- Department of Plant Sciences, University of Saskatchewan, Saskatoon, SK, Canada
An increase in intensive cropping would benefit society by providing food to a growing population, and vegetable production is an excellent example of intensive cropping systems that are indeed on the rise. Vegetable cropping systems are high-input and generally require large quantities of fertilization, frequent irrigation, and repeated tillage operations. Consequently, an increase in global vegetable production may have seriously negative impacts on soil health and ecosystem services. Yet, not only maintaining but improving soil health is critical to enhancing the sustainability of food production systems. Previous agricultural research mainly focused on field crop systems and largely ignored vegetable cropping systems; consequently, this represents a conspicuous research gap, one that must be addressed in order to make progress toward sustainable food production. Here, we review the literature to gain a better understanding of how management has influenced various soil health indices (soil biology, chemistry, and physical dynamics) and to evaluate the implications for soil ecosystem services in vegetable cropping systems. We found that alternative modifications to conventional vegetable production systems, which resemble methods used in organic or conservation agriculture, tended to improve aspects of soil health. For example, soil amendments generally improved soil chemical and nutrient indices of health—soil carbon levels and nitrogen reserves in particular. Incorporation of cover crops to vegetable crop rotations tended to improve nitrogen recycling via reduced nitrate leaching risks, increased soil carbon levels, and weed suppression. Reduced tillage systems were rare, presenting an important challenge and opportunity for further improving soil health dynamics in vegetable production. Notably, adopting alternative practices generally had no effect on crop yields, which implies little risk of yield penalties when agronomic management is carefully planned. Our results indicate that future sustainable vegetable cropping systems may embody a blend between organic and conventional ideologies to better maintain or improve soil ecosystem functioning.
Introduction
One of the greatest challenges facing humanity today is the urgent need to provide nutritious food to over 9 billion people. This challenge is 2-fold because not only must food production expand and intensify, it must do so in a way that does not compromise the very ecosystem services that are necessary for life on earth. Some of the most important ecosystem services are provided by healthy soils, such as carbon sequestration, nutrient supply, and water regulation. But these ecosystem services are easily disturbed through modifications of soil physical, chemical and biological conditions with cropping intensification (Tilman et al., 2002; Power, 2010). Decades of previous research has led to great success in improving methods of crop production while minimizing impact on soil health and ecosystem services, notably, the adoption of conservation agriculture with reduced tillage, reduced frequency of fallowing, and reduced fertilizer applications via strategic crop rotations or pre-plant soil tests (Doran, 2002; Hobbs, 2007; Knowler and Bradshaw, 2007). However, these successes apply only to field crops of grain, cereal, and oilseed crops—~70% of which are produced for the purpose of animal feed (Cassidy et al., 2013) rather than nutritious crops, such as vegetables, for human consumption.
Global vegetable production is rapidly expanding (FAO, 2017) due to health guidelines that recommend an increase in vegetable consumption (WHO, 2003). However, due to vegetable crop growth requirements and inherent nutritious nature, soils under vegetable crop production are managed extremely intensively; vegetable production requires much higher inputs (such as, fertilizers, irrigation, and frequent/deep tillage operations Jackson et al., 2004; Willekens et al., 2014; Congreves and Van Eerd, 2015) compared to field crops. For some vegetable crops, very little organic matter is returned to the soil after harvest (Jackson et al., 2004; Willekens et al., 2014; Brennan and Acosta-Martinez, 2017). Consequently, vegetable production may have seriously negative impacts on soil health and ecosystem services. Unfortunately, mainstream agricultural research has largely ignored soil health in vegetable cropping systems, and this represents a conspicuous research gap; one that must be addressed in order to make progress toward feeding a growing population sustainably.
Literature on soil attributes in vegetable cropping systems may not be as prevalent as for field cropping systems, but it nonetheless exists under various guises. Vegetable agronomy research is published across a wide range of disparate fields (bioresource science, horticultural sciences, agricultural sciences, ecology, land degradation science, geochemistry, soil science, plant science, urban agriculture, etc.) which is likely a factor explaining why very few research reviews (none that we are aware of) have been conducted to comprehensively evaluate how vegetable management practices influence soil health indicators. Research on soil attributes in various vegetable crop systems must be synthesized in order to understand which type of management practices best promote soil health and long-term intensive agricultural sustainability, and to identify where gaps in our understanding exist.
Soil quality and soil health are often viewed as interchangeable terms; here we use the term soil health because it encompasses the integrated and complex functions that soils provide e.g., carbon sequestration, nutrient cycling, water regulation and filtration, and biological diversity. Soil health is a broad concept and it is challenging to measure and interpret. By examining soil with an ecological perspective, i.e., considering soil physical, chemical, and biological properties, one can approach a better understanding of the various indices of soil health.
Organic farming is often practiced through an ecological perspective (Scialabba and Müller-Lindenlauf, 2010). However, while highly contextual and dependent on crop type, organic yields are typically lower than conventional yields (Seufert et al., 2012)—a result which compromises societal or economic benefits despite potential environmental benefits of organic management. A recent global meta-analysis showed greater yield reductions for vegetable crops than for cereals, oilseeds, and fruits under organic relative to conventional management (Seufert et al., 2012). Even with considerable yield concerns, the detail remains that organic systems have a strong potential for building soil health indices such as soil fertility and organic matter (Scialabba and Müller-Lindenlauf, 2010). Thus, perhaps a blend between organic and conventional management is a more compelling approach to maintain or improve soil health indices while maintaining crop yields, rather than strictly organic management in vegetable cropping systems. For example, if the system is not limited by nutrient inputs perhaps organic or conservation practices are key to improving soil health indices without negatively impacting vegetable yields. Here, we pose the question whether or not alternative management practices—which may resemble organic or conservation agriculture—have the potential to improve soil health indices relative to conventional vegetable crop management, without negatively impacting crop yields. If so, to what extent and which types of alternative practices have the greatest potential for improving soil health indices? To lay the foundation for better understanding soil health in intensive vegetable cropping systems, here, we present the first review to synthesize how alternative management practices influence crop yields and soil health by evaluating soil biological indices, soil chemical composition and nutrient availability, and soil physical parameters.
Materials and Methods
Literature representing modern and westernized vegetable cropping systems across the Americas and Europe was compiled by using electronic search engines, mainly Google Scholar, Web of Science, and institutional library search engines with a focus on field research since 2000. The literature search was conducted from August 2017 to January 2018. Keywords included: soil health, soil quality, soil health indices, soil biology, soil chemistry, and/or soil physics; and were used in combination with keywords such as: vegetable, vegetable cropping systems, horticulture, intensive agriculture, and/or intensive cropping systems. Earlier papers that fit our parameters were identified as citations within the compiled papers. As part of our data compilation and in preparation for data analysis, the following factors were categorized in our database: vegetable crop species, trial location, the type of soil measurements (by listing the soil physical, biological and/or chemical attributes measured), the duration of the trial in years, type of experimental manipulation (amendment, tillage, cover crop, and/or crop rotation trials) with treatments listed (Table 1).
Of the studies compiled in our literature database, many were included in the meta-analysis. For studies to be included in the meta-analysis, there had to be a control treatment compared to experimental treatments, and the control had to be consistently defined across studies. Since we were interested in comparing the performance of conventional practices to alternative management, we defined the control treatments for each type of experimental manipulation. The controls included: un-amended treatments, no cover crop treatments (bare or fallow), and conventional tillage treatments (plow, cultivation, or disking). These controls were compared to manipulations that were: amended with organic material, included cover crops in the rotation, and implemented reduced tillage, respectively. Data were compiled from the literature database by extracting published values that were directly available from the tables and text. We did not extract data that was in figure-form. When studies reported multiple experimental results, e.g., multi-year, multi-horizon, or multi-measurements, data selected were refined based on longest experimental treatment time, topsoil ( < 30 cm), and/or most common soil properties. Data were assigned to categorical indices for physical, chemical or biological soil properties. Although the analytical methods to acquire the data may have differed, we were more focused on the overall direction and magnitude of the effect of management on each soil property. As examples, the extraction solution for pH measurements tended to vary; the measurements for soil carbon included loss on ignition and elemental analysis; soil nitrogen was measured by total Kjeldahl methods and elemental analysis methods, while nitrate data included measurements from lysimeters, extracts from ion resin membranes and from the soil. We considered total carbon and nitrogen as chemical indices rather than biological properties. For biological indices of soil health, microbial populations were determined by direct counts, colony forming units, rDNA, and phospholipid fatty acids (PLFA) while community level physiological profiles (CLPP) were primarily determined by the Biolog technique.
