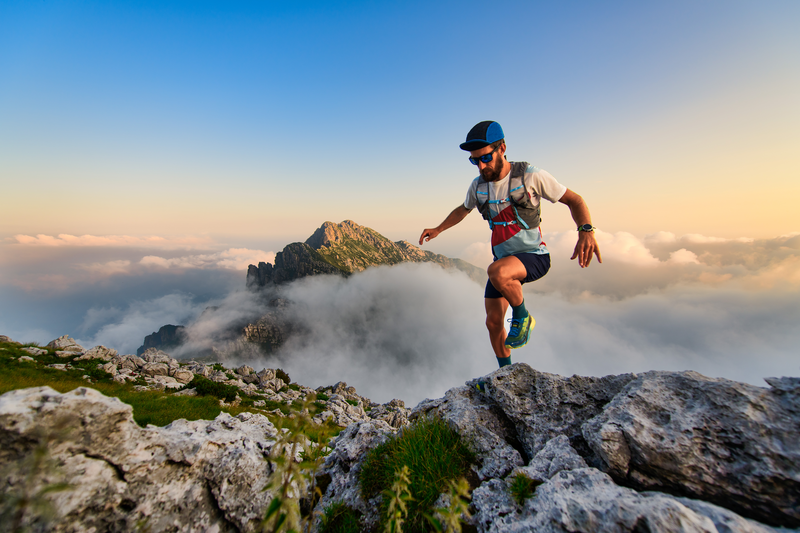
95% of researchers rate our articles as excellent or good
Learn more about the work of our research integrity team to safeguard the quality of each article we publish.
Find out more
ORIGINAL RESEARCH article
Front. Environ. Sci. , 01 February 2019
Sec. Freshwater Science
Volume 7 - 2019 | https://doi.org/10.3389/fenvs.2019.00009
This article is part of the Research Topic Aquatic Habitat Ecology & Conservation: Continental and Marine Ecosystems Connectivity View all 17 articles
The distribution of Andean freshwater fishes is the result of the interaction of historical and contemporary factors such as basin geomorphology and the physicochemical characteristics of water bodies. Dramatic changes along river networks due to waterfalls or dams generate abrupt changes in longitudinal slopes that function as ecological barriers to dispersal and thus have an effect on the composition and richness of fish assemblages. We expect the amount of variation in beta diversity along the elevation gradient (between 700 and 3,500 m a.s.l.) of the eastern slope of the Cauca River basin to be explained by changes in the aquatic environment and connectivity among sites. We measured connectivity in terms of the distance along the stream channel between sampling sites considering changes in slope. We used a Generalized Dissimilarity Model (GDM) to evaluate the contribution of connectivity and other water mass characteristics (dissolved oxygen, pH, conductivity, temperature, and elevation) in predicting changes in beta diversity. The GDM models explained 33% of the total deviance in species turnover, suggesting that there are additional variables that have not been considered, such as available habitats along the rivers. Elevation was the variable with the largest relative importance in the model and connectivity explained only seven percent of the total variance when all sites were included. However, when only the sampling sites in the headwater streams were included (the most geographically isolated sites), the GDM models explained 51% of the total deviance and the contribution due to connectivity increased. Isolation of stream headwaters, in conjunction with extreme conditions present at high elevations may influence the fish assemblage turnover. Our results provide evidence that elevation has a strong influence on beta diversity of Andean fish assemblages. Species turnover upstream above 1,200 m a.s.l. is strongly influenced by channel connectivity and there are additional environmental variables that need to be included in the models to improve their prediction power.
Freshwater fish represent nearly 10% of all vertebrate species in the world (Vari and Malabarba, 1998) and each continent has a distinctive fauna, which is the result of a long geological history of isolation due to physical barriers and the adaptation of each of the various groups to particular biotic and abiotic environmental conditions (Lévêque et al., 2008). The composition and structure of communities has also been associated with elevation gradients and changes in environmental factors along the cline. The pattern observed has been a decrease in species richness and increase of endemism with the increase in elevation (Lomolino, 2001). At a local scale, the composition of fish communities has been the result of these same conditions (Albert and Carvalho, 2011); however, their relative importance varies (Cilleros et al., 2016). In the South American Andes, for instance, studies on fish assemblages have identified elevational climatic gradients as the main drivers of change in composition and species richness (Pouilly et al., 2006; Jaramillo-Villa et al., 2010; Carvajal-Quintero et al., 2015; De La Barra et al., 2016). These analyses did not consider connectivity throughout the channel within a basin as a possible factor that could contribute to shaping the composition of fish assemblages.
