- 1Nature Coast Biological Station, Institute of Food and Agricultural Sciences, University of Florida, Cedar Key, FL, United States
- 2Dauphin Island Sea Lab, Dauphin Island, AL, United States
Estuaries of the northern Gulf of Mexico contain an abundance of habitat-forming submerged aquatic vegetation (SAV) that provide refuge and protection for a variety of freshwater, estuarine, and marine organisms. However, many of these estuaries now contain numerous exotic species, the ultimate impacts of which are unclear. In the Mobile-Tensaw Delta, located in the upper portion of Mobile Bay, Alabama (USA), Eurasian milfoil (Myriophyllum spicatum, hereafter referred to as Myriophyllum) is now the most dominant submerged macrophyte. Myriophyllum is a structurally-complex macrophyte with the potential to dramatically alter estuarine food webs through reduced encounter rates between predators and their prey and other mechanisms. Previously, we surveyed faunal communities using throw traps, trawls, cores, and suction sampling to compare milfoil assemblages with other native macrophytes to explore the interactive role of hydrology, diel periodicity, and macrophyte presence in influencing community structure. Here, we use this previously collected data to generate preliminary food web analyses to determine if milfoil, due to its high complexity, creates a “trophic dead end” and limits higher trophic level production. We found the number of nodes, links, linkage density, and connectance to all be greater in milfoil than Vallisneria americana (hereafter referred to as Vallisneria), indicating that a diverse, productive, and highly connected food web exists in this invasive habitat.
Introduction
Estuarine ecosystems, located in the ecotones between marine and fresh waters and exposed to a range of dynamic stressors, including fluctuating environmental conditions (e.g., tides, salinity) and anthropogenic development (e.g., habitat loss, shoreline modification, altered hydrology), may be at great risk from successful invasions of non-native species. Invasion theory, established by Elton (1958), suggests that the alteration of native ecosystems can reduce invasion resistance of the ecosystem which, when coupled with high invasive propagule pressure from the proximity to urban centers and dispersal vectors such as commercial shipping, may facilitate the establishment of invasive species in estuaries. After gaining a foothold in these dynamic ecosystems, it is hypothesized that their competitive advantages will further enable their establishment and proliferation, creating a threat to biodiversity (Lodge, 1993; Vitousek et al., 1997; Cohen and Carlton, 1998; Everett, 2000) and ecosystem services provided by species-rich native ecosystems (Mack et al., 2000; Zedler and Kercher, 2004).
In northern Gulf of Mexico estuaries, a variety of invaders are now established at a wide range of trophic levels (Duffy and Baltz, 1998; Hicks et al., 2001; Peterson et al., 2005; Martin et al., 2010) and reported to have a negative impact on biodiversity. At the level of primary producer, however, several recent studies have indicated that plant invasions can supplement species richness in some invaded estuarine ecosystems (Cleland et al., 2004; Smith et al., 2004; Capers et al., 2007; Martin and Valentine, 2012). Invasive species that either create new habitats, or alter environmental conditions in existing habitats, have been hypothesized to have disproportionately negative effects on food webs (Crooks, 2002). In some cases, invasive vegetation can be structurally complex and larger than native species (Posey, 1988; Posey et al., 1993; Duffy and Baltz, 1998; Chaplin and Valentine, 2009). Structurally complex invasive plants can also reduce the foraging efficiency of higher order predators through the creation of interstitial spaces reducing encounter rates between predators and their prey (Nelson, 1979; Heck and Thoman, 1981; Martin and Valentine, 2011; Valinoti et al., 2011). As a result, the invasion of structurally complex macrophytes may limit energy flow to lower levels and alter trophic interactions in estuaries and thus represent a “trophic dead-end,” with fewer pathways and less energy transfer among adjacent trophic levels in successfully invaded estuarine food webs (Chaplin and Valentine, 2009).