For the meta-analysis, we used response ratio (RR) values that described the treatment effect relative to the control. The RR values were determined as follows:
according to previously published methodology (Hedges et al., 1999; Maynard et al., 2014). The RR gives a unitless measure of treatment effects to compare across a wide array of experiments. The RR values above or below 1 indicate treatment responses greater than or lower than the control treatments, respectively. When the 95% confidence intervals of the RR mean do not overlap 1, then the treatment effect can be considered as significant.
Results
Body of Literature
Of the 60 publications collected, most research relied on short-term (2 to 4-year) field experiments or built off of medium-term (5 to 10-year) trials. The majority of papers collected (78%) evaluated soil chemical indicators of soil health, while 67% measured soil biological indicators, and relatively fewer studies (24%) collected soil physical measures of soil health (Table 1). Chemical and nutrient indices tended to focus on soil pH, EC, soil C, and N pools; biological indices primarily included microbial biomass, PLFA profiles, and soil nematodes; while physical attributes included bulk density, soil aggregate stability, texture, and moisture dynamics (Table 1). The seemingly heavy focus on nutrient and microbial and aspects of soil health may signify that there is more interest in understanding how to manage chemical and biological aspects in intensively managed vegetable crop soils. However, the relatively fewer studies on soil physics may not necessarily indicate there is poor interest in maintaining the physical aspects of soil health; rather, it is possible that soil physics literature might be less likely to use the keywords we searched with (e.g., soil health, soil quality, vegetable crop systems, etc.).
As for the type of manipulations that were described in the research we reviewed, 58% of the studies focused on the influence of amendments (manure, compost, biochar, green manure, etc.), 42% evaluated the effect of cover crops, while only 18% assessed the impact of different tillage systems in vegetable crop rotations (Table 1). Of the reviewed research, 105 different vegetable crops were studied. Those that were studied at the highest frequency, whether in the crop rotation or the crop in focus, included solanaceous crops (tomato, pepper, eggplant, and common potato) and cole crops (broccoli, cabbage, cauliflower, Brussels sprouts, kohlrabi), followed by cucurbit crops (cucumber, squash, zucchini, melon, pumpkin), grasses (entirely sweet corn) and green/salad (lettuce, spinach, chard) crops (Figure 1). Root (carrot, sweet potato, beet), bulb (onion, garlic), and pulse crops (bean, pea) occurred at a relatively lower frequency (Figure 1). Our results from these studies were determined by evaluating the RR of alternative management vs. conventional management and were deemed significant when their 95 % confidence interval did not overlap with 1. While numerous studies indicated the collection of soil health indicator data and met our criteria for inclusion in the meta-analysis, not all fully reported these data. Table 1 indicates which studies were included in the meta-analysis, and the number of comparisons that were used for the meta-analysis for each soil health indicator is presented as a number within the symbols on Figures 2, 3.
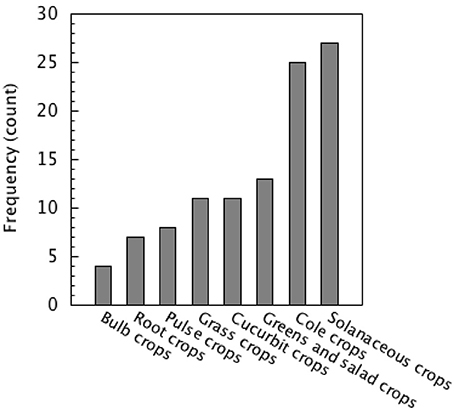
Figure 1. Frequency of various vegetable crops that were evaluated in the literature; classified according to use, botany or a combination of use/botany.
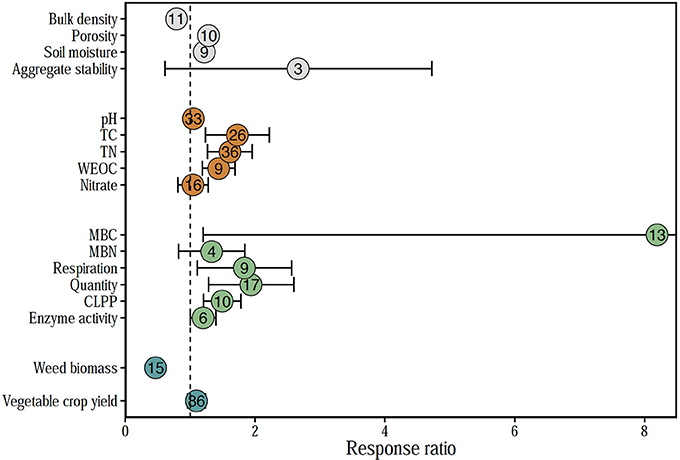
Figure 2. Effects of alternative management practices on soil physical, chemical and biological properties as indicators of soil health. All points are presented as Response Ratios (RR) with the 95 % confidence interval and the respective number of comparisons (n) presented inside the circle. TC, total carbon; TN, total nitrogen; WEOC, water extractable organic carbon; MBC, microbial biomass carbon; MBN, microbial biomass nitrogen; CLPP, community level physiological profile.
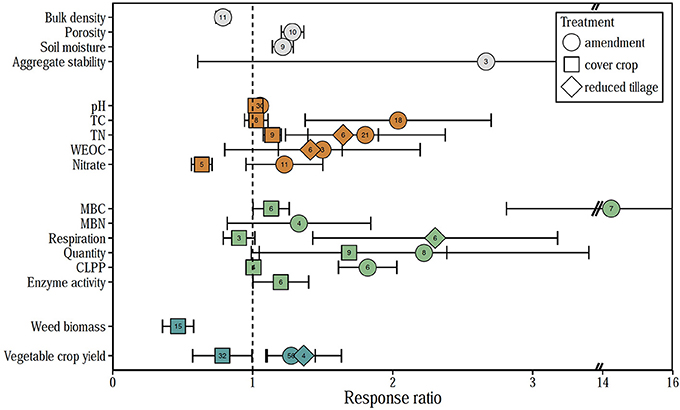
Figure 3. Effects of alternative management practices on soil physical, chemical and biological properties as indicators of soil health separated by management treatment (organic amendment, cover crop, reduced tillage). All points are presented as Response Ratios (RR) with the 95% confidence interval and the respective number of comparisons (n) presented inside the circle. TC, total carbon; TN, total nitrogen; WEOC, water extractable organic carbon; MBC, microbial biomass carbon; MBN, microbial biomass nitrogen; CLPP, community level physiological profile.
Alternative Management
Alternative management practices were defined as practices that generally deviated from conventional operations such as applying organic amendments, integrating cover crops into the crop rotation, or implementing methods of reduced tillage. Crop rotation was included within the experimental framework of all of the literature we reviewed but not as a main treatment effect. Hence, we did not focus the effect of crop rotation on soil health indices, but rather we focused on the effects of other treatments implemented within various crop sequences and rotations. The results of these practices were first considered in aggregate and, by and large, the results from our meta-analysis demonstrate that alternative management positively influences soil health indices (Figure 2). For instance, desirable affects surfaced due to alternative management, such as: reduced soil bulk density, increased soil porosity, increased soil water holding capacity, increased soil aggregate stability, increased soil carbon and nitrogen reservoirs, increased microbial indices (activity and community diversity), and reduced weed pressure. Notably, alternative management did not significantly influence vegetable crop yields (Figure 2).
Amendments
Organic carbon amendments tended to change soil properties in vegetable cropping systems (Figure 3) and were the main driver for improved soil health indices observed in our aggregate meta-analysis (Figure 2). The breadth of amendment types that were studied reflects the diversity of organic carbon material available for potentially improving soil health; ranged from various types of composts (plant-based, manure, or vermi-compost) (e.g., Morra et al., 2010), to biochar (e.g., Dumontet et al., 2017), and to used cooking oil (e.g., Congreves et al., 2014). There were also a variety of intended purposes for applying these amendments to soils in vegetable production, such as hypothesized improvements in soil organic matter levels (Morra et al., 2010), potential enhancements in microbial diversity (Dumontet et al., 2017), and reductions in postharvest N losses (Congreves et al., 2014).
Although relatively few studies focused on the soil physical aspects of soil health, when physical attributes were reported, there was a general improvement (Figure 3) including: decreased soil bulk density (0.79 RR), increased soil porosity (1.28 RR), and increased soil moisture (1.22 RR). A greater number of organic amendment studies reported data on soil chemical properties than soil physical properties. Meta-analysis of these data clearly indicated that amendments tended to increase soil carbon levels (2.04 RR; Figure 3). However, the soil nitrogen indices sometimes remained unchanged (Suddick and Six, 2013), or were sometimes increased (Warman, 2005; Ferreras et al., 2006; Hepperly et al., 2009; Beniston et al., 2016) therefore our results did not indicate as large an increase in RR (1.80) for total nitrogen or soil nitrate (1.23 RR) as for total carbon (Figure 3).