The dendritic nature of fluvial networks creates preferential or obligatory connections for fish and aquatic taxa (Cote et al., 2009); thus, the spatial positioning of habitats within a river network could play a crucial role in the way communities are structured through processes of dispersal and environmental selection (Altermatt, 2013). Furthermore, longitudinal fragmentation, known as the natural discontinuity of fluvial networks, caused by natural waterfalls and rapids may act as ecological barriers which limit the process of fish dispersal and even promote speciation events in fragmented sub-drainages (Dias et al., 2013). As a result, the degree of connectivity within a given fluvial network should be an important mechanism that promotes speciation, extinction, and migration in evolutionary time-scales (Dias et al., 2013).
Both theoretical and empirical studies show that dispersal through dendritic systems exerts an influence on the spatial distribution of diversity (Altermatt, 2013). Evidence from fish communities at high elevations in the Andes suggests that headwater streams have different compositions despite having similar environments (Carvajal-Quintero et al., 2015). Therefore, the connectivity of the fluvial network could play an important role in the composition of these assemblages. With this in mind, this study analyzes the variation in the composition of fish communities in the Cauca River basin (northern Colombia's second most important river) considering the local environmental conditions along the elevational gradient, and also quantifies the spatial connectivity between fish assemblage locations.
Beta (β) diversity, defined as the temporal or spatial variation in species composition, provides a fundamental tool to quantify the distribution of diversity at a regional level, which has implications in ecosystem functioning and conservation (Socolar et al., 2016). β diversity may reflect two types of phenomena: spatial species turnover and community nestedness (Baselga, 2010). Nestedness occurs when the sites with less species richness are a subgroup of species that are present in successively richer sites, whereas turnover is when a species in a specific site is substituted by other species (Leprieur et al., 2011). This partition of β diversity into its independent components makes it possible to find suitable spatial conservation strategies that cannot be identified through the analysis of general β diversity (Angeler, 2013). For example, if the largest part of β diversity refers to nested communities, this would allow for the prioritization of a small number of sites with more species, whereas a high turnover would require the conservation of a greater amount of sites, though not necessarily the richest (Baselga, 2010; Guareschi et al., 2015; Loiseau et al., 2017).
Understanding the effects of the local abiotic conditions in conjunction with connectivity in dendritic systems at the local and regional levels is necessary in order to have a better grasp on ecosystem ecology and how it can be managed effectively (Barletta et al., 2010). With this in mind, in this study we seek to (i) quantify β diversity and its two components (turnover and nestedness) amongst local fish communities, and (ii) determine the contribution of fluvial connectivity, elevation, and physicochemical water conditions to explain the variation in β diversity of the fish fauna along the Cauca River basin in the northwestern Andes. We expect species turnover to be determined largely by changes in elevation gradient as has been reported by other authors (Carvajal-Quintero et al., 2015; De La Barra et al., 2016), and that fluvial connectivity and water conditions complement the importance of elevation as a predictor of species turnover amongst Andean fish assemblages. Finally, we expect a special importance of connectivity for headwater fish assemblages that are the most isolated and often with a highly differentiated biota (Albert and Carvalho, 2011; Carvajal-Quintero et al., 2015).
Colombia is a topographically diverse country, where the western region is predominantly mountainous as the Andes subdivide into three mountain ranges separated by two valleys through which the Magdalena and Cauca rivers run. Our study takes place in the Cauca River basin, specifically between 4°47′54″-5°32′21″N and 75°59′53″-75°16′34″W (Figure 1A). This region is characterized by a bimodal rainfall pattern and is made up of numerous valleys, plateaus, and smaller mountain ranges which result in varying climates ranging from temperate in the mountains to warm, semi-humid climates in the Cauca valley. We used 45 sampling sites between 700 and 3,500 m a.s.l. along the main Cauca River basin and in its tributary basins, including Risaralda, Campoalegre, Opiramá, Tapias, Pozo, and Supía rivers.
Figure 1. (A) Hydrographic network of the study area. Circle size indicates species richness at each sampling site. Blue circles indicate sites that were used as headwater streams in the GDM. (B) Cost surface.
This study was carried out with recommendations and approval of the Ethics Committee for Animal Experimentation from the Universidad de Antioquia (CEEA). Fish were captured during the first low-flow period of 2015 between February and March in longitudinal transects of 100 meters. The fishing effort at each site consisted in 30 throws using cast nets with different mesh sizes (0.5, 1, 2 cm) and an area sweep for 60 min using electrofishing.
All captured specimens were recorded; some were released, while others were fixed in 10% formaldehyde, taken to the lab, stored in 75% alcohol, and identified using taxonomic keys and compared with identified specimens and deposited in the fish collection of the Universidad de Antioquia CIUA. The identified records are available from the Global Biodiversity Information Facility (URL: https://www.gbif.org).
