- 1Department of Biological Sciences (DBS), Pwani University, Kilifi, Kenya
- 2Department of Biochemistry & Biotechnology (DBB), Pwani University, Kilifi, Kenya
- 3Pwani University Bioscience Research Centre (PUBReC), Pwani University, Kilifi, Kenya
Marine biodiversity is under increasing threat as the area covered by corals diminishes under pressure from climate change and human activities, most of which lead to marine pollution. In Kenya, marine protected areas (MPAs) are the key strategy used to protect coral reefs and biodiversity. However, MPAs' effectiveness to prevent pollution of the reefs has not been specifically assessed. We determined if the levels of surrogates of human-source pollution, i.e., E. coli and nutrient concentrations on Kenyan coral reefs, varied with increasing levels of marine protection at the Kilifi creek (least protection), Malindi Reserve (moderate protection), and Kuruwitu Conservancy (strictest protection). The most probable number (MPN) of E. coli was estimated by serial dilution while nitrate and orthophosphate concentrations were determined spectrophotometrically. As protection increased from “least,” to “moderate” and “strictest,” E. coli concentrations (MPN/100 mL) decreased from 29, to 16 and undetectable, while mean orthophosphate concentrations increased from 0.326, to 0.422 and 0.524 mg/L, respectively. Mean nitrate concentrations, on the other hand, showed no trend with protection. These results suggest the potential of marine protection to mitigate coral reef pollution, especially from microbes. They also point to the possibility that multiple sources of pollution exist on which marine protection may have little or no effect. Significantly, this pilot study points to the need for improved study design to definitively determine the role MPAs may play in protecting against pollution.
Introduction
The Western Indian Ocean (WIO) hosts the second largest coral reef biodiversity globally (Obura, 2012) and holds potential wealth for the region due to the significant economic role that oceans play to support jobs, tourism, and fisheries. Surprisingly, the blue economy of the WIO region remains underdeveloped.
The oceans' economic value depends on healthy coral reefs (Obura et al., 2017), which cover only 0.1% of the ocean floor but host at least 25% of all marine biodiversity (Hoegh-guldberg et al., 2017), provide goods and services such as seafood, recreational possibilities, coastal protection, and aesthetic and cultural benefits (Moberg and Folke, 1999). However, coral reef ecosystems are increasingly under threat mainly from climatic changes as evidenced by mass coral bleaching and/or death following episodes of elevated sea surface temperatures (Hughes et al., 2017). The situation is aggravated by overfishing and pollution (Hughes et al., 2003) from human activities on the coast zone leading to a decrease in the abundance of coral reef species. While corals may recover from bleaching episodes (Hughes et al., 2003; West and Salm, 2003), nutrient influx, especially of nitrate and phosphate, is thought to increase susceptibility to bleaching (Wiedenmann et al., 2013), compromising this resilience (Hall et al., 2018). The scale of human impacts is, therefore, bound to intensify over time as temperatures are expected to keep rising, while the human population also increases on coastal zones attracted by the goods and services of the marine ecosystems (Neumann et al., 2015).
Over the past 50 years, Kenya has mitigated anthropogenic impacts on its coastal and marine resources, through MPAs as the primary marine ecosystems management strategy (Samoilys and Obura, 2011). Two classes of MPAs, differing in the level of protection, were established and enforced by the Kenya Wildlife Services (KWS): marine parks' “no-take” areas protected from all forms of consumptive utilization, and marine reserves which are contiguous to parks acting as buffers. Here, artisanal, but not commercial fishing is allowed (Tuda and Omar, 2012). Owing to the success of MPAs, local communities are increasingly establishing and enforcing conservation areas termed as “locally managed marine areas” (LMMAs) which adopt the “no-take” protection management plan, like marine parks. Most LMMAs are administered with financial and/or technical support from non-governmental organizations and/or the government.
The primary goal for the implementation of MPAs and LMMAs is biodiversity conservation and the regulation of fisheries. These efforts have generally been successful, especially in increased and improved fish populations (Ransom and Mangi, 2010; Samoilys and Obura, 2011). While these strategies seem effective to curb the direct impacts of human-related pressures such as overfishing and fishery-related damages, it is necessary to interrogate their effectiveness on subtler effects that may result from spatially removed urbanization, tourism development, agriculture, and industrialization. Globally, these activities are major drivers of marine pollution (Shahidul Islam and Tanaka, 2004) posing health risks to fishery products, consumers and recreational users on the coasts (Kilinc and Besler, 2014), as well as leading to the death of corals and other reef organisms (Hipsey et al., 2008).
