- 1World Wildlife Fund, Global Science, Washington, DC, United States
- 2The Nature Conservancy, North America Region, Helena, MT, United States
- 3WWF-Mexico, Mexico City, Mexico
Water-management infrastructure, such as dams, diversions, and levees, provides important benefits to society, including energy, flood management, and water supply, but this infrastructure is a primary cause of the decline of freshwater ecosystems and the services they provide. Due to these declines, recent attention has focused on improving the environmental performance of water infrastructure, such as modifying the location, design, or operation of infrastructure to maintain or restore environmental flows. Despite growing attention to the importance of environmental flows, and continued advancement in flow assessment methods, implementation of flow protection or restoration has lagged expectations. In this paper we describe how pursuing environmental flows at the scale of infrastructure systems, rather than individual sites, such as a dam, offers two pathways to increased implementation of environmental flows. First, policy and management mechanisms that apply to large areas—river basins or political jurisdictions—can catalyze large-scale implementation of flow protection or restoration. We provide two examples of system-scale policy and management mechanisms: flow protection policies and system-scale hydropower planning and management. Although system-scale policy and management offer a clear path to large-scale implementation, there will continue to be a need for flow implementation that occurs at smaller scales, such as a high priority river reach. The second pathway focuses on implementation at that scale—such as environmental flow releases from a dam or small set of dams—but embeds dam reoperation or site-scale flow implementation within reoperation of the larger systems of resource management within which the dam or ecosystem is located. These systems of resource management can encompass various sectors and here we provide examples of dam reoperation or flow implementation facilitated by solutions that included changes to the management of (1) water supply systems; (2) floodplains; and (3) irrigation systems. We illustrate both of these system-scale pathways through a set of case studies, drawn primarily from North America, each of which includes an example of current implementation.
Introduction
Rivers and river-dependent ecosystems, such as floodplains and estuaries, support immense biological diversity and productivity. They also are among the most important ecosystems for providing economically and socially important services to people, such as fisheries, flood-recession agriculture, regulation of water quality and quantity, and attenuation of floods (Costanza et al., 1997; Opperman et al., 2017b). Although water infrastructure, such as dams and levees, provides important benefits such as hydroelectric energy, flood management, and water supply, this infrastructure can also degrade freshwater ecosystems by disrupting connectivity and altering the movement of water, sediment and organisms through river basins. Due to this fragmentation, river-floodplain disconnection, and flow alteration, water infrastructure is one of the primary contributors to the dramatic global declines in freshwater biodiversity and the loss of ecosystem services such as fisheries (Tockner and Stanford, 2002; UNEP, 2010; McDonald et al., 2012).
Due to these declines, growing attention has focused on improving the environmental performance of water infrastructure, such as implementing environmental flows that maintain or restore the flow regime of a river or other aquatic ecosystem (Poff et al., 2017). The flow regime has a strong influence on freshwater ecosystem processes and consists of the pattern of water discharge or level over time including events such as low flows, small floods, and large floods (Poff et al., 1997; Postel and Richter, 2003). Environmental flows can be implemented by managing water withdrawals or diversions, changing operations of a dam, or by other changes to the design or siting of infrastructure, including policies or decisions that maintain free-flowing conditions on a river.
Despite growing attention to the importance of environmental flows, and continued advancement in flow assessment methods, implementation of flow protection or restoration has lagged expectations (Horne et al., 2017). This paper reviews the potential for system-scale approaches to increase the rate and geographic scope of implementation of environmental flows. We consider two system-scale pathways. First, policy and management mechanisms that apply to large areas—river basins or political jurisdictions—can catalyze widespread implementation of flow protection or restoration (Duane and Opperman, 2010; Poff et al., 2010). Examples of these mechanisms include regional flow protection policies and system-scale hydropower planning and management. Although system-scale policy and management mechanisms can promote large-scale implementation of flow management, there will continue to be a need for environmental flow implementation that occurs at smaller scales, such as a high priority river reach. The second pathway focuses on implementation at that scale—such as environmental flow releases from a dam or small set of dams—but embeds flow implementation within reoperation of the larger systems of resource management within which the dam operates and/or the ecosystem is located (Richter and Thomas, 2007). These systems of resource management can encompass various sectors including water supply, flood, and floodplain management and irrigation.
We illustrate both of these system-scale pathways through 10 case studies, drawn primarily from North America, each of which includes an example of current implementation.
System-Scale Policy and Management
Implementation of Regional Flow Protection Standards
Poff et al. (2010) noted that only a small fraction of river kilometers worldwide has environmental flow protections in place. Given the rapid development of water infrastructure and growing extraction of water, they asserted that regional approaches to setting environmental flow standards (e.g., for scales ranging from large river basins to countries) would accelerate the designation of these flow standards at a pace and geographic scope commensurate with the management need. The Ecological Limits of Hydrologic Alteration framework (ELOHA; Poff et al., 2010) is a flexible framework for determining scientifically based environmental flow recommendations at a regional scale. ELOHA draws on existing hydrologic and biological information and rests on the premise that, although each river is distinct, many exhibit similar environmental responses to flow alteration. Therefore, scientists can determine flow-ecology relationships for groups of ecologically similar rivers, rather than for one river at a time.
Translating these regional flow recommendations into implementation requires corresponding policies to establish flow standards for specific river reaches based on their ecological condition goals, similar to how many water quality programs regulate contaminant concentrations according to water quality attainment goals. Below we provide two case studies of system-scale flow protection policies (one at a sub-national level and one at a national level) that have implemented flow protection based on regionally determined flow standards.
Connecticut—Regionalized Dam Operating Rules
Regionalizing environmental flow management has the potential to minimize environmental impacts of new developments, direct development to less sensitive areas, and prioritize flow restoration efforts (Poff et al., 2010). Several states within the United States are accomplishing these objectives through new streamflow criteria or standards, expressed as limits on hydrologic alteration (Kendy et al., 2012). Streamflow standards, which apply to water bodies, are implemented as operating rules that apply to dams or as withdrawal permits that apply to water users (Kendy et al., 2012).
Generally, water withdrawal limits protect existing streamflow from future development. In contrast, restoring depleted flows may require additional policies, such as Oregon's Conserved Water Statute, which explicitly re-allocates water saved through conservation measures to environmental flow (Aylward, 2008; Kendy et al., 2018).
Streamflow standards that regulate dam operating rules can both protect and restore streamflow. A 2005 statute required the Connecticut Department of Energy and Environmental Protection (DEEP) to develop environmental flow regulations for all 4,386 kilometers of rivers and streams in the state while also providing for secure water use. The process was informed by a policy advisory committee, a Commissioner's advisory committee and a technical advisory committee, along with formal public comments. The process was accompanied by high-visibility advocacy campaigns and culminated in 2011 with the issuance of final regulations (Smith, 2012).
The regulations contain three primary components: (1) narrative streamflow standards that apply to all rivers and streams; (2) a goal classification process, which associates every stream segment in the state with one of four environmental flow standards that it must meet; and (3) detailed reservoir operating rules, called release requirements, associated with each goal class (Connecticut Department of Energy Environmental Protection, 2017). The regulated reservoirs primarily store domestic water supplies.
Narrative streamflow standards describe four stream condition classes ranging from Class 1, which is free-flowing, to Class 4, which is substantially altered to meet human water needs. Dams on Class 4 river reaches are required to achieve Class 3 conditions to the best of their ability. A stream condition goal classification is assigned to each stream and river reach by DEEP through a detailed public process, accounting for 18 specific factors, such as the presence of sensitive species, existing water withdrawals, and existing and planned development upstream of the reach. To date, two of the state's five river basins have formally adopted stream condition goal classifications.
Reservoir release requirements mimic natural conditions. The advisory committees adapted the ELOHA framework to develop the requirements, using flow-response curves for fluvial-dependent species in the northeastern United States (Vokoun and Kanno, 2009, 2010; Armstrong et al., 2010, 2011). Water suppliers used a Safe Yield Wizard (Vogel et al., 2007) to calculate impacts of proposed release requirements on the amount of water available to supply customers. Dams on Class 1 streams may not actively manipulate reservoir storage. Dams on class 2 streams must release at least 75% of their reservoir inflows at all times, thereby maintaining some degree of natural flow variability. Release requirements for dams on class 3 streams, which include almost all dams of any significant size, depend on the time of year (bioperiod, or biologically based season lasting from 1 to 4 months) and whether inflows are low or high. Inflow levels are defined as a function of average inflows over the preceding 2 weeks (Smith, 2012).
Release requirements are expressed as bioperiod exceedance probabilities, rather than as volumetric discharge, so they apply consistently to the entire regulated community, while acknowledging that different types of streams vary in their flow characteristics. For example, the relative volume of a Q95 flow (discharge that is exceeded 95% of the time) of a high-baseflow river differs substantially from that of a flashy river (Smith, 2012).
Exceptions to the regulations ensure water reliability for communities and flexibility during drought. For example, the regulations allow for time-limited release reductions to ensure that public water suppliers maintain an adequate margin of safety. Release requirements also can be reduced during drought, in concert with implementation of water-use restrictions. Critical to the regulations' acceptance was a provision that dam operators have 10 years from the time from which a stream is classified until they must fully comply with the regulations (Smith, 2012). This gives water providers sufficient time to make structural modifications to dams and/or to find additional supplies if necessary.
In practice, about 23 major dams and reservoirs are changing their operations because of the regulations (David Sutherland, The Nature Conservancy (TNC), personal communication). Just as importantly, the regulations ensure that thousands of river kilometers will always have environmental flows.
