Corrigendum: Intact Forest in Selective Logging Landscapes in the Tropics
- 1Faculty of Engineering and IT, School of Information, Systems and Modelling, University of Technology, Sydney, NSW, Australia
- 2Department of Mechanical Engineering, Politeknik Negeri Medan, Medan, Indonesia
- 3Biofuel Engine Research Facility, Queensland University of Technology, Brisbane, QLD, Australia
- 4Department of Mechanical Engineering, COMSATS University Islamabad, Sahiwal, Pakistan
Biodiesel is one of the potential alternative energy sources that can be derived from renewable and low-grade origin through different processes. One of the processes is alcoholysis or transesterification in the presence of a suitable catalyst. The catalyst can be either homogeneous or heterogeneous. This article reviews various catalysts used for biodiesel production to date, presents the state of the art of types of catalysts, and compares their suitability and associated challenges in the transesterification process. Biodiesel production using homogeneous and heterogeneous catalysis has been studied extensively, and novel heterogeneous catalysts are being continuously investigated. Homogeneous catalysts are generally efficient in converting biodiesel with low free fatty acid (FFA) and water containing single-origin feedstock. Heterogeneous catalysts, on the other hand, provide superior activity, range of selectivity, good FFA, and water adaptability. The quantity and strengths of active acid or basic sites control these properties. Some of the heterogeneous catalysts such as zirconia and zeolite-based catalysts can be used as both basic and acidic catalyst by suitable alteration. Heterogeneous catalysts from waste and biocatalysts play an essential role in attaining a sustainable alternative to traditional homogeneous catalysts for biodiesel production. Recently, high catalytic efficiency at mild operating conditions has drawn attention to nanocatalysts. This review evaluates state of the art and perspectives for catalytic biodiesel production and assesses the critical operational variables that influence biodiesel production along with the technological solutions for sustainable implementation of the process.
Introduction
The exigency of energy, limited reserve, the rapidly rising price of petroleum oil, and the deleterious effect of greenhouse gases have dictated to steer our attention toward alternative sources of energy. The quest for eco-friendly technology is driving the research initiatives to find potential energy sources that are renewable, biodegradable non-toxic, and mostly carbon neutral (Arbab et al., 2015). Historically, fossil fuels have played a vital role in global energy demand (Jayed et al., 2009). The diesel engine, named after its inventor Rudolf Diesel, was patented in 1892 and catered this energy demand substantially ever since. Being the powerhouse of heavy-duty and commercial transport vehicles has been the most important use of diesel engines, and the importance is increasing consistently. The diesel engine is the most efficient type of internal combustion engine, offering excellent fuel economy and low carbon dioxide (CO2) emission (Fattah et al., 2018). While diesel engines are arguably superior to any other power-producing device for the transportation sector in terms of efficiency, torque, and overall drivability, they suffer from inferior performance in terms of emissions (Silitonga et al., 2013a).
Biodiesel is a renewable energy source that can replace fossil-based diesel and can reduce the drawbacks of diesel emission (Abedin et al., 2014). Diesel is obtained by fractional distillation from crude petroleum oil that typically contains a mixture of pure hydrocarbon molecules (no oxygen molecule) that range in size from 8 to 21 carbon atoms. Biodiesel, on the other hand, consists of long-chain hydrocarbons with an ester functional group (–COOR). Thus, it is defined as mono-alkyl esters of long-chain fatty acids derived from various feedstocks, namely, plant oils, animal fats, or other lipids, also known as triacylglycerides (TAGs), or more simply, triglycerides (Hoekman and Robbins, 2012). Biodiesel is produced using the transesterification or alcoholysis process, which is usually facilitated by acids, bases, enzymes, and other type and form of catalysts (Ong et al., 2014). The catalysts can be either in a homogeneous or in a heterogeneous phase as of the reactants. If the catalyst remains in the same phase (usually liquid) to the reactants during alcoholysis, then that is the homogeneous catalyst. If the catalyst is in a different phase (usually non-liquid) to the reactants, then that is the heterogeneous catalyst (Ruhul et al., 2015). The appropriate catalyst selection depends on several factors, namely, the amount of free fatty acids (FFAs) in the oil, the water content, etc.
