- International Centre for Integrated Mountain Development, Kathmandu, Nepal
The mountain cryosphere provides fresh water and other ecosystem services to half of humanity. The loss of the mountain cryosphere due to global warming is already evident in many parts of the world and has direct implications to people living in mountain areas and indirect implications to those who live downstream of glaciated river basins. Despite the growing concerns, the relationship between cryosphere change and human society has yet to be assessed systematically. A better understanding of how cryosphere change affects human systems and human security would provide much needed support to the planning of global and regional actions to mitigate impacts and facilitate adaptation. This paper synthesizes the current evidence for and potential impacts of cryosphere change on water, energy, food, and the environment in different mountain regions in the world. The analysis reveals that the changes in the cryosphere and the associated environmental change have already impacted people living in high mountain areas and are likely to introduce new challenges for water, energy, and food security and to exacerbate ecosystem and environmental degradation in the future. The effects of cryospheric changes are also likely to extend to downstream river basins where glacier melt contributes significantly to dry season river flows and supports irrigation, fisheries, and navigation, as well as water supply to many big cities. Appropriate adaptive and mitigative measures are needed to prevent risks and uncertainties from being further compounded.
Introduction
Mountains provide multiple ecosystem services vital for human well-being (Millennium Ecosystem Assessment, 2005; Messerli et al., 2007). Many of these services are related to the cryosphere—glaciers, ice sheets, snow cover, river and lake ice, permafrost, and seasonally frozen ground (Huggel et al., 2015). Mountains are often referred to as “water towers for humanity (Messerli et al., 2007) as the mountain cryosphere can store vast amounts of the Earth's freshwater (Viviroli et al., 2011; DellaSala, 2013). The cryosphere regulates the climatic conditions of the Earth system, while seasonal melting of mountain snow and ice supplies freshwater for more than half of the world's population (Qin and Ding, 2010; Xiao et al., 2015; Milner et al., 2017).
Globally, glaciers, permafrost, and other ice masses in mountain areas are thinning or declining in response to global warming and the associated changes in air temperature and precipitation (IPCC, 2014; Zongxing et al., 2016; Milner et al., 2017, see also Table S1). Deglaciation rates in the high mountains have accelerated in recent decades, with both the area and volume of glaciers declining, with only a few exceptions (Bolch et al., 2012; Rabatel et al., 2013). An estimate of global glacier change indicated a mass loss rate of 259 ± 28 Gt yr−1 between 2003 and 2009, with global runoff from glaciers exceeding 1,350 km3 yr−1 (Bliss et al., 2014). In the Andes, Bolivian glaciers have lost nearly 50% of their mass over the last 50 years (Rangecroft et al., 2013). The glacier on Mount Kilimanjaro in Tanzania has lost 85% of its area over the last 100 years, and it is unlikely that any ice will remain on it by 2060 (Cullen et al., 2013). Glaciers in the Alps have lost between 30 and 40% of their surface area and about half of their volume since 1900 (Paul et al., 2007; Beniston et al., 2018). Similarly, glaciers in the Tien Shan mountains in China have lost 6–18% of their area between 1961 and 2012 (Farinotti et al., 2015), especially in the Northern regions where loss of area ranges from 0.36 to 0.76% annually (Sorg et al., 2014).
The Hindu Kush-Himalayan (HKK) region is known as the “Third Pole” as it stores more snow and ice than anywhere else in the world outside the polar regions. But here too glaciers have been retreating very fast (Xu et al., 2009; Bolch et al., 2012; Laghari, 2013; Moors and Stoffel, 2013; Bahuguna et al., 2014). Glaciers in the Ganges, Indus, and Brahmaputra river basins are reported to be losing 24 ± 2 Gt of ice per year, equivalent to around 10% of the global loss of glacier mass between 2003 and 2009 (Gardner et al., 2013). In a recent comprehensive assessment, Bolch et al. (2019) concluded with high confidence that the trend of glacier changes—thinning, retreating, and losing mass—in the Himalayan region is expected to continue. Kranaijenbrink et al. (2017) projected that an increase of global temperature by 1.5°C would warm the high mountains of Asia by 2.1 ± 0.1°C, which would lead to the disappearance of 49 ± 7% to 64 ± 5% of glaciers by the end of the century. Even if the Paris Agreement's goal of limiting global warming to 1.5°C is realized, one-third of the Himalayan ice-volume is expected to disappear. Böhner and Lehmkuhl (2005) predicted that by 2100, glaciated regions in the Himalayas will decrease by 43–81% under the annual mean temperature increases described in the IPCC SRES scenarios.
Scientists predict that about 50% of the glaciers in high mountain Asia, 80% of glaciers in the low latitudes of the South American Andes (Table S2) and European Alps, and 30% of glaciers in Alaska may disappear by the end of the 21st century (Huss et al., 2017).
Glaciers have a profound influence on streamflow, river runoff and water quantity and quality, and changes on it will have a wide range of impacts (Lafreniere and Sharp, 2005; Stahl and Moore, 2006; Bliss et al., 2014). Receding glaciers and less snowfall are expected to have implications on hydrologic patterns of our water systems with significance for mountain and downstream populations.
Natural and human systems are closely interconnected; changes in the physical systems of the cryosphere is expected to have an impact on both natural ecosystems and dependent human systems with impacts on socio-economic conditions, livelihoods, infrastructure, and living conditions (Kaltenborn et al., 2010; Carey et al., 2017; Mark et al., 2017). It is clear that mountain populations will directly feel the impact of such changes when water sources change for irrigation and drinking, and there are increased threats of flooding or drought. However, the effects of cryospheric change extend significantly beyond the mountain regions (Milner et al., 2017). The cryosphere is a major source of water for hydropower, surface and groundwater irrigation for agriculture, livestock production, forestry, fisheries, and drinking water supplies (Hovelsrud and Smit, 2010; Huggel et al., 2015; Vincent et al., 2019).
Accelerated melting of mountain glaciers is likely to increase surface water flow in the short term but lead to a gradual decrease in the volume of water in glacier-fed rivers and streams in the longer term (Shrestha, 2005; Kaltenborn et al., 2010; IPCC, 2014; Sorg et al., 2014; Vuille et al., 2018). There are likely to be marked changes in the seasonal availability of water, with reduced storage of winter precipitation in the form of snow, and resultant reduction in water flow in the dry season (Fountain et al., 2012), without the buffering capacity of glacier melt. In combination with other factors, water shortages may affect agriculture and crop production and increase food insecurity.
The accelerated glacier melting is expected to increase the formation of glacial lakes and may exacerbate various water-induced natural hazards such as Glacial Lake Outburst Floods (GLOFs), increasing the susceptibility of infrastructure and loss of lives and property (Kaltenborn et al., 2010; Khanal et al., 2015). Thawing of permafrost may also release methane gas which can significantly contribute to atmospheric warming, while melting of snow, ice, and soil increases erodibility, leading to subsidence and infrastructure failure (Fountain et al., 2012). Changes in the cryosphere could impact tourism, energy generation, industrial production, socio-cultural structures, and human security, and hinder economic growth and exacerbate human risk (Biemans et al., 2013; IPCC, 2014; Carey et al., 2017). The impacts are expected to affect both mountain communities and those further downstream (Bury et al., 2011; Brown et al., 2015; Milner et al., 2017) and are expected to cause chain reactions in the social, cultural, economic, and environmental sectors at multiple spatial and temporal scales.
Over the last few decades, with growing concern about climate change and its impacts there has been a concerted effort to understand more about cryosphere change. While serious concern is consistently raised about the melting of ice reserves, in fact little attention has been paid to the human impacts. The possible social and economic consequences have yet to be systematically documented (IPCC, 2014; Carey et al., 2017). Better understanding of how cryosphere change affects human systems and human security is important for global and regional actions to mitigate impacts and facilitate adaptation.
Although the 4th and 5th IPCC assessment reports highlight the importance of “increasing emphasis on human beings, their role in managing resources and natural systems, and the societal impacts of climate change” (IPCC, 2014), the reports up to now provide only limited information on the relationship between cryosphere change and human society. This paper fills this gap by synthesizing the impacts of cryosphere change on human systems, particularly on water, food, energy, and the environment, both in high mountain areas and downstream.
Understanding the Linkages Between Cryosphere Change and Human Impact
The cryosphere is an integral component of the Earth's natural system which interlinks and interacts with biological and human systems (Qin and Ding, 2010; Xiao et al., 2015). Different components of the cryosphere (e.g., glaciers, snow cover, and permafrost) are interconnected and play an integrated role in the climate system and local ecosystems (Derksen et al., 2012). Thus, changes in the mountain cryosphere can have significant consequences on terrestrial ecosystems, hydrology, and human systems (Bales et al., 2006; Derksen et al., 2012; Cramer et al., 2014). Understanding the impacts of cryosphere change on human society, therefore, requires examining the complex linkages between the natural environment and humans, their processes, direct and indirect feedback, and effects at multiple scales (Warner, 2010; Derksen et al., 2012; Huggel et al., 2015). Changes in the cryosphere or in its size and/or certain characteristics of its components can affect the local and regional physical environment as well as the ecosystems and services people receive from them (Warner, 2010; Fountain et al., 2012; Berman and Schmidt, 2019). These changes can affect human society directly through changes in hydrology, streamflow, ground water recharge, air temperature, disasters, living conditions, infrastructure and transportation, and indirectly through the effects on changing ecosystems and their services (Millennium Ecosystem Assessment, 2005; Fountain et al., 2012).
The effects of cryosphere changes are non-linear, interactive and highly complex, and often cascade from one stress to another, one sector to another, and one place to another (Galaz et al., 2011; Cramer et al., 2014; Lawrence et al., 2018). For instance, the melting of glaciers and the resultant glacial lake outburst floods (GLOFs) can trigger various other events. Such events may aggravate land degradation, increase variations in the hydrological regime and degrade biodiversity, which in turn can increase socioeconomic vulnerabilities (McDowell et al., 2013; Carey et al., 2017). Manifestations of vulnerabilities in one sector or place can cause vulnerabilities in other places. For instance, changes in the hydrological regime, especially in the dry season, may affect agriculture, which may cause livelihood stress and compel poor farmers to migrate elsewhere, causing further social stress (Barnett and Adger, 2007; IPCC, 2014). The impacts are often indirect, invisible or distant, and their full extent is not immediately clear (Alcamo and Olesen, 2012; Lawrence et al., 2018). Some impacts are sudden and immediate, while others, such as the changing structure and function of ecosystems and the resultant loss of ecosystem services available to humans (Grimm et al., 2013), may emerge gradually over several years and decades (Alcamo and Olesen, 2012). Impacts can also be seasonal with strong inter-annual variability (Dirmeyer and Brubaker, 2007). However, humans too can have profound impacts on the cryosphere in various ways. Water quantity and quality, for instance, can be impacted (de Jong et al., 2009) when humans adopt unsustainable adaptation practices, such as artificial snowmaking and constructing ski runs equipped with pipelines and reservoirs to promote winter tourism (de Jong, 2011, 2015).
The effects of cryosphere change extend well beyond their origins. Initially, accelerated glacier melt may increase a glacier's contribution to river flow as a result of its negative mass balance (Lutz et al., 2016), but the effect is temporary and will decline with decreasing glacier size (Pouyaud et al., 2005). Rapid cryosphere changes are likely to impact local water availability and quality, and exacerbate disasters which in turn may affect water, livelihood, food, and energy security at a regional scale (Biemans et al., 2013; IPCC, 2014). Figure 1 summarizes the conceptual linkages between physical cryosphere change, the ecosystem, and human systems in a schematic form.
