, pastures, and forest plantations), unmanaged areas (natural regeneration and degraded forest), and conservation areas (undisturbed forests).
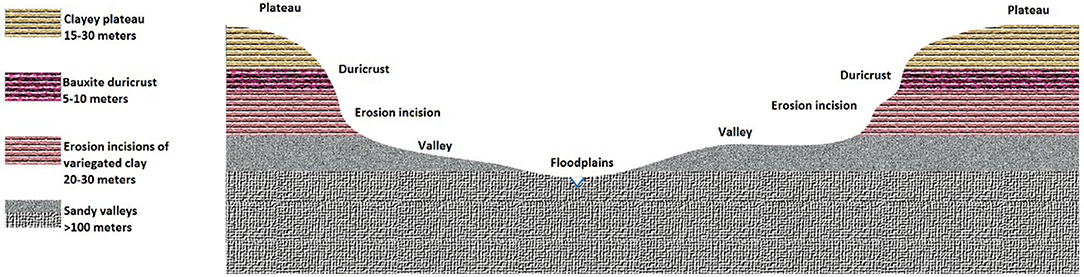
Figure 1. Geological profile and pedo-morphological units in Paragominas in the northeast state of Pará, Brazil.
Research Approach
We define a landscape as the spatial organization of biophysical characteristics and land uses driven by ecological processes and management practices (Poccard-Chapuis et al., 2014). Land-use decisions, however, are mostly made at the farm scale and public policies are regulating these decisions. In this conception, socio-economic, institutional, and environmental dimensions interact across farm, landscape, and municipal scales composing a socio-ecological system (Gallopín et al., 2001). We use the conceptual framework of Functional Land Management (FLM) (Schulte et al., 2014, 2015) to spatially identify areas where land is being used and managed in such a way that ecosystem services provision is not optimal and can be enhanced. By recognizing soil multifunctionality, FLM aims at the optimization- rather than maximization- of soil-based ecosystem services, given the difficulty of maximizing all functions at the same time across scales. We refer to “supply” as the provision of soil-based ecosystem services as a function of biophysical characteristics, land use and management; whereas “demand” is derived from agro-environmental policies acting as proxies of societal expectations (Schulte et al., 2015).
We considered pedo-morphology and land use as the two main drivers of the supply of ecosystem services in Paragominas. Thus, in order to manage the complexity of the socio-ecological system at the municipal level (19,342 km2), we first categorized the region into landscape units (LU) defined as the combination of pedo-morphological conditions (Figure 1) and land-use categories (Schulte et al., 2014; Coyle et al., 2016). To assess the ecosystem services supply for each LU, we reviewed the literature on the link between the provision of ecosystem services, pedo-morphological conditions, and land use. We assumed that these two bio-physical conditions (i.e., pedo-morphology and land use) will be the prime drivers of ecosystem services supply at the landscape scale because of their link with soil texture and land cover, respectively. First, we focused on the relation between each one of the ecosystem services and soil textural class as it conditions soil carbon storage capacity, soil fertility, and the likelihood that habitats for conservation will be competing with agricultural production in the region. We selected only studies from the Amazon and Cerrado biome except for the soil texture-soil biodiversity link as we did not find a relevant study for Brazil. This first literature review allowed us to qualitatively assess the (spatial) heterogeneity of the supply in relation to soil texture in order to build an informed categorization of LUs and capture general trends at the municipality level in relation to pedo-morphology.
A second literature review was conducted to assess the local delivery of CS and HB at land-use level using studies conducted in Paragominas. Carbon storage for major land use/land cover types was assessed using the Invest Carbon Model (Sharp et al., 2014). We estimated carbon stocks by summing secondary data on soil carbon and aboveground biomass. Data on carbon in belowground biomass, dead and harvested wood were not available and were therefore not included in the analysis. For the supply of HB, we derived an index based on recorded number of species for birds, dung beetles, orchid plant bees, and ants for each land use type (i.e., α-biodiversity at the site level) reported in the literature. To calculate indices, we took the highest value for each indicator specified in Table 1 and assigned this a value of 1. The remaining values were divided by the maximum value to generate a relative scale with a maximum value of 1. Concerning CP, we selected the three main commodities produced in the region: soybean, beef and wood products, and assessed their gross profit margin and investment return period as indicators of commodity supply. We chose economic indicators because these capture one of the three main dimensions to assess productive performance of land use systems (besides the ecological and agronomic dimension; Murray et al., 2016), and can be quantified for crop, beef, and forest production. We estimated the gross profit margin for each commodity based on official exports statistics for the year 2017, and other sources from scientific and gray literature reporting on internal markets, production costs, and profit margins in Brazil from 2004 to 2018 (Table 2).
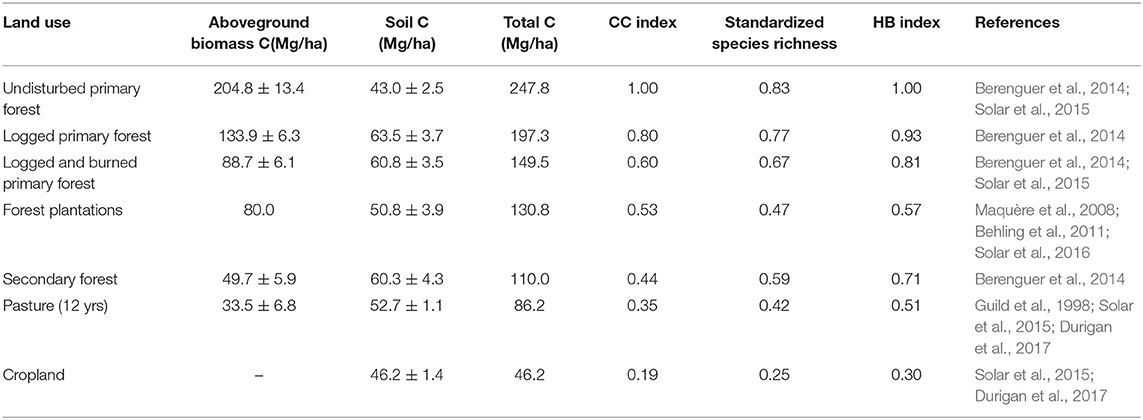
Table 1. Indices for carbon storage and habitat for biodiversity, based on values for indicators from secondary data.
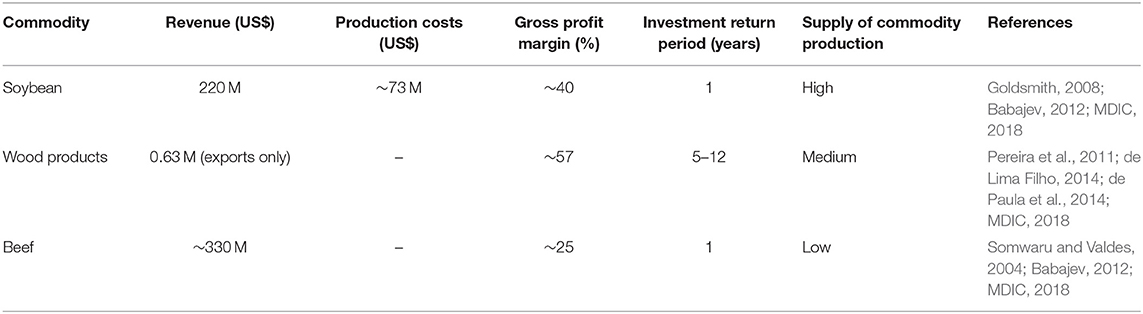
Table 2. Estimated revenue, production costs, gross profit margin, investment return periods, and relative level of production supply for the main commodities in Paragominas for the 2004–2018 period.
To frame the societal demands for ecosystem services delivery, we conducted reviews of the relevant policy frameworks related to CS, HB, and CP in Brazil. These included national policies, state legislation, and municipal regulations. We selected policies that explicitly state specific targets for our three ecosystem services. The selection of the demand indicators was based on these targets. In the case of CS, the targets include reducing national carbon emissions by 37% in 2025 in relation to 2005, and a ban on deforestation at the municipal level. The demand for HB was taken as the mandate to preserve 50–80% of each rural property as a forest conservation area, whereas the demand for CP was derived from the state-level targets aiming at annual production increases until 2030. We also took into account road infrastructure as underlying drivers of agricultural intensification.
Once indicators and values were developed and estimated both for supply and demand, we generated maps for the supply of ecosystem services by intersecting a map of pedo-morphological units (which encompasses the corresponding soil textural categories) with a map of land use of Paragominas in Esri ARCGIS 10.4. This generated a map of LUs that then were attributed the indices derived from the literature (Figure 2) and illustrated in our supply matrix (Figure 3). Regarding the demand maps, the spatial variation was not defined based on the LU classification, but rather on the targets established in the policies and their application at the municipal level.
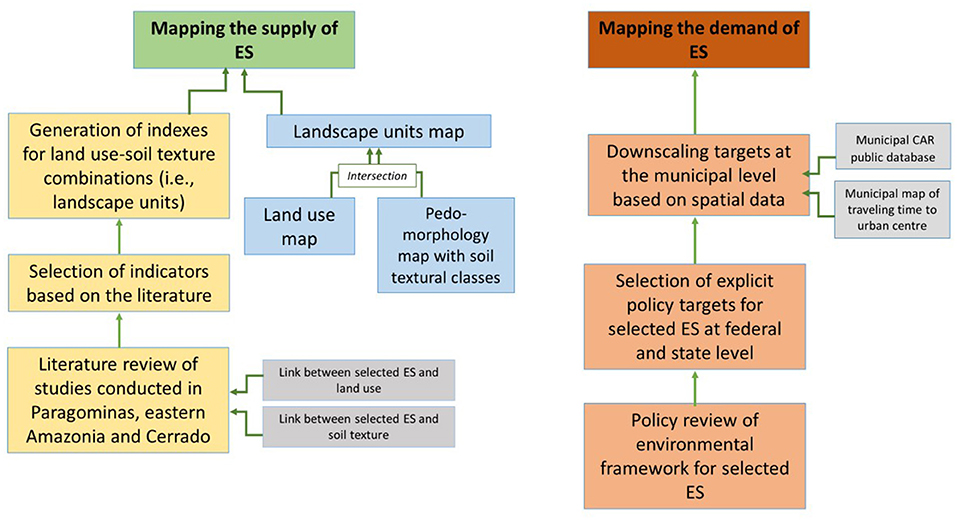
Figure 2. Diagram of methodological steps followed to map the supply and demand of ecosystem services in Paragominas.