Homogeneous catalysts are generally efficient in converting biodiesel with low FFA and water containing single-origin feedstock (Silitonga et al., 2013a). Oils with higher FFA content lead to the formation of soap, consequently affecting the activity of the catalyst (Fattah et al., 2014a). Besides, the catalyst is partially miscible in biodiesel and miscible in glycerol, which results in problems of product separation from the reactant mixture (Tan et al., 2019). Heterogeneous catalysts, on the other hand, provides high activity, selectivity, and water adaptability due to the presence of a large number of active acid or basic sites. Various reviews have been published before on the topic of catalysts, especially on heterogeneous catalysts. Table 1 presented below summarizes some of the critical review articles in the last decade, along with their brief introductions. The novelty aspect of this article is to review the works of many researchers on the development of various homogeneous and heterogeneous catalysts used for biodiesel production to date. This article presents different types of catalysts and compares their suitability and associated challenges in the transesterification process with an emphasis on the catalytic activity, selectivity, catalyst loading, and reusability.
Biodiesel Production Using Alcoholysis
The conventional process for biodiesel production is transesterification or alcoholysis (typically methanolysis), by which the triglycerides are reacted with alcohols (typically methanol), in the presence of a catalyst, either homogeneous or heterogeneous, as a reaction promoter, to produce fatty acid alkyl esters [typically fatty acid methyl esters (FAME)] (Mahlia et al., 2020). Transesterification consists of several consecutive, reversible, and catalyzed reactions where the triglycerides are converted to diglycerides, monoglyceride, and finally glycerin (also known as glycerol) stepwise (Ong et al., 2019). Generally, biodiesel is produced using a single-step transesterification reaction catalyzed by alkali catalysts. However, depending on FFA and water content, a two-step reaction might be required where acid catalyzed alcoholysis, also known as esterification, precedes the transesterification process (Ashraful et al., 2014). The schematic diagram for one- and two-step biodiesel production is shown in Figure 1. The property standard of petroleum diesel and biodiesel, according to the American Society for Testing and Materials (ASTM) and European Standard (EN) are shown in Table 2.
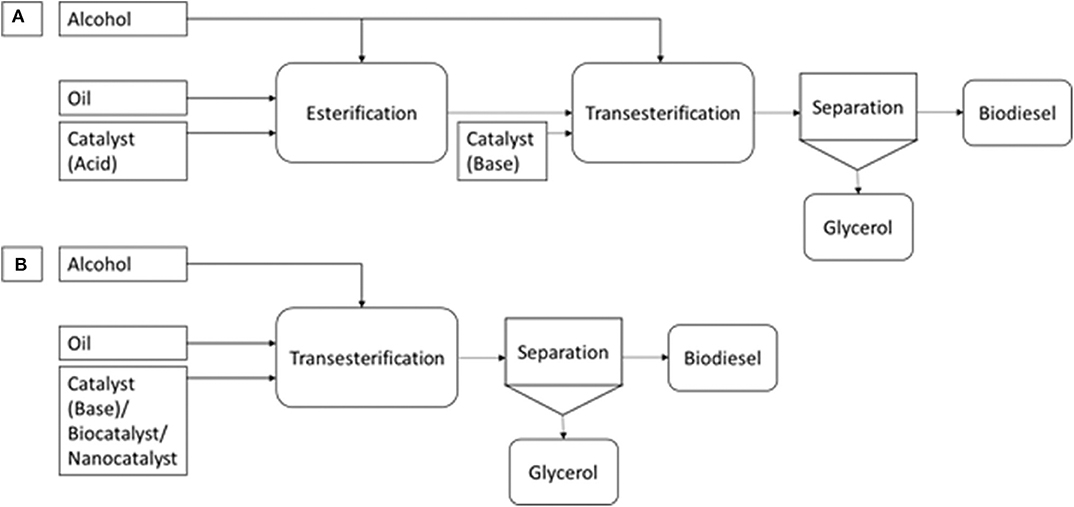
Figure 1. Biodiesel production from feedstock: (A) two-step process; (B) one-step process (Fattah et al., 2014d; Mofijur et al., 2014).
