- 1Institute of Bio- and Geosciences–Agrosphere (IBG-3), Forschungszentrum Jülich GmbH, Jülich, Germany
- 2Research Unit for Comparative Microbiome Analysis, Helmholtz Zentrum München, Neuherberg, Germany
- 3Key Laboratory of Arable Land Conservation (Middle and Lower Reaches of Yangtze River), Ministry of Agriculture, College of Resources and Environment, Huazhong Agricultural University, Wuhan, China
Nitrite () and hydroxylamine (NH2OH) are important intermediates of the nitrogen (N) cycle in soils. They play a crucial role in the loss of nitrous oxide (N2O) and nitric oxide (NO) from soil due to their high reactivity. In this study, we collected soil samples from three ecosystems (grassland, arable land, and forest with a riparian zone) and explored the contribution of and NH2OH to N2O formation in the different soils after exposure to oxic or anoxic pre-treatment. In addition, the importance of abiotic processes on the N2O formation from the two intermediates was studied by irradiating the soil samples with γ-irradiation. Our results demonstrate that addition induced the largest N2O production in the grassland soil, followed by the forest and arable soils. Only 9–39% of the produced N2O after addition came from abiotic processes. NH2OH addition increased N2O emissions the most from the arable soil, followed by the grassland and forest soils. The conversion of NH2OH to N2O was mostly (73–93%) abiotic. Anoxic pre-treatment decreased N2O production from NH2OH remarkably, especially for the grassland soil, while it increased N2O production from for most of the soils. Correlation analysis showed that effects on N2O production were strongly correlated to content in soils with anoxic pre-treatment, while NH2OH effects on N2O production were strongly correlated to soil Mn and C content in soils with oxic pre-treatment. Our results indicate that NH2OH plays an important role for abiotic N2O formation in soils with low C and high Mn content, while the effect of was important mainly during biotic N2O production. Anoxic periods prior to N addition may increase the contribution of , but reduce the contribution of NH2OH, to soil N2O formation.
Introduction
Nitrous oxide (N2O) is an important greenhouse gas that also contributes to the depletion of the ozone layer. Soils are the most important source of N2O, but the exact mechanisms responsible for N2O production in soils are not fully clarified yet. In general, nitrification, and denitrification are the two main source processes of N2O (Hu et al., 2015). These two pathways not only utilize enzymes that catalyze N2O production, but also provide substrates, e.g., hydroxylamine (NH2OH), nitrite (), and nitric oxide (NO), which can be released to the environment and form N2O chemically, i.e., so-called coupled biotic-abiotic N2O production (Liu et al., 2017a). Therefore, studying the biotic pathways and abiotic processes of N2O production based on NH2OH and are necessary for the understanding of N2O production mechanisms.
and NH2OH are important nitrification intermediates responsible for soil N2O production. Both are very reactive with relatively high self-decomposition rates dependent on soil pH, metals and organic matter. In oxic soils without fertilizer application and drought, is rarely accumulated due to the faster oxidation of to nitrate () than oxidation of ammonia (NH3) to during nitrification (Robertson and Groffman, 2007). However, high concentrations can be found after fertilizer application and drought (Ma et al., 2015; Homyak et al., 2016; Liu et al., 2018). The other reactive nitrification intermediate, NH2OH, is even more reactive and unstable in its natural environment (Butler and Gordon, 1986). At neutral or slightly alkaline pH, about 30% of NH2OH degrades within 3 h at room temperature in seawater samples at micromolar concentrations (Butler and Gordon, 1986). Nevertheless, NH2OH has been detected in cultures of ammonia oxidizers (Liu et al., 2017a) and heterotrophic nitrifiers (Daum et al., 1998), and acid forest soils (Liu et al., 2014).
For a long time, had been considered as a common compound for N2O production either via biological or abiotic processes. can be reduced biologically to N2O either by reductase through either “classical” denitrification or a pathway called “nitrifier denitrification” (Wrage et al., 2001), as well as biologically or chemically by Fe2+ with the help of iron oxidizers and other microorganisms (Kampschreur et al., 2011). Moreover, soil organic matter (SOM) can also react chemically with to form N2O (Stevenson and Swaby, 1964). However, the contribution of NH2OH to N2O formation has been neglected until recently, although it was shown that N2O can be formed both biologically by the enzyme NH2OH oxidoreductase (Ritchie and Nicholas, 1972) and chemically by O2 and several other soil oxidants (e.g., MnO2 and Fe3+) (Bremner, 1997; Heil et al., 2016).
Different soil preconditions may have a strong impact on biotic and abiotic N2O formation from and NH2OH in soil. For example, quality and quantity of dissolved organic matter (DOM) may have strong effects on N2O formation from NH2OH and . Soils rich in DOM, especially in phenolic lignin derivatives, may favor N2O formation from (Stevenson and Swaby, 1964; Wrage et al., 2001; Wei et al., 2017), but may decrease N2O formation from NH2OH, as NH2OH binds readily to carbonyl groups of organic matter to form oximes (Thorn et al., 1992; Liu et al., 2017b). Moreover, the content and oxidation state of transition metals may also affect the formation of N2O from and NH2OH. In soil samples with high Fe and Mn content, the oxidized form will promote the conversion of NH2OH to N2O, whereas under reduced conditions the formation of N2O from will be favored (Heil et al., 2016).
Therefore, in this paper, we compared the contribution of and NH2OH to N2O formation in the same soils. We collected soil samples from forest, grassland, and arable land with large ranges of C and Mn contents as well as pH. Oxic or anoxic pre-incubations were carried out. The effect of sterilization with γ-irradiation was scrutinized to quantify the relevance of abiotic processes. We hypothesized that (1) NH2OH plays a more important role in N2O production in soils with higher Mn and lower SOM content, whereas contributes more to N2O formation in soils with higher SOM and Fe content; (2) anoxic pre-incubation increases the contribution of to soil N2O formation, but decreases the contribution of NH2OH to soil N2O formation; (3) the contribution of NH2OH to N2O formation is mainly from abiotic processes, while there is a mixed contribution of biotic pathways and abiotic processes to N2O formation from .