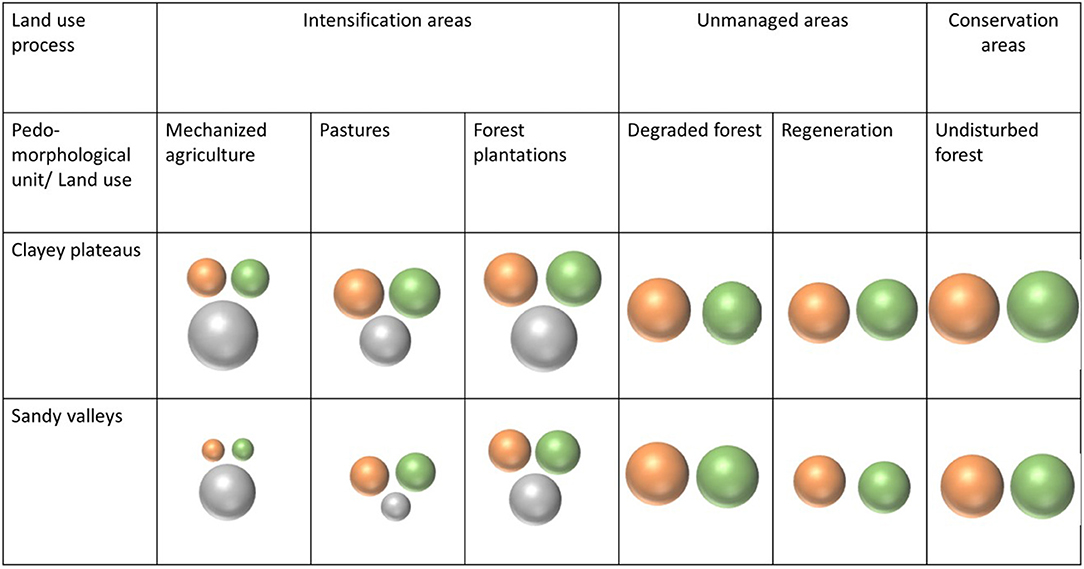
Figure 3. Supply matrix of ecosystem services in Paragominas for different pedo-morphological units and land uses associated with different land use processes. Supply of carbon storage (brown), habitat for biodiversity (green), and commodity production (gray) are indicated by bubbles, and bubble size is indicative for relative supply level. This analysis is informed by a literature review (Tables 1, 2) and personal observations.
Finally, to explore land use pathways to enhance landscape multifunctionality, we integrated the supply and demand maps for CS, HB and CP (Figure 5) to generate a trade-off map for these ecosystem services (Figure 6). We first standardized the supply and demand maps for CS, HB, and CP by generating maps with z-scores at the pixel level (630 × 630 m = 40 ha). Then the z-score transformed demand and supply maps were subtracted for each ecosystem service to reveal areas where supply meets or fails demand. The spatial distribution of trade-offs between CS, HB and CP were then integrated in a single map by systematically considering all eight (23) combinations of potential (mis)matches between CS, HB, and CP (Figure 4). All calculations were conducted in ArcGIS 10 using the raster calculator.
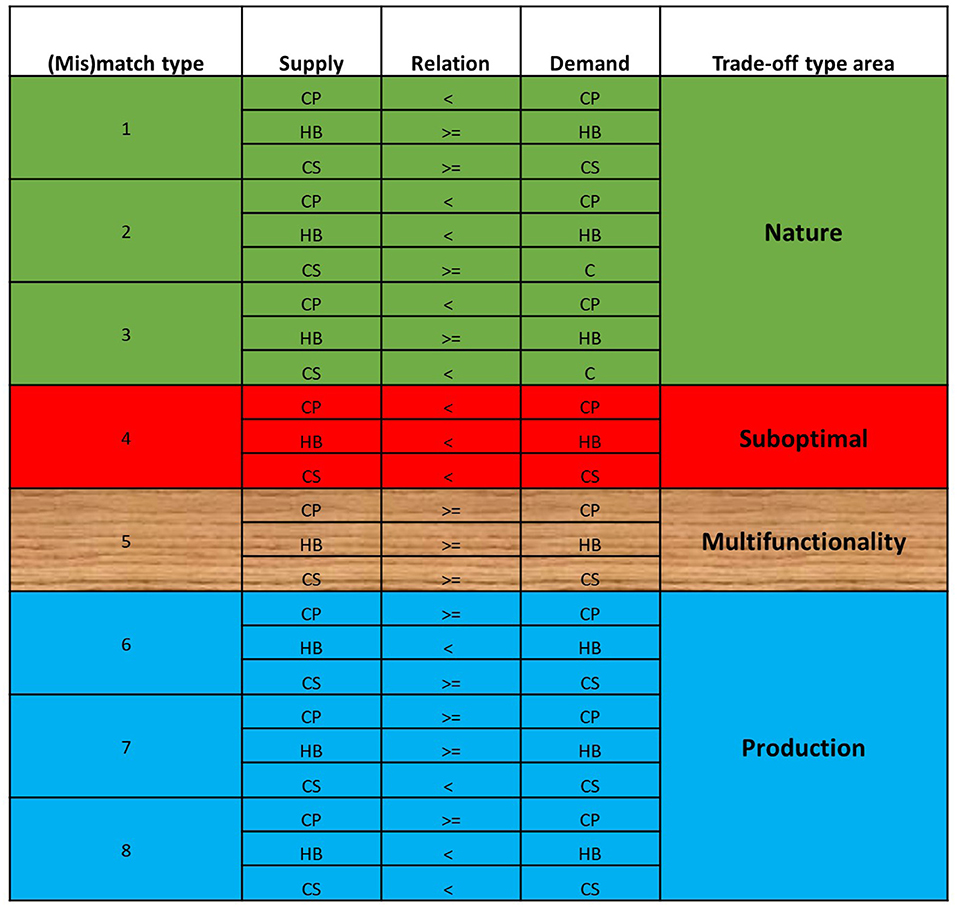
Figure 4. Overview of possible combinations of (mis)matches between carbon storage (CS), habitat for biodiversity (HB), and commodity production (CP), and associated trade-offs.
Supply of Soil-Based Ecosystem Services
Clay content is an important factor driving carbon dynamics and storage in tropical soils whereby clay content is usually positively associated with soil carbon storage capacity (Feller and Beare, 1997; Six et al., 2002; Telles et al., 2003). For example, in a study conducted on arable and native vegetation land in the Cerrado biome, Neto et al. (2010) reported a significant linear increase of soil organic carbon (0–20 cm) with clay content. In a study conducted in eastern Amazonia, Silver et al. (2000) reported significantly greater soil carbon content in clayey soils than in sandy soils at 20 cm. Another study conducted in clayey and sandy soils of the same forest in the Amazonia state, reported that at 40 cm depth, the soil carbon stocks in Oxisols (61–71 Mg C ha−1) were significantly higher than in Spodosols and Ultisols with higher sand content (46 and 42 Mg C ha−1) (Telles et al., 2003). Overall, these studies indicate that soils with higher clay content in the region tend to store more carbon and for longer times.
Regarding CS and land use, in a forest degradation gradient in Santarém, western Pará, Brazil, undisturbed primary forest had the highest stocks of soil carbon, followed by secondary forest, while disturbance by fire significantly reduced soil carbon (Durigan et al., 2017). Soil carbon stocks were not affected by the conversion of primary forest to pastures but decreased significantly when conversion to cropland took place (Durigan et al., 2017). Along a similar forest degradation gradient in Paragominas, carbon stocks in aboveground vegetation, dead wood, litter and soil (upper 30 cm) decreased from undisturbed forest (276 Mg C ha−1) to logged forest (238 Mg C ha−1), logged and burned forest (187 Mg C ha−1), and secondary forest (125 Mg C ha−1) (Berenguer et al., 2014). Furthermore, Sommer et al. (2000) reported significant changes in soil carbon and root biomass after undisturbed forest (196 Mg C ha−1) was converted to annual cropping agriculture (146–167 Mg C ha−1), but no significant difference when converted to slash and burn agriculture (185 Mg C ha−1). Data from these studies indicate that there are major differences in the amount of carbon undisturbed forests store as compared to croplands Intermediate values are reported for other land uses.
Soil texture in combination with climate and management are also important factors influencing the activity of soil macro-fauna that produce biogenic structures (Brussaard et al., 2007). Moreover, soil texture also has been reported to be an important regulator of microbial communities in the soil after deforestation. For instance, Crowther et al. (2014) found that clayey soil textures act as a buffer for micro-organisms against altering soil moisture conditions, pH, and nutrients concentrations that may result from forest removal. They reported minimal differences of microbial communities between forest and grassland soils in 11 sites across the United States. The same authors compared their results in the United States to those of Rodrigues et al. (2013) who found that the mean bacterial richness in the soil increased by 47 taxa after forest clearance in western Amazonia. Crowther et al. (2014) found that the species richness of bacteria in the clayey Brazilian soils is relatively stable across sites with different clay textures. These results point at the apparent protective effect of clay textures to micro-fauna even after deforestation. Furthermore, Hassink et al. (1993) reported a higher grazing pressure by nematodes on bacteria in sandy soils than in clayey soils, which in turn was linked to higher N mineralization rates per bacterium. It is recognized that micro-organisms, such as bacteria, might stimulate nutrient cycling, as many are considered to be keystone species (Lupatini et al., 2014). As in the case of CS, clayey textures and their higher soil organic matter content appear to provide protective effects to both soil micro- and macro-fauna.