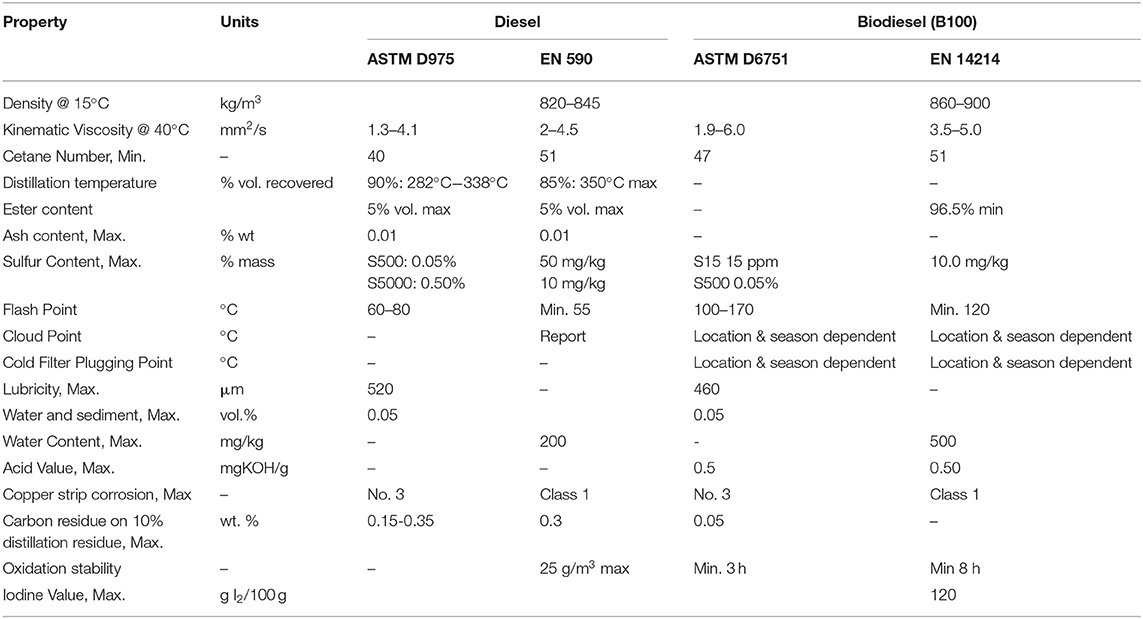
Table 2. Comparison of standards for diesel and biodiesel ASTM and EN standards (Fattah et al., 2013; Knothe and Razon, 2017).
Different Catalysts for Biodiesel Production
The presence of catalyst increases the rate of the reaction, thereby increasing the yield of the product. Various catalysts are used in the transesterification process for biodiesel production. As discussed previously, the catalysts used for the transesterification reaction are intricate to the group. However, based on previous review articles, these can be divided into four major categories, namely, homogeneous catalysts, heterogeneous catalysts, biocatalysts, and nanocatalysts (Shan et al., 2018; Akubude et al., 2019), which can be further classified into different subgroups. The classification is shown in Figure 2.