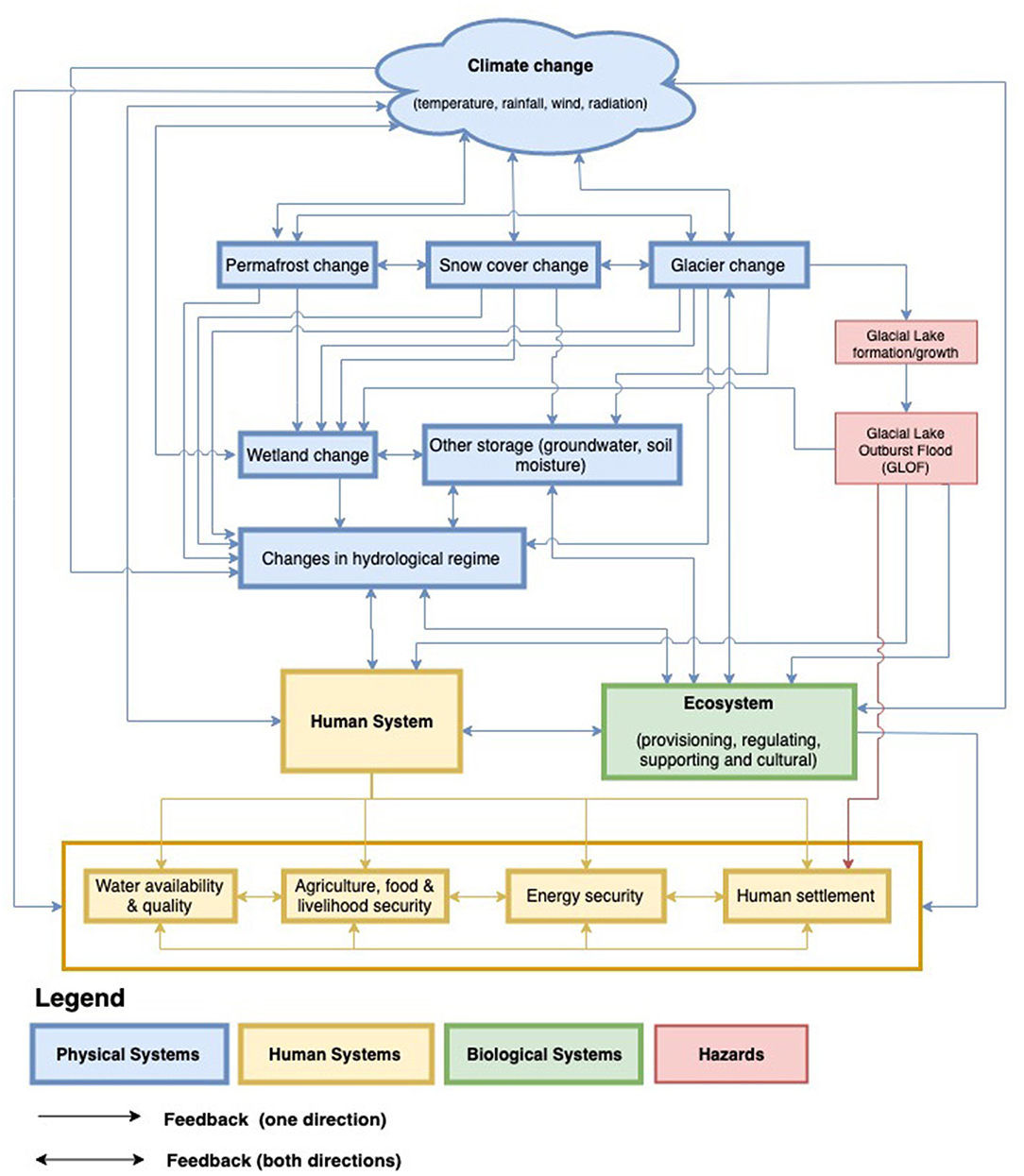
Figure 1. Conceptual linkages between cryosphere change and human systems. Source: Developed by authors.
Methods
To synthesize the information, we adopted a systematic review approach. The literature search was guided by two broad questions: (1) What are the observed impacts of cryosphere change on water, food, energy security, and the ecosystem in high mountain areas? And (2) what are the expected impacts of cryosphere change on water, energy, food security, and the environment in the downstream glaciated river basins? Our primary focus areas were the Andes, Alps, Tien Shan, and Himalaya mountain ranges, however we also used examples from other mountain regions, such as the Rocky Mountains.
We collected information from diverse sources, but our primary focus was on peer-reviewed journal articles. As many journal articles do not touch upon social and economic aspects of cryosphere change, we also included book chapters, research reports, government documents, and conference papers. The keywords used in search string terms included combinations of “cryosphere change”, “social impacts,” “economic impacts,” “glacier melting,” “snowfall,” “permafrost,” “streamflow,” “river runoff,” “water availability,” “irrigation water,” “agriculture,” “hydropower,” “tourism,” “livestock,” “fisheries,” “livelihood,” “human security,” “disasters,” “GLOF,” “ecosystem,” and “environment.” We selected documents based on the following criteria: (1) the documents recorded or referred to the observed and projected impacts of cryosphere change; (2) the documents cover any of the mountain ranges of Andes, Alps, Tien Shan, Himalaya, and other high mountain regions; (3) the documents were published in the English language. The main exclusion criteria were (1) the documents referred to impacts that were not, fully or partly, related or attributed to the changes of any components of cryosphere; (2) documents were not written in the English language.
We began the search with Scopus but expanded to Google Scholar and ResearchGate to fill in the gaps. While searching, we also looked at titles, abstracts, and keywords to avoid irrelevant documents in addition to the inclusion exclusion criteria stated above. We found 390 unduplicated documents over a period of 25 years (1994–2019) relevant to our research questions. These 390 documents were studied thoroughly, keeping the inclusion criteria, research questions and relevance in mind. Unsuitable documents were thereafter dropped. This resulted in 185 non-duplicated papers that were suitable to use in this study. We used these documents to identify social and economic implications of cryosphere change for people living in mountain areas and the downstream river basins, particularly on water, energy, food security, and the environment. The next section presents the result of the analysis.
Results: Hydrological, Socio-economic, and Ecological Impacts
Cryospheric Change Shifting Hydrological Regimes
Much of the human impacts of cryospheric change will be reflected in water systems as people are highly dependent on river flow and groundwater for cities, irrigation, and energy, much of which has its source in mountain areas. There is already mounting pressure in many areas of the world due to limited water resources, and too little or too much water, available at different times will stretch water management capabilities. Moreover, there is another pressing challenge to keep enough water in the environment to maintain a range of aquatic ecosystem services including fisheries and to provide enough outflow into oceans to keep saltwater intrusion at bay (Forslund et al., 2009).
Each river system in each mountain will have a different response to cryospheric change due to different hydrological systems. However, as ice and snow turn into a liquid state due to global warming, the contribution to streamflow from the cryosphere will increase up to a point in time when ice reserves shrink to such a size that release of water slows down, and the contribution starts to diminish (Huss and Hock, 2018; Vuille et al., 2018). This turnover point, or “peak water” will happen in different mountain regions at different times. Many glaciers have already reached their peak contribution to stream flow in the Andes (Baraer et al., 2015; Huss et al., 2017; Vuille et al., 2018) and most regions of British Columbia (Stahl and Moore, 2006). In the Hindu Kush Himalayan region, most glaciers are yet to reach their peak runoff, and for many this is expected to occur at the middle of this century (Bolch et al., 2019), snowfall pattern is changing (Viste and Sorteberg, 2015).
Typically, annual river hydrographs show a pattern of peak discharge coinciding with high rainfall or snow or glacier melt. There are also low flow periods, and societies have learned to store water, for example with reservoirs, to level out any imbalance so that water is available throughout the year. Nevertheless, water shortages could increase vulnerability through increased dependence on reservoirs during water shortages (Di Baldassarre et al., 2018). Societies have also adopted their practices to grow irrigated crops when water is available. The cryosphere has an important role to play in storing water, often releasing it during the dry season. Losing this natural water storage will require further adaption by people to cope with climate change.
Ten large socio-economically and culturally important rivers have their origin in the Hindu Kush Himalaya. The rivers flowing within South Asia are dominated by monsoon patterns, swelling during the summer monsoon rainy season but drying up during the remaining 6 to 8 months. These heavily populated regions depend heavily on this water, and people have adapted to these wet and dry cycles. With not much reservoir storage, people have turned to groundwater to meet their needs, a situation that is not sustainable over large parts of South Asia. The cryosphere releases water during the dry months, providing a precious resource when it is most needed. In addition to cryosphere change, the region will also face a change in rainfall patterns, with the annual volume of rain expected to increase in the future but with more intense rainfall events surrounded by drought periods. The buffering effect of the cryosphere reserves is expected to be lost.
Alterations of the hydrological regimes brought about by cryospheric and climate changes may affect society and the economy by reducing water availability across different sectors (Barnett et al., 2005; Berman and Schmidt, 2019) as well as by intensifying water-induced natural hazards including floods, droughts, avalanches, and landslides (Haeberli and Whiteman, 2015; Stäubli et al., 2018; Allen et al., 2019). Due to floods, avalanches and landslides, along with other climatic stresses, mountain regions have incurred significant economic losses over the last 30 years (from 1985 to 2014). It is estimated that such losses amount to up to US$ 45 billion in the Hindu-Kush Himalaya region, US$ 7 billion in the European Alps, and US$ 3 billion in the Andes (Stäubli et al., 2018). Economic loss and damages due to cryospheric-induced disasters are also reported in other mountain regions and are projected to further increase. The Zhangzangbo GLOF in Tibet, China, in 1981 killed 200 people and incurred an estimated economic loss of US$ 456 million. It caused severe damage to the Nepal–China Highway, which incurred a cost of US $3 million to rebuild (Mool et al., 2001). Similarly, the Dig Tsho GLOF in the Khumbu Himal of Nepal in 1985 damaged a hydropower plant and other properties and its estimated economic loss was US$ 500 million (Shrestha et al., 2010).
Cryospheric-induced disasters are projected to increase in the future and will require additional costs in risk reduction measures and maintenance, as well as protection of infrastructure, going as far as relocation of communities to safer places. For instance, the adaptation cost of digging a canal into the Tsho Rolpa glacier in Nepal to lower the glacial lake costed US$ 3 million in 2002 (Bajracharya, 2010). Cryosphere-induced disasters have also increased livelihood stress and physical risk and affected human settlement. Due to several GOLFs and disruption of irrigation canals, the entire villages of Passu and the communities of Northern Borith and Ghulkin in northern Pakistan were forced to migrate to higher ground (Parveen et al., 2015).
Moreover, the degradation of permafrost and consequent land and soil instabilities may bring additional challenges to the existing infrastructure, including roads, dams, and buildings. For instance, roads in the Northwest Territories of Canada have become unreliable with the operating seasons being considerably shortened owing to thawing of permafrost. This has affected food transport to the northern communities as well as domestic and international trade (Warren and Lemmen, 2014). The Diavic diamond mine, for instance, incurred an additional expense of $11.25 million to fly 15 million liters of fuel when the operational season of the road was reduced from 70 to 42 days (Warren and Lemmen, 2014).
In addition to the damages caused by natural disasters, shifting hydrological regimes may cause additional risk and challenges, affecting the society and economy in multiple ways. The following sections describe the key challenges besides observed and potential impacts of cryospheric changes.
Cryospheric Change Brings Challenges to Water Security
Accelerated glacier melting and changes in the cryosphere pose a risk to future water security, as about two billion people currently live in water-stressed areas (UNESCO World Water Assessment Programme, 2019). In high mountain areas, people depend to a great extent on snow, ice, and glacier meltwater to be used for drinking water, domestic use, agriculture, livestock, and other economic activities. In several regions, including the Hindu Kush Himalayas and tropical Andes, water scarcity is likely to increase regardless of the climate change scenario and speed and magnitude of glacier retreat, owing to population growth, urbanization and industrialization (Buytaert and De Bièvre, 2012; Buytaert et al., 2017). Cryosphere shrinkages, however, will compound the problem. Glaciers, an important source of water, have been retreating in highland Peru since 1962, in turn reducing dry season stream flow. As a result, local communities are experiencing water shortages in the dry season (Bury et al., 2011) (Table S2). In the Himalayan region as well, many areas are already experiencing water shortages (Tiwari and Joshi, 2015) which are projected to increase in the future. The Indus and Ganges basins in particular are likely to face increased risks and uncertainties in water availability (Immerzeel et al., 2010; Bolch et al., 2012). In the Tien Shan region of China, a 20% reduction of glacier volume was observed for 446 glaciers between 1964 and 2004, which is already impacting the sustainability of the region's water sources (Wang et al., 2014). Table 1 presents some of the evidence for impacts that have already been observed.
Although these individual pieces of evidence available are small and not representative, collectively they clearly indicate that cryospheric change has already impacted water availability for irrigation, agriculture, and livestock in some parts of the Himalayas and Andes as well as water quality in some parts of the Alps, Himalayas, and Western United States. These impacts are expected to intensify in the future due to projected changes of the cryosphere.
Cryospheric Change May Affect Downstream Water Availability
While mountain communities are the most affected, downstream communities will also be affected by cryosphere change as they rely to a considerable extent on mountain areas for water, and a reduction in glacial coverage after peak water is expected to lead to low flows and drought conditions in the long run (Van Tiel et al., 2018). For instance, more than 800 million people live in the catchments of the Indus, Ganges, and Brahmaputra rivers, with a vast majority living in the plains. They all rely to varying extents on the water released from glaciers for drinking, food production, and others, especially during the dry season (Immerzeel et al., 2010; Kaser et al., 2010; Lutz et al., 2014; Rasul, 2014). The Himalayan cryosphere contributes to as much as 70% of the Indus River System, which flows on the plains (Tahir et al., 2015) and supports large irrigation systems. Changes in the cryosphere in high mountain areas are likely to have serious implications for these downstream populations (Barnett et al., 2005; Milner et al., 2017), especially during the dry season when water demand for agriculture and energy is high.