Concerning HB and land use, the α-diversity of birds, dung beetles, orchid plant bees and ants declines from a gradient of undisturbed forests to mechanized agriculture in Paragominas (undisturbed primary forest > logged primary forest > logged and burnt primary forest > secondary forest > pastures > mechanized agriculture) (Solar et al., 2015, 2016). In addition, Barlow et al. (2016) reported that forest fragmentation and disturbance was associated with the loss of 46–61% of the biodiversity conservation value of forests in Paragominas. Rodrigues et al. (2013), found that in western Amazonia bacterial communities were significantly higher in α-biodiversity in pasture soils than in forest soils. Nevertheless, the opposite was the case for β-biodiversity (i.e., differentiation across space) suggesting a process of biotic homogenization after forest conversion that leads to endemism loss and invasion of broad range taxa. Conversion of primary forests to pastures also has been found to reduce fungal richness and affect community fungal composition of specialized species in the Amazonian region (Mueller et al., 2016). These data were used in our study to estimate HB indices for land use (Table 1).
We also used soil texture as an indicator of agricultural soil quality since clayey soils in the Paragominas region have a greater inherent fertility and water holding capacity, as well as slower rates of nutrient leaching than sandy soils. For this reason, mechanized annual cropping such as soybean, maize, and sorghum production, and forest plantations are typically located on clayey soils, whereas sandy and gravel soils are used for pastures (Piketty et al., 2015). Due to this distribution, our assessment distinguishes areas of crop production located on the plateaus where clayey soils are predominant, areas of forest plantations located both on plateaus and on sandy valleys, and pastures located mainly in the sandy valleys.
The capacity of different land uses to produce soybeans, beef and wood products were assessed in terms of gross profit margin. In 2017, Paragominas soybean exports amounted a total of US$219 million (MDIC, 2018). On the other hand, operation costs of no-till, genetically modified soybean in different parts of Brazil ranges from US$112–132 ton−1 (Goldsmith, 2008; Babajev, 2012). These figures give an estimated production cost of $80 million (exported net weight of soybeans from Paragominas in 2017 was 604 thousand tons). Including transportation costs, estimated at 85$ ton−1 for Mato Grosso (Goldsmith, 2008) signifies a gross profit margin of around 40%. Concerning beef production, the herd in Paragominas consisted of 253 thousand heads in 2017, with an export revenue of US$12.2 million (MDIC, 2018). The total weight of exported frozen meat and edible offal in 2017 was 1,893 and 599 tons, respectively (MDIC, 2018). Using conversion factors of 61% for frozen meat and 42% for edible offal (Holland et al., 2014) we estimated a total of 3,156 and 1,437 tons of live weight, respectively. Taking 545 kg as an average weight for live animal means that the number of heads devoted for exports was around 11,000, equivalent to roughly 4% of the total livestock in Paragominas. The other largest fraction of beef production was destined to local processors in other municipalities such as Belém and Castanhal. Taking US$2 kg−1 (Globo, 2014) as a general market price in the region, this signifies a revenue between US$ 290–320 million. Summing up exports and local market indicates that revenues from beef production in Paragominas amounts to roughly US$330 million. While no data were available on the fraction of the animals that are not exported and remain as livestock or as a commodity in the local market, Somwaru and Valdes (2004) reported a gross profit margin of 24% for beef production in Paragominas. Finally, exports concerning forest products including wood, wood products and charcoal amounted to a total of US$0.64 million in 2017 (MDIC, 2018). In the state of Mina Gerais, the gross profit margin of eucalyptus plantations was estimated at 57% during a period of 7 years equivalent to a mean yearly profit of 8% (de Lima Filho, 2014). Another feasibility study for eucalyptus plantations in the Amazonia reported for a 5-year rotation plantation a profitability index ([Net Present Value + initial investment]/initial investment]) of 4.97. A profitability index larger than 1 indicates the financial attractiveness of a project; however, the payback period of a eucalyptus plantation in the Amazonia is around 5–7 years when destined for pulp and 12 years when destined for energy. Other forest species such as paricá (Schizolobium amazonicum Huber ex. Ducke) are used in the region for plywood production with investment return periods of 7 years in Paragominas (de Paula et al., 2014).
These economic data indicate that soybean has the highest supply of commodity production based on a 40% profit margin and a short period on return on investment (Table 2). Forest plantations can have a high profit margin (57%) but have a return on investment period ranging from 5 to 12 years. Therefore, we rank forest plantations as a medium supply of commodity production. Finally, beef production is ranked as low supply of commodity due to a relative low profit margin. Indeed, the greater profitability of soybean is one of the factors hindering beef production and restoration of degraded pastures in eastern Amazonia (Santos et al., 2007). It is important to acknowledge that this classification depends on price volatility. Due to its inelastic demand soybean is considered as one of the most volatile agricultural commodities. Beef, in contrast, is much less volatile (OECD/FAO, 2018).
Demand for Soil-Based Ecosystem Services
National policies established in the Climate Change Policy (Lei 12.187/2009), the Plan for Prevention and Control of Deforestation in the Legal Amazonia (PPCDAm) and the Low Carbon Agriculture Plan (Plano ABC) of Brazil, include targets to reduce carbon emissions by 37% from 2005 levels by 2025, to end illegal deforestation, to restore 12 M ha of forest by 2030, to restore 15 M ha of degraded pastures by 2030, and to implement 5 M ha of crop-livestock-forest integrated systems by 2030. At the state level, the Plan for Prevention, Control and Alternatives for Deforestation of Pará State (PPCAD-PA) translates this into a 42% reduction target for deforestation in the period 2016–2020 in relation to the previous period from 2011 to 2015. Furthermore, the Green Municipality project in Paragominas advanced a zero-deforestation pact among an array of stakeholders since 2009, which was renewed in 2016 (Vilhena, 2016).
A crucial aspect for our analysis was to determine the implications of national targets at the local level in the absence of an explicit municipal target concerning carbon storage. Paragominas' agricultural sector gross domestic product represents <0.5% (US$95 million in 2016; IBGE, 2018) of the national agricultural primary sector estimated at US$77 billion in 2017 (Ministério da Agricultura, 2016). A calculation based on this proportion would equate to a target of 0.67–0.81 million tons CO2eq for Paragominas (the mitigation potential of the national agricultural sector amounts to 133.9–162.9 M tons CO2eq as estimated in Plano ABC). An estimation based on agricultural area results in a similar value of 0.5% of the total agricultural land in Brazil. These estimates show that based on these criteria, the national target would correspond to a municipal target in the order of magnitude of 0.7 M tons of CO2eq. We did not further identify a spatial variation of this target within the municipality.
The demand for habitat for biodiversity was informed by the habitat requirements stated in the Forest Code (Código Florestal, Lei n° 12.651). It stipulates that all rural properties in the Amazon spare conservation areas known as Legal Reserves equivalent to 80% of the property and preserve all riparian forest (known as APPs, permanent conservation areas) within a distance of 30, 50, 100, 200, and 500 m from rivers, depending on their length. An exception to this rule is contemplated in the Decree No. 7.130/2010, which concerns the Ecological-Economic Zoning. In practice this often means that Legal Reserves within productive areas make up for 50% of the farm. We framed the demand for HB in relation to the regularization plan of each rural property in order to comply with the 80/50% legal requirement.
Regarding the demand for CP, the state development plan, Plano Pará 2030, targets yearly increases of 15–18 and 2–4% for soybean and beef production until 2030, respectively. An important factor for delivering on the intensification of commodity production is market access (Piketty et al., 2015). Paragominas is located near the intersection of two interstate roads of which the federal road BR0-10 is a major transport route for agricultural produce. In recent years, mechanized agriculture and forest plantations have expanded predominantly in areas of clayey soils close to the Paragominas urban center due to easy road access (Piketty et al., 2015). We accounted for these trends by generating a map indicating traveling-time (areas of 1, 2–3, and >3 h) to the city of Paragominas near the main interstate road (BR0-10) to distinguish areas where the demand to increase production is more likely to materialize. Such increases in CP can only take place in areas already cleared since opening new forest areas is forbidden. We identified those areas, where the policy targets to increase production will be more likely to manifest in the landscape, by intersecting our traveling-time map with non-forested areas.
Results
Mapping the Supply and Demand for Ecosystem Services
We identified critical areas of carbon storage to mitigate carbon emissions from deforestation and forest degradation (Figure 5A). Using the Carbon Invest Model and secondary data, total C stocks in Paragominas in the soil and aboveground biomass were estimated at 279 million Mg C. Areas covered by undisturbed forests were estimated to store 247.8 Mg C ha−1, while the lowest values were modeled for croplands (46.2 Mg C ha−1). This indicates that undisturbed forests in Paragominas store 142 million Mg of carbon. Since the demand for CS has been downscaled from the national to the municipal level there is no spatial variation for demand of CS (Figure 5D).
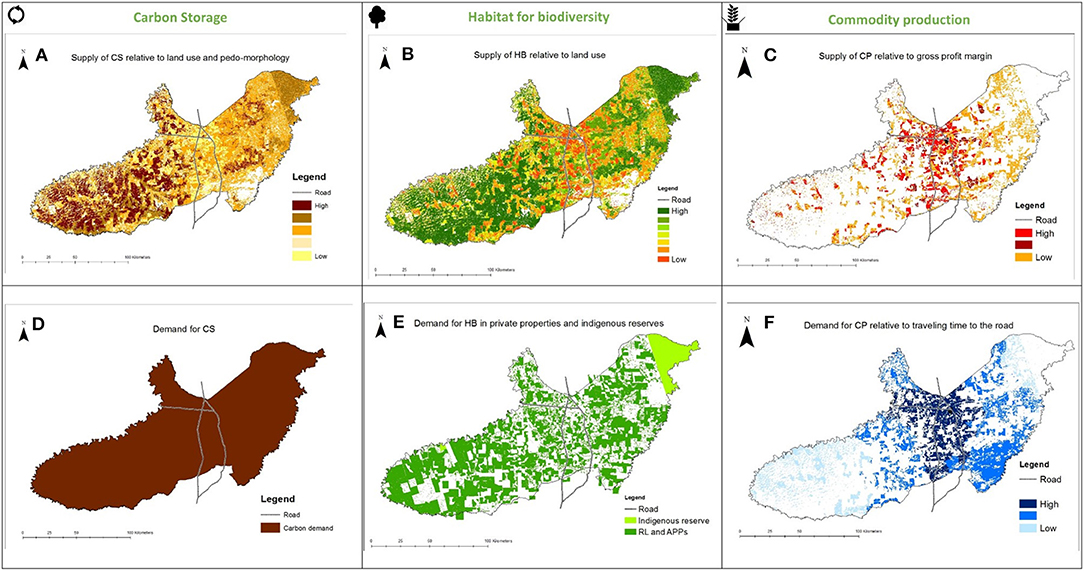
Figure 5. Maps of supply (A–C) and demand (D–F) of carbon storage, habitat for biodiversity, and commodity production in Paragominas.