Soil microbial biomass was a commonly studied attribute and it was found to significantly increase due to additions of organic amendment (14.25 RR), although there was considerable variability among amendment trials (Figure 3). Other measures of biological activity, such as heterotrophic colony forming units, microbial respiration, Biology substrate use, Biology species identification, and PLFAs were used to determine changes to soil health following amendments and, when reported, were found to have results greater than their conventional management treatment (RR > 1) (Figure 2). Of interest, it was CLPP results which indicated significantly different soil biological activity under alternative management relative to conventional practice (Figure 3). Finally, our meta-analysis suggested that vegetable crop yields were significantly greater due to organic carbon amendment (Figure 3).
Cover Crops
Common cover crops included grasses (e.g., winter rye), legumes (e.g., hairy vetch, Austrian winter pea), brassicas (e.g., fodder radish), or mixtures of two or three of these types (Bending et al., 2004; Altieri et al., 2011; Belfry et al., 2017). Frequently, cover crops were planted following summer vegetable harvest with cover crop termination in the subsequent spring prior to planting. However, there were also cases where cover crops were planted as short-season summer crops to provide weed suppression and to supply soil nitrogen credits for fall-planted vegetable crops in the southeastern USA (Creamer and Baldwin, 2000).
Based on our literature review and meta-analysis, incorporating cover crops generally improved certain aspects of soil health compared to the conventional practice without cover crops (Figure 3). Soil chemical indicators of pH and soil carbon for cover crop treatments were not different from conventional management practices (RR = 1). However, soil total nitrogen levels were significantly greater (1.14 RR) with vs. without cover crop treatments, while soil nitrate measurements were significantly lower under cover cropping than their conventional management counterparts (0.64 RR) (Figure 3). Unfortunately for this meta-analysis there were no reports of soil physical properties with cover crop treatments.
Of the cover crop research collated here, 60% reported some measure of soil microbial biomass but no straightforward or significant response emerged (Figure 3). Cover crops sometimes increased microbial biomass (Wyland et al., 1996; Bandick and Dick, 1999; Maul et al., 2014; Brennan and Acosta-Martinez, 2017), decreased it (Bending et al., 2004), and mixed results were otherwise observed (Schutter et al., 2001; Marinari et al., 2015). This variable response for soil biological properties contributed to a lack of significant differences between cover crop and conventional management for various biological activity indicators such as soil respiration RR < 1, CLLP RR = 1 and RR > 1 for enzyme activity and microorganism quantity. In contrast to the soil biological results, including cover crop in vegetable cropping systems clearly decreased weed pressure (0.47 RR). While vegetable crop yields were lowered due to the presence of cover crops in the system (0.78 RR), the yield reduction was not significant (Figure 3).
Reduced Tillage
Of the studies we reviewed, few investigated the effects of tillage practices on intensive vegetable cropping systems (Table 1) and only one study fully reported their soil attribute results (Roper et al., 2017). Data from Roper et al. (2017) was included in our meta-analysis because it encompassed numerous long-term trials. Based on Roper and colleagues' work, reduced tillage significantly increased soil total nitrogen (1.65 RR), soil microbial respiration (2.30 RR) and crop yield (1.36 RR) compared to more intensive and conventional tillage practices (Figure 3).
Discussion
Crop Rotation
Undesirable and negative implications of continuously cropping the same species year after year are well documented, such as declined levels of soil organic matter, decreased aggregate stability, increased soil bulk density, increased soil erosion, increased disease prevalence, reduced crop yields, etc. (Ketcheson, 1980; Saini and Grant, 1980; Mohr et al., 2011; Munkholm et al., 2013; Agneessens et al., 2014; McDaniel et al., 2014; Gaudin et al., 2015; Tiemann et al., 2015). Consequently, crop rotations have been consistently recommended as a best management practice to improve production and help conserve soil quality (Doran et al., 1996; Karlen et al., 1997; Doran and Zeiss, 2000; Tilman et al., 2002). Yet, recent demand for biofuel production may pressure farmers to continuously cultivate the same high-energy crop year after year; there is potential that continuous cropping may increase in the future, despite the negative consequences on soil (McDaniel et al., 2014). Currently, it remains common practice to implement crop rotations in vegetable cropping systems, as reflected in all the studies compiled for this review.
Amendments
Our meta-analysis demonstrated that vegetable crop yields were significantly increased due to organic carbon amendments (Figure 3), likely a result of the additional nutrients supplied with the amendments and the improved soil and growing conditions provided. One compost amendment study even concluded that there was a causal relationship between a soil quality index and the yield of potato and beets (D'Hose et al., 2014). Based on the reviewed literature, all studies reported increased or similar crop yields with soil amendments compared to the un-amended controls—expect one. The distinctive case involved potato and corn production on sandy loam in Nova Scotia with municipal solid waste compost amendments compared to standard fertilizer applications; the authors suggested that mixing the compost with inorganic fertilizer would likely alleviate nutrient limitations and yield penalties (Mkhabela and Warman, 2005).
After 9-years of annual organic carbon amendments, heterotrophic colony forming units increased by 5-fold on a sandy soil in Florida (Ozores-Hampton et al., 2011). Not only was there increased microbial activity, but microbial populations were more likely to oxidize a wider range of carbon substrates when amendments were applied to a tomato rotation, compared to an un-amended control on a silty loam soil in Valenzano, Italy (Dumontet et al., 2017). The only case where an amendment decreased microbial biomass was for a relatively low application rate of poultry litter (Ninh et al., 2015).
The large RR and variation for soil microbial biomass (Figures 2, 3) was primarily due to the inclusion of an urban soil restoration study (Beniston et al., 2016). What might have been interpreted as outliers for soil microbial biomass or some physical attributes are essentially an extreme, but nonetheless important, case of soil restoration where land was converted from abandoned urban housing lots to intensive vegetable agricultural in Ohio (Beniston et al., 2016). These results emphasize the positive influence organic carbon amendments on soil physical properties. In their 1-year trial, the un-amended control had a bulk density of 1.46 Mg m−3 with only 0.30% water stable aggregates, while the compost amended soil (15 kg m−2 of composted leaves and grass clippings) decreased the soil bulk density to 1.05 Mg m−3 and increased soil aggregation to 0.49% (Beniston et al., 2016). Making use of urban soils for local food production is a unique niche for vegetable systems—one that this is increasing in popularity and demand; thus, represents an important and timely direction for new research on soil health.
Interpreting the influence of amendments on soil inorganic nitrogen (i.e., ) measurements can be challenging. In some cases, greater inorganic concentrations after amendment applications were interpreted as beneficial for soil health, i.e., greater nitrate availability for plant growth (Ninh et al., 2015), but in other cases it was interpreted as increased potential for nutrient losses with a negative impact on the environment (Evanylo et al., 2008). A number of studies explored how carbon amendments immobilize inorganic nitrogen during periods when there is a high risk of nitrogen loss (Congreves et al., 2013, 2014; Congreves and Van Eerd, 2015). A finer-scale understanding of how amendments influence the temporal dynamics for soil inorganic nitrogen availability is key for linking its influence to aspects of soil health i.e., enhancing nutrient reservoirs for plant growth, improving nitrogen sequestration for mitigating losses to the environment, and the balance between these soil functions, etc.
Manure amendments in vegetable cropping systems were commonly researched (Table 1). In some cases, poultry manure amendments increased soil inorganic nitrogen levels during the growing season, which translated to increased vegetable crop yields (Ninh et al., 2015). Yet, due to the nitrogen rich and the readily mineralizable nature of poultry manure (i.e., low C:N ratios), others cautioned an increased potential for nitrate leaching after poultry litter amendments, and suggested that poultry litter should be mixed with other high carbon material (i.e., compost, yard waste, leaves) to reduce the risk for nitrogen losses (Evanylo et al., 2008; Hepperly et al., 2009). Although poultry manure tends to be a rich source of nitrogen, which may bode well for offsetting fertilizer demands for vegetable crops—and most tend to require high levels of nitrogen fertilizer (Congreves and Van Eerd, 2015)—it may also prime soil microbial biomass, resulting in excess mineralization of soil nitrogen stocks (Fontaine et al., 2003). Mixing poultry manure with higher C:N material, such as composted material, may promote a more moderated mineralization response sufficient for crop production.