Cryospheric Change Compounds Urban Water Challenges
The water supply for many large cities and towns originates, at least in part, from the cryosphere (Vergara et al., 2007; Kinouchi et al., 2019). The Andes, for example, supply 15% of the total water to the large cities of La Paz and El Alto in Bolivia, 20% to the city of Huarez, Peru, and 5% to Ecuador's capital city of Quito. The glaciers of Cordillera Real supply 30–40% of the potable water to La Paz and El Alto (Vergara et al., 2007). Seasonality is also significant, with glaciers contributing to critical low flow during less favorable periods. The three main dams that supply La Paz and El Alto no longer receive runoff from glaciers and have almost run dry. The two Tuni-Condoriri glaciers which provide part of the water for El Alto and La Paz lost 39% of their area between 1983 and 2006 (Buxton and Escobar, 2013). The Chacaltaya glacier, another source for these cities, is expected to completely disappear within 15 years (Buxton and Escobar, 2013). Recession of Andean glaciers will affect the water supplies to major cities, putting populations and food supplies at risk in Argentina, Bolivia, Chile, Colombia, Ecuador, Peru, and Venezuela (Vuille et al., 2018), clearly an area of major concern.
Even in the HKH region, many big cities in mountain and downstream areas depend partially on glacier and snowmelt water for municipality water supply. For example, the glacier-fed Melamchi River supplies 3.5 million liters of water per day to a population of 600,000 in the Melamchi Valley and is planned to supply 170 million liters per day to more than 1.5 million or more people in the capital city of Kathmandu (Khadka and Khanal, 2008). High-mountain villages are directly dependent on melt water for domestic water supply and irrigation, and some villages in the high mountain areas of northern Pakistan have relocated to other places as glacier supplies have diminished and irrigation systems have been disrupted (Parveen et al., 2015).
Lowland areas are some of the most densely populated areas in the world. Moving downstream from mountains, rainwater becomes a more important contributor to river flow. Nevertheless, large and growing cities will require as much water as possible throughout the year. In India, large cities such as Haridwar, Varanasi, Patna, Kanpur, Allahabad, Munger, Bhagalpur, Delhi, Agra, Mathura, and Kolkata are all located along the Ganges and its tributaries, with huge populations depending at least partly on glacier and snow melt that feed the rivers and recharge groundwater. The Simly Dam water reservoir for Islamabad in Pakistan is supplied by the Soan River, and water seepage from the glacier-fed Indus Basin Irrigation System is an important source of water for southern Punjab (Jehangir et al., 1998).
With growing populations becoming wealthier and urbanized, the demand for water will continue to rise, and providing adequate water to the growing population will be a challenging task in the future. The contribution from the cryosphere varies depending on the situation, and in most instances very little is known about the direct contribution. However, this change in flow pattern due to vanishing cryospheric water will add complexity to an already water-stressed situation. It can cause conflicts between different water users (Gleick, 2014) as happened in Peru in 2008 when farmers and a hydroelectricity company disagreed on the operation of the Lake Paron Reservoir (French et al., 2015; Vuille et al., 2018).
Cryospheric Change May Affect Water Quality and Health
Glaciers can regulate the influence of harmful contaminants through storage in snowpacks, which prolongs the opportunities for volatilization and other transformations (Grannas et al., 2013), but as glaciers recede this effect will be reduced. Similarly, glacier retreat and longer-term decline in streamflow could reduce the capacity of streams to dilute pollutants such as industrial effluent and non-point source contaminants such as agrichemicals (Moore et al., 2009; Brown et al., 2015). In Peru, it is estimated that an additional cost of US$ 100 million will be required to purify the deteriorating water quality resulting from cryospheric changes (Vergara et al., 2007).
Glacial meltwater could also potentially contribute to water pollution. Melting glaciers represent a secondary source of previously deposited and stored persistent organic pollutants (POPs), which are now being released back into the environment due to the accelerated melting induced by global warming (Donald et al., 1999; Blais et al., 2001; Bettinetti et al., 2008; Bizzotto et al., 2009; Bogdal et al., 2009). The release of historically deposited pollutants could significantly reduce water quality downstream (Milner et al., 2017). An unexpected increase in environmental pollution by POPs such as polychlorinated biphenyls (PCBs) and dichlorodiphenyl trichloroethane (DDT) was reported in a study of Lake Oberaar in Switzerland, which is directly fed by meltwater from the adjacent Oberaar Glacier (Bogdal et al., 2009), and an increase in levels of PCB was reported in Lake Stein in Switzerland, which is fed by the Stein Glacier. In Lake Iseo in northern Italy, the highest levels of DDT contamination were found during the period corresponding to the greatest glacier retreat (Bettinetti, 2011), and in a number of alpine lakes, POP concentration levels were related to the volume of meltwater (Morselli et al., 2014). Blais et al. (2001) found that 50–90% of POPs in glacier lakes originated from glacial meltwater. In the Himalayas, pollutant loads released by glaciers melting during the dry season can exceed the loads from other sources in the catchment areas of river basins (Sharma et al., 2015).
Many communities living in the high mountains of developing countries depend on glaciers and streams for drinking water. Even in developed countries, indigenous people use glacier melt water for drinking. For instance, a majority of the indigenous population in Iqaluit, Canada, prefer collecting freshwater from natural sources such as streams, lakes, and icebergs. With the thawing of permafrost, they are now at risk of being exposed to contaminated water and water-borne pathogens (Warren and Lemmen, 2014). Deteriorating water quality resulting from changes in the cryosphere may directly affect human health, while the reduction in water supply and agricultural production resulting from glacier recession may also have health impacts (Singh et al., 2010).
Cryospheric Change as a Risk for Food Security
The high mountain areas in developing countries still have a considerable population dependent on agriculture, forestry, fisheries, pasture, and tourism for their livelihoods and food security, and depend on snow and glacier melt water for irrigation and subsistence agriculture for maintaining soil moisture and vegetation growth and groundwater recharge (Bury et al., 2013; Paudel and Andersen, 2013; Parveen et al., 2015; Clouse et al., 2016). High mountain agriculture and agro-pastoralism are particularly vulnerable to changes in the cryosphere as they depend on spring and summer runoff from melting snow and ice for irrigation (Dame and Nusser, 2011; Parveen et al., 2015; Clouse et al., 2016).
Some regions of the Himalayas, such as Afghanistan, northern Pakistan, and north western India, are completely dependent on snow and glacial meltwater for irrigation (Parveen et al., 2015; Clouse et al., 2016; Mukherji et al., 2019). In northern India, the gradual recession of low-lying glaciers over the last three decades has reduced the supply of irrigation meltwater available to farmers and caused chronic drought in many subsistence agricultural villages (Grossman, 2015). In Ladakh, India, agricultural production is entirely based on irrigation. Channels divert meltwater from glaciers and snowfields or, where topography allows, from the main rivers to settlements (Dame and Nusser, 2011; Vincent et al., 2019). In Gilgit-Baltistan in Pakistan, glacier and snowmelt water irrigates 66% of the total irrigated land, and in Melamchi Valley in Nepal this number is 100% (Khadka and Khanal, 2008; McDowell et al., 2013). Melting glaciers, thinning snow cover and changing precipitation patterns are affecting subsistence agriculture and the agro-pastoral system in many high mountain areas of the world (Table 2).
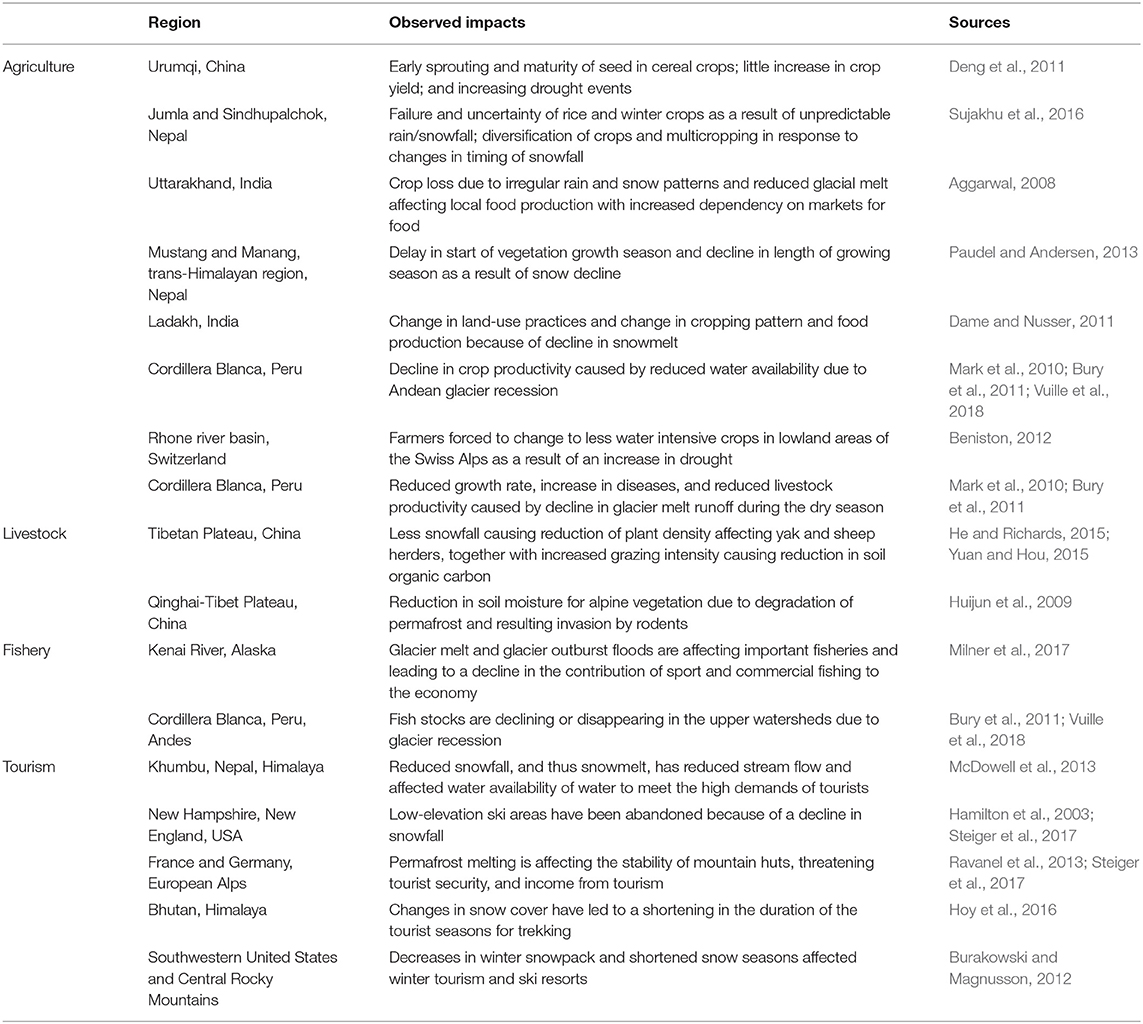
Table 2. Cryospheric change and its impacts on agriculture, livestock, fisheries, tourism, and food security.
Besides high mountain areas, many irrigation systems in the plains rely on glacier- and snow-fed rivers (Mukherji et al., 2015; Schoolmeester et al., 2018). Glacial melt water is used for irrigation mainly in the Himalayas, the Andes, the Alps, and in the Central Asias Tien Shan and Pamir mountains (Punkari et al., 2014; Mukherji et al., 2015; Buytaert et al., 2017; Vincent et al., 2019). The Indus Basin Irrigation System, for instance, is fed by water from six glacier- and snow-fed tributaries; it irrigates 80% of the total cultivated area of Pakistan (ICIMOD, 2010), which produces 85% of rice and wheat as well as all the sugar in the country, among others (Qureshi, 2011). Similarly, the Bhakra-Nangal irrigation system in Himachal Pradesh, India, supplies irrigation water to fields in Haryana, Punjab, and Rajasthan, producing a large amount of food. It has contributed significantly to making India self-sufficient in food grain and reducing hunger and food insecurity (Malik, 2008). In the Andes, surface glacial meltwater sustains farming in the Cordillera Blanca valleys and commercial agriculture in the lower watershed (Somers et al., 2016). In the Andes, glacial melt contributes to 45–100% of the irrigation water, irrigating 402,000 hectares of land in Bolivia, 79,300 hectares in Peru, 655 hectares in Chile, and 429 hectares in Argentina (Schoolmeester et al., 2018).
Over recent decades, reduced snow and loss of glacier mass has reduced water supplies in many subsistence agricultural villages across the Ecuadorian, Peruvian, and Bolivian Andes (Table 2). The canal water levels in the Yanamarey watershed of the Cordillera Blanca in Peru have been dropping over the past few years, especially during the dry season. It has become increasingly difficult to get sufficient water to maintain crop productivity (Bury et al., 2011). The frequency and intensity of low temperature frosts have also increased, which further damages crops. Households in the Cordillera Blanca in Peru reported that increasing climatic extremes and recession of the Andean glaciers have led to a decline in crop productivity, which poses a considerable risk to household food security (Mark et al., 2010; Vuille et al., 2018).