Each site was georeferenced and the following environmental variables were recorded using a Multi-Probe System (YSI 556 MPS): dissolved oxygen (mg/l), water temperature (°C), pH, and conductivity (μScm−1) (see Table S1 in Supplementary Material). Even though sampling effort was the same for all sites, we conducted individual based rarefaction curves for each site to get an idea of sampling efficacy using the Vegan package (Oksanen et al., 2018) in software R (R Core Team, 2018).
For the purpose of this study, we defined connectivity among sampling sites as the degree in which the fluvial network facilitates or prevents movement between them (Tonkin et al., 2018). We used the measure of effective resistance, based on electrical circuit theory, as an indicator of connectivity between two sites. A high resistance value indicates little connectivity between sites. To quantify resistance, we generated a cost surface with low resistances assigned to habitats that are most permeable to movement, and high resistances assigned to poor dispersal habitat or to movement barriers (Shah and McRae, 2008). To accomplish this, we used the elevation raster available from the PALSAR radar system at a spatial resolution of 12.5 m to calculate slope angles in degrees, and determined drainages (Figure 1B) using the hydrology toolset from ArcMap 10.2 software (ESRI, 2011). The final cost surface was the result of the sum of both surfaces. Since the drainages are used for transit, all cells along the drainage were assigned a value of 1 while all other cells were discarded. The slope values varied from 0 to 90 degrees, where a 90-degree watercourse slope is vertical.
With the cost surfaces along the drainage system we calculated the fluvial network resistance to organism movement, with the software Circuitscape (Shah and McRae, 2008). This program calculates the connectivity between sites based on an analogy with electrical circuit theory, (McRae and Shah, 2011). Connectivity is quantified as the amount of current conducted from one site to another. The resistance of a resistor is the reciprocal of its conductance and can be thought of as representing isolation or movement cost between nodes. This measure considers not only the least cost path but all possible paths between a pair of sites, which might be more suitable as a measure of connectivity in biological systems (McRae et al., 2008). In our particular case, we used the program output file that reporting effective resistances between all pairs of focal nodes in the pairwise model.
In order to identify whether the longitudinal fragmentation caused by changes in elevation influences β diversity among fish communities, we calculated the Sorensen dissimilarity index (βsor) and its components: turnover (βsim) and nestedness (βnes; Equation 1) using the Betapart package (Baselga and Orme, 2012) in R software (R Core Team, 2018). This index is based on presence-absence matrices and determines which of the components (βsim or βnes) underlies variations in β diversity through the following equation:
where βsor is the Sorensen dissimilarity and is made up of the Simpson similarity (βsim), which consists of the substitution of species in one site for different species in another site (species replacement) describing a spatial turnover that is not influenced by differences in the species richness of each community, and βnes, which is the nestedness that occurs when sites with less species richness are a subgroup of the species at the sites with higher species richness (Leprieur et al., 2011; Baselga and Orme, 2012).
To explore which potential environmental and spatial variables may drive the variation in β diversity (βsor) among sites, we used a Generalized Dissimilarity Model (GDM). A GDM is a technique, which allows one to model the spatial variation in biodiversity based on the variation in environmental conditions, including means of spatial isolation, such as geographic distance. The model can predict the change in the composition of communities, based on a curvilinear relationship with the predictor variables (Ferrier et al., 2007). This type of relationship between change in composition and change in the ecological environment is more adequate since betadiversity reaches a maximum when two sites do not share species while ecological dissimilarity can always increase. In its traditional version, this model uses pairs of geographical coordinates to calculate distances in a straight line. This would not fit our purpose since we were interested in calculating distances along the drainage network and considering slope effects. Thus, we used the resistance matrix derived from Circuitscape as input in a non-metric multidimensional scaling (NMDS) analysis with the aim of transforming the resistance matrix into points on a two-dimensional space. The coordinates obtained through NMDS for each of the points were used to calculate euclidean distances for the GDM model. In addition to the distances calculated, the following continuous predictor variables were used in the GDM: dissolved oxygen, pH, elevation, and conductivity.
Two models were constructed; one used all 45 sampling sites, while the other only used the farthest sites from each of the drainage branches (seven sampling sites). The first model sought to capture variables that allow predicting most of the variation in the fish community composition, whereas the second model sought to evidence the isolation effect generated by the distance and accumulation of physical barriers for fish displacement.
In the 45 sampling sites, 2,475 specimens were recorded from 43 species grouped in five orders, 13 families, and 29 genera. The rarefaction curves from most sampling sites presented asymptotic behavior (see Figure S1 in Supplementary Material), which can be interpreted as evidence that sampling was representative, at least during the time intervals in which samples were taken. The greatest species richness (16 species) was found in the Supía River at 759 m a.s.l., whereas the sites where only one species was collected were at elevations above 1,400 m a.s.l., including La Leona stream, and the high parts of Pozo River, Tapias River, and Chinchiná River (see Table S1 in Supplementary Material, Figure 1). Headwater fish assemblages had on average two species and were characterized by a prevalence of species of the genus Astroblepus (60%).