Materials and Methods
Soil Collection
Soils were collected from three field sites of the Terrestrial Environmental Observatory (TERENO) (www.tereno.net) from the Eifel/Lower Rhine Valley, Germany, including a coniferous forest (Wüstebach; 50° 30′ 10″ N, 6° 19′ 50″ E), an extensive grassland (Rollesbroich; 50° 37′ 18″ N, 6° 18′ 15″ E) and an arable land (Selhausen; 50° 52′ 10″ N, 6° 27′ 4″ E). The coniferous forest site is located in the low mountain ranges of the Eifel National Park, 630 m above mean sea level, with a tributary of the river Rur basin flowing through it. The site is dominated by Norway spruce (Picea abies (L.) H. Karst). The hillslopes are characterized by Cambisol and Planosol, whereas the riparian zone is dominated by Gleysol and Histosol. The texture of soil in this forest is silty clay loam. The mean annual rainfall is about 1,400 mm, and the mean annual temperature is around 7°C. The vast majority of the precipitation occurred in the form of rain. The grassland site is located in the Northern Eifel region with smooth meadow grassland. Dominant soils at this site are (gleyic) Cambisol, Stagnosol, and Cambisol-Stagnosol with a silt loam texture. The climate is temperate maritime with a mean annual temperature and rainfall at the grassland site of 7.7°C and 1,033 mm, respectively, for the period from 1981 to 2000. The agricultural site is dominated also by (gleyic) Cambisol and (gleyic) Luvisol with a silt loam texture, and regularly cultivated with sugar beet, wheat, and oilseed rape, depending on the year. Mean annual temperature and rainfall at the cropland site are 9.8°C and 690 mm, respectively.
For grassland and arable land, mixed soil samples from five points for each site (~1.5 kg each) were collected from an area of 0.5 hectare from the top 15 cm in January 2016. Due to the strong spatial heterogeneity of soil properties at the forest site (Liu et al., 2016), six soil samples (~3 kg) were collected in January 2016 from the humus-rich layer (Oa horizon, depth 3–5 cm) of five sampling points (F1, F2, F3, F4, and F5) of the forest upland area and in addition from one sampling point of the forest riparian zone (FR) within an area of ~27 hectare of the forested Wüstebach catchment. The riparian zone represented only a small part of the forest, therefore we did not consider spatial heterogeneity of the riparian zone in contrast to the whole forest, and sampled only one point in the riparian zone area. Soil samples were transferred to the laboratory at the day of sampling. In the laboratory, fresh samples (except for the riparian zone sample) were passed through a 2-mm sieve, and coarse plant residues (including roots) and stones were manually removed.
Oxic and Anoxic Pre-treatment of Soil
For the anoxic pre-treatment, about 600 g fresh soil from each sampling site, stored at 4°C for 3 days after sampling in open plastic bags, was put into a 1 liter glass bottle and sealed with a rubber plug within a plastic lid. The gravimetric water content (w/w, water/dry soil) of the fresh soils was around 59–108% for the forest, 22% for the grassland, and 10% for arable land. The bottles were then evacuated for 10 min and refilled with helium to 0.4 bar overpressure. This procedure was repeated three times. Then the bottles were incubated with helium as headspace gas at ambient pressure at room temperature for 1 week. For the oxic pre-treatment, another ~600 g fresh soil was put in large open plastic bags and kept under oxic conditions at room temperature for 1 week. All plastic bags were stored in a large plastic box to reduce air flow and further reduce soil water evaporation. All soil samples were freeze-dried immediately after the oxic/anoxic pre-incubations to preserve the chemical status of the soil samples until further treatment. One side effect of freeze-drying could have been that this process led to a disruption of soil aggregates, which would have made more of the substrates soluble when the solution was added, and would have led to an overestimation of the N2O production from (via both biotic and abiotic pathways), but an underestimation of the N2O production from NH2OH (via abiotic processes). After freeze-drying, half of each oxic and anoxic pre-incubated soil was transferred to 50-ml falcon tubes and sterilized with γ-irradiation (Best Theratronics, Canada) for 14 h (total dose: 11 kGy), and the other half of each soil was kept in ziplock bags at room temperature (21 ± 1°C). The success of the sterilization process was checked by plating soil slurries after the sterilization on R2A medium and incubated for 24 h at 25°C. No growth of bacteria or fungi was observed (data not shown).
Addition of NH2OH and to Freeze-Dried Soils
About 1.4 g of freeze-dried soil with and without γ-irradiation were weighed into 22-ml gas chromatography (GC) vials (VWR International, Darmstadt, Germany), followed by the addition of either H2O, or and NH2OH solutions to reach around 40% water-holding capacity (WHC) and 1 μg N g−1 dry soil (for and NH2OH). The water was added to reactivate microbial activity (Morillas et al., 2015). The concentration in the freeze-dried soil was around 0–0.3 mg kg−1 dry soil. The added N amount corresponded to content in soil with fertilizer application (Shen et al., 2003; Venterea et al., 2003), and was assumed also realistic for NH2OH in soils with fertilizer application, as concentrations of 0.3–34.8 μg N kg−1 dry soil had been observed at this forest site (Liu et al., 2014). Solid MnO2 (Merck, Darmstadt, Germany) was added to the soil with NH2OH addition to explore the effect of MnO2 on NH2OH-to-N2O conversion in soil with both oxic and anoxic pre-treatment. The added Mn amount amounted to 0.1% (w/w) of soil dry weight, while the natural Mn content of the soil samples of this study ranged between 0.015 and 0.194% (w/w) (Table 1). Each treatment was carried out in triplicate. In total, there were 96 vials used for each soil. All vials were closed gas-tight immediately after addition of the N solution and MnO2 with butyl septa and aluminum crimp caps (VWR International). Half (48) of the vials were incubated aerobically at room temperature (21 ± 1°C) for 1 h, and the other half (48) were incubated aerobically for 7 h.