We conducted a pilot study to assess whether area-based marine protection may have the potential to curb human-source pollution. In coral reefs under differing levels of protection, we measured concentrations of surrogates for human-source pollution including, E. coli, a conventional indicator for fecal pollution and the subsequent public health risk from pathogenic microorganisms (Fremaux et al., 2009). We also measured nitrate and phosphate as they are known to be increased, in marine environments, by human activities (Jickells, 1998; Wiedenmann et al., 2013; Sayadi et al., 2016). Furthermore, nitrate and phosphate are important for the optimum physiological functioning of coral symbionts and in determining coral reef resilience (Rosset et al., 2017) whereby their enrichment is thought to increase susceptibility to bleaching (Wiedenmann et al., 2013), compromise resilience, promote algal dominance over corals (Hall et al., 2018) and suppress calcification (Kinsey and Davies, 1979).
Materials and Methods
Study Sites
Sampling was done between March and April 2018 during the long rains season at three sites on the northern coast of Kenya: (1) Kuruwitu Conservancy, an LMMA administered “no-take” area since 2006, had the “strictest” protection safeguarded from all forms of consumptive utilization and human activities, save for research and tourism; (2) Malindi Marine Reserve, established in 1968 (Lambo and Ormond, 2006), was considered to be under “moderate” protection—the reserve is contiguous to Malindi Marine Park where it acts as a buffer to the “no-take” area. Here, artisanal fishing as well as research and tourism is allowed, but commercial forms of utilizations are prohibited (Tuda and Omar, 2012); and (3) Kilifi creek is not protected hence was designated the “least protected”—it is open to fishing, recreation, and experiences effluents from surrounding hotels and residential houses.
Sampling
Duplicate samples of 1L seawater each were collected in the morning between 08:00 and 12:00 at 1–2 meters depth at low tide within 15–20 cm of the dominant species of coral (Acropora spp.) 500–2,000 m from the shore. The samples were then transported on ice to the laboratory for processing within 2 h of sampling.
Laboratory Methods
Nitrate concentration was determined with ultraviolet spectrophotometry at 275 nm. Phosphate concentration was derived from orthophosphate colorimetric measurement at 690 nm using the Stannous Chloride method (APHA et al., 1999). Concentrations of viable E. coli cells were estimated by a three-tube most probable number (MPN) method as described by Vincy et al. (2015).
Results
E. coli concentrations decreased with increased levels of protection. At Kuruwitu, the site with the strictest protection, E. coli was not detected (<3 MPN/100 mL), whilst Malindi (moderate protection) recorded 16 MPN/100 mL and Kilifi (no protection) 29 MPN/100 mL. Between-site differences of E. coli density were not statistically significant (Figure 1).
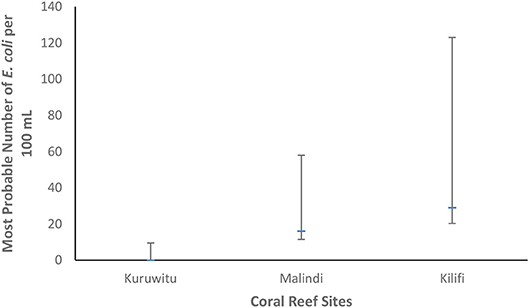
Figure 1. E. coli concentrations (MPN/100 mL) in water sampled at three different coral reef sites. Error bars indicate 95% confidence intervals.
Orthophosphate mean concentrations differed significantly among sites, increasing with the degree of marine protection (Table 1): the highest concentration, 0.524 mg/L, was observed at Kuruwitu followed by Malindi (0.422 mg/L) and Kilifi (0.326 mg/L) (P < 0.01). Nitrate mean concentrations also differed significantly among sites but did not correlate with the level of protection. Kuruwitu registered 0.566 mg/L, Kilifi 0.188, and Malindi, 1.402 (P < 0.01).