Regarding the supply of HB, undisturbed forest supports the highest supply of HB (Figure 5B). These areas account for 36% of the total municipality. Areas of lower habitat quality are located mostly close to the city within 1 h away from the main road where agriculture dominates the landscape. Concerning the demand, the total area of Legal Reserves and APPs stipulated in the regularization plans of farms in Paragominas adds up to 1.02 M ha (Figure 5E) and represents 52% of the municipal area. The total forest area in Paragominas is 56% (INPE, 2018), which suggests that in terms of forested area the demand for HB is met in the region.
Regarding CP, the highest supply in terms of gross profit margin takes place on clayey soils located near the main road with soybean production. Forest plantations, which provide intermediate supply, are also concentrated in the central area of the municipality, while pastures for beef production are mostly distributed to the east, in areas more than 1 h away from the road (Figure 5C). Targets framed at the state level represent the demand to increase CP. In areas where mechanization and access to inputs and markets is less costly the demand is higher (Figure 5F). Nevertheless, considering the projected yearly increase of crop and livestock production up to 2030, intensification could also increase in more remote areas. Furthermore, the demand for CP could turn into pressure on forested areas that are located on clayey soils close to the city.
Potential Spatial (Mis)matches and Trade-Offs
Non-CP areas where the supply of CS and HB meet the demand (Mismatch type 1 in Figure 4) represent 32% of the analyzed area (Figure 6). These are areas covered by undisturbed forest on clayey plateaus and on sandy soils (i.e., “Nature areas” in Figure 6). “Production areas” (Mismatch type 8 in Figure 4) represent 18% of the area (shown in blue in Figure 6). These are pastures in sandy valleys and croplands on top of the plateaus. Here, CP is happening at the expense of CS and HB. “Suboptimal areas” (Mismatch type 4 in Figure 4 and indicated in red in Figure 6), represent 49% of the total area and are likely suboptimal for CP as these areas are mostly composed by pastures in floodplains and plateaus and agriculture in sandy valleys. These areas can also be sub-optimal for CS and HB because of forest degradation or abandonment (Figure 6). The identification of trade-off areas in conjunction with the supply-demand maps were the basis to identify possible pathways for landscape multifunctionality (Table 3).
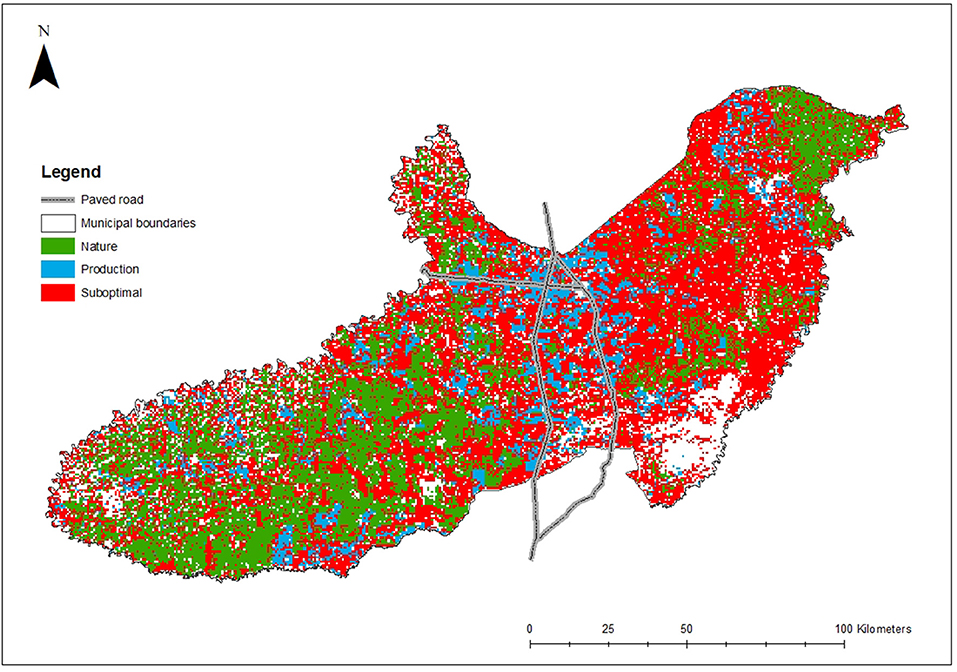
Figure 6. Areas of potential (mis)matches and trade-offs between ecosystem services according to pedo-morphological units and land uses in Paragominas.
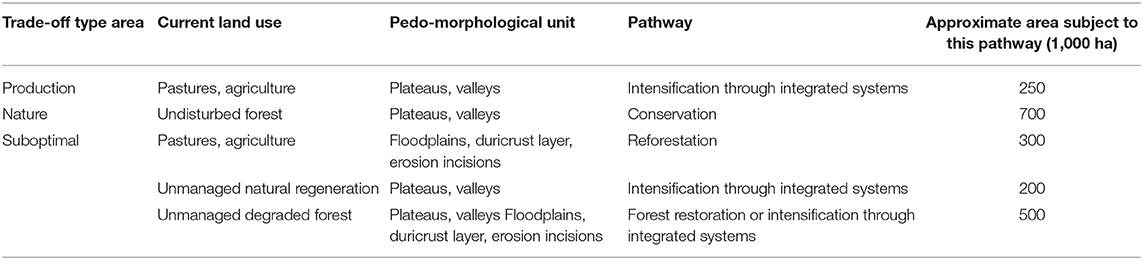
Table 3. Pathways to optimize ecosystem services in Paragominas in terms of current land use and pedo-morphological unit.
Discussion
Meeting Carbon Storage and Biodiversity Conservation Targets
Our analysis indicates that undisturbed forests fulfill the demand for carbon storage (Figure 5A). These critical areas, however, are located on clayey soils that are also suitable for grain production. Thus, even though extensive deforestation has been controlled and minimized in the region, these forests may face pressure in the near future due to forest degradation (Berenguer et al., 2014). Conversely, other land uses such as degraded pastures and croplands with lower carbon stocks offer potential to meet the further demand for carbon storage. For example, it has been estimated that land-use conversion in the tropics, from cropland to pastures, to forest plantations and to secondary forests can increase soil carbon by 20–50% (Guo and Gifford, 2002). Similarly, restoring degraded pastures and implementing silvo-pastoral systems has a storage potential of 1.0 and 1.7 tons C ha−1 year−1, respectively (Gurgel and Costa, 2015). Other studies suggest that integrated silvo-pastoral systems in the Amazonia can store an additional amount of soil carbon (at 1 m depth) of up to 1.9 tons C ha−1 compared to extensive traditional pastures (de Moura Oliveira et al., 2018). Paragominas possesses 300,000 ha of degraded pastures that, if managed and restored, would represent a carbon storage potential of 0.3–0.5 M tons C year−1 (Gurgel and Costa, 2015). This means that Paragominas could theoretically contribute its share of the national target (0.81 million tons of CO2 eq) in only 2–3 years.
Concerning HB, in 2010, 56% of Paragominas was covered by forest (INPE, 2018), 51% consisted of primary forest (including degraded forest), and 5% of natural regeneration (Nunes et al., 2015). According to the Rural Environmental Registry (CAR), 67% of the rural properties of Paragominas comply with the Forest Code requirements, 31% have some pending issue, and only 2% of the properties have been assessed as not meeting the requirements. If we compare these records with the available data from our literature review, we can notice that, for example, most of the rural properties located close to the main road comply with the legislation However, according to the secondary data it is precisely in these areas where the lowest levels of biodiversity can be found (Figure 5B). This suggests that the current legislation was not framed in the most pertinent way to effectively safeguard HB. Indeed, there is an increasing concern that Legal Reserves tend to be disconnected from the network of protected areas (Siqueira et al., 2015) because there are no explicit policy targets concerning forest habitat connectivity at the landscape scale that take into account forest patch size, patch shape, and landscape fragmentation (Oakleaf et al., 2017).
Landscape Multifunctionality, (Mis)matches, and Trade-Offs
In this paper we argue that the trade-offs between ecosystem services in the region emerged from historical agrarian dynamics, i.e., colonization by pioneers from other regions of the country, characterized by land-use changes that ignored key landscape characteristics. It is in this context that we envision a multifunctional landscape as one that matches supply and demand of different ecosystem services through synergistic interactions between multiple ecosystem services. However, attaining these synergies is difficult in complex agroecosystems and therefore FLM aims for the spatial optimization rather than the ubiquitous maximization of all ecosystem services (Schulte et al., 2015).
Our notion of landscape multifunctionality aligns with what Manning et al. (2018) define as ecosystem service multifunctionality as opposed to ecosystem function multifunctionality. The former concerns the supply of an ecosystem service according to the value assigned by human judgement, whereas the latter focuses on the ecological processes. Furthermore, following the classification of landscape multifunctionality proposed by Mastrangelo et al. (2014) (i.e., pattern-based multifunctionality, process-based multifunctionality, socially relevant process-based multifunctionality), our approach is based on a spatial approach in order to identify priority areas (i.e., trade-offs and mismatches) where interventions via land-use change and management practices could be pertinent. However, we also integrate social elements by taking the policy framework as proxies of societal demands, and by taking into account aspects such as infrastructure and markets access. Additionally, our supply matrix (Figure 3) illustrates the concept of landscape multi-functionality in terms of natural processes linked to pedo-morphology and land use driving the supply of each ecosystem service. By applying the FLM framework we integrate elements of the main three multifunctionality categories defined by Mastrangelo et al. (2014) despite the constraints of primary data availability and direct stakeholder participation for ecosystem services selection.