Transesterification or alcoholysis can be catalyzed both homogeneously and heterogeneously. When catalyzed homogeneously, the reactions are faster typically and require lower loading than that of heterogeneously catalyzed ones. One major drawback of homogeneous catalysts is that the separation of these catalysts from the medium is intricate and often non-economical; as such, reuse of these is often impossible. Apart from that, several washing steps associated with the catalyst removal from the product results in the consumption of water, often deionized, and significant generation of wastewater (De Lima et al., 2016). On the other hand, heterogeneous catalysts are in a different phase than the reaction system, which allows for the removal of catalyst at various stages. These can be reused subsequently without intensive washing steps. Besides, high-purity glycerine can be obtained compared to that of homogeneous catalysis due to considerably fewer dissolved ions, allowing for further use in industrial processes. For the advantages mentioned above, transesterification using heterogeneous catalysts has received increased attention over the past decade (Lam et al., 2010; Ling et al., 2019). However, partial leaching of the active sites, destruction of the catalyst microstructure, and organic deposition from the reaction mixture pose a problem for the applicability of these catalysts (Zhang et al., 2020). Therefore, the synthesis of active reusable heterogeneous catalysts is a great challenge in biodiesel production.
Homogeneous Catalysts
Homogeneous catalysis involves a sequence of reactions that is catalyzed by a chemical that is in the same phase as the reaction system. The most preferred catalyst used for the production of biodiesel is the homogeneous catalyst, as they are simple to use and require less time to achieve a complete reaction. Both acidic and basic catalysts come under this category. Homogeneous catalysts are usually dissolved in a solvent that is in the same phase with all reactants.
Base Catalyst
Homogeneous base catalysts are an alkaline liquid such as alkali metal-based hydroxides, namely, sodium or potassium hydroxide; alkali metal-based oxides such as sodium and potassium methoxides; and carbonates. Base catalysts have high activity in transesterification (Endalew et al., 2011). Metallic hydroxides are frequently used as catalysts due to lower prices but generally possess lower activity than alkoxides. It was reported that the rate of base catalyzed reaction is 4,000 times faster than that of the acid-catalyzed one (De Lima et al., 2016). A known drawback is that the oil containing significant amounts of FFA cannot be converted into biodiesels completely but remains as soap in vast quantities (Helwani et al., 2009). Up to ~5% FFAs, the reaction can still be catalyzed with an alkali catalyst, but an additional amount of catalyst is required to compensate for the catalyst lost to soap (Gerpen, 2005). Most studies recommend that the FFA content should be <2 wt.% for biodiesel production using the homogeneous catalyst.
Acid Catalyst
The esterification process is catalyzed by Brønsted acids, preferably by sulfonic and sulfuric acids as well as hydrochloric acid (Schuchardt et al., 1998). These catalysts produce very high yields in alkyl esters. However, the reactions are slower compared to alkali catalyzed reactions, making the process economically confronting due to the increased energy requirements (Silitonga et al., 2020). Homogeneous acid catalysis is insensitive to FFA content and can catalyze both esterification and transesterification reactions. Despite these added advantages, homogeneous acid catalysis presents the same separation issues as homogeneous base catalysis. Some investigations using homogeneous catalysts to produce biodiesel from different biodiesel feedstocks are shown in Table 3.
Heterogeneous Catalysts
Heterogeneous catalysts are in a phase or state different from those of the reactants. These are the type of catalysts that creates active sites with its reactants regularly during a reaction (Melero et al., 2009). Heterogeneously catalyzed methanolysis reaction is very complex because it occurs in a three-phase system consisting of a solid (heterogeneous catalyst) and two immiscible liquid phases (oil and methanol). Some side reactions such as saponification of glycerides and methyl esters and neutralization of FFAs by catalyst also occur concurrently with methanolysis. The main disadvantages of this catalysis include elevated temperatures and higher oil/alcohol ratios than that of the homogeneous catalysis. Other advantages include ease in separation and purification, as well as superior reusability of the catalyst, etc. Heterogeneous catalysts can be divided into acid and base catalysts. These catalysts can be classified as Brønsted or Lewis catalysts (Di Serio et al., 2008). However, in many cases, both types of sites could be present; as such, some of the catalysts can be used as a catalyst to both kind of reactions.
