- 1Agricultural Centre for Sustainable Energy Systems (ACSES), Department of Agriculture and the Environment, Harper Adams University, Newport, United Kingdom
- 2Rika BioFuels Limited, Middlesex, United Kingdom
Oat husks are produced during the milling process of oats. Oat husks are a lignocellulosic material that have the potential for valorization thereby improving the circular economy of agricultural by-products. However, due to the high lignocellulosic content, there are limited valorization pathways for oat husks. To improve the anaerobic digestibility of oat husks, pre-treatment was investigated as a method to aid valorization. A novel extrusion process was used in an attempt to fragment the lignocellulosic structure of oat husks prior to anaerobic digestion. The extrusion pre-treatment was investigated to determine the effect it may have on altering the methane yield and digestibility of oat husks. Biochemical methane potential assays were undertaken using oat husks with no pre-treatment and extruded oat husks. These assays demonstrated that extruded oat husks produced a significantly higher methane yield of 264 ml/gVS fed, which was 27% greater than the methane yield produced from the untreated oat husks. Similarly, the total solids degradation was also significantly higher for extruded oat husks treatment compared to the untreated oat husks. Overall, the extrusion process demonstrated an increased methane yield for oat husks compared to previously published data. The biomethane potential tests suggest that extruded oat husks would be a feedstock suitable for anaerobic digestion.
Introduction
As the European Union (EU) moves toward a climate-neutral economy, the 2030 Climate and Energy Policy Framework has set targets for EU members to reduce their greenhouse gas emissions by 40% of 1990 emission levels before 2030. The Framework also requires that renewable energy should provide at least 32% of all energy generated and 14% of all transport fuels. To negate the food vs. fuels debate, by 2030 first generation biofuels will be gradually phased out of renewable energy targets (European Commission, 2014). Therefore, anaerobic digestion (AD) plants are considering a wide range of organic feedstocks that can be used for methane production to contribute to achieving these 2030 targets (Dumas et al., 2015). Feedstocks include purpose grown crops, for example, sugar beet, rye grass, and agricultural by-products, for example, chicken manure and straw (Scarlat et al., 2019). Interest is growing in the use of high lignocellulosic feedstock for AD, as these feedstocks are readily available in large quantities and are low cost (Peng et al., 2016).
Globally, 3,700 Mt/year dry matter (DM) of straw is produced predominantly from maize, rice, wheat, soybean, sugarcane, and barley (Bentsen et al., 2014). Within Europe, straw production comprises from barley, wheat, rye, oats, and other combined cereals (Elbersen et al., 2012), with estimated total straw yields for Europe varying from 200 to 457 (with Ukraine) Mt/year DM (Fischer et al., 2010; Scarlat et al., 2010). This variation in total straw DM yields is due to changing regional crop yields, harvested areas and the species grown as it can alter the straw to grain ratio (Scarlat et al., 2010). For example, the straw to grain ratio for wheat can vary from 0.5 to 2.37 kg of straw per kg of grain harvested and oats have a straw to grain ratio of 0.75–2.0 kg of straw per kg of grain harvested (Bedoić et al., 2019). Straw as an agricultural by-product has multiple different uses (Scarlat et al., 2019). Conventionally, straw is used as feed and bedding for animals, frost prevention for overwintering crops and as a mulch and soil remediator (Gallegos et al., 2017; Scarlat et al., 2019). Furthermore, straw can be used for bioenergy production (bioethanol, biodiesel or methane) (Ferreira et al., 2014). The use of straw for bioenergy production is gradually increasing and within the EU its use is most common in Denmark, Spain and then the United Kingdom (Scarlat et al., 2019). Alongside straw production, other cereal by-products are produced from processing the cereal grains. During the milling process, grains have to be dehulled to expose the endosperm (Decker et al., 2014). The husk to grain ratio varies per crop, for example, oat husks produce 0.25–0.32 kg of husk per kg of grain harvested and rice produces 0.04–0.36 kg of husks per kg of grain harvested (Bedoić et al., 2019). Barbieri et al. (2020) estimated that in 2019, Europe produced 10 million tons of wheat husks alone. Husks represent a significant quantity of agricultural by-products with a high lignocellulosic content and low digestibility (Decker et al., 2014). Husks are traditionally used as a human food source (if finely ground) (Decker et al., 2014), as animal bedding and feed (Bledzki et al., 2010). They are also landfilled and incinerated for energy and heat recovery (Marques et al., 2020). Potential future options currently being researched for husk use include as a replacement composite material, as biodegradable packaging material (Bledzki et al., 2010) and in phytochemical production (Marques et al., 2020).