Despite progress in the science and practice of environmental flows, the vast majority of global rivers remain unprotected from flow alteration (Poff et al., 2010). Meanwhile, pressure on rivers from water infrastructure and extraction is increasing. Through a transparent, inclusive policy process, Connecticut has implemented environmental flows for every river and stream in the state in a timeframe and at a cost comparable to environmental flow implementation for some individual river reaches.
Environmental Water Reserves in Mexico
Mexico's National Water Law was passed in 1992 and has language requiring “sustainable extraction.” However, for the first 15 years following its passage, the Water Law did not translate into formal management of river flows in Mexico. At the start of the last decade the National Water Commission (CONAGUA) sought to clarify water availability and water rights, finding that water consumption exceeded 40% of mean annual flow in eight of CONAGUA's 13 hydrological administrative regions, an area representing 75% of Mexico's Gross Domestic Product (Barrios et al., 2015).
In 2005, the Gonzalo Río Arronte Foundation (FGRA) and WWF-Mexico formed an alliance to explore new water management models for Mexico, including an assessment of how environmental flows could be determined and managed at the national scale. Pilot studies were conducted in three basins that spanned the range of hydrological conditions in Mexico: the Conchos, San Pedro-Mezquital, and Copolita-Zimatan-Huatuculco basins. From this project, FGRA and WWF proposed to CONAGUA a “norm,” or standard, for determining environmental flows (Barrios et al., 2015).
The environmental flow standard emphasized a set of scientific principles rather than requiring specific methods for determining environmental flow levels. The standard also recommended a three-level hierarchical approach to match the intensity of methods to the degree of certainty required. Further, the standard was based on a concept of maintaining balance between flow protection and water use, with the balance set along a continuum determined by the value of environmental resources and the level of demand for water (Barrios et al., 2015). The Mexican environmental flow standard was published in 2012 and ratified in 2017.
To translate environmental flow determinations into improved water management and protection of flows, FGRA and WWF joined with CONAGUA and the National Commission of Natural Protected Areas (CONANP) to launch a National Water Reserves Program (NWRP). The goals of the NWRP were to: (1) establish a national system of water reserves; (2) demonstrate that water reserves could support healthy river functions; and (3) build capacity in Mexico to implement environmental flows (Barrios et al., 2015). The concept of “water reserves”—a set volume of water dedicated to a specific use such as urban water supply—was used to establish an “environmental water reserve” (EWR), defined as an annual volume of water that must be left in the river to support ecological function and not available for allocation to any other purpose, similar to the ‘ecological reserve' concept pioneered in South African water law (King and Brown, 2006).
Under the NWRP, a team of scientists conducted a study of Mexico's 730 river basins to identify candidate basins where an EWR could be established relatively quickly and where government and stakeholders could develop experience implementing environmental flows. Further, the early establishment of an EWR in these basins could allow these basins to avoid the overallocated condition common to many regions in Mexico. Criteria included the proportion of water still available, water demands, the presence of infrastructure projects and the presence of natural protected areas and Ramsar sites (Barrios et al., 2015). Through this process, 189 candidate basins were identified in 2011 (Figure 1; Harwood et al., 2017).
A system was established to formalize EWRs. An environmental flow proposal is developed at the basin scale following the environmental flow standard. The flow proposal is then evaluated and discussed through formal consultations with water management entities and the general public, resulting in a Technical Justification Study that CONAGUA provides to the Executive Branch as a recommendation. Based on this recommendation, an Executive Decree can formally establish an EWR (Barrios et al., 2015). In September 2014, the first EWR was formally established for 11 basins that are sub-basins within the San Pedro-Mezquital Basin. Demonstrating that the EWR can influence decisions about infrastructure and flow management, the environmental review process for a hydropower dam on the San Pedro was halted because it would not have been able to be operated in a manner consistent with the EWR (Harwood et al., 2017).
The Government of Mexico is now pursuing one of the largest programs to establish environmental flow protections in the world. The Mexico National Water Plan 2013–2018 calls for the establishment of 189 water reserves that, due to hydrological connectivity, could reach up to 356 basins that represent 40% of the national territory. WWF is now presenting the Environmental Water Reserve concept as a model and is working with partners to promote water reserve policies in other countries in Latin America (Bolivia, Colombia, Ecuador, Guatemala, and Peru; Harwood et al., 2017).
System-Scale Planning and Management of Hydropower
Hydropower currently provides 16% of global electricity generation and global hydropower capacity is projected to increase by at least 50% by 2050 (International Energy Association, 2014). Although hydropower provides low-carbon energy, representing the majority of renewable electricity generation, the dams associated with hydropower can cause substantial negative impacts to the environmental and social resources of rivers (World Commission on Dams, 2000; Scudder, 2012). For example, hydropower dams can alter the flow regime of rivers and serve as barriers to migratory aquatic species.
In the context of hydropower, environmental flows can be protected or restored through a range of mechanisms, including: (1) planning for new hydropower that incorporates flow standards into decisions about siting and operation to ensure that new dams and reservoirs are consistent with the standard. In terms of siting, this can include requirements that a river, or section of river, remain free-flowing (i.e., free of dams); (2) reoperation of existing reservoirs to reduce hydrological alteration and release environmental flows; (3) strategic removal of dams to restore free-flowing conditions to a river. Below we review system-scale approaches to hydropower planning and management that can be used to implement environmental flow management across large areas (e.g., the scale of a country).
National Relicensing Policy to Implement Environmental Flows From Existing Dams
In the United States, non-federal hydropower dams (i.e., those owned by a state government or private company) are regulated by the Federal Energy Regulatory Commission (FERC), created by the Federal Power Act of 1920. FERC issues 30–50 year licenses to hydropower projects and, prior to license expiration, projects must undergo a relicensing process. The FPA was amended in 1986 with the Electric Consumers Protection Act (ECPA) which required FERC to give “equal consideration” to conservation and recreational uses of rivers alongside hydropower production (Gillilan and Brown, 1997). Following passage of ECPA, the periodic relicensing of projects provides an opportunity to reassess their impacts and benefits and to ensure that projects comply with new regulatory requirements issued since the previous license was granted, such as the Clean Water Act (CWA) and Endangered Species Act (ESA). During relicensing processes, other Federal agencies, such as the U.S. Fish and Wildlife Service, have “conditioning authority” through which they can issue conditions that FERC must incorporate into a license (Pollak, 2007), including conditions that require changes to project design (e.g., a retrofit to include a fish passage structure) or to its operation (e.g., the release of environmental flows).
In addition to this legal role for agencies, relicensing processes also provide a formal role for consultation with stakeholders including non-governmental organizations (NGOs) that represent environmental, cultural, or recreational interests (e.g., NGOs that represent anglers and boaters). The FERC licensing process has continued to evolve, and dam operators now generally pursue a new license through the Integrated Licensing Process (ILP), which is structured to promote consistent engagement of agencies and stakeholders throughout the process. Under the ILP, most relicensing processes now culminate in a settlement agreement—a legally binding instrument that is negotiated and signed by a licensee and parties that generally include federal, tribal and/or state agencies and NGOs. A settlement agreement describes the terms for the license, including components such as required dam operations and environmental flows (Hydropower Reform Coalition, 2005).
Environmental flows are a common mitigation requirement of renewed FERC licenses. Schramm et al. (2016) reviewed 309 licenses issued between 1998 and 2013 and found that the most common category of mitigation focused on how projects manage hydrology, with 82% of the licenses requiring environmental flows (most of the exceptions were projects for which an environmental flow would not be appropriate, such as projects within an irrigation canal or projects added to a federally owned dam where the flow regime is set by the federal agency, not by the licensee). Nearly 40% of the licenses stipulated that the project would be operated as “run of river” (outflow equals inflow), though note that some definitions of run of river allow for within-day storage and peaking operations. Among the projects not categorized as run of river, most of the environmental flow requirements were for minimum flows with relatively few requirements to manage ramping rates (~11% of licenses) or for flood or “flushing” flows (~6% of projects).
Through the FERC relicensing process, nearly all non-federal hydropower projects in the United States will have some degree of environmental flow management, and that management will continue to be revisited every few decades. Although studies do not yet exist to provide general results or trends on the environmental performance of flow requirements under FERC licenses, a number of individual results show the potential for improved environmental and social outcomes. For example, on the Roanoke River (Virginia and North Carolina, USA), a settlement agreement for a FERC license was signed in 2017 that formalized a new flow regime, based on the results of an adaptive management research program (Pearsall et al., 2005), to improve ecosystem conditions in the river and floodplain. In the Skagit River (Washington, USA), FERC conditions for license renewal required adjustments to hydropower dam operations to improve spawning habitat for salmon, resulting in a significant increase in successful spawning (Connor and Pflug, 2004). Nationally, a number of projects that previously were operated for peaking were moved to run of river operations following relicensing; Jager and Bevelhimer (2007) review 28 cases of this change.
Finally, evidence suggests that these are not isolated examples. The Hydropower Reform Coalition (HRC) is an umbrella organization for NGOs that engage in relicensing processes across the U.S. According to the HRC's website, they have signed on to 200 settlement agreements, representing 20,000 MW of hydropower capacity. Through these settlement agreements, HRC-member NGOs have pursued improvements to flow regimes, fish passage, and other environmental improvements. As described below, the FERC relicensing process has been used to catalyze dam removal and some projects show the potential of relicensing being used to trigger system-scale management of a river basin.