Livestock and Pastoralism
Animal husbandry is an important component of livelihoods and food security in high mountain areas, where animals are a source of food, skin, and wool and are also used for transport. Drying up of watering points due to cryosphere change could affect livestock production and food security. For example, in Wyoming in the USA, the cattle industry depends on glacial melt as a stable source of water during the growing season. However, the glacier surface area decreased on average by 32% between 1985 and 2005, and streamflow was reduced by 10% (Cheesbrough et al., 2009). Similarly, diminishing spring flows in the upper reaches of the Querococha watershed in the Cordillera Blanca in Peru, due to recession of the Andean glaciers, have negatively affected pasture health and grass productivity (Bury et al., 2011), while reduction in dry season water availability for livestock has also affected livestock productivity (Mark et al., 2010; Vuille et al., 2018). In the Nagqu Prefecture in Tibet Autonomous Region in China, the plant density in meadows has declined, in part due to cryosphere change, and this, together with increasing grazing intensity, has negatively affected yak and sheep herders (He and Richards, 2015) (Table 2). Overall, cryosphere change could have both positive and negative effects on agro pastoral livelihoods depending on the region, and more studies are needed to understand the possibilities.
Tourism
Tourism is an important source of income and livelihoods in mountain areas and supports a billion-dollar outdoor recreation service (Burakowski and Magnusson, 2012) but is being negatively affected by lower snowfall and receding glaciers (Table 2). In 2007, the Pastoruri Glacier in Huascarán National Park in Peru was closed to tourists because of its rapid recession, and tourist numbers have fallen from an estimated 100,000 in the 1990s to 34,000 in 2012 (Taj, 2013). Glacier ski resorts established in the 1970s and 1980s in Austria in the European Alps are now facing challenges related to the loss of glacier area thickness, decreased summer skiing season, and stagnation of tourist numbers (Falk, 2016). Similarly, the Chacaltaya ski resort in Bolivia, once declared the world's highest ski resort, closed recently due to glacier recession. This trend can also be witnessed in Ontario and Quebec in Canada, with a 10–15% decrease in ski tourism between 2000 and 2010 (Warren and Lemmen, 2014). In Switzerland, ski areas are projected to decrease to 63% in a +2°C scenario and to 44% in a +4°C scenario, in reference to 85% in 1961–1990. Similarly, in Japan a 30% decline in skier visits is projected in a +3° scenario (Steiger et al., 2017). Similarly, the snowmobile industry is becoming increasingly vulnerable due to a lack of natural snow and skiing is becoming increasingly vulnerable due to dependency on snowmaking (Warren and Lemmen, 2014). Winter tourism in the United States is also at risk due climate-induced cryospheric change (Burakowski and Magnusson, 2012).
Fisheries
Many communities who depend on fishing as a source of livelihood could be affected by changes in glacier melt (Lehodey et al., 2006; Brander, 2007). For example, fish stocks in the Yanamarey watershed in Peru have declined or completely disappeared due to seasonal reductions in fish habitat in the upper watershed resulting from glacier recession (Bury et al., 2011), which has affected the state economy. Increased glacier melting and glacier outburst floods in the Kenai river in Alaska have affected fisheries worth USD 70 million annually (Milner et al., 2017), while fish production in rivers from the Himalayan mountains has also become vulnerable (Allison et al., 2009), threatening income generation and dietary protein. Similarly, the warming of rivers and lakes has led to an expansion of invasive species in the Arctic and Continental Great Lakes ecoregions, which has increased commercial fishing but threatened the food security of the indigenous people, who for millennia depended on subsistence fishing (Warren and Lemmen, 2014).
Cryospheric Change as a Risk to Energy Security
Hydropower is an important source of energy, supplying about 16% of the world's electricity, and as much as 100% in Bhutan and Norway, 93% in Nepal, 59% in Canada, with up to 90% in Quebec, British Columbia, Manitoba, Newfoundland and Labrador, 33% in Pakistan (Shrestha et al., 2016) 80% in Peru, 50% in Ecuador, and a considerable amount in many Andean countries (Vergara et al., 2007). Water for these hydropower reservoirs and plants often originates from glacier and snow melt in high elevation areas, which is likely to be affected by climate change and accelerated glacier melting (Cruz et al., 2007; Boehlert et al., 2016; Turner et al., 2017). It is difficult to ascertain the exact impact of glacier recession on hydropower as the impacts can vary substantially across a region and even within countries, but different studies predict that it may eventually lead to marked impacts on the seasonality and volume of streamflow. The impact is likely to be greatest on small-scale “run of the river” hydropower plants (Kopytkovskiy et al., 2015) with little or no storage, which is a common type in the Himalayan region (Boehlert et al., 2016; Turner et al., 2017). While melting glaciers may increase water availability for hydropower at the initial stage, an increase in volume from meltwater does not necessarily lead to an increase in production of energy due to spillage effects from hydropower reservoirs (Warren and Lemmen, 2014; Tarroja et al., 2016). Reduction in dry season runoff can reduce hydropower production from these plants, as the initial increase in annual flow from glaciers will not compensate for decreased water availability during the dry season (Rees et al., 2004; Tarroja et al., 2016). For instance, severe drought in California in the United States resulting from higher temperatures and less snowfall has reduced hydroelectric generation by two-thirds from 2011 to 2015 (Gonzalez et al., 2018).
Climate change is also expected to increase the frequency and magnitude of extreme events, which is likely to increase the risk to hydropower plants from flash floods, debris flows, and similar events. Energy infrastructure such as transmission lines and powerhouses can be destroyed by GLOFs, which are also projected to increase. The Dig Tsho GLOF in Nepal in 1985 destroyed a hydropower plant worth USD 1.3 million, while a glacial fall near the Chorabari glacier, India, in June 2013 caused an outburst that damaged hydropower projects in Uttarakhand (Schwanghart et al., 2016).
The energy sector is likely to suffer due to additional risk and loss of potential opportunities due to cryospheric changes. In Peru, the cost of glacier shrinkage for the energy sector was estimated to be about US$ 740 million annually (Vergara et al., 2007), with a reduction of 11–15% in the production of electricity (Vincent et al., 2019). If glaciers from the Cordillera Blanca retreat, it would reduce meltwater runoff in the Cañón del Pato hydropower plant in Rio Santa by 50% and energy output would be reduced by about 300 GWh, and if the glaciers would disappear it would further decrease by about 600 GWh (Condom et al., 2012). Similarly, Switzerland, which generates over half of its energy from hydropower, is projected to lose about 21% of its annual inflow in the 2031–2050 period compared to 1991–2010, and further reduction in hydropower potential due to cryospheric shrinkages is expected (Gaudard et al., 2013). Additional investment will be required to repair, maintain, and adapt hydropower infrastructure due to cryosphere-induced disasters (Berman and Schmidt, 2019) and increased stress and demand for energy. For instance, Peru will be required to invest about US $1 billion per gigawatt for thermal-based power to compensate for the loss of hydroelectricity (Vergara et al., 2007).
Cryospheric Change May Affect the Ecosystem and Environment
Ecosystems accustomed to long frozen periods are vulnerable to warmer temperatures. Cryosphere recession has serious implications for mountain ecosystems because of the effect on micro-climate, hydrology, vegetation, and the carbon balance (Fountain et al., 2012; Williams et al., 2015) and can also affect the ecosystems and environment downstream (Zhao-ping et al., 2010). Ice loss affects ecosystems directly through the loss of physical habitat and through alterations in thermal conditions, and indirectly by altering light and nutrient supply to primary producers (Fountain et al., 2012). Permafrost degradation can change soil moisture content and soil nutrient availability and influence species composition (Zhao-ping et al., 2010). The potential impacts include fragmentation of animal and plant communities and development of new assemblages, disruption of seasonally synchronized phonological connections among species, and losses in biodiversity with associated changes in ecosystem function (Parmesan, 2006), all of which can lead to environmental degradation. Permafrost recession can also affect slope stability and carbon balance, the release of trace gases, and hydrology (Slaymaker and Kelly, 2007).
Vegetation Patterns and Changing Ecosystem Productivity
Growth conditions for plants and the food chain for animals are directly affected by the thickness and duration of winter snow and its effect on soil water availability, energy input, and thermal conditions for biomass production. Winter snow cover is of great importance for soil processes (Edwards et al., 2007; DeBeer et al., 2016). Climate warming could reduce snowpack, which can lead to colder soils and an increase in the frequency of freeze-thaw cycles. Shorter duration of snow cover directly influences the length of the growing season and the phenology of plant production and consumption (Gottfried et al., 2012; Warren and Lemmen, 2014). Permafrost degradation exacerbates surface erosion (Grannas et al., 2013; Warren and Lemmen, 2014). The disappearance of perennial ice and degradation of permafrost is likely to be accompanied by progressive drying of the surface and a decrease in vegetation cover. These factors will increase the effects of aeolian (wind) action and elevate the potential risk of desertification (Wang and French, 1994, 1995).
Upward Shifts of Species and Communities
Upward shifts of species and communities in response to a reduction in the cryosphere and warming climate are already evident in some mountain environments (Telwala et al., 2013). An analysis of worldwide tree line data indicated that the tree line had moved uphill in 52% of sites, and down in only 1% (Barros et al., 2017). In the Alps, upward movement of some plant taxa at a rate of 1–4 m per decade has been observed at higher elevations, together with a loss of some taxa that were formerly restricted to these elevations (Watson and Haeberli, 2004). Furthermore, concerning the riverine biodiversity, species of cold-adapted invertebrates from higher elevations are increasingly being displaced with the expansion of less cryophilic species due to a decrease in glacier runoff to streams, thus changing the hierarchical habitat template (Wilhelm et al., 2013).
Greenhouse Gases and the Carbon Pool
Large carbon pools have accumulated in the wetlands of the world's cold regions, primarily in peatlands. Much of this carbon is sequestered in permafrost (Jin et al., 1999; Schuur et al., 2008). Permafrost degradation is likely to increase the emission of major greenhouse gases from these layers, leading to further changes in the climate (Cheng and Wu, 2007). Nitrogen leaching from soils has also been reported to increase alongside a decrease in snowpack, which is potentially attributable to reduced root uptake and/or to physical (rather than microbial) degradation of soil organic matter (Freppaz et al., 2008). Recession of the cryosphere and resultant changes in vegetation could have a marked impact on the atmospheric exchange of greenhouse gases such as carbon dioxide and methane, which will impact ecosystem functioning (Callaghan et al., 2013). As mentioned above, there are considerable deposits of pollutants, especially POPs, stored in glacier ice. These are not only significant for water; melting of Arctic glaciers has released massive amounts of POPs back into the atmosphere, leading to a slight increase in the concentration of a variety of POPs in the Arctic atmosphere over the past 20 years (Ma et al., 2011), and a similar effect can be expected in other regions.
Discussion
The evidence presented in “observed impacts” and “potential future impacts” has important implications on water management, food security, energy security, and environmental management. Existing trends and future projections suggest that the cryosphere is likely to shrink substantially in the coming decades at the current global warming rate. This is expected to change hydrological regimes in glacier-dependent regions and affect river and stream flow, bringing additional risks and uncertainties to food, water, and energy security while exacerbating ecosystem and environmental degradation. Some of these effects are already being observed though their characteristics and magnitude of risks vary considerably across sectors and mountain regions (Table 3).
Although effects are difficult to quantify and existing studies are limited in scope, it is clear that cryosphere change will have a considerable impact on water availability in the future, both upstream and downstream. Evidence suggests that the impact will be more severe in arid and semi- arid mountain regions (Messerli et al., 2007), where peak water has already been reached and communities are already under water stress, and in high mountain areas in developing countries where people rely on cryosphere resources and ecosystems for their livelihoods heavily. Since glaciers are still melting with increased precipitation, the impacts are not clearly evident downstream, and the shortage of water availability is likely to emerge only gradually as glaciers increasingly vanish (Huss et al., 2017; Vuille et al., 2018). Furthermore, cryosphere change may bring additional risks to urban water supply, particularly in the Andes and the Himalayas, where the water supply of many big cities depends partly on water from glacier and snowmelt. As urbanization rapidly increases, cryosphere loss will pose management challenges for urban planners and managers in many mountain regions, particularly in the Andes and the Himalayas.
The agriculture sector is already experiencing adverse effects and is likely to be severely affected in the future. The effect of cryosphere shrinkage is likely to be felt beyond the mountain regions along the glaciated river basins. Changes in water availability due to cryospheric and climatic factors may immediately affect agricultural productivity in upstream areas and downstream areas in the long run. Glacier and snowmelt water contributes considerably to the supply of dry season irrigation water in the river basins of the Indus, Upper-Ganges, Upper-Brahmaputra, and Yangtze, which are the bread basket for billions of people in Asia (Siderius et al., 2013; Vincent et al., 2019). Similarly, the tourism sector, particularly winter sports in the Alps and North America, have already been affected negatively by snow reduction. In the future the industry is expected to become unviable, causing many to become jobless. Glacier shrinkage can also affect hydropower production in certain regions upon reaching peak water, impacting both energy security and mitigation of greenhouse gas emissions, thus posing challenges to reducing greenhouse gas emission and achieving the goals of the Paris Agreement (Gonzalez et al., 2018).