Furthermore, our trade-off map integrates the analysis of CS, HB, and CP by dividing the region into three main types of landscape configurations (Figure 6). Suboptimal areas cover around half of the municipality but encompasses a variety of land uses and covers located in different pedo-morphological areas (Table 3). “Production” and “Nature areas” cover the other half of the region and are typically spatially separated, often located 1 h away and more than 3 h away from the urban center, respectively. We could conjecture that the spatial patterns of these two areas follow a land sparing arrangement. Phalan (2018) defines land sparing as the conservation intervention to increase agricultural productivity and free up land for habitat conservation. Areas of high-intensity practices in Paragominas are expected to be incompatible with biodiversity conservation (due to practices such as heavy use of pesticides) and tend to have a relatively coarse spatial grain (e.g., the average rural property size in the region is 800 ha). At the municipal scale, this results in an emergent pattern of relatively large areas with intensified agricultural production that are spatially separated from high quality habitats for biodiversity conservation. The caveat of this spatial arrangement in Paragominas is that a pure land sparing configuration in tropical contexts is generally followed by a transition to a cleared landscape (Angelsen and Kaimowitz, 2001). Although environmental legislation in Brazil may prevent large-scale forest clearing, forest degradation and habitat disconnection may still be considered a threat to biodiversity conservation in the region.
Our spatially explicit categorization of landscape units and the (mis)matches and trade-offs between ecosystem services may guide the implementation of measures to reorganize the landscape. Our analysis is based on what Driscoll et al. (2013) denominates the “patch-matrix model” of fragmented landscapes, i.e., patches of native vegetation surrounded by a highly modified matrix due to agriculture. Our analysis points out the importance of managing this matrix in order to match supply and demand of ecosystem services. Specifically for biodiversity, the same authors suggest that managing the matrix in a fragmented landscape can have effects on movement and dispersal, resource availability, and the abiotic environment. Therefore, one strategy in Paragominas should be to make the matrix structurally more similar to the remaining forest patches. In this way the landscape could benefit from incorporating a land-sharing approach in “Production” areas to increase forest connectivity in order to enhance species dispersal, food availability and improve microclimatic conditions. This could also be the case for some “Suboptimal” areas where forest enrichment, reforestation, afforestation, or the incorporation of integrated systems such as silvo-pastures or agroforestry could be designed toward a land-sharing approach. A focus on the catchment level may be required to explore new conservation strategies that take into account matrix heterogeneity in space and time, and adaptation of species to changing landscape conditions (Driscoll et al., 2013). Such measures to make the matrix resemble patches of natural vegetation could simultaneously increase the supply of carbon storage.
On the other hand, a land-sparing format for biodiversity conservation could be kept in “Nature areas” and in “Suboptimal” areas that are unsuitable for agricultural production. This mosaic of land-sharing/sparing based on our maps could reconcile what some authors point out as the false dichotomy between land sharing and sparing (Renwick and Schellhorn, 2016). Kremen (2015) calls this a “both-and” design characterized by the establishment of large protected areas able to host endemism and specialist species, and a matrix of agricultural landscapes surrounding conservation areas allowing for species dispersal and room for human activities.
Pathways to Match Supply and Demand of Ecosystem Services
Following this “both-and” logic, we distinguish two pathways to match supply and demand of ecosystem services, while minimizing trade-offs in Paragominas. A first pathway centers on agricultural management at the field scale. For example, land for soybean production under conservation agriculture practices is expected to yield different results on the supply of CS or HB compared to soybean monoculture under conventional practices (Corbeels et al., 2006; de Pontes et al., 2017). Thus, a farmer aiming to increase grain production on a clayey plateau can adopt intensification measures such as precision agriculture, no-tillage, or crop-livestock integration to increase commodity production without compromising the supply of CS and HB (Corbeels et al., 2006; Maia et al., 2010; Sentelhas et al., 2015; Gil et al., 2016). A second example includes pastures that are integrated into silvo-pastoral systems to increase the production of meat and timber, while at the same time increasing CS and HB. Tree-livestock-crop integrated systems may foster positive interactions between different ecosystem services and resource-use efficiency in the Amazon and the Cerrado (Campos et al., 2015; da Conceição et al., 2017; Stark et al., 2017; Gil et al., 2018). Considering the supply matrix (Figure 3), this pathway means that within a landscape unit (e.g., mechanized agriculture on clayey plateaus) the supply of all ecosystem services can be increased (i.e., the size of the bubbles) by adopting management practices that modify soil properties as well as energy, nutrient and water fluxes at the field and farm level. This in turn, can lead to an agricultural matrix that resembles as much as possible the ecological functionality of the forest. Importantly, the absence of management as a result of abandonment can also play a role in the supply of ecosystem services in the region. For instance, unmanaged pastures can lead to natural regeneration and subsequent increases in botanic biodiversity (Poccard-Chapuis et al., 2014). Conversely, the absence of management of degraded forest can have detrimental effects on carbon stocks due to the incidence of forest fires (Berenguer et al., 2014).
A second pathway concerns land-use changes. In terms of the supply matrix (Figure 3), this implies a displacement from one landscape unit to another, e.g., from natural regeneration in clayey soils to crop land on clayey soils. These land-use changes should aim at increasing forest connectivity. Under this scenario, trade-offs between CP and CS-HB are minimized by prioritizing conservation in selected areas that have less potential to produce commodities (i.e., “Suboptimal” area). Suboptimal areas where production has less potential (i.e., natural regeneration in sandy valleys close to water bodies) could also be prioritized for conservation to minimize trade-offs at the landscape scale. This pathway, however, has the challenge of managing at a higher scale than the farm, the main operational level of functional organization in the region (Poccard-Chapuis et al., 2014).
A critical point for optimizing ecosystem services is to distinguish where to follow which pathway. This revolves around the question of where the clayey soils, roads, forests, and cleared areas are located because this will determine the aptitude of a particular area to perform certain ecosystem service. Therefore, optimization of the landscape means that intensification activities with emphasis on integrated systems should be placed in open areas without forests located on plateaus and in valleys (“Production” areas and “Suboptimal” areas with natural regeneration). These areas account for 20–25% of the municipality. Reforestation should be prioritized to increase forest connectivity in areas with low production potential, such as “Suboptimal” areas on floodplains (10–15% of the municipality). “Nature” areas (a third of the total area) should be kept protected, while forest restoration should be implemented in areas of forest degradation with low production potential covering between 10 and 15% of the territory. This leaves between 15 and 20% of “Suboptimal” areas with degraded forest located on plateaus or in valleys that could have potential for production or forest restoration (Table 3).
As pointed out by Schulte et al. (2015), it is important to acknowledge that FLM is not a call for a top-down approach to impose a zoning in the region. Rather, we intend to offer tools that foment land-use planning discussions that go beyond the farm level and take into consideration biophysical aspects that require a landscape approach, for example, at the catchment level. The development and implementation of pathways through the identification of trade-off areas may involve a highly complex governance challenge that requires the engagement of all relevant actors in the region. Given the recent success story of a societal agreement toward environmental compliance in Paragominas through the “Green Municipality” project (Viana et al., 2016), the region appears in a good position to embark in such a task.
The development of these pathways is the subject of further research under the approach of what Huppes and Ishikawa (2007) define as environmental cost-effectiveness; that is, an environmental prime as the criteria to rearrange the landscape. These could potentially counter the “artificialization” of the landscape in Paragominas (Poccard-Chapuis et al., 2015) and move toward more endogenous landscape systems that are better at fulfilling the demands for ecosystem services in a way that is both ecologically and economically sustainable. One of the main challenges in this regard is to make the jurisdictional approach operational to attain landscape governance given the diversity of scales, actors, and demands (Pacheco et al., 2017). What are the options within conventional, resource-intensive agriculture to introduce spatial changes oriented toward the transition of food systems? Can land-use planning offer a way to contribute to this transition? Does matching the supply and demand of ecosystem services offer a model of ecological intensification in a tropical post-forest frontier? As pointed out by Tittonell (2014), processes of ecological intensification require a landscape approach that takes nature as an active ally. Contrasting the supply and demand for ecosystem services offers a hint of what the current role of nature is in the landscape of Paragominas and the possible pathways to be implemented at the farm level to “work with nature” and attain landscape multifunctionality.
Limitations
A few limitations in our approach emerge from our conceptualization of landscape multifunctionality. First, we established a (mis)match in terms of policy targets that we take as a proxy of societal demands (Schulte et al., 2015). These targets were established at federal and state level and do not necessarily reflect the actual demand and use of ecosystem services by local stakeholders. In the original case study conducted in Ireland, FLM takes policy targets as societal demands as Ireland has policies explicitly stating agricultural and environmental targets. However, the representativeness of policies in a relatively small country like Ireland as compared to a country with continental scales such as Brazil can vary greatly. For example, in 2018, the Economist Intelligence Unit's Democracy Index placed Ireland in the 6th place worldwide with a score of 8.33 for political participation, while Brazil ranks number 50 with a score of 5.00 (The Economist Intelligence Unit, 2019). Assuming the same policy-making process in such a different context as Brazil can be misleading but given the complexity of all the different governance layers that affect land use in eastern Amazonia, these policy targets become a useful starting point to examine the relationships between international, national, and local demands that shape the landscape of Paragominas. This paper thus, does not consider direct stakeholder participation because it attempts to provide an overarching panorama of the landscape in terms of ecosystem services and environmental policies. Further studies on the comparison of policy targets and the perception and actual demand of ecosystem services by stakeholders could provide valuable insights to what extent demands for ecosystem services at policy levels align with demands by other stakeholders.
A second limitation of our study is that we considered only three particular ecosystem services. The selected ecosystem services have the advantage that their supply can be upscaled with a reasonable degree of reliability from a landscape unit to the whole landscape. Other ecosystem services, such as nutrient cycling, water regulation, pollination, and pest control and cultural ecosystem services are equally important but involve spatial aspects or complexities that make upscaling from plot to landscape level fraught with uncertainties (Manning et al., 2018). For example, insect-mediated ecosystem services, such as natural pest control is governed by the dispersal ability and behavior of natural enemies, which are species-specific and hard to generalize (Karp et al., 2018). Ecosystem services associated with water and nutrient flows pertain complexities, such as vertical distances from tree to soil, livestock mobility, and surface infiltration (Poccard-Chapuis et al., 2014; Lavelle et al., 2016). Cultural ecosystem services are often bundled with food values and are difficult to localize and to correlate with other ecosystem services (Cooper et al., 2016). Our three focal ecosystem services on the other hand, can be quantified at large spatial scales and enables identifying “pressure points” (i.e., mismatches) at relevant spatial scales for policy making.