The average β diversity (βsor) among all sites was 0.93 ± 0.002, and the beta diversity component due to turnover (βsim) was 0.867 ± 0.007, while the component due to nestedness (βnes) was only 0.063 ± 0.006. The same pattern was observed when performing the analysis only with the headwater sites. Average beta diversity (βsor) was 0.8184 ± 0.03, and turnover (βsim) was the most important component (0.6860 + 0.05), while nestedness (βnes) was relatively low (0.13 + 0.25). In other words, the replacement of species is the component that most contributes to the general beta diversity of the area (Figure 2). The low percentage of β diversity that was not originated by differences in species composition is related to nestedness.
Figure 2. Density plots with the distribution of general beta diversity (βsor, solid line) and its nestedness component (βnes, dashed gray line) and turnover component (βsim, dashed black line). (A) Results for all sampling sites and (B) results for headwater stream sites only.
The GDM conducted for all sampling sites explained 33.14% of the total deviance in β diversity, whereas, if conducted using only the farthest sampling sites in each of the sub-basins, 51% of the deviance was explained.
In terms of the relative importance of each predictor variable, results differed between models (Figure 3). In the model including all sampling sites, the most important variable was elevation, followed by pH, dissolved oxygen, and resistance last, whereas in the model that used only headwater stream sites, resistance was the most important variable, followed by elevation. Conductivity does not seem to contribute to either of the models as all of the coefficients assigned to this I-spline equaled 0.
Figure 3. I-splines generated for each environmental variable from the GDM model to predict beta diversity. The maximum height of each curve indicates the magnitude of turnover in fish communities with respect to that variable. The relative magnitude among variables within a model is indicative of the relative importance among variables to explain the changes in β diversity while maintaining the remaining variables constant. The form of each function indicates the rate of turnover throughout the gradient. (A) Results of model with all sampling sites (B), and results only including only headwater stream sites.
Results of our study support the hypothesis that both environmental gradients and connectivity exert an effect on the composition of fish communities, but their relative contributions vary along the drainage system. Connectivity exerts a greater contribution to explain beta diversity when only headwater streams are used, while changes in elevation explain variation among most other sites. This is partly related to the contrasting structure of river networks between high and low elevation sites. While there is a high degree of connectivity in water systems in lowland areas (e.g., <500 m), rivers and streams in the Andes are isolated and discontinuous (Schaefer, 2011) partly due to waterfalls and chutes that act as natural barriers within the fluvial network that affect the degree of connectivity and configure permeability and the availability of habitats for freshwater organisms (Rahel, 2007; Dias et al., 2013). As a result, the degree of connectivity within a fluvial network is an important mechanism that promotes speciation, extinction, and migration in evolutionary time-scales (Dias et al., 2013), with particular relevance in headwater streams. On the other hand, changes in elevation, which are related to changes in temperature, vegetation, and substrate conditions, may exert significant differential selective pressures that account for a major part of the variation in fish assemblage composition. Unlike elevation and connectivity, which significantly contribute to explain the variation in β diversity in this system, changes in local physicochemical water conditions, such as pH, dissolved oxygen, and temperature, seem to contribute little (Figure 3). The high variability of the water conditions at sampling sites is associated to seasonal precipitation changes, soil types, and the riparian forest cover, amongst others, which were not measured in this study. This variation could have reduced the influence of these variables in the model.
Beta diversity among the fish communities of the Cauca River basin was mainly characterized by strong species replacement. Our results support those obtained by Leprieur et al. (2011) on a global scale, where the variation in β diversity in South America is mainly caused by turnover. This same result was found in other studies conducted in freshwater systems at the local level where the dominant component in terms of β diversity is turnover and where the nestedness component is generally low (Tisseuil et al., 2012; Angeler, 2013; Jamoneau et al., 2018). The nestedness component is characteristic of lowland communities (Granado-Lorencio et al., 2012; <500 m, Henriques-Silva et al., 2018), and has been observed in other water organisms, such as diatoms (Jamoneau et al., 2018). According to the network position hypothesis (Brown and Swan, 2010), the communities of downstream stretches are increasingly influenced by effects of mass due to their position in the fluvial network, which facilitates dispersal. Migratory fish are a good example of species with strong dispersal capacity that can homogenize fish assemblages especially at low to intermediate elevations (Ríos-Pulgarín et al., 2008; Jiménez-Segura et al., 2014). Only one migratory species—Brycon henni—was recorded in this survey and is characterized by short, lateral migration patterns (Builes and Uran, 1974). We recovered a low nestedness component because our sampling sites were located at elevations above 700 m. The spatial patterns of beta diversity evidenced are consistent with a scenario of differential selection along the elevation gradient that suggests a steady change in composition along the gradient (Vellend, 2016), and isolation in conjunction with extreme selective pressures at headwater sites.