Base Catalyst
A heterogeneous base catalyst aims to overcome constraints such as saponification that hinders the separation of glycerol from the methyl ester layer, associated with the usage of a homogeneous base catalyst. These catalysts also show superior catalytic activity under mild conditions (Calero et al., 2014). These catalysts have many other advantages, namely, non-corrosiveness, environmental friendliness, and less problems in disposal. In addition, they are easily separated from the reaction environment and can be designed to give higher activity, selectivity and longer catalyst lifetime (Liu et al., 2008b). Many metal-based oxides, including alkali metal, alkaline earth metal, and transition metal oxides have been used as catalyst for the transesterification process of oils. The structure of metal oxides consists of positive metal ions (cations) that possess Lewis acid characteristics and negative oxygen ions (anions) that possess Brønsted base characteristics (Di Serio et al., 2008; Zabeti et al., 2009). Sometimes, two or more types of metal oxides are combined to be used as catalysts. Other types of catalysts that predominantly work as base heterogeneous catalysts are boron group-based and waste-based catalysts (Semwal et al., 2011).
Alkaline earth and alkali metal-based catalyst
Metal-based oxides are the most commonly exploited as a heterogeneous catalyst for transesterification. The surface structure of a metal oxide is presented in Figure 3. Zhang et al. (1988) demonstrated the basic properties of alkaline earth metal oxides using temperature-programmed desorption (TPD) of adsorbed carbon dioxide analysis. They showed that the amount of basic sites per unit weight approximately corresponds to that of the surface area and follows the sequence: CaO > MgO > SrO > BaO. The oxides of stronger basic sites promote the reaction more effectively.
Among the metal-based catalysts, CaO has been the most studied catalyst material for biodiesel production, as it presents many advantages, namely, long catalyst life, relatively high basic strength, high activity, and low solubility in methanol and requires only moderate reaction conditions (Roschat et al., 2016; Latchubugata et al., 2018). Arun et al. (2017) studied the biodiesel production from Terminalia belerica and Garcinia gummi-gutta using calcinated CaO as a heterogeneous catalyst. The calcination process was done in a muffle furnace at 605°C for 5 h, which was preceded by drying in an oven at 125°C for 12 h. The transesterification was carried out at 60°C, 9:1 M methanol/oil ratio, and 2% w/v catalyst for 3 h. Properties of biodiesel produced from these feedstocks were within the range suggested by the American Society for Testing and Materials (ASTM) standard establishing a successful production. Roschat et al. (2016) carried out the transesterification of palm olein oil (FFAs of 0.29 mg KOH/g) using hydrated lime-derived calcium oxide (CaO) as a catalyst. The catalyst was prepared by drying overnight in an oven at 100°C, crushed and sieved, and then calcined in a furnace at different temperatures (700, 800, and 900°C) in the air for 3 h. A biodiesel yield of 97.20% was achieved for 800°C calcined sample. This catalyst could be reused for at least five times without significant yield deterioration (more than 90%).
Du et al. (2019) synthesized three novel carbon-based MgO solid bases using sol–gel and calcination method for biodiesel production from castor oil. These catalysts were identified as MgO-PVA-800, MgO-UREA-800, and MgO-GLY-800, where 800 indicates the calcination temperature, and PVA, UREA, and GLY stands for polyvinyl acetate, urea, and ethylene glycol, respectively. The transesterification was carried out at 12:1 M ethanol/oil ratio, 6 wt.% catalyst concentration at 75°C. The highest conversion rate of 96.5% was obtained with MgO-UREA-800 after 50 min under these conditions. The biodiesel yield for MgO-PVA-800 and MgO-GLY-800 was 93.6 and 91.8%, respectively, for the same reaction time. Manríquez-Ramírez et al. (2013) studied the catalyst activity of impregnated MgO with KOH, NaOH, and cerium nitrate. The basic strength of the samples followed the order: MgO–KOH > MgO–NaOH > MgO–CeO2 as determined by Fourier-transform infrared spectroscopy (FTIR). The reaction was carried out at 60°C with oil/methanol ratio of 4:1, and the biodiesel yields after 1 h of reaction were 44, 56, 78, and <99% for MgO and MgO–CeO2, MgO–NaOH, and MgO–KOH, respectively. It has been reported that MgO has low activity in transesterification of vegetable oils to biodiesel (Liu et al., 2007).