Dam Removal to Restore Free-Flowing Rivers
The United States Federal Energy Regulatory Commission has asserted the right to require a dam to be removed if it is in the public interest (Bowman, 2002), with the prominent example of Edwards Dam on the Kennebec River (Maine, USA). In other cases, dam owners have decided that the mitigation conditions required for license renewal, particularly for fish passage, were too expensive and so they pursued options for dam removal; examples include the Sandy River (Oregon, USA) and the Klamath River (California, USA). On the Penobscot River (Maine, USA) the FERC relicensing process was used to implement a basin-scale solution to balancing hydropower generation with dam removal to restore free-flowing river conditions. This section explores how the FERC relicensing process can be applied at a system scale to catalyze dam removal and restoration of free-flowing rivers.
The Penobscot River basin (22.3 million hectares) is the largest in Maine and second largest in the Northeastern United States. Migratory fish—including Atlantic salmon and American shad—provided the primary source of protein for the Penobscot Indian Nation and, following European settlement, supported a large commercial fishing industry. Beginning in the 1820s, dams began to be developed on the Penobscot with observers recording immediate negative impacts on migratory fish: “a great many shad and alewives lingered about the dam and died there, until the air was loaded with the stench” (Foster and Atkins, 1869). In 2000, the National Marine Fisheries Service listed the Penobscot River run of Atlantic salmon as endangered under the U.S. Endangered Species Act.
Individual dams on the Penobscot underwent numerous relicensing processes in the twentieth century but these were contentious and failed to resolve conflicts between migratory fish and hydropower generation. Early in the twenty-first century, PPL Corporation acquired the major dams on the Penobscot mainstem and, by replacing multiple dam owners with a single owner, creating the enabling conditions for a broader solution. Diverse parties negotiated the major conservation and energy issues; these included PPL Corporation, the Penobscot Indian Nation, the state of Maine, the Department of the Interior (Bureau of Indian Affairs, US Fish and Wildlife Service, National Park Service), and five non-profit conservation organizations (American Rivers, Atlantic Salmon Federation, Maine Audubon, Natural Resources Council of Maine, and Trout Unlimited). In October 2003, the parties reached a conceptual agreement to balance hydropower generation and restoration of migratory fish habitat, and a year later, filed the Lower Penobscot River Comprehensive Settlement Accord with FERC. The major components of the accord included dam removal and fish passage projects to restore free-flowing rivers and fish habitat, changes to the design, and/or operation of remaining dams to maintain hydropower generation, and new licenses for PPL's dams. Under the Accord, PPL granted to the newly formed Penobscot River Restoration Trust (“the Trust”) a 5-year option to purchase three dams for ~US$25 million and the Trust subsequently exercised that option. The Trust is composed of the Penobscot Indian Nation and the five conservation NGOs involved in the negotiation, with TNC joining the Trust in 2006.
By 2013 two mainstem dams had been removed (Figure 2). The power plant at a third dam was decommissed and, in 2016, a “nature-like” fish bypass, which physically mimics a stream, was completed to allow even weak-swimming fish to move both upstream and downstream past the dam (the dam remained because the local community preferred current river levels above the dam). PPL also committed to improving fish passage at other remaining dams in the basin. Following completion of dam removal and fish passage improvements, the accessible network of large river and stream channels will increase by an order of magnitude: from 60 to 615 km (Figure 2; Opperman et al., 2017a). Some fish populations have shown an immediate response to the increased habitat with river herring numbers increasing to 600,000 in 2015 and 1.8 million in 2016, 135 times greater than before the dam removals (Miller, 2015). Species such as salmon will require greater time to respond to the new habitat but, based on the increase in connected habitat, biologists estimate that Atlantic salmon will increase from a few thousand to 12,000 (Opperman et al., 2011).
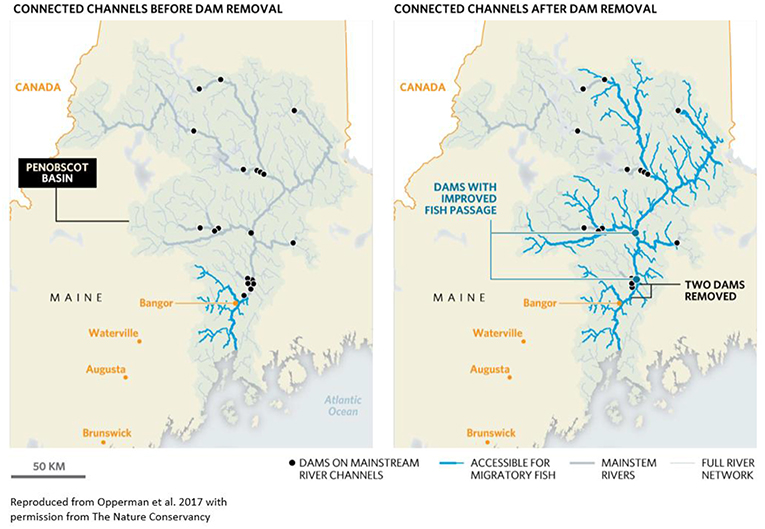
Figure 2. The Penobscot River (Maine USA) and the length of channel accessible to migratory fish before and after dam removal. Reproduced from Opperman et al. (2017a) with permission from The Nature Conservancy.
In addition to these dramatic increases in fish and fish habitat, the agreement will at least maintain the previous level of power generation from the Penobscot and PPL's projections indicate that total generation from the basin may increase after the project (Opperman et al., 2017a). The generation lost due to dam removal will be offset through several changes to design and operation of remaining dams that were approved under the new licenses.
In the section “National Relicensing Policy to Implement Environmental Flows From Existing Dams”, we characterized FERC relicensing, a national policy, as a system-scale mechanism to promote widespread implementation of environmental flows. FERC relicensing was the national policy context for the Penobscot restoration. However, by addressing most of the major infrastructure within a river basins, the Penobscot project showed the potential for FERC relicensing to also function as a mechanism to promote reoperation of a system, such as a river basin, similar to mechanisms described in section “Implementing Environmental Flows by Reoperating A System.” To date, the Penobscot is one of the few examples of where FERC relicensing has been applied to multiple projects in a coordinated manner to achieve results at a basin scale. Opperman et al. (2011) and Opperman et al. (2017a) offer a range of recommendations for how FERC relicensing can be adapted to promote basin-scale solutions. Owen and Apse (2014) describe how policies for environmental trading could be applied to allow system-scale solutions that balance power generation with dam removal and restoration of free-flowing rivers.
The potential gains, in terms of protecting or restoring environmental flows, from system-scale mechanisms to reoperate basins (e.g., through FERC relicensing or environmental trading) are quite large. For example, Kuby et al. (2005) investigated the potential to expand salmon habitat in the Willamette River basin (Oregon, USA) through dam removal. They modeled various removal options from among 150 dams in the basin and quantified tradeoffs between power generation, water storage and the length of connected channel network accessible to salmon. They reported that removal of only 12 dams could reconnect 52 percent of channel length in the basin with a loss of <2 percent of the basin's hydropower and water-storage capacity. This situation—in which a relatively small proportion of hydropower capacity causes a high proportion of the fragmentation of a basin's channel network—is likely common in many basins around the world, particularly those with a legacy of smaller and older dams. In the Duero basin (Spain), small hydropower dams are responsible for most of the disconnection while contributing very little to the total generation from the basin (Mayor et al., 2017). The data from these basins suggest that considerably large restoration gains could be achieved through strategic dam removal with relatively small losses in generation and/or water storage and thus that policy mechanisms that promote dam removal through system-scale relicensing, mitigation, or environmental trading could produce large benefits in terms of restoring free-flowing conditions in rivers.
Planning for New Hydropower to Incorporate Environmental Flow Protections
Economic growth is driving an increase in dam construction in the later-developing world including a rapid expansion of hydropower (Zarfl et al., 2015). Although development of water-management infrastructure can help countries meet important objectives for water and energy, a proliferation of new dams could lead to significant negative impacts to river ecosystems and people that depend on them, particularly because the current expansion of dams is occurring primarily in those river basins with the greatest diversity of aquatic species and highest productivity of fish harvests (Opperman et al., 2015, 2017a) which provide livelihoods and food security to millions of rural people (Richter et al., 2010). Opperman et al. (2015) found that the projected hydropower development by 2050 could fragment or alter flows, or both, on 300,000 km of river channel worldwide.
Policies that promote system-scale planning of hydropower could reduce impacts on rivers while achieving targets for renewable energy. Opperman et al. (2015, 2017a) describe a framework for system-scale planning, Hydropower by Design (HbD), which is defined as “a comprehensive and system-scale approach to hydropower planning and management that fully integrates other economic priorities and environmental and social issues from the earliest stages to promote sustainability and optimize delivery of benefits.” HbD influences environmental flow management by guiding decisions on siting (where future dams are developed and where free-flowing conditions are maintained) and design and operation. Opperman et al. (2015) conducted global modeling of projected hydropower development to 2040 and found that widespread application of HbD could reduce impacts on connectivity and flow on approximately 100,000 km of river channel globally. Opperman et al. (2017a) provide more detail on the technical, policy, and planning mechanisms that can be used within HbD and a set of case studies that show how application of HbD can result in greater length of free-flowing rivers or implementation of environmental flows during dam development within a basin. Several of the case studies demonstrate that these balanced outcomes for energy and environmental benefits can also produce economic benefits for countries, through better coordination of infrastructure investments, and financial benefits for developers and investors, primarily through improved risk management.