N2O Analysis
The gas in the headspace of the sample vials was analyzed for N2O using a gas chromatograph (Clarus 580, PerkinElmer, Rodgau, Germany) equipped with an electron capture detector (ECD) and flame ionization detector (FID) for N2O and CO2 detection, respectively. The N2O emission rate was calculated according to the following equation:
where E is the N2O emission (ng N g−1 dry soil); the factor two is used as a constant to the ratio of N2O-N to N2O; C' is the N2O mixing ratio in the vial headspace (nL L−1); V is the volume of vial headspace (L); Vm is the molar volume of N2O at standard pressure and room temperature (L mol−1); M is molar mass of nitrogen (g mol−1); Wds is the mass of the dry soil (g). The instrument was calibrated each day using five different standard gases with 0.25, 0.50, 0.75, 1.00, and 5.00 μL L−1 N2O, balanced with N2 (99.999% purity, Linde, Munich, Germany).
Soil Chemical Analyses
Chemical properties of the soils with oxic and anoxic pre-treatment were analyzed before the incubation. The elemental composition of the organic materials was analyzed by using inductively coupled plasma optical emission spectrometry (ICP-OES). Briefly, 100 mg of sample material were mixed with 3 ml HNO3 and 2 ml H2O2, heated in the microwave at 800 W for 30 min. The mixtures were subsequently filled up to 14 ml and diluted 10-fold with deionized water followed by the ICP-OES measurement. Total C and N contents were determined with an elemental analyzer (vario EL Cube, Elementar Analysensysteme GmbH, Langenselbold, Germany).
In addition, mineral N and the quality and quantity of soil DOM were analyzed to determine the effects of anoxic pre-treatment on the DOM dynamics. The mineral N ( and ) contents were analyzed with ion chromatography (ICS-3000 for , DX-500 for ; Dionex, Sunnyvale, CA, USA). and were extracted with 1 M KCl (dry soil: solution = 1:10 w/w) and shaken for 24 h. Soil pH was measured by shaking soil with 1 M KCl (dry soil: solution = 1:10 w/w). was extracted according to the method of Homyak et al. (2015) and measured by ion chromatography. NH2OH was extracted and measured according to the method of Liu et al. (2014). Dissolved organic carbon (DOC) and dissolved total nitrogen (DTN) were extracted with deionized water (dry soil: water = 1:2.5 for grassland and arable land soils, and 1:5 for forest and riparian soils) by shaking for 1 h at 200 rpm. DOC and DTN were then analyzed with a TOC-TN analyzer (Shimadzu Corp., Kyoto, Japan). In addition, for characterization of aromatic substances the absorbance of the DOC extract at 254 nm (A254) was determined with UV-VIS spectrometry (DU 800, Beckman Coulter, Inc., United States) and a path length of 1 cm.
Data Analyses
The effects of and NH2OH on N2O emission were calculated by subtracting N2O emission after water addition only (as control) from the N2O emission in response to and NH2OH addition. Contribution of abiotic processes to formation of N2O from NH2OH and was calculated as follows:
where R means the contribution (%) of abiotic conversion of NH2OH to N2O and of to N2O; E1′ (ng g−1) represents the N2O production after NH2OH or NO2- addition to soil after γ-irradiation; E0′ (ng g−1) represents the N2O production after H2O addition to soil after γ-irradiation; E1 (ng g−1) represents the N2O production after NH2OH or NO2- addition to soil without γ-irradiation; E0 (ng g−1) represents the N2O production after H2O addition to soil without γ-irradiation.
Analysis of variance (ANOVA) was used to test the main factors, i.e., soil type, oxic, and anoxic pre-treatment, N addition and γ-irradiation, and their interactions for significance (P < 0.05) of their effect on N2O production using the R software package (version 3.4.3). Box-Cox transformation of N2O data was performed before the ANOVA test. Fisher's Least Significant (P < 0.05) Difference test was used to test means of the effects for significant (P < 0.05) differences. Spearman's rank correlation analysis was performed between variables C, N, C/N, Fe, Mn, pH, DOC, DTN, A254, and for the oxic and anoxic pre-treatment using Origin Pro V. 2015.
Results
Effect of NH2OH and Addition on N2O Production in Different Oxic Pre-treated Soils
addition increased N2O production in the grassland soil significantly (P < 0.05), whereas it had only minor effect in the other soil samples (Figure 1A). In the grassland soil with oxic pre-treatment, 30.4% of the had been converted to soil N2O within 7 h, assuming that all the N2O came from the added . Even with water addition only, grassland soil which was pretreated under oxic conditions showed a strong rewetting effect, with a large N2O production of 512.1 μg N kg1 dry soil after 7 h (Table S1, supplementary information). For the forest soils, the N2O formation after addition amounted to about 40 μg N kg−1 dry soil after 7 h, which was 13% of the grassland soil N2O production. In general, the N2O production after addition from the riparian and arable soils after oxic pre-treatment was significantly (P < 0.05) lower than from the other soil samples.
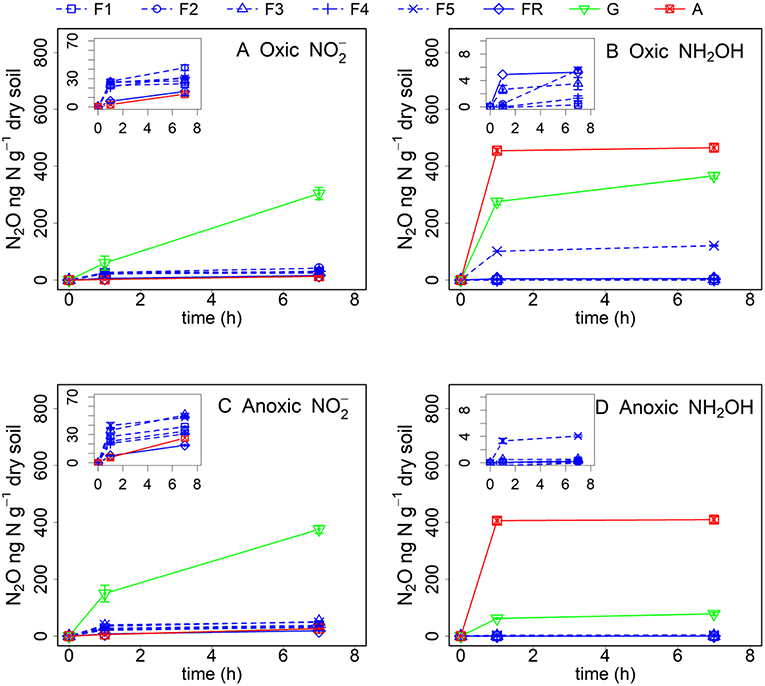
Figure 1. Net N2O (ng N g−1 dry soil) production in forest (F1, F2, F3, F4, F5, and FR), grassland (G) and arable land (A) soils without γ-irradiation after (A: oxic, C: anoxic pre-incubation) and NH2OH (B: oxic, D: anoxic pre-incubation) addition. Net N2O production was calculated by subtracting mean N2O emission values after addition of pure water (as control) from the N2O emission values after addition of or NH2OH solution. The values are presented as mean ± standard deviation (SD, n = 3).