Conclusion and Recommendations
Findings of this study indicate that the growing risks for water, food, and energy security, together with increasing risk of disasters, are expected to increase economic, social, and environmental vulnerabilities and to introduce new threats to human security (Matthew et al., 2009). The risks emerging from shrinkage of the cryosphere are adding challenges for sustainable development, particularly in achieving certain sustainable development goals (SDGs) such as ensuring water availability (SDG 6), achieving food security (SDG 2), ensuring access to affordable energy for all (SDG 7), and combating climate change (SDG 13).
Cryosphere loss is therefore likely to have far-reaching impacts on societies, the economy, the environment, and ecosystems if appropriate measures are not taken urgently. The adaptation measures taken so far are limited, fragmented, and narrowly focused (McDowell et al., 2019; Rasul et al., 2019). A comprehensive risk management plan at different levels will be needed to minimize the adverse impacts. Adaptation to climatic change vulnerability should be integrated into planning and management of socioeconomic development, and appropriate adaptive and mitigative measures to prevent risks and uncertainties from being further compounded urgently need to be addressed. Cryosphere change may otherwise undermine development efforts aimed at reducing poverty and achieving food, water, and energy security by further triggering social conflicts and human insecurity.
Limitations and Further Research Needs
Quantification of the economic and social effects of cryosphere change is important in informing public policies and influencing adaptation decisions. This study, however, was not able to quantify the effects, owing to gaps in data, as most of the documents available are qualitative with limited scope and fail to develop a coherent approach. Further research is needed to quantify, assess, and predict the impacts of cryosphere change on the environment, society, and the economy and its effects on human security, outmigration, and social conflicts. Research is also needed to reduce uncertainties in projected changes in the cryosphere, their potential impacts and their long-term consequences on society, the economy, and the environment. More interdisciplinary studies are required to better understand how the effects of cryosphere change cascade to the socio-economic sectors, increasing risks and human vulnerabilities, and what sustainable adaptation measures can be adopted on the long-run (de Jong, 2015).
Recommendations
While designing public policies and strategies to deal with the increasing uncertainty emerging from cryosphere shrinkage, a collaborative and integrated approach that considers the impact of water stress on agriculture, energy, food, and the ecosystem needs to be considered. The main points to consider are as follows:
• While cryospheric change affects water availability, managing the future water challenges will require both demand and supply management. Sustainable water storage will be critical for managing seasonal water variability, as cryosphere change is expected to affect the amount and seasonality of flow in most rivers. Reservoirs, however, should be managed carefully to avoid adverse effects on the environment and human settlement (Di Baldassarre et al., 2018). This is particularly important in regions like the HKH where seasonality in water availability is high and water storage is gaining importance.
• Efforts should be made to manage demand for water by improving water-use efficiency and reducing waste through appropriate policy incentives. As agriculture is the largest user of water, efforts need to be made to reduce water demand for irrigation by improving irrigation methods, changing cropping patterns and the crop calendar, and introducing other water-efficient methods.
• To deal with the possible impacts on hydropower generation and energy security, cryosphere change needs to be taken into consideration in designing hydropower plants. Efforts should be made to develop more efficient hydropower turbines and to reduce surface evaporation from reservoirs as well as to enhance energy efficiency and reduce wasteful consumption.
• Disaster response and preparedness need to be improved through better monitoring of risks and planning for risk management and by engaging multiple stakeholders. Governments have an important role to play in raising awareness about present and future impacts and vulnerabilities, building adequate capacity to cope with impacts, and in helping to put existing adaptation capacities into action. It is also necessary to improve research capacity to understand, assess, and predict impacts so that appropriate response measures can be developed.
• Measures need to be taken to facilitate adaptation of high-mountain agriculture, fisheries, and livestock with the changes in snow and ice cover and their shifting patterns.
• The mitigation of cryosphere change mainly depends on actions to reduce greenhouse gas emissions, which will require substantial decarbonization of the global economy and energy system. Urgent global action is critical to reducing global warming. Addressing the impacts of cryosphere shrinkage will require regional and international cooperation to share experiences and knowledge as well as monitoring risks and facilitating adaption and mitigation measures.
Data Availability
All datasets generated for this study are included in the manuscript and/or the Supplementary Files.
Author Contributions
GR: conceptualization, data collection, data analysis, writing, and revising. DM: conceptualization and writing.
Funding
This work was supported by the core funds of ICIMOD contributed by the governments of Afghanistan, Australia, Austria, Bangladesh, Bhutan, China, India, Myanmar, Nepal, Norway, Pakistan, Sweden, and Switzerland.
Conflict of Interest Statement
The authors declare that the research was conducted in the absence of any commercial or financial relationships that could be construed as a potential conflict of interest.
Acknowledgments
We extend our sincere thanks to the handling Editor Carmen de Jong and two reviewers Martin John Siegert and Zoe Courville for their constructive and insightful comments which helped to improve the paper. Authors benefited from the comments from Alexander Milner, University of Birmingham, and Eklabya Sharma from ICIMOD on earlier version of the paper. Prakriti Gurung and Sakhie Pant assisted in collecting literature and Sam Inglis in preparing the figure. The views and interpretations in this publication are those of the authors and are not attributable to the ICIMOD.
Supplementary Material
The Supplementary Material for this article can be found online at: https://www.frontiersin.org/articles/10.3389/fenvs.2019.00091/full#supplementary-material
References
Aggarwal, P. K. (2008). Global climate change and Indian agriculture: impacts, adaptation and mitigation. India J. Agr. Res. 78, 911−919.
Allen, S. K., Zhang, G., Cuaresma, J. C., and Bolach, T. (2019). Potentially dangerous glacial lakes across the Tibetan Plateau revealed using a large-scale automated assessment approach. Sci. Bull. 64, 435–445. doi: 10.1016/j.scib.2019.03.011
Allison, E. H., Perry, A. L., Badjeck, M. C., Neil Adger, W., Brown, K., Conway, D., et al. (2009). Vulnerability of national economies to the impacts of climate change on fisheries. Fish Fish. 10, 173–196. doi: 10.1111/j.1467-2979.2008.00310.x
Bahuguna, I. M., Rathore, B. P., Brahmbhatt, R., and Sharma, M. C. (2014). Are the Himalayan glaciers retreating? Curr. Sci. India 106, 1008–1013.
Bajracharya, S. R. (2010). Glacial lake outburst flood disaster risk reduction activities in Nepal, Inter. J. Eros. Contr. Eng. 3, 92–101. doi: 10.13101/ijece.3.92
Bales, R. C., Molotch, N. P., Painter, T. H., Dettinger, M. D., Rice, R., and Dozier, J. (2006). Mountain hydrology of the western United States. Water Resour. Res. 42, 1–13. doi: 10.1029/2005WR004387
Baraer, M., McKenzie, J., Mark, B. G., Gordon, R., Bury, J., Condom, T., et al. (2015). Contribution of groundwater to the outflow from ungauged glacierized catchments: a multi-site study in the tropical Cordillera Blanca, Peru. Hydrol. Proc. 29, 2561–2581. doi: 10.1002/hyp.10386
Barnett, J., and Adger, W. N. (2007). Climate change, human security and violent conflict. Polit. Geogr. 26, 639–655. doi: 10.1016/j.polgeo.2007.03.003
Barnett, T. P., Adam, J. C., and Lettenmaier, D. P. (2005). Potential impacts of a warming climate on water availability in snow-dominated regions. Nature 438, 303–309. doi: 10.1038/nature04141
Barros, C., Guéguen, M., Douzet, R., Carboni, M., Boulangeat, I., Zimmermann, N. E., et al. (2017). Extreme climate events counteract the effects of climate and land-use changes in Alpine tree lines. J. Appl. Ecol. 54, 39–50. doi: 10.1111/1365-2664.12742
Beniston, M. (2012). Is snow in the Alps receding or disappearing? Wiley Interdiscip. Rev. Clim. Change 3, 349–358. doi: 10.1002/wcc.179
Beniston, M., Farinotti, D., Stoffel, M., Andreassen, L. M., Coppola, E., Eckert, N., et al. (2018). The European mountain cryosphere: a review of its current state, trends, and future challenges. Cryosphere 12, 759–794. doi: 10.5194/tc-12-759-2018
Beniston, M., and Stoffel, M. (2014). Assessing the impacts of climatic change on mountain water resources. Sci. Tot. Environ. 493, 1129–1137. doi: 10.1016/j.scitotenv.2013.11.122
Beniston, M., Stoffel, M., and Hill, M. (2011). Impacts of climatic change on water and natural hazards in the Alps: can current water governance cope with future challenges? Examples from the European “ACQWA” project. Environ. Sci. Policy 14:734. doi: 10.1016/j.envsci.2010.12.009
Berman, M., and Schmidt, J. I. (2019). Economic effects of climate change in Alaska. Weather Clim. Soc. 11, 245–258. doi: 10.1175/WCAS-D-18-0056.1
Bettinetti, R. (2011). Sediment analysis to support the recent glacial origin of DDT pollution in Lake Iseo (Northern Italy). Chemosphere 85, 163–169. doi: 10.1016/j.chemosphere.2011.06.037
Bettinetti, R., Quadroni, S., Galassi, S., Bachetta, R., Bonardi, L., and Vailati, G. (2008). Is meltwater from Alpine glaciers a secondary DDT source for lakes. Chemosphere 73, 1027–1031. doi: 10.1016/j.chemosphere.2008.08.017
Biemans, H., Speelman, L. H., Ludwig, F., Moors, E. J., Wiltshire, A. J., Kumar, P., et al. (2013). Future water resources for food production in five South Asian river basins and potential of adaptation options–a modelling study. Sci. Tot. Environ. 468–469, S117–S131. doi: 10.1016/j.scitotenv.2013.05.092
Bizzotto, E. C., Villa, S., Vaj, C., and Vighi, M. (2009). Comparison of glacial and non-glacial-fed streams to evaluate the loading of persistent organic pollutants through seasonal snow/ice melt. Chemosphere 74, 924–930. doi: 10.1016/j.chemosphere.2008.10.013
Blais, J. M., Schindler, D. W., Muir, D. C., Sharp, M., Donald, D., Lafreniére, M., et al. (2001). Melting glaciers: a major source of persistent organochlorines to subalpine Bow Lake in Banff National Park, Canada. Ambio 30, 410–415. doi: 10.1579/0044-7447-30.7.410
Bliss, A., Hock, R., and Radić, V. (2014). Global response of glacier runoff to twenty-first century climate change. J. Geophys. Res. Earth 119, 717–730. doi: 10.1002/2013JF002931
Boehlert, B., Strzepek, K. M., Gebretsadik, Y., Swanson, R., McCluskey, A., Neumann, J. E., et al. (2016). Climate change impacts and greenhouse gas mitigation effects on U.S. hydropower generation. Appl. Energy 183, 1511–1519. doi: 10.1016/j.apenergy.2016.09.054
Bogdal, C., Schmid, P., Zennegg, M., Anselmetti, F. S., Scheringer, M., and Hungerbühler, K. (2009). Blast from the past: melting glaciers as a relevant source for persistent organic pollutants. Environ. Sci. Technol. 43, 8173–8177. doi: 10.1021/es901628x
Böhner, J., and Lehmkuhl, F. (2005). Environmental change modelling for central and high Asia: pleistocene, present and future scenarios. Boreas 34, 220–231. doi: 10.1080/03009480510012917
Bolch, T., Kulkarni, A., Kääb, A., Huggel, C., Paul, F., Cogley, J., et al. (2012). The state and fate of Himalayan Glaciers. Science 336, 310–314. doi: 10.1126/science.1215828
Bolch, T., Shea, J. M., and Liu, S. (2019). Status and Change of the HKH Cryosphere in Hindu Kush Himalayan Monitoring and Assessment Programme. Cham: Springer Nature. doi: 10.1007/978-3-319-92288-1_7
Brander, K. (2007). Global fish production and climate change. PNAS 104, 19709–19714. doi: 10.1073/pnas.0702059104
Brown, L. E., Dickson, N. E., Carrivick, J. L., and Füreder, L. (2015). Alpine river ecosystem response to glacial and anthropogenic flow pulses. Freshw. Sci. 34, 1201–1215. doi: 10.1086/683062
Brown, T. C., Mahat, V., and Ramirez, J. A. (2019). Adaptation to future water shortages in the United States caused by population growth and climate change. Earth Fut. 7, 219–234. doi: 10.1029/2018EF001091
Burakowski, E., and Magnusson, M. (2012). Climate Impacts on the Winter Tourism Economy in the United States. New York, NY: Natural Resources Defense Council.