Discussion
The coral reef sites studied differed in both surrogates of human-source pollution investigated. As expected, E. coli concentrations decreased as the level of protection increased. Conversely, mean orthophosphate concentrations increased with increasing protection while mean nutrient concentration did not correlate with protection. These observations can be explained by the wide and differing range of human activities taking place around each study site, the level of marine protection and the proximity of each sampling site to a town.
In urban settings, wastewater and solid waste disposal is the principal cause of microbial pollution and nutrient loading (particularly nitrate) in the ocean (Wakida and Lerner, 2005; Okuku et al., 2011). The Kilifi site, which registered the highest E. coli and nitrate concentrations is a creek dividing two business centers, Mnarani and Kilifi towns, which together constitute the capital of Kilifi County. The creek is surrounded by resorts and residential houses leaking and/or disposing of wastewater into the ocean, and the beach is frequented by both foreign and local residents and holiday makers for recreation. In contrast, Malindi Marine Park and Kuruwitu Conservancy have limited, if any, exposure to urban pollution due to their distance from towns and levels of protection; the Malindi site (moderate protection) is about 10 km from the town, and Kuruwitu (highest protection) about 23 km from Kilifi town.
The Kuruwitu site shares a beach with several affluent residential homes with swimming pools, lush lawns utilizing fertilizers, as well as commercial farms. The conservancy administration reported of having had to seek the intervention of National Environmental Management Agency (NEMA) to stop residents from discharging swimming pools wastewaters directly into the ocean during the initiation phase of the LMMA. This suggests that the level of marine protection may also explain the variations in E. coli concentrations observed.
Agriculture-related activities are the main contributors of both orthophosphates and nitrate in rural settings (Coulter et al., 2004). We expected Malindi (where the Sabaki river meets the ocean) to contain higher mean nutrient concentrations than Kuruwitu since the Sabaki river runs through a catchment area with farming activities. However, the opposite was observed. Run-off from Kuruwitu lawns and commercial farms may explain the elevated orthophosphate and nitrate concentrations compared to Malindi where little or no farming occurs in the immediate vicinity of the sampling site. Furthermore, the Sabaki river has been shown to have the lowest discharge in the ocean during the north-eastern to south-eastern Monsoon transition period (March–April), which was when this study sampled. Most river sediment was deposited along the beach and intertidal area, and not in the neritic zone where this study sampled (Munyao et al., 2003). These observations support our results, assuming that nutrients are deposited in a similar manner as sediments.
There are no universal E. coli and nutrients references for tropical coral reefs but in comparison to local standards elsewhere, the levels observed in this study are of public and environmental health concern. Although the E. coli concentration was lower than the threshold allowable for bathing beaches in parts of Europe (Baudart et al., 2009), it may have been much higher at the shore close to the point where the pollutants entered the sea as bacterial numbers would decrease as they moved toward the open ocean due to tidal dilution and the bactericidal activity of seawater (Vaccaro et al., 1950). The nutrient concentrations assessed were also high, consistent with eutrophication (Okuku et al., 2011) or may have been the result of resuspended sediments (which accumulate nutrients at higher concentrations than seawater) following disturbances caused by rains and/or sampling activities. This finding is alarming and needs to be confirmed by a long-term follow-up study with supplementary data on the possible impact of the nutrient elevations on reef biodiversity.
This pilot study indicated that human activities around the Kenyan Coastal zone likely increase pollution that may impact coral reef health. An exact cause for the observed variations may not be conclusively specified yet, but the likelihood of marine protection in mitigating microbial pollution is suggested. This study, therefore, confirms the need to research the topic, and informs on critical areas of consideration in designing an effective study to definitively determine the role, if any, of MPAs in curbing marine pollution.
Data Availability
The datasets generated for this study are available on request to the corresponding author.
Author Contributions
AK, SdV, and SW contributed conception and design of the study. AK and SW performed sample collection and laboratory analysis. SW performed data analysis. AK wrote the first draft of the manuscript. SdV and SW wrote sections of the manuscript. All authors contributed to manuscript revision, read and approved the submitted version.
Conflict of Interest Statement
The authors declare that the research was conducted in the absence of any commercial or financial relationships that could be construed as a potential conflict of interest.