There are few applied examples of this comprehensive approach to system planning. The Mexican Environmental Water Reserves program (described in “Environmental Water Reserves in Mexico”) illustrates how a regional to national environmental flow management scheme can provide some of the outcomes associated with hydropower system planning. For example, where relevant, new infrastructure proposals, such as a hydropower dam, must demonstrate that the construction and operation of the proposed project can be consistent with a designated Environmental Water Reserve (EWR). In some cases, the EWR may influence license conditions (e.g., design or operation requirement to ensure consistency) for a project that goes forward. If a project cannot be managed in a way consistent with the EWR it may be halted, such as was the case for the proposed dam on the San Pedro, described in the section “Environmental Water Reserves in Mexico”. In this way, application of the EWR can influence infrastructure siting and operation decisions, illustrating that incorporation of flow standards into infrastructure planning and licensing could be a vehicle for widespread implementation of environmental flow management.
National policies for hydropower planning and development in Norway illustrate several of the mechanisms and potential benefits and outcomes of system-scale planning for hydropower. Hydropower provides nearly all (99%) of Norway's electricity and most of its large rivers have been dammed. By the 1970s, Norway had developed approximately half of its estimated hydropower potential and proposals to construct new hydropower projects began to generate opposition from environmental organizations, indigenous groups, and other stakeholders. Managers and political leaders recognized that a project-by-project approach to hydropower development could not effectively resolve conflicts and identify options that balanced the diverse values of the country's rivers (Huse, 1987). In response, Norway passed a set of policies encompassing river protection and hydropower site selection that collectively created a system-scale framework that guides how hydropower is developed and managed.
Through several legislative actions in the 1970s and 1980s, Norway established a national Protection Plan for Watercourses and a Master Plan for Water Resources. The Master Plan incorporated economic, social, and environmental criteria into a method for ranking individual hydropower projects with a goal of minimizing impacts on other resources for a given generation target. Based on these rankings, the Master Plan included a category for high impact projects that would not receive regulatory approval. The Protection Plan for Watercourses identified a set of rivers to be protected that, along with the Master Plan's identification of areas that could not be developed, has grown to include nearly 400 rivers or parts of rivers. The basins of these protected rivers encompass 40% of Norway's area and represent ~25% of Norway's hydropower potential (Stensby and Pedersen, 2007). Norway's national policies that govern hydropower planning have reduced conflict over hydropower and illustrate how a system-scale approach to infrastructure planning and licensing can catalyze large-scale protection of free-flowing rivers.
Implementing Environmental Flows by Reoperating a System
Although national policies can promote widespread implementation of environmental flows, flow management at the site-scale, such as a river with high conservation values or competing economic sectors, will remain necessary in many places. A wide variety of constraints can limit the ability to achieve environmental flow implementation at a site, such as reoperating a single dam to restore flow to a section of river (Konrad, 2010). The range of environmental flows that a dam can release can be limited by the economic purposes for which the dam was built; for example, if implementation would impact existing water rights or power purchase agreements. Overcoming constraints for implementation at a site may thus require moving beyond reoperation of a dam and/or site-scale flow implementation to “reoperation” of the larger management systems in which the dam or ecosystem is located (Richter and Thomas, 2007). In the case studies below, we explore how reoperation of systems of water storage, irrigation, and flood and floodplain management can facilitate the implementation of environmental flows.
Coordination of Multiple Dam Operations
Expanding beyond a single dam to a cascade or system of dams can increase the likelihood of overcoming constraints to environmental flow implementation. By considering more than one dam, water managers can take advantage of a greater range of options and synergies. This case study (summarized from Konrad, 2010) examines how coordination of dam operations on the Yakima River (Washington state, USA) facilitated environmentally beneficial reoperation without impacting water-management objectives in a way that would not have been possible with a single dam (Konrad, 2010).
The Yakima River is a tributary to the Columbia River that supports endangered salmon populations. Spring snowmelt runoff in the Yakima River basin is stored in dams operated by the U.S. Bureau of Reclamation (the Bureau), such as the Cle Elum Reservoir, for release during the irrigation season. These irrigation releases result in elevated flows through the summer and early fall. Salmon begin to spawn toward the end of the irrigation season and the elevated flows induce salmon to build redds (sites where eggs are buried in gravel for incubation) along channel margins that will become dry as soon as the flows drop at the end of the irrigation season. Preventing the de-watering of redds would require dropping flows to their stable, fall low-flow levels before salmon begin to spawn and prior to the end of the irrigation season, interfering with the primary purpose of the Yakima system of water storage and delivery.
To prevent redd de-watering while maintaining irrigation deliveries, the Bureau developed an approach that required coordinated operations of two dams. Just prior to salmon spawning in the Upper Yakima, the Cle Elum Dam drops its release of water to stable low-flow levels that can sustain spawning throughout the incubation period. As releases from the Cle Elum drop, Tieton Dam on the Tieton River, a tributary to the Yakima, greatly increases its flow release level to meet irrigation demand in the lower Yakima. This so-called “flip-flop” coordinated operation achieves the fulfillment of both environmental and water-supply goals with a solution that could not have been achieved at a single dam (Figure 3). The Bureau developed this solution in response to a Federal court decision in 1980 that supported the treaty rights of the Yakima tribe to retain access to salmon for harvest (MacDonnell, 1999).
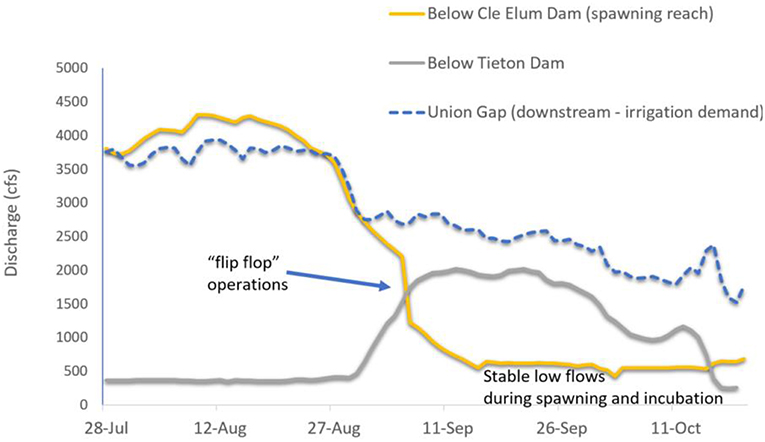
Figure 3. The “flip-flop” hydrograph produced by coordinated management of reservoirs in the Yakima River basin (Washington, USA). Chinook salmon spawn in the upper reaches of the Yakima River, below Cle Elum Dam, beginning in early September. Under previous management, flow below Cle Elum Dam would remain high in September, to support irrigation in the lower river, and then begin to decline as irrigation demand declines. However, this pattern of flows would expose and dry out salmon redds along the river margin that were laid in September. Under the new coordinated management, the Cle Elum reservoir rapidly reduces discharge (beginning late August) to reach a stable flow level that can persist throughout the spawning season (mid-September to mid-October for the year shown, 2012). At the same time, discharge from the Tieton Dam increases so that the flows in the downstream section with irrigation demand can remain higher through September, before declining in October [Flow data from USGS gage 12500450 (Union Gap) and US Bureau of Reclamation: Yakima River at Cle Elum and Tieton River below Tieton Diversion Canal].
Modeling results from other basins provide further support that moving from a single dam to a system of dams increases management flexibility and can increase the likelihood of implementing environmental flows while maintaining, or even improving, the primary designated purposes of the dam system. Lee et al. (2010) investigated optimization of hydropower and flood control within the system of dams on the Colombia River, including modeled changes to hydrology from climate change. They found that, compared to fixed status quo flood control release curves, optimized release curves for a system of dams could allow for increased generation while maintaining equivalent levels of flood-risk management. Further, under these optimized release curves, more water would be available in the system in the later summer for flows to maintain fish habitat. Modeling reoperation options for a cascade of dams on the Tana River (Kenya) identified options that could increase hydropower generation and the release of flood flows that could benefit downstream floodplain fisheries and livestock grazing (Opperman et al., 2017a; McCartney, in preparation).
Integration of Water Management Sectors: Dam Operation, Water Allocation, and Irrigation Supply
Dams are not the only cause of flow alteration, nor is dam re-operation the only solution. In over-allocated systems, environmental flow implementation means reducing water withdrawals, potentially at the expense of water-dependent economic production. Case studies are emerging, however, which demonstrate that win-win solutions can be found by integrating water management sectors. In our first example, environmental flows were secured for the Colorado River Delta (Sonora and Baja California, Mexico) through a combination of water markets and international diplomacy and delivered through a combination of dam and irrigation infrastructure re-operation. In our second example, Whychus Creek in Oregon, USA, irrigation infrastructure upgrades benefited farmers while providing environmental flows.
Colorado River Delta
Diversions from the Colorado River and its tributaries irrigate more than 50 million acres (20 million hectares) of cropland and supply water for 40 million people in the US and Mexico, most of whom reside outside the basin. As a result, the river rarely reaches its once-productive delta and estuary. What remains of the delta's riparian corridor is a dry, sandy channel flanked by desert, punctuated by patches of remnant riparian habitat maintained by irrigation drainage (Figure 4).
