- 1Department of Chemical Analytics and Biogeochemistry, Leibniz-Institute of Freshwater Ecology and Inland Fisheries (IGB), Forschungsverbund Berlin e.V., Berlin, Germany
- 2Department of Bioscience, Aarhus University, Silkeborg, Denmark
- 3Institute of Landscape Ecology and Site Evaluation, University of Rostock, Rostock, Germany
- 4School of Natural Sciences, Bangor University, Bangor, United Kingdom
There have been widespread attempts to rewet peatlands in Europe and elsewhere in the world to restore their unique biodiversity as well as their important function as nutrient and carbon sinks. However, changes in hydrological regime and therefore oxygen availability likely alter the abundance of enzyme-inhibiting polyphenolic compounds, which have been suggested as a “latch” preventing large amounts of carbon from being released into the atmosphere by microbial mineralization. In recent years, a variety of factors have been identified that appear to weaken that latch including not only oxygen, but also pH. In minerotrophic fens, it is unknown if long-term peat mineralization during decades of drainage and intense agricultural use causes an enrichment or a decline of enzyme-inhibiting polyphenols. To address this, we collected peat samples and fresh roots of dominating plants (i.e., the peat parent material) from the upper 20 cm peat layer in 5 rewetted and 6 natural fens and quantified total phenolic content as well as hydrolysable and condensed tannins. Polyphenols from less decomposed peat and living roots served partly as an internal standard for polyphenol analysis and to run enzyme inhibition tests. As hypothesized, we found the polyphenol content in highly decomposed peat to be eight times lower than in less decomposed peat, while condensed tannin content was 50 times lower in highly degraded peat. In addition, plant tissue polyphenol contents differed strongly between peat-forming plant species, with the highest amount found in roots of Carex appropinquata at 450 mg g−1 dry mass, and lowest in Sphagnum spp. at 39 mg g−1 dry mass: a 10-fold difference. Despite large and clear differences in peat and porewater chemistry between natural and rewetted sites, enzyme activities determined with Fluorescein diacetate (FDA) hydrolysis and peat degradation were not significantly correlated, indicating no simple linear relationship between polyphenol content and microbial activity. Still, samples with low contents of polyphenols and condensed tannins showed the highest microbial activities as measured with FDA.
Introduction
Despite covering only 2–3% of the land surface, peatlands store approximately one third of global soil carbon (C; Gorham, 1991; Jenkinson et al., 1991; Yu et al., 2010). Due to the anaerobic conditions in waterlogged peat, the rate of production of organic matter exceeds its decomposition (Pind et al., 1994; Williams and Yavitt, 2003; Freeman et al., 2004). Peatlands also play a significant role in water cycling and nutrient retention in river catchments (Verhoeven et al., 2006) and often support unique biodiversity (Zerbe et al., 2013). However, despite their importance from local to global scales, a significant loss in global peatland area continues (Joosten, 2010; IPCC, 2014) and attempts to restore these important ecosystems are widespread. In NE Germany, more than 30,000 hectares of drained minerotrophic (groundwater-fed) peatlands (i.e., fens) have been rewetted over the last 30 years to restore their ecological functions (Zak et al., 2018). Due to their extended drainage history, a re-establishment of their original state is not expected in a human life time perspective (Zak et al., 2018), but they may eventually return to a carbon neutral state (Joosten et al., 2015). The post-rewetting dynamics of carbon cycling in fens are, however, still poorly constrained.
The peatland carbon imbalance is regulated by the prevalence of polyphenolic compounds that inhibit microbial carbon mineralization and the enzymes that act upon them (Freeman et al., 2001). In ombrotrophic (rain-fed) peatlands (bogs), the enzyme phenol oxidase represents a key regulator for microbial carbon mineralization (Freeman et al., 2001, 2004). Phenol oxidase depends on molecular oxygen (O2) and is highly active under drained conditions. This increased activity reduces the amount of polyphenolic compounds and limits their inhibiting effect on microbial decomposition. In contrast, when oxygen is depleted under waterlogged conditions, phenol oxidase is inactive, polyphenols accumulate, and microbial carbon mineralization becomes limited. This mechanism is so effective that it has been termed an “enzymic latch” on the peatland carbon store (Freeman et al., 2001). The “enzymic latch” theory is still discussed widely, with some suggesting greater complexity than previously assumed based on research in tropical peats (Hall et al., 2014) and temperate systems (Fenner and Freeman, 2011; Brouns et al., 2016; Bonnett et al., 2017).
Despite its possible central role for organic matter decomposition in bogs, the “enzymic latch” has rarely been investigated in fens (but see Pinsonneault et al., 2016). This mechanism may be particularly relevant in previously drained fens, because prolonged drainage exposes the peat surface to oxygen and allows phenol oxidase to activate, essentially enhancing peat decomposition that may even continue under rewetted conditions, as long as polyphenol content is considerably reduced. In addition, drainage leads to warming of the soil. This may further weaken the “latch” since temperature seems to be an important control on phenol oxidase activity across peatland types (Pinsonneault et al., 2016). A biogeochemical legacy of draining is well-documented in several other aspects of fen carbon and nutrient dynamics. For example, a strong increase in methane emissions is typically measured in rich fens after rewetting (Hahn-Schöfl et al., 2011; Franz et al., 2016). Elevated concentrations of dissolved organic carbon, ammonium, and phosphate at levels of one to three orders higher compared to pristine systems have been measured in porewaters of the upper degraded peat layers of rewetted fens (Zak and Gelbrecht, 2007), indicating potentially increased microbial turnover of organic matter in degraded topsoil of formerly drained fens. Laboratory incubations under stationary conditions have shown that microbially mediated redox processes are more intense in highly decomposed vs. less decomposed peat, partly explained by enhanced availability of nutrients and terminal electron acceptors (Zak and Gelbrecht, 2007; Cabezas et al., 2012). On the contrary, there is evidence that the general availability of organic substrate within the decomposed peat layer is lower because it has already undergone mineralization over decades of drainage, and it is expected to be more recalcitrant due to the enrichment of polyphenols, in particular of lignin and tannin, that can inhibit enzymatic systems important for microbial carbon cycling (Bader et al., 2018).
Polyphenols are a large and diverse group of organic substances in which at least two hydroxyl (OH-) groups are bound to aromatic ring structures. They are typical plant metabolites that are synthesized to support a variety of physiological functions such as morphology and energy metabolism (e.g., pigments), structure (e.g., lignin), and pathogen or predator resistance (e.g., flavonoids and tannins; Bravo, 1998). Tannins are of particular ecological importance, as these high-molecular weight polyphenols (500–3,000 Da; Swain, 1979) possess protein deactivation potential by forming insoluble protein complexes, and therefore affect many microbial processes that are enzyme-mediated (Bravo, 1998). They are the fourth-most abundant class of biochemical compounds in terrestrial biomass after cellulose, hemicelluloses, and lignin, and are found in the bark, wood, leaves, fruits, and roots of a wide variety of vascular plants (Cowan, 1999; Hernes and Hedges, 2000; Mueller-Harvey, 2001). Due to their multiple phenolic hydroxyl groups and high molecular weights, tannins, in contrast to low molecular weight phenolic acids such as gallic, vanillic, or ferulic acids, may limit litter decomposition more effectively in a number of different ways: (1) by complexing or deactivating microbial exoenzymes, (2) by complexing metals essential to enzyme activity, (3) by direct toxicity to microbes, (4) by themselves being resistant to decomposition, (5) by sequestering proteins in tannin-protein complexes that are resistant to decomposition and finally, (6) by coating other compounds, such as cellulose, and protecting them from microbial attack (Kraus et al., 2003). To unravel the importance of these single processes, it seems mandatory to complement bulk polyphenol analysis by further characterizing the biochemical properties of the polyphenol assemblage (Mole and Waterman, 1987; Appel, 1993; Nelson et al., 1997; Hättenschwiler and Vitousek, 2000; Rautio et al., 2007). Analytically, tannins can be divided into two major groups: hydrolysable tannins and condensed tannins (Figure 1); the latter also termed proanthocyanidins (PAs). Hydrolysable tannins are composed of gallic acid or hexahydroxydiphenic acid esterified to a sugar moiety while condensed tannins are polymers of three ring flavan-3-ols joined with C-C bounds.