Bury, J., Mark, B. G., Carey, M., Young, K. R., McKenzie, J. M., Baraer, M., et al. (2013). New geographies of water and climate change in Peru: Coupled natural and social transformations in the Santa River watershed. Ann. Assoc. Am. Geogr. 103, 363–374. doi: 10.1080/00045608.2013.754665
Bury, J. T., Mark, B. G., McKenzie, J. M., French, A., Baraer, M., Huh, K. I., et al. (2011). Glacier recession and human vulnerability in the Yanamarey watershed of the Cordillera Blanca, Peru. Clim. Change 105, 179–206. doi: 10.1007/s10584-010-9870-1
Buxton, N., and Escobar, M. (2013). Water Scarcity, Climate Change and Bolivia: Planning for Climate Uncertainties. Available online at: https://www.sei.org/publications/water-scarcity-climate-change-and-bolivia-planning-for-climate-uncertainties/ (accessed April 20, 2019).
Buytaert, W., and De Bièvre, B. (2012). Water for cities: The impact of climate change and demographic growth in the tropical Andes. Water Resour. Res. 48:8503. doi: 10.1029/2011WR011755
Buytaert, W., Moulds, S., Acosta, L., De Bievre, B., Olmos, C., Villacis, M., et al. (2017). Glacial melt content of water use in the tropical Andes. Environ. Res. Lett. 12:114014. doi: 10.1088/1748-9326/aa926c
Callaghan, T. V., Jonasson, C., Thierfelder, T., Yang, Z., Hedenås, H., Johansson, M., et al. (2013). Ecosystem change and stability over multiple decades in the Swedish subarctic: complex processes and multiple drivers. Philos. Trans. Royal Soc. B 368:20120488. doi: 10.1098/rstb.2012.0488
Carey, M., Molden, O., Rasmussen, M., Jackson, M., Nolin, A. W., and Mark, B. G. (2017). Impacts of glacier recession and declining meltwater on mountain societies. Ann. Assoc. Am. Geogr. 107, 350–359. doi: 10.1080/24694452.2016.1243039
Chauhan, M. (2010). A perspective on watershed development in the Central Himalayan State of Uttarakhand, India. Int. J. Ecol. Environ. Sci. 36, 253–269
Cheesbrough, K., Edmunds, J., Tootie, G., Kerr, G., and Pochop, L. (2009). Estimated Wind River range (Wyoming, USA) glacier meltwater contributions to agriculture. Remote Sens. 1, 818–828. doi: 10.3390/rs1040818
Cheng, G., and Wu, T. (2007). Responses of permafrost to climate change and their environmental significance, Qinghai-Tibet Plateau. J. Geophys. Res. 112, 1–10. doi: 10.1029/2006JF000631
Chevallier, P., Pouyaud, B., Suarez, W., and Condom, T. (2011). Climate change threats to environment in the tropical Andes: glaciers and water resources. Reg. Environ. Change 11, 179–187. doi: 10.1007/s10113-010-0177-6
Clouse, C. (2016). Frozen landscapes: climate-adaptive design interventions in Ladakh and Zanskar. Landsc. Res. 41, 821–837. doi: 10.1080/01426397.2016.1172559
Clouse, C., Anderson, N., and Shippling, T. (2016). Ladakh's artificial glaciers: climate-adaptive design for water scarcity. Clim. Dev. 9, 428–438. doi: 10.1080/17565529.2016.1167664
Condom, T., Escobar, M., Purkey, D., and Pouget, J. (2012). Simulating the implications of glaciers' retreat for water management: a case study in the Rio Santa basin, Peru. Water Int. 37, 442–459. doi: 10.1080/02508060.2012.706773
Cramer, W., Yohe, G. W., Auffhammer, M., Huggel, C., Molau, U., Dias, M. D. S., et al. (2014). “Detection and attribution of observed impacts,” in Climate Climate Change 2014: Impacts, Adaptation, and Vulnerability, eds C.B. Field, V.R. Barros, D.J. Dokken, K.J. Mach, M.D. Mastrandrea, T.E. Bilir, M. Chatterjee, K.L. Ebi, Y.O. Estrada, R.C. Genova, B. Girma, E.S. Kissel, A.N. Levy, S. MacCracken, P.R. Mastrandrea, and L.L. White (Cambridge University Press), 979–1038.
Cruz, R. V., Harasawa, H., Lal, M., Wu, S., Anokhin, Y., Punsalmaa, B., et al. (2007). “Asia,” Climate Change 2007: Impacts, Adaptation and Vulnerability. Contribution of Working Group II to the Fourth Assessment Report of the IPCC. Cambridge University Press.
Cullen, N. J., Sirguey, P., Molg, T., Kaser, G., Winkler, M., and Fitzsimons, S. J. (2013). A century of ice retreat on Kilimanjaro: the mapping reloaded. Cryosphere 7, 419–431. doi: 10.5194/tc-7-419-2013
Dame, J., and Nusser, M. (2011). Food security in high mountain regions: agricultural production and the impact of food subsidies in Ladakh, Northern India. Food Secur. 3, 179–194. doi: 10.1007/s12571-011-0127-2
de Jong, C. (2011). “Artificial production of snow, encyclopedia of snow, ice and glaciers,” in Earth Sciences Series, eds U. K. Haritashya, P. Singh, and V. P. et Singh (Dordrecht: Springer, 61–66. doi: 10.1007/978-90-481-2642-2
de Jong, C. (2015). Challenges for mountain hydrology in the third millennium. Front. Environ. Sci. 3:38. doi: 10.3389/fenvs.2015.00038
de Jong, C., Lawler, D., and et Essery, R. (2009). Mountain hydroclimatology and snow seasonality–perspectives on climate impacts, snow seasonality and hydrological change in mountain environments in mountain hydroclimatology and snow seasonality. Hydrol. Proc. 23, 955–961. doi: 10.1002/hyp.7193
DeBeer, C. M., Wheater, H. S., Carey, S. K., and Chun, K. P. (2016). Recent climatic, cryospheric, and hydrological changes over the interior of western Canada: a review and synthesis. Hydrol. Earth Syst. Sci. 20, 1573–1598. doi: 10.5194/hess-20-1573-2016
DellaSala, D. A. (2013). Global Change. Reference Module in Earth Systems and Environmental Sciences. Elsevier. doi: 10.1016/B978-0-12-409548-9.05355-0
Deng, M., Zhang, H., Mao, W., and Wang, Y. (2011). Public perceptions of cryosphere change and the selection of adaptation measures in the Ürümqi River Basin. Adv. Clim. Change Res. 2, 149–158. doi: 10.3724/SP.J.1248.2011.00149
Derksen, C., Smith, S. L., Sharp, M., Brown, L., Howell, S., Copland, L., et al. (2012). Variability and change in the Canadian cryosphere. Clim. Change 115, 59–88. doi: 10.1007/s10584-012-0470-0
Di Baldassarre, G., Wanders, N., AghaKouchak, A., Kuil, L., Rangecroft, S., Veldkamp, T. I., et al. (2018). Water shortages worsened by reservoir effects. Nat. Sustain. 1:617. doi: 10.1038/s41893-018-0159-0
Dirmeyer, P. A., and Brubaker, K. L. (2007). Characterization of the global hydrologic cycle from a back-trajectory analysis of atmospheric water vapor. J. Hydrometeorol. 8, 20–37. doi: 10.1175/JHM557.1
Donald, D. B., Syrgiannis, J., Crosley, R. W., Holdsworth, G., Muir, D. C., Rosenberg, B., et al. (1999). Delayed deposition of organochlorine pesticides at a temperate glacier. Environ. Sci. Technol. 33, 1794–1798. doi: 10.1021/es981120y
Edwards, A. C., Scalenghe, R., and Freppaz, M. (2007). Changes in the seasonal snow cover of alpine regions and its effect on soil processes: a review. Quatern Int. 162, 172−181. doi: 10.1016/j.quaint.2006.10.0
Falk, M. (2016). The stagnation of summer glacier skiing. Tour. Anal. 21, 117–122. doi: 10.3727/108354216X14537459509053
Farinotti, D., Longuevergne, L., Moholdt, G., Duethmann, D., Mölg, T., Bolch, T., et al. (2015). Substantial glacier mass loss in the Tien Shan over the past 50 years. Nat. Geosci. 8, 716–722. doi: 10.1038/ngeo2513
Forslund, A., Renöfält, B. M., Barchiesi, S., Cross, K., Davidson, S., Farrell, T., et al. (2009). Securing Water for Ecosystems and Human Well-being: The Importance of Environmental Flows. Swedish Water House Report, 24.
Fountain, A. G., Campbell, J. L., Schuur, E. A. G., Stammerjohn, S. E., Williams, M. W., and Ducklow, H. W. (2012). The disappearing cryosphere: impacts and ecosystem responses to rapid cryosphere loss. Bioscience 62, 405–415. doi: 10.1525/bio.2012.62.4.11
French, A., Barandiaran, J., and Rampini, C. (2015). “Contextualizing conflict: vital waters and competing values in glaciated environments,” in The High Mountain Cryosphere: Environmental Changes and Human Risks, eds C. Huggel, M. Carey, J. Clague, and A. Kääb. (Cambridge: Cambridge University Press). 315–336.
Freppaz, M., Celi, L., Marchelli, M., and Zanini, E. (2008). Snow removal and its influence on temperature and N dynamics in alpine soils (Vallee d'Aoste, northwest Italy), plant nutrition and soil. Science 171, 672–680. doi: 10.1002/jpln.200700278
Galaz, V., Moberg, F., Olsson, E. K., Paglia, E., and Parker, C. (2011). Institutional and political leadership dimensions of cascading ecological crises. Public Adm. 89, 361–380. doi: 10.1111/j.1467-9299.2010.01883.x
Gardner, A. S., Moholdt, G., Cogley, J. G., Wouters, B., Arendt, A. A., Wahr, J., et al. (2013). A reconciled estimate of glacier contributions to sea level rise: 2003 to 2009. Science 340, 852–857. doi: 10.1126/science.1234532
Gaudard, L., Gilli, M., and Romerio, F. (2013). Climate change impacts on hydropower management. Water Res. Manag. 27, 5143–5156. doi: 10.1007/s11269-013-0458-1
Gleick, P. H. (2014). Water, drought, climate change, and conflict in Syria. Weather Clim. Soc. 6, 331–340. doi: 10.1175/WCAS-D-13-00059.1
Gonzalez, P., Garfin, G. M., Breshears, D. D., Brooks, K. M., Brown, H. E., Elias, E. H., et al. (2018). “Southwest,” in Impacts, Risks, and Adaptation in the United States: Fourth National Climate Assessment, Vol. II, eds D. R. Reidmiller, C. W. Avery, D. R. Easterling, K. E. Kunkel, K. L. M. Lewis, T. K. Maycock, and B. C. Stewart (Washington, DC: U.S. Global Change Research Program), 1101–1184.
Gottfried, M., Pauli, H., Futschik, A., Akhalkatsi, M., Barančok, P., Alonso, J. L. B., et al. (2012). Continent-wide response of mountain vegetation to climate change. Nat. Clim. Chang. 2, 111–115. doi: 10.1038/nclimate1329
Grannas, A. M., Bogdal, C., Hageman, K. J., Halsall, C., Harne, T., Hung, H., et al. (2013). The role of the global cryosphere in the fate of organic contaminants. Atmos. Chem. Phys. 13, 3271–3305. doi: 10.5194/acp-13-3271-2013
Grimm, N. B., Chapin, I. I. I. F. S, Bierwagen, B., et al. (2013). The impacts of climate change on ecosystem structure and function. Front. Ecol. Environ. 11:120282. doi: 10.1890/120282
Grossman, D. (2015). As Himalayan Glacier melts, two towns face the fall out. Yale Environment 360. Yale school of Forestry and Environment Studies. Available online at: http://e360.yale.edu/features/as_himalayan_glaciers_melt_two_towns_face_the_fallout
Haeberli, W., and Whiteman, C. (2015). “Snow and ice-related hazards, risks, and disasters: a general framework,” in Snow and Ice-Related Hazards, Risks and Disasters, (Academic Press), 1–34. doi: 10.1016/B978-0-12-394849-6.00001-9
Hamilton, L. C., Rohall, D. E., Brown, B. C., Hayward, G., and Keim, B. D. (2003). Warming winters and New Hampshire's lost ski areas: an integrated case study. Int. J. Sociol. Soc. Policy 23, 52–73. doi: 10.1108/01443330310790309
He, S., and Richards, K. (2015). Impact of meadow degradation on soil water status and pasture management: a case study in Tibet. Land Degrad. Dev. 26, 468–479. doi: 10.1002/ldr.2358
Hovelsrud, G. K., and Smit, B. (2010). Community Adaptation and Vulnerability in the Arctic Regions. Berlin: Springer Publishers.
Hoy, A., Katel, O., Thapa, P., Dendup, N., and Matschullat, J. (2016). Climatic changes and their impact on socio-economic sectors in the Bhutan Himalayas: an implementation strategy. Reg. Environ. Change. 16, 1401–1415. doi: 10.1007/s10113-015-0868-0
Huggel, C. M., Carey, J. J., and Clague, Kääb, A. (2015). The High-Mountain Cryosphere: Environmental Changes and Human Risks. Cambridge: Cambridge University Press, 1–371.