Roschat et al. (2018) studied the biodiesel production process of palm oil using strontium oxide (SrO) via the ethanolysis reaction. The optimum reaction condition for this work was determined experimentally as 80°C reaction temperature, ethanol/oil ratio of 12:1 M, catalyst loading amount of 5 wt.%, and reaction time of 3 h. This resulted in a yield of 98.2% fatty acid ethyl ester. The catalyst can be reused for five times with more than 90% yield. Liu et al. (2007) studied the transesterification of soybean oil to biodiesel using SrO as a solid base catalyst. They proposed the mechanism of the catalytic reaction of SrO, which creates basic intermediate compounds, thereby making it a solid base catalyzed reaction. The results showed that biodiesel yield was <95% at temperatures below 70°C within 30 min. The reusability of SrO was high and could maintain sustained activity even after 10 cycles.
Mootabadi et al. (2010) carried out ultrasonic-assisted transesterification of palm oil using different alkaline earth metal oxides such as CaO, SrO, BaO, etc. as catalysts. They varied the reaction parameters, namely, reaction time of 10–60 min, alcohol/oil ratio of 3:1–15:1 M, catalyst loading of 0.5–3%, and ultrasonic amplitudes of 25–100%. Their results opposed the previously reported activity ranking of the catalysts as CaO < SrO < BaO. At optimum test conditions, 95% yield was reached in 1 h. When non-optimized conditions were used, the yield of 77.3, 95.2, and 95.2% was reported for CaO, SrO, and BaO catalysts, respectively, for 1 h reaction time. Dai et al. (2018) prepared lithium-based catalyst, lithium–iron oxide (LiFe5O8-LiFeO2) by homogeneously mixing Fe2O3 in an aqueous solution of Li2CO3 and then heating this in a muffle furnace at different calcination temperatures and cooling to room temperature afterwards. The catalysts were then used to produce biodiesel from soybean oil where reactions were carried out at 65°C for 2 h utilizing methanol/oil ratio 36:1 M and 8 wt.% catalyst loading. The maximum conversion of 96.5% was achieved when the catalyst calcination temperature of 800°C was used. Moreover, the catalyst can be reused for at least five runs, maintaining the biodiesel yields close to 94%.
Mixed metal-based catalyst
Mixed metal-based oxides are predominantly used as base catalyst depending on the catalyst mixture. Their basicity can be tuned by altering their chemical composition and synthesis procedure (Teo et al., 2017). The type of synthesis method, activation temperature, and structure have a strong influence in the final basicity of the mixed oxides (Mckenzie et al., 1992; Tichit et al., 1995). They were sometimes used as a mixed acid–base catalyst by some researchers. Salinas et al. (2018) studied the catalytic activity of 1–5 wt.% La2O3 in ZrO2 mixed metal oxides produced using sol–gel method and calcined at 600°C in biodiesel production from canola oil through methanolysis reaction. The sol–gel method allows for improving catalytic activity by modifying acid–base properties. It was found that La2O3 doped in ZrO2 forms a monoclinic-ZrO2 structure and enhances its basicity, which plays a key role in catalysis, and 3 wt.% La2O3 was the optimum percentage for the transesterification of canola oil. Limmanee et al. (2013) studied the catalytic activity of nanocrystallite CaMgZn mixed oxides as heterogeneous catalyst prepared using coprecipitation method using Na2CO3 as a precipitant in the synthesis of palm kernel oil biodiesel via methanolysis. They reported a maximum yield of 97.5 wt.% over the CaMgZn mixed oxide, prepared with the Ca/Mg/Zn ratio of 3:1:1 under the Na2CO3 concentration of 0.75 mol/L and the CaMg(CO3)2 and CaZn(CO3)2 metal ions ratio of 1.0 when the reaction conditions were methanol/oil ratio of 20:1 M, 6 wt.% catalyst and 60°C. Santiago-Torres et al. (2014) studied Na2ZrO3 as a basic catalyst for the transesterification of soybean oil. They reported a maximum biodiesel conversion efficiency of 98.3% at 3% of catalyst in 3 h of reaction time at 65°C reaction temperature. Lee et al. (2015) synthesized bifunctional acid–base catalyst comprising of mixed metal oxides of Ca and La (CaO–La2O3) at various Ca/La atomic ratios via coprecipitation. This integration enhanced the catalytic activity due to the dispersion of CaO on the composite surface, thereby increasing the surface acidic and basic sites compared to individual oxides. They reported the highest biodiesel yield of 98.76% under conditions of 160°C, 3 h, 25:1 M methanol/oil ratio, and 3 wt.% catalyst.