Because estuaries in the northern Gulf of Mexico serve as nursery grounds that fuel some of the most productive commercial and recreational fisheries in the world (Chesney et al., 2000; Lellis-Dibble et al., 2008), the alteration of food web function in this area is of great concern. Here, we use estimates of shelter-seeking fauna from previous literature in Mobile Bay, Alabama (USA) to construct a preliminary food web to assess the impacts of one widespread invasive submerged aquatic vegetation (SAV), Eurasian milfoil Myriophyllum spicatum, on oligohaline estuarine food web function via a comparison with an abundant native species of vegetation (wild celery, Vallisneria americana hereafter referred to as Vallisneria) (Figure 1). The overarching goal of this research is to provide an initial assessment of the potential impacts of invasive Eurasian milfoil (hereafter referred to as Myriophyllum) on food web function in the estuaries of the northcentral Gulf of Mexico.
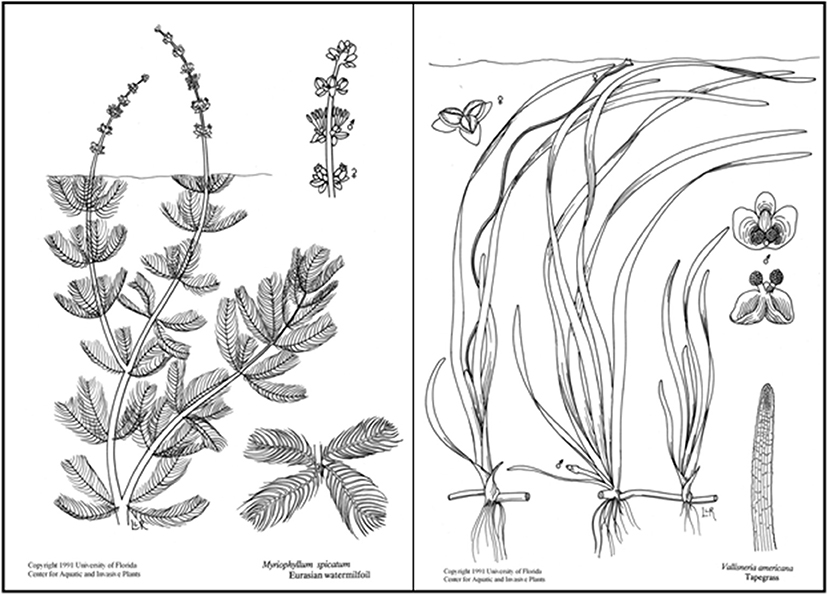
Figure 1. SAV species compared in this study are the complex invasive species Myriophyllum spicatum (left) and structurally simpler native species Vallisneria americana (right). Line drawings used with permission from UF/IFAS Center for Aquatic and Invasive Plants.
Methods
Study Site
The Mobile-Tensaw Delta (MTD), located at the upper end of Mobile Bay in lower Alabama, encompasses an area of over 1100 km2, from the convergence of the Tombigbee and Alabama Rivers south to Mobile Bay. The watershed drains ~15% of the United States' freshwater, ranking as the fourth largest drainage delta in terms of discharge, before entering the Gulf of Mexico (Gastaldo, 1985). The landscape shifts from forested wetlands in the north to tidal freshwater and oligohaline marshes as the MTD empties into Mobile Bay to the south. Salinities in the southernmost portion of the area is highly variable, from freshwater or low salinity (2 Practical Salinity Units, PSU) during the spring to 18 PSU or higher in the fall. Tidal heights vary on average 40-cm (Martin and Valentine, 2014a,b).
Widespread throughout the MTD in shallow waters are as many as 24 species of SAV (Stout et al., 1982; Vittor, 2003), the most abundant of which are Myriophyllum and Vallisneria. Although annually variable, as much as 80% coverage of the submerged landscape has been estimated to be covered by Myriophyllum (Vittor, 2003; Chaplin and Valentine, 2009; Martin and Valentine, 2012). Myriophyllum is a structurally complex species of SAV commonly encountered in freshwater and coastal ecosystems in North America, including estuaries of the Gulf of Mexico (Duffy and Baltz, 1998; Frazer et al., 2006; Martin and Valentine, 2011). The dense beds formed by Myriophyllum have the potential to alter the composition of faunal assemblages due to their increased complexity and density, especially compared to the structurally simpler native habitats, such as Vallisneria (Chaplin and Valentine, 2009; Goecker et al., 2009). While much is known about the aesthetic effects of Myriophyllum on freshwater ecosystems, and its effects on food web structure and function in freshwater systems (Smith and Barko, 1990), its food web effects in coastal systems, especially in the Gulf of Mexico, remain less clear and the goal of this study is to perform a preliminary assessment of Myriophyllum's food web.
