- 1CDISE Department, Skolkovo Institute of Science and Technology, Moscow, Russia
- 2Department of Compositional Materials, Moscow Aviation Institute, Moscow, Russia
- 3Faculty of Soil Science, Lomonosov Moscow State University, Moscow, Russia
- 4Department of Biology, Pirogov Russian National Research Medical University, Moscow, Russia
- 5A.N. Severtsov Institute of Ecology and Evolution, Moscow, Russia
There is a growing awareness among farmers about the importance of soil for sustaining crop production and soil health. Marked interests in “environmentally-friendly” soil fertilization in agriculture has attracted significant attention in the last decades. A wide range of commercial humic products (HPs) are used as soil supplements to improve the nutrient utilization efficiency of plants as well as to increase crop production. However, the implications of increased HPs use for soil biology are being questioned, but a comprehensive review on this topic is lacking. The aims of this review are to understand behavior of HPs applied to soil, with a special focus on environmental protection issues, and to summarize the available data how HPs may influence the soil microbial communities, including fungi and bacteria strains. The interaction between HPs and soil inhabitants is highly complex and is controversial to a certain extent for several reasons. First, applying HPs may promote specific bacteria communities (Acidobacteria, Actinobacteria, Bacteriodeites, etc.) rather than other microbiome species. Soil enzymes such as laccase, peroxidase and dehydrogenase were seemingly affected as well. Applying HPs may result in the microbial respiration of CH4, preventing the production of CO2 from microbial respiration. These results would potentially lead to an overall decrease in the microbial respiration of CH4, which is associated with a deficit in CO2. Furthermore, HPs can control the nematode population in different soils. Finally, applying HPs in terms of physiological functioning can also have various consequences for different groups of soil fungi. However, the conditions that promote specific effects (chemical composition of HPs, environmental conditions, etc.) have yet to be investigated. Given the surveyed data, we conclude that there are gaps in the current knowledge of this topic. We propose an integrated approach including a targeted research involving not only species of plants but also the integrated chemical, toxicological and biological analyses as a useful approach of soil health protection.
Introduction
Healthy and fertile soil are fundamental for food security and agricultural sustainability, yet it remains a challenge due to poor management and environmental changes (Doran and Safley, 1997; Moebius-Clune, 2016). Increasingly, soils become degraded and marginal for production. This situation is exacerbated by the need to increase food production to feed an increasing global population. Consequently, there is a common interest in the development and implementation of sustainable agricultural practices (Quintero-Angel and González-Acevedo, 2018; Gazzola et al., 2019). Such practices need to maintain the ability of the soil to produce food, while also delivering other ecosystem services such as regulation and storage of nutrients and carbon.
Among many different carbon-based substances for soil health improvement, humic-based soil amendments are accessible due to the industrial request for efficient and environmentally friendly soil supplements. Nowadays, the HPs market size is estimated to cross 1 billion US$ by 2024 (Pulidindi and Pandey, 2017) because they are using worldwide as soil conditioners and in cropland agriculture (Olk et al., 2018). According to Billingham (2012) humic products (HPs) are materials “that are often sold as soil amendments with or without accompanying plant nutrients.” Industrial technologies of the production of HPs differ from research analytical techniques used for the isolation of humic substances (HS). In general, they are manufactured by alkaline extraction from various organic matter resources, including lignite, peat, composts and organic waste materials (Perminova et al., 2005), but the resulted HPs show various properties (Yakimenko and Terekhova, 2011; Yakimenko et al., 2018). Practically, commercial HPs usually contain between 15 and 85% of HS (Yakimenko and Terekhova, 2011; Lamar et al., 2014).
In the context of potential effects of HPs on living organisms, there is a promising concept of emergent properties. According to this, the complexity of HPs as a whole substances is essential for their performance in compare with information about their separate components (Yakhin et al., 2016). In plant science, HPs are considered belonging to plant biostimulants, which are defined as “any substance or microorganism applied in minor quantities to plants with the aim of enhancing nutrition efficiency, abiotic stress tolerance, and crop quality traits, regardless of nutrients content” (Calvo et al., 2014; Canellas et al., 2015; Shah et al., 2018). A later definition underlines that a biostimulant is “a formulated product of biological origin that improves plant productivity as a consequence of the novel or emergent properties of the constituents, and not as a sole consequence of the presence of known essential plant nutrients, such as plant growth regulators, or plant protective compounds” (du Jardin, 2015; Yakhin et al., 2016). The benefits of applying HPs to enhance crop productivity have been well documented in the literature (Albiach et al., 2001; Quilty and Cattle, 2011; Tan, 2014). The positive effect of HPs on plant growth is commonly associated not only with the direct interaction of HS molecules with the plant root (“hormone-like activity”) and the activation of physiological processes in the plant, but also through exhibiting multiple indirect effects. For example, HPs buffer pH, increase water retention and mobilize nutrients availability. In respect to plants, both stimulating and inhibiting effects of HPs are illustrated in some comprehensive reviews (Canellas and Olivares, 2014; Rose et al., 2014; Nardi et al., 2016; Shah et al., 2018). At the same time, HPs may be promising remediation agents to degraded lands, due their ability to improve physical, chemical and biological properties of soil (Piccolo and Stevenson, 1982; Khaled and Fawy, 2011; Pukalchik M. et al., 2017; Tregubova et al., 2017).
However, the general picture of HPs influencing soil health is neither unambiguously positive nor simple. Besides the effects on plant productivity, the ubiquitous use of HPs in agriculture may bring specific changes to the soil food chain and microbial community. Being biostimulants, HPs can provide either stimulating or toxic effects on microorganisms, fungi and soil fauna. When applied as soil amendments, HPs provide a certain influence on the biological activity of the soil as a whole. For example, Kulikova et al. (2005) emphasized that the complexity of HPs structure and the initial experimental conditions bring a broad range of observed biological effects, which may promote the toxicity of HPs. Understanding of their potential effects on soil biota is of particular importance for soil health since it is mostly affected as a result of the field application of HPs. Moreover, HPs can exert the dose-toxicity effect phenomenon, which would result from an increase in application rates and may induce soil toxicity on living organisms, thereby damaging the environment (Guo et al., 2019).
Overall, the issue of HPs safety with respect to soil health should not be dismissed, yet should always be considered when applying amendments to the HPs. The main objective of this literature review concerns the analysis of peer-reviewed data on the role of HPs in biological and ecotoxicological soil properties. The aim of the review is to understand the behaviors of HPs applied to the soil, with a special focus on the protection of soil health and key ecosystem health indicators.
Effects of Humic Products on Soil Biota
Effects on Soil Microbial Activity and Functional Characteristics of Soil
Soil heterotrophic organisms play a crucial role in decomposing the organic matter, retaining C and N in their biomass and releasing CO2, CH4, NO2 back to the atmosphere (Le Mer and Roger, 2001; Trumbore, 2006; Zhu et al., 2013). Due to their gradual decomposition kinetics, inputs of HPs continuously provide nutrients utilized by microbial communities (Murphy et al., 2004). For that reason, HPs tend to act as slow-release biostimulants for microorganisms. Table 1 provides selected examples of how HP impacts specific soil microbial and enzyme activities, with associated soil processes given and a link to the impact on soil health (Table 1).