In this study we found an important contribution of βsim to β diversity, which is often associated with climatic gradients (McCain, 2005; Bryant et al., 2008). Nonetheless, we propose that aside from the contribution of climate gradients, connectivity due to the dendritic structure of the basins and principally driven by headwater assemblages (Tonkin et al., 2018) also contributes to the high relative importance of turnover. Headwater sites are characterized by low species richness (Figure 1) but very distinctive compositions. Headwater assemblages are important because, even though they present low α diversity, they contribute to a high γ diversity with a group of endemic species (Clarke et al., 2008; Carvajal-Quintero et al., 2015). In the case of the Andes, the headwater communities are mainly composed of species belonging to the genera Astroblepus and Trichomycterus (Barletta et al., 2010; Jiménez-Segura et al., 2016), which have been recorded at up to 3,300 m a.s.l (Maldonado et al., 2011). Besides the importance of these assemblages due to their distinctive composition, the functional traits present in these species reflect unique adaptations required by the fish in order to inhabit water bodies characterized by extreme environmental conditions, such as low temperatures, high slopes, and fast-flowing waters. Fish from the Astroblepus genus are characterized by depressed bodies, lips specialized for adhering to substrates, dorsolateral eyes, and well-developed pectoral, pelvic, and caudal fins, which provide greater stability on the substrate and allow for rapid, explosive movements (De Crop et al., 2013; Conde-Saldaña et al., 2017). This genus presents a complex history where divergence and dispersal along the drainage basins are the main factors explaining their distribution; they are usually species that are endemic or unique to a particular river or its adjacent systems (Schaefer et al., 2011).
Defining conservation areas to protect species as well as endemisms within fluvial networks, should consider not only the number of drainages but also the probability of connection among them. Our results indicate that low-order drainages are the most isolated and present a number of endemic species. The challenge lies not only in determining how many patches to conserve, but also which ones. In Colombia, ca. 25,711 km2 at elevations above 1,000 m are under some type of protection. Thus, an important next step is to identify the water bodies that exist within the current protected areas and determine whether it is necessary to complement the existing reserve network with new areas or if the current protected network is accomplishing our conservation goals. It is important to keep in mind that our analyses were conducted in a small part of the central branch of the Andes and that the ichthyofauna of the other two branches may differ in terms of species composition (Jiménez-Segura et al., 2014). Therefore, it will be necessary to increase the extent of this analysis in order to obtain a broader perspective on the conservation strategies that must be implemented in the northwestern Andean region.
In conclusion, we identified patterns in the spatial distribution of β diversity that support a role for environmental gradients and connectivity at the local level, and we suggest that the effects of connectivity are magnified in headwater streams. Even though most studies along mountain sides identify climate gradients as ultimate drivers of variation in community properties, we were able to unmask the effects of other variables such as connectivity among sites, considering that euclidean geographic distances are not adequate to describe the complex structure of fluvial networks (Henriques-Silva et al., 2018). Our models explained only 33 and 51% of the variation in β diversity, which suggests that there may be other variables that have not yet been considered; for example, our analyses did not take into account the one-directional flow of water nor other environmental variables, such as the watercourse width, depth, and stream bed composition. We suggest that future studies include these variables as well as the interaction between topography and water mass (water level and average velocity of the water bodies). Regarding composition, it is important to take samples during other seasons and at lower elevations in order to detect the influence of migratory species, which, due to their movement capacity, would participate in different communities depending on the time of year. Continuing to model these scenarios will improve our understanding of the system behavior and allow us to identify the possible effects of basin fragmentation and climate change in Andean fish communities.
All authors conceived the presented idea. JP suggested the type of analysis and wrote parts of the manuscript. JH-P performed the analysis. LJ-S helped in data interpretation and wrote parts of the manuscript. DR-S provided orientation in the manuscript style. All authors discussed the results and contributed to the final manuscript.
The authors declare that the research was conducted in the absence of any commercial or financial relationships that could be construed as a potential conflict of interest.
The authors would like to thank CORPOCALDAS for its financial support, as well as Oscar Ospina Herrera for his continued aid during collection. Thanks to Juan Guillermo Ospina and Juan Pablo Londoño for their field work, fish identification, and input for the development of this proposal. Thanks to the GIUA ichthyology group and its CIUA ichthyological collection. Thanks to Humberto Vergara for providing consultation regarding analysis of geographical information systems. Finally we would like to comment on their comments and to Donald Taphorn for grammar reviews.
The Supplementary Material for this article can be found online at: https://www.frontiersin.org/articles/10.3389/fenvs.2019.00009/full#supplementary-material
Albert, J. S., and Carvalho, T. P. (2011). “Neogene assembly of modern faunas,” in Historical Biogeography of Neotropical Freshwater Fishes, eds R. E. Reis and J. S. Albert (Berkeley, CA; Los Angeles, CA; London: University of California Press), 118–136.