Previous Literature
Several studies have quantified various aspects of the organisms residing in Myriophyllum and Vallisneria in the MTD (Table 1). Chaplin and Valentine (2009) quantified secondary production and invertebrate assemblages using cores and found elevated levels driven primarily by very high densities of amphipods. Martin and Valentine (2011) reported that the fishes and macroinvertebrates inhabiting Myriophyllum, quantified using throw traps, were more abundant and had a significantly different community structure from those found in native Vallisneria beds. Hydrologically isolated areas within the region can also have impacts on the faunal communities, as demonstrated by a drop sampling approach (Rozas et al., 2013). Finally, the structure provided by Myriophyllum can be extremely dense, trapping organic matter which, when coupled with plant respiration, can trigger low oxygen events in some areas and impact organism captured through suction sampling (Kauffman et al., 2018). Generally, this anoxia is limited to nighttime hours and only in Myriophyllum beds. Using the data collected for these studies, we used a social network modeling (Borgatti et al., 2002) to perform initial assessments of the food web structure in both Myriophyllum and Vallisneria.
Food Web Analyses
Using presence/absence of species composition, we used the tools of social network analysis to generate a binary food web for organisms captured within each SAV (Myriophyllum and Vallisneria). Networks were created using UCINET software (Borgatti et al., 2002; McCann et al., 2017). Each network was visualized by creating a circular graph using the NETDRAW feature found in UCINET software. While this approach is most commonly used in social sciences, it has been applied to ecological systems (Christian et al., 2005; Fletcher et al., 2011; McCann et al., 2017). Advantages of this approach are that it provides accurate predictions of structure, requires less data, and is flexible with the types of data used (Fletcher et al., 2011). As a result, this approach may be advantageous for some questions not requiring a full diet model (such as Ecopath with Ecosim). Diet information was attained from the author's unpublished gut content data from this area, literature-based estimates, and best professional judgement (Chaplin, 2002; Christian and Allen, 2014).
From the networks generated by the modeling efforts, a variety of performance metrics were generated for both the Myriophyllum and Vallisneria habitats. The number of nodes was defined as the number of individual species/taxonomic groups present in each network. The number of links estimated the probable number of trophic interactions (i.e., lines that connect nodes) occurring within the two habitats. The estimated linkage density for each SAV was defined as the average number of links per node, calculated as L/S, where L was the number of links and S was the number of species or nodes. Finally, connectance (or the proportion of possible links that are realized) was determined for each network by calculating the maximum connectance as S(S-1)/2, where S is the number of species or nodes. Then the connectance in each network was calculated as L/(S(S-1)/2), where S is the number of species or nodes and L is the number of links.
Results
The food webs generated for Myriophyllum (Figure 2) and Vallisneria (Figure 3) show that each habitat is productive and supports a diverse food web. However, the model-generated food web performance metrics showed that Myriophyllum exceeds that of Vallisneria in all comparisons. In general, there were more nodes and links in Myriophyllum habitats than Vallisneria (nodes: 75 compared to 68 and links: 310 compared to 197 respectively). The 9.33% increase in nodes in Myriophyllum led to a 36.45% more links. This corresponded to a 29.78% increase in the linkage density in Myriophyllum (4.13), when compared to Vallisneria (2.90). Finally, these performance metrics led to a 23.21% increase in connectance for Myriophyllum (0.056) over Vallisneria (0.043). Given the increased nodes, links, linkage density, and connectance in Myriophyllum, we surmise that these initial, preliminary food web metrics indicate that Myriophyllum may not be the trophic dead ends as might be predicted based on traditional invasion theory. Rather, Myriophyllum seems to support a robust, highly connected food web when compared to the dominant native species, Vallisneria.