Huijun, J., He, R. X., Cheng, G. D., Wu, Q. B., Wang, S. L., Lu, L. Z., et al. (2009). Changes in frozen ground in the source area of the Yellow River on the Qinghai-Tibet Plateau, China, and their eco-environmental impacts. Environ. Res. Lett. 4:045206. doi: 10.1088/1748-9326/4/4/045206
Huss, M., Bookhagen, B., Huggel, C., Jacobsen, D., Bradley, R. S., Clague, J. J., et al. (2017). Toward mountains without permanent snow and ice. Earth Fut. 5, 418–435. doi: 10.1002/2016EF000514
Huss, M., and Hock, R. (2018). Global-scale hydrological response to future glacier mass loss. Nat. Clim. Chang. 8, 135–140. doi: 10.1038/s41558-017-0049-x
ICIMOD (2010). Glacial Melt and Downstream Impacts on Indus Dependent Water Resources and Energy. Kathmandu.
Immerzeel, W. W., van Beek, L. P. H., and Bierkens, M. F. P. (2010). Climate change will affect the Asian water towers. Science 328, 1382–1385. doi: 10.1126/science.1183188
IPCC (2014). Climate Change 2014: Synthesis Report. Contribution of Working Groups I, II and III to the Fifth Assessment Report of the Intergovernmental Panel on Climate Change, eds R. K. Pachauri and L. A. Meyer. Geneva: Core Writing Team, IPCC.
Jehangir, W. A., Mudasser, M., Hassan, M., and Ali, Z. (1998). Multiple Uses of Irrigation Water in the Hakra-6/R Distributary Command Area, Punjab, Pakistan. Report R-61, Pakistan National Program. Lahore: International Water Management Institute.
Jin, H. J., Wu, J., Cheng, G. D., Nakano, T., and Sun, G. Y. (1999). Estimation of CH4 emissions from cold wetland ecosystems on the Tibetan Plateau. J. Glaciol. 21, 339–350.
Kaltenborn, B. P., Nellemann, C., and Vistnes, I. I. (2010). High mountain glaciers and climate change. Challenges to human livelihoods and adaptation, Arendal: UNEP-GRID Arendal.
Kaser, G., Grosshauser, M., and Marzeion, B. (2010). Contribution potential of glaciers to water availability in different climate regimes. Proc. Natl. Acad. Sci. U. S. A. 107, 20223–20227. doi: 10.1073/pnas.1008162107
Khadka, R. B., and Khanal, A. B. (2008). Environmental management plan (EMP) for Melamchi water supply project, Nepal. Environ. Monit. Assess. 146, 225–234. doi: 10.1007/s10661-007-0074-8
Khanal, N. R., Mool, P. K., Shrestha, A. B., Rasul, G., Ghimire, P. K., Shrestha, R. B., et al. (2015). A comprehensive approach and methods for glacial lake outburst flood risk assessment, with examples from Nepal and the transboundary area. Int. J. Water Resour. D. 31, 219–237. doi: 10.1080/07900627.2014.994116
Kinouchi, T., Nakajima, T., Mendoza, J., and Asaoka, Y. (2019). Water security in high mountain cities of the Andes under a growing population and climate change: A case study of La Paz and El Alto, Bolivia. Water Sec. 6:100025. doi: 10.1016/j.wasec.2019.100025
Kopytkovskiy, M., Geza, M., and McCray, J. E. (2015). Climate-change impacts on water resources and hydropower potential in the Upper Colorado River Basin. J. Hydrol. 3, 473–493. doi: 10.1016/j.ejrh.2015.02.014
Kranaijenbrink, P. D. A., Bierkens, M. F. P., Lutz, A. F., and Immerzeel, W. W. (2017). Impact of a global temperature rise of 1.5 degrees Celsius on Asia's glaciers. Nature 549, 257–260. doi: 10.1038/nature23878
Lafreniere, M., and Sharp, M. (2005). A comparison of solute fluxes and sources from glacial and non-glacial catchments over contrasting melt seasons. Hydrol. Process. 19, 2991–3012. doi: 10.1002/hyp.5812
Laghari, J. R. (2013). Climate change: melting glaciers bring energy uncertainty. Nature 502, 617–618. doi: 10.1038/502617a
Lawrence, J., Blackett, P., Cradock-Henry, N., and Nistor, B. J. (2018). Climate Change: The Cascade Effect. Cascading Impacts and Implications for Aotearoa New Zealand. Wellington: Deep South Challenge.
Lehodey, P., Alheit, J., Barange, M., Baumgartner, T., Beaugrand, G., Drinkwater, K., et al. (2006). Climate variability, fish, and fisheries. J. Clim. 19, 5009–5030. doi: 10.1175/JCLI3898.1
Lutz, A., Immerzeel, W. W., Bajracharya, S. R., Litt, M., and Shrestha, A. (2016). Impact of Climate Change on the Cryosphere, Hydrological Regimes and Glacial Lakes of the Hindu Kush Mimalayas: A Review Of Current Knowledge. ICIMOD Research Report 2016/3. Kathmandu: ICIMOD.
Lutz, A. F., Shrestha, A. B., Bierkens, M. F. P., Immerzeel, W. W., and Shrestha, A. B. (2014). Consistent increase in High Asia's runoff due to increasing glacier melt and precipitation. Nat. Clim. Change 4, 587–592. doi: 10.1038/nclimate2237
Ma, J., Hung, H., Tian, C., and Kallenborn, R. (2011). Revolatilization of persistent organic pollutants in the Arctic induced by climate change. Nat. Clim. Change 1, 256–260. doi: 10.1038/nclimate1167
Malik, R. (2008). Growth Impacts of Development and Management of Water Resources in Proceedings: Annual IWMI-TATA Partners Meet. Hyderabad: International Water Management Institute.
Mark, B., Bury, J., McKenzie, J., French, A., and Baraer, M. (2010). Climate change and tropical andean glacier recession: evaluating hydrologic changes and livelihood vulnerability in the Cordillera Blanca, Peru. Ann. Assoc. Am. Geogr. 100, 794–805. doi: 10.1080/00045608.2010.497369
Mark, B. G., French, A., Baraer, M., Carey, M., Bury, J., Young, K. R., et al. (2017). Glacier loss and hydro-social risks in the Peruvian Andes. Glob. Planet Change 159, 61–76. doi: 10.1016/j.gloplacha.2017.10.003
Matthew, R., Barnett, J., McDonald, B., and O'Brien, K. (2009). Global Environmental Change and Human Security. London: The MIT Press.
McDowell, G., Ford, J. D., Lehner, B., Berrang-Ford, L., and Sherpa, A. (2013). Climate-related hydrological change and human vulnerability in remote mountain regions: a case study from Khumbu, Nepal. Reg. Environ. Change 13, 299–310. doi: 10.1007/s10113-012-0333-2
McDowell, G., Huggel, C., Frey, H., Wang, F. M., Cramer, K., and Ricciardi, V. (2019). Adaptation action and research in glaciated mountain systems: are they enough to meet the challenge of climate change? Glob. Environ. Change 54, 19–30. doi: 10.1016/j.gloenvcha.2018.10.012
Messerli, B., Viviroli, D., Dürr, H., Meybeck, M., and Weingartner, R. (2007). Mountains of the world, water towers for humanity: typology, mapping, and global significance. Water Resourc. Res. 43, 1–13. doi: 10.1029/2006WR005653
Millennium Ecosystem Assessment (2005). Ecosystems and Human Well-being: Synthesis. Washington, DC: Island Press.
Milner, A. M., Khamis, K., Battin, T. J., Brittain, J. E., Barrand, N. E., Füreder, L., et al. (2017). Glacier shrinkage driving global changes in downstream systems. Proc. Natl. Acad. Sci. U S A, 114, 9770–9778. doi: 10.1073/pnas.1619807114
Mool, P. K., Wangda, D., Bajracharya, S. R., Joshi, S. P., Kunzang, K., and Gurung, D. R. (2001). Inventory of Glaciers, Glacial Lakes and Glacial Lake Outburst Floods: Monitoring and Early Warning Systems in the Hindu Kush-Himalayan Region. Kathmandu: ICIMOD.
Moore, R. D., Fleming, S. W., Menounos, B., Wheate, R., Fountain, A., Stahl, K., et al. (2009). Glacier change in western North America: influences on hydrology, geomorphic hazards and water quality. Hydrol. Process. 23, 42–61. doi: 10.1002/hyp.7162
Moors, E. J., and Stoffel, M. (2013). Changing monsoon patterns, snow and glacial melt, its impacts and adaptation options in northern India: setting the stage. Sci. Total Environ. 468–469, S1–S3. doi: 10.1016/j.scitotenv.2013.10.033
Morselli, M., Semplice, M., Villa, S., and Di Guardo, A. (2014). Evaluating the temporal variability of concentrations of POPs in a glacier-fed stream food chain using a combined modeling approach. Sci. Total Environ. 493, e571–e579. doi: 10.1016/j.scitotenv.2014.05.150
Mukherji, A., Molden, D., Nepal, S., Rasul, G., and Wagnon, P. (2015). Himalayan waters at the crossroads: issues and challenges. Int. J. Water Resour. Development. 31, 151–160. doi: 10.1080/07900627.2015.1040871
Mukherji, A., Sinisalo, A., Nüsser, M., Garrard, R., and Eriksson, M. (2019). Contributions of the cryosphere to high mountain communities of the Hindu Kush Himalaya: a review. Reg. Environ. Change 19, 1311–1326. doi: 10.1007/s10113-019-01484-w
Nüsser, M., Dame, J., Kraus, B., Baghel, R., and Schmidt, S. (2018). Socio-hydrology of “artificial glaciers” in Ladakh, India: assessing adaptive strategies in a changing cryosphere. Reg. Environ. Change. 19, 1327–1337. doi: 10.1007/s10113-018-1372-0
Nüsser, M., and Schmidt, S. (2017). Nanga parbat revisited: evolution and dynamics of sociohydrological interactions in the Northwestern Himalaya. Ann. Assoc. Am. Geogr. 107, 403–415. doi: 10.1080/24694452.2016.1235495
Parmesan, C. (2006). Ecological and evolutionary responses to recent climate change. Annu. Rev. Ecol. Evol. Sci. 37, 637–669. doi: 10.1146/annurev.ecolsys.37.091305.110100
Parveen, S., Winiger, M., Schmidt, S., and Nüsser, M. (2015). Irrigation in Upper Hunza: evolution of socio-hydrological interactions in the Karakoram, northern Pakistan. Erdkunde 69, 69–85. doi: 10.3112/erdkunde.2015.01.05
Paudel, K., and Andersen, P. (2013). Response of rangeland vegetation to snow cover dynamics in Nepal Trans Himalaya. Clim. Change 117, 149–162. doi: 10.1007/s10584-012-0562-x
Paul, F., Kaab, A., and Haeberli, W. (2007). Recent glacier changes in the Alps observed by satellite: consequences for future monitoring strategies. Global Planet Change 56, 111–122. doi: 10.1016/j.gloplacha.2006.07.007
Pouyaud, B., Zapata, M., Yerren, J., Gomez, J., Rosas, G., Suarez, W., et al. (2005). On the future of the water resources from glacier melting in the Cordillera Blanca, Peru. Hydrol. Sci. J. 50, 999–1022. doi: 10.1623/hysj.2005.50.6.999
Punkari, M., Droogers, P., Immerzeel, W, Korhonen, N., Lutz, A., and Venäläinen, A. (2014). “Climate Change and Sustainable Water Management in Central Asia,” ADB Central and West Asia Working Paper Series, No 5 (Manila: Asian Development Bank).
Qin, D., and Ding, Y. (2010). Key issues on cryospheric changes, trends and their impacts. Adv. Clim. Change Res. 1, 1–10. doi: 10.3724/SP.J.1248.2010.00001
Qureshi, A. S. (2011). Water management in the Indus Basin in Pakistan: challenges and opportunities. Mt. Res. Dev. 31, 252–260. doi: 10.1659/MRD-JOURNAL-D-11-00019.1
Rabatel, A., Francou, B., Soruco, A., Gomez, J., Cáceres, B., Ceballos, J. L., et al. (2013). Current state of glaciers in the tropical Andes: a multi-century perspective on glacier evolution and climate change. The Cryosphere 7, 81–102. doi: 10.5194/tc-7-81-2013
Rangecroft, S., Harrison, S., Anderson, J., Magrath, A., Castel, P., and Pacheco, P. (2013). Climate change and water resources in arid mountains: an example from the Bolivian Andes. Ambio 42, 852–863. doi: 10.1007/s13280-013-0430-6
Rasul, G. (2014). Food, water, and energy security in South Asia: a nexus perspective from the Hindu Kush Himalayan region. Environ. Sci. Policy 39, 35–48. doi: 10.1016/j.envsci.2014.01.010
Rasul, G., Pasakhala, B., Mishra, A., and Pant, S. (2019). Adaptation to mountain cryospheric change: issues and challenges. Clim. Dev. 16. doi: 10.1080/17565529.2019.1617099
Ravanel, L., Deline, P., Lambiel, C., and Vincent, C. (2013). Instability of a high alpine rock ridge: the lower Arete des Cosmiques, Mont Blanc Massif, France. Geogr. Ann. A 95, 51–66. doi: 10.1111/geoa.12000
Rees, H. G., Holmes, M. G. R., Young, A. R., and Kansaker, S. R. (2004). Recession-based hydrological models for estimating low flows in ungauged catchments in the Himalayas. Hydrol. Earth Syst. Sci. Discuss. 8, 891–902. doi: 10.5194/hess-8-891-2004
Schmid, P., Bogdal, C., Bluthgen, N., Anselmetti, F. S., Zwyssig, A., and Hungerbuhler, K. (2011). The missing piece: sediment records in remote mountain lakes confirm glaciers being secondary sources of persistent organic pollutants. Environ. Sci. Technol. 45, 203–208. doi: 10.1021/es1028052
Schoolmeester, T., Johansen, K. S., Alfthan, B., Baker, E., Hesping, M., and Verbist, K. (2018). The Andean and Glacier Water Atlas - The Impact of Glacier Retreat on Water Resources. UNESCO and GRID-Arendal.