Cover Crops
Cover crops are plants that are grown between the periods when main cash crops are produced (when the soil would otherwise be left bare) for purposes other than harvesting for direct commercial value, i.e., for altering soil physical, chemical or biological properties, to minimize erosion, or to improve soil fertility. While it has been long known that cover crops can influence soil properties (Masiunas, 1998), there are a number of variables that determine the resulting effects, such as the species mixture and composition, frequency of cover crops in rotation, timing of planting, timing of termination, surface-placed vs. incorporation of cover crop residues, selection of species that overwinter vs. winterkill in cold climates, different growth forms or nutrient acquisition strategies, etc.
Our findings agree with a previous literature review focused on cover crops by McDaniel et al. (2014) that included field crops and vegetable crops. However, based on our analysis, the influence of cover crops on most biological aspects of soil health were not marked (Figure 3). Although not always representative of total microbial biomass, the diversity of soil microorganisms might be more important for soil health by providing either redundant or distinct microbial functions for decomposition and nutrient cycling. Interestingly, after a 5-year conversion from conventional to organic vegetable production—which included cover cropping—the soil microbial biomass was similar or lower under organic compared to the conventional management but the functional diversity of the microbial communities differed (Bending et al., 2004). Changes in microbial community are likely driven by a number of factors; recent research demonstrated successional changes are driven by season, by location within the soil profile (i.e., bulk soil or rhizosphere), and by the previous crop (Maul et al., 2014). Not only were there quantitative differences in bacterial (16s) rDNA in an intensively managed tomato system (Maul et al., 2014), but there were also differences in soil microbial community structure when vegetables were produced after rye or vetch cover crops compared to plastic mulch without a cover crop (plasticulture being a common practice for vegetable cropping systems). However, despite the changes in soil biological indices, it did not translate into differences in subsequent tomato yield (Maul et al., 2014). Thus, soil management may influence certain aspects of soil functioning (i.e., the microbial functional diversity underlying nutrient cycling) without influencing other aspects of soil functioning (i.e., crop production), emphasizing the complex interplay among factors that regulate various capacities of the soil to function.
An earlier meta-analysis focused on cover crops primarily in field cropping systems (though a small number of vegetable trials were considered) demonstrated reductions in soil nitrate leaching due to cover cropping (Tonitto et al., 2006). In the Northern Hemisphere, cover crops are generally seeded in the fall after the main crop is harvested. Oftentimes, this period coincides with relatively high soil nitrate concentrations due to readily mineralizable and N-rich crop residues (Congreves and Van Eerd, 2015), depending on soil type and land use legacy. In Salinas Valley broccoli production, Wyland et al. (1996) nicely illustrated the seasonal fluctuations in soil nitrate concentrations and the effect that cover cropping (lacy phacelia and rye cover crops) had on reducing nitrate leaching compared to conventional practices (fallow) (9.1 and 7.4 vs. 25.5 g -N leached m−2, respectively) using ion exchange membranes. By reducing the potential for nitrate leaching and by conserving nitrogen that would have otherwise been lost, cover crops may indirectly provide nitrogen credits to subsequent vegetable crop production.
Greater yields were observed when broccoli was produced after cover cropping compared to the conventional practice in Salinas Valley, without imposing increased financial costs (Wyland et al., 1996). Similarly in Brazil, vegetable yields were equivalent to or higher with cover crops vs. without (Altieri et al., 2011); in eastern Canada, growing cover crops in a tomato rotation resulted in greater crop profit margins (Belfry et al., 2017) and 7–22% greater crop yields (Chahal and Van Eerd, 2018) compared to conventional management without cover cropping. The yield benefits were likely due to soil nutrient credits provided from the mineralization of cover crop biomass prior to or during tomato production (Belfry et al., 2017). Further, the tomato yield benefits with vs. without cover crops in the crop rotation after 6-years were linked to 8.4–9.3% greater surface soil organic carbon concentrations (Chahal and Van Eerd, 2018). Decreased weed abundance might also contribute to yield benefits, as cover crop mulches may provide a physical barrier to weed growth and thereby improve vegetable crop production (Altieri et al., 2011). Thus, cover crops may benefit multiple soil health functions such as carbon sequestration, mitigating nutrient losses and supporting crop production. Measuring mulitiple soil ecosystem functions and interpreting the influence of cover cropping is not straightforward, however; commercial “overall soil health” tests (such as the Haney Soil Health Test, the Solvita Test, and the Solvita Labile Amino N Test) may not be the most appropriate method, as suggested by Chahal and Van Eerd (2018).
Despite the potential for vegetable yield benefits due to cover crops, we found a number of observations where vegetable crop yields were reduced due to cover cropping (Figure 3). Instances of reduced vegetable crop yields were largely associated with a very short period between cover crop termination and subsequent main crop planting (i.e., vegetable main crops were planted immediately upon cover crop termination), and where there was a marked shift in typical crop planting dates (i.e., a delay of 2–5 weeks after recommended planting dates) (Leavitt et al., 2011). In contrast, the studies which did not show yield penalties (Wyland et al., 1996; Altieri et al., 2011; Belfry et al., 2017) were within the window of typical planting dates but also incorporated a strategic 2 to 6-week period between cover crop termination and main crop planting, which likely improved synchrony between mineralization and crop nutrient demand without delaying recommended crop planting dates. Hence, carefully planning agronomic management of cover crop termination and the timing of subsequent vegetable crop planting may be a determining factor in the successful use of cover crops.
Tillage Systems
Intensive tillage has long been recognized as detrimental to soil health (Carter and Sanderson, 2001; Carter et al., 2009), especially soil physical changes (Jackson et al., 2003). Few articles specifically investigated tillage effects, or explored alternate tillage impacts on soil health indices or crop production in vegetable cropping systems—only five of 60 articles focused on the topic (Table 1). Conservation tillage is difficult to implement in intensive vegetable cropping systems for a number of reasons including: the importance of close soil contact for seed germination, importance of tillage for weed management, and the interference of surface stubble or crop residues with transplant machinery. Despite these issues, Brainard et al. (2013) presents a thoughtful introduction on converting from conventional to reduced tillage (chisel, strip, and no-till) in vegetable cropping systems.
Soil health indicators (e.g., aggregate stability) improved with strip-tillage in a silt loam trial on Rhode Island and, while decreased vegetable yields were noted, the authors posit that improved weed management in their strip tillage treatment might have lessened yield reductions (Pieper et al., 2015). Reduced tillage has been suggested as a viable alternate to conventional tillage in vegetable cropping systems in North Carolina, possibly leading to improved soil conditions (Roper et al., 2017). However, soil health tests such as the Cornell Soil Health Test and the Haney Soil Health Test were unable to distinguish amongst long-term intensive vs. conservation tillage practices (Roper et al., 2017), suggesting the sensitivity of such tests to changes in management may be limited—a finding that echoes the conclusions drawn by Chahal and Van Eerd (2018) for cover cropping systems. Accurate assessment of soil health is critical as a recent study by Tsiafouli et al. (2015) demonstrated that cropping and tillage intensification decreased soil food web diversity with consequences on ecosystem functioning, and this effect was similarly manifested across numerous climatic regions. While a complete transition from intensive tillage to no-till may not be readily practical for vegetable cropping systems, the frequency, depth, and timing of tillage operations could potentially be modified to minimize negative effects on soil health indices.
Putting the Pieces Together: Additive and Synergistic Effects
Although our aggregate meta-analysis (Figure 3) illustrated that components of alternative management practices generally improved soil health, characterizing the additive or synergistic effect of combining different alternative management practices is challenging and much more complex. Few studies directly evaluated the combination of these practices let alone reported on the response to soil physical, chemical, and biological indicators of soil health. In general, for this literature review, indices of soil health improved by incorporating amendments, integrating cover cropping and reducing tillage in intensive vegetable agricultural management. However, the meta-analysis does not capture whether there are additive or synergistic impacts on soil health indices when multiple alternative management practices are implemented.
In combining alternative management practices, one study that focused on Hawaii zucchini production evaluated the combination of sunhemp cover cropping and vermi-compost tea amendments, and found improved nitrogen supply, supression of parasitic nematodes, and enhanced bacterivorous nematodes (Wang et al., 2014). In Michigan, the addition of compost and rye cover cropping in a tomato production system did not affect nematode populations, but increased soil microbial biomass, respiration and crop yields (Nair and Ngouajio, 2012). In a 3-year on-farm comparison of conventional vs. alternate farming systems that included both compost amendments and cover cropping in the San Joaquin Valley of California, increased soil carbon contents were observed (Andrews et al., 2002). Even with disparate amendments and cover crop species (Andrews et al., 2002), the combination of these two alternative management practices resulted in general improvements for soil carbon concentration (9.9 vs. 8 g kg−1), soil nitrogen concentration (0.83 vs. 0.70 g kg−1), microbial biomass carbon (256 vs. 192 mg kg−1), bulk density (1.27 vs. 1.30 g cm−3), and water stable aggregates (83.1 vs. 82.8 %).