In contrast, NH2OH addition induced the highest N2O production in the arable soil, followed by the grassland soil and the forest soil from sampling point F5 for soil samples with oxic pre-treatment. The conversion of NH2OH to N2O ranged from 12 to 47% for the arable, grassland and F5 forest soils within 7 h, assuming that all the N2O came from the added NH2OH. NH2OH addition had only a minor effect on the other forest soil samples during the whole incubation period (Figure 1B). Compared to the effect of , NH2OH had a larger effect on N2O production in the arable soil and in forest soil F5. Moreover, N2O was produced very quickly in the first hour after NH2OH addition.
Effect of Anoxic Pre-incubation on N2O Production From NH2OH and Addition
Anoxic pre-incubation increased soil concentration up to 7-fold, with the largest concentration (195 mg N kg−1 dry soil) in the grassland soil (Table 1), and decreased concentration in most of the soil samples (except forest sample F5) to nearly zero. The quality (reflected in the A254 value) and quantity of DOM (reflected in the concentrations of DOC and DTN) varied substantially after anoxic pre-incubation between the different soil samples, with 5–42% higher DOC content compared to soil samples with oxic pre-incubation. The A254 value followed a trend very similar to DOC, indicating that more aromatic substances were available in dissolved form after anoxic pre-incubation. The difference in DTN between the different treatments was not as pronounced as for DOC and A254.
Anoxic pre-treatment of the soil samples had a significant (P < 0.05) effect on soil N2O emission after the addition of NH2OH and . For the grassland and arable soil, anoxic pre-incubation resulted in a significant (P < 0.05) increase of N2O production during the first hour after addition (Figure 1C, Table 2). For the forest soil, anoxic pre-incubation increased N2O production from samples F1, F3, F5, and FR after addition compared to the oxic pre-treatment, but decreased the effect of on N2O production in F2 and F4. In terms of NH2OH, anoxic pre-treatment had a negative effect on the N2O production after NH2OH addition in most of soil samples, especially in those with a large NH2OH effect after oxic pre-incubation, i.e., grassland and F5 forest soils, but had a relatively small, but significant (P < 0.05) effect on the N2O production after NH2OH addition in arable soil (Table 2). Anoxic pre-incubation decreased N2O production only by 12% in arable soil, but by 79% in grassland soil after NH2OH addition after 7 h of incubation (Figure 1D, Table 2).
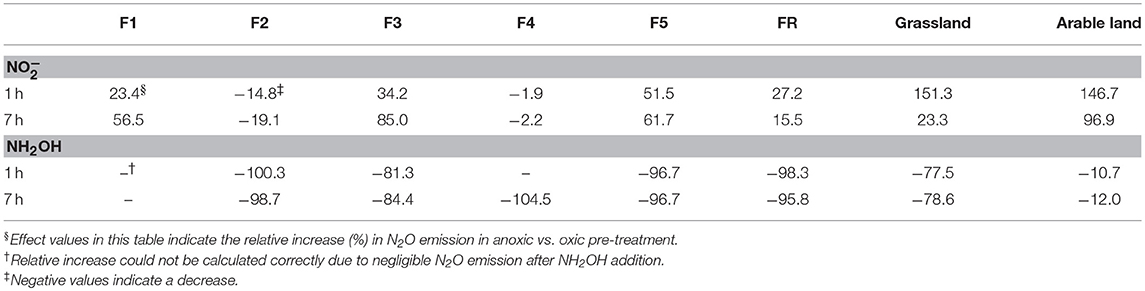
Table 2. Effect (%, n = 3) of anoxic pre-treatment on soil N2O emissions after 1 and 7 h of incubation of soils with and NH2OH additions.
Contribution of Abiotic Pathways to N2O Production From NH2OH and Addition
Abiotic pathways contributed to 9–39% of soil N2O production within 7 h after addition from the different soils after oxic pre-incubation, but contributed to 73–93% of soil N2O production within 7 h after NH2OH addition in the arable land, grassland and the F5 soil (Figure 2, Table 3). For the soil samples with anoxic pre-incubation, abiotic pathways contributed to 14–49% of -induced N2O production after 7 h, but contributed to 67–99% of N2O production only after NH2OH addition in the grassland, arable, and F5 soil in the same time period. In general, abiotic pathways played a more important role in N2O production after NH2OH addition compared to addition in the tested soils.