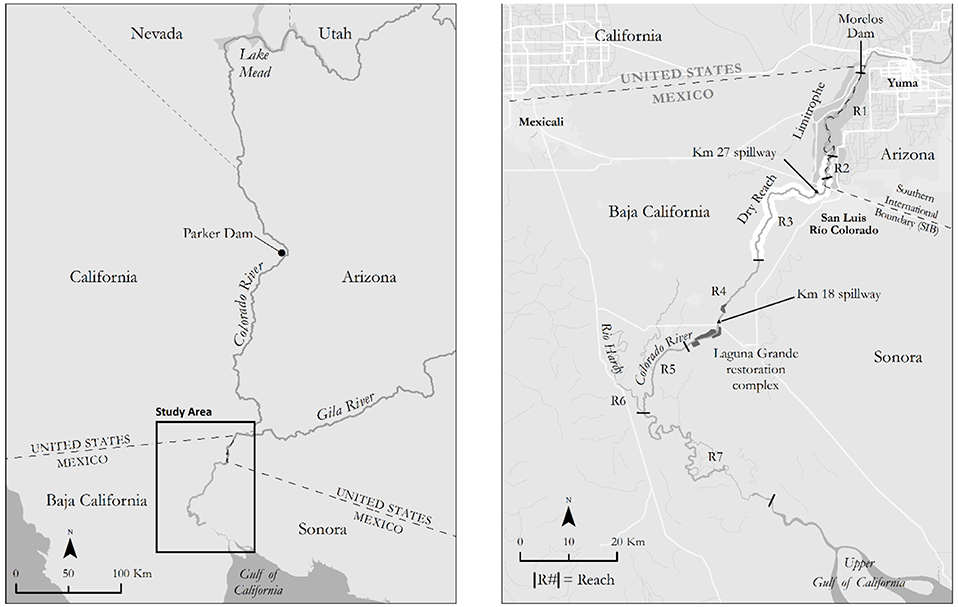
Figure 4. Riparian corridor in the Colorado River delta. Under Minute 319, environmental water was delivered to the river via Morelos Dam and Kms 18 and 27 canal spillways. Modified from Pitt and Kendy (2017) and used with permission from the authors.
In the late 1990s, a coalition of NGOs mobilized to restore riparian habitat along the corridor, which is a critical stopover for migratory birds along the Pacific Flyway (Pitt, 2001). Restoring the riparian habitat would require delivering more water to the channel and riparian corridor. But, with climate change exacerbating water shortages in the already over-allocated basin, water users and managers did not support dedicating scarce water to ecosystem restoration. A system-scale approach was required both to acquire the necessary water and to deliver it to the river and delta.
By the 2000s, water levels in Lake Mead, the largest reservoir in the United States, were declining rapidly, raising concerns among water users and decision makers across the basin. Mexico, which by treaty receives a set 1.5 million acre-feet (1.85 cubic kilometers) of Colorado River annually, was critical to a solution, but historical distrust between the US and Mexico precluded productive negotiations. The NGOs conducted shuttle diplomacy in exchange for a seat at the negotiating table (King et al., 2014). Ultimately, in 2012, the two federal governments signed Minute 319, a binational agreement that established a set of measures to share both shortages and surpluses. Among these measures, the Minute allowed Mexico to store water in Lake Mead and allocated environmental water to restore riparian habitat in the delta (International Boundary and Waters Commission (IBWC), 2012). By addressing system-scale water shortages, delta restoration became part of a basinwide solution instead of an isolated problem.
Because the Colorado River is over-allocated, simply re-operating a dam would not procure new water for the environment. The environmental water had to be acquired from existing users. Minute 319 identified two sources. First, the NGO coalition established a water trust, which purchases water rights from willing sellers in the irrigated Mexicali Valley surrounding the riparian corridor. This water is delivered to the riparian corridor as “base flows” to sustain native habitat. Second, the US and Mexican governments invested in irrigation efficiency projects in the Mexicali Valley, enabling Mexico to reduce its water demands. The water conserved through these projects is stored in Lake Mead; in 2014, some of this water was released as an environmental pulse flow into the delta. Thus, the integration of two water management approaches—water markets and irrigation efficiency improvements—freed up the water for environmental flows.
Finally, delivering the environmental water required a system-scale approach. Minute 319 negotiators envisioned the pulse flow being released from Morelos Dam, on the US-Mexico border. However, the binational science team, which designed and monitored the pulse flow, predicted that releases from Morelos would be too small to traverse a 40 km (25-mile) dry river reach without seeping into the depleted aquifer below. In order for the water to reach targeted restoration sites and to recede slowly enough to maintain growth of riparian seedlings, a portion of it was delivered through irrigation canals that bypassed the dry reach (Figure 4; Pitt and Kendy, 2017). In the end, this novel combination of water deliveries via irrigation infrastructure and dam releases was crucial to the pulse flow's success in restoring riparian habitat (Kendy et al., 2017). Improving environmental performance meant re-operating a system; not just a dam.
The benefits of restoring flows to the Colorado River delta ranged from local to binational. Locally, residents in riverside towns enjoyed seeing water in their river, many for the first time in their lives. Surveys indicated resounding support for the pulse flow across genders, age groups, educational levels, and socioeconomic conditions (Kendy et al., 2017). Mexicali farmers, who previously viewed any water in the river as wasted, saw that the river and the farms could share the water. In the US, Mexico's stored water in Lake Mead forestalled a water crisis, giving the lower basin states more time to develop comprehensive drought contingency plans.
Whychus Creek
From its glacial headwaters in Cascade Range of Oregon (USA), Whychus Creek flows 35 miles through forested mountains and sagebrush steppe before joining the Deschutes River, a tributary to the Columbia River. Prior to development, natural flows in Whychus Creek supported a diverse assemblage of riverine species, including steelhead trout (Oncorhynchus mykiss), now listed as a threatened species under the U.S. Endangered Species Act. However, by 1913, irrigators were granted water rights to divert more than 200 cubic feet per second (cfs) (5.7 m3/s), exceeding the natural streamflow in dry and normal years. As a result, a 5-mile (8 km) reach of Whychus Creek often ran dry (Aylward and O'Connor, 2017).
Beginning in the 1990s, conservationists engaged local water users in a water transaction program to restore environmental flows. As the largest water right holder on the creek, Three Sisters Irrigation District (TSID) has been an active participant in the program. From 2000 through 2015 the program secured approximately 30 cfs (0.85 m3/s) of water rights by shifting agricultural diversions to environmental flows in Whychus Creek, at a total cost of USD $17 million. Two-thirds of the water was acquired through collaborations with the TSID to eliminate water conveyance losses by installing pipes in place of ditches and canals and upgrading other irrigation infrastructure, and legally transferring the saved water to instream water rights.
As a result, the attainment of environmental flow goals has increased markedly (Figure 5), improving aquatic health in Whychus Creek (Mazzacano, 2015), while simultaneously benefitting irrigators. The conserved water projects upgraded the irrigation district's infrastructure, reducing transmission losses, thereby increasing the reliability of its water deliveries and allowing farmers to maintain crop production while diverting less water. Furthermore, the irrigation district has retained a portion of the water saved by water use efficiency projects, enhancing the security of the remaining irrigation rights.
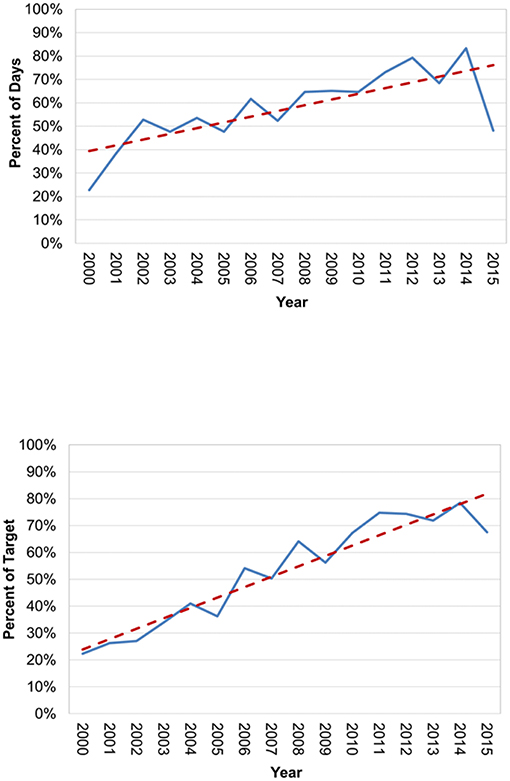
Figure 5. Attainment of the environmental flow target in Whychus Creek, Oregon, expressed as a percent of days per year (above) and as a percent of the target volume (below). Stakeholders established the flow target of 33 cfs (0.93 m3/s) based on ecological criteria. Dashed lines are linear regressions. Modified from Kendy et al. (2018) and used with permission of the authors.
Several conditions unique to Whychus Creek enabled this win-win solution. First, the physical configuration of the hydrologic system allowed flows to be restored without reducing agricultural water consumption. Historically, irrigation water conveyance losses returned to the creek downstream from the dewatered reach of concern to a consistently perennial reach. Therefore, the irrigation conveyance improvements financed by the transaction program enabled irrigators to reduce withdrawals without simultaneously reducing return flows to the dewatered reach. Reducing withdrawals from Whychus Creek left more water in the dewatered reach when it was most needed, without reducing crop production.
Second, Oregon law supports water transaction strategies for restoring instream flow. Unlike most western United States, Oregon allows water users to reduce return flows, even if downstream water users rely upon them. Additionally, Oregon's Conserved Water Statute stipulates that a portion of the water saved through irrigation efficiency improvements must go the environment. Finally, Oregon law allows the rights to saved water to be transferred to high-priority instream flow rights, thereby preventing other users from diverting it for their own use.
Restoring 30 cfs to Whychus Creek came at a relatively high cost ($17 million USD); as in much of the western US there are few opportunities for restoration using low-cost water. By working together, conservationists and irrigators achieved both of their objectives—environmental flow restoration for the environment, and irrigation-supply reliability and economic sustainability for farmers. Although neither sector alone could afford to pay $17 million, the multi-sector, multi-objective nature of the transactions attracted the funding from both private and government sources.