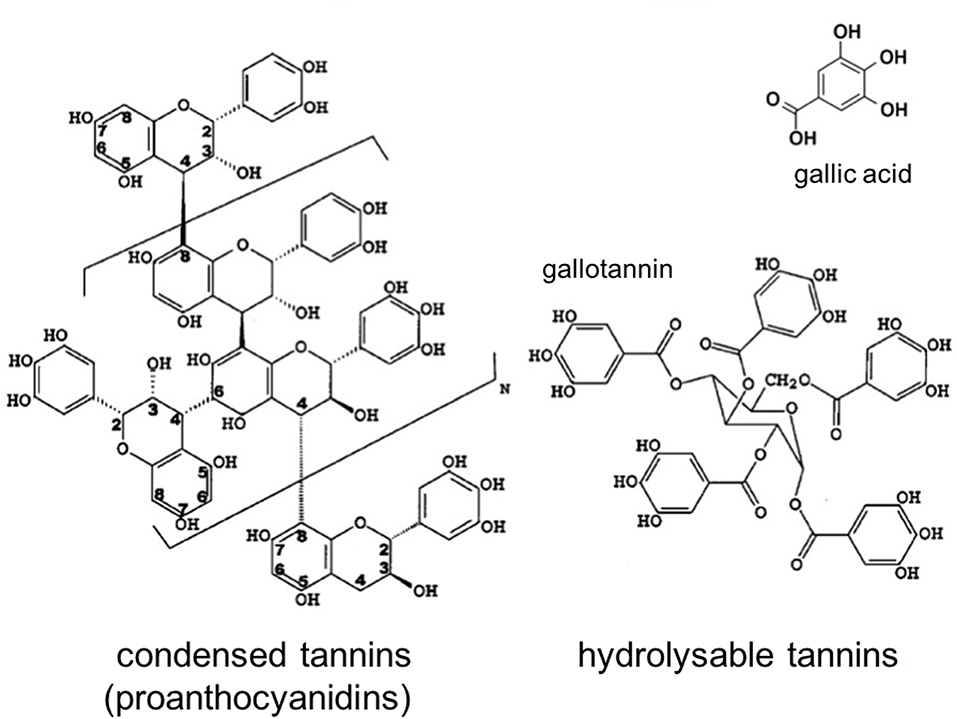
Figure 1. Structure of condensed and hydrolysable tannins (modified after Hernes and Hedges, 2000).
Due to these unique properties of polyphenols, we expect that their effect on carbon turnover should be similar to the “enzyme latch” described in bogs. We hypothesize that highly degraded peat of rewetted fens contains less enzyme-inhibiting polyphenols compared to less decomposed peat of pristine fens due to break up of polyphenols during the drainage phase. We analyzed fen peat substrates as well as peat-forming plant tissues from rewetted and pristine fens for representative polyphenol fractions—total phenol content, hydrolysable and condensed tannins—and for bulk microbial activity. Further, we tested microbial inhibition with PAs and characterized the peat samples chemically.
Methods
Study Sites and Sampling
The investigated fens are situated along freshwater sources in the postglacial landscape of northeast Germany and northwest Poland (Figure 2). The rewetted fens Hasenfelde, Sauwinkel, Menzlin, Zarnekow, and Jargelin have been subject to long-term drainage and were used mostly as grassland over several decades. At the time of sampling, their upper soil layer therefore consisted of highly decomposed peat until a depth of ~0.3 m, followed by less decomposed peat until a depth of up to 10 m (Zak and Gelbrecht, 2007). Three to Twelve years after rewetting, all sites were dominated by helophytes such as Phragmites australis and Typha latifolia in permanent flooded areas, and by Phalaris arundinacea in areas experiencing wet-dry cycles (Zak et al., 2015).
The hydrology of the sites Dollgensee, Töpchin, Gützkow, Triebschsee, and Rzecin was not or only slightly disturbed by drainage or similar measures in the past. In all areas the peat at the soil surface was only slightly decomposed, i.e., H1–4 according to the von Post humification scale (Von Post, 1922). All of these near to pristine peatlands can be classified as mesotrophic subneutral fens and are dominated by brown mosses (e.g., Bryum pseudotriquetum, Campylium stellatum, Plagiomnium elatum), and low sedges like Carex hostiana, C. flacca, and C. panacea (Zak et al., 2010). Furthermore, sparse stands of Phragmites australis occur in all the pristine sites, which represents the main peat-forming plant apart from Carex spp. and brown mosses. For the rewetted sites, the peat parent material was not recognizable any more due to the amorphous character of the soil, however deeper layers which were unaffected by water table drawdown indicate the same peat-parent material as in the undisturbed sites.
Peat samples were collected in triplicate from the top layers using a knife (ca. 10–20 cm depth) and transported to the lab within 1 day in an insulated cooler with ice, and analyzed for the characterization of total microbial activity immediately or frozen at −18°C for polyphenol analysis. Select data on porewater chemistry and peat characteristics are compiled in Tables 1, 2. A detailed description of conducted bulk peat analyses (total contents of phosphorus, carbon, nitrogen, iron, and organic matter), porewater sampling, and chemical analysis of dissolved matter concentrations (phosphate, ammonium, dissolved organic carbon, sulfate, iron, and calcium) can be found in Zak et al. (2010). Furthermore, plant samples (roots or moss litter) were taken from site Triebschsee (Carex appropinquata, brown mosses) and an additional natural poor fen, Kablow-Ziegelei (Eriophorum angusitfolium, Vaccinium oxycoccos, Sphagnum spp.) and treated analogously to the peat samples.
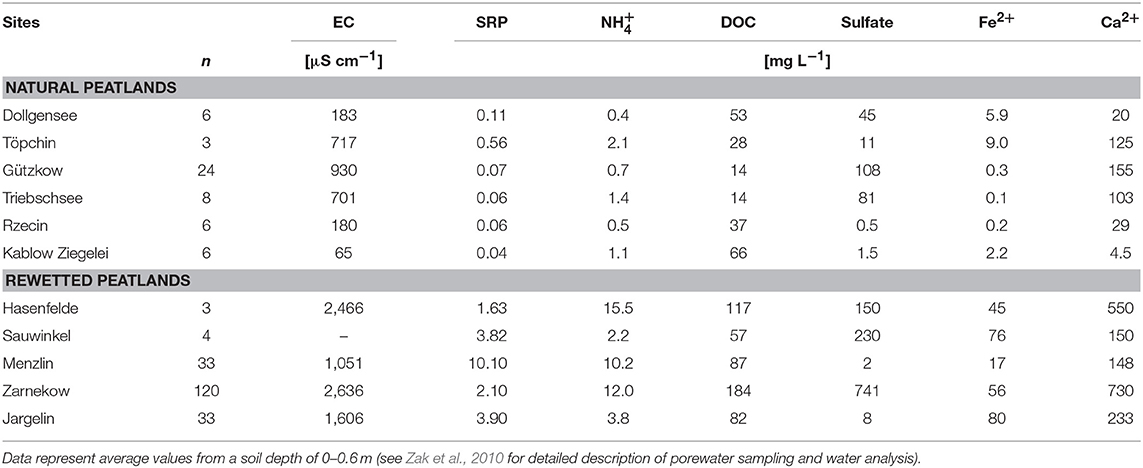
Table 1. Select data on porewater chemistry (EC—electrical conductivity, SRP—soluble reactive phosphorus).
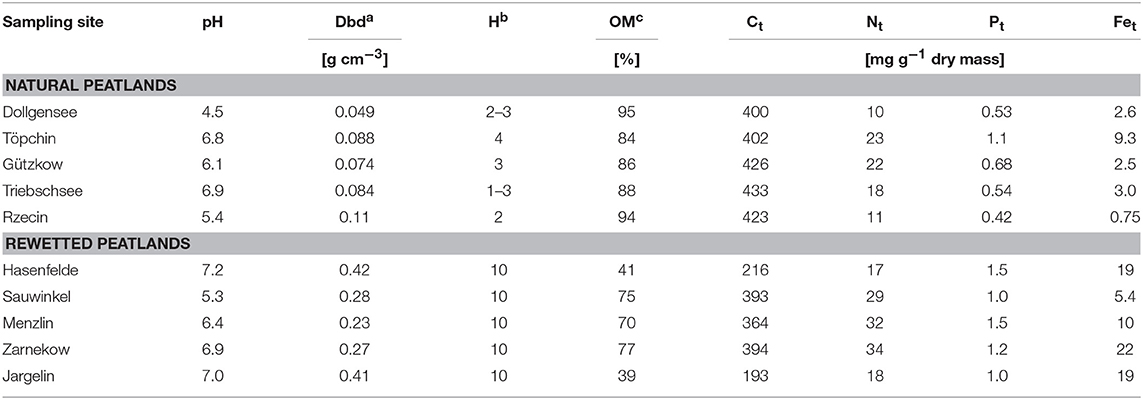
Table 2. Selected peat characteristics of sampling sites: (Ct, Nt, Pt, and Fet–total contents of carbon, nitrogen, phosphorus, and iron), dry bulk density (Dbd) calculated from the dry matter according to Scheffer and Blankenburg (1993), the degree of peat decomposition (H) according to von Post-scale (Von Post, 1922) and organic matter (OM) in % of dry matter determined by loss on ignition.
Polyphenol Analysis
Sample Preparation and Extraction of Phenolic Substances
For the extraction of phenols, we followed the protocol of Hagerman (2002) taking into the account that phenols are not stable at light and higher temperature with slight adaptations. Thus, after removing coarse living plant roots, collected peat samples were freeze-dried, finely ground in an agate ball mill, and stored at 18°C in the dark. Phenolic substances were extracted with 10 mL 70% aqueous acetone (v/v) three times sequentially from 0.2 g of the lyophilized peat material in screw cap plastic tubes. During extraction, tubes were suspended for 20 min in an ultrasonic water bath cooled to temperatures below 20°C. The used sonicator allowed samples to be maintained in the dark during the extraction period. The extracts were decanted after centrifugation at 10,600 g for 5 min (Universal 30F, Hettich, Tuttlingen, Germany) and combined in Erlenmeyer flasks on ice for further analysis. For the acid butanol assay and for purification of PAs from the extracts, 2 mg ascorbic acid L−1 were initially added to the 70% aqueous acetone (v/v) to prevent oxidation of the extracted PAs. No ascorbic acid was added when extracts were used for the analysis of total phenolics since ascorbic acid strongly interferes with the color reaction by reducing the Folin-Ciocalteau reagent (Box, 1983; Georgé et al., 2005).