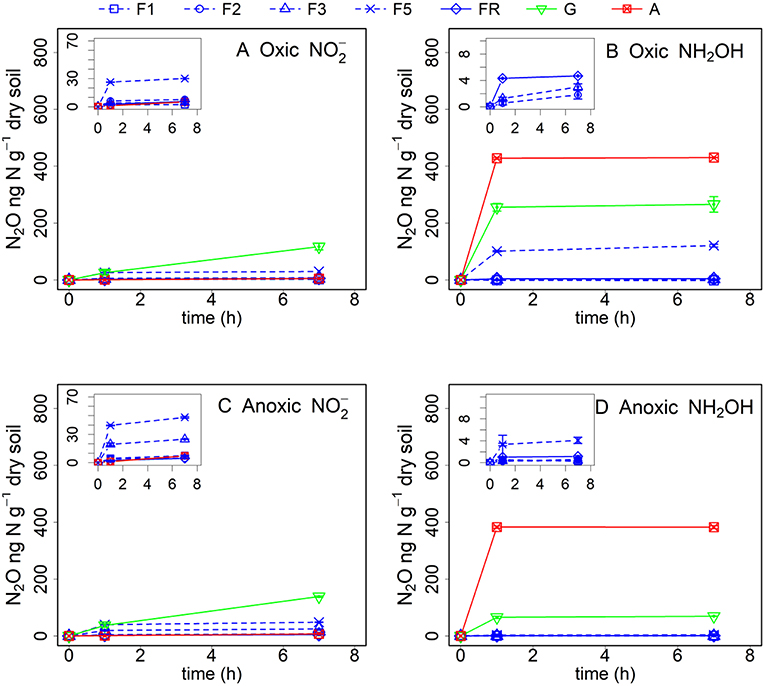
Figure 2. Net N2O (ng N g−1 dry soil) production in forest (F1, F2, F3, F5, and FR), grassland (G) and arable land (A) soils after γ-irradiation and (A: oxic, C: anoxic pre-incubation) and NH2OH (B: oxic, D: anoxic pre-incubation) addition. Net N2O production was calculated by subtracting mean N2O emission values after addition of pure water (as control) from the N2O emission values after addition of or NH2OH solution. The values are presented as mean ± standard deviation (SD, n = 3).
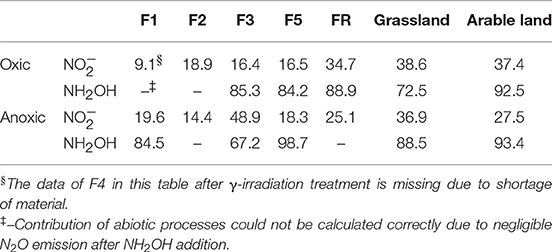
Table 3. Contribution (%, n = 3) of abiotic pathways to soil N2O emissions after 7 h incubation of soils with addition of aqueous solutions of NH2OH or to soil samples with oxic or anoxic pre-incubation and freeze-drying treatment.
Controlling Factors of N2O Production in Response to NH2OH and Addition to Soils After Oxic and Anoxic Pre-incubation
Correlation analysis showed that soil Mn, C, N, DOC, and A254 content as well as pH were important variables responsible for soil N2O formation from NH2OH in the tested soils (Table 4). The NH2OH-to-N2O conversion was positively and significantly correlated with soil Mn content and pH, but negatively and significantly correlated with soil C, N, and DOC content, and A254. Soil N2O production after addition was found to be significantly (r = 0.93, P < 0.05) correlated with content, and only marginally (r = 0.69, P = 0.06) correlated with soil Fe content after anoxic pre-treatment. No significant correlation was observed between the -to-N2O conversion and any soil properties after oxic pre-treatment.
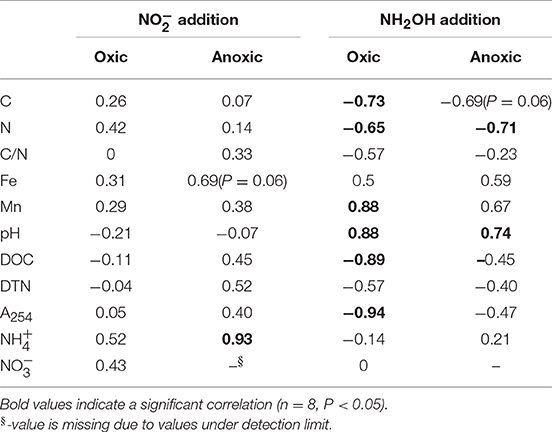
Table 4. Spearman's correlation coefficients between soil N2O emissions and soil properties after 7 h of incubation.
The addition of MnO2 increased the NH2OH-to-N2O conversion in all soil samples after oxic or anoxic pre-incubation (Figure 3). However, addition of MnO2 increased the NH2OH-to-N2O conversion more in the soil with oxic pre-incubation (especially during the first hour after NH2OH addition) compared to the soil with anoxic pre-incubation. Only N2O emission from soil F3 with anoxic pre-incubation was largely affected by the addition of MnO2 (as high as 2.5 mg kg−1 N after 7 h), which disappeared completely after γ-irradiation (data not shown). The NH2OH-to-N2O conversion of the grassland soil, F5 and other forest soil samples after anoxic pre-incubation also increased after MnO2 addition, but was still much lower than with NH2OH addition only after oxic pre-incubation.
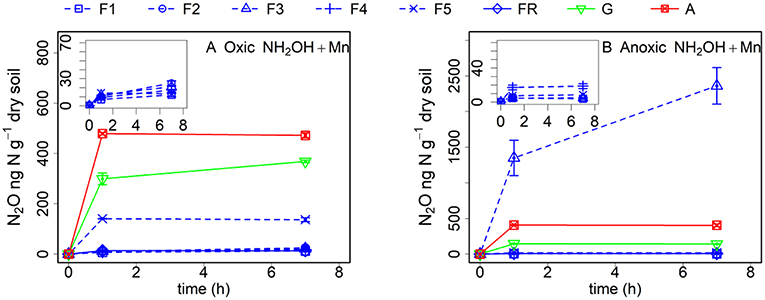
Figure 3. Soil N2O production (ng N g−1 dry soil) after MnO2 and NH2OH addition after oxic (A) and anoxic (B) pre-incubation for samples from forest (F1, F2, F3, F4, F5, and FR), grassland (G) and arable land (A) without γ-irradiation. The values are presented as mean ± standard deviation (SD, n = 3).