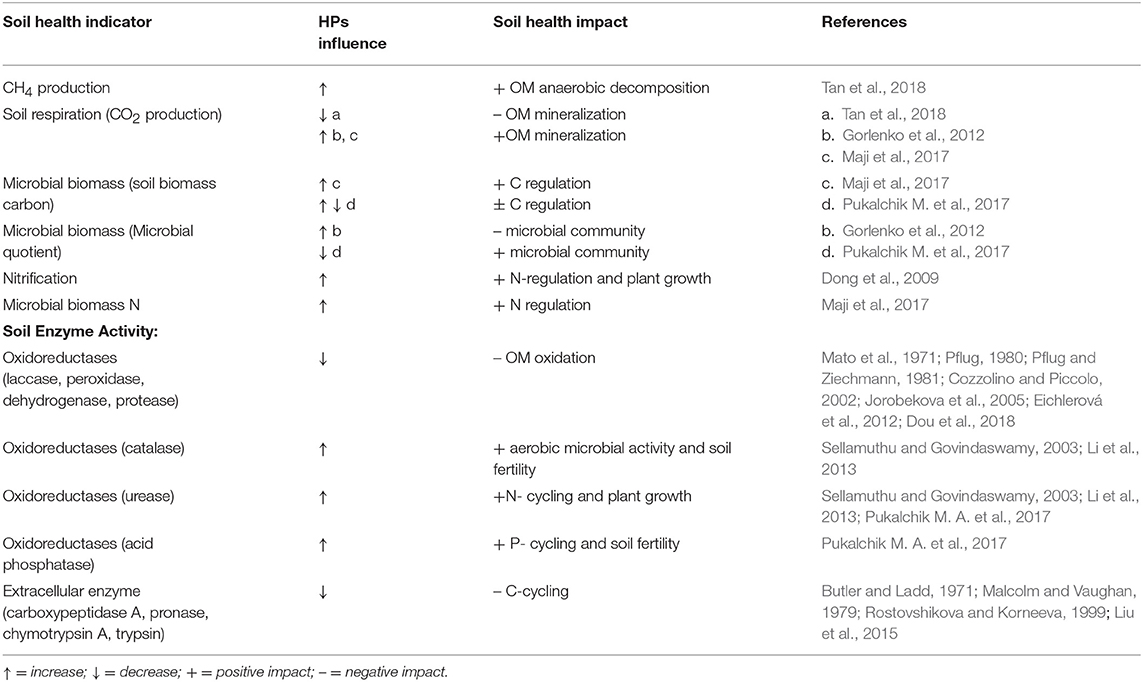
Table 1. Impacts of HPs on key biological processes in soil (C and N, enzyme activities) and functional effects on soil health.
The introduction of HPs in soils induced considerable cascading effects on soil C and N transformation processes. The mechanism of the influence of HPs may be linked with redox-active functional groups of HPs, which can be readily oxidized and subsequently act as electron donors for bacterial respiration, with subsequent changes in CO2 and CH4 production (Lovley et al., 1999; Coates et al., 2002). For example, Tan et al. (2018), following the application of three different HPs, highlighted CH4 emissions in paddy and wetland soils, thus decreasing CO2 emissions. According to those authors, that effect would account for not only for the ability of HPs to accept electrons but also trigger the anaerobic oxidation of CH4. Quite the opposite results were published by Gorlenko et al. (2012), where the application of 0.1, 0.5, and 1 g•kg−1 of commercial HPs, manufactured from brown coal, markedly increased emissions of CO2 from a model soil mixture. Only small doses of 0.1 g/kg HPs exhibited a positive trend for the soil microbial community, considering indicators such as biodiversity, metabolic activity, and stability of the soil microbial community. The highest applications induced a decrease in microbial biodiversity during the 45 day experiment. Maji et al. (2017) showed that applying HPs through enriched vermicompost (comprising 39.9% of HP, application dose is 9 g•kg−1 in soil) promoted soil CO2 respiration, microbial biomass carbon, and microbial biomass nitrogen values, unlike a traditionally used vermicompost and an NPK-fertilizer. Moreover, the highest rhizosphere fungal and bacterial populations were detected in the HPs-enriched vermicompost treatment. In our recent studies, we exploited Cu, Zn, Cd and Cr-contaminated soils in pilot greenhouse experiments and observed a positive short-term effect (0–60 days) produced by a commercial Na-humate (0.05, 0.5 and 1%) on a microbial metabolic quotient and microbial biomass carbon (Pukalchik M. et al., 2017).
The application of HPs has substantial impacts on soil biochemical processes, in particular on soil enzymes (see Table 1). The addition of HPs may affect the supply of C and N to plants and thus the processes of organic matter decomposition in soil. According to Wallenstein and Burns (2011), the following parts of microbial cells are mostly subjected to interactions with HPs: cytoplasm of microbial cells and the outer surface of the cells. The HPs may affect fungal spores, protozoal cysts and bacterial spores, which would constitute enzyme-substrate complexes and co-polymerized complexes with soil organic matter; they could subsequently be absorbed on the surface of clay minerals. Therefore, HPs can be involved in a plethora of processes and interactions within soil extracellular enzyme complexes.
On the one hand, HPs can be degraded by enzymes in the course of oxidative catalysis. In case the redox potential of laccases is too small (4,500–800 mv) to oxidize the C-C bonds in the HPs directly, HPs oxidize a variety of organic molecules in the soil, creating extracellular diffusible radicals which can depolymerize HPs (Brenelli et al., 2018). The redox potential of peroxidase is up to 1,490 mv. Moreover, peroxidases include Fe-containing groups that use H2O2 as an electron acceptor, enabling them to directly oxidize C-C bonds (Wang et al., 2012). Dehydrogenases that transfer hydride groups from a substrate to an acceptor such as NAD+ play a key role in the decomposition and mineralization of aromatic compounds (Bugg et al., 2011). The activity of these oxidative enzymes usually decreases upon addition of HPs. The reduction of laccase activity in the presence of HP was observed by Eichlerová et al. (2012); according to Dou et al. (2018), HP-induced inhibition may be linked with the oxidation of phenoxyl radicals. The peroxidase activity may be inhibited by (i) competitive interactions between the donor substrate NADH in peroxidase and HP (Pflug, 1980) and (ii) the fact that hydrogen bonds may confer conformational rigidity to humic associations and depress molecular reactivity (Cozzolino and Piccolo, 2002). The decrease in dehydrogenase activities was due to the competition of the forward reaction enzyme-HP (Pflug and Ziechmann, 1981). Applying HPs may also inhibit the activity of proteases in the soil through non-specific mechanisms. According to Jorobekova et al. (2005), enzyme-inhibitor complexes are formed by electrostatic, hydrophobic and other types of intermolecular interactions. A strong linear correlation between oxidase activities and the HPs phenolic content, as well as the C:H ratio and C and N content were shown by Mato et al. (1972). The recent data regarding the correlation between HPs and the activity of the soil enzyme pool suggested that HPs increased the catalase and urease activities in soil (Sellamuthu and Govindaswamy, 2003; Li et al., 2013). Our recent study, which involved a model of artificial soil and HPs of different origin, has also shown that HPs can impact the activities of acid phosphatase and urease while having no influence on soil dehydrogenase activity (Pukalchik M. A. et al., 2017).
On the other hand, HPs may be involved in specific mechanisms of binding reactions, which hamper access to the substrate for the enzymes active site. As a result, there was an observed decrease in activities of the different extracellular enzymes (Butler and Ladd, 1971; Vaughan and Ord, 1980; Allison, 2006). According to Butler and Ladd (1971), the content of carboxyl groups was responsible for carboxypeptidase A, pronase, chymotrypsin A and trypsin inhibitions in the soil. In case of phosphatase, a decrease in enzymatic activity did not correlate with C, H, and N ratios, and the content of ash and even carboxyl and phenol in groups (Malcolm and Vaughan, 1979). Still, low molecular weight components of HPs were particularly active (Mato et al., 1972; Nardi et al., 2007), while high molecular weight components had residual activity (Butler and Ladd, 1971). The data above evidence that molecular weight conditions influence the biological behavior of these substances. The inhibiting effect of HPs may be linked to hydrophobicity properties (Liu et al., 2015). The results on which the hypothesis was advanced had been published earlier by Rostovshikova and Korneeva (1999), who showed that molecular and hydrophobicity fractions of humic acids in HPs have the most considerable influence on enzyme hydrolysis.