Isolation of Proanthocyanidins
PAs were obtained from peat samples of the five pristine sites following Giner-Chavez et al. (1997), where water-solved PAs are precipitated with trivalent ytterbium acetate, with slight modifications. The extraction procedure for obtaining of PAs was performed as described before with the exception that 1 g instead of 0.2 g of the lyophilized peat were extracted in order to yield higher amounts of tannins. The extraction was performed in six parallel runs to gain sufficient tannin material from each peat soil. After combination the extracts were washed three times with 50 ml n-hexane to remove lipids, and three times with 50 ml ethyl acetate to remove other remaining non-tannin components from the crude peat extract. Rotoevaporation was used in addition to separating funnels to remove acetone and traces of organic solvents yielding approximately 40 mL of an aqueous tannin solution. Ten ml 0.1 M ytterbium acetate (Yb(C2H2O2)3 · 4H2O) and 2 ml 0.5 M triethanolamine as buffer (N(CH2CH2OH)3) were added to this solution and the extracts were stored in the dark for 20 h at 4°C to precipitate solved tannins. After centrifugation, the pellet was washed twice with 20 mL of 70% acetone (v/v) and PAs were recovered from the formed complexes using cation exchange resin (Nyamambi et al., 2000). For this purpose, tannin ytterbium complexes were mixed with 2 g exchange resin (Amberlite IRP-64, Sigma-Aldrich, Schnelldorf, Germany) and with three times 40 mL 70% acetone (v/v) sequentially in screw top glass tubes placed in a cooled sonicator for 20 min. Free PAs left in solution were then separated from the resin by centrifugation and filtration, from acetone by rotoevaporation and freeze dried to yield solid samples.
The PAs were used to determine their inhibitory activity on enzymes originating from peat, and as internal standards for colorimetric analyses. Analysis of UV spectra of the colored anthocyanidins formed during the acid butanol assay were conducted to verify the quality of isolated PAs and their suitability as internal standards (Figure 3).
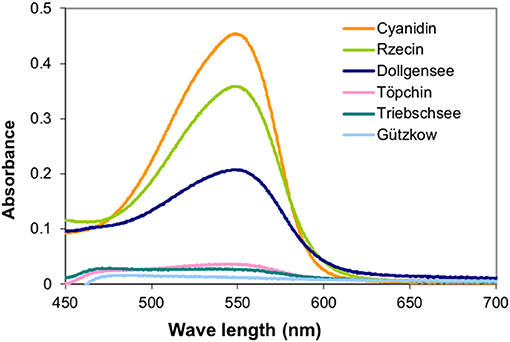
Figure 3. UV spectra of the colored anthocyanidins formed during the acid butanol assay were conducted to verify the quality of separated PAs and their suitability as internal standards for pristine peatlands.
Quantification of Total Phenolics
The simple, non-specific Folin-Ciocalteau assay quantifies total concentrations of phenolic hydroxyl groups in plant extracts (Box, 1983). The method was slightly modified most notably by using only half of the given volumes and reducing reaction times to 30 min (Hilt et al., 2006). 0.3–0.5 mL aliquots of the combined extracts were increased to volumes of 5 ml with deionized water in 10 mL screw cap glass tubes to obtain absorbance below 0.5. After addition of 0.75 ml of sodium carbonate solution (75 g Na2CO3 L−1) and 0.25 ml Folin-Ciocalteau reagent (Merck KGaA, Darmstadt, Germany), tubes were vortexed for 5 s. Absorbance was read at 750 nm (Photometer Spekol 221, Iskra Elektronik, Stuttgart, Germany) exactly 30 min after addition of the Folin-Ciocalteau reagent. The assay was calibrated with tannic acid (Fluka/Sigma-Aldrich, Munich, Germany) and tannins isolated from peat material. Analyses for polyphenols were carried out on three technical replicates per field sample. The same holds for all steps regarding the general chemical characterization of the field samples.
Quantification of Hydrolysable Tannins
Hydrolysable tannins were measured after saponisation and extraction after Luthria and Pastor-Corrales (2006) modified or peat soils. Specifically, 0.2 g of the lyophilized and grounded peat was digested by adding 2.5 mL 2 M NaOH containing 10 M EDTA and 1% ascorbic acid in an ultrasonic water bath at 45°C. The extraction was conducted with 4 mL ethyl acetate after acidification with 7.2 mL 2 M HCl. After shaking for 30 min the samples were centrifuged at 10,600 g for 5 min. The extraction was repeated one time and combined supernatants were dried by rotoevaporation under nitrogen atmosphere. Capturing was done with 300 μL 0.1% formic acid. Analyses were conducted using High-Performance Liquid Chromatography (Gynkotek) with UV detection at 280 nm. Separation was achieved using the column Phenomex Prodigy PH3 5 μ (250 × 4.6 mm) at constant flow mode and temperature (1.9 mL min−1, 25°C). The mobile phase was a constant mixture of methanol/0.1 % HCOOH (80%/20%, v/v). For calibration, gallic acid was used (Fluka/Sigma-Aldrich, Munich, Germany).
Quantification of Condensed Tannins
Condensed tannins or PAs were, respectively, measured with the acid butanol method (Porter et al., 1986), by adding 6 mL acid butanol reagent (nbutanol/concentrated HCl, 95:5 by volume) and 0.2 mL iron reagent (2% ferric ammonium sulfate dodecahydrate salt, FeNH4(SO2)4 · 12H2O, in 2 M HCl) to 1 ml extract solution. Samples were then vortexed for 5 s and placed for 50 min in a water bath at 95°C. After cooling the samples to room temperature, absorbance was measured at 550 nm (Photometer Spekol 221, Iskra Elektronik, Stuttgart, Germany). Since samples from different peat soils were already colored to different extents before heating, absorbance was also read before the production of colored anthocyanidins and subtracted from the values measured after heating. This approach led to a conservative estimate of tannin concentrations, since some chromophores might have been destroyed upon heating (Hagerman, 2002). Analyses of UV spectra (200–700 nm) were conducted to confirm the subtraction of background color. The acid butanol assay was calibrated with cyanidin chloride (Carl Roth, Karlsruhe, Germany) and the tannins isolated from peat material.
Microbial Activity in Peat Soils
Fluorescein diacetate (FDA) hydrolysis was performed to measure the enzyme activity of microbes according to Schnürer and Rosswall (1982) and optimized for peat analysis. Always, ca. 0.6 g (±0.1 g) fresh peat were directly weighed into screw cap glass tubes out of ca. 15 g fresh bulk peat sample. The samples were mixed with 4 mL potassium phosphate buffer (pH 7.6; 8.7 g K2HPO4 and 1.3 g KH2PO4 in 1 L distilled water), 1 mL distilled water, and 0.3 mL FDA solution (1 mg FDA in 1 mL acetone). Peat slurries were shaken then for 25 min on a rotary shaker and centrifuged at 4,200 g for 5 min (Heraeus Labfuge 400, DJB Labcare, Buckinghamshire, England). 0.5 mL of the supernatant was diluted with 1.5 mL potassium phosphate buffer solution before measurement at 490 nm on a spectrophotometer (Photometer Spekol 221, Iskra Elektronik, Stuttgart, Germany). Blanks were measured without the addition of FDA to correct for background absorbance and concentrations of released fluorescein were determined by calibration with a fluorescein sodium salt solution (C20H10Na2O5, Sigma Aldrich, Schnelldorf, Germany). Hydrolysis rates of FDA were expressed as μg fluorescein h−1 mg−1 oven dry (105°C) peat. Dry matter of aliquots withdrawn from the slurry and of weighed peat soils from pristine sites were measured for each analytical run.
Inhibition of Microbial Activity With Proanthocyanidins
To evaluate the inhibitory activity of PAs, the FDA hydrolysis assay was performed as described above, adding PAs dissolved in 1 mL distilled water to a 4 mL slurry just before starting the hydrolysis reaction with FDA solution. Absorbance of formed fluorescein at 490 nm was related to a reference sample that did not contain any tannin and results were expressed in percentage inhibition. PAs from the five pristine sites as well as tannic acid (Fluka/Sigma-Aldrich, Munich, Germany) as model substance were added to yield final concentrations of 100 mg L−1 in the test tubes. This concentration corresponded to a tannin/peat ratio of ~1:140. Additionally, PAs from Rzecin and tannic acid were added to yield concentration related inhibition with concentrations between 0–150 mg L−1 and 0–400 mg L−1, respectively. All inhibition tests were conducted with highly decomposed peat material from a long-term experiment containing peat from Zarnekow (Zak and Gelbrecht, 2007). Analyses of microbial activities and inhibition of microbial activity was carried out on four to five technical replicates of each field sample.
Statistical Analysis
Statistical tests were performed using SPSS 14.0 for windows (SPSS Inc., 2005) and R (R Core Team, 2018). On the one hand, we simply compared the variables introduced above across two peatland types—degraded and near-natural—treating the samples from the different sites as replicates among peatland types. We analyzed whether there were significant differences between samples from these two peatland types with respect to the inhibitory activity of proanthocyanidins on enzyme-activity in samples, the amounts of phenolics as well as of hydrolysable and condensed tannins, and microbial activity using either one-way ANOVA or alternatively the parameter free Kruskal–Wallis test when conditions for the ANOVA were not satisfied. Significance was tested against an α level of 0.05.