Discussion
The Importance of and NH2OH on Biotic and Abiotic N2O Formation in Different Soils
Our results showed that the role of and NH2OH in N2O production was strongly dependent on soil properties. The by far largest amount of N2O was produced in non-γ-irradiated grassland soil after addition, much higher than in all other soils (Figures 1A,C). About 30% of the added was converted to N2O in the grassland soil with oxic pre-incubation after 7 h of incubation, assuming that all the N2O produced came from the added . This large and quick N2O pulse could easily lead to the assumption of abiotic processes, e.g., chemodenitrification, being responsible for the N2O production upon addition of . However, γ-irradiation decreased the N2O production after addition to soil with oxic pre-incubation by 72% (Figure 2, Table 3), indicating that biotic pathways played a more important role in N2O production after addition compared to abiotic processes. It was reported that nitrifier denitrification involving biological reduction can play an important role in soil N2O emissions, especially in grassland and arable soil with large nitrifier activity (Wrage et al., 2001, 2004). Thus, the large pulse of N2O production with addition in the grassland soil could be due to nitrifier denitrification. In contrast, the smaller effect of addition on N2O production in forest soils was at first unexpected, as there was more carbon available in the forest soils than in the grassland soil for biotic (denitrification) and abiotic (chemodenitrification) pathways leading to N2O production. One possible reason responsible for the smaller N2O production in the forest soils could be that more NO instead of N2O was produced as it mostly decomposes to NO and NO2 at low pH (Davidson, 1992; Venterea et al., 2005). This assumption is supported by the fact that in our study the forest soil was acidic with pH values lower than 3.5 for most of the samples and more NO instead of N2O was produced in this forest site during our former research (Wei et al., 2017).
In contrast to , large N2O production was observed after NH2OH addition to the arable land, grassland, and the forest soil sample F5, and only negligible amounts of N2O were produced in the other forest soils (Figures 1B,D). Abiotic reactions played a much larger role in the case of NH2OH addition compared to addition. Comparison of the results from the experiments with γ-irradiated and non-irradiated soils revealed that most of the N2O from NH2OH was chemically produced, contributing 73–93% to the total conversion of NH2OH to N2O for the arable, F5 and grassland soil. We found larger Mn content in soils of the grassland, the arable land and the F5 forest sub-sample (Table 1), and a positive and significant correlation was observed between soil N2O production in response to NH2OH addition and Mn content (Table 4). This is in accordance with previous findings, which identified the chemical reaction between MnO2 and NH2OH as important factor for abiotic N2O production in soil (Bremner, 1997; Heil et al., 2015). The contradictory observation that the forest soil with the largest Mn content (F5) had a lower N2O production upon NH2OH addition than the grassland and cropland soils can be explained with the inhibitory effect of SOM on the abiotic conversion of NH2OH to N2O, as the effect of NH2OH on soil N2O emissions had been found earlier to be related to SOM quantity, quality and Mn content, with larger NH2OH-to-N2O conversion ratios in soils with higher Mn content and lower soil organic C content or, more specifically, lower content of carbonyl groups to which NH2OH could bind chemically (Liu et al., 2017b).
Effect of Soil Redox History on N2O Formation From and NH2OH
Despite their reactivity, the two N intermediates NH2OH and may accumulate in soils under anoxic conditions. NH2OH accumulation in anoxic sediment slurries has been observed in a preliminary experiment (Figure S1, supporting information), while transient accumulation as well as the absence of have been reported in soil slurries during anaerobic incubation (Clément et al., 2005). In the present study, the concentration, especially of the grassland soil, increased largely with anoxic pre-incubation (Table 1). Soil organic matter quality, transition metal redox state, and pH may change remarkably at this low redox potential (Dassonville and Renault, 2002). According to thermodynamic theory, the following sequential reduction of electron acceptors is observed with decreasing redox potential: O2, , MnO2, Fe2O3, , and CO2 reduction (Froelich et al., 1979) during respiratory or other dissimilatory processes. Thus, Fe2+, Mn2+, and fermented organic matter would accumulate during anoxic pre-incubation. Furthermore, the transient occurrence of reactive C substances could have reversed the effects of NH2OH and addition, as a transient increase in reactive C rich in carbonyl groups would preferentially react with NH2OH and decrease N2O production from abiotic conversion of NH2OH, while a transient increase in reactive phenolic compounds would lead to preferential reaction with to produce N2O chemically.
We hypothesized that anoxic pre-incubation would lead to higher N2O release after addition and less N2O release after NH2OH addition due to the accumulation of more reduced substances. Our results suggest that anoxic pre-incubation increased N2O production after addition in most of the soils. The stimulatory effect of anoxic pre-incubation on N2O production could be due to the increased contribution of N2O production via chemodenitrification, as more reduced transition metal ions, e.g., Fe2+, may accumulate after anoxic pre-incubation, which could have explained the observed very fast abiotic N2O production. However, since most of the N2O produced after addition came from biotic pathways, anoxic pre-incubation may have increased denitrification activity by stimulating denitrifier growth during the anoxic phase, leading to a positive effect of anoxic pre-incubation on N2O production via in soils.
Anoxic pre-incubation had an even more pronounced effect on N2O production after NH2OH addition, with a significant reduction of N2O production in the forest soil F5 and the grassland soil (97 and 79%, respectively), in accordance with our hypothesis, but with only a small effect (12%) on the arable soil after 7 h. As a strong oxidant, most of the MnO2 should have been reduced to Mn2+ during the anoxic pre-incubation period according to the large increase in (Table 1), which may indicate a soil redox potential that was lower than +200 mV (Froelich et al., 1979). In those soil samples with high C content, the organic carbon can be used by microorganisms that reduce Fe3+ or Mn4+ instead of oxygen (Lovley et al., 2004). The lower effect of the anoxic pre-treatment on the conversion of NH2OH to N2O in the arable soil could be attributed to the lower C content in this soil, where less Mn4+ would be reduced to Mn2+. To further explore the effect of MnO2 on the NH2OH-to-N2O conversion ratio, we added 0.1% (w/w) MnO2-Mn (equal to the Mn content of the grassland soil) to the soil samples with both oxic and anoxic pre-treatment. We hypothesized that this amount of MnO2 addition would increase the NH2OH-to-N2O conversion ratio of the soil samples pre-incubated under anoxic conditions. However, only the grassland soil and forest soils FR and F5 showed a larger Mn effect after anoxic pre-treatment (by comparing Figures 1D, 3B), but the added Mn amount could not make up the reduction in N2O production caused by the anoxic pre-incubation, despite the large increase of N2O production from F3 with anoxic pre-incubation (comparing Figures 1B, 3B).