Cereal straws and husks are rich in the structural polysaccharides, cellulose (30–40%) and hemicellulose (20–30%); they also contain large quantities of lignin (15–20%) making it difficult for microbes to digest lignocellulosic substrates anaerobically (Yao et al., 2018; Lui et al., 2019; Barbieri et al., 2020). Hydrolysis of these longer-chain polysaccharides to monomers for biogas production can take several days and is often the rate limiting stage of AD (Lui et al., 2019). Previously published data of biochemical methane potential (BMP) assays of wheat straw (no pre-treatment) demonstrated methane yields ranging from 162 to 233 ml/g volatile solids (VS) (Ferreira et al., 2014; Yao et al., 2018; Lui et al., 2019), depending on the origin and the maturity of the wheat straw (Dumas et al., 2015).
To increase the anaerobic digestibility of these materials, pre-treatment is required (Gomez-Tovar et al., 2012). Various different pre-treatment methods exist and are being further developed to include physical/mechanical, chemical, and/or biological pre-treatments (Gallegos et al., 2017; Abraham et al., 2020). Pre-treatments increase enzyme and microbial access and enhance degradation, often involving particle size reductions which increase surface area, reduce crystallinity, and/or disrupt the lignin-hemicellulose cross-linkages (Dumas et al., 2015). With regard to mechanical processing, there are a number of novel processes methods that have a high technology readiness level 8–9. These include various milling procedures, extrusion, ultrasound, cavitation, irradiation, microwaves, and steam explosion (Schumacher et al., 2014; Lui et al., 2019). Mechanical pre-treatment methods do not create any toxic process compounds, with grinding and milling the most common techniques used (Abraham et al., 2020). Mechanical pre-treatment methods tend to be reliable (Abraham et al., 2020), however they typically have high investment and maintenance costs. They also require high energy inputs and this may have limited their commercial uptake (Gallegos et al., 2017). Numerous studies have investigated a range of mechanical pre-treatment technologies across different agricultural cereal by-products, with many studies demonstrating an increased methane yield from AD in comparison to untreated feedstocks. Gallegos et al. (2017) demonstrated an increased methane yield of 244 ml/gVS fed from ground (<0.2 mm) wheat straw compared to chopped (2 mm) wheat straw of 179 ml/gVS fed. Similarly, Dumas et al. (2015) produced a high methane yield of 306 ml/gVS fed by grinding wheat straw to 759 µm. In continuous digestion trials, as particle size decreased (759–88 µm) methane yield increased, however if the wheat straw was ground further (<88 µm) there was no increase in methane yields noted (Dumas et al., 2015). Furthermore, Ferreira et al. (2014) demonstrated that a similar methane yield can be achieved from chopped wheat straw (30–50 mm) 239 ml/gVS fed compared to ground wheat straw (<1 mm) of 245 ml/gVS fed. Grinding wheat straw too excessively is thought to be detrimental to methane yield as decreased particle size increases the rate of volatile fatty acids, inhibiting methanogenesis (Dumas et al., 2015). Kusch et al. (2011) demonstrated that by grinding oat husks, methane yield was increased to 242 ml/gVS fed compared to the untreated oat husks of 202 ml/gVS fed. This increased methane yield originated from the hemicellulose and cellulose components of the ground oat husks (Kusch et al., 2011).
This research examines a novel mechanical pre-treatment, the Bio-Extruder, developed in Germany (Lehmann UMT GmbH, Pöhl, Germany) and marketed by Rika BioFuels Limited in the United Kingdom. The technology is marketed under the BioExtruder trade name. It consists of a counter rotating dual-screw auger to undertake both mechanical and thermal fragmentation, reducing the structure and particle size of organic materials. The BioExtruder can process a range of different organic feedstocks suitable for AD, including wheat straw, ensiled wheat straw (Gallegos et al., 2017), solid manures (Lehmann and Eberhard, 2012), maize, and other crop silages (Rika BioFuels, 2017). Additionally, it can process feedstocks as received or mixed with digestate to hydrothermally fragment them. With an anticipated 50–60 kW/h required to process a ton of wheat straw fresh weight, the Bio-Extruder’s energy input is low compared to milling technologies. Unlike milling technologies, the Bio-Extruder produces little or no dust, reducing potential fire risk. It also has a small operating footprint and is self-cleaning, with low maintenance costs and an estimated 2–4 years return on investment (Lehmann and Eberhard, 2012; Rika BioFuels, 2017).