A third limitation of this study is our reliance on only secondary data. By restricting our literature review on studies from the Amazon and Cerrado biome we harnessed the context-specificity of CS and HB data as much as possible. The supply of CP was estimated in part from studies from more remote areas and from different years over the last 15 years. Our results, therefore, should be interpreted with caution, and used as indicators of spatial patterns (i.e., areas of trade-offs between ecosystems), rather than interpreted as geo-referenced quantification of ecosystem services.
Conclusions
In this paper we defined landscape multifunctionality in terms of the values that are assigned to different functions by different stakeholders. However, the landscape of Paragominas is conditioned by different sets of values: from environmental to productivity-oriented, from global to local, from a desire to urbanize to a call for pristine wilderness in the region. How can the landscape satisfy all these values?
Combining land-sparing and sharing formats can generate a multifunctional mosaic that satisfies as much values as possible in line with the philosophy of Functional Land Management. This could also provide enough flexibility to cope with the bio-physical heterogeneity, while taking into account aspects such as land tenure and accessibility. This strategy, however, may require that conservation areas are delimitated beyond the boundaries of a single rural property (e.g., the watershed level).
Ultimately, the multifunctionality of the landscape ought to be constructed by the decision makers of the region who are local farmers in the first place deciding based on individual values. In this paper we provide a delimitation of three areas at the municipal level characterized by different trade-offs between ecosystem services. This is intended as a source of terms, concepts and data, around which stakeholders can gather around in order to engage in a complex decision-making process toward enhancing landscape multi-functionality in Paragominas.
Data Availability Statement
The datasets generated for this study are available on request to the corresponding author.
Author Contributions
DP and RS conceived the idea. DP and FB conducted the analysis. DP, FB, RP-C, MC, PT, and RS wrote the manuscript.
Conflict of Interest
The authors declare that the research was conducted in the absence of any commercial or financial relationships that could be construed as a potential conflict of interest.
Acknowledgments
We thank two reviewers and the editor whose suggestions helped improve this manuscript. This work has been supported by the European Commission under the Erasmus Mundus Joint Doctorate Program AgTraIn (Agricultural Transformation by Innovation) and by the EU Horizon 2020 LANDMARK (Land Management: Assessment, Research, Knowledge Base) project.
References
Angelsen, A., and Kaimowitz, D. (2001). Agricultural Technologies and Tropical Deforestation. New York, NY: CABI. doi: 10.1079/9780851994512.0000
Azevedo, A. A., Rajão, R., Costa, M. A., Stabile, M. C. C., Macedo, M. N., dos Reis, T. N. P., et al. (2017). Limits of Brazil's Forest Code as a means to end illegal deforestation. Proc. Natl. Acad. Sci. U.S.A. 114, 7653–7658. doi: 10.1073/pnas.1604768114
Babajev, N. (2012). Financial analysis of soybean and beef production chains between the EU and Latin America (M.Sc. thesis Business Economics). Wageningen University and Research.
Barlow, J., Lennox, G. D., Ferreira, J., Berenguer, E., Lees, A. C., Nally, R. M., et al. (2016). Anthropogenic disturbance in tropical forests can double biodiversity loss from deforestation. Nature 535, 144–147. doi: 10.1038/nature18326
Barretto, A. G. O. P., Berndes, G., Sparovek, G., and Wirsenius, S. (2013). Agricultural intensification in Brazil and its effects on land-use patterns: an analysis of the 1975–2006 period. Glob. Chang. Biol. 19, 1804–1815. doi: 10.1111/gcb.12174
Barthem, R., Charvet-Almeida, P., Montag, L., and Lanna, A. (2004). Global International Waters Assessment Amazon Basin, GIWA Regional Assessment 40 b. GIWA Regional Assessment Reports, 76.
Behling, M., Piketty, M. G., Morello, T. F., Bouillet, J.-P., Laclau, J.-P., and Mesquita Neto, F. (2011). Eucalyptus Plantations and the Steel Industry in Amazonia - A Contribution From the 3-PG Model. Available online at: https://inis.iaea.org/collection/NCLCollectionStore/_Public/48/026/48026267.pdf?r=1&r=1
Berenguer, E., Ferreira, J., Gardner, T. A., Aragão, L. E. O. C., De Camargo, P. B., Cerri, C. E., et al. (2014). A large-scale field assessment of carbon stocks in human-modified tropical forests. Glob. Chang. Biol. 20, 3713–3726. doi: 10.1111/gcb.12627
Brussaard, L., Pulleman, M. M., Ouédraogo, É., Mando, A., and Six, J. (2007). Soil fauna and soil function in the fabric of the food web. Pedobiologia 50, 447–462. doi: 10.1016/j.pedobi.2006.10.007
Campos, D. T., Stieven, A. C., and Wruck, F. J. (2015). “Microbial biomass carbon dynamics in soil under crop-livestock-forest in northern of Mato Grosso, Brazil,” in Embrapa Arroz e Feijão-Resumo em Anais de Congresso (ALICE) (Brasilia: World Congress on Integrated Crop-Livestock-Forest Systems).
Cooper, N., Brady, E., Steen, H., and Bryce, R. (2016). Aesthetic and spiritual values of ecosystems: recognising the ontological and axiological plurality of cultural ecosystem ‘services’. Ecosyst. Services 21, 218–229. doi: 10.1016/j.ecoser.2016.07.014
Corbeels, M., Scopel, E., Cardoso, A., Bernoux, M., Douzet, J.-M., and Neto, M. S. (2006). Soil carbon storage potential of direct seeding mulch-based cropping systems in the Cerrados of Brazil. Glob. Chang. Biol. 12, 1773–1787. doi: 10.1111/j.1365-2486.2006.01233.x
Coyle, C., Creamer, R. E., Schulte, R. P. O., O'Sullivan, L., and Jordan, P. (2016). A Functional Land Management conceptual framework under soil drainage and land use scenarios. Environ. Sci. Policy 56, 39–48. doi: 10.1016/j.envsci.2015.10.012
Crowther, T. W., Maynard, D. S., Leff, J. W., Oldfield, E. E., McCulley, R. L., Fierer, N., et al. (2014). Predicting the responsiveness of soil biodiversity to deforestation: a cross-biome study. Glob. Chang. Biol. 20, 2983–2994. doi: 10.1111/gcb.12565
da Conceição, M. C., MATOS, E. S., Bidone, E. D., Rodrigues, R., and Cordeiro, R. C. (2017). Changes in soil carbon stocks under Integrated Crop-Livestock-Forest system in the Brazilian Amazon Region. Agric. Sci. 8, 904–913. doi: 10.4236/as.2017.89066
de Lima Filho, R. R. (2014). Rentabilidade da Produção de Eucalipto no Brasil [Online]. Scot Consultoria: Scot Consultoria. Available online at: https://www.scotconsultoria.com.br/noticias/artigos/39247/rentabilidade-da-producao-de-eucalipto-no-brasil.htm (accessed November 21, 2018).
de Moura Oliveira, J., Madari, B. E., de Melo Carvalho, M. T., Assis, P. C. R., Silveira, A. L. R., de Leles Lima, M., et al. (2018). Integrated farming systems for improving soil carbon balance in the southern Amazon of Brazil. Region. Environ. Change 18, 105–116. doi: 10.1007/s10113-017-1146-0
de Paula, M. T., Pontes, A. N., Ferreira Filho, H. R., Cardoso, L. A., Silva, I. M., Damasceno, M. C. S., et al. (2014). Economic viability of production of tree paricá (Schizolobium amazonicum Huber ex. Ducke) of reforestation project in the municipality paragominas-PA, Brazil. J. Life Sci. 8, 967–971. doi: 10.17265/1934-7391/2014.12.006
de Pontes, J. S., Oehl, F., Pereira, C. D., de Toledo Machado, C. T., Coyne, D., da Silva, D. K. A., et al. (2017). Diversity of arbuscular mycorrhizal fungi in the Brazilian's Cerrado and in soybean under conservation and conventional tillage. Appl. Soil Ecol. 117–118, 178–189. doi: 10.1016/j.apsoil.2017.04.023
Driscoll, D. A., Banks, S. C., Barton, P. S., Lindenmayer, D. B., and Smith, A. L. (2013). Conceptual domain of the matrix in fragmented landscapes. Trends Ecol. Evol. 28, 605–613. doi: 10.1016/j.tree.2013.06.010
Durigan, M. R., Cherubin, M. R., de Camargo, P. B., Ferreira, J. N., Berenguer, E., Gardner, T. A., et al. (2017). Soil organic matter responses to anthropogenic forest disturbance and land use change in the Eastern Brazilian Amazon. Sustainability 9:379. doi: 10.3390/su9030379
Eitelberg, D. A., van Vliet, J., and Verburg, P. H. (2015). A review of global potentially available cropland estimates and their consequences for model-based assessments. Glob. Chang. Biol. 21, 1236–1248. doi: 10.1111/gcb.12733
Fearnside, P. (2017). Business as Usual: A Resurgence of Deforestation in the Brazilian Amazon [Online]. Yale Environment 360: Yale School of Forestry & Environmental Studies. Available online at: https://e360.yale.edu/features/business-as-usual-a-resurgence-of-deforestation-in-the-brazilian-amazon (accessed October 1, 2019). doi: 10.1093/acrefore/9780199389414.013.102
Feller, C., and Beare, M. (1997). Physical control of soil organic matter dynamics in the tropics. Geoderma 79, 69–116. doi: 10.1016/S0016-7061(97)00039-6
Gallopín, G., Funtowicz, S., O'Connor, M., and Ravetz, J. (2001). Science for the twenty-first century: from social contract to the scientific core. Int. Soc. Sci. J. 53, 219–229. doi: 10.1111/1468-2451.00311
Gil, J. D., Garrett, R. D., Rotz, A., Daioglou, V., Valentim, J., Pires, G. F., et al. (2018). Tradeoffs in the quest for climate smart agricultural intensification in Mato Grosso, Brazil. Environ. Res. Lett. 13:064025. doi: 10.1088/1748-9326/aac4d1
Gil, J. D. B., Garrett, R., and Berger, T. (2016). Determinants of crop-livestock integration in Brazil: evidence from the household and regional levels. Land Use Policy 59, 557–568. doi: 10.1016/j.landusepol.2016.09.022
Globo (2014). Falta de bovinos para abate aumenta preços da arroba em Mato Grosso [Online]. Agrodebate: Globo Agrodebate. Available online at: http://g1.globo.com/mato-grosso/agrodebate/noticia/2014/11/falta-de-bovinos-para-abate-aumenta-precos-da-arroba-em-mato-grosso.html (accessed December 2, 2018).