Schuur, E. A., Bockheim, J., Canadell, J. G., Euskirchen, E., Field, C. B., Goryachkin, S. V., et al. (2008). Vulnerability of permafrost carbon to climate change: Implications for the global carbon cycle. Bioscience 58, 701–714. doi: 10.1641/B580807
Schwanghart, W., Worni, R., Huggel, C., Stoffel, M., and Korup, O. (2016). Uncertainty in the Himalayan energy–water nexus: Estimating regional exposure to glacial lake outburst floods. Environ. Res. Lett. 11:074005. doi: 10.1088/1748-9326/11/7/074005
Sharma, B. M., Nizzetto, L., Bharat, G. K., Tayal, S., Melymuk, L., Sánka, O., et al. (2015). Melting Himalayan glaciers contaminated by legacy atmospheric depositions are important sources of PCBs and high-molecular-weight PAHs for the Ganges floodplain during dry periods. Environ. Pollut. 206, 588–596. doi: 10.1016/j.envpol.2015.08.012
Shrestha, A. B., Eriksson, M., Mool, P., Ghimire, P., Mishra, B., and Khanal, N.R. (2010). Glacial lake outburst flood risk assessment of Sun Koshi basin, Nepal. Geomat. Nat. Hazards Risk 1, 157–169. doi: 10.1080/19475701003668968
Shrestha, K. L. (2005). Global change impact assessment for Himalayan mountain regions for environmental management and sustainable development. Glob. Environ. Res. 9, 69–81.
Shrestha, S., Bajracharya, A., and Babel, M. (2016). Assessment of risks due to climate change for the Upper Tamakoshi Hydropower Project in Nepal. Clim. Risk Manag. 14, 27–41. doi: 10.1016/j.crm.2016.08.002
Siderius, C., Biemans, H., Wiltshire, A., Rao, S., Franssen, W. H. P., Kumar, P., et al. (2013). Snowmelt contributions to discharge of the Ganges. Sci. Total Environ. 468–469, S93–S101. doi: 10.1016/j.scitotenv.2013.05.084
Singh, S. P., Singh, V., and Skutsch, M. (2010). Rapid warming in the Himalayas: ecosystem responses and development options. Clim. Dev. 2, 221–232. doi: 10.3763/cdev.2010.0048
Slaymaker, O., and Kelly, R. E. J. (2007). The Cryosphere and Global Environmental Change. Environmental Systems and Global Change Series 1. Malden, MA:Blackwell Publishing.
Somers, L. D., Gordon, R. P., McKenzie, J. M., Lautz, L. K., Wigmore, O., Glose, A., et al. (2016). Quantifying groundwater–surface water interactions in a proglacial valley, Cordillera Blanca, Peru. Hydrol. Process. 30, 2915–2929. doi: 10.1002/hyp.10912
Sorg, A., Huss, M., Rohrer, M., and Stoffel, M. (2014). The days of plenty might soon be over in glacierized Central Asian catchments. Environ. Res. Lett. 9:104018. doi: 10.1088/1748-9326/9/10/104018
Stahl, K., and Moore, R. D. (2006). Influence of watershed glacier coverage on summer streamflow in British Columbia, Canada. Water Resour. Res. 42:W06201. doi: 10.1029/2006WR005022
Stäubli, A., Nussbaumer, S. U., Allen, S. K., Huggel, C., Arguello, M., Costa, F., et al. (2018). Analysis of weather-and climate-related disasters in mountain regions using different disaster databases. in Climate Change, Extreme Events and Disaster Risk Reduction, eds S. Mal, R. B. Singh, and C. Huggel (Cham: Springer, 17–41.
Steiger, R., Scott, D., Abegg, B., Pons, M., and Aall, C. (2017). A critical review of climate change risk for ski tourism. Curr. Issues Tour. 5, 1–37. doi: 10.1080/13683500.2017.1410110
Stewart, I. T., Cayan, D. R., and Dettinger, M. D. (2005). Changes toward earlier streamflow timing across western North America. J. Clim. 18, 1136–1155. doi: 10.1175/JCLI3321.1
Sujakhu, N. M., Ranjitkar, S., Niraula, R. R., Pokharel, B. K., Schmidt-Vogt, D., and Xu, J. (2016). Farmers' perceptions of and adaptations to changing climate in the Melamchi valley of Nepal. Mt. Res. Dev. 36. 15–30. doi: 10.1659/MRD-JOURNAL-D-15-00032.1
Tahir, A. A., Chevallier, P., Arnaud, Y., Ashraf, M., and Bhatti, M. T. (2015). Snow cover trend and hydrological characteristics of the Astore River basin (Western Himalayas) and its comparison to the Hunza basin (Karakoram region). Sci. Tot. Environ. 505, 748–761. doi: 10.1016/j.scitotenv.2014.10.065
Taj, M. (2013). Peru Uses Climate Twist to Lure Tourists to Shrinking Glacier. Reuters. Available online at: http://www.reuters.com/article/us-peru-glacier-idUSBRE9A907Y20131110 (accessed March 31, 2017).
Tarroja, B., AghaKouchak, A., and Samuelsen, S. (2016). Quantifying climate change impacts on hydropower generation and implications on electric grid greenhouse gas emissions and operation. Energy 111, 295–305. doi: 10.1016/j.energy.2016.05.131
Telwala, Y., Brook, B. W., Manish, K., and Pandit, M. K. (2013). Climate-induced elevational range shifts and increase in plant Species richness in a Himalayan biodiversity epicentre. PLoS ONE 8:e57103. doi: 10.1371/journal.pone.0057103
Tiwari, P., and Joshi, B. (2015). Climate change and rural out-migration in Himalaya. Change Adapt. Socioecol. Syst. 2, 8–2. doi: 10.1515/cass-2015-0002
Turner, S. W. D., Ng, J. Y., and Galelli, S. (2017). Examining global electricity supply vulnerability to climate change using a high-fidelity hydropower dam model. Sci Tot. Environ. 590:663e75. doi: 10.1016/j.scitotenv.2017.03.022
UNESCO World Water Assessment Programme (2019). The United Nations World Water Development Report 2019: Leaving No One Behind. Paris: UNESCO.
Van Tiel, M., Teuling, A. J., Wanders, N., Vis, M. J., Stahl, K., and Van Loon, A. F. (2018). The role of glacier changes and threshold definition in the characterisation of future streamflow droughts in glacierised catchments. Hydrology Earth Syst. Sci. 22, 463–485. doi: 10.5194/hess-22-463-2018
Vergara, W., Deeb, A., Valencia, A., Bradley, R., Francou, B., Zarzar, A., et al. (2007). Economic impacts of rapid glacier retreat in the Andes. EOS 88, 261–263. doi: 10.1029/2007EO250001
Vincent, A., Violette, S., and Adalgeirsdottir, G. (2019). Groundwater in catchments headed by temperate glaciers: A review. Earth Sci. Rev. 188, 59–76. doi: 10.1016/j.earscirev.2018.10.017
Viste, E., and Sorteberg, A. (2015). Snowfall in the Himalayas: an uncertain future from a little-known past. Cryosphere 9, 1147–1167. doi: 10.5194/tc-9-1147-2015
Viviroli, D., Archer, D. R., Buytaert, W., Fowler, H. J., Greenwood, G., Hamle, A. F., et al. (2011). Climate change and mountain water resources: overview and recommendations for research, management and policy. Hydrol. Earth Syst. Sci. Discuss. 15, 471–504. doi: 10.5194/hess-15-471-2011
Vuille, M., Carey, M., Huggel, C., Buytaert, W., Rabatel, A., Jacobsen, D., et al. (2018). Rapid decline of snow and ice in the tropical Andes–Impacts, uncertainties and challenges ahead. Earth Sci. Rev. 176, 195–213. doi: 10.1016/j.earscirev.2017.09.019
Wang, B. L., and French, H. M. (1994). Climate controls and high-altitude permafrost, Qinghai– Xizang (Tibet) Plateau, China. Permafrost Periglac 5, 87–100. doi: 10.1002/ppp.3430050203
Wang, B. L., and French, H. M. (1995). Permafrost on the Tibet Plateau, China. Quat. Sci. Rev.14, 255–274. doi: 10.1016/0277-3791(95)00006-B
Wang, L., Li, Z., Wang, F., and Edwards, R. (2014). Glacier shrinkage in the Ebinur lake basin, Tien Shan, China, during the past 40 years. J. Glaciol. 60, 245–254. doi: 10.3189/2014JoG13J023
Warner, K. (2010). Global environmental change and migration: Governance challenges. Glob. Environ. Change 20, 402–413. doi: 10.1016/j.gloenvcha.2009.12.001
Warren, F. J., and Lemmen, D. S. (2014). Canada in a Changing Climate: Sector Perspectives on Impacts and Adaptation. Ottawa, ON: Government of Canada, 286. Available online at: https://www.weadapt.org/sites/weadapt.org/files/2017/february/canadasectorperspectivesfull-report_eng_0.pdf#page=70
Watson, R. T., and Haeberli, W. (2004). Environmental threats, mitigation strategies and high-mountain areas. Ambio Special Rep. 13, 2–10.
Wilhelm, L., Singer, G. A., Fasching, C., Battin, T. J., and Besemer, K. (2013). Microbial biodiversity in glacier-fed streams. Isme J. 7, 1651–1660. doi: 10.1038/ismej.2013.44
Williams, M. W., Seastedt, T. R., Bowman, W. D., McKnight, D. M., and Suding, K. N. (2015). An overview of research from a high elevation landscape: the Niwot Ridge, Colorado Long Term Ecological Research programme. Plant Ecol. Divers. 8, 597–605. doi: 10.1080/17550874.2015.1123320
Xiao, C., Wang, S., and Qin, D. (2015). A preliminary study on cryosphere service function and its value estimation. Adv. Clim. Change Res. 12, 45–52. doi: 10.1016/j.accre.2015.11.004
Xu, J., Grumbine, R. E., Shrestha, A., Eriksson, M., Yang, X., Wang, Y., et al. (2009). The melting Himalayas: cascading effects of climate change on water, biodiversity, and livelihoods. Conserv. Biol. 23, 520–530. doi: 10.1111/j.1523-1739.2009.01237.x
Yuan, H., and Hou, F. (2015). Grazing intensity and soil depth effects on soil properties in alpine meadow pastures of Qilian Mountain in northwest China. Acta Agr. Scand. B S P. 65, 222–232. doi: 10.1080/09064710.2014.992940
Zhao-ping, Y., Hua, O. Y., Xing-Liang, X., Lin, Z., Ming-hua, S., and Caiping, Z. (2010). Effects of permafrost degradation on ecosystems. Acta Ecol. Sin. 30, 33–39. doi: 10.1016/j.chnaes.2009.12.006
Keywords: cryospheric change, water security, food security, energy security, climate change, environmental degradation, adaption measures, SDGs
Citation: Rasul G and Molden D (2019) The Global Social and Economic Consequences of Mountain Cryospheric Change. Front. Environ. Sci. 7:91. doi: 10.3389/fenvs.2019.00091
Received: 22 April 2019;
Published: 21 June 2019.
Edited by:
Carmen De Jong, Université de Strasbourg, FranceReviewed by:
Martin John Siegert, Imperial College London, United KingdomZoe Courville, Cold Regions Research and Engineering Laboratory, United States
Copyright © 2019 Rasul and Molden. This is an open-access article distributed under the terms of the Creative Commons Attribution License (CC BY). The use, distribution or reproduction in other forums is permitted, provided the original author(s) and the copyright owner(s) are credited and that the original publication in this journal is cited, in accordance with accepted academic practice. No use, distribution or reproduction is permitted which does not comply with these terms.
*Correspondence: Golam Rasul, golam.rasul@icimod.org