Limited research to date has been undertaken using the BioExtruder, particularly for the valorization of oat husks. Therefore, the aim of this research was to test the novel pre-treatment technology for processing United Kingdom grown oat husks as an agricultural by-product that could be valorized through AD. In our research have processed oat husks using the bio-extrusion technology and thereafter, used BMP assays to determine if pre-treatment increased biogas yield and anaerobic digestibility compared to untreated oat husks. The research was intended as a pre-requisite to the inclusion of pre-treated oat husks in a pilot plant anaerobic digester.
Materials and Methods
Extrusion Process
In this research we used a mini 3 kW/h research scale BioExtruder to process 2 kg of oat husks. The 3 kW/h BioExtruder (Figure 1A) was [approximately 1.4 m × 1.2 m × 0.8 m (height × length × width)] mounted onto a wooden pallet to enable moving. A feed hopper was located at the top left-hand side of the BioExtruder to enable feedstocks to be fed to the counter-rotating screws beneath. A counter rotating dual-screw auger was located in the horizontal plane beneath the feed hopper. Figure 1B displays the counter-rotating screw-auger from the largest commercial scale BioExtruder, model number MSZB 110e. The counter rotating dual-auger screws ran continuously to mechanically fragment the feedstocks. The mechanical fragmentation process generates heat within the feedstock, causing thermal fragmentation. The quantity of heat generated is dependent upon the feedstock processed. A combination of mechanical and thermal fragmentation reduced the structure and particle size of the feedstock, which moved down the length of the counter rotating dual-augers and discharged to a collection bucket.
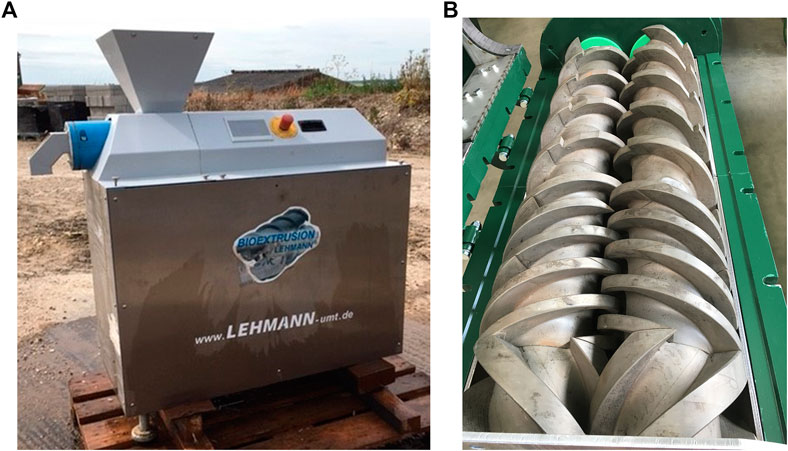
FIGURE 1. A picture of the (A) The mini 3kW/h BioExtruder, (B) the counter rotating dual-screw auger from a commercial scale BioExtruder (model number MSZB 110e).
Oat husks were presoaked prior to processing. This was to improve the flow of the oat husks through the extruder. Dry oat husks tended to drop through the augers too quickly, reducing contact time for fragmentation. Oat husks were soaked in water (30% w/v) for 1 h prior to extrusion. The BioExtruder was fed at a continuous rate, with it taking only a few seconds to process approximately 5 g of oat husks (total 2 kg processed) and a small amount of steam could be seen evaporating from the exit of the BioExtruder with the processed oat husks. The extent of heat generated caused by processing is not known, but less heat was generated with oat husks in comparison to the processing of other dry feedstocks, for example, wheat straw.