Discussion
Among the myriad of stressors facing coastal ecosystems, invasive species have been posited to be among the most pervasive consequence of elevated levels of anthropogenic activities in estuaries (Mack et al., 2000). With the increasing human population in coastal areas, and subsequent increases in disturbances accompanying this encroachment, invasive species now join a long list of stressors affecting coastal food webs (Ruiz et al., 1999) including eutrophication (de Mutsert et al., 2016), climate change (Heck et al., 2015), hurricanes (Anton et al., 2009), and oil spills (McCann et al., 2017; Martin and Swenson, 2018), among others.
Here, we used the results of several recent studies comparing Myriophyllum and Vallisneria faunal composition in upper Mobile Bay and the lower MTD, Alabama, to evaluate potential food web impacts due to the proliferation of this notorious invader. Given the increased nodes, links, linkage density, and connectance in Myriophyllum, we surmise that these initial, preliminary food web performance metrics indicate that Myriophyllum may not be a trophic dead end as many predicted and the increased structural complexity does support a highly connected food web compared relative to the dominant native species, Vallisneria. We note that the density, and thus structural complexity, of these habitats vary greatly within this invaded system such that a range of complexities are present (Martin and Valentine, 2012; Martin et al., 2012).
We highlight that these analyses are preliminary, with these studies encompassing a large temporal interval (almost a decade), over which changes may have occurred and be integrated within the generated models. Likewise, short-term changes in habitat community composition may also occur, as demonstrated by Kauffman et al. (2018) with migration into/out of these habitats occurring over a diel cycle, further biasing the model. We would contend, however, that this work is conservative to this bias by incorporating presence/absence of all organisms captured over the study period into these analyses. Finally, this study uses a binary, unweighted links to establish food web connections and future research should be aimed at determining carbon flow originating from Myriophyllum and true, weighted links in this food web (e.g., using biomarkers such as isotopic and/or fatty acid approaches). Moreover, incorporating abundance into more traditional food web analyses such as Ecopath with Ecosim may yield further insight into ecosystem functioning in these invaded ecosystems.
Throughout the United States, the invasion of Myriophyllum has been largely widespread and represents a significant threat to aquatic environments, largely due to the fast growth rates resulting in large canopies and areal coverage of this invader. These changes can impact key ecosystem functions such as environmental conditions and water quality (Frodge et al., 1990; Unmuth et al., 2000) and significantly alter a range of variables impacting community composition and food webs, including habitat preference, herbivory, foraging, and decomposition (Martin and Valentine, 2011; Steele et al., 2018). Based on the impacts to freshwater ecosystems, it is reasonable to assume that Myriophyllum might also have negative impacts in estuaries, although to date these have not been observed (Duffy and Baltz, 1998; Martin and Valentine, 2011, 2012). Still, a more detailed assessment of impacts is necessary to gain a more comprehensive view of the ecological impacts of this invader. We suggest that additional food web studies are necessary to fully understand Myriophyllum's impacts in coastal areas, such coupled gut/dietary analyses with biomarker approaches and how ecologically relevant the documented food web changes are for energy flow. Incorporating multiple trophic levels, in addition to spatial and temporal variability in communities and patterns of vegetation will likely significantly enhance our understanding of estuarine invaders and encourage more cognizant management approaches to prevent and eradicate these invaders.
Data Availability Statement
The datasets for this manuscript are not publicly available because Datasets are part of previously published literature found at the following links: https://link.springer.com/article/10.1007/s12237-010-9274-5; https://www.int-res.com/abstracts/meps/v592/p97-108/; https://link.springer.com/article/10.1007/s12237-008-9117-9; https://www.int-res.com/abstracts/meps/v492/p9-20/. Requests to access the datasets should be directed to CM, bWFydGluLmNoYXJsZXMud0BnbWFpbC5jb20=.