On the other hand, we investigated the relationships between the total concentrations of phenolic hydroxyl groups (A750) and condensed tannins (A550), the estimated total microbial activity according to the FDA analysis (FDA), and the multivariate characteristics of the peat (OM, C:N, C, N, P, Fe:P, P, bulk density, von Post scale) with NMDS (non-metric multi-dimensional scaling) ordination. NMDS allows for the exploration of multivariate data in low dimensional space. The NMDS based on the characteristics of the peat was performed with function metaMDS() of package vegan (Oksanen et al., 2017) for R with default settings. Sub-sequentially we fitted the above stated variables to the ordination result using the function envfit() of package vegan. This approach is closely related to the concept of multiple regression and allows to investigate the possible relationship of a number of predictors on the multivariate configuration of the peat samples defined by all their relevant characterizing variables. The significance of relationships was determined with the build in permutation test of envfit() (999 runs). Variables with p < 0.05 were deemed significantly related to the ordination configuration.
To test whether microbial activity was determined by inhibitory compounds (A750, A550) and/or some of the other peat characterizing variables we constructed a multiple linear regression with estimated total microbial activity (FDA) as response and all variables that were identified as significant in the NMDS as drivers. The primary model was then further simplified using the function step() in R that runs an iterative approach of combined forward and backward selection, using the lowest AIC as selection criterion for deciding on the final regression equation.
Results
Proanthocyanidins
Extraction of PAs from the different slightly decomposed peat soils yielded <1% of initial peat material on a dry matter basis. Although precipitation of tannins with trivalent ytterbium was not used for gravimetric quantification, it was a good indicator of tannin concentration in washed extract solutions. Extracts from peat of the pristine sites Rzecin and Dollgensee almost immediately showed precipitation of tannin-ytterbium complexes whereas peat extracts from Gützkow precipitated small amounts only perceptible after centrifugation. Calibrations of the acid butanol and the Folin-Ciocalteau assay with separated tannins are presented in Figure 4. Separated tannins from Gützkow, Triebschsee and Töpchin were characterized by particularly low absorbance values (<0.035 at 550 nm) in the acid butanol assay. Analysis of UV spectra showed that, in the case of tannins isolated from Gützkow peat, no absorbance maximum could be detected at 550 nm (Figure 3) although tannin concentrations were as high as 0.61 mg mL−1 (Figure 4). In the cases of Triebschsee and Töpchin, absorbance maxima could be identified but were not significantly different from background absorbance. Therefore, only calibrations for peat from the sites Rzecin and Dollgensee were used as internal standards for the quantification of PAs. All tannins, however, showed relevant concentrations of total phenolics during the Folin-Ciocalteau assay. Comparison of internal standards and the commercially available standards cyanidin chloride and tannic acid illustrates the advantage of internal standards. In any case, quantification with cyanidin or tannic acid would lead to a considerable underestimation (80–97%) of phenolic concentrations in peat soils (Table 3). Unfortunately, the purification of internal standards is impractical when peat samples contain only small amounts of tannins. Consequently, as no adequate internal standards could be separated for each peat soil, especially not for highly decomposed peat, further comparisons of concentrations were conducted with values expressed in absorbance units (AU) per dry mass (AU750/AU550 g−1 DM). However, to enable comparison with other studies, AU values were translated to commonly used tannic acids equivalents and cyanidin equivalents (TAE/CAE g−1 DM) as summarized in Table 3.
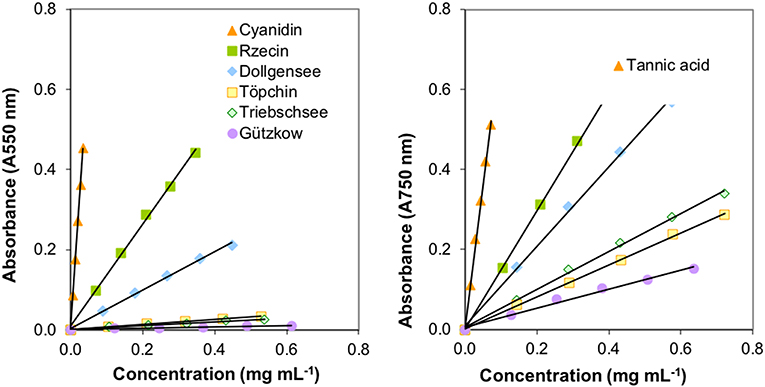
Figure 4. Calibrations of the acid butanol (left image) and the Folin-Ciocalteau assay (right image) with isolated tannins from less decomposed peat of pristine peatlands.
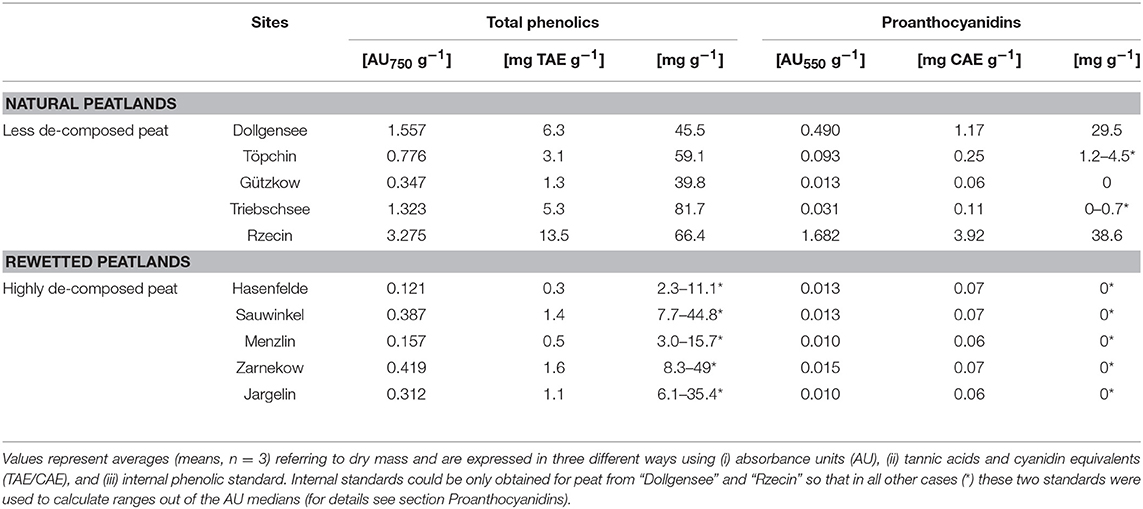
Table 3. Total phenolics and proanthocyanidins in differently decomposed peat soils referring to dry mass.
Polyphenol Contents
Absorbance values of extractable total phenolics and PAs ranged between 0.121–3.275 AU750 g−1 DM and 0.01–1.682 AU550 g−1 DM, respectively (Table 3). Hydrolysable tannins were not detectable in any of the peat samples. Both total phenolics and PAs were significantly higher in peat soils from pristine sites than in peat soils from degraded sites, with a strong correlation between both factors (Spearman's R 0.92). Notably, peat from Gützkow did not show higher values in comparison to the degraded sites and peat from Triebschsee had comparatively high concentration of total phenolics not reflected in high PA concentrations. The two sites Rzecin and Dollgensee stood out by their 139- and 40-fold higher concentrations of extractable PAs compared to degraded sites. Calibration of the acid Butanol and Folin-Ciocalteau assay with the same internal standard allowed an estimate of the size of the PA fraction in terms of total phenolics for peat from Rzecin and Dollgensee. Here, proanthocyanidins accounted for 58 and 65% of total phenolics, respectively, with total phenolics representing 6% of peat soil organic matter on an average.
Microbial Activity and Inhibition
The microbial activity of studied peat soils measured as hydrolysis rate of FDA ranged between 0.4 and 1.57 μg fluorescein h−1 mg−1 DM (Figure 5). The production of fluorescein was not significantly lower in the slightly decomposed peat soils, although Dollgensee and Triebschsee clearly had the lowest rates of FDA hydrolysis. However, peat from Gützkow showed very high activities for an only slightly decomposed peat soil, with presumably low microbial activities, and had the second highest activity after Menzlin.
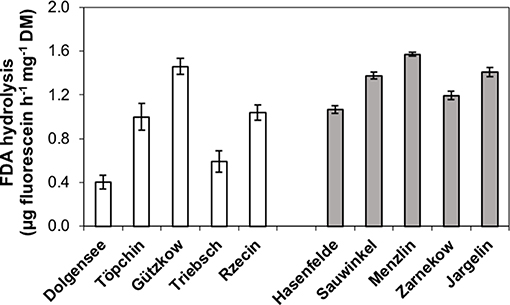
Figure 5. Microbial activity of studied peat soils measured as hydrolysis rate of fluorescein diacetate (FDA) for pristine peatlands with less decomposed peat (white bars) and rewetted peatlands with highly decomposed peat (gray bars).