It was reported that large amounts of fermented substances could accumulate during anoxic incubation (Dassonville and Renault, 2002). In the present study, we found more DOC and dissolved aromatic substances (represented as A254) in the soil samples with anoxic pre-incubation than with oxic pre-incubation (Table 1). The change of soil DOC quality and quantity could be responsible for the difference in N2O production after NH2OH addition to soils with different redox history, as the increase in DOC and aromatic substances after anoxic pre-incubation would increase the likelihood of fast binding of NH2OH to organic compounds once added to the soil, and lead to a lower availability of NH2OH for the reaction with MnO2 to produce N2O. Therefore, the absence of a MnO2 addition effect on the NH2OH-to-N2O conversion ratio could be due to the accumulation of fermented substances that can quickly react with NH2OH.
Conclusions
In summary, we show that the response of soil N2O production to the addition of the reactive intermediates NH2OH and of microbial N metabolism depends on the soil precondition, i.e., oxic vs. anoxic. The addition of increased N2O emissions mainly from biotic pathways, while the addition of NH2OH increased N2O from abiotic processes. Anoxic pre-incubation decreased N2O emissions in the NH2OH treatment, while it increased N2O emissions after addition. Soil properties, especially the DOM, Fe, and Mn content, have strong effects on the contribution of NH2OH and to N2O formation. This study emphasizes the higher importance of NH2OH for abiotic N2O production compared to in soils with high Mn content and less C content under oxic conditions, which may give useful information for the understanding of N2O formation mechanisms and prediction of N2O emission in such soils.
Author Contributions
NB and SL designed the experiment. SL and MS carried out the experiment and analyzed the data. NB, SL, MS, RH, and HV wrote the paper.
Conflict of Interest Statement
The authors declare that the research was conducted in the absence of any commercial or financial relationships that could be construed as a potential conflict of interest.
Acknowledgments
The authors wish to thank Daniela Gesekus and Volker Nischwitz for the analysis of soil basic properties and mineral N, and Diana Hofmann, Martina Krause, and Andrea Kubica for their assistance in the TOC/TN analysis. This study was supported by the Chinese Scholarship Council (scholarship no. 201206760007). This manuscript is based on the chapter six of a PhD thesis published in the online library of University of Bonn (Liu, 2017).
Supplementary Material
The Supplementary Material for this article can be found online at: https://www.frontiersin.org/articles/10.3389/fenvs.2019.00047/full#supplementary-material
References
Bremner, J. M. (1997). Sources of nitrous oxide in soils. Nutr. Cycl. Agroecosys. 49, 7–16. doi: 10.1023/A:1009798022569
Butler, J. H., and Gordon, L. I. (1986). An improved gas chromatographic method for the measurement of hydroxylamine in marine and fresh waters. Mar. Chem. 19, 229–243. doi: 10.1016/0304-4203(86)90025-3
Clément, J.-C., Shrestha, J., Ehrenfeld, J. G., and Jaffé, P. R. (2005). Ammonium oxidation coupled to dissimilatory reduction of iron under anaerobic conditions in wetland soils. Soil Bio. Biochem. 37, 2323–2328. doi: 10.1016/j.soilbio.2005.03.027
Dassonville, F., and Renault, P. (2002). Interactions between microbial processes and geochemical transformations under anaerobic conditions: a review. Agronomie. 22, 51–68. doi: 10.1051/agro:2001001
Daum, M., Zimmer, W., Papen, H., Kloos, K., Nawrath, K., and Bothe, H. (1998). Physiological and molecular biological characterization of ammonia oxidation of the heterotrophic nitrifier Pseudomonas putida. Curr. Microbiol. 37, 281–288. doi: 10.1007/s002849900379
Davidson, E. A. (1992). Pulses of nitric oxide and nitrous oxide flux following wetting of dry soil: an assessment of probable sources and importance relative to annual fluxes. Ecol. Bull. 42, 149–155.
Froelich, P. N., Klinkhammer, G. P., Bender, M. L., Luedtke, N. A., Heath, G. R., Cullen, D., et al. (1979). Early oxidation of organic matter in pelagic sediments of the eastern equatorial Atlantic: suboxic diagenesis. Geochim. Cosmochim. Acta 43, 1075–1090. doi: 10.1016/0016-7037(79)90095-4
Heil, J., Liu, S., Vereecken, H., and Brüggemann, N. (2015). Abiotic nitrous oxide production from hydroxylamine in soils and their dependence on soil properties. Soil Bio. Biochem. 84, 107–115. doi: 10.1016/j.soilbio.2015.02.022
Heil, J., Vereecken, H., and Brüggemann, N. (2016). A review of chemical reactions of nitrification intermediates and their role in nitrogen cycling and nitrogen trace gas formation in soil. Eur. J. Soil Sci. 67, 23–39. doi: 10.1111/ejss.12306
Homyak, P. M., Blankinship, J. C., Marchus, K., Lucero, D. M., Sickman, J. O., and Schimel, J. P. (2016). Aridity and plant uptake interact to make dryland soils hotspots for nitric oxide (NO) emissions. Proc. Natl. Acad. Sci. U.S.A. 113, E2608–E2616. doi: 10.1073/pnas.1520496113
Homyak, P. M., Vasquez, K. T., Sickman, J. O., Parker, D. R., and Schimel, J. P. (2015). Improving nitrite analysis in soils: drawbacks of the conventional 2 M KCl extraction. Soil Sci. Soc. Am. J. 79, 1237–1242. doi: 10.2136/sssaj2015.02.0061n
Hu, H. W., Chen, D., and He, J. Z. (2015). Microbial regulation of terrestrial nitrous oxide formation: understanding the biological pathways for prediction of emission rates. FEMS Microbiol. Rev. 39, 729–749. doi: 10.1093/femsre/fuv021
Kampschreur, M. J., Kleerebezem, R., de Vet, W. W. J. M., and van Loosdrecht, M. C. M. (2011). Reduced iron induced nitric oxide and nitrous oxide emission. Water Res. 45, 5945–5952. doi: 10.1016/j.watres.2011.08.056
Liu, S. (2017). Coupled Biotic-Abiotic Mechanisms of Nitrous Oxide Production in Soils During Nitrification Involving the Reactive Intermediates Hydroxylamine and Nitrite. [Dissertation]. [Bonn(IL)]: University of Bonn.