Effects on Diversity of Soil Microbial Community
HP's amendments usually affect the bacterial community more than soil fungi or actinomycetes (Dong et al., 2009; Puglisi et al., 2009). In a series of column experiments in which the 16S rRNA gene hybridization method was used, Van Trump et al. (2011) showed that Acidobacteria, Actinobacteria, Bacteroidetes, Firmicutes, and Proteobacteria were greatly affected by the addition of HPs. In a recent study, Yuan et al. (2017) obtained similar results in a model based on experiment with biofilms from HPs on the electrodes. The added layer of HPs specifically promoted Proteobacteria, Firmicutes, and Bacteroidetes. According to Schoebitz et al. (2016), adding 15 g of HP from leonardite shale to blueberry seedlings growing in andosol (TOC 5.77 g•kg−1, pH 6.74) did not change the fungal communities in the rhizosphere, yet altered the bacterial population. The opposite effect was observed by Sellamuthu and Govindaswamy (2003), who examined the changes in the microbial community growing in a sandy clay loam soil. The soil had been amended with four rates (10, 20, 30, and 40 kg•ha−1) of K-enriched HP addition in a 30 day pot experiment sown with sugarcane. For all HP-treatments, no effect was recorded in the bacteria population, whereas there was a substantial increase in the total amount of fungi and actinomycetes. Interestingly, the two higher rates accentuated these effects; the total amount of fungi in the rhizosphere increased up to 150% with 30 kg•ha−1 HPs as compared to the control group, while the 30 kg•ha−1 HP-induced variable increased only 76%. This finding could be explained by just a few microbial groups (e.g., bacteria). Nevertheless, more research needs to be conducted in this area before forming any conclusions.
Effects on Soil Invertebrates
The application of HPs to soil systems also affects mesofauna. Among all the effects upon soil fauna, the interaction of earthworms and nematodes with HPs appears to be the best-studied. Earthworms evidently ingest HPs particles. The passage of HPs through the digestive tract of the earthworm Eisenia fetida and Eisenia andrei influenced the average molecular weights of the HPs, particularly causing them to decrease (Tikhonov et al., 2011). Other authors have proposed that HPs may successfully mitigate pollutant-induced toxicity on these species. Shen et al. (2015) used cultivated soil spiked with Ni (100 and 1 mg/kg) and deltamethrin, examining remediation activities of HPs (0, 0.5, 1, and 3%) on the E. fetida oxidative and DNA damages over the course of the 48 day test. Any increase in the density of the earthworm would lead to an improvement of soil health. The presence of HPs successfully mitigated the damage inflicted by nickel and deltamethrin on DNA, proteins and lipid membranes. However, the damage caused by the lipid membrane was prevented most effectively when the content of HPs was 0.5 or 1%, while damage to DNA was prevented most effectively with 3% of HP. However, the 3% of HPs also negatively affected lipid membranes of earthworms. In addition, He et al. (2017) detected a protective effect of HPs from Ni contamination of Enchytraeus crypticus in a model soil system. The most prominent decrease in sub-lethal concentration of Ni for E. cripticus was observed after 4, 7, and 14 days of exposure. The preceding authors proposed that HPs could mitigate and delay the disruption of ion-regulatory mechanisms (e.g., plasma Na+), contributing to reduced physiological stress. In a recent study, 0.1 and 1% of HPs successfully promoted the E. fetida survival rate and cocoon production in a 60 day greenhouse experiment (Pukalchik et al., 2018).
Data on the response of nematodes to HPs is limited. Some studies reported the possibilities of HPs to control nematode populations in soils for agriculture purposes to prevent plant roots. For instance, (Kesba and Al-Shalaby, 2008) tested commercial HPs with and without nutrients suppliers against the root-knot nematode in sandy loam, sandy and clay soils. They observed that HP supplemented with micro-elements (Fe, Mn, Cu) was notably better than pure HP and HP+NPK in reducing the number of surviving juveniles, achieving the highest percentage of nematode inhibition (49.2%). Jothi and Poornima (2017), reported that 2 g•kg−1 of HPs in soil reduced the nematode population 2-fold in a clay loam soil. Similar results were reported by Seenivasan and Senthilnathan (2017), who observed a marked drop of the nematode population Meloidogyne incognita. The soil density, egg population and reproduction rate dropped by 53.5–56.7%, 61.9–63.8%, and 55.7–56.6%, respectively, when exposed to the commercial humic product (0.04, 0.08, 0.2, and 0.4% in a sandy loam soil). This study highlighted that HPs can act as an effective agent for biological control. They also stated that HPs under the field conditions may cause drastic changes in the soil food web; the complexity of those processes require detailed investigations.
Effects of Humic Products on Pure Cultures of Bacteria and Fungi
Apart from the physical and chemical components, the soil system includes complex fungal and bacterial communities. As such, the biological effects of each species on the environment are difficult to specify. So that, artificial liquid media for growing individual species is usually used to elucidate the interaction between HPs and fungal and bacterial metabolisms, as well as the biological effects produced by HPs themselves (Crowther et al., 2018). Recently, some works analyzed the influence of HPs on pure cultures of microorganisms in artificial media have received attention while explaining interactions. Overall, HPs produce both beneficial and non-beneficial effects on the microorganisms isolated from soil habitats and cultivated on different artificial media, such as Czapek's medium with sugars (Tikhonov et al., 2010; Fedoseeva et al., 2018), potato dextrose agar (PDA) (Pascual et al., 2002; Loffredo et al., 2007, 2008; Loffredo and Senesi, 2009) and under other artificial conditions.
Humic products produce an stimulatory effect on microorganisms (Kirschner et al., 1999; Gryndler et al., 2005; Tikhonov et al., 2010; Kanaparthi and Conrad, 2015). Major direct effects of HP on microorganisms include the stimulation of biomass growth, which is due to the biosynthetic activity that regulates the metabolism of a cell (Kirschner et al., 1999; Kulikova et al., 2005; Tikhonov et al., 2010). The HPs stimulated the growth of the intestinal bacteria strains Agromyces spp., Aminobacter vorans, Arthrobacter spp., Bacillus spp, Kocuria palustris, Microbacterium sp., Nocardioides spp., Oxalobacter sp., Pseudomonas spp., Rhodococcus sp., Sphingopyxis spp., and Streptomyces spp. (Tikhonov et al., 2010). HPs from peat promoted the growth of hydrocarbon-oxidizing Acinetobacter sp. bacteria (Tikhonov et al., 2010). Kanaparthi and Conrad (2015) showed that HPs isolated from a lake sediment significantly enhanced the growth and activity of autotrophic, nitrate-dependent-oxidizing Thiobacillus sp. microorganisms. HPs extracted from soil stimulated root colonization by extra radical mycelium of the mycorrhizal fungus (Gryndler et al., 2005). The addition of HPs activate molecular signaling for the transition from growth forms to dormant forms (such as spores and cysts) in the growth media, while improving the development of bacteria in the soil (Stevenson et al., 2004). Furthermore, HPs could be utilized by humus-reducing microorganisms as terminal acceptors supporting their microbial growth (Zhao et al., 2017). The degree of decomposition of HPs positively correlated with the accumulation of fungal biomass (Fedoseeva et al., 2018).
A number of studies reported that HPs considerable inhibit the growth of different fungal and bacteria species (Pascual et al., 2002; Gryndler et al., 2005; Loffredo et al., 2007, 2008; Fedoseeva et al., 2009; Loffredo and Senesi, 2009) (Table 2). The HPs extracted from soil decreased the populations of saprophytic microorganisms, such as the fungi Paecilomyces lilacinus and an unidentified actinomycete in the cultivation system (Gryndler et al., 2005). To confirm those results, the mycelial growth and conidial germination of two forms of pathogenic Fusarium oxysporum were investigated in the soil and composts (Loffredo et al., 2007). Furthermore, the growth and sclerotial formation of pathogenic Sclerotinia sclerotiorum and two antagonistic Trichoderma species (Loffredo et al., 2008), as well as the mycelial growth of pathogenic stains from Pythium ultimum and F. oxysporum (Loffredo and Senesi, 2009), were assessed.