All extracted tannins from pristine sites generated a significant inhibition of FDA hydrolysis when added to highly decomposed Zarnekow peat, with the exception of tannins from Gützkow peat (Figure 6). Significant inhibitions ranged between 12.3 and 17.9% and were achieved without any preliminary incubation times of tannins mixed with peat material. These values were lower compared to the inhibition produced by the addition of tannic acid as model substance (30.1%). Suppression of FDA hydrolysis with tannins was poorly reproducible between runs, most probably because of the great heterogeneity of the analyzed peat material. We attribute varying inhibition to the fact that inhibition tests with 100 mg tannins L−1 were below saturation point (Figure 7).
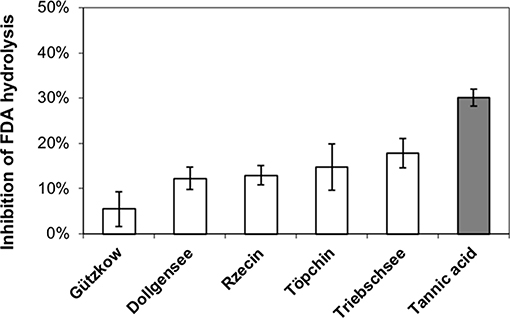
Figure 6. Inhibition of fluorescein diacetate (FDA) hydrolysis in highly decomposed peat from rewetted peatland “Zarnekow” using isolated tannins from less decomposed peat of pristine peatlands (white bars) and commercial tannic acid standard (gray bar).
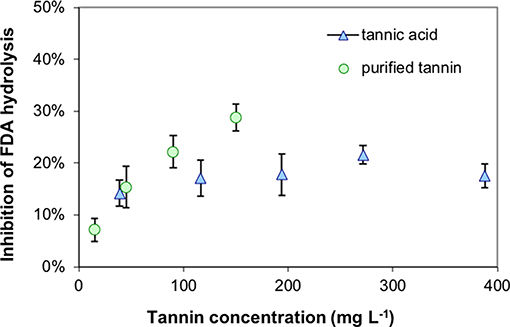
Figure 7. Suppression of fluorescein diacetate (FDA) hydrolysis with increasing concentrations of tannins at the example of highly decomposed peat from rewetted peatland “Zarnekow” using commercial tannic acid and a isolated tannin of less decomposed peat from pristine peatland “Rzecin”.
Relationships Between Variables
The peat from the pristine and the altered sites are clearly separated in the NMDS plot (Figure 8) while the generalizing characteristic of the pH value (size of bubbles in Figure 8) doesn't seem to play an important role. The iron contents in the sites, mainly the Fe2+ concentrations in the porewater, have a strong influence on the ordination pattern and are associated with relatively low contents of organic matter (OM) in the peat. A similar negative relationship appears for the microbial activity (as measured with FDA) and the two variables representing total phenolic compounds (A750) and condensed tannins (A550) that were fitted post-hoc to the ordination and point at opposite directions (Figure 8). Interestingly these variables don't follow the pristine—rewetted gradient but are almost orthogonal to the latter. Only A750 is significantly related to the ordination configuration. While C and N in the peat show a similar direction like the organic matter, the CN ratio increases with the content of polyphenolics. Soluble reactive phosphorus increases in the opposite direction and thus relates well to increased microbial activity (Figure 8). We tested all variables for relationships to the microbial activity. Only three showed significant relation according to singular linear models: N content, A550, and A750 after omitting Rzecin which had a much lower Fe content (with high coefficient of variation) and a much higher total phenolic content (A750) than all other samples. Only total polyphenol content (A750) had a strong negative linear relationship with microbial activity (R2 = 0.83, p < < 0.001; Figure 9). This result was confirmed by the multiple regression approach. While N content, A750 and A550 (including a possible interaction between the latter) entered the analysis, the final model contained only total polyphenol content as a driver and was, thus, essentially the model depicted in Figure 9.
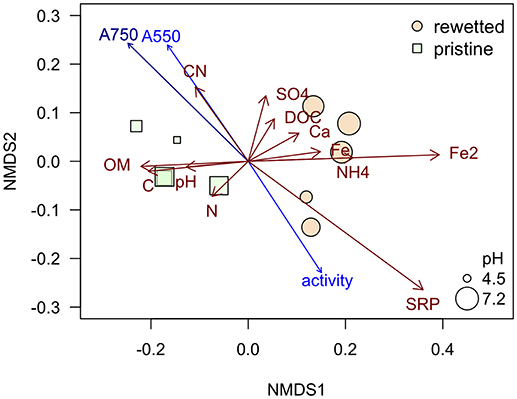
Figure 8. NMDS plot based on the chemical characteristics of the peat and porewater analyses. The NMDS was run with defaults using function metaMDS of package vegan in R. Final stress was 0.059. Red arrows represent the included variables with vector lengths ≥0.1. Blue arrows are the post-hoc fitted variables on phenolic compounds and FDA, dark blue represents a variable that is significantly related to the ordination configuration with p < 0.05.
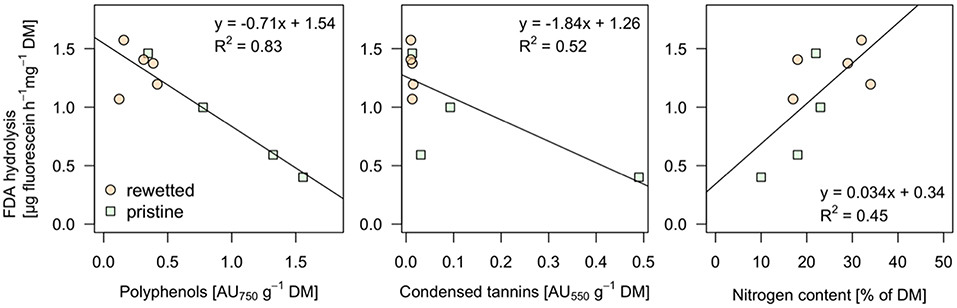
Figure 9. Significant linear relationships between microbial activity and other measured variables. The microbial activity was determined as enzyme activity using fluorescein diacetate (FDA) hydrolysis. Values are given on dry mass (DM) basis. The complete variable set (peat chemistry, pore water chemistry, and potentially inhibiting substances) were tested. Only relationships with linear models significant with p < 0.05 are displayed.
Discussion
Supporting our initial hypothesis, we found that highly degraded peat contained eight times lower levels of total polyphenols and 50 times lower levels of condensed tannins than less decomposed peat. Thus, draining and subsequent peat degradation effectively removes major parts of the tannin stock of fens by former mentioned oxidation processes and eliminates their potential function as an “enzymic latch” on the fen carbon store. The total phenolic contents of less decomposed peat from the pristine fens in this study (Table 3) corresponded well with concentrations of less decomposed Sphagnum peat (ca. 4–8 mg TAE g−1 DM) from different European peatlands (Dorrepaal et al., 2005; Bragazza and Freeman, 2007). Since peat-parent material in our study was mostly polyphenol-rich Carex spp. (Table 4) higher contents could be expected but certain Carex species might differ strongly regarding polyphenol contents/composition and some part of polyphenols might be decomposed during peat formation (see below). The concentrations in peat porewater were much higher and ranged up to 100 mg L−1. To put this into perspective, inhibitory effects on hydrolase activity were already recorded under phenolic contents between 2 and 5 mg L−1 for a Welsh peatland (Freeman et al., 2004). Soil pore water was not analyzed for polyphenols in this study, however according to the amount of water-extractable polyphenols of 0.7 mg TAE g−1 DM and a dry bulk density of 100 g L−1, as determined for peat from Dollgensee (results not shown) inhibition-relevant porewater concentration of about 70 mg TAE L−1 can be expected.
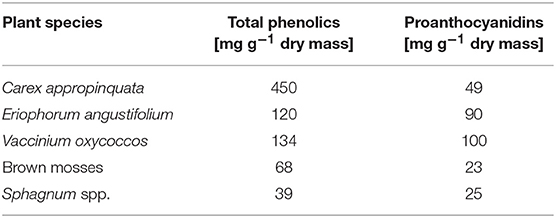
Table 4. Average contents of total phenolics and proanthocyanidins (median, n = 3) in roots from selected peat-forming vascular plant and from moss litter (for plant sampling sites see section Study sites and sampling).
Naturally, the complex nature of polyphenols with potentially more than 8,000 individual molecule structures cannot be reflected in the analysis of total phenolics. Nevertheless, this unspecific proxy has shown some good correlation with decomposition patterns in peatland studies (Dorrepaal et al., 2005; Bragazza and Freeman, 2007; Bragazza et al., 2007) and has proven to be a suitable predictor for tannin inhibition of microbial growth of ruminal bacteria (Nelson et al., 1997). Individual processes such as litter decay, however, may depend much more on the type of polyphenol, rather than just on the total content. Despite their large diversity, their functioning with regards to metabolic processes can be classified as “ineffective,” “inhibiting,” or “promoting” (e.g. Bonnett et al., 2017). The latter refers inter alia to their ability to mediate redox-processes in soils as described for the quinone already in the beginning of the Twentieth century (Erdtman, 1933a,b). Likewise, the inhibitory properties of phenolics are well-known even for more than 100 years for sphagnum-derived phenolic acids as earlier reviewed by Verhoeven and Liefveld (1997). The suppression of microbial activity in Sphagnum peat was partially attributed to a tanning-like process involving 5-keto-D-mannurionic acid, associated with contributing to the famous preservation property of Sphagnum bogs (Vanbreemen, 1995). In vascular plant-dominated minerotrophic peatlands, tannins e.g. derived from Eriophorum spp. (Williams et al., 2000), could play an equally important role in the inhibition of microbial activity. Accordingly, it seems advantageous to obtain more detailed characterization of the biochemically diverse tannin fraction than to measure total phenolic contents alone.