Ethics Statement
While this manuscript does involve animal subjects, the analysis performed here only used data from previously published literature and no field collections were performed for this modeling approach.
Author Contributions
CM and JV conceived the study and contributed to manuscript revision, read, and approved the submitted version. CM performed analysis and wrote the first draft.
Funding
While this study was unfunded, the data collected and cited from previous literature was funded through a variety of sources including the Mobile Bay National Estuary Program, Northern Gulf Institute, Alabama Center for Estuarine Studies, Dauphin Island Sea Lab, and University of South Alabama.
Conflict of Interest
The authors declare that the research was conducted in the absence of any commercial or financial relationships that could be construed as a potential conflict of interest.
Acknowledgments
We thank numerous people for their dedication and effort in collecting data used in these analyses, as well as the discussions and guidance of many others, including: Derrick Blackmon, Katherine Blankenhorn, Glen Chaplin, Mike Dardeau, Marissa Dueker, Alan Gunter, Ken Heck, Taylor Kauffman, Larissa Lee, Shanna Madsen, Sean Powers, Riikka Puntila, Susan Sklenar, LaTina Steele, and staff at the Dauphin Island Sea Lab. We thank the reviewers for their constructive comments which improved the manuscript.
References
Anton, A., Cebrian, J., Duarte, C. M., Heck, J., Kenneth, L., and Goff, J. (2009). Low impact of Hurricane Katrina on seagrass community structure and functioning in the northern Gulf of Mexico. Bull. Mar. Sci. 85, 45–59. Available online at: https://www.ingentaconnect.com/content/umrsmas/bullmar/2009/00000085/00000001/art00004
Borgatti, S. P., Everett, M. G., and Freeman, L. C. (2002). Ucinet for Windows: Software for Social Network Analysis. Harvard, MA: Analytic Technologies.
Capers, R. S., Selsky, R., Bugbee, G. J., and White, J. C. (2007). Aquatic plant community invasibility and scale-dependent patterns in native and invasive species richness. Ecology 88, 3135–3143. doi: 10.1890/06-1911.1
Chaplin, G. I. (2002). Effects of habitat complexity, created by native and exotic macrophytes, on secondary production and the transfer of energy to higher order consumers in the lower Mobile-Tensaw Delta, Alabama (MS thesis). University of South Alabama, Mobile, United States.
Chaplin, G. I., and Valentine, J. F. (2009). Macroinvertebrate production in the submerged aquatic vegetation of the Mobile–Tensaw Delta: effects of an exotic species at the base of an estuarine food web. Estuaries Coasts 32, 319–332. doi: 10.1007/s12237-008-9117-9
Chesney, E. J., Baltz, D. M., and Thomas, R. G. (2000). Louisiana estuarine and coastal fisheries and habitats: perspectives from a fish's eye view. Ecol. Appl. 10, 350–366. doi: 10.1890/1051-0761(2000)010[0350:LEACFA]2.0.CO;2
Christian, R. R., and Allen, D. M. (2014). Linking hydrogeomorphology and food webs in intertidal creeks. Estuaries Coasts 37, 74–90. doi: 10.1007/s12237-013-9657-5
Christian, R. R., Baird, D., Luczkovich, J., Johnson, J. C., Scharler, U. M., and Ulanowicz, R. E. (2005). “Role of network analysis in comparative ecosystem ecology of estuaries,” in Aquatic Food Webs: An Ecosystem Approach, eds A. Belgramo, U. M. Scharler, J. Dunne, and R. E. Ulanowicz (New York, NY: Oxford University Press), 25–40. doi: 10.1093/acprof:oso/9780198564836.003.0004
Cleland, E. E., Smith, M. D., Andelman, S. J., Bowles, C., Carney, K. M., Claire Horner-Devine, M., et al. (2004). Invasion in space and time: non-native species richness and relative abundance respond to interannual variation in productivity and diversity. Ecol. Lett. 7, 947–957. doi: 10.1111/j.1461-0248.2004.00655.x
Cohen, A. N., and Carlton, J. T. (1998). Accelerating invasion rate in a highly invaded estuary. Science 279, 555–558. doi: 10.1126/science.279.5350.555
Crooks, J. A. (2002). Characterizing ecosystem-level consequences of biological invasions: the role of ecosystem engineers. Oikos 97, 153–166. doi: 10.1034/j.1600-0706.2002.970201.x