Experimental Design
The BMP assays anaerobically digested untreated and extruded oat husks to determine the effect of the extrusion process on biogas yield and digestibility. The experimental design was a 2× factorial comparison of untreated and extruded oat husks. Additionally, cellulose controls (to standardize biological sludge activity biogas yield per gVS fed making it comparable to other published cellulose control data) and blank assays (to determine biogas yield from the digestate alone) were included. All BMP assays were replicated three times (12 BMP assays in total) and distilled water was used to standardize the working volume (51 ml) of all assays. All BMP assays were randomly allocated a bottle number and randomly distributed throughout the incubator. BMP assays were undertaken at mesophilic temperature (35°C) and operated for 46 days until biogas production had ceased.
Preparation of Biochemical Methane Potential Components
Seven days prior to the start of the experiment, 4 L of sewage sludge digestate were collected from the local sewage works and sieved (600 µm) to remove the larger particle and left to degas for 7 days before the experiment commenced.
Experimental Routine
The sewage sludge and two oat husk samples (untreated and extruded) were analyzed for total solids (TS) and VS content, as a proportion of TS content (Table 1) (for methodology, see section Experimental Routine). Each BMP assay was loaded on an inoculum:feed substrate VS ratio of 2:1 (VS added 1.14:0.57 g per assay) (Table 2) (Angelidaki et al., 2009). After BMP assays were assembled, the BMP bottles were sealed, flushed with nitrogen for 10 min to remove air from the headspace and the pressure in each bottle equalized to atmospheric pressure. The bottles were placed in a pre-warmed incubator at 35°C and biogas pressure and volume were measured as required throughout the experiment. Biogas volume and composition (methane and carbon dioxide) were measured using a pressure transducer and micro-GC (see section Experimental Routine). To determine the endpoint of the experiment, biogas pressure readings were taken every 1–2 days between experimental days 34–46. The rate to biogas pressure increase was recorded but the biogas was left within the bottle, as the daily quantity of biogas produced was not sufficient to accurately measure biogas composition. At the end of the experiment, digestate was analyzed for pH, TS, and VS contents (see section Experimental Routine).
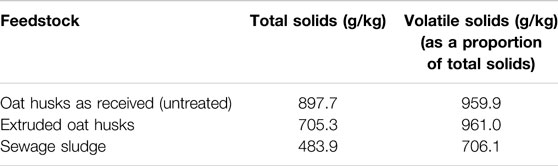
TABLE 1. Compositional analyzes of oat husks as received (untreated) and following the BioExtruder pre-treatment technology.
Chemical Analyzes
Biogas pressure of BMP assays were measured using a pressure transducer (Gems Sensors and Controls, Basingstoke, United Kingdom) fitted with a three-way tap to a hypodermic needle (23G × 1 inch) and a gas syringe (adapted from Theodorou et al., 1994). The pressure transducers’ hypodermic needle was inserted through the butyl rubber stopper of the bottle and the pressure recorded. The biogas was released into the syringe and the volume recorded before discarding the biogas. Mean blank assay biogas volumes were later subtracted from the treatment biogas yields of each treatment to determine the biogas produced from oat husks only. Biogas composition for methane and carbon dioxide concentrations were analyzed using an Agilent 490 micro-GC (Agilent Technologies LDA UK Limited, Stockport, United Kingdom), with dual columns (molsieve column for methane and a CP-PoraPLOT U column for carbon dioxide). The injector and columns were pre-heated to 60 and 80°C, respectively. Biogas was sampled for 10 ms onto the molsieve (backflush set to 34 s) and 100 ms for the PoraPLOT. The sample was transferred using helium and analyzed for 115 s at a pressure of 120 kPa. Biogas volumes were corrected to standard temperature (0°C) and pressure (1 atm).
Digestate pH was analyzed using an integrated pH and temperature probe (Jenway, Stone, United Kingdom) calibrated daily. The TS and VS content of the digestates and feedstocks were analyzed using standard methods (APHA, 1989). VS is expressed as a proportion of the TS content. TS and VS degradation rates were calculated relative to the TS and VS contents entering the experiment (IN) compared to the digestate composition (OUT) at the end of the experiment.
Statistical Analyzes
The experiment was analyzed as a 2× factorial ANOVA with three replicates per treatment. All statistical analyzes were conducted using GenStat version 18, with a significance level of p < 0.05, using Fisher’s Least Significant Difference.
Results
From visual inspection, in comparison to untreated oat husks, extruded oat husks, were reduced in particle size and had increased surface area. They produced significantly greater yields for total biogas (p < 0.001), methane (p < 0.001), and carbon dioxide (p = 0.025) compared to the untreated oat husks (Table 3). The extruded oat husks produced 29% more total biogas, 27% more methane, and 43% more carbon dioxide, compared to untreated oat husks.