Altermatt, F. (2013). Diversity in riverine metacommunities: a network perspective. Aquatic Ecol. 47, 365–377. doi: 10.1007/s10452-013-9450-3
Angeler, D. G. (2013). Revealing a conservation challenge through partitioned long-term beta diversity: increasing turnover and decreasing nestedness of boreal lake metacommunities. Divers. Distrib. 19, 772–781. doi: 10.1111/ddi.12029
Barletta, M., Jaureguizar, A. J., Baigun, C., Fontoura, N. F., Agostinho, A. A., Almeida-Val, V. M., et al. (2010). Fish and aquatic habitat conservation in South America: a continental overview with emphasis on neotropical systems. J. Fish Biol. 76, 2118–2176. doi: 10.1111/j.1095-8649.2010.02684.x
Baselga, A. (2010). Partitioning the turnover and nestedness components of beta diversity. Glob. Ecol. Biogeogr. 19, 134–143. doi: 10.1111/j.1466-8238.2009.00490.x
Baselga, A., and Orme, C. D. L. (2012). Betapart: an R package for the study of beta diversity. Methods Ecol. Evol. 3, 808–812. doi: 10.1111/j.2041-210X.2012.00224.x
Brown, B. L., and Swan, C. M. (2010). Dendritic network structure constrains metacommunity properties in riverine ecosystems. J. Anim. Ecol. 79, 571–580. doi: 10.1111/j.1365-2656.2010.01668.x
Bryant, J. A., Lamanna, C., Morlon, H., et al. (2008). Microbes on mountainsides: contrasting elevational patterns of bacterial and plant diversity. Proc. Natl. Acad. Sci. U.S.A. 105, 11505–11511. doi: 10.1073/pnas.0801920105
Builes, J., and Uran, A. (1974). Estudio del ciclo sexual de la Sabaleta Brycon henni Eigenmann. Su comportamiento y fecundación artificial. Actual. Biológicas 7, 2–12.
Carvajal-Quintero, J. D., Escobar, F., Alvarado, F., Villa-Navarro, F. A., Jaramillo-Villa, Ú, and Maldonado-Ocampo, J. A. (2015). Variation in freshwater fish assemblages along a regional elevation gradient in the northern Andes, Colombia. Ecol. Evol. 5, 2608–2620. doi: 10.1002/ece3.1539
Cilleros, K., Allard, L., Grenouillet, G., and Brosse, S. (2016). Taxonomic and functional diversity patterns reveal different processes shaping European and Amazonian stream fish assemblages. J. Biogeogr. 43, 1832–1843. doi: 10.1111/jbi.12839
Clarke, A., Mac Nally, R., Bond, N., and Lake, P. S. (2008). Macroinvertebrate diversity in headwater streams: a review. Freshw. Biol. 53, 1707–1721. doi: 10.1111/j.1365-2427.2008.02041.x
Conde-Saldaña, C. C., Albornoz-Garzón, J. G., López-Delgado, E. O., and Villa-Navarro, F. A. (2017). Ecomorphological relationships of fish assemblages in a trans-Andean drainage, Upper Magdalena River Basin, Colombia. Neotrop. Ichthyol. 15, 1–12. doi: 10.1590/1982-0224-20170037
Cote, D., Kehler, D. G., Bourne, C., and Wiersma, Y. F. (2009). A new measure of longitudinal connectivity for stream networks. Landsc. Ecol. 24, 101–113. doi: 10.1007/s10980-008-9283-y
De Crop, W., Pauwels, E., Van Hoorebeke, L., and Geerinckx, T. (2013). Functional morphology of the Andean climbing catfishes (Astroblepidae, Siluriformes): alternative ways of respiration, adhesion, and locomotion using the mouth. J. Morphol. 274, 1164–1179. doi: 10.1002/jmor.20169
De La Barra, J., Aguilera, G., Maldonado, M., and Pouilly, M. (2016). ¿Qué factores determinan la distribución altitudinal de los peces de ríos tropicales andinos? Rev. Biol. Trop. 64, 173–192. doi: 10.15517/rbt.v64i1.18576
Dias, M. S., Cornu, J. F., Oberdorff, T., Lasso, C. A., and Tedesco, P. A. (2013). Natural fragmentation in river networks as a driver of speciation for freshwater fishes. Ecography. 36, 683–689. doi: 10.1111/j.1600-0587.2012.07724.x