Goldsmith, P. D. (2008). “21 - Soybean production and processing in Brazil,” in Soybeans Chemistry, Production, Processing, and Utilization, eds L. A. Johnson, P. J. White, and R. Galloway (Urbana, IL: AOCS Press), 773–798. doi: 10.1016/B978-1-893997-64-6.50024-X
Guild, L. S., Kauffman, J. B., Ellingson, L. J., Cummings, D. L., Castro, E. A., Babbitt, R. E., et al. (1998). Dynamics associated with total aboveground biomass, C, nutrient pools, and biomass burning of primary forest and pasture in Rondônia, Brazil during SCAR-B. J. Geophys. Res. 103, 32091–32100. doi: 10.1029/98jd00523
Guo, L. B., and Gifford, R. M. (2002). Soil carbon stocks and land use change: a meta-analysis. Glob. Chang. Biol. 8, 345–360. doi: 10.1046/j.1354-1013.2002.00486.x
Gurgel, A. C., and Costa, C. F. (2015). Análise dos Recursos do Programa ABC: Foco na Amazônia Legal: Potencial de Redução de GEE e Estudo de Caso Sobre o Programa ABC em Paragominas. Relatório 4, ABC Observatório Agricultura de Baixo Carbono, 55.
Hassink, J., Bouwman, L. A., Zwart, K. B., Bloem, J., and Brussaard, L. (1993). Relationships between soil texture, physical protection of organic matter, soil biota, and c and n mineralization in grassland soils. Geoderma 57, 105–128. doi: 10.1016/0016-7061(93)90150-J
Holland, R., Loveday, D., and Ferguson, K. (2014). “How much meat to expect from a beef carcass,” in UT Extension PB1822 (University of Tennessee Institute of Agriculture).
Holt-Giménez, E., Shattuck, A., Altieri, M., Herren, H., and Gliessman, S. (2012). We already grow enough food for 10 billion people… and still can't end hunger. J. Sustain. Agric. 36, 595–598. doi: 10.1080/10440046.2012.695331
Huppes, G., and Ishikawa, M. (2007). “An introduction to quantified eco-efficiency analysis,” in Quantified Eco-Efficiency: An Introduction with Applications, eds G. Huppes and M. Ishikawa (Dordrecht: Springer Netherlands), 1–38. doi: 10.1007/1-4020-5399-1_1
IBGE (2016). Pará > Paragominas > Municipal Agricultural Production - Temporary Crops - 2014 [Online]. IBGE. Available online at: http://www.cidades.ibge.gov.br/xtras/temas.php?lang=_EN&codmun=150550&idtema=149&search=par%C3%A1%7Cparagominas%7Cmunicipal-agricultural-production-temporary-crops (accessed February 25, 2016).
IBGE (2018). Produto Interno Bruto dos Municípios [Online]. IBGE. Available online at: https://www.ibge.gov.br/estatisticas-novoportal/economicas/contas-nacionais/9088-produto-interno-bruto-dos-municipios.html?=&t=resultados (accessed November 27, 2018).
INPE (2018). Monitoramento da Floresta Amazônica Brasileira por Satélite [Online]. INPE. Available online at: http://www.obt.inpe.br/OBT/assuntos/programas/amazonia/prodes (accessed November 2, 2018).
Karp, D. S., Chaplin-Kramer, R., Meehan, T. D., Martin, E. A., DeClerck, F., Grab, H., et al. (2018). Crop pests and predators exhibit inconsistent responses to surrounding landscape composition. Proc. Natl. Acad. Sci. U.S.A. 115, E7863–E7870. doi: 10.1073/pnas.1800042115
Kremen, C. (2015). Reframing the land-sparing/land-sharing debate for biodiversity conservation. Ann. N. Y. Acad. Sci. 1355, 52–76. doi: 10.1111/nyas.12845
Laurent, F., Poccard-Chapuis, R., Plassin, S., and Pimentel Martinez, G. (2017). Soil texture derived from topography in North-eastern Amazonia. J. Maps 13, 109–115. doi: 10.1080/17445647.2016.1266524
Lavelle, P., Dolédec, S., de Sartre, X. A., Decaëns, T., Gond, V., Grimaldi, M., et al. (2016). Unsustainable landscapes of deforested Amazonia: an analysis of the relationships among landscapes and the social, economic and environmental profiles of farms at different ages following deforestation. Glob. Environ. Change 40, 137–155. doi: 10.1016/j.gloenvcha.2016.04.009
Lupatini, M., Suleiman, A. K. A., Jacques, R. J. S., Antoniolli, Z. I., de Siqueira Ferreira, A., Kuramae, E. E., et al. (2014). Network topology reveals high connectance levels and few key microbial genera within soils. Front. Environ. Sci. 2:10. doi: 10.3389/fenvs.2014.00010
Macedo, M. N., DeFries, R. S., Morton, D. C., Stickler, C. M., Galford, G. L., and Shimabukuro, Y. E. (2012). Decoupling of deforestation and soy production in the southern Amazon during the late 2000s. Proc. Natl. Acad. Sci. U.S.A. 109, 1341–1346. doi: 10.1073/pnas.1111374109
Maia, S. M. F., Ogle, S. M., Cerri, C. C., and Cerri, C. E. P. (2010). Changes in soil organic carbon storage under different agricultural management systems in the Southwest Amazon Region of Brazil. Soil Till. Res. 106, 177–184. doi: 10.1016/j.still.2009.12.005
Manning, P., van der Plas, F., Soliveres, S., Allan, E., Maestre, F. T., Mace, G., et al. (2018). Redefining ecosystem multifunctionality. Nat. Ecol. Evol. 2, 427–436. doi: 10.1038/s41559-017-0461-7
Maquère, V., Laclau, J.-P., Bernoux, M., Saint-Andre, L., Gonçalves, J. L. M., Cerri, C. C., et al. (2008). Influence of land use (savanna, pasture, Eucalyptus plantations) on soil carbon and nitrogen stocks in Brazil. Eur. J. Soil Sci. 59, 863–877. doi: 10.1111/j.1365-2389.2008.01059.x
Mastrangelo, M., Weyland, F., Villarino, S., Barral, M., Nahuelhual, L., and Laterra, P. (2014). Concepts and methods for landscape multifunctionality and a unifying framework based on ecosystem services. Landsc. Ecol. 29, 345–358. doi: 10.1007/s10980-013-9959-9
MDIC (2018). Comex stat. Exports and imports of Cities [Online]. Ministério da Indústria, Comércio Exterior e Serviços: Ministério da Indústria, Comércio Exterior e Serviços. Available online at: http://comexstat.mdic.gov.br/en/municipio (accessed November 23, 2018).
Ministério da Agricultura, Pecuária e Abastecimento. (2016). Agropecuária Cresceu 13% em 2017 [Online]. Ministério da Agricultura, Pecuária e Abastecimento.,Available online at: http://www.agricultura.gov.br/noticias/agropecuaria-cresceu-13-em-2017 (accessed December 12, 2018).
Mueller, R. C., Rodrigues, J. L. M., Nüsslein, K., and Bohannan, B. J. M. (2016). Land use change in the Amazon rain forest favours generalist fungi. Funct. Ecol. 30, 1845–1853. doi: 10.1111/1365-2435.12651
Murray, F., Baldi, G., von Bernard, T., Viglizzo, E. F., and Jobbágy, E. G. (2016). Productive performance of alternative land covers along aridity gradients: ecological, agronomic and economic perspectives. Agricult. Syst. 149, 20–29. doi: 10.1016/j.agsy.2016.08.004
Nepstad, D., McGrath, D., Stickler, C., Alencar, A., Azevedo, A., Swette, B., et al. (2014). Slowing Amazon deforestation through public policy and interventions in beef and soy supply chains. Science 344, 1118–1123. doi: 10.1126/science.1248525
Nepstad, D., Uhl, C., and Serrão, E. A. S. (1991). Recuperation of a degraded Amazonian landscape: forest recovery and agricultural restoration. Ambio 20, 248–255.
Nepstad, D., Verssimo, A., Alencar, A., Nobre, C., Lima, E., Lefebvre, P., et al. (1999). Large-scale impoverishment of Amazonian forests by logging and fire. Nature 398, 505–508. doi: 10.1038/19066
Neto, M. S., Scopel, E., Corbeels, M., Cardoso, A. N., Douzet, J.-M., Feller, C., et al. (2010). Soil carbon stocks under no-tillage mulch-based cropping systems in the Brazilian Cerrado: an on-farm synchronic assessment. Soil Till. Res. 110, 187–195. doi: 10.1016/j.still.2010.07.010
Nunes, S., Barlow, J., Gardner, T., Sales, M., Monteiro, D., and Souza, C. (2019). Uncertainties in assessing the extent and legal compliance status of riparian forests in the eastern Brazilian Amazon. Land Use Policy 82, 37–47. doi: 10.1016/j.landusepol.2018.11.051
Nunes, S. S., Barlow, J. O. S., Gardner, T. A., Siqueira, J. V., Sales, M. R., and Souza, C. M. (2015). A 22 year assessment of deforestation and restoration in riparian forests in the eastern Brazilian Amazon. Environ. Conserv. 42, 193–203. doi: 10.1017/S0376892914000356
Oakleaf, J. R., Matsumoto, M., Kennedy, C. M., Baumgarten, L., Miteva, D., Sochi, K., et al. (2017). LegalGEO: conservation tool to guide the siting of legal reserves under the Brazilian Forest Code. Appl. Geogr. 86, 53–65. doi: 10.1016/j.apgeog.2017.06.025
OECD/FAO, (ed.). (2018). OECD-FAO Agricultural Outlook 2018-2027. Paris; Rome: OECD Publishing; Food and Agriculture Organization of the United Nations.