Research in Salinas Valley, California determined that both soil carbon and microbial biomass carbon increased with yard-waste compost amendments and cover cropping in a vegetable crop rotation on a sandy soil (Brennan and Acosta-Martinez, 2017). But, cover cropping had a greater influence than the amendment on the soil food web; therefore, the authors recommended that farmers in the region should focus more on cover cropping than compost additions to improve soil health (Brennan and Acosta-Martinez, 2017). Other research in Salinas Valley reported on the potential of nematodes to be an integrative indicator of soil health because soils with cover crop treatments resulted in a greater variety of nematode types without impacting crop yields (Ferris et al., 2012). Further research demonstrated that compost amendments combined with cover cropping treatments had a subtle increase or no effect on soil phosphorus dynamics, possibly due to relatively high background levels of soil phosphorous (Maltais-Landry et al., 2015). However, when poultry litter compost was applied to the soil as a nitrogen fertilizer (without a cover crop), it resulted in even higher phosphorus levels that were considered excessive (Maltais-Landry et al., 2015). Therefore, investigating the use of cover crops for not only phosphorus mobilization but also as catch crops has been suggested (Maltais-Landry et al., 2016).
Three publications in our literature database investigated the integration of tillage systems and amendment treatments; one on a sandy loam in Belgium (Willekens et al., 2014) and the others on a silt loam in Illinois (Ugarte and Wander, 2013; Ugarte et al., 2013). Soil biological health indices sometimes improved following the transition to alternative management (reduced tillage); however, there were no observed differences in soil physical or chemical attributes among organic amendment or disturbance treatments (Ugarte and Wander, 2013). A soil biological indicator, nematode composition was found to change seasonally but also with management history where communities were more abundant in the less disturbed treatments (Ugarte et al., 2013). In Belgium, both the addition of compost and reduced tillage increased surface soil carbon concentration and soil microbial bacteria and fungi concentrations without affecting crop yields, but the reduced tillage system was considered more important than the compost amendments for its ability to improve soil health indices (Willekens et al., 2014).
In our literature review, only two trials included all four components of alternative management practices as part of their research. First, in southern Uruguay, on small holding farms with silt clay loam soils or clay soils, a multi-year study was undertaken to investigate adapting multiple alternative management practices to individual farm issues (Alliaume et al., 2013). Initial measurements from this study reported that 20-years of intensive vegetable production decreased the proportion of soil aggregates, available water content and soil carbon compared to a paired undisturbed reference soil (Alliaume et al., 2013). Shifting management practices (which included decreasing crop area, changing field slope, additions of green manure, or changes to crop rotations) increased both labor productivity (Dogliotti et al., 2014) and vegetable yield, while also improving soil health through increased soil carbon concentrations (Alliaume et al., 2013). On a sandy loam trial in Denmark, alternative management options were also being investigated for reducing inputs while improving nutrient cycling and pest management through the use of organic matter amendments, cover crops and reduced tillage (Thorup-Kristensen et al., 2012). In their study, vegetable yields were not impacted and there were no significant differences in pests or diseases among organic and conventional management practices; however, the use of fertility building cover crops, rather than the addition of amendments, were found to improve the soil nutrient balances (Thorup-Kristensen et al., 2012).
Theoretical Framework for Integrating Alternative Management Practices
Based on the relative influence that each alternative management practice had on various aspects of soil health (Figures 2, 3) and the literature review of the synergistic or additive effects described herein, we provide a decision framework for farmers and policy makers to consider when adopting alternative management practices: (i) crop rotation (ii) soil amendments (iii) cover crops, and (iv) reduced tillage (Figure 4). Crop rotation forms the basis, while organic amendments appear to be the first key to improving soil physical, chemical, and biological health indices. Cover crops offer additional benefits such as weed suppression, mitigating nitrate loss, or countering excessive levels of nutrients from soil amendments. Reduced tillage shows some promise in improving soil health, but may be considered after amendments and cover crops due to lack of research or lack of viable tillage alternatives. As illustrated by our conceptual model (Figure 4), we hypothesize that the greatest improvements in soil functioning under intensive vegetable production can be achieved by systematically combining different alternative management practices. In other words, the more pieces of this management puzzle are put together, the greater the corresponding improvement in soil health may ensue (Figure 4). In contrast to strictly implementing organic agriculture practices that limit the amounts and types of external inputs—thereby risking yield reductions (Seufert et al., 2012)—this blended approach may reduce the reliance on external inputs (e.g., fertilization, irrigation, herbicides, and pesticides) to maintain or improve both soil health and crop yields in intensive vegetable cropping systems.
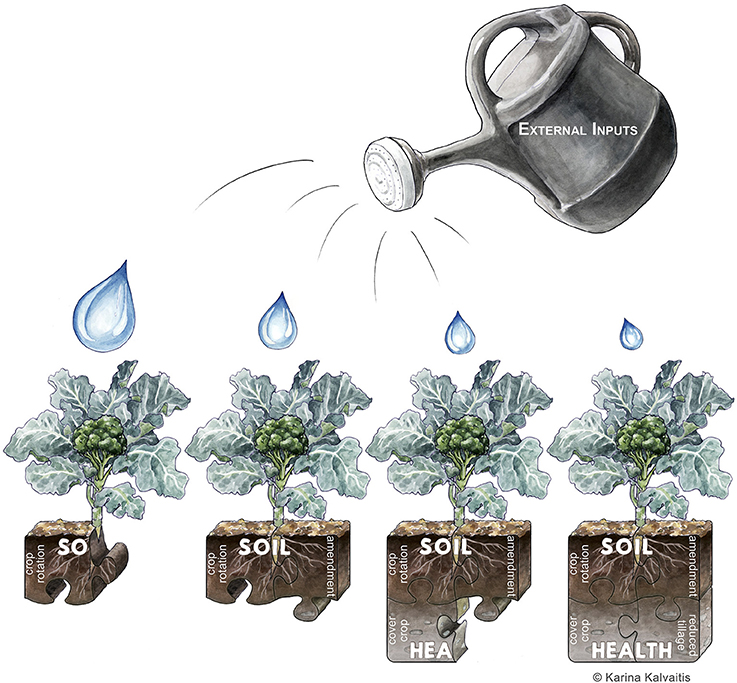
Figure 4. Conceptual model toward improved soil health in intensive vegetable cropping systems. Published with permission from artist.
The incorporation of alternative practices into conventional vegetable cropping systems may be viewed as a melding between organic and conventional ideologies, and it may represent a direction toward increasing soil health indices without compromising productivity in intensive cropping systems. Organic agricultural principles typically consider environmental factors such as biodiversity, integration, natural plant nutrition and pest management, etc. and often include amendments, green manure, or cover cropping, or reduced forms of tillage—practices that were historically alternative to conventional practices. However, future sustainable food production may not be classified as wholly organic or conventional, but rather a combination of both ideologies. Our results provide a clear example of how alternative practices (that may seem organic-based in nature) might be positively integrated into conventional cropping systems to improve soil health indices, and possibly contribute toward improving the sustainability of intensive food production.
Knowledge Gaps and Limitations
Our meta-analysis was limited based on the results from other published research. This constraint yielded an uneven distribution of crop types. The frequency of certain vegetable crop types that were studied in published literature (Figure 1) may have reflected market trends, and/or implies that research on soil health research may be particularly urgent for crop types that tend to have a high nutrient (and fertilizer) demand (i.e., solanaceous, cole, corn, and cucurbit crop typically require 120 to > 200 kg N ha−1), thereby emphasizing the importance of researching soil nutrient reservoirs as an aspect of soil health.
A number of research gaps appeared as a consequence of this literature review. There is a notable absence of long-term studies (10–20-years); while this is understandable due to the elevated logistical and monetary resources that are needed to fund long-term research, maintaining long-term trials in vegetable production will improve the scientific capacity to measure differences in soil health indices. Because long periods are often required before changes in soil can be detected, the knowledge and understanding of ecology, soil science, and plant science has benefited from a number of long-term ecological research (LTER) trials (Hobbie et al., 2009). In the field of agriculture, LTER has mainly focused on field cropping systems; long-term trials focused on vegetable cropping systems are lacking. This limits our ability to accurately project how management and climate change may influence food production and soil health indices in the long run.
Results from studies on intercropping were also notably absent in the literature review. Intercropping, or polycropping, could be an important component to soil health in vegetable cropping systems due to the low-acreage and intensive nature of vegetable production; this warrants future research. Determining viable modifications or alternate tillage approaches for specific vegetable systems remains a conspicuous research gap and will require extensive further research.