de Mutsert, K., Steenbeek, J., Lewis, K., Buszowski, J., Cowan, J. H. Jr., and Christensen, V. (2016). Exploring effects of hypoxia on fish and fisheries in the northern Gulf of Mexico using a dynamic spatially explicit ecosystem model. Ecol. Model. 331, 142–150. doi: 10.1016/j.ecolmodel.2015.10.013
Duffy, K. C., and Baltz, D. M. (1998). Comparison of fish assemblages associated with native and exotic submerged macrophytes in the Lake Pontchartrain estuary, USA. J. Exp. Mar. Biol. Ecol. 223, 199–221. doi: 10.1016/S0022-0981(97)00166-4
Elton, C. S. (1958). The Ecology of Invasions by Plants and Animals. University of Chicago Press. doi: 10.1007/978-1-4899-7214-9
Everett, R. A. (2000). Patterns and pathways of biological invasions. Trends Ecol. Evol. 15, 177–178. doi: 10.1016/S0169-5347(00)01835-8
Fletcher, R. J., Acevedo, M. A., Reichert, B. E., Pias, K. E., and Kitchens, W. M. (2011). Social network models predict movement and connectivity in ecological landscapes. Proc. Natl. Acad. Sci. U.S.A. 108, 19282–19287. doi: 10.1073/pnas.1107549108
Frazer, T. K., Notestein, S. K., Jacoby, C. A., Littles, C. J., Keller, S. R., and Swett, R. A. (2006). Effects of storm-induced salinity changes on submersed aquatic vegetation in Kings Bay, Florida. Estuaries Coasts 29, 943–953. doi: 10.1007/BF02798655
Frodge, J. D., Thomas, G. L., and Pauley, G. B. (1990). Effects of canopy formation by floating and submergent aquatic macrophytes on the water quality of two shallow Pacific Northwest lakes. Aquat. Bot. 38, 231–248 doi: 10.1016/0304-3770(90)90008-9
Gastaldo, R. A. (1985). Plant Accumulating Deltaic Depositional Environments Mobile Delta, Alabama. Geological Survey of Alabama.
Goecker, M. E., Valentine, J. F., Sklenar, S. A., and Chaplin, G. I. (2009). Influence from hydrological modification on energy and nutrient transference in a deltaic food web. Estuaries Coasts 32, 173–187. doi: 10.1007/s12237-008-9105-0
Heck, K. L. Jr, Fodrie, F. J., Madsen, S., Baillie, C. J., and Byron, D. A. (2015). Seagrass consumption by native and a tropically associated fish species: potential impacts of the tropicalization of the northern Gulf of Mexico. Mar. Ecol. Prog. Ser. 520, 165–173. doi: 10.3354/meps11104
Heck, K. L. Jr, and Thoman, T. A. (1981). Experiments on predator-prey interactions in vegetated aquatic habitats. J. Exp. Mar. Biol. Ecol. 53, 125–134. doi: 10.1016/0022-0981(81)90014-9
Hicks, D. W., Tunnell, J. W. Jr., and McMahon, R. F. (2001). Population dynamics of the nonindigenous brown mussel Perna perna in the Gulf of Mexico compared to other world-wide populations. Mar. Ecol. Prog. Ser. 211, 181–192. doi: 10.3354/meps211181
Kauffman, T. C., Martin, C. W., and Valentine, J. F. (2018). Hydrological alteration exacerbates the negative impacts of invasive Eurasian milfoil Myriophyllum spicatum by creating hypoxic conditions in a northern Gulf of Mexico estuary. Mar. Ecol. Prog. Ser. 592, 97–108. doi: 10.3354/meps12517
Lellis-Dibble, K. A., McGlynn, K. E., and Bigford, T. E. (2008). Estuarine Fish and Shellfish Species in US Commercial and Recreational Fisheries: Economic Value as an Incentive to Protect and Restore Estuarine Habitat. Washington, DC: NOAA Technical Memo NMFSF/SPO-90; U.S. Department of Commerce. Available online at: http://www.habitat.noaa.gov/pdf/publications_general_estuarinefishshellfish.pdf
Lodge, D. M. (1993). Biological invasions: lessons for ecology. Trends Ecol. Evolution 8, 133–137. doi: 10.1016/0169-5347(93)90025-K
Mack, R. N., Simberloff, D., Mark Lonsdale, W., Evans, H., Clout, M., and Bazzaz, F. A. (2000). Biotic invasions: causes, epidemiology, global consequences, and control. Ecol. Appl. 10, 689–710. doi: 10.1890/1051-0761(2000)010[0689:BICEGC]2.0.CO;2
Martin, C. W., Bayha, K. M., and Valentine, J. F. (2012). Establishment of the invasive island apple snail Pomacea insularum (Gastropoda: Ampullariidae) and eradication efforts in Mobile, Alabama, USA. Gulf Mexico Sci. 30:5.