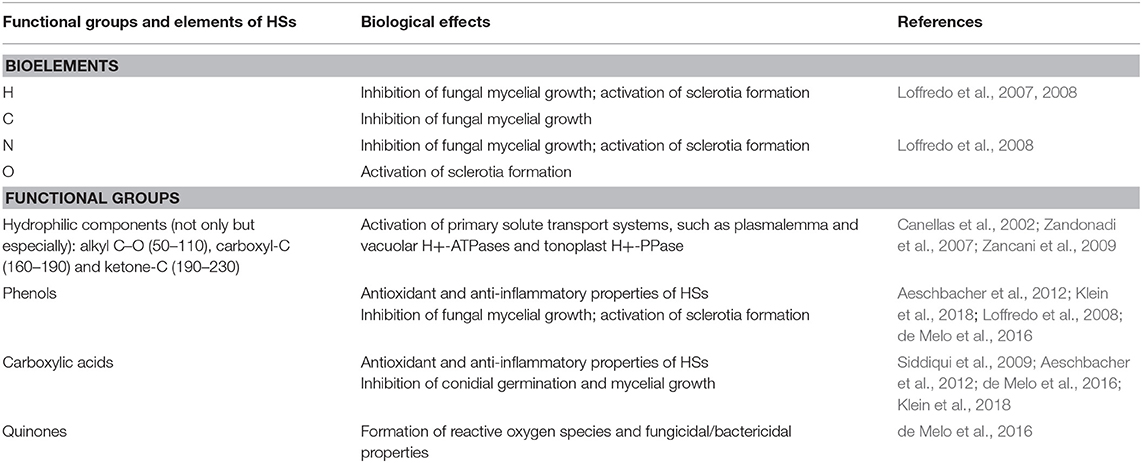
Table 2. Functional groups and elements of HPs and effects on biological characteristics of microorganisms.
In general, HPs exhibit a stimulatory effect on antagonistic species, such as those representatives of plant pathogens. However, the effect on T. harzianum was less sensitive than T. viride in the presence of HPs in the growth medium (Loffredo et al., 2007, 2008). Furthermore, A. alternata was sensitive to some HS types extracted from cambisols (Moliszewska and Pisarek, 1996). However, Loffredo et al. (2008) observed that various environmentally safe humic fractions and bioactive natural organic molecules should be considered as a possible alternative to the large number of inorganic and organic chemical fungicides that control the phytopathogenic fungi in the soil. Moreover, fungicide activity exhibited by HPs against phytopathogenic fungi Botrytis cinerea, Rhizoctonia cereals, Fusarium graminearum, and Phytophthora infestans was confirmed with field research (Wu et al., 2016). However, should be considers as an alternative, Fedoseeva et al. (2017) found that sporulation of phytopathogenic A. alternata was stimulated by HP from lignosulfonate. A wide variety of the observed effects may account for the multiple functions of HPs and the variability of soil microorganisms. Several mechanisms that are stimulated by HPs or inhibited by microorganisms are not fully understood, although a series of studies have shown the existence of a close connection between chemical and functional properties of HPs and the effects that they produce.
Quite often, artificial media fails to reproduce the endogenous abiotic and biotic conditions required for the microbial growth. However, this is a vital prerequisite for comprehensively studying the chemical, physical and biological processes of the environment where microbes live. It is important to apply this knowledge to the interaction of the aforementioned factors with the growth of microorganisms (Pham and Kim, 2012).
Probable Action Path-Ways of HPs at the Cellular Level
HPs can influence various cell components such as plasma membrane, mitochondria and DNA containing components. The physiological influence of HPs is determined by their heterogenic composition that vary according to the origin, process of obtainment, and functional groups present in their structures, such as quinones, phenols, carboxylic acids, and hydrophilic groups (Zancani et al., 2009; de Melo et al., 2016). The physiological influence of HPs on plant growth was correlated mostly with specific bioactive molecules content than with their size (Canellas et al., 2010). However, these authors mark that “molecules may be dynamically released from humic superstructures and exert their bioactivity weaker is the humic conformational stability as that obtained in the separated size-fractions.”
Some studies reveal that the response of certain physiological groups of HPs exhibit micellar-like properties and act as one kind of surfactants (Visser, 1985; de Melo et al., 2016; Xie et al., 2017). HPs could form micelle-like structures in neutral to acidic conditions due to their amphiphilic character (de Melo et al., 2016). According to Xie et al. (2017) the treatment of bacterial surfaces by the enhanced sorption properties of HAs promotes their interactions with bacteria. The core results demonstrated that the presence of humic acids significantly affected bacterial transport and deposition due to changes in the physicochemical properties of bacteria (Yang et al., 2016).
Quinones are responsible for the formation of reactive oxygen species in HPs, which are useful for wound healing and have fungicidal/bactericidal properties (de Melo et al., 2016). Furthermore, phenols and carboxylic acids are responsible for various other functions, such as antioxidant and anti-inflammatory properties of HSs (Aeschbacher et al., 2012; de Melo et al., 2016; Klein et al., 2018). In particular, the presence of phenolic groups in HPs provides antioxidant properties due to their free radical scavenging capacity (de Melo et al., 2016). Stress factors can induce excessive production of reactive oxygen species, resulting in the disintegration of lipid membrane components due to lipid peroxidation. Therefore, the protective effect of HSs is considered to be due to the presence of phenolic fragments, which provide antioxidant activity (Kulikova et al., 2018). This response of HSs appears to be comparable to those of natural reactive oxygen species-neutralizing antioxidants, such as flavonoids, phenolic acids and stilbenes (Fujita et al., 2006).
HPs are able to activate primary solute transport systems, such as plasmalemma and vacuolar H+-ATPases and tonoplast H+-PPase (Zandonadi et al., 2007). In the presence of HPs, and particularly the most hydrophilic fraction, other biochemical plant parameters were modified. In that study, Glu-6-P and ATP concentrations, SAP activity (evaluated as phytate hydrolysis), total cellular P and the basal oxygen consumption by suspension cells all increased (Zancani et al., 2009). HPs were found to promote cell energy as inducers of plasma membrane H+-ATPase synthesis (Canellas et al., 2002). This enzyme cleaves ATP molecules and generates the electrochemical gradient that provides energy to secondary cell transporters (Morsomme and Boutry, 2000).
Moreover, HPs can efficiently facilitate extracellular electron transfer with the participation of out-membrane cytochrome c (Cyt c), which interacts with humic acid via electrostatic or hydrogen-bonding interactions to form organic complexes (Tang et al., 2014). Additionally, as a ubiquitous biogeochemical, HPs can induce the DNA-methylation in the cladocerans (Menzel et al., 2011). DNA-methylation is one pathway of epigenetic programming of gene expression and can alter the stress response, presumably including exposure to synthetic xenobiotic chemicals.
Therefore, we can resume that, according to their heterogenic composition, HPs can express various properties at the cellular and organismal level (Table 2). Despite the contrasting data, it is evident that antibacterial and antifungal effects are more likely to have quinone groups. Numerous attempts have been done to reveal the structure-property-function relationship in humic substances to explain their biological activity (Muscolo et al., 2006; Garcia et al., 2016; Shah et al., 2018). However, a main driving force of the influence of HPs to different organisms are not revealed. Consequently, the contemporary concept of HPs as a biostimulants can better explain their effects on living organisms.