Pacheco, P., Hospes, O., and Dermawan, A. (2017). Zero Deforestation and Low Emissions Development: Public and Private Institutional Arrangements Under Jurisdictional Approaches. Bogor: Center for International Forestry Research (CIFOR).
Pereira, T., Rangel, R., Rodrigues, A., Azevedo, R., and Pena, H. (2011). Análise de Viabilidade Econômica de uma Plantação de Eucalipto no Pará-Amazônia-Brasil. Málaga: Observatorio de la Economía Latinoamericana.
Phalan, B. T. (2018). What have we learned from the land sparing-sharing model? Sustainability 10:1760. doi: 10.3390/su10061760
Piketty, M.-G., Poccard-Chapuis, R., Drigo, I., Coudel, E., Plassin, S., Laurent, F., et al. (2015). Multi-level governance of land use changes in the Brazilian Amazon: Lessons from Paragominas, State of Pará. Forests 6, 1516–1536. doi: 10.3390/f6051516
Poccard-Chapuis, R., Carvalho, S., Burlamaqui, A., Navegantes, L., Plassin, S., El Husny, J., et al. (2015). Des cendres de la forêt à l'économie verte, l'évolution agraire en Amazonie orientale traduit-elle un mouvement d'intensification écologique. Fourrages 222, 125–133. Available online at: http://agritrop.cirad.fr/589696/1/F222%20-Poccard.pdf
Poccard-Chapuis, R., Navegantes Alves, L., Grise, M. M., Bâ, A., Coulibaly, D., Ferreira, L. A., et al. (2014). Landscape characterization of integrated crop-livestock systems in three case studies of the tropics. Renew. Agricult. Food Syst. 29, 218–229. doi: 10.1017/S174217051400009X
Renwick, A., and Schellhorn, N. (2016). “A perspective on land sparing versus land sharing,” in Learning From Agri-Environment Schemes in Australia, eds D. Ansell, F. Gibson, and D. Salt (Acton, ACT: ANU Press), 117–126. doi: 10.22459/LFAESA.05.2016.09
Rodrigues, J. L. M., Pellizari, V. H., Mueller, R., Baek, K., Jesus, E. C., Paula, F. S., et al. (2013). Conversion of the Amazon rainforest to agriculture results in biotic homogenization of soil bacterial communities. Proc. Natl. Acad. Sci. U.S.A. 110, 988–993. doi: 10.1073/pnas.1220608110
Rodrigues, L. W., Maklouf, C. E. J., and Texeira, S. L.G. (2014). Diagnóstico Agrícola do Município de Paragominas, PA. Belém: Empraba Amazônia Oriental.
Rodrigues, T. E., Silva, R. C., da Silva, J. M., de Oliveira, J. R. C., Gama, J. R. F., and Valente, M. A. (2003). Caracterização e Classificação dos Solos do Município de Paragominas, Estado do Pará. Belém: Embrapa Amazônia Oriental-Documentos (INFOTECA-E).
Santos, M., da Santos, J., Cunha, S., and Santana, A. (2007). Mercado e Dinâmica Local da Cadeia Produtiva da Pecuária de Corte na Região Norte. Belém: BASA.
Schielein, J., and Börner, J. (2018). Recent transformations of land-use and land-cover dynamics across different deforestation frontiers in the Brazilian Amazon. Land Use Policy 76, 81–94. doi: 10.1016/j.landusepol.2018.04.052
Schulte, R. P., Bampa, F., Bardy, M., Coyle, C., Creamer, R. E., Fealy, R., et al. (2015). Making the most of our land: managing soil functions from local to continental scale. Front. Environ. Sci. 3:81. doi: 10.3389/fenvs.2015.00081
Schulte, R. P., Creamer, R. E., Donnellan, T., Farrelly, N., Fealy, R., O'Donoghue, C., et al. (2014). Functional land management: a framework for managing soil-based ecosystem services for the sustainable intensification of agriculture. Environ. Sci. Policy 38, 45–58. doi: 10.1016/j.envsci.2013.10.002
Sentelhas, P. C., Battisti, R., Camara, G. M. S., Farias, J. R. B., Hampf, A. C., and Nendel, C. (2015). The soybean yield gap in Brazil - magnitude, causes and possible solutions for sustainable production. J. Agric. Sci. 153, 1394–1411. doi: 10.1017/S0021859615000313
Sharp, R., Tallis, H., Ricketts, T., Guerry, A., Wood, S., Chaplin-Kramer, R., et al. (2014). InVEST User's Guide. Stanford, CA: The Natural Capital Project.
Silver, W. L., Neff, J., McGroddy, M., Veldkamp, E., Keller, M., and Cosme, R. (2000). Effects of soil texture on belowground carbon and nutrient storage in a lowland Amazonian forest ecosystem. Ecosystems 3, 193–209. doi: 10.1007/s100210000019
Siqueira, A., Ricaurte, L. F., Borges, G. A., Nunes, G. M., and Wantzen, K. M. (2015). The role of private rural properties for conserving native vegetation in Brazilian Southern Amazonia. Region. Environ. Change 18, 21–32. doi: 10.1007/s10113-015-0824-z
Six, J., Conant, R. T., Paul, E. A., and Paustian, K. (2002). Stabilization mechanisms of soil organic matter: implications for C-saturation of soils. Plant Soil 241, 155–176. doi: 10.1023/A:1016125726789
Solar, R. R. C., Barlow, J., Andersen, A. N., Schoereder, J. H., Berenguer, E., Ferreira, J. N., et al. (2016). Biodiversity consequences of land-use change and forest disturbance in the Amazon: a multi-scale assessment using ant communities. Biol. Conserv. 197(Suppl. C), 98–107. doi: 10.1016/j.biocon.2016.03.005
Solar, R. R. C., Barlow, J., Ferreira, J., Berenguer, E., Lees, A. C., Thomson, J. R., et al. (2015). How pervasive is biotic homogenization in human-modified tropical forest landscapes? Ecol. Lett. 18, 1108–1118. doi: 10.1111/ele.12494
Sommer, R., Denich, M., and Vlek, P. L. G. (2000). Carbon storage and root penetration in deep soils under small-farmer land-use systems in the Eastern Amazon region, Brazil. Plant Soil 219, 231–241. doi: 10.1023/A:1004772301158
Somwaru, A., and Valdes, C. (2004). Brazil's Beef Production and Its Efficiency: A Comparative Study of Scale Economies. West Lafayette, IN: Global Trade Analysis Project (GTAP).
Stark, F., González-García, E., Navegantes, L., Miranda, T., Poccard-Chapuis, R., Archimède, H., et al. (2017). Crop-livestock integration determines the agroecological performance of mixed farming systems in Latino-Caribbean farms. Agron. Sustain. Dev. 38:4. doi: 10.1007/s13593-017-0479-x
Telles, E. C. C., de Camargo, P. B., Martinelli, L. A., Trumbore, S. E., da Costa, E. S., Santos, J., et al. (2003). Influence of soil texture on carbon dynamics and storage potential in tropical forest soils of Amazonia. Glob. Biogeochem. Cycles 17, 9/1–9/12. doi: 10.1029/2002GB001953
The Economist Intelligence Unit (2019). Democracy Index 2018: Me Too? Political Participation, Protest and Democracy. The Economist.
Tittonell, P. (2014). Ecological intensification of agriculture—sustainable by nature. Curr. Opin. Environ. Sustain. 8, 53–61. doi: 10.1016/j.cosust.2014.08.006
Townsend, A. R., Cleveland, C. C., Houlton, B. Z., Alden, C. B., and White, J. W. (2011). Multi-element regulation of the tropical forest carbon cycle. Front. Ecol. Environ. 9:47. doi: 10.1890/100047
Uhl, C., and Kauffman, J. B. (1990). Deforestation, fire susceptibility, and potential tree responses to fire in the Eastern Amazon. Ecology 71, 437–449. doi: 10.2307/1940299
UNEP FAO, and UNFF. (2009). “Vital forest graphics,” in Forest, eds L. Christian, M. L. Wilkie, I. Ruceveska, and Mita Sen (Nairobi: UNEP; FAO; UNEP/GRID-Arendal; UNFF Secretariat).
Viana, C., Coudel, E., Barlow, J., Ferreira, J., Gardner, T., and Parry, L. (2016). How does hybrid governance emerge? Role of the elite in building a Green Municipality in the Eastern Brazilian Amazon. Environ. Policy Govern. 26, 337–350. doi: 10.1002/eet.1720
Vilhena, L. (2016). Paragominas Renova Compromisso com o Meio Ambiente [Online]. Prefeitura de Paragominas. Available online at: http://paragominas.pa.gov.br/noticias/meio_ambiente/82-paragominas_renova_compromisso_com_o_meio_ambiente (accessed November 16, 2018).
WWF (2019). Amazon deforestation [Online]. WWF. Available online at: https://wwf.panda.org/our_work/forests/deforestation_fronts2/deforestation_in_the_amazon/ (accessed December 15, 2018).
Keywords: landscape multi-functionality, ecosystem services, pedo-morphology, land use, policy targets, land-use pathways
Citation: Pinillos D, Bianchi FJJA, Poccard-Chapuis R, Corbeels M, Tittonell P and Schulte RPO (2020) Understanding Landscape Multifunctionality in a Post-forest Frontier: Supply and Demand of Ecosystem Services in Eastern Amazonia. Front. Environ. Sci. 7:206. doi: 10.3389/fenvs.2019.00206
Received: 12 June 2019; Accepted: 20 December 2019;
Published: 21 January 2020.
Edited by:
André Mascarenhas, Humboldt University of Berlin, GermanyReviewed by:
Constantina Alina Hossu, University of Bucharest, RomaniaAlexandros Gasparatos, The University of Tokyo, Japan
Copyright © 2020 Pinillos, Bianchi, Poccard-Chapuis, Corbeels, Tittonell and Schulte. This is an open-access article distributed under the terms of the Creative Commons Attribution License (CC BY). The use, distribution or reproduction in other forums is permitted, provided the original author(s) and the copyright owner(s) are credited and that the original publication in this journal is cited, in accordance with accepted academic practice. No use, distribution or reproduction is permitted which does not comply with these terms.
*Correspondence: Felix J. J. A. Bianchi, felix.bianchi@wur.nl