Martin, C. W., and Swenson, E. M. (2018). Herbivory of oil-exposed submerged aquatic vegetation Ruppia maritima. PLoS ONE 13:e0208463. doi: 10.1371/journal.pone.0208463
Martin, C. W., and Valentine, J. F. (2011). Impacts of a habitat-forming exotic species on estuarine structure and function: an experimental assessment of Eurasian milfoil. Estuaries Coasts 34, 364–372. doi: 10.1007/s12237-010-9274-5
Martin, C. W., and Valentine, J. F. (2012). Eurasian milfoil invasion in estuaries: physical disturbance can reduce the proliferation of an aquatic nuisance species. Mar. Ecol. Prog. Ser. 449, 109–119. doi: 10.3354/meps09515
Martin, C. W., and Valentine, J. F. (2014a). Sexual and asexual reproductive strategies of invasive Eurasian milfoil (Myriophyllum spicatum) in estuarine environments. Hydrobiologia 727, 177–184. doi: 10.1007/s10750-013-1798-9
Martin, C. W., and Valentine, J. F. (2014b). Tolerance of embryos and hatchlings of the invasive apple snail Pomacea maculata to estuarine conditions. Aquatic Ecol. 48, 321–326. doi: 10.1007/s10452-014-9486-z
Martin, C. W., Valentine, M. M., and Valentine, J. F. (2010). Competitive interactions between invasive Nile tilapia and native fish: the potential for altered trophic exchange and modification of food webs. PLoS ONE 5:e14395. doi: 10.1371/journal.pone.0014395
McCann, M. J., Able, K. W., Christian, R. R., Fodrie, F. J., Jensen, O. P., Johnson, J. J., et al. (2017). Key taxa in food web responses to stressors: the Deepwater Horizon oil spill. Front. Ecol. Environ. 15, 142–149. doi: 10.1002/fee.1474
Nelson, W. G. (1979). An analysis of structural pattern in an eelgrass (Zostera marina L.) amphipod community. J. Exp. Mar. Biol. Ecol. 39, 231–264. doi: 10.1016/0022-0981(79)90129-1
Peterson, M. S., Slack, W. T., and Woodley, C. M. (2005). The occurrence of non-indigenous Nile tilapia, Oreochromis niloticus (Linnaeus) in coastal Mississippi, USA: ties to aquaculture and thermal effluent. Wetlands 25, 112–121. doi: 10.1672/0277-5212(2005)025[0112:TOONNT]2.0.CO;2
Posey, M. H. (1988). Community changes associated with the spread of an introduced seagrass, Zostera japonica. Ecology 69, 974–983. doi: 10.2307/1941252
Posey, M. H., Wigand, C., and Stevenson, J. C. (1993). Effects of an introduced aquatic plant, Hydrilla verticillata, on benthic communities in the upper Chesapeake Bay. Estuar. Coast. Shelf Sci. 37, 539–555. doi: 10.1006/ecss.1993.1072
Rozas, L. P., Martin, C. W., and Valentine, J. F. (2013). Effects of reduced hydrological connectivity on the nursery use of shallow estuarine habitats within a river delta. Mar. Ecol. Prog. Ser. 492, 9–20. doi: 10.3354/meps10486
Ruiz, G. M., Fofonoff, P., Hines, A. H., and Grosholz, E. D. (1999). Non-indigenous species as stressors in estuarine and marine communities: assessing invasion impacts and interactions. Limnol. Oceanogr. 44(3 Pt 2), 950–972.