Conclusions
Soil is the main target for HP application, given their well-known role as soil supplements and plant biostimulants. Overall, the majority of papers reported a positive impact on crop yields when HPs applied at recommended field-application rates. Meanwhile, the other side of this coin is the unpredictable influence of HPs to soil health and non-targeted organisms, such as soil microorganisms and mesofauna. In general, most studies suggest that the impact of HP's application on soil biological indicators are minor and temporary (Figure 1). Here, we present comprehensive review about the influence of HPs on C and N cycling, enzyme activities and growth and reproduction of earthworm and nematode populations. Stimulatory effect on microbial biomass, as well as inhibitory effects on different fungal and bacteria species were also pointed. Although HPs can be grouped according to chemical composition, source of HPs and formulation process, this does not guarantee that they will have similar impacts on soil organisms. Finally, we support the idea to attribute HPs into the class of biostimulants and to recognize that “this classic reductionist biology/chemistry may indeed be insufficient to explain their biological complexity” (Yakhin et al., 2016). One of the main matter that have to be improved in the assessment of HPs is the development of a detailed procedure, which will include not only plant species, but also various indicators of soil quality and different bioassay tests (for example, invertebrates, soil bacteria and fungi, etc.).
Author Contributions
All authors contributed equally to the development and writing of the manuscript and MP led the writing team.
Conflict of Interest Statement
The authors declare that the research was conducted in the absence of any commercial or financial relationships that could be construed as a potential conflict of interest.
Acknowledgments
This research was funded by the Russian Science Foundation (grant # 18-74-00015). We also thank reviewers for critical review of this manuscript.
References
Aeschbacher, M., Graf, C., Schwarzenbach, R.P., and Sander, M. (2012). Antioxidant properties of humic substances. Environ. Sci. Technol. 46, 4916–4925. doi: 10.1021/es300039h
Albiach, R., Canet, R., Pomares, F., and Ingelmo, F. (2001). Organic matter components, aggregate stability and biological activity in a horticultural soil fertilized with different rates of two sewage sludges during ten years. Biores. Technol. 77, 109–114. doi: 10.1016/S0960-8524(00)00166-8
Allison, S. D. (2006). Soil minerals and humic acids alter enzyme stability: implications for ecosystem processes. Biogeochemistry 81, 361–373. doi: 10.1007/s10533-006-9046-2
Billingham, K. (2012). Humic Products - Potential or Presumption for Agriculture. Orange, NSW: NSW Dept Primary Industries.
Brenelli, L., Squina, F.M., Felby, C., and Cannella, D. (2018). Laccase-derived lignin compounds boost cellulose oxidative enzymes AA9. Biotechnol. Biofuel. 11:10. doi: 10.1186/s13068-017-0985-8
Bugg, T. D., Ahmad, M., Hardiman, E. M., and Singh, R. (2011). The emerging role for bacteria in lignin degradation and bio-product formation. Curr. Opin. Biotechnol. 22, 394–400. doi: 10.1016/j.copbio.2010.10.009
Butler, J., and Ladd, J. (1971). Importance of the molecular weight of humic and fulvic acids in determining their effects on protease activity. Soil Biol. Biochem. 3, 249–257. doi: 10.1016/0038-0717(71)90021-6
Calvo, P., Nelson, L., and Kloepper, J. W. (2014). Agricultural uses of plant biostimulants. Plant Soil 383, 3–41. doi: 10.1007/s11104-014-2131-8
Canellas, L. P., Fa3anha, A. O., Olivares, F. L., and Fa3anha, A. R. (2002). Humic acids isolated from earthworm compost enhance root elongation, lateral root emergence, and plasma membrane H+-ATPase activity in maize roots. Plant Physiol. 130, 1951–1957 doi: 10.1104/pp.007088
Canellas, L. P., and Olivares, F. L. (2014). Physiological responses to humic substances as plant growth promoter. Chem. Biol. Technol. Agr. 1:3. doi: 10.1186/2196-5641-1-3
Canellas, L. P., Olivares, F. L., Aguiar, N. O., Jones, D. L., Nebbioso, A., Mazzei, P., et al. (2015). Humic and fulvic acids as biostimulants in horticulture. Sci. Hortic. 196, 15–27. doi: 10.1016/j.scienta.2015.09.013
Canellas, L. P., Piccolo, A., Dobbss, L. B., Spaccini, R., Olivares, F. L., Zandonadi, D. B., et al. (2010). Chemical composition and bioactivity properties of size-fractions separated from a vermicompost humic acid. Chemosphere 78, 457–466. doi: 10.1016/j.chemosphere.2009.10.018
Coates, J. D., Cole, K. A., Chakraborty, R., O'Connor, S. M., and Achenbach, L. A. (2002). Diversity and ubiquity of bacteria capable of utilizing humic substances as electron donors for anaerobic respiration. Appl. Environ. Microbiol. 68, 2445–2452. doi: 10.1128/AEM.68.5.2445-2452.2002
Cozzolino, A., and Piccolo, A. (2002). Polymerization of dissolved humic substances catalyzed by peroxidase. effects of pH and humic composition. Org. Geochem. 33, 281–294. doi: 10.1016/S0146-6380(01)00160-7
Crowther, T. W., Boddy, L., and Maynard, D. S. (2018). The use of artificial media in fungal ecology. Fungal. Ecol. 32, 87–91. doi: 10.1016/j.funeco.2017.10.007
de Melo, B. A., Motta, F. L., and Santana, M. H. (2016). Humic acids: structural properties and multiple functionalities for novel technological developments. Mater. Sci. Eng. C Mater. Biol. Appl. 62, 967–974. doi: 10.1016/j.msec.2015.12.001
Dong, L., Córdova-Kreylos, A. L., Yang, J., Yuan, H., and Scow, K. M. (2009). Humic acids buffer the effects of urea on soil ammonia oxidizers and potential nitrification. Soil Biol. Biochem. 41, 1612–1621. doi: 10.1016/j.soilbio.2009.04.023
Doran, J. W., and Safley, M. (1997). “Defining and assessing soil health and sustainable productivity,” in Biological Indicators of Soil Health, eds C. Pankhurst, B. M. Doube, V. V. S. R. Gupta (Wallingford, NY: CAB International), 1–28.
Dou, R. -N., Wang, J. -H., Chen, Y. -C., and Hu, Y. -Y. (2018). The transformation of triclosan by laccase: effect of humic acid on the reaction kinetics, products and pathway. Environ. Pollut. 234, 88–95. doi: 10.1016/j.envpol.2017.10.119
du Jardin, P. (2015). Plant biostimulants: definition, concept, main categories and regulation. Scientia. Horticult. 196, 3–14. doi: 10.1016/j.scienta.2015.09.021
Eichlerová, I., Šnajdr, J., and Baldrian, P. (2012). Laccase activity in soils: Considerations for the measurement of enzyme activity. Chemosphere 88, 1154–1160. doi: 10.1016/j.chemosphere.2012.03.019
Fedoseeva, E., Patsaeva, S., and Terekhova, V. (2009). Effect of potassium humate on some of the physiological characteristics of microscopic fungi with different pigments. Micol. Phytopathol. 43, 243–250.
Fedoseeva, E., Stepanov, A., Yakimenko, O., Patsaeva, S., Freidkin, M., Khundzua, D., et al. (2018). Biodegradation of humic substances by microscopic filamentous fungi: chromatographic and spectroscopic proxies. J. Soils Sediments 19, 2676–2687. doi: 10.1007/s11368-018-2209-7
Fedoseeva, E. V., Terekhova, V. A., Khundzhua, D. A., Pukalchik, M. A., and Patsaeva, S. V. (2017). “Study of antibiotic and probiotic activities of humic preparationsto soil micromycetes,” in SUITMA 9. 9th international congress Soils of Urban Industrial Traffic Mining and Military Areas (Moscow: Urbanization: a challenge and an opportunity for soil functions and ecosystem services), 22–26, 294–297.