The better solubility of hydrolysable tannins in water and their distinct complexing behavior, which depends on prevailing pH and redox conditions (Martin and Martin, 1983; Appel, 1993), promote them as potent enzyme inhibitors. On the other hand, their stability even in the form of tannin-protein-complexes may be too low to persist in the long term (Bravo, 1998; Fierer et al., 2001). According to the absence of hydrolysable tannins in all tested peat soils, the inhibiting role of tannins must be solely attributed to the group of condensed tannins. Unfortunately, there is no reference for PA concentrations in peat, but some information is available for plant samples. For example, for conifers, the proportion was higher than 50% of total phenolic content, similar to peat samples from the natural sites Dollgensee and Rzecin. While our data analysis shows that isolated PAs from less decomposed peat of natural peatlands inhibited the activity of hydrolases (Figure 7), enzyme activities did not differ significantly between different degraded peat samples (Figure 5). Still, at least when correcting for an obvious outlier we find a strong linear relationship between total polyphenol content and microbial activity (Figure 9), supporting the validity of the “enzymic latch” for fen peat. However, the linear relationship between the content of condensed tannins and microbial activity hinges on one data point only and the data rather suggest a threshold below which microbial activity can go up.
We assume that enzyme activity levels and decomposition of organic matter are not only related to the presence or absence of polyphenols, but are a concert of multiple factors and processes which is also represented in the direction of the dichotomy between microbial activity and the inhibitory compounds in the NMDS plot which does not follow the gradient from pristine to altered sites that is otherwise well-represented along the first NMDS axis. Yet another limiting factor is the availability of resources (C and other nutrients) for decomposing organisms (Yavitt et al., 2005). Litter decomposition thus depends on substrate quality, e.g., C:N ratio, and lignin content. The bulk parameter C:N ratio scales well with the amount of potentially inhibitory substances in the NMDS. Polymers such as cellulose and hemicelluloses are readily degraded by microbial exoenzyme systems into energy-yielding dimers and monomers. Lignin or lignin-like phenolic polymers as found in Sphagnum are more recalcitrant in comparison.
Plant litter with high nutrient concentration generally decomposes relatively fast (Couteaux et al., 1995; Laiho, 2006). In Sphagnum litter, Bragazza et al. (2007) found the polyphenol/nutrient ratio to be one of the primary parameters controlling decomposition. Unlike in pristine bog peatlands, the decomposition of organic matter in the degraded and rewetted fens is strongly controlled by the biogeochemical regime including the elevated availability of electron acceptors like ferric iron and sulfate, enhanced nutrient availability, and circum-neutral pH (Zak and Gelbrecht, 2007; Brouns et al., 2014). Respiration measurements with different organic substrates from rewetted peatlands suggest that degraded peat without any fresh plant-derived material is relatively inert in terms of decomposition, whereas significant anaerobic CO2 and CH4 production in peat only occurs when enough labile organic matter is available (e.g., from remaining roots or root exudates; Hahn-Schöfl et al., 2011). The role of polyphenols for the decomposition of fresh aboveground litter and from wetland plants was tested by anaerobic incubation experiments showing that the availability of nutrients rather than polyphenol contents were significant factors (Zak et al., 2015). The polyphenol concentrations of tested plant litter samples ranged between 14 and 68 mg g−1 DM and were, thus, at the lower level of root and moss litter from this study (39–450 mg g−1 DM, Table 3). Unfortunately, the proportion of tannins was not quantified in this work, however it was interesting to note that in non-peat forming plants (Phalaris arundinacea, Ceratophyllum demersum, Typha latifolia) the content of polyphenols was reduced between 50 and 95%, while in the peat forming plants (Phragmites australis, Carex riparia) the reduction was much lower (<20% after 150 days).
At time of peat sampling, non-peat forming plants still dominated the studied rewetted sites. After 10 to 20 years of rewetting, however, a distinct succession toward peat-forming reed communities is evident, while the re-colonization with brown mosses and low sedges characterized by higher polyphenol contents seems to be retarded over several decades, depending on the drainage and land use history (Zerbe et al., 2013; Zak et al., 2015) as observed in other rewetting projects (Koch et al., 2017). Also, if peat forming plants are re-established the quality and quantity of polyphenols in plant tissues might differ substantially from their natural counterparts as long as nutrient levels are higher as under pre-drained conditions. Low nutrient levels are known for promoting the production of secondary metabolites inter alia to protect the plants against herbivory or pathogens (Verhoeven and Liefveld, 1997). All things considered, it remains uncertain about how long it would take for enzyme-inhibiting polyphenols in soils to reach concentrations that would have a significant impact on microbial metabolism. For such an assessment it might also be considered that phenol oxidases could be activated under oxygen-free conditions in case of elevated concentrations of other electron acceptors, such as sulfate and oxidized but soluble or less crystalline ferric iron (Van Bodegom et al., 2005; Fenner and Freeman, 2011). Thus, elevated sulfate concentrations in soil water of the pristine sites Gützkow and Triebschsee (>80 mg L−1, Table 1) might be responsible for the comparatively low polyphenol contents in peat from such sites. Eventually, there might be other anaerobic decomposition pathways depending on certain abiotic factors and/or presence of fungal and bacterial communities (e.g., Fuchs et al., 2011) which needs detailed consideration in order to better understand different patterns of organic matter decomposition in peatlands.
Conclusions
The drainage of fens and their use as grassland over approximately three decades caused a significant loss of condensed tannins. Accordingly, organic matter decomposition may continue at about the same pace as before the rewetting because of the (almost) absence of the “latch” provided by the phenols. Despite their presumed role as enzyme-inhibiting substances, hydrolysable tannins may play an insignificant role in decomposition processes since they do not accumulate in the peat in contrast to condensed tannins. Their capacity to reduce the microbial enzyme activity was clearly confirmed in assays using tannins from less decomposed peat of pristine fens. However, the substantial differences in polyphenol contents, in particular for condensed tannins, did not correspond with significant differences in hydrolytic enzyme activities in highly decomposed peat compared to less decomposed peat. Future research must clarify if and how long it may take after rewetting for polyphenol content and composition to return to those of pristine fens, and whether the repression of hydrolase enzymes eventually resumes, as this will be important for the long-term carbon accumulation. According to the high complexity of possible metabolic processes and driving factors both under aerobic and anaerobic conditions the measurement of single parameters like redox-conditions, nutrient availability or pH will be not sufficient to make a reasonable estimate of carbon turnover in rewetted peatlands. However, it can be presumed that the re-establishment of peat-forming Carex species or brown mosses characterized by high tannin contents could represent the switch for the “enzymic latch” mechanism. Another important precondition might be a significant reduction of electron acceptor availability and nutrient levels (Brouns et al., 2016). Altogether, there is evidence that polyphenol elimination by fen drainage must be ameliorated before rehabilitation of rewetted peatlands in the context of carbon cycling can be considered a success in decades to come. The removal of the degraded peat layer can be a suitable method to re-establish low-nutrient conditions and facilitate the re-colonization by peat-forming plants within few years only (Zak et al., 2017). However, top soil removal can be a rather expensive measure and, particularly if the objective of the peatland restoration is to target mitigation of climate change, its carbon footprint needs to be carefully assessed (Zak et al., 2018). Alternatively, harvesting of wetland helophytes and their commercial use (Wichmann, 2017) can be another viable option to support the succession toward natural conditions but again longer time periods (>>10 years) must be tolerated (Zak et al., 2014).
Data Availability Statement
The datasets generated and analyzed for this study can be found in an electronic supplement to this article (Data_Supplement-Zak_et_al.xlsx in Supplementary Material).
Author Contributions
DZ and CR designed the study and CR conducted the experiments. CR and GJ performed data analysis. DZ and CR compiled the manuscript. All authors contributed to the text.
Funding
The study was supported by Deutsche Forschungsgemeinschaft (DFG), Grant/Award Number: ZA 742/2-1; Department of Environment of Mecklenburg-Vorpommern, Grant/Award Number: LUNG-230-5325.60-7-34; European Agriculture Guidance and the Guarantee Fund, Grant/Award Number: LUNG-230-5325.60-7-34. Finalization of the study was funded by the European Social Fund (ESF) and the Ministry of Education, Science and Culture of Mecklenburg-Western Pomerania within the scope of the project WETSCAPES (ESF/14-BM-A55-0030/16). VU was financed within the framework of the Research Training Group Baltic TRANSCOAST funded by the DFG under grant number GRK 2000.
Conflict of Interest
The authors declare that the research was conducted in the absence of any commercial or financial relationships that could be construed as a potential conflict of interest.
Acknowledgments
Many thanks to all colleagues of the Department of Chemical Analytics and Biogeochemistry of the Leibniz-Institute of Freshwater Ecology and Inland Fisheries supporting the comprehensive chemical analyses and for their technical assistance during fieldwork. In particular we gratefully acknowledge Jörg Gelbrecht for advice and help with peat and plant sampling. Steffen Vogler, Etienne Brunet, and Angela Krüger are acknowledged for conducting microbial activity tests and phenol analysis.