TABLE 3. The effect of the BioExtruder pre-treatment technology on the mean total biogas, methane and carbon dioxide yields (ml/gVS fed) from oat husks, minus the blank assay biogas yields.
The cumulative methane yield (ml/gVS fed) increased steadily from the beginning of the experiment, with a greater methane yield produced from the extruded oat husks treatment compared to the untreated oat husks (Figure 2). Between days 34–46, biogas pressure measurements recorded a gradual reduction in daily biogas volume and this determined the experimental endpoint. The cellulose control demonstrated >80% methane yield compared to the theoretical cellulose methane yield of 415 ml/gVS fed (Filer et al., 2019), demonstrating that the sewage sludge was biologically active.
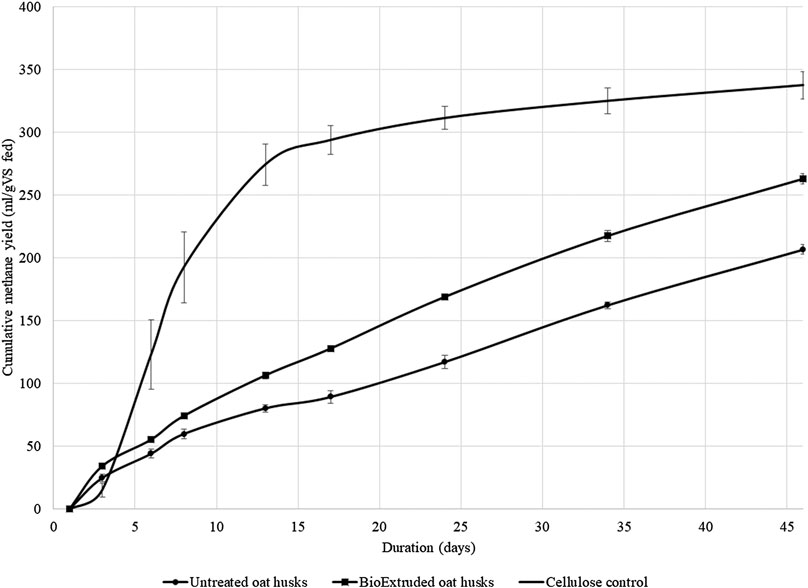
FIGURE 2. The mean cumulative methane yield from oat husks (untreated and pre-treated via the BioExtruder) and cellulose controls. Digestate blank assay methane yields were subtracted from both treatment yields.
At the end of the experiment, the pH was not significantly different between the two oat husks treatments. However, at the end of the experiment, the pH of both oat husk treatments (at 7.76) were reduced in comparison to the initial sewage sludge pH and blank assays pH (Table 4). Feedstock degradability was measured through TS and VS conversion to biogas. There was a significantly (p = 0.020) higher TS degradability from extruded oat husks (20.4%) compared to the untreated oat husks (15.5%) (Table 4). This equates to a 31.6% improvement in the TS degradation by processing oat husk using the BioExtruder. This greater TS degradation rate was reflected in the significantly higher biogas yields for the extruded treatment. Although VS degradability was also higher for the extruded oat husk treatment, there was no significant effect on VS degradability. This was due to both treatments having a greater variation in VS degradation rates between replicates (Table 4).

TABLE 4. The effect of the BioExtruder pre-treatment technology on the mean pH, total solids (TS) and volatile solid (VS) degradation rates (%) (VS is expressed as a proportion of TS content) at the end of the biochemical methane potential assays.
Discussion
To date, limited research has been undertaken on the AD of oat husks and the suitability of oat straw for AD (Kusch et al., 2011). The BioExtruder was effective at altering the physical structure of the oat husks, reducing the particle size and increasing the surface area. As the oat husks were soaked in water prior to extrusion, the processed oat husks retained some of this additional water post extrusion. This was demonstrated by the lower TS content of the extruded oat husks, but similar VS content of both untreated and extruded oat husks (Table 1).