Smith, C. S., and Barko, J. W. (1990). Ecology of Eurasian watermilfoil. J. Aquat. Plant Manag. 28, 55–64.
Smith, J. E., Hunter, C. L., Conklin, E. J., Most, R., Sauvage, T., Squair, C., et al. (2004). Ecology of the invasive red alga Gracilaria salicornia (Rhodophyta) on O'ahu, Hawai'i. Pac. Sci. 58, 325–343. doi: 10.1353/psc.2004.0023
Steele, L., Ray, C., and Guidone, M. (2018). High phenolic content fails to deter mesograzer consumption of Myriophyllum spicatum (Eurasian watermilfoil) in New England. Aquatic Ecol. 52, 255–267. doi: 10.1007/s10452-018-9661-8
Stout, J. P., Lelong, M. J., Dowling, H. M., and Powers, M. T. (1982). Wetland habitats of the Alabama coastal zone. Part III: An inventory of wetland habitats of the Mobile-Tensaw River Delta. Alabama Coastal Area Board Technical Report, No 81-49A, 25
Unmuth, J. M., Lillie, R. A., Dreikosen, D. S., and Marshall, D. W. (2000). Influence of dense growth of Eurasian watermilfoil on lake water temperature and dissolved oxygen. J. Freshwater Ecol. 15:497–503 doi: 10.1080/02705060.2000.9663772
Valinoti, C. E., Ho, C. K., and Armitage, A. R. (2011). Native and exotic submerged aquatic vegetation provide different nutritional and refuge values for macroinvertebrates. J. Exp. Mar. Biol. Ecol. 409, 42–47. doi: 10.1016/j.jembe.2011.08.001
Vitousek, P. M., Mooney, H. A., Lubchenco, J., and Melillo, J. M. (1997). Human domination of Earth's ecosystems. Science 277, 494–499. doi: 10.1126/science.277.5325.494
Vittor, B. A. (2003). Benthic Mapping of Submerged Aquatic Vegetation in Mobile Bay and Adjacent Waters of Coastal Alabama. Mobile, AL: Barry A. Vittor and Associates, Inc.
Keywords: Vallisneria, watermilfoil, habitat, exotic, network model
Citation: Martin CW and Valentine JF (2019) Does Invasion of Eurasian Milfoil Myriophyllum spicatum Lead to a “Trophic Dead End” and Reduced Food Web Complexity in Gulf of Mexico Estuarine Food Webs? Front. Environ. Sci. 7:166. doi: 10.3389/fenvs.2019.00166
Received: 12 March 2019; Accepted: 03 October 2019;
Published: 22 October 2019.
Edited by:
Robert Ptacnik, Wasser Cluster Lunz, AustriaReviewed by:
Luis Zambrano, National Autonomous University of Mexico, MexicoMarcelo Bertellotti, Centro Para el Estudio de Sistemas Marinos (CONICET), Argentina
Copyright © 2019 Martin and Valentine. This is an open-access article distributed under the terms of the Creative Commons Attribution License (CC BY). The use, distribution or reproduction in other forums is permitted, provided the original author(s) and the copyright owner(s) are credited and that the original publication in this journal is cited, in accordance with accepted academic practice. No use, distribution or reproduction is permitted which does not comply with these terms.
*Correspondence: Charles W. Martin, bWFydGluLmNoYXJsZXMud0BnbWFpbC5jb20=