Supplementary Material
The Supplementary Material for this article can be found online at: https://www.frontiersin.org/articles/10.3389/fenvs.2019.00147/full#supplementary-material
References
Appel, H. M. (1993). Phenolics in ecological interactions - the importance of oxidation. J. Chem. Ecol. 19, 1521–1552. doi: 10.1007/BF00984895
Bader, C., Müller, M., Schulin, R., and Leifeld, J. (2018). Peat decomposability in managed organic soils in relation to land use, organic matter composition and temperature. Biogeosciences 15, 703–719. doi: 10.5194/bg-15-703-2018
Bonnett, S. A. F., Maltby, E., and Freeman, C. (2017). Hydrological legacy determines the type of enzyme inhibition in a peatlands chronosequence. Scient. Rep. 7, 1–14. doi: 10.1038/s41598-017-10430-x
Box, J. D. (1983). Investigation of the folin-ciocalteau phenol reagent for the determination of polyphenolic substances in natural-waters. Water Res. 17, 511–525. doi: 10.1016/0043-1354(83)90111-2
Bragazza, L., and Freeman, C. (2007). High nitrogen availability reduces polyphenol content in Sphagnum peat. Sci. Total Environ. 377, 439–443. doi: 10.1016/j.scitotenv.2007.02.016
Bragazza, L., Siffi, C., Iacumin, P., and Gerdol, R. (2007). Mass loss and nutrient release during litter decay in peatland: the role of microbial adaptability to litter chemistry. Soil Biol. Biochem. 39, 257–267. doi: 10.1016/j.soilbio.2006.07.014
Bravo, L. (1998). Polyphenolics, dietary sources, metabolism and nutritional significance. Nutr. Rev. 56, 257–267. doi: 10.1111/j.1753-4887.1998.tb01670.x
Brouns, K., Keuskamp, J. A., Potkamp, G., Verhoeven, J. T. A., and Hefting, M. M. (2016). Peat origin and land use effects on microbial activity, respiration dynamics and exo-enzyme activities in drained peat soils in the Netherlands. Soil Biol. Biochem. 95, 144–155. doi: 10.1016/j.soilbio.2015.11.018
Brouns, K., Verhoeven, J. T. A., and Hefting, M. M. (2014). Short period of oxygenation releases latch on peat decomposition. Sci. Total Environ. 481, 61–68. doi: 10.1016/j.scitotenv.2014.02.030
Cabezas, A., Gelbrecht, J., Zwirnmann, E., Barth, M., and Zak, D. (2012). Effects of degree of peat decomposition, loading rate and temperature on dissolved nitrogen turnover in rewetted fens. Soil Biol. Biochem. 48, 182–191. doi: 10.1016/j.soilbio.2012.01.027
Couteaux, M. M., Bottner, P., and Berg, B. (1995). Litter decomposition, climate and litter quality. Trends Ecol. Evol. 10, 63–66. doi: 10.1016/S0169-5347(00)88978-8
Cowan, M. M. (1999). Plant products as antimicrobial agents. Clin. Microbiol. Rev. 12, 564–582. doi: 10.1128/CMR.12.4.564
Dorrepaal, E., Cornelissen, J. H. C., Aerts, R., Wallen, B., and van Logtestijn, R. S. P. (2005). Are growth forms consistent predictors of leaf litter quality and decomposability across peatlands along a latitudinal gradient? J. Ecol. 93, 817–828. doi: 10.1111/j.1365-2745.2005.01024.x
Erdtman, H. G. H. (1933a). Studies on the formation of complex oxidation and condensation products of phenols. A contribution to the investigation of the origin and nature of humic acid. 1. Studies of the reactivity of simple monocyclic quinones. Proc. Roy. Soc. A 143,177–191. doi: 10.1098/rspa.1933.0212
Erdtman, H. G. H. (1933b). Studies on the formation of complex oxidation and condensation products of phenols. 2. Coupling of simple phenols and quinones to diphenyl derivatives. Proc. Roy. Soc. A 143, 191–222. doi: 10.1098/rspa.1933.0213
Fenner, N., and Freeman, C. (2011). Drought-induced carbon loss in peatlands. Nat. Geosci. 4, 895–900. doi: 10.1038/ngeo1323
Fierer, N., Schimel, J. P., Cates, R. G., and Zou, J. (2001). Influence of balsam poplar tannin fractions on carbon and nitrogen dynamics in Alaskan taiga floodplain soils. Soil Biol. Biochem. 33,1827–1839. doi: 10.1016/S0038-0717(01)00111-0
Franz, D., Koebsch, F., Larmanou, E., Augustin, J., and Sachs, T. (2016). High net CO2 and CH4 release at a eutrophic shallow lake on a formerly drained fen. Biogeosciences 13, 3051–3070. doi: 10.5194/bg-13-3051-2016
Freeman, C., Ostle, N., and Kang, H. (2001). An enzymic 'latch' on a global carbon store - A shortage of oxygen locks up carbon in peatlands by restraining a single enzyme. Nature 409:149. doi: 10.1038/35051650
Freeman, C., Ostle, N. J., Fenner, N., and Kang, H. (2004). A regulatory role for phenol oxidase during decomposition in peatlands. Soil Biol. Biochem. 36,1663–1667. doi: 10.1016/j.soilbio.2004.07.012
Fuchs, G., Boll, M., and Heider, J. (2011). Microbial degradation of aromatic compounds – from one strategy to four. Nat. Rev. Microbiol. 9, 803–816. doi: 10.1038/nrmicro2652
Georgé, S., Brat, P., Alter, P., and Amiot, M. J. (2005). Rapid determination of polyphenols and vitamin C in plant-derived products. J. Agric. Food Chem. 53, 1370–1373. doi: 10.1021/jf048396b
Giner-Chavez, B. I., van Soest, P. J., Robertson, J. B., Lascano, C., Reed, J. D., and Pell, A. N. (1997). A method for isolating condensed tannins from crude plant extracts with trivalent ytterbium. J. Sci. Food Agric. 74, 359–368. doi: 10.1002/(SICI)1097-0010(199707)74:3<359::AID-JSFA811>3.0.CO;2-C
Gorham, E. (1991). Northern peatlands role in the carbon cycle and probable responses to climatic warming. Ecol. Appl. 1, 182–195. doi: 10.2307/1941811
Hagerman, A. E. (2002). The Tannin Handbook. Available online at: https://www.users.miamioh.edu/hagermae/ (accessed September 10, 2019).
Hahn-Schöfl, M., Zak, D., Minke, M., Gelbrecht, J., Augustin, J., and Freibauer, A. (2011). Organic sediment formed during inundation of a degraded fen grassland emits large fluxes of CH4 and CO2. Biogeosciences 8, 1539–1550. doi: 10.5194/bg-8-1539-2011
Hall, S. J., Treffkorn, J., and Silver, W. L. (2014). Breaking the enzymatic latch: impacts of reducing conditions on hydrolytic enzyme activity in tropical forest soils. Ecology 95, 2964–2973. doi: 10.1890/13-2151.1
Hättenschwiler, S., and Vitousek, P. M. (2000). The role of polyphenols in terrestrial ecosystem nutrient cycling. Trends Ecol. Evol. 15, 238–243. doi: 10.1016/S0169-5347(00)01861-9
Hernes, P. J., and Hedges, J. I. (2000). Determination of condensed tannin monomers in environmental samples by capillary gas chromatography of acid depolymerization extracts. Analyt. Chem. 72, 5115–5124. doi: 10.1021/ac991301y
Hilt, S., Ghobrial, M. G., and Gross, E. M. (2006). In situ allelopathic potential of Myriophyllum verticillatum (Halorgagaceae) against selected phytoplankton species. J. Phycol. 42, 1189–1198. doi: 10.1111/j.1529-8817.2006.00286.x
IPCC (2014). 2013 Supplement to the 2006 IPCC Guidelines for National Greenhouse Gas Inventories. Wetlands: IPCC.
Jenkinson, D. S., Adams, D. E., and Wild, A. (1991). Model estimates of CO2 emissions from soil in response to global warming. Nature 351, 304–306. doi: 10.1038/351304a0
Joosten, H. (2010). The Global Peatland CO2 Picture: Peatland Status and Drainage Related Emissions in All Countries of the World. Netherlands: Wetland International, Ede.
Joosten, H., Brust, K., Couwenberg, J., Gerner, A., Holsten, B., Permien, T., et al. (2015). MoorFutures® Integration of Additional Ecosystem Services (Including Biodiversity) Into Carbon Credits – Standard, Methodology and Transferability to Other Regions. Bonn: Bundesamt für Naturschutz (Federal Ministry for the Environment, BfN).