Extrusion of oat husks demonstrated significantly higher biogas yields and TS degradation rate compared to untreated oat husks. The significant improvement in TS degradability of extruded oat husks demonstrates the ability of the BioExtruder to fragment oat husks and to improve methanogenesis. The pH value for both treatments at the start and end of the experiment were suitable for methanogenesis. The rate of biogas production was faster at the start of the experiment for the extruded oat husks, but thereafter the rate of biogas production stabilized for both treatments throughout the remainder of the BMP experiment. The untreated oat husks in this research produced similar methane yields (207.5 ml/gVS fed) compared to those previously published; 202 ml CH4/gVS fed (Kusch et al., 2011). Kusch et al. (2011) demonstrated that the rate of methane production for the first 30 days of AD could be increased by grinding oat husks to <1 mm. This produced an increased methane yield of 242 ml/gVS fed, over a 49 days experimental period (Kusch et al., 2011). This value, 242 ml/gVS fed, was less than the methane yield obtained from BioExtruded oat husks of 264 ml/gVS fed, over a similar same time period.
Previous research has stated that some pre-treatment technologies are energy intensive (Maurya et al., 2015) and insufficient methane yields are obtained from the pre-treatment feedstocks. Therefore, the overall processes have not been energetically favorable (Abraham et al., 2020). Rika BioFuels anticipate the BioExtruder to have an energy demand of 50–60 kW/h per ton of wheat straw fresh weight processed (Rika BioFuels, Personal Communication). There is limited information published on the energy demand of other pre-treatment technologies (Schumacher et al., 2014). However, Schumacher et al. (2014) estimated energy usage to vary from 0.4 to 16.7 kWh/kgTS for agitator bead mills and approximately 2 kWh/kgTS of feedstock processed through thermal and high-pressure pre-treatments (Schumacher et al., 2014). In comparison to the technologies investigated in the Schumacher et al. (2014) study, it would suggest that the BioExtruder was energy efficient compared to other pre-treatment technologies. However further research is required to quantify this.
The experimental period of the BMP reflected the hydraulic retention time commercial anaerobic digesters are operated at. The cellulose control had peaked by the end day 34 of the experiment, with little additional biogas produced. If the BMP experiment was continued for longer, the untreated oat husks may have produced further methane, although at a very slow rate. The aim of this experiment was to replicate commercial practice within the experimental design. Reducing the time taken to digest recalcitrant feedstocks, like oat husks, is an advantage to AD plant operators because the biogas yield could be increased per m3 of digester tank. Furthermore, the increased methane yield of extruded recalcitrant feedstocks could substitute the use of other more expensive feedstocks. Additionally, new AD plants that used extruded feedstocks could reduce their digestion tank sizes while achieving the same biogas output (Dumas et al., 2015). In this context, the BioExtruder investigated in this research may be a commercially valuable tool to assist AD plant profitability and contribute to oat husk valorization and the circular economy. Further work is now required to investigate longer-term continuous digestion of extruded feedstocks. This will provide an assessment of longer-term digestion stability and methane yields. Additionally, an energy assessment of the BioExtruder process is needed to determine commercial viability.
Conclusions
This research has demonstrated that the 3 kW/h mini BioExtruder can process oat husks and alter their physical composition to improve anaerobic digestibility. The extruded oat husks BMP demonstrated increased methane yields compared to oat husks without pre-treatment. This technology could enable a greater use of oat husks in AD, improving the circular economy and helping the United Kingdom to meet the 2030 Climate and Energy Policy Framework targets.
Data Availability Statement
The raw data supporting the conclusions of this article will be made available by the authors, without undue reservation.
Author Contributions
MK contributed to the conceptualization, methodology development, data curation and analyzes, and drafting the manuscript. MM contributed to the methodology development, resource allocation, data curation, and reviewing the manuscript. HHA led the funding acquisition and contributed to project management and reviewing the manuscript. JF contributed to funding acquisition and reviewing the manuscript. MT contributed to the conceptualization, funding acquisition, and reviewing the manuscript.
Funding
Funding was kindly provided by Rika BioFuels Limited for the oat husks BMP assays and the Department of Transport through the Future Fuels for Flight and Freight Competition (F4C), grant number F4C1-004 for background understanding.
Conflict of Interest
Authors HHA and JF were employed by the company Rika BioFuels Limited.
The remaining authors declare that the research was conducted in the absence of any commercial or financial relationships that could be construed as a potential conflict of interest.
Acknowledgments
Thanks to Gregory Krupnikovs and Giles Houston from Rika BioFuels Limited for their help with operating the BioExtruder and their involvement with the continuous digestion experiment.