Another notable research gap is the limited knowledge on the magnitude of greenhouse gas emissions in vegetable cropping systems. Linking soil health indices or management to greenhouse gas emissions in vegetable cropping systems is needed to better understand the contribution of vegetable crops to global greenhouse gas inventories, and to develop improved strategies for mitigating emissions in intensive cropping systems. Further, endeavors to characterize soil health indices at the molecular level, like organic matter composition, are limited. An improved understanding of stable soil organic matter formation, soil organic matter accumulation and loss is needed to develop improved soil conservation strategies for intensive vegetable cropping systems.
Conclusions
Vegetable cropping systems are inherently intensive by nature and therefore present considerable concern for soil degradation and crop resiliency. Previous research has demonstrated a dilemma for this type of nutrient-demanding cropping system: while implementing organic management is a convincing option for improving soil health, organic management significantly risks vegetable crop yield reductions due to restrictions on the amounts and types of external inputs. Instead of continuing the ideologically disparate “organic vs. conventional” argument, we evaluated the influence of “alternative” management options that included soil amendments, cover cropping, and reduced tillage—practices that resemble organic or conservation agriculture but without imposing external input limitations—as compared to conventional management on soil health indices and crop production in intensive vegetable production. Via a systematic review, alternative management practices generally improved physical, chemical, and biological aspects of soil health without negatively affecting vegetable crop yields, as compared to conventional management. Soil amendments tended to improve soil physical, chemical, and biological properties to the greatest extent; while the incorporation of cover crops reduced nitrate leaching, increased soil carbon levels, and weed suppression. It is evident that reduced tillage systems remain a challenge for vegetable crop production. Our results indicate that future sustainable vegetable cropping systems may embody a blend of management ideologies (i.e., organic and conventional) in order to best maintain or improve soil ecosystem functioning without negatively impacting crop productivity. While we hypothesize that multiple alternative management practices may have an additive or synergistic effect on soil health and crop productivity (Figure 4), more research is needed to better characterize the complex interactions among alternative management practices and their influence on vegetable cropping systems. Researchers are encouraged to consider this relatively understudied sub-field of agriculture to contribute to the development of sustainable food production; and researchers and growers alike are urged to focus on management practices that build soil health and simultaneous reduce the reliance on external inputs in vegetable cropping systems.
Author Contributions
KC conceptualized the review, secured the funding, collected the literature, and co-wrote the paper. CN conducted the analyses, helped in collecting literature, made the figures, and co-wrote the paper.
Conflict of Interest Statement
The authors declare that the research was conducted in the absence of any commercial or financial relationships that could be construed as a potential conflict of interest.
Acknowledgments
The authors sincerely thank the Soil Health Institute (USA) for the funds awarded to support this literature review. Special thanks to graphic artist Karina Kalvaitis for illustrating the conceptual figure.
References
Abawi, G. S., and Widmer, T. L. (2000). Impact of soil health management practices on soilborne pathogens, nematodes and root diseases of vegetable crops. Appl. Soil Ecol. 15, 37–47. doi: 10.1016/S0929-1393(00)00070-6
Agneessens, L., De Waele, J., and De Neve, S. (2014). Review of alternative management options of vegetable crop residues to reduce nitrate leaching in intensive vegetable rotations. Agronomy 4, 529–555. doi: 10.3390/agronomy4040529
Alliaume, F., Rossing, W. A. H., García, M., Giller, K. E., and Dogliotti, S. (2013). Changes in soil quality and plant available water capacity following systems re-design on commercial vegetable farms. Eur. J. Agron. 46, 10–19. doi: 10.1016/j.eja.2012.11.005
Altieri, M. A., Lana, M. A., Bittencourt, H. V., Kieling, A. S., Comin, J. J., and Lovato, P. E. (2011). Enhancing crop productivity via weed suppression in organic no-till cropping systems in Santa Catarina, Brazil. J. Sustain. Agric. 35, 855–869. doi: 10.1080/10440046.2011.588998
Andrews, S. S., Mitchell, J. P., Mancinelli, R., Karlen, D. L., Hartz, T. K., Horwath, W. R., et al. (2002). On-farm assessment of soil quality in California's central valley. Agron. J. 94, 12–23. doi: 10.2134/agronj2002.1200
Bandick, A. K., and Dick, R. P. (1999). Field management effects on soil enzyme activities. Soil Biol. Biochem. 31, 1471–1479. doi: 10.1016/S0038-0717(99)00051-6
Baum, C., ElTohamy, W., and Gruda, N. (2015). Increasing the productivity and product quality of vegetable crops using arbuscular mycorrhizal fungi: a review. Sci. Horticult. 187, 131–141. doi: 10.1016/j.scienta.2015.03.002
Baumann, K., Dignac, M. F., Rumpel, C., Bardoux, G., Sarr, A., Steffens, M., et al. (2013). Soil microbial diversity affects soil organic matter decomposition in a silty grassland soil. Biogeochemistry 114, 201–212. doi: 10.1007/s10533-012-9800-6
Belfry, K. D., Trueman, C., Vyn, R. J., Loewen, S. A., and Van Eerd, L. L. (2017). Winter cover crops on processing tomato yield, quality, pest pressure, nitrogen availability, and profit margins. PLoS ONE 12:e0180500. doi: 10.1371/journal.pone.0180500
Bending, G. D., Turner, M. K., Rayns, F., Marx, M. C., and Wood, M. (2004). Microbial and biochemical soil quality indicators and their potential for differentiating areas under contrasting agricultural management regimes. Soil Biol. Biochem. 36, 1785–1792. doi: 10.1016/j.soilbio.2004.04.035
Beniston, J. W., Lal, R., and Mercer, K. L. (2016). Assessing and managing soil quality for urban agriculture in a degraded vacant lot soil. Land Degradation Dev. 27, 996–1006. doi: 10.1002/ldr.2342
Bonanomi, G., D'Ascoli, R., Scotti, R., Gaglione, S. A., Caceres, M. G., Sultana, S., et al. (2014). Soil quality recovery and crop yield enhancement by combined application of compost and wood to vegetables grown under plastic tunnels. Agricult. Ecosyst. Environ. 192, 1–7. doi: 10.1016/j.agee.2014.03.029
Brainard, D. C., Peachey, R. E., Haramoto, E. R., Luna, J. M., Brainard, D. C., Peachey, R. E., et al. (2013). Weed ecology and nonchemical management under strip-tillage : implications for Northern, U.S. Veg. Crop. Syst. Weed Technol. 27, 218–230. doi: 10.1614/WT-D-12-00068.1
Brennan, E. B., and Acosta-Martinez, V. (2017). Cover cropping frequency is the main driver of soil microbial changes during six years of organic vegetable production. Soil Biol. Biochem. 109, 188–204. doi: 10.1016/j.soilbio.2017.01.014
Brennan, E. B., and Boyd, N. S. (2012). Winter cover crop seeding rate and variety affects during eight years of organic vegetables: I. cover crop biomass production. Agron. J. 104, 684–698. doi: 10.2134/agronj2011.0330
Carter, M., and Sanderson, J. (2001). Influence of conservation tillage and rotation length on potato productivity, tuber disease and soil quality parameters on a fine sandy loam in eastern Canada. Soil Til. Research 63, 1–13. doi: 10.1016/S0167-1987(01)00224-0
Carter, M. R., Noronha, C., Peters, R. D., and Kimpinski, J. (2009). Influence of conservation tillage and crop rotation on the resilience of an intensive long-term potato cropping system: restoration of soil biological properties after the potato phase. Agric. Ecosyst. Environ. 133, 32–39. doi: 10.1016/j.agee.2009.04.017
Cassidy, E. S., West, P. C., Gerber, J. S., and Foley, J. A. (2013). Redefining agricultural yields: from tonnes to people nourished per hectare. Environ. Res. Lett. 8:34015. doi: 10.1088/1748-9326/8/3/034015
Chahal, I., and Van Eerd, L. L. (2018). Evaluation of commercial soil health tests using a medium-term cover crop experiment in a humid, temperate climate. Plant Soil 427, 351–367. doi: 10.1007/s11104-018-3653-2
Collange, B., Navarrete, M., Peyre, G., Mateille, T., and Tchamitchian, M. (2011). Root-knot nematode (Meloidogyne) management in vegetable crop production: The challenge of an agronomic system analysis. Crop Protect. 30, 1251–1262. doi: 10.1016/j.cropro.2011.04.016
Congreves, K. A., and Van Eerd, L. L. (2015). Nitrogen cycling and management in intensive horticultural systems. Nutr. Cycling Agroecosyst. 102, 299–318. doi: 10.1007/s10705-015-9704-7
Congreves, K. A., Voroney, R. P., and Van Eerd, L. L. (2014). Amending soil with used cooking oil to reduce nitrogen losses after cole crop harvest: a 15N study. Nutr. Cycling Agroecosyst. 100, 257–271. doi: 10.1007/s10705-014-9643-8
Congreves, K. A., Vyn, R. J., and Van Eerd, L. L. (2013). Evaluation of post-harvest organic carbon amendments as a strategy to minimize nitrogen losses in cole crop production. Agronomy 3, 181–199. doi: 10.3390/agronomy3010181
Creamer, N. G., and Baldwin, K. R. (2000). An evaluation of summer cover crops for use in vegetable production systems in North Carolina. Hortscience 35, 600–603.