References
APHA AWWA, and AEF. (1999). Standard Methods for the Examination of Water and Wastewater, 20th Edn. Washington DC: American Public Health Association.
Baudart, J., Servais, P., De Paoli, H., Henry, A., and Lebaron, P. (2009). Rapid enumeration of Escherichia coli in marine bathing waters : potential interference of nontarget bacteria. J. Appl. Microbiol. 107, 2054–2062. doi: 10.1111/j.1365-2672.2009.04392.x
Coulter, C. B., Kolka, R. K., and Thompson, J. A. (2004). Water quality in agricultrual, urban, and mixed land use watersheds. J. Am. Water Resour. Assoc. 40, 1593–1601. doi: 10.1111/j.1752-1688.2004.tb01608.x
Fremaux, B. J., Gritzfeld, J., Boa, T., and Yost, C. K. (2009). Evaluation of host-specific bacteroidales 16S RRNA gene markers as a complementary tool for detecting fecal pollution in a prairie watershed. Water Res. 43, 4838–4849. doi: 10.1016/j.watres.2009.06.045
Hall, E. R., Muller, E. M., Goulet, T., Bellworthy, J., Ritchie, K. B., and Fine, M. (2018). Eutrophication may compromise the resilience of the red sea coral Stylophora pistillata to global change. Mar. Pollut. Bull. 131, 701–711. doi: 10.1016/j.marpolbul.2018.04.067
Hipsey, M. R., Antenucci, J. P., and Brookes, J. D. (2008). A generic, process-based model of microbial pollution in aquatic systems. Water Resour. Res. 44, 1–26. doi: 10.1029/2007WR006395
Hoegh-guldberg, O., Poloczanska, E. S., Skirving, W., and Dove, S. (2017). Coral reef ecosystems under climate change and ocean acidification. Front. Mar. Sci. 4:158. doi: 10.3389/fmars.2017.00158
Hughes, T. P., Baird, A. H., Bellwood, D. R., Card, M., Connolly, S. R., Folke, C., et al. (2003). Climate change human impacts and the resilience of coral reefs. Science 301, 929–934. doi: 10.1126/science.1085046
Hughes, T. P., Kerry, J. T., Álvarez-Noriega, M. Á. R., Jorge, G., Anderson, K. D., Baird, A. H., et al. (2017). Global warming and recurrent mass bleaching of corals. Nature 543, 373–377. doi: 10.1038/nature21707
Jickells, T. D. (1998). Nutrient biogeochemistry of the coastal zone. Science 281, 217–221. doi: 10.1126/science.281.5374.217
Kilinc, B., and Besler, A. (2014). The occurrence of enteric bacteria in marine environment and pollution. Mar. Sci. Technol. Bull. 3, 39–43. Available online at: http://www.masteb.com/archive/2014-volume-3-issue-2/2014-volume-3-issue-2-page-39-43/
Kinsey, D. W., and Davies, P. J. (1979). Effects of elevated nitrogen and phosphorus on coral reef growth. Limnol. Oceanogr. 24, 935–940. doi: 10.4319/lo.1979.24.5.0935
Lambo, A. L., and Ormond, R. F. (2006). Continued post-bleaching decline and changed benthic community of a Kenyan coral reef. Mar. Pollut. Bull. 52, 1617–1624. doi: 10.1016/j.marpolbul.2006.05.028
Moberg, F., and Folke, C. (1999). Ecological goods and services of coral reef ecosystems. Ecol. Econ. 29, 215–233. doi: 10.1016/S0921-8009(99)00009-9
Munyao, T. M., Tole, P., and Jungerius, P. D. (2003). Sabaki River Transport and Deposition in the Indian Ocean. Recent Advances in Coastal Ecology, Studies From Kenya. African Studies Centre, 119–132.
Neumann, B., Vafeidis, A. T., Zimmermann, J., and Nicholls, R. J. (2015). Future coastal population growth and exposure to sea-level rise and coastal flooding - a global assessment. PLoS ONE 10:e0118571. doi: 10.1371/journal.pone.0118571
Obura, D. (2012). The diversity and biogeography of Western Indian Ocean reef-building corals. PLoS ONE 7:e45013. doi: 10.1371/journal.pone.0045013
Obura, D., Gudka, M., Bijoux, J., Freed, S., Gian, S. B., Maharavo, J., et al. (2017). Coral Reef Status Report for the Western Indian Ocean. Global Coral Reef Monitoring Network (GCRMN)/International Coral Reef Initiative (ICRI), 140.