Fujita, M., Fujita, Y., Noutoshi, Y., Takahashi, F., Narusaka, Y., Yamaguchi-Shinozaki, K., et al. (2006). Cross talk between abiotic and biotic stress responses: a current view from the points of convergence in the stress signaling networks. Curr. Opin. Plant Biol. 9, 436–442. doi: 10.1016/j.pbi.2006.05.014
Garcia, A. C., de Souza, L. G. A., Pereira, M. G., Castro, R. N., Garcia-Mina, J. M., Zonta, E., et al. (2016). Structure-property-function relationship in humic substances to explain the biological activity in plants. Sci. Rep. 6:20798. doi: 10.1038/srep20798
Gazzola, P., Del Campo, A. G., and Onyango, A. (2019). Going green vs going smart for sustainable development: Quo vadis? J. Clean. Product. 214, 881–892. doi: 10.1016/j.jclepro.2018.12.234
Gorlenko, M. V., Yakimenko, O. S., Golichenkov, M. V., and Kostina, N. V. (2012). Functional biodiversity of soil microbe colonies affected by organic substrates of different kinds. Moscow University Soil Science Bulletin 67, 71–78. doi: 10.3103/S0147687412020032
Gryndler, M., Hršelová, H., Sudová, R., Gryndlerová, H., Rezáčová, V., and Merhautová, V. (2005). Hyphal growth and mycorrhiza formation by the arbuscular mycorrhizal fungus Glomus claroideum BEG 23 is stimulated by humic substances. Mycorrhiza 15, 483–488. doi: 10.1007/s00572-005-0352-7
Guo, X., Liu, H., and Wu, S. (2019). Humic substances developed during organic waste composting: formation mechanisms, structural properties, and agronomic functions. Sci. Total Environ. 662, 501–510. doi: 10.1016/j.scitotenv.2019.01.137
He, E., Qiu, H., Qiu, R., Devresse, Q., and Van Gestel, C. A. M. (2017). Time-dependent uptake and toxicity of nickel to Enchytraeus crypticus in the presence of humic acid and fulvic acid: effect of DOC on uptake and toxicity of Ni with time. Environ. Toxicol. Chem. 36, 3019–3027. doi: 10.1002/etc.3870
Jorobekova, S., Kydralieva, K., and Hudaybergenova, E. (2005). “Influence of metal ions on the activity of soil humic-enzyme complexes,” in Use of Humic Substances to Remediate Polluted Environments: From Theory to Practice. eds I. V. Perminova, K. Hatfield, N. Hertkorn (Berlin; Heidelberg: Springer-Verlag), 329–341.
Jothi, G., and Poornima, K. (2017). Potassium humate for the management of root knot nematode in tomato (Lycopersicon esculentum). J. Entomol. Zool. Stud. 5, 646–648
Kanaparthi, D., and Conrad, R. (2015). Role of humic substances in promoting autotrophic growth innitrate-dependent iron-oxidizing bacteria. Syst. Appl. Microbiol. 38, 184–188. doi: 10.1016/j.syapm.2015.02.009
Kesba, H. H., and Al-Shalaby, M. L. M. (2008). Survival and reproduction of meloidogyne incognita on tomato as affected by humic acid. Nematology 10, 243–249. doi: 10.1163/156854108783476304
Khaled, H., and Fawy, H. (2011). Effect of different levels of humic acids on the nutrient content, plant growth, and soil properties under conditions of salinity. Soil Water Res. 6, 21–29. doi: 10.17221/4/2010-SWR
Kirschner, R. A. Jr., Parker, B. C., and Falkinham, J. O. (1999). Humic and fulvic acids stimulate the growth of Mycobacterium avium. FEMS Microbiol. Ecol. 30, 327–332. doi: 10.1111/j.1574-6941.1999.tb00660.x
Klein, O. I., Kulikova, N. A., Filimonov, I. S., Koroleva, O. V., and Konstantinov, A. I. (2018). Long-term kinetics study and quantitative characterization of the antioxidant capacities of humic and humic-like substances. J. Soils Sediment. 18, 1355–1364. doi: 10.1007/s11368-016-1538-7
Kulikova, N. A., Filippova, O. I., and Perminova, I. V. (2018). Protective activity of humic substances in wheat seedlings in water deficit conditions. Moscow Univ. Soil Sci. Bull. 73, 76–80. doi: 10.3103/S0147687418020047
Kulikova, N. A., Stepanova, E. V., and Koroleva, O. V. (2005). Mitigating activity of humic substances: direct influence on biota. in Use of Humic Substances to Remediate Polluted Environments: From Theory to Practice, eds Perminova IV, Hatfield K, Hertkorn N (eds). Springer-Verlag, Berlin/Heidelberg, 285–309 doi: 10.1007/1-4020-3252-8_14
Lamar, R. T., Olk, D. C., Mayhew, L., and Bloom, P. R. (2014). A new standardized method for quantification of humic and fulvic acids in humic ores and commercial products. J. AOAC Internatl. 97, 721–730. doi: 10.5740/jaoacint.13-393
Le Mer, J., and Roger, P. (2001). Production, oxidation, emission and consumption of methane by soils: a review. Eur. J. Soil Biol. 37, 25–50. doi: 10.1016/S1164-5563(01)01067-6
Li, Y., Tan, W., and Koopal, L. K. (2013). Influence of soil humic and fulvic acid on the activity and stability of lysozyme and urease. Environ. Sci. Technol. 47, 5050–5056. doi: 10.1021/es3053027
Liu, K., Chen, Y., Xiao, N., Zheng, X., and Li, M. (2015). Effect of humic acids with different characteristics on fermentative short-chain fatty acids production from waste activated sludge. Environ. Sci. Technol. 49, 4929–4936. doi: 10.1021/acs.est.5b00200
Loffredo, E., Berloco, M., Casulli, F., and Senesi, N. (2007). In vitro assessment of the inhibition of humic substances on the growth of two strains of Fusarium oxysporum. Biol. Fertil. Soils 43, 759–769. doi: 10.1007/s00374-006-0160-z
Loffredo, E., Berloco, M., and Senesi, N. (2008). The role of humic fractions from soil and compost in controlling the growth in vitro of phytopathogenic and antagonistic soil-borne fungi. Ecotoxicol. Environ. Saf. 69, 350–357. doi: 10.1016/j.ecoenv.2007.11.005
Loffredo, E., and Senesi, N. (2009). In vitro and in vivo assessment of the potential of compost and its humic acid fraction to protect ornamental plants from soil-borne pathogenic fungi. Sci. Horticult. 122, 432–439. doi: 10.1016/j.scienta.2009.05.030
Lovley, D. R., Fraga, J. L., Coates, J. D., and Blunt-Harris, E. L. (1999). Humics as an electron donor for anaerobic respiration. Environ. Microbiol. 1, 89–98. doi: 10.1046/j.1462-2920.1999.00009.x
Maji, D., Misra, P., Singh, S., and Kalra, A. (2017). Humic acid rich vermicompost promotes plant growth by improving microbial community structure of soil as well as root nodulation and mycorrhizal colonization in the roots of Pisum sativum. Appl. Soil Ecol. 110, 97–108. doi: 10.1016/j.apsoil.2016.10.008
Malcolm, R. E., and Vaughan, D. (1979). Humic substances and phosphatase activities in plant tissues. Soil Biol. Biochem. 11, 253–259. doi: 10.1016/0038-0717(79)90070-1
Mato, M. C., Fábregas, R., and Méndez, J. (1971). Inhibitory effect of soil humic acids on indoleacetic acid-oxidase. Soil Biol. Biochem. 3, 285–288. doi: 10.1016/0038-0717(71)90037-X
Mato, M. C., Olmedo, M. G., and Méndez, J. (1972). Inhibition of indoleacetic acid-oxidase by soil humic acids fractionated on sephadex. Soil Biol. Biochem. 4, 469–473. doi: 10.1016/0038-0717(72)90062-4