D'Hose, T., Cougnon, M., De Vliegher, A., Vandecasteele, B., Viaene, N., Cornelis, W., et al. (2014). The positive relationship between soil quality and crop production: a case study on the effect of farm compost application. Appl. Soil Ecol. 75, 189–198. doi: 10.1016/j.apsoil.2013.11.013
Dogliotti, S., García, M. C., Peluffo, S., Dieste, J. P., Pedemonte, A. J., Bacigalupe, G. F., et al. (2014). Co-innovation of family farm systems: a systems approach to sustainable agriculture. Agric. Syst. 126, 76–86. doi: 10.1016/j.agsy.2013.02.009
Doran, J. W., and Zeiss, M. R. (2000). Soil health and sustainability: managing the biotic component of soil quality. Appl. Soil Ecol. 15, 3–11. doi: 10.1016/S0929-1393(00)00067-6
Doran, J. W., Sarrantonio, M., and Liebig, M. A. (1996). Soil health and sustainability. Adv. Agron. 56, 1–54. doi: 10.1016/S0065-2113(08)60178-9
Doran, J. W. (2002). Soil health and global sustainability: translating science into practice. Agric. Ecosyst. Environ. 88, 119–127. doi: 10.1016/S0167-8809(01)00246-8
Drewry, J., Curran-Cournane, F., Taylor, M., and Lynch, B. (2015). “Soil quality monitoring across land uses in four regions: implications for reducing nutrient losses and for national reporting,” in Moving Farm Systems to Improved Nutrient Attenuation, 1–14.
Dumontet, S., Cavoski, I., Ricciuti, P., Mondelli, D., Jarrar, M., Pasquale, V., et al. (2017). Metabolic and genetic patterns of soil microbial communities in response to different amendments under organic farming system. Geoderma 296, 79–85. doi: 10.1016/j.geoderma.2017.02.025
Evanylo, G., Sherony, C., Spargo, J., Starner, D., Brosius, M., and Haering, K. (2008). Soil and water environmental effects of fertilizer-, manure-, and compost-based fertility practices in an organic vegetable cropping system. Agric. Ecosyst. Environ. 127, 50–58. doi: 10.1016/j.agee.2008.02.014
FAO (2017). Food and Agriculture Organization of the United Nations, Statistics Division. Available online at: http://www.fao.org/statistics/en/
Ferreras, L., Gomez, E., Toresani, S., Firpo, I., and Rotondo, R. (2006). Effect of organic amendments on some physical, chemical and biological properties in a horticultural soil. Bioresour. Technol. 97, 635–640. doi: 10.1016/j.biortech.2005.03.018
Ferris, H., Sánchez-Moreno, S., and Brennan, E. B. (2012). Structure, functions and interguild relationships of the soil nematode assemblage in organic vegetable production. Appl. Soil Ecol. 61, 16–25. doi: 10.1016/j.apsoil.2012.04.006
Fontaine, S., Mariotti, A., and Abbadie, L. (2003). The priming effect of organic matter: a question of microbial competition? Soil Biol. Biochem. 35, 837–843. doi: 10.1016/S0038-0717(03)00123-8
Gardner, M., and Sarrantonio, M. (2012). Cover crop root composition and density in a long-term vegetable cropping system trial. J. Sustain. Agricult. 36, 719–737. doi: 10.1080/10440046.2012.672548
Gaudin, A. C., Tolhurst, T. N., Ker, A. P., Janovicek, K., Tortora, C., Martin, R. C., et al. (2015). Increasing crop diversity mitigates weather variations and improves yield stability. PLoS ONE 10:e0113261. doi: 10.1371/journal.pone.0113261
Hedges, L. V., Gurevitch, J., and Curtis, P. S. (1999). The meta-analysis of response ratio in experimental ecology. Ecology 80, 1150–1156.
Hepperly, P., Lotter, D., Ulsh, C. Z., Seidel, R., and Reider, C. (2009). Compost, manure and synthetic fertilizer influences crop yields, soil properties, nitrate leaching and crop nutrient content. Compost Sci. Utilization 17, 117–126. doi: 10.1080/1065657X.2009.10702410
Hobbie, J. E., Carpenter, S. R., Grimm, N. B., Gosz, J. R., and Seastedt, T. R. (2009). The US long term ecological research program. BioScience 53, 21–32. doi: 10.1641/0006-3568(2003)053[0021:TULTER]2.0.CO;2
Hobbs, P. R. (2007). Conservation agriculture: what is it and why is it important for future sustainable food production? J. Agric. Sci. 145, 127–137. doi: 10.1017/S0021859607006892
Jackson, L. E., Calderon, F. J., Steenwerth, K. L., Scow, K. M., and Rolston, D. E. (2003). Responses of soil microbial processes and community structure to tillage events and implications for soil quality. Geoderma 114, 305–317. doi: 10.1016/S0016-7061(03)00046-6
Jackson, L., Ramirez, I., Yokota, R., Fennimore, S., Koike, S., Henderson, D., et al. (2004). On-farm assessment of organic matter and tillage management on vegetable yield, soil, weeds, pests, and economics in California. Agric. Ecosyst. Environ. 103, 443–463. doi: 10.1016/j.agee.2003.11.013
Karlen, D. L., Mausbach, M. J., Doran, J. W., Cline, R. G., Harris, R. F., and Schuman, G. E. (1997). Soil quality: a concept, definition, and framework for evaluation (A Guest Editorial). Soil Sci. Soc. Am. J. 61, 4. doi: 10.2136/sssaj1997.03615995006100010001x
Ketcheson, J. W. (1980). Long-range effects of intensive cultivation and monoculture on the quality of Southern Ontario soils. Can. J. Soil Sci. 60, 403–410. doi: 10.4141/cjss80-045
Knowler, D., and Bradshaw, B. (2007). Farmers' adoption of conservation agriculture: a review and synthesis of recent research. Food Policy 32, 25–48. doi: 10.1016/j.foodpol.2006.01.003
Leavitt, M. J., Sheaffer, C. C., Wyse, D. L., and Allan, D. L. (2011). Rolled winter rye and hairy vetch cover crops lower weed density but reduce vegetable yields in no-tillage organic production. HortScience 46, 387–395.