Integrating Environmental Flows With Flood and Floodplain Management
While most cases in this paper focus on various aspects of water management, system reoperation can also encompass land management, incorporating interventions such as increasing irrigation efficiency or groundwater recharge. In this section, we examine how management of floodplains and multipurpose reservoirs can be integrated to enable environmental flow management.
Floodplains are among the most diverse and productive ecosystems on the planet (Opperman et al., 2017b) and floodplain productivity and diversity is influenced strongly by river flows (Opperman et al., 2010). The Brisbane Declaration emphasized that environmental flows can be used to restore floodplains and that implementation of environmental flows may require coordination with floodplain management (Brisbane Declaration, 2007; Arthington et al., 2018).
Opperman et al. (2017a) describe an approach to enable environmental flows, and other benefits, through the reoperation of flood management, including floodplains. Key components of the approach include: (1) reduce reservoir storage allocated to flood management; (2) compensate for the reduction of flood-management storage through investments on the floodplain that will maintain or improve flood safety, relative to the status quo; and (3) apply the increased reservoir storage, made available through reduction of flood storage, to produce additional economic and environmental benefits. These economic benefits can include water supply, hydropower generation, or recreation. The environmental benefits can include increasing the frequency of meeting environmental flow objectives.
In 1998, TNC and the U.S. Army Corps of Engineers began collaborating on an environmental flow program for the Green River (Kentucky, USA), a tributary to the Ohio River notable for its high richness of freshwater species, including 151 fish species (12 endemic), and 71 mussel species. Through collaboration of scientists and dam operators, the Corps began implementing a new flow regime that improved habitat conditions, particularly to promote spawning of fish and mussels. To implement environmental flows, reservoir operations at Green River Dam were modified to include (1) a reduction of the flood pool; (2) delaying the drawdown that occurred each autumn, prior to season of higher flood risk; and (3) extending the period of reservoir refilling in the spring. After a program of experimental flow releases, the Corps formalized the new reservoir operation, including environmental flows, through a revision to the dam's water control plan. In addition to environmental benefits, the new operation would extend the recreation season on the reservoir by 6 weeks (Warner et al., 2014).
The integration of floodplain management with reservoir management played a role in the implementation of environmental flows. The Green River Dam's primary purpose was flood-risk management and, to implement the environmental flow regime, reservoir operations needed to change in a way that reduced flood storage. The flood pool was reduced by 1.3 m (meaning the reservoir surface is 1.3 m higher during the season focused on flood management), reducing the flood storage volume by 5%. Further, the new flow regime allowed an increase in the maximum allowed discharge by 20–30% (depending on season) (Warner et al., 2014). Through modeling of reservoir operations and downstream hydraulics, managers realized that a limited number of properties would be affected by the changed reservoir operations. To maintain equivalent levels of flood protection, the Corps, TNC, and partners carried out targeted and voluntary floodplain acquisitions and easements downstream of the reservoir, through a mix of public and private funding. Through this process, changing the management of specific floodplain parcels increased the operational flexibility of the dam and facilitated the release of environmental flows (Warner et al., 2011).
The Green River example illustrates how changed management on a floodplain can facilitate reservoir reoperation. In that case, the change in floodplain management was relatively simple, involving only a few properties. Implementation at larger scales would require considerably more complex and extensive changes in floodplain management. Opperman et al. (2017a) and The Nature Conservancy (2012) summarize research on potential large-scale implementation of this concept for the Yangtze (China), Savannah (Georgia and South Carolina, USA), and Mokelumne (California, USA) rivers.
Recommendations and Conclusions
In the developed world, nearly all large rivers have been affected by water-management infrastructure (Nilsson et al., 2005) and infrastructure is expanding rapidly in parts of the later-developing world (Zarfl et al., 2015). While infrastructure is an important foundation of modern economies and poverty reduction, it is also a primary contributor to the decline of freshwater ecosystems and the services they provide to society. Vörösmarty et al. (2010) demonstrated that water security for people increases, and freshwater biodiversity decreases, in direct proportion to the investment in water-management infrastructure. Thus, to achieve economic development that is sustainable, society must find solutions to reduce the environmental impacts of existing infrastructure and to ensure that new infrastructure is built in a way that is as compatible as possible with healthy rivers and the provision of services, such as fisheries.
Maintaining or restoring environmental flows provides a specific example of this challenge. In this paper, we propose that this challenge can best be met by pursuing solutions at the scale of systems rather than the scale of individual projects. System-scale approaches include policies and practices that integrate flow protection into planning and regulatory and management regimes. Through these policies and practices, environmental flow management can be implemented through management (e.g., the release of environmental flows from dams or restrictions on diversions) or by directing new development away from rivers with natural flow regimes (e.g., free-flowing rivers). Mexico's Environmental Water Reserves and national hydropower planning in Norway both illustrate how environmental objectives can be integrated into infrastructure planning, environmental review, and licensing to protect the natural flow regime in undammed rivers.
In addition to policies and practices that can achieve impact at large scales, there will remain a need for strategies focused on managing flow regimes at specific high priority sites. System-scale solutions can also catalyze implementation at the site scale, such as securing flow releases from an individual dam, through strategies that look for solutions at the scale of the overarching management systems within which an individual dam operates. For example, improving flood-risk management within a floodplain can increase the management flexibility of an upstream reservoir and improve its ability to release environmental flows. Management focused on irrigation efficiency or the trading of water allocations can also free up water that can then be used to achieve a flow restoration objective.
The case studies presented here share several themes. First, the system scale affords a broader set of potential solutions than could have been achieved at a single project such as a dam. Not only does this allow for more meaningful environmental restoration, these solutions also often achieve conservation outcomes without compromising the management purposes of the infrastructure.
Second, these balanced outcomes are often possible through spatial partitioning within a river basin, with some locations supporting conservation objectives and some supporting more intense management. For example, Konrad (2010) notes that the “flip flop” flow management within the Yakima system increases flow distortions on the Tieton River. However, this flow distortion in one location of the basin allows a more beneficial flow regime in the upper Yakima, which supports the most valuable spawning habitat in the basin. The Penobscot Project resulted in removal of the dams that posed the greatest challenge to fish migration, leaving these areas as unfragmented rivers that prioritize natural flow and fish habitat, while a dam on a side channel, with much lower impacts on migratory fish habitat, emphasizes hydropower and increases energy generation. The conceptual foundation of hydropower by design supports this spatial partitioning by identifying those locations that should be avoided and those locations where dam development is more appropriate.
Although this paper features a number of applied examples, implementation of environmental flows—at site or system scales—lags expectations and objectives, due to a variety of constraints. Below we describe these constraints while offering several recommendations to overcome them and to promote broader implementation.
Recommendations
Tailor Technical Requirements and Level of Data Needed for the Specific Context
One of the constraints to broader uptake of regional flow protection standards (see section “Implementation of Regional Flow Protection Standards”) is that these processes are viewed as being data intensive and overly complicated. However, as demonstrated by the case study on environmental water reserves in Mexico (Environmental Water Reserves in Mexico) and an application of ELOHA that guided flow policy for the Susquehana River Basin Commission (DePhilip and Moberg, 2010), regional flow protection standards can be based on relatively simple approaches, where appropriate. Another article in this special issue (Opperman et al., 2018) focuses on how implementation of environmental flows can be facilitated by processes that tailor the methods and level of data required to the specific context.
System-Scale Approaches Require Conveners Who Can Overcome Siloed River Management
River management is often characterized by fragmented authority of agencies or other parties that do not communicate or collaborate effectively. Processes to explore and implement new system-scale approaches will require an organization, or organizations, that can overcome this fragmentation and convene the necessary agencies and stakeholders. This convening role can be played by non-governmental organizations, as demonstrated by WWF for environmental water reserves and TNC for state flow protection standards (section “Implementation of Regional Flow Protection Standards”).
Embed System-Scale Approaches Within the Policy and Regulatory Framework for Water Resource Management
A clear mandate within the water resource regulatory framework provides managers with the tools and structures to implement system-scale approaches for environmental flow implementation, such as water allocation rules that require protection of environmental flows. As demonstrated with water reserves in Mexico, integrating these protections into allocation rules and then implementing them in places that are not yet in conflict over allocation provides a path to proactive and widespread uptake of flow protections.
Implement Periodic Relicensing of Existing Hydropower Projects to Integrate Environmental Flows Into Existing Hydropower Projects
In most countries with mature systems of hydropower, there is little opportunity to revise operations, other than through litigation or specific legislation. Periodic relicensing, such as that administered by FERC in the U.S., is an example of a policy that can compel widespread reoperation of existing facilities, including implementation of environmental flows (see section “System-Scale Planning and Management of Hydropower”). In certain cases, relicensing can trigger dam removal and the restoration of free-flowing conditions on rivers. Overall, relicensing allows for a rebalancing of river management objectives within systems that have already been developed, and, though generally focused on individual projects, relicensing has the potential to achieve system-scale objectives (Opperman et al., 2011). However, relatively few countries currently require periodic relicensing of hydropower projects. Pittock and Hartmann (2011) recommend broader uptake of relicensing to increase the sustainability of hydropower and to increase dams' resilience to climate change.
Multilateral Institutions Can Promote System Planning for New Infrastructure
The regions of the world undergoing rapid development of water-management infrastructure, including dams, encompass many countries in which the governments have relatively low capacity for planning or regulating. In these situations, development generally occurs on a project-by-project basis, with projects selected by developers based on their access to a site and financing. With limited government capacity for planning and licensing, other mechanisms are needed to promote system-scale and strategic infrastructure planning and development. For example, multilateral financial institutions can fund mechanisms such as an early planning facility or Strategic Environmental Assessment (SEA) to provide a system-scale framework which can inform the selection of individual projects, both to achieve sustainable development and to reduce investment risk (Opperman et al., 2017a).