Menzel, S., Bouchnak, R., Menzel, R., and Steinberg, C. E. W. (2011). Dissolved humic substances initiate DNA-methylation in cladocerans. Aqua. Toxicol. 105, 640–642. doi: 10.1016/j.aquatox.2011.08.025
Moebius-Clune, B. N. (2016). Comprehensive Assessment of Soil Health. The Cornell Framework Manual Cornell University
Moliszewska, E., and Pisarek, I. (1996). Influence of humic substances on the growth of two phytopathogenic soil fungi. Environ. Lnt. 22, 579–584. doi: 10.1016/0160-4120(96)00048-7
Morsomme, P., and Boutry, M. (2000). The plant plasma-membrane H-ATPase: structure, function and regulation. Biochim. Biophys. Acta. 1465, 1–16. doi: 10.1016/S0005-2736(00)00128-0
Murphy, D. V., Stockdale, E. A., Brookes, P. C., and Goulding, K. W. T. (2004). Impact of microorganisms on chemical transformations in soil. in Soil Biological Fertility, L. K. Abbott, D. V. Murphy, (eds). Springer Netherlands, Dordrecht, 37–59 doi: 10.1007/978-1-4020-6619-1_3
Muscolo, A., Sidari, M., Attinà, E., Francioso, O., Tugnoli, V., and Nardi, S. (2006). Biological activity of humic substances is related to their chemical structure. Soil Sci. Soc. Am. J. Abst. 71, 75–85 doi: 10.2136/sssaj2006.0055
Nardi, S., Muscolo, A., Vaccaro, S., Baiano, S., Spaccini, R., and Piccolo, A. (2007). Relationship between molecular characteristics of soil humic fractions and glycolytic pathway and krebs cycle in maize seedlings. Soil Biol. Biochem. 39, 3138–3146. doi: 10.1016/j.soilbio.2007.07.006
Nardi, S., Pizzeghello, D., Schiavon, M., and Ertani, A. (2016). Plant biostimulants: physiological responses induced by protein hydrolyzed-based products and humic substances in plant metabolism. Sci. Agric. 73, 18–23. doi: 10.1590/0103-9016-2015-0006
Olk, D. C., Dinnes, D. L., Scoresby, R., Callaway, C. R., and Darlington, J. W. (2018). Humic products in agriculture: potential benefits and research challenges—a review. J Soils Sediments 18, 2881–2891. doi: 10.1007/s11368-018-1916-4
Pascual, J. A., Garcia, C., Hernandez, T., Lerma, S., and Lynch, J. M. (2002). Effectiveness of municipal waste compost and its humic fraction in suppressing Pythium ultimum. Microb. Ecol. 44, 59–68. doi: 10.1007/s00248-001-0040-x
Perminova, I.V., Hatfield, K., and Hertkorn, N. (2005). Use of humic substances to remediate polluted environments: from theory to practice. Nato Sci. Series 52:506. doi: 10.1007/1-4020-3252-8
Pflug, W. (1980). Effect of humic acids on the activity of two peroxidases. Zeitschrift für Pflanzenernährung und Bodenkunde 143, 432–440. doi: 10.1002/jpln.19801430409
Pflug, W., and Ziechmann, W. (1981). Inhibition of malate dehydrogenase by humic acids. Soil Biol. Biochem. 13, 293–299. doi: 10.1016/0038-0717(81)90065-1
Pham, V. H., and Kim, J. (2012). Cultivation of unculturable soil bacteria. Trends Biotechnol. 30, 475–484. doi: 10.1016/j.tibtech.2012.05.007
Piccolo, A., and Stevenson, F.J. (1982). Infrared spectra of Cu2+, Pb2+ and Ca2+ complexes of soil humic substances. Geoderma 27, 195–208. doi: 10.1016/0016-7061(82)90030-1
Puglisi, E., Fragoulis, G., Ricciuti, P., Cappa, F., Spaccini, R., Piccolo, A., et al. (2009). Effects of a humic acid and its size-fractions on the bacterial community of soil rhizosphere under maize (Zea mays L.). Chemosphere 77, 829–837. doi: 10.1016/j.chemosphere.2009.07.077
Pukalchik, M., Mercl, F., Panova, M., Terekhova, V. A., and Tlustos, P. (2017). The improvement of multi-contaminated sandy loam soil chemical and biological properties by the biochar, wood ash, and humic substances amendments. Environ. Pollut. 229, 516–524. doi: 10.1016/j.envpol.2017.06.021
Pukalchik, M., Mercl, F., Terekhova, V.A., and Tlustoš, P. (2018). Biochar, wood ash and humic substances mitigating trace elements stress in contaminated sandy loam soil: evidence from an integrative approach. Chemosphere 203, 228–238. doi: 10.1016/j.chemosphere.2018.03.181
Pukalchik, M. A., Panova, M. I., Terekhova, V. A., Yakimenko, O. S., and Fedoseeva, E. V. (2017). Effect of humic preparations on the activity of soil enzymes in model experiment. Agrochemistry 8, 84–91. doi: 10.7868/S0002188117080105
Pulidindi, K., and Pandey, H. (2017). Humic Acid Market Size By Application (Agriculture, Ecological Bioremediation, Horticulture, Dietary Supplements), Industry Analysis Report, Regional Outlook (U.S., Canada, Germany, UK, France, Spain, Italy, China, India, Japan, Australia, Indonesia, Malaysia, Brazil, Mexico, South Africa, GCC), Growth Potential, Price Trends, Competitive Market Share & Forecast, 2017–2024
Quilty, J., and Cattle, S. (2011). Use and understanding of organic amendments in Australian agriculture: a review. Soil Res. 49, 1–26. doi: 10.1071/SR10059
Quintero-Angel, M., and González-Acevedo, A. (2018). Tendencies and challenges for the assessment of agricultural sustainability. Agricult. Ecosyst. Environ. 254, 273–281. doi: 10.1016/j.agee.2017.11.030
Rose, M. T., Patti, A. F., Little, K. R., Brown, A. L., Jackson, W. R., and Cavagnaro, T. R. (2014). A meta-analysis and review of plant-growth response to humic substances: practical implications for agriculture. Adv. Agron. 124, 37–89. doi: 10.1016/B978-0-12-800138-7.00002-4
Rostovshikova, I.N., and Korneeva, G.A. (1999). The role of humic substances in enzymatic destruction of organic matter. Biol. Bull. 26, 291–296
Schoebitz, M., López, M., Serrí, H., Martinez, O., and Zagal, E. (2016). Combined application of microbial consortium and humic substances to improve the growth performance of blueberry seedlings. J. Soil. Sci. Plant Nutr. 16, 1010–1023. doi: 10.4067/S0718-95162016005000074
Seenivasan, N., and Senthilnathan, S. (2017). Effect of humic acid on meloidogyne incognita (Kofoid & White) chitwood infecting banana (Musa spp.). Int. J. Pest. Manage 64, 1–9. doi: 10.1080/09670874.2017.1344743
Sellamuthu, K.M., and Govindaswamy, M. (2003). Effect of fertilizer and humic acid on rhizosphere microorganisms and soil enzymes at an early stage of sugarcane growth. Sugar Tech. 5, 273–277. doi: 10.1007/BF02942484
Shah, Z. H., Rehman, H. M., Akhtar, T., Alsamadany, H., Hamooh, B. T., et al. (2018). Humic substances: determining potential molecular regulatory processes in plants. Front Plant Sci. 9:263. doi: 10.3389/fpls.2018.00263
Shen, C. C., Shen, D. S., Shentu, J. L., Wang, M. Z., and Wan, M. Y (2015). Could humic acid relieve the biochemical toxicities and DNA damage caused by nickel and deltamethrin in earthworms (Eisenia foetida)? Environ. Sci. 17, 2074–2081. doi: 10.1039/C5EM00288E
Siddiqui, Y., Meon, S., Ismail, R., Rahmani, M., and Ali, A. (2009). In vitro fungicidal activity of humic acid fraction from oil palm compost. Int. J. Agric. Biol. 11, 448–452.