Maltais-Landry, G., Scow, K., Brennan, E., and Vitousek, P. (2015). Long-term effects of compost and cover crops on soil phosphorus in Two California agroecosystems. Soil Sci. Soc. Am. J. 79, 688–697. doi: 10.2136/sssaj2014.09.0369
Maltais-Landry, G., Scow, K., Brennan, E., Torbert, E., and Vitousek, P. (2016). Higher flexibility in input N: P ratios results in more balanced phosphorus budgets in two long-term experimental agroecosystems. Agricult. Ecosyst. Environ. 223, 197–210. doi: 10.1016/j.agee.2016.03.007
Marinari, S., Mancinelli, R., Brunetti, P., and Campiglia, E. (2015). Soil quality, microbial functions and tomato yield under cover crop mulching in the Mediterranean environment. Soil Tillage Res. 145, 20–28. doi: 10.1016/j.still.2014.08.002
Masiunas, J. B. (1998). Journal of vegetable crop production production of vegetables using cover crop and living mulches—a review. J. Veg. Crop Production 4, 11–31. doi: 10.1300/J068v04n01_03
Maul, J. E., Buyer, J. S., Lehman, R. M., Culman, S., Blackwood, C. B., Roberts, D. P., et al. (2014). Microbial community structure and abundance in the rhizosphere and bulk soil of a tomato cropping system that includes cover crops. Appl. Soil Ecol. 77, 42–50. doi: 10.1016/j.apsoil.2014.01.002
Maynard, D. G., Paré, D., Thiffault, E., Lafleur, B., Hogg, K. E., and Kishchuk, B. (2014). How do natural disturbances and human activities affect soils and tree nutrition and growth in the Canadian boreal forest? Environ. Rev. 22, 161–178. doi: 10.1139/er-2013-0057
McDaniel, M. D., Grandy, A. S., Tiemann, L. K., and Weintraub, M. N. (2014). Crop rotation complexity regulates the decomposition of high and low quality residues. Soil Biol. Biochem. 78, 243–254. doi: 10.1016/j.soilbio.2014.07.027
Mkhabela, M. S., and Warman, P. R. (2005). The influence of municipal solid waste compost on yield, soil phosphorus availability and uptake by two vegetable crops grown in a Pugwash sandy loam soil in Nova Scotia. Agric. Ecosyst. Environ. 106, 57–67. doi: 10.1016/j.agee.2004.07.014
Mohr, R. M., Volkmar, K., Derksen, D. A., Irvine, R. B., Khakbazan, M., McLaren, D. L., et al. (2011). Effect of rotation on crop yield and quality in an irrigated potato system. Am. J. Potato Res. 88, 346–359. doi: 10.1007/s12230-011-9200-9
Morra, L., Pagano, L., Iovieno, P., Baldantoni, D., and Alfani, A. (2010). Soil and vegetable crop response to addition of different levels of municipal waste compost under Mediterranean greenhouse conditions. Agron. Sustain. Dev. 30, 701–709. doi: 10.1051/agro/2009046
Munkholm, L. J., Heck, R. J., and Deen, B. (2013). Long-term rotation and tillage effects on soil structure and crop yield. Soil Till. Res. 127, 85–91. doi: 10.1016/j.still.2012.02.007
Nair, A., and Ngouajio, M. (2012). Soil microbial biomass, functional microbial diversity, and nematode community structure as affected by cover crops and compost in an organic vegetable production system. Appl. Soil Ecol. 58, 45–55. doi: 10.1016/j.apsoil.2012.03.008
Ninh, H. T., Grandy, A. S., Wickings, K., Snapp, S. S., Kirk, W., and Hao, J. (2015). Organic amendment effects on potato productivity and quality are related to soil microbial activity. Plant Soil 386, 223–236. doi: 10.1007/s11104-014-2223-5
Ozores-Hampton, M., Stansly, P. A., and Salame, T. P. (2011). Soil chemical, physical, and biological properties of a sandy soil subjected to long-term organic amendments. J. Sustain. Agric. 35, 243–259. doi: 10.1080/10440046.2011.554289
Pieper, J. R., Brown, R. N., and Amador, J. A. (2015). Effects of three conservation tillage strategies on yields and soil health in a mixed vegetable production system. HortScience 50, 1770–1776.
Power, A. G. (2010). Ecosystem services and agriculture: tradeoffs and synergies. Philos. Trans. R. Soc. Lond. B Biol. Sci. 365, 2959–2971. doi: 10.1098/rstb.2010.0143
Roper, W. R., Osmond, D. L., Heitman, J. L., Wagger, M. G., and Reberg-Horton, S. C. (2017). Soil health indicators do not differentiate among agronomic management systems in North Carolina Soils. Soil Sci. Soc. Am. J. 81, 828. doi: 10.2136/sssaj2016.12.0400
Saini, G. R., and Grant, W. J. (1980). Long-term effects of intensive cultivation on soil quality in the potato-growing areas of New Brunswick (Canada) and Maine (U.S.A.). Can. J. Soil Sci. 60, 421–428. doi: 10.4141/cjss80-047
Schutter, M. E., Sandeno, J. M., and Dick, R. P. (2001). Seasonal, soil type, and alternative management influences on microbial communities of vegetable cropping systems. Biol. Fertil. Soils 34, 397–410. doi: 10.1007/s00374-001-0423-7
Scialabba, N. E.-H., and Müller-Lindenlauf, M. (2010). Organic agriculture and climate change. Renew. Agric. Food Syst. 25, 158–169. doi: 10.1017/S1742170510000116
Seufert, V., Ramankutty, N., and Foley, J. A. (2012). Comparing the yields of organic and conventional agriculture. Nature 485, 229–232. doi: 10.1038/nature11069
Smith, R., Cahn, M., Hartz, T., Love, P., and Farrara, B. (2016). Nitrogen dynamics of cole crop production: implications for fertility management and environmental protection. HortScience 51, 1586–1591. doi: 10.21273/HORTSCI11335-16
Suddick, E. C., and Six, J. (2013). An estimation of annual nitrous oxide emissions and soil quality following the amendment of high temperature walnut shell biochar and compost to a small scale vegetable crop rotation. Sci. Total Environ. 465, 298–307. doi: 10.1016/j.scitotenv.2013.01.094
Thorup-Kristensen, K., Dresbøll, D. B., and Kristensen, H. L. (2012). Crop yield, root growth, and nutrient dynamics in a conventional and three organic cropping systems with different levels of external inputs and N re-cycling through fertility building crops. Eur. J. Agron. 37, 66–82. doi: 10.1016/j.eja.2011.11.004
Tiemann, L. K., Grandy, A. S., Atkinson, E. E., Marin-Spiotta, E., and McDaniel, M. D. (2015). Crop rotational diversity enhances belowground communities and functions in an agroecosystem. Ecol. Lett. 18, 761–771. doi: 10.1111/ele.12453
Tilman, D., Cassman, K., Matson, P., and Naylor, R. (2002). Agricultural sustainability and intensive production practices. Nature 418, 671–677. doi: 10.1038/nature01014
Tonitto, C., David, M. B., and Drinkwater, L. E. (2006). Replacing bare fallows with cover crops in fertilizer-intensive cropping systems: a meta-analysis of crop yield and N dynamics. Agric. Ecosyst. Environ. 112, 58–72. doi: 10.1016/j.agee.2005.07.003
Tsiafouli, M. A., Thébault, E., Sgardelis, S. P., de Ruiter, P. C., van der Putten, W. H., Birkhofer, K., et al. (2015). Intensive agriculture reduces soil biodiversity across Europe. Global Change Biol. 21, 973–985. doi: 10.1111/gcb.12752
Ugarte, C. M., and Wander, M. M. (2013). The influence of organic transition strategy on chemical and biological soil tests. Renew. Agric. Food Syst. 28, 17–31. doi: 10.1017/S1742170511000573
Ugarte, C. M., Zaborski, E. R., and Wander, M. M. (2013). Nematode indicators as integrative measures of soil condition in organic cropping systems. Soil Biol. Biochem. 64, 103–113. doi: 10.1016/j.soilbio.2013.03.035
Wang, K. H., Radovich, T., Pant, A., and Cheng, Z. (2014). Integration of cover crops and vermicompost tea for soil and plant health management in a short-term vegetable cropping system. Appl. Soil Ecol. 82, 26–37. doi: 10.1016/j.apsoil.2014.05.003
Warman, P. R. (2005). Soil fertility, yield and nutrient contents of vegetable crops after 12 years of compost or fertilizer amendments. Biol. Agric. Hortic. 23, 85–96. doi: 10.1080/01448765.2005.9755310
Willekens, K., Vandecasteele, B., Buchan, D., and De Neve, S. (2014). Soil quality is positively affected by reduced tillage and compost in an intensive vegetable cropping system. Appl. Soil Ecol. 82, 61–71. doi: 10.1016/j.apsoil.2014.05.009
Wyland, L. J., Jackson, L., Chaney, W. E., Klonsky, K., Koike, S. T., and Kimple, B. (1996). Altering surface soil dynamics with winter cover crops in a vegetable cropping system: impacts on yield, nitrate leaching, pests and management costs. Agric. Ecosyst. Environ. 59, 1–17. doi: 10.1016/0167-8809(96)01048-1
Keywords: soil health, vegetable production, carbon sequestration, soil nutrients, organic agriculture, organic amendments, cover crops, reduced tillage
Citation: Norris CE and Congreves KA (2018) Alternative Management Practices Improve Soil Health Indices in Intensive Vegetable Cropping Systems: A Review. Front. Environ. Sci. 6:50. doi: 10.3389/fenvs.2018.00050
Received: 27 March 2018; Accepted: 22 May 2018;
Published: 12 June 2018.
Edited by:
Luuk Fleskens, Wageningen University & Research, NetherlandsReviewed by:
Rosa Francaviglia, Consiglio per la Ricerca in Agricoltura e l'analisi Dell'economia Agraria (CREA), ItalyRok Mihelic, University of Ljubljana, Slovenia
Copyright © 2018 Norris and Congreves. This is an open-access article distributed under the terms of the Creative Commons Attribution License (CC BY). The use, distribution or reproduction in other forums is permitted, provided the original author(s) and the copyright owner are credited and that the original publication in this journal is cited, in accordance with accepted academic practice. No use, distribution or reproduction is permitted which does not comply with these terms.
*Correspondence: Katelyn A. Congreves, kate.congreves@usask.ca