Innovative Financial Mechanisms Can Help Achieve System-Scale Outcomes by Sharing Costs and Benefits Across Stakeholders
Implementing environmental flows by reoperating components of a larger system (section “Implementing Environmental Flows by Reoperating a System”) will generally require changes to how costs and benefits are distributed across stakeholders in a system; in other words, there may be winners and losers under the new arrangement, creating barriers to implementation. Overcoming these barriers may require innovative financial mechanisms that can compensate those who will incur costs, potentially paid in part by those who will experience benefits. For example, the environmental flow program at Wychus Creek (Whychus Creek) was facilitated by a mechanism that funded irrigation upgrades for farmers linked to requirements that the saved water would remain in the creek.
The science and practice of environmental flows has recognized the need to move beyond site-specific flow management and toward management of appropriate flow regimes across broad spatial areas, as articulated in the concept of ELOHA (Poff et al., 2010). Ultimately, the best opportunities for meeting demands for energy and water while maintaining healthy rivers and the services they provide will arise through system-scale approaches that coordinate infrastructure location, operations, and the integration of green and engineered infrastructure.
Author Contributions
JO conceptualized the paper, was lead author on the Introduction and Conclusions and wrote the case studies on relicensing, dam removal, hydropower planning, the Yakima River, and floodplain management. EK helped conceptualize and edit the paper and wrote the case studies on ELOHA, Connecticut, Colorado River Delta, and Whychus Creek. EB wrote the case study on Water Reserves in Mexico and contributed to the Conclusions.
Conflict of Interest Statement
The authors declare that the research was conducted in the absence of any commercial or financial relationships that could be construed as a potential conflict of interest.
References
Armstrong, D. S., Richards, T. A., and Brandt, S. L. (2010). Preliminary Assessment of Factors Influencing Riverine Fish Communities in Massachusetts. U.S. Geological Survey Open-File Report 2010-1139. Available online at: http://pubs.usgs.gov/of/2010/1139/
Armstrong, D. S., Richards, T. A., and Levin, S. B. (2011). Factors Influencing Riverine Fish Assemblages in Massachusetts. U.S. Geological Survey Scientific-Investigations Report 2011-5193. Available online at: http://pubs.usgs.gov/sir/2011/5193/
Arthington, A. H., Bhaduri, A., Bunn, S. E., Jackson, S., Tharme, R. E., Tickner, D., et al. (2018). The Brisbane declaration and global action agenda on environmental flows. Front. Environ. Sci. 6:45. doi: 10.3389/fenvs.2018.00045
Aylward, B. (2008). Restoring Water Conservation Savings to Oregon's Rivers: A Review of Oregon's Conserved Water Statute. Bend: Ecosystem Economics. Available online at: http://www.ampinsights.com/s/Conserved-Water.pdf
Aylward, B., and O'Connor, R. (2017). Whychus Flow Restoration: An Environmental Water Transactions Case Study. Portland: AMP Insights. Available online at: http://www.ampinsights.com/s/Whychus.pdf
Barrios, E., Salinas Rodríguez, S. A., Martínez, A., López Pérez, M., Villón Bracamonte, R. A., and Rosales Ángeles, F. (2015). National Water Reserves Program in Mexico: Experiences With Environmental Flows and the Allocation of Water for the Environment. Inter-American Development Bank.
Bowman, M. B. (2002). Legal perspectives on dam removal: this article outlines the legal issues associated with dam removal and examines how environmental restoration activities such as dam removal fit into the existing US legal system. Bioscience 52, 739–747. doi: 10.1641/0006-3568(2002)052[0739:LPODR]2.0.CO;2
Brisbane Declaration (2007). “The Brisbane declaration: environmental flows are essential for freshwater ecosystem health and human well-being,” in Declaration of the 10th International River Symposium and International Environmental Flows Conference, 3–6 September 2007 (Brisbane, QLD).
Connecticut Department of Energy Environmental Protection (2017). Connecticut Streamflow Standards and Regulations. Available online at: http://www.ct.gov/deep/cwp/view.asp?a=2719&q=434018&deepNav_GID=1654%20 (accessed August 24, 2017).
Connor, E. J., and Pflug, D. E. (2004). Changes in the distribution and density of pink, chum, and Chinook salmon spawning in the upper Skagit River in response to flow management measures. N. Am. J. Fisheries Manag. 24, 835–852. doi: 10.1577/M03-066.1
Costanza, R., d'Arge, R., de Groot, R., Farber, S., Grasso, M., Hannon, B., et al. (1997). The value of the world's ecosystem services and natural capital. Nature 387, 253–260. doi: 10.1038/387253a0
DePhilip, M., and Moberg, T. (2010). Ecosystem Flow Recommendations for the Susquehanna River Basin: Report to the Susquehanna River Basin Commission and U.S. Army Corps of Engineers. Arlington, VA: The Nature Conservancy, 96 p + appendices.
Duane, T. P., and Opperman, J. J. (2010). Comparing the conservation effectiveness of private water transactions and public policy reforms in the conserving California landscapes initiative. Water Policy 12, 913–931. doi: 10.2166/wp.2010.115
Foster, N., and Atkins, C. (1869). Second Report of the Commissioners of Fisheries of the State of Maine. Augusta, ME: Owen and Nash, Printers to the State.
Gillilan, D. M., and Brown, T. C. (1997). Instream Flow Protection: Seeking a Balance in Western Water Use. Washington, DC: Island Press.
Harwood, A., Johnson, S., Richter, B., Locke, A., Yu, X. Z., and Tickner, D. (2017). Listen to the River: Lessons From a Global Review of Environmental Flow Success Stories. Woking: WWF-UK.
Horne, A. C., O'Donnell, E. L., Acreman, M., McClain, M. E., Poff, N. L., Webb, J. A., et al. (2017). “Moving forward-the implementation challenge for environmental water management” in Water for the Environment: From Policy and Science to Implementation and Management, eds A. C. Horne, J. A. Webb, M. J. Stewardson, B. Richter, and M. Acreman (Amsterdam: Elsevier Inc), 649–673. doi: 10.1016/B978-0-12-803907-6.00027-9
Huse, S. (1987). The Norwegian river protection scheme: a remarkable achievement of environmental conservation. Ambio 16, 304–308.
Hydropower Reform Coalition (2005). Citizen Toolkit for Effective Participation in Hydropower Relicensing. Washington, DC. Available online at: http://www.hydroreform.org/hydroguide
International Boundary and Waters Commission (IBWC) (2012). Interim International Cooperative Measures in the Colorado River Basin through 2017, Minute 319. El Paso, TX; Ciudad Juarez; Chihuahua.
Jager, H. I., and Bevelhimer, M. S. (2007). How run-of-river operation affects hydropower generation and value. Environ. Manage. 40, 1004–1015. doi: 10.1007/s00267-007-9008-z
Kendy, E., Apse, C., and Blann, K. (2012). A Practical Guide to Environmental Flows for Policy and Planning, With Nine Case Studies From the United States. The Nature Conservancy. Available online at: http://www.conservationgateway.org/ConservationPractices/Freshwater/EnvironmentalFlows/MethodsandTools/ELOHA/Documents/Practical%20Guide%20Eflows%20for%20Policy-low%20res.pdf
Kendy, E., Aylward, B., Ziemer, L. S., Richter, B. D., Colby, B. G., Grantham, T. E., et al. (2018). Water transactions for streamflow restoration, water supply reliability, and rural economic vitality in the western United States. J. Am. Water Res. Assoc. 54, 487–504. doi: 10.1111/1752-1688.12619
Kendy, E., Flessa, K. W., Schlatter, K. J., de la Parra, C. A., Hinojosa-Huerta, O. M., Carrillo-Guerrero, Y. K., et al. (2017). Leveraging environmental flows to reform water management policy: lessons learned from the 2014 Colorado River delta pulse flow. Ecol. Eng. 106, 683–694. doi: 10.1016/j.ecoleng.2017.02.012
King, J., and Brown, C. (2006). Environmental flows: striking the balance between development and resource protection. Ecol. Soc. 11:26.
King, J. S., Culp, P. W., and de la Parra, C. (2014). Getting to the right side of the river: lessons for binational cooperation on the road to Minute 319. Univ. Denv. Water L. Rev. 18:77.
Konrad, C. P. (2010). “Water management for river conservation: lessons from outside of the Colorado River Basin for moving from sites to systems,” in Proceedings of the Colorado River Basin Science and Resource Management Symposium, November 18-20, 2008 (Scottsdale, AZ: U.S. Geological Survey Scientific Investigations Report 2010-5135), 77–83.
Kuby, M., Fagan, W., ReVelle, C., and Graf, W. L. (2005). A multiobjective optimization model for dam removal: an example trading off salmon passage with hydropower and water storage in the Willamette basin. Adv. Water Resour. 28, 845–855. doi: 10.1016/j.advwatres.2004.12.015
Lee, S.-Y., Fitzgerald, C. J., Hamlet, A. F., and Burges, S. J. (2010). Daily time-step refinement of optimized flood control rule curves for a global warming scenario. J. Water Res. Plann. Manag. 137.4, 309–317. doi: 10.1061/(ASCE)WR.1943-5452.0000125
MacDonnell, J. L. (1999). From Reclamation to Sustainability: Water, Agriculture, and the Environment in the American West. Boulder, CO: University Press of Colorado.