Stevenson, B. S., Eichorst, S. A., Wertz, J. T., Schmidt, T.M., and Breznak, J.A. (2004). New strategies for cultivation and detection of previously uncultured microbes. Appl. Environ. Microb. 70, 4748–4755. doi: 10.1128/AEM.70.8.4748-4755.2004
Tan, K.H. (2014). Humic Matter in Soil and the Environment: Principles and Controversies, Second Edition. Boca Raton, FL: CRC Press, Taylor & Francis Group.
Tan, W., Jia, Y., Huang, C., Zhang, H., Li, D., Zhao, X., et al. (2018). Increased suppression of methane production by humic substances in response to warming in anoxic environments. J. Environ. Manage 206, 602–606. doi: 10.1016/j.jenvman.2017.11.012
Tang, J., Liu, Y., Yuan, Y., and Zhou, S. (2014). Humic acid-enhanced electron transfer of in vivo cytochrome c as revealed by electrochemical and spectroscopic approaches. J. Environ. Sci. 26, 1118–1124 doi: 10.1016/S1001-0742(13)60518-5
Tikhonov, V., Byzov, B., Zavgorodnyaya, Y., and Demin, V. (2011). Earthworms as modifiers of the structure and biological activity of humic acids. Biol. Bull. 38, 14–24. doi: 10.1134/S1062359010061032
Tikhonov, V. V., Yakushev, A. V., Zavgorodnyaya, Y. A., Byzov, B. A., and Demin, V. V. (2010). Effects of humic acids on the growth of bacteria. Eurasian Soil Sci. 43, 305–313. doi: 10.1134/S1064229310030087
Tregubova, P. N., Koptsik, G. N., Stepanov, A. A., Smirnova, I. E., Kadulin, M. S., Turbaevskaya, V. V., et al. (2017). Application of humic substances in the remediation of heavy-metal-polluted soils of the subarctic zone of the kola peninsula. Moscow Univ. Soil Sci. Bull. 72, 207–214. doi: 10.3103/S0147687417050064
Trumbore, S. (2006). Carbon respired by terrestrial ecosystems - recent progress and challenges. Glob. Change Biol. 12, 141–153. doi: 10.1111/j.1365-2486.2006.01067.x
Van Trump, J. I., Wrighton, K. C., Thrash, J. C., Weber, A. K., Andersen, G., and Coates, J. (2011). Humic acid-oxidizing, nitrate-reducing bacteria in agricultural soils. mBio 2, e00044-11. doi: 10.1128/mBio.00044-11
Vaughan, D., and Ord, B.G. (1980). An effect of soil organic matter on invertase activity in soil. Soil Biol. Biochem. 12, 449–450. doi: 10.1016/0038-0717(80)90026-7
Visser, S. A. (1985). Physiological action of humic substances on microbial cells. Soil Biol. Biochem. 17, 457–462 doi: 10.1016/0038-0717(85)90009-4
Wallenstein, M. D., and Burns, R. G. (2011). “Ecology of extracellular enzyme activities andorganic matter degradation in soil: a complex community-driven process,” in Dick RP ed Methods of Soil Enzymology. ,Madison, WI: Soil Science Society of America), 35–55.
Wang, G., Post, W. M., Mayes, M. A., Frerichs, J., and Jagadamma, S. (2012). Parameter estimation for models of ligninolytic and cellulolytic enzyme kinetics. Soil Biol. Biochem. 48, 28–38. doi: 10.1016/j.soilbio.2012.01.011
Wu, M., Song, M., Liu, M., Jiang, C., and Li, Z. (2016). Fungicidal activities of soil humic/fulvic acids as related to their chemical structures in greenhouse vegetable fields with cultivation chronosequence. Sci. Rep. 6:32858. doi: 10.1038/srep32858
Xie, Y., Gu, Z., Hertath, H., He, C., Wang, F., Jiang, H., et al. (2017). Evaluation of bacterial biodegradation and accumulation of phenanthrene in the presence of humic acid. Chemosphere. 184, 482–488. doi: 10.1016/j.chemosphere.2017.06.026
Yakhin, O., Lubyanov, A. A., Yakhin, I. A., and Brown, P. H. (2016). Biostimulants in plant science: a global perspective. Front. Plant Sci. 7:2049. doi: 10.3389/fpls.2016.02049
Yakimenko, O. S., Khundzhua, D., Izosimov, A., Yuzhakov, V., and Patsaeva, S. V. (2018). Source indicator of commercial humic products: UV-vis and fluorescence proxies. J Soils Sediments 4, 1279–1291. doi: 10.1007/s11368-016-1528-9
Yakimenko, O. S., and Terekhova, V. A. (2011). Humic preparations and the assessment of their biological activity for certification purposes. Eurasian Soil Sci. 44, 1222–1230. doi: 10.1134/S1064229311090183
Yang, H., Ge, Z., Wu, D., Tong, M., and Ni, J. (2016). Cotransport of bacteria with hematite in porous media: effects of ion valence and humic acid. Water Res. 88, 586–594. doi: 10.1016/j.watres.2015.10.052
Yuan, Y., Cai, X., Wang, Y., and Zhou, S. (2017). Electron transfer at microbe-humic substances interfaces: electrochemical, microscopic and bacterial community characterizations. Chem. Geol. 456, 1–9. doi: 10.1016/j.chemgeo.2017.02.020
Zancani, M., Petrussa, E., Krajnáková, J., Casolo, V., Spaccini, R., Piccolo, A., et al. (2009). Effect of humic acids on phosphate level and energetic metabolism of tobacco BY-2 suspension cell cultures. Environ. Exp. Botany 65, 287–295. doi: 10.1016/j.envexpbot.2008.09.012
Zandonadi, D. B., Canellas, L. P., and Fac, A. R. (2007). Indolacetic and humic acids induce lateral root development through a concerted plasmalemma and tonoplast H+ pumps activation. Planta. 225, 1583–1595 doi: 10.1007/s00425-006-0454-2
Zhao, X., He, X., Xi, B., Gao, R., Tan, W., Zhang, H., et al. (2017). Response of humic-reducing microorganisms to the redox properties of humic substance during composting. Waste Manage. 70, 37–44. doi: 10.1016/j.wasman.2017.09.012
Keywords: humic substances, microbial activity, mesofauna, bacteria, fungi, bioassay, biodiversity, toxicity
Citation: Pukalchik M, Kydralieva K, Yakimenko O, Fedoseeva E and Terekhova V (2019) Outlining the Potential Role of Humic Products in Modifying Biological Properties of the Soil—A Review. Front. Environ. Sci. 7:80. doi: 10.3389/fenvs.2019.00080
Received: 18 December 2018; Accepted: 21 May 2019;
Published: 07 June 2019.
Edited by:
Luiz Fernando Wurdig Roesch, Federal University of Pampa, BrazilReviewed by:
Ademir Araujo, Federal University of Piauí, BrazilMaría Teresa Gómez-Sagasti, University of the Basque Country, Spain
Copyright © 2019 Pukalchik, Kydralieva, Yakimenko, Fedoseeva and Terekhova. This is an open-access article distributed under the terms of the Creative Commons Attribution License (CC BY). The use, distribution or reproduction in other forums is permitted, provided the original author(s) and the copyright owner(s) are credited and that the original publication in this journal is cited, in accordance with accepted academic practice. No use, distribution or reproduction is permitted which does not comply with these terms.
*Correspondence: Maria Pukalchik, M.Pukalchik@skoltech.ru; Pukalchik.Maria@gmail.com