Okuku, E. O., Ohowa, B., Mwangi, S. N., Munga, D., Kiteresi, L. I., Wanjeri, V. O., et al. (2011). Sewage pollution in the coastal waters of Mombasa City, Kenya: a norm rather than an exception. Int. J. Environ. Res. 5, 865–874. doi: 10.22059/IJER.2011.444
Ransom, K. P., and Mangi, S. C. (2010). Valuing recreational benefits of coral reefs: the case of mombasa marine national park and reserve, Kenya. Environ. Manage. 45, 145–154. doi: 10.1007/s00267-009-9402-9
Rosset, S., Wiedenmann, J., Reed, A. J., and D'Angelo, C. (2017). Phosphate deficiency promotes coral bleaching and is reflected by the ultrastructure of symbiotic dinoflagellates. Mar. Pollut. Bull. 118, 180–187. doi: 10.1016/j.marpolbul.2017.02.044
Samoilys, M. A., and Obura, D. O. (2011). Marine Conservation Successes in Eastern Africa. CORDIO Status Report 2011, 28.
Sayadi, M. H., Ahmadpour, N., Capoorchali, M. F., and Rezaei, M. R. (2016). Removal of nitrate and phosphate from aqueous solutions by microalgae: an experimental study. Glob. J. Environ. Sci. Manage. 2, 357–364. doi: 10.22034/GJESM.2016.02.04.005
Shahidul Islam, M., and Tanaka, M. (2004). Impacts of pollution on coastal and marine ecosystems including coastal and marine fisheries and approach for management: a review and synthesis. Mar. Pollut. Bull. 48, 624–649. doi: 10.1016/j.marpolbul.2003.12.004
Tuda, A., and Omar, M. (2012). Protection of marine areas in Kenya. George Wright Forum 29, 43–50. Available online at: http://www.georgewright.org/291tuda.pdf
Vaccaro, R. F., Briggs, M. P., Carey, L., and Ketchum, B. H. (1950). Viability of Escherichia coli in Sea water. Am. J. Public Health 40, 1257–1266. doi: 10.2105/AJPH.40.10.1257
Vincy, M. V., Brilliant, R., and Pradeepkumar, A. R. (2015). Prevalence of indicator and pathogenic bacteria in a tropical river of Western Ghats, India. Appl. Water Sci. 7, 833–844. doi: 10.1007/s13201-015-0296-9
Wakida, F. T., and Lerner, D. N. (2005). Non-agricultural sources of groundwater nitrate: a review and case study. Water Res. 39, 3–16. doi: 10.1016/j.watres.2004.07.026
West, J. M., and Salm, R. V. (2003). Resistance and resilience to coral bleaching: implications for coral reef conservation and management. Conserv. Biol. 17, 956–967. doi: 10.1046/j.1523-1739.2003.02055.x
Keywords: MPA (marine protected area), E. coli—Escherichia coli, nutrients (nitrogen and phosphorus), coral reefs, pollution
Citation: Kaimba A, de Villiers S and Wambua S (2019) Does Protection of Marine Areas Safeguard Coral Reefs From Human-Source Pollution? Front. Environ. Sci. 7:89. doi: 10.3389/fenvs.2019.00089
Received: 12 February 2019; Accepted: 27 May 2019;
Published: 14 June 2019.
Edited by:
Xiaoshou Liu, Ocean University of China, ChinaReviewed by:
Chun-hua Li, Chinese Research Academy of Environmental Sciences, ChinaWenzhe Xu, Tianjin University of Science and Technology, China
Copyright © 2019 Kaimba, de Villiers and Wambua. This is an open-access article distributed under the terms of the Creative Commons Attribution License (CC BY). The use, distribution or reproduction in other forums is permitted, provided the original author(s) and the copyright owner(s) are credited and that the original publication in this journal is cited, in accordance with accepted academic practice. No use, distribution or reproduction is permitted which does not comply with these terms.
*Correspondence: Sammy Wambua, sammywambua@gmail.com