Mayor, B., Rodríguez-Muñoz, I., Villarroya, F., Montero, E., and López-Gunn, E. (2017). The role of large and small scale hydropower for energy and water security in the Spanish Duero Basin. Sustainability 9:1807. doi: 10.3390/su9101807
Mazzacano, C. A. (2015). Effectiveness Monitoring in Whychus Creek; Benthic Macroinvertebrate Communities in 2005, 2009, and 2011–2015. Portland: The Xerces Society for Invertebrate Conservation. Available online at: http://www.upperdeschuteswatershedcouncil.org/wp-content/uploads/2016/08/Effectiveness-Monitoring-in-Whychus-Creek_FINAL.pdf
McDonald, R. I., Olden, J. D., Opperman, J. J., Miller, W. M., Fargione, J., Revenga, C., et al. (2012). Energy, water and fish: biodiversity impacts of energy-sector water demand in the United States depend on efficiency and policy measures. PLoS ONE 7:e50219. doi: 10.1371/journal.pone.0050219
Miller, K. (2015). Two Years After Dams' Removal, Penobscot River Flourishes. Portland Press Herald. Available online at: http://www.pressherald.com/2015/09/27/a-river-revived-the-penobscot-river-two-years-after-dams-removal/
Nilsson, C., Reidy, C. A., Dynesius, M., and Revenga, C. (2005). Fragmentation and flow regulation of the world's large river systems. Science 308, 405–408. doi: 10.1126/science.1107887
Opperman, J., Grill, G., and Hartmann, J. (2015). The Power of Rivers: Finding Balance Between Energy and Conservation in Hydropower Development. Washington, DC: The Nature Conservancy.
Opperman, J. J., Hartmann, J., Raepple, J., Angarita, H., Beames, P., Chapin, E., et al. (2017a). The Power of Rivers: a Business Case. Washington DC: The Nature Conservancy.
Opperman, J. J., Kendy, E., Tharme, R. E., Warner, A. T., Barrios, E., and Richter, B. D. (2018). A three-level framework for assessing and implementing environmental flows. Front. Environ. Sci. 6:76. doi: 10.3389/fenvs.2018.00076
Opperman, J. J., Luster, R., McKenney, B. A., Roberts, M., and Meadows, A. W. (2010). Ecologically functional floodplains: connectivity, flow regime, and scale. J. Am. Water Res. Assoc. 46, 211–226. doi: 10.1111/j.1752-1688.2010.00426.x
Opperman, J. J., Moyle, P. B., Larsen, E. W., Florsheim, J. L., and Manfree, A. D. (2017b). Floodplains: Processes and Management for Ecosystem Services. Berkeley, CA: University of California Press.
Opperman, J. J., Royte, J., Banks, J., Rose Day, L., and Apse, C. A. (2011). The Penobscot River (Maine, USA): a basin-scale approach to balancing power generation and ecosystem restoration. Ecol. Soc. 16:7. doi: 10.5751/ES-04117-160307
Pearsall, S. H., McCrodden, B. J., and Townsend, P. A. (2005). Adaptive management of flows in the lower Roanoke River, North Carolina, USA. Environ. Manage. 35, 353–367. doi: 10.1007/s00267-003-0255-3
Pitt, J. (2001). Can we restore the Colorado River Delta? J. Arid Environ. 49, 211–220. doi: 10.1006/jare.2001.0843
Pitt, J., and Kendy, E. (2017). Shaping the 2014 Colorado River delta pulse flow: rapid environmental flow design for ecological outcomes and scientific learning. Ecol. Eng. 106, 704–714. doi: 10.1016/j.ecoleng.2016.12.002
Pittock, J., and Hartmann, J. (2011). Taking a second look: climate change, periodic relicensing and improved management of dams. Marine Freshw. Res. 62, 312–320. doi: 10.1071/MF09302
Poff, N. L., David Allan, J., Bain, M. B., Karr, J. R., Prestegaard, K. L., Richte, B. D., et al. (1997). The natural flow regime. Bioscience 47, 769–784. doi: 10.2307/1313099
Poff, N. L., Richter, B. D., Arthington, A. H., Bunn, S. E., Naiman, R. J., Kendy, E., et al. (2010). The Ecological Limits of Hydrological Alteration (ELOHA): a new framework for developing regional environmental flow standards. Freshw. Biol. 55, 147–170. doi: 10.1111/j.1365-2427.2009.02204.x
Poff, N. L., Tharme, R. E., and Arthington, A. H. (2017). “Evolution of environmental flows assessment science, principles, and methodologies. Chapter 11,” in Water for the Environment: From Policy and Science to Implementation and Management. eds A. C. Horne, J. A. Webb, M. J. Stewardson, B. Richter, and M. Acreman (Elsevier Inc.), 361–398. doi: 10.1016/B978-0-12-803907-6.00011-5
Pollak, D. (2007). S.D. Warren and the erosion of federal preeminence in hydropower regulation. Ecol. Law Q. 34, 763–800. doi: 10.15779/Z38B26Q
Postel, S., and Richter, B. (2003). Rivers for Life: Managing Water for People and Nature. Washington, DC: Island Press.
Richter, B. D., Postel, S., Revenga, C., Scudder, T., Lehner, B., Churchill, A., et al. (2010). Lost in development's shadow: the downstream human consequences of dams. Water Alt. 3, 14–42. doi: 10.4000/studifrancesi.7101
Richter, B. D., and Thomas, G. A. (2007). Restoring environmental flows by modifying dam operations. Ecol. Soc. 12:12. doi: 10.5751/ES-02014-120112
Schramm, M. P., Bevelhimer, M. S., and DeRolph, C. R. (2016). A synthesis of environmental and recreational mitigation requirements at hydropower projects in the United States. Environ. Sci. Policy 61, 87–96. doi: 10.1016/j.envsci.2016.03.019
Scudder, T. T. (2012). The Future of Large Dams: Dealing with Social, Environmental, Institutional and Political Costs. Abingdon: Taylor & Francis.
Smith, M. P. (2012). “Connecticut statewide environmental flow regulations,” in A Practical Guide to Environmental Flows for Policy and Planning, With Nine Case Studies From the United States, eds E. Kendy, C. Apse, K. Blann (The Nature Conservancy), 29–32. Available online at: http://www.conservationgateway.org/ConservationPractices/Freshwater/EnvironmentalFlows/MethodsandTools/ELOHA/Documents/Practical%20Guide%20Eflows%20for%20Policy-low%20res.pdf
Stensby, K. E., and Pedersen, T. S. (2007). Role of Hydropower in Norway. Water Framework Directive and Hydropower. Berlin June 4-5, 2007. Oslo: Norwegian Water Resources and Energy Directorate.
The Nature Conservancy (2012). Increasing Social, Economic, and Environmental Benefits through integrated Reservoir and Floodplain Management: Improving Return on Investment in Water Infrastructure. Washington, DC: TNC.
Tockner, K., and Stanford, J. A. (2002). Riverine floodplains: present state and future trends. Environ. Conserv. 29, 308–330. doi: 10.1017/S037689290200022X
Vogel, R. M., Sieber, J., Archfield, S. A., Smith, M. P., Apse, C. D., and Huber-Lee, A. (2007). Relations among storage, yield and instream flow. Water Resour. Res. 43:W05403. doi: 10.1029/2006WR005226
Vokoun, J. C., and Kanno, Y. (2009). Evaluating Effects of Water Withdrawals and Impoundments on fiSh Assemblages in Connecticut Streams. University of Connecticut, Department of Natural Resources and the Environment College of Agriculture and Natural Resources
Vokoun, J. C., and Kanno, Y. (2010). Evaluating effects of water withdrawals and impoundments on fish assemblages in southern New England streams. Fisheries Manag. Ecol. 17, 272–283. doi: 10.1111/j.1365-2400.2009.00724.x
Vörösmarty, C. J., McIntyre, P. B., Gessner, M. O., Dudgeon, D., Prusevich, A., Green, P., et al. (2010). Global threats to human water security and river biodiversity. Nature 467:555. doi: 10.1038/nature09440
Warner, A., Opperman, J. J., and Pietrowsky, R. (2011). A call to enhance the resiliency of the nation's water management. J. Water Res. Plann. Manag. 2011, 305–308. doi: 10.1061/(ASCE)WR.1943-5452.0000151
Warner, A. T., Bach, L. B., and Hickey, J. T. (2014). Restoring environmental flows through adaptive reservoir management: planning, science, and implementation through the Sustainable Rivers Project. Hydrol. Sci. J. 59, 770–785. doi: 10.1080/02626667.2013.843777
World Commission on Dams (2000). Dams and Development: A New Framework for Decision-Making. Available online at: http:www.dams.org
Keywords: river restoration, water management, environmental flows, hydropower, dam removal
Citation: Opperman JJ, Kendy E and Barrios E (2019) Securing Environmental Flows Through System Reoperation and Management: Lessons From Case Studies of Implementation. Front. Environ. Sci. 7:104. doi: 10.3389/fenvs.2019.00104
Received: 09 February 2018; Accepted: 19 June 2019;
Published: 09 August 2019.
Edited by:
Robert Alexander Speed, Badu Advisory Pty Ltd, AustraliaReviewed by:
Paul Benjamin Sayers, University of Oxford, United KingdomBrian Douglass Richter, Sustainable Waters, United States
Copyright © 2019 Opperman, Kendy and Barrios. This is an open-access article distributed under the terms of the Creative Commons Attribution License (CC BY). The use, distribution or reproduction in other forums is permitted, provided the original author(s) and the copyright owner(s) are credited and that the original publication in this journal is cited, in accordance with accepted academic practice. No use, distribution or reproduction is permitted which does not comply with these terms.
*Correspondence: Jeffrey J. Opperman, jeff.opperman@wwf.org