Koch, M., Koebsch, F., Hahn, J., and Jurasinski, G. (2017). From meadow to shallow lake: monitoring secondary succession in a coastal fen after rewetting by flooding based on aerial imagery and plot data. Mires Peat 19, 1–17. doi: 10.19189/MaP.2015.OMB.188
Kraus, T. E. C., Dahlgren, R. A., and Zasoski, R. J. (2003). Tannins in nutrient dynamics of forest ecosystems - a review. Plant Soil 256, 41–66. doi: 10.1023/A:102620651
Laiho, R. (2006). Decomposition in peatlands: reconciling seemingly contrasting results on the impacts of lowered water levels. Soil Biol. Biochem. 38, 2011–2024. doi: 10.1016/j.soilbio.2006.02.017
Luthria, D. L., and Pastor-Corrales, M. A. (2006). Phenolic acids content of fifteen dry edible bean (Phaseolus vulgaris L.) varieties. J. Food Comp. Anal. 19, 205–2011. doi: 10.1016/j.jfca.2005.09.003
Martin, J. S., and Martin, M. M. (1983). Tannin assays in ecological-studies - precipitation of ribulose-1,5-bisphosphate carboxylase oxygenase by tannic-acid, quebracho, and oak foliage extracts. J. Chem. Ecol. 9, 285–294. doi: 10.1007/BF00988046
Mole, S., and Waterman, P. G. (1987). A critical analysis of techniques for measuring tannins in ecological-studies II. Tech. Biochem. Def. Tann. Oecol. 72, 148–156. doi: 10.1007/BF00385059
Mueller-Harvey, I. (2001). Analysis of hydrolysable tannins. Anim. Feed Sci. Technol. 91, 3–20. doi: 10.1016/S0377-8401(01)00227-9
Nelson, K. E., Pell, A. N., Doane, P. H., GinerChavez, B. I., and Schofield, P. (1997). Chemical and biological assays to evaluate bacterial inhibition by tannins. J. Chem. Ecol. 23, 1175–1194. doi: 10.1023/B:JOEC.0000
Nyamambi, B., Ndlovu, L. R., Read, J. S., and Reed, J. D. (2000). The effects of sorghum proanthocyanidins on digestive enzyme activity in vitro and in the digestive tract of chicken. J. Sci. Food Agric. 80, 2223–2231. doi: 10.1002/1097-0010(200012)80:15<2223::AID-JSFA768>3.0.CO;2-I
Oksanen, J., Blanchet, F. G., Friendly, M., Kindt, R., Legendre, P., McGlinn, D., et al. (2017). Vegan: Community Ecology Package, R package version 2.4-5. Available online at: https://CRAN.R-project.org/package=vegan (accessed September 10, 2019).
Pind, A., Freeman, C., and Lock, M. A. (1994). Enzymatic degradation of phenolic materials in peatlands - measurement of phenol oxidase activity. Plant Soil 159, 227–231. doi: 10.1007/BF00009285
Pinsonneault, A. J., Moore, T. R., and Roulet, N. T. (2016). Temperature the dominant control on the enzyme-latch across a range of temperate peatland types. Soil Biol. Biochem. 97, 121–130. doi: 10.1016/j.soilbio.2016.03.006
Porter, L. J., Hrstich, L. N., and Chan, B. G. (1986). The conversion of procyanidins and prodelphinidins to cyanidin and delphinidin. Phytochemistry 25, 223–230. doi: 10.1016/S.0031-9422(00)94533-3
R Core Team (2018). R: A Language and Environment for Statistical Computing. Vienna: R Foundation for Statistical Computing. Available online at: https://www.R-project.org/ (accessed September 10, 2019).
Rautio, P., Bergvall, U. A., Karonen, M., and Salminen, J. P. (2007). Bitter problems in ecological feeding experiments: commercial tannin preparations and common methods for tannin quantifications. Biochem. System. Ecol. 35, 257–262. doi: 10.1016/j.bse.2006.10.016
Scheffer, B., and Blankenburg, J. (1993). The determination of the bulk-density of peat soils. Agribiol. Res. Zeitschr. Agrarbiol. Agrik. Okol. 46, 46–53.
Schnürer, J., and Rosswall, T. (1982). Fluorescein diacetate hydrolysis as a measure of total microbial activity in soil and litter. Appl. Environ. Microbiol. 43, 1256–1261.
Swain, T. (1979). “Tannins and lignins,” in Herbivores: Their Interaction With Secondary Plant Metabolites, eds G. Rosenthal and D. H. Janzen (New York, NY: Academic Press, 657–682.
Van Bodegom, P. M., Broekman, R., Van Dijk, J., Bakker, C., and Aerts, R. (2005). Ferrous iron stimulates phenol oxidase activity and organic matter decomposition in waterlogged Wetlands. Biogeochemistry 76, 69–83. doi: 10.1007/s10533-005-2053-x
Vanbreemen, N. (1995). How Sphagnum bogs down other plants. Trends Ecol. Evol. 10, 270–275. doi: 10.1016/0169-5347(95)90007-1
Verhoeven, J. T., and Liefveld, W. M. (1997). The ecological significance of organochemical compounds in Sphagnum. Acta Bot. Neerl. 46, 117–130. doi: 10.1111/plb.1997.46.2.117
Verhoeven, J. T. A., Arheimer, B., Yin, C. Q., and Hefting, M. M. (2006). Regional and global concerns over wetlands and water quality. Trends Ecol. Evol. 21, 96–103. doi: 10.1016/j.tree.2005.11.015
Von Post, L. (1922). Sveriges geologiska undersöknings torvinventering och några av dess hittills vunna resultat. Sven. Mosskulturfören. Tidskr 1, 1–27.
Wichmann, S. (2017). Commercial viability of paludiculture: a comparison of harvesting reeds for biogas production, direct combustion, and thatching. Ecol. Eng. 103, 497–505. doi: 10.1016/j.ecoleng.2016.03.018
Williams, C. J., Shingara, E. A., and Yavitt, J. B. (2000). Phenol oxidase activity in peatlands in New York state: response to summer drought and peat type. Wetlands 20, 416–421. doi: 10.1672/0277-5212(2000)020[0416:POAIPI]2.0.CO;2
Williams, C. J., and Yavitt, J. B. (2003). Botanical composition of peat and degree of peat decomposition in three temperate peatlands. Ecoscience 10, 85–95. doi: 10.1080/11956860.2003.11682755
Yavitt, J. B., Williams, C. J., and Wieder, R. K. (2005). Soil chemistry versus environmental controls on production of CH4 and CO2 in northern peatlands. Eur. J. Soil Sci. 56, 169–178. doi: 10.1111/j.1365-2389.2004.00657.x
Yu, Z., Loisel, J., Brosseau, D. P., Beilman, D. W., and Hunt, S. J. (2010). Global peatland dynamics since the last glacial maximum. Geophys. Res. Lett. 37:L13402. doi: 10.1029/2010GL043584
Zak, D., and Gelbrecht, J. (2007). The mobilisation of phosphorus, organic carbon and ammonium in the initial stage of fen rewetting (a case study from NE Germany). Biogeochemistry 85, 141–151. doi: 10.1007/s10533-007-9122-2
Zak, D., Gelbrecht, J., Zerbe, S., Shatwell, T., Barth, M., Cabezas, A., et al. (2014). How helophytes influence the phosphorus cycle in degraded inundated peat soils – implications for fen restoration. Ecol. Eng. 66, 82–90. doi: 10.1016/j.ecoleng.2013.10.003
Zak, D., Goldhammer, T., Cabezas, A., Gelbrecht, J., Gurke, R., Wagner, C., et al. (2018). Top soil removal reduces water pollution from phosphorus and dissolved organic matter and lowers methane emissions from rewetted peatlands. J. Appl. Ecol. 55, 311–320. doi: 10.1111/1365-2664.12931.
Zak, D., Meyer, N., Cabezas, A., Gelbrecht, J., Mauersberger, R., Tiemeyer, B., et al. (2017). Topsoil removal to minimize internal eutrophication in rewetted peatlands and to protect downstream systems against phosphorus pollution: a case study from NE Germany. Ecol. Eng. 103, 488–496. doi: 10.1016/j.ecoleng.2015.12.030.
Zak, D., Reuter, H., Augustin, J., Shatwell, T., Barth, M., Gelbrecht, J., et al. (2015). Changes of the CO2 and CH4 production potential of rewetted fens in the perspective of temporal vegetation shifts. Biogeosciences 12, 2455–2468. doi: 10.5194/bg-12-2455-2015
Zak, D., Wagner, C., Payer, B., Augustin, J., and Gelbrecht, J. (2010). Phosphorus mobilization in rewetted fens: the effect of altered peat properties and implications for their restoration. Ecol. Appl. 20, 1336–1349. doi: 10.1890/08-2053.1
Keywords: decomposition, enzyme inhibition, microbial activity, peatland restoration, Sphagnum, tannins
Citation: Zak D, Roth C, Unger V, Goldhammer T, Fenner N, Freeman C and Jurasinski G (2019) Unraveling the Importance of Polyphenols for Microbial Carbon Mineralization in Rewetted Riparian Peatlands. Front. Environ. Sci. 7:147. doi: 10.3389/fenvs.2019.00147
Received: 19 May 2019; Accepted: 17 September 2019;
Published: 04 October 2019.
Edited by:
Colin McCarter, University of Toronto Scarborough, CanadaReviewed by:
James McLaughin, Ontario Ministry of Natural Resources, CanadaJos Thomas Antonius Verhoeven, Utrecht University, Netherlands
Copyright © 2019 Zak, Roth, Unger, Goldhammer, Fenner, Freeman and Jurasinski. This is an open-access article distributed under the terms of the Creative Commons Attribution License (CC BY). The use, distribution or reproduction in other forums is permitted, provided the original author(s) and the copyright owner(s) are credited and that the original publication in this journal is cited, in accordance with accepted academic practice. No use, distribution or reproduction is permitted which does not comply with these terms.
*Correspondence: Dominik Zak, ZG96QGJpb3MuYXUuZGs=