References
Abraham, A., Mathew, A. K., Park, H., Choi, O., Sindhu, R., Parameswaran, B., et al. (2020). Pretreatment strategies for enhanced biogas production from lignocellulosic biomass. Bioresour. Technol. 301, 122725. doi:10.1016/j.biortech.2019.122725
Angelidaki, I., Alves, M., Bolzonella, D., Borzacconi, L., Campos, J. L., Guwy, A. J., et al. (2009). Defining the biomethane potential (BMP) of solid organic wastes and energy crops: a proposed protocol for batch assays. Water Sci. Technol. 59 (5), 927–934. doi:10.2166/wst.2009.040
APHA (1989). Standard methods for the examination of water and wastewater. 17th Edn. Washington DC: American Public Health Association.
Barbieri, V., Lassinantti Gualtieri, M., and Siligardi, C. (2020). Wheat husk: a renewable resource for bio-based building materials. Construct. Build. Mater. 251, 118909. doi:10.1016/j.conbuildmat.2020.118909
Bedoić, R., Ćosić, B., and Duić, N. (2019). Technical potential and geographic distribution of agricultural residues, co-products and by-products in the European Union. Sci. Total Environ. 686, 568–579. doi:10.1016/j.scitotenv.2019.05.219
Bentsen, N. S., Felby, C., and Thorsen, B. J. (2014). Agricultural residue production and potentials for energy and materials services. Prog. Energy Combust. 40, 59–73. doi:10.1016/j.pecs.2013.09.003
Bledzki, A. K., Mamun, A. A., and Volk, J. (2010). Physical, chemical and surface properties of wheat husk, rye husk and soft wood and their polypropylene composites. Compos. Appl. Sci. Manuf. 41, 480–488. doi:10.1016/j.compositesa.2009.12.004
Decker, E. A., Rose, D. J., and Stewart, D. (2014). Processing of oats and the impact of processing operations on nutrition and health benefits. Br. J. Nutr. 112, S58–S64. doi:10.1017/S000711451400227X
Dumas, C., Silva Ghizzi Damasceno, G., Barakat, A., Carrère, H., Steyer, J.-P., and Rouau, X. (2015). Effects of grinding processes on anaerobic digestion of wheat straw. Ind. Crop. Prod. 74, 450–456. doi:10.1016/j.indcrop.2015.03.043
Elbersen, B., Startisky, I., Hengeveld, G., Schelhass, M. J., and Han, N. (2012). Atlas of EU biomass potentials. Available at: https://ec.europa.eu/energy/intelligent/projects/sites/iee-projects/files/projects/documents/biomass_futures_atlas_of_technical_and_economic_biomass_potential_en.pdf (Accessed August 10, 2020).
European Commission (2014). A policy framework for climate and energy in the period from 2020 to 2030 (COM(2014) 15 final). Communication from the commission to the European parliament, the council, the European economic and social committee and the committee of the regions. https://eur-lex.europa.eu/legal-content/EN/TXT/?uri=COM:2014:15:FIN.