Liu, S., Berns, A. E., Vereecken, H., Wu, D., and Brüggemann, N. (2017b). Interactive effects of MnO2, organic matter and pH on abiotic formation of N2O from hydroxylamine in artificial soil mixtures. Sci. Rep. 7:39590. doi: 10.1038/srep39590
Liu, S., Han, P., Hink, L., Prosser, J. I., Wagner, M., and Brggemann, N. (2017a). Abiotic conversion of extracellular NH2OH contributes to N2O emission during ammonia oxidation. Environ. Sci. Technol. 51, 13122–13132. doi: 10.1021/acs.est.7b02360
Liu, S., Herbst, M., Bol, R., Gottselig, N., Pütz, T., Weymann, D., et al. (2016). The contribution of hydroxylamine content to spatial variability of N2O formation in soil of a Norway spruce forest. Geochim. Cosmochim. Acta 178, 76–86. doi: 10.1016/j.gca.2016.01.026
Liu, S., Schloter, M., and Brüggemann, N. (2018). Accumulation of NO2- during drying period stimulates soil N2O emissions by subsequent rewetting. Eur. J. Soil Sci. 69, 936–946. doi: 10.1111/ejss.12683
Liu, S., Vereecken, H., and Brüggemann, N. (2014). A highly sensitive method for the determination of hydroxylamine in soils. Geoderma 232–234, 117–122. doi: 10.1016/j.geoderma.2014.05.006
Lovley, D. R., Holmes, D. E., and Nevin, K. P. (2004). Dissimilatory Fe(III) and Mn(IV) Reduction. Adv. Microb. Physiol. 49, 219–286. doi: 10.1016/S0065-2911(04)49005-5
Ma, L., Shan, J., and Yan, X. (2015). Nitrite behavior accounts for the nitrous oxide peaks following fertilization in a fluvo-aquic soil. Biol. Fert. Soils. 51, 563–572. doi: 10.1007/s00374-015-1001-8
Morillas, L., Durán, J., Rodríguez, A., Roales, J., Gallardo, A., Lovett, G. M., et al. (2015). Nitrogen supply modulates the effect of changes in drying–rewetting frequency on soil C and N cycling and greenhouse gas exchange. Glob. Change Biol. 21, 3854–3863. doi: 10.1111/gcb.12956
Ritchie, G., and Nicholas, D. (1972). Identification of the sources of nitrous oxide produced by oxidative and reductive processes in Nitrosomonas europaea. Biochem. J. 126, 1181–1191. doi: 10.1042/bj1261181
Robertson, G., and Groffman, P. (2007). “Nitrogen transformations,” in Soil Microbiology, Ecology, and Biochemistry, 4th Edn. ed E. A. Paul (Burlington, MA: Academic Press), 421–446.
Shen, Q., Ran, W., and Cao, Z. (2003). Mechanisms of nitrite accumulation occurring in soil nitrification. Chemosphere 50, 747–753. doi: 10.1016/S0045-6535(02)00215-1
Stevenson, F., and Swaby, R. (1964). Nitrosation of soil organic matter: I. Nature of gases evolved during nitrous acid treatment of lignins and humic substances. Soil Sci. Soc. Am. J. 28, 773–778. doi: 10.2136/sssaj1964.03615995002800060027x
Thorn, K. A., Arterburn, J. B., and Mikita, M. A. (1992). Nitrogen-15 and carbon-13 NMR investigation of hydroxylamine-derivatized humic substances. Environ. Sci. Technol. 26, 107–116. doi: 10.1021/es00025a011
Venterea, R. T., Groffman, P. M., Verchot, L. V., Magill, A. H., Aber, J. D., and Steudler, P. A. (2003). Nitrogen oxide gas emissions from temperate forest soils receiving long-term nitrogen inputs. Glob. Change Biol. 9, 346–357. doi: 10.1046/j.1365-2486.2003.00591.x
Venterea, R. T., Rolston, D. E. E., and Cardon, Z. G. (2005). Effects of soil moisture, physical, and chemical characteristics on abiotic nitric oxide production. Nutr. Cycl. Agroecosys. 72, 27–40. doi: 10.1007/s10705-004-7351-5
Wei, J., Amelung, W., Lehndorff, E., Schloter, M., Vereecken, H., and Brüggemann, N. (2017). N2O and NOx emissions by reactions of nitrite with soil organic matter of a Norway spruce forest. Biogeochemistry 132, 325–342. doi: 10.1007/s10533-017-0306-0
Wrage, N., Velthof, G. L., Laanbroek, H. J., and Oenema, O. (2004). Nitrous oxide production in grassland soils: assessing the contribution of nitrifier denitrification. Soil Bio. Biochem. 36, 229–236. doi: 10.1016/j.soilbio.2003.09.009
Keywords: nitrification intermediate, reactive N, abiotic process, chemodenitrification, anoxic, γ-irradiation
Citation: Liu S, Schloter M, Hu R, Vereecken H and Brüggemann N (2019) Hydroxylamine Contributes More to Abiotic N2O Production in Soils Than Nitrite. Front. Environ. Sci. 7:47. doi: 10.3389/fenvs.2019.00047
Received: 18 January 2019; Accepted: 27 March 2019;
Published: 17 April 2019.
Edited by:
Alexandre J. Poulain, University of Ottawa, CanadaReviewed by:
Wenzhi Liu, Wuhan Botanical Garden, Chinese Academy of Sciences, ChinaPertti Juhani Martikainen, University of Eastern Finland, Finland
Copyright © 2019 Liu, Schloter, Hu, Vereecken and Brüggemann. This is an open-access article distributed under the terms of the Creative Commons Attribution License (CC BY). The use, distribution or reproduction in other forums is permitted, provided the original author(s) and the copyright owner(s) are credited and that the original publication in this journal is cited, in accordance with accepted academic practice. No use, distribution or reproduction is permitted which does not comply with these terms.
*Correspondence: Shurong Liu, shurongliu.edu@gmail.com
†Present Address: Shurong Liu, Division of Microbial Ecology, Department of Microbiology and Ecosystem Science, Centre for Microbiology and Environmental Systems Science, University of Vienna, Vienna, Austria