Ferrier, S., Manion, G., Elith, J., and Richardson, K. (2007). Using generalized dissimilarity modelling to analyse and predict patterns of beta diversity in regional biodiversity assessment. Divers. Distrib. 13, 252–264. doi: 10.1111/j.1472-4642.2007.00341.x
Granado-Lorencio, C., Gulfo, A., Alvarez, F., Jiménez-Segura, L. F., Carvajal-Quintero, J. D., and Hernández-Serna, A. (2012). Fish assemblages in floodplain lakes in a Neotropical river during the wet season (Magdalena River, Colombia). J. Trop. Ecol. 28, 271–279. doi: 10.1017/S0266467412000181
Guareschi, S., Bilton, D. T., Velasco, J., Millán, A., and Abellán, P. (2015). How well do protected area networks support taxonomic and functional diversity in non-target taxa? The case of Iberian freshwaters. Biol. Conserv. 187, 134–144. doi: 10.1016/j.biocon.2015.04.018
Henriques-Silva, R., Logez, M., Reynaud, N., Tedesco, P. A., Brosse, S., Januchowski-Hartley, S. R., et al. (2018). A comprehensive examination of the network position hypothesis across multiple river metacommunities. Ecography 41, 1–11. doi: 10.1111/ecog.03908
Jamoneau, A., Passy, S. I., Soininen, J., Leboucher, T., and Tison-Rosebery, J. (2018). Beta diversity of diatom species and ecological guilds: response to environmental and spatial mechanisms along the stream watercourse. Freshw. Biol. 63, 62–73. doi: 10.1111/fwb.12980
Jaramillo-Villa, U., Maldonado-Ocampo, J. A., and Escobar, F. (2010). Altitudinal variation in fish assemblage diversity in streams of the central Andes of Colombia. J. Fish Biol. 76, 2401–2417. doi: 10.1111/j.1095-8649.2010.02629.x
Jiménez-Segura, L. F., Galvis-Vergara, G., Cala-Cala, P., García-Alzate, C. A., López-Casas, S., Ríos-Pulgarín, M. I., et al. (2016). Freshwater fish faunas, habitats and conservation challenges in the Caribbean river basins of north-western South America. J. Fish Biol. 89, 65–101. doi: 10.1111/jfb.13018
Jiménez-Segura, L. F., Restrepo-Santamaría, D., López-Casas, S., Delgado, J., Valderrama, M., Álvarez, J., et al. (2014). Ictiofauna Y desarrollo del sector hidroeléctrico en la cuenca del río magdalena-cauca, colombia luz. Biota Colomb. 15, 3–25. doi: 10.21068/c0001
Leprieur, F., Tedesco, P. A., Hugueny, B., Beauchard, O., Dürr, H. H., Brosse, S., et al. (2011). Partitioning global patterns of freshwater fish beta diversity reveals contrasting signatures of past climate changes. Ecol. Lett. 14, 325–334. doi: 10.1111/j.1461-0248.2011.01589.x
Lévêque, C., Oberdorff, T., Paugy, D., Stiassny, M. L. J., and Tedesco, P. A. (2008). Global diversity of fish (Pisces) in freshwater. Hydrobiologia 595, 545–567. doi: 10.1007/s10750-007-9034-0
Loiseau, N., Legras, G., Kulbicki, M., Mérigot, B., Harmelin-Vivien, M., Mazouni, N., et al. (2017). Multi-component β-diversity approach reveals conservation dilemma between species and functions of coral reef fishes. J. Biogeogr. 44, 537–547. doi: 10.1111/jbi.12844
Lomolino, M. V. (2001). Elevation gradients of species-density: historical and prospective views. Glob. Ecol. Biogeogr. 10, 3–13. doi: 10.1046/j.1466-822x.2001.00229.x
Maldonado, M., Maldonado-Ocampo, J., a Ortega, H., Encalada, A. C., Carvajal-Vallejos, F. M., Rivadeneira, J. F., et al. (2011). “Biodiversity in aquatic systems of the tropical andes,” in Climate Change and Biodiversity in the Tropical Andes, eds S. K Herzog, R. Martínez, P. M. Jørgensen, and H. Tiessen. IAI - Inter-American Institute for Global Change Research. 276–294.