Ferreira, L. C., Nilsen, P. J., Fdz-Polanco, F., and Pérez-Elvira, S. I. (2014). Biomethane potential of wheat straw: influence of particle size, water impregnation and thermal hydrolysis. Chem. Eng. J. 242, 254–259. doi:10.1016/j.cej.2013.08.041
Filer, J., Ding, H. H., and Chang, S. (2019). Biochemical methane potential (BMP) assay method for anaerobic digestion research. Water 11 (5), 921. doi:10.3390/w11050921
Fischer, G., Prieler, S., van Velthuizen, H., Lensink, S. M., and de Wit, M. (2010). Biofuel production potentials in Europe: sustainable use of cultivated land and pastures. Part I: land productivity potentials. Biomass Bioenergy 34, 159–172. doi:10.1016/j.biombioe.2009.07.008
Gallegos, D., Wedwitschka, H., Moeller, L., Zehnsdorf, A., and Stinner, W. (2017). Effect of particle size reduction and ensiling fermentation on biogas formation and silage quality of wheat straw. Bioresour. Technol. 245, 216–224. doi:10.1016/j.biortech.2017.08.137
Gomez-Tovar, F., Celis, L. B., Razo-Flores, E., and Alatriste-Mondragón, F. (2012). Chemical and enzymatic sequential pretreatment of oat straw for methane production Francisco. Bioresour. Technol. 116, 372–378. doi:10.1016/j.biortech.2012.03.109
Kusch, S., Schumacher, B., Oechsner, H., and Schäfer, W. (2011). Methane yield of oat husks. Biomass Bioenerg. 35, 2627–2633. doi:10.1016/j.biombioe.2011.02.044
Lehmann, T., and Eberhard, F. (2012). Straw instead of field crops. Landtechnik 67 (5), 358–360. doi:10.15150/lt.2012.57910.1628/002268812803506162
Liu, T., Zhou, X., Li, Z., Wang, X., and Sun, J. (2019). Effects of liquid digestate pretreatment on biogas production for anaerobic digestion of wheat straw. Bioresour. Technol. 280, 345–351. doi:10.1016/j.biortech.2019.01.147
Marques, G., Rencoret, J., Gutiérrez, A., and del Río, J. C. (2020). Lipophilic compounds from maize fiber and rice husk residues – An abundant and inexpensive source of valuable phytochemicals. Ind. Crop. Prod. 146, 112203. doi:10.1016/j.indcrop.2020.112203
Maurya, D. P., Singla, A., and Negi, S. (2015). An overview of key pretreatment processes for biological conversion of lignocellulosic biomass to bioethanol. 3 Biotech 5, 597–609. doi:10.1007/s13205-015-0279-4
Peng, X., Nges, I. A., and Liu, J. (2016). Improving methane production from wheat straw by digestate liquor recirculation in continuous stirred tank processes. Renew. Energy 85, 12–18. doi:10.1016/j.renene.2015.06.023
Rika BioFuels (2017). BioExtruder. Available at: https://www.bioextruder.co.uk/ (Accessed June 17, 2020).Google Scholar
Scarlat, N., Fahl, F., Lugato, E., Monforti-Ferrario, F., and Dallemand, J. F. (2019). Integrated and spatially explicit assessment of sustainable crop residues potential in Europe. Biomass Bioenerg. 122, 257–269. doi:10.1016/j.biombioe.2019.01.021
Scarlat, N., Martinov, M., and Dallemand, J.-F. (2010). Assessment of the availability of agricultural crop residues in the European Union: potential and limitations for bioenergy use. J. Waste Manag. 30, 1889–1897. doi:10.1016/j.wasman.2010.04.016
Schumacher, B., Wedwitschka, H., Hofmann, J., Denysenko, V., Lorenz, H., and Liebetrau, J. (2014). Disintegration in the biogas sector – Technologies and effects. Bioresour. Technol. 168, 2–6. doi:10.1016/j.biortech.2014.02.027
Theodorou, M. K., Williams, B. A., Dhanoa, M. S., McAllan, A. B., and France, J. (1994). A simple gas production method using a pressure transducer to determine the fermentation kinetics of ruminant feeds. Anim. Feed Sci. Technol. 48, 185–197. doi:10.1016/0377-8401(94)90171-6
Keywords: anaerobic digestion, extrusion, bioextruder, pre-treatment, oat husks, circular economy, valorization, biochemical methane potential
Citation: Kirby ME, Mirza MW, Hoskyns-Abrahall H, Fenwick J and Theodorou MK (2020) Increasing the Methane Potential of Oat Husks Using a Novel Extrusion Pre-Treatment Technology Prior to Anaerobic Digestion. Front. Energy Res. 8:579034. doi: 10.3389/fenrg.2020.579034
Received: 01 July 2020; Accepted: 01 September 2020;
Published: 05 October 2020.
Edited by:
David M. Wall, University College Cork, IrelandReviewed by:
Ao Xia, Chongqing University, ChinaBernadette McCabe, University of Southern Queensland, Australia
Copyright © 2020 Kirby, Mirza, Hoskyns-Abrahall, Fenwick and Theodorou. This is an open-access article distributed under the terms of the Creative Commons Attribution License (CC BY). The use, distribution or reproduction in other forums is permitted, provided the original author(s) and the copyright owner(s) are credited and that the original publication in this journal is cited, in accordance with accepted academic practice. No use, distribution or reproduction is permitted which does not comply with these terms.
*Correspondence: Marie E. Kirby, bWtpcmJ5QGhhcnBlci1hZGFtcy5hYy51aw==