McCain, C. M. (2005). Elevational gradients in diversity of small mammals. Ecology 86, 366–372. doi: 10.1890/03-3147
McRae, B. H., Dickson, B. G., Keitt, T. H., and Shah, V. B. (2008). Using circuit theory to model connectivity in ecology, evolution, and conservation. Ecology 89, 2712–2724. doi: 10.1890/07-1861.1
McRae, B. H., and Shah, V. B. (2011). Circuitscape User Guide. ONLINE. Santa Barbara: The University of California. Available online at: http://www.circuitscape.org
Oksanen, J., Friendly, F. G. B. M., Kindt, R., Legendre, P., McGlinn, D., Minchin, P. R., et al. (2018). Vegan: Community Ecology Package. R package version 2.5-3. Available online at: https://cran.r-project.org/package=vegan%0A
Pouilly, M., Barrera, S., and Rosales, C. (2006). Changes of taxonomic and trophic structure of fish assemblages along an environmental gradient in the Upper Beni watershed (Bolivia). J. Fish Biol. 68, 137–156. doi: 10.1111/j.0022-1112.2006.00883.x
R Core Team (2018). R: A Language and Environment for Statistical Computing. Vienna: R Foundation for Statistical Computing. Available online at: https://www.R-project.org/
Rahel, F. J. (2007). Biogeographic barriers, connectivity and homogenization of freshwater faunas: it's a small world after all. Freshw. Biol. 52, 696–710. doi: 10.1111/j.1365-2427.2006.01708.x
Ríos-Pulgarín, M. I., Jiménez-Segura, L. F., Palacio, J. A., and Ramírez-Restrepo, J. J. (2008). Comunidad de peces en la ciénaga de Ayapel, cambios espacio-temporales en su asociación. Actual. Biol. 30, 29–53.
Schaefer, S. (2011). “The Andes: riding the tectonic uplift,” in Historical Biogeography of Neotropical Freshwater Fishes, eds R. E. Reis and J. S. Albert (Berkeley, CA; Los Angeles, CA; London: University of California Press), 259–278.
Schaefer, S. A., Chakrabarty, P., Geneva, A. J., and Sabaj Pérez, M. H. (2011). Nucleotide sequence data confirm diagnosis and local endemism of variable morphospecies of Andean astroblepid catfishes (Siluriformes: Astroblepidae). Zool. J. Linn. Soc. 162, 90–102. doi: 10.1111/j.1096-3642.2010.00673.x
Shah, V. B., and McRae, B. (2008). “Circuitscape: a tool for landscape ecology,” in Proceedings of the 7th Python in Science Conference, eds G. Varoquaux, T. Vaught, and J. Millman (Pasadena, CA), 62–65.
Socolar, J. B., Gilroy, J. J., Kunin, W. E., and Edwards, D. P. (2016). How should beta-diversity inform biodiversity conservation? Trends Ecol. Evol. 31, 67–80. doi: 10.1016/j.tree.2015.11.005
Tisseuil, C., Leprieur, F., Grenouillet, G., Vrac, M., and Lek, S. (2012). Projected impacts of climate change on spatio-temporal patterns of freshwater fish beta diversity: a deconstructing approach. Glob. Ecol. Biogeogr. 21, 1213–1222. doi: 10.1111/j.1466-8238.2012.00773.x
Tonkin, J. D., Altermatt, F., Finn, D. S., Heino, J., Olden, J. D., Pauls, S. U., et al. (2018). The role of dispersal in river network metacommunities: patterns, processes, and pathways. Freshw. Biol. 63, 141–163. doi: 10.1111/fwb.13037
Vari, R. P., and Malabarba, L. R. (1998). “Neotropical ichthyology: an overview,” in Phylogeny and Classification of Neotropical Fishes, eds L. R. Malabarba, R. E. Reis, R. Vari, Z. M. S. Lucena, and C. A. S. Lucena (Porto Alegre: Edipucrs), 1–11.
Keywords: tropics, freshwater, beta diversity, river connection, teleostei
Citation: Herrera-Pérez J, Parra JL, Restrepo-Santamaría D and Jiménez-Segura LF (2019) The Influence of Abiotic Environment and Connectivity on the Distribution of Diversity in an Andean Fish Fluvial Network. Front. Environ. Sci. 7:9. doi: 10.3389/fenvs.2019.00009
Received: 02 November 2018; Accepted: 15 January 2019;
Published: 01 February 2019.
Edited by:
Mario Barletta, Universidade Federal de Pernambuco (UFPE), BrazilReviewed by:
Nelson Ferreira Fontoura, Pontifícia Universidade Católica do Rio Grande do Sul, BrazilCopyright © 2019 Herrera-Pérez, Parra, Restrepo-Santamaría and Jiménez-Segura. This is an open-access article distributed under the terms of the Creative Commons Attribution License (CC BY). The use, distribution or reproduction in other forums is permitted, provided the original author(s) and the copyright owner(s) are credited and that the original publication in this journal is cited, in accordance with accepted academic practice. No use, distribution or reproduction is permitted which does not comply with these terms.
*Correspondence: J. Herrera-Pérez, anVsaWFuYS5oZXJyZXJhLnBAZ21haWwuY29t
Disclaimer: All claims expressed in this article are solely those of the authors and do not necessarily represent those of their affiliated organizations, or those of the publisher, the editors and the reviewers. Any product that may be evaluated in this article or claim that may be made by its manufacturer is not guaranteed or endorsed by the publisher.
Research integrity at Frontiers
Learn more about the work of our research integrity team to safeguard the quality of each article we publish.