Transition metal-based catalyst
Titanium oxide (TiO2) and zinc oxide (ZnO) are among the transition metal oxides that are used as a heterogeneous base catalyst for biodiesel production (Yoo et al., 2010). Kaur et al. (2018) synthesized tungsten (W) supported TiO2/SiO2 catalyst using the sol–gel method to study transesterification of waste cottonseed oil to produce biodiesel. The complete transesterification reaction was achieved in 4 h with 1:30 M oil/methanol ratio, 5 wt.% catalyst at 65°C. They also reported good reusability with the catalyst being active in four catalytic runs without significant reduction in activity. Madhuvilakku and Piraman (2013) studied the catalytic activity of mixed oxides of Ti and Zn (TiO2-ZnO) and ZnO by employing these in palm oil transesterification process. Biodiesel yield of 92% was attained with 200 mg catalyst loading of TiO2-ZnO, 6:1 M methanol/oil ratio, and 60°C reaction temperature in 5 h. They also reported a better yield compared to ZnO catalyst (83%).
Boron group-based catalyst
Boron group-based compounds, especially alumina (Al2O3), are widely utilized for supporting different metal oxides, halides, nitrates, and alloys (Chouhan and Sarma, 2011). Kesserwan et al. (2020) reported on novel hybrid CaO/Al2O3 aerogel to catalyze the methanolysis of waste cooking oil. The catalyst was prepared by the rapid epoxide-initiated sol–gel process followed by calcination at 700°C under supercritical carbon dioxide conditions. They reported that, at optimized reaction condition of 11:1 M methanol/oil ratio, 1 wt.% of 3:1 CaO/Al2O3 calcined aerogel, 65°C reaction temperature, and 4 h reaction time results in a maximum biodiesel yield of 89.9%. Benjapornkulaphong et al. (2009) studied various Al2O3-supported alkali and alkali earth metal nitrates, namely, LiNO3/Al2O3, NaNO3/Al2O3, Ca(NO3)2/Al2O3, KNO3/Al2O3, and Mg(NO3)2/Al2O3 produced by impregnation method followed by calcination at 450–850°C. They employed these to catalyze the methanolysis of palm kernel oil and coconut oil. They found that calcination temperature affects the yield significantly with Ca(NO3)2/Al2O3 and LiNO3/Al2O3 yielding <90% biodiesel when calcined at 450°C, and higher calcination temperature dropped the biodiesel yield. They recommended a catalyst amount of 10 wt.% and 15–20 wt.% of Ca(NO3)2/Al2O3 catalyst for transesterification of palm kernel oil and coconut oil, respectively, when methanol/oil ratio of 65:1 M, temperature of 60°C, and reaction time of 3 h were used.
Hydrotalcite-based catalyst
“Hydrotalcites are a class of anionic and basic clays with a general formulas of Mx3+ (OH2)x+(Ax/n)n−. yH2O where M2+ and M3+ are divalent and trivalent metals, respectively, An−(, , Cl−, ) is an n-valent anion, and x usually has a value between 0.25 and 0.33” (Helwani et al., 2009; Endalew et al., 2011). Hydrotalcites are predominantly used as heterogeneous base catalyst. The advantages of these catalysts are their tunable high basic strength obtained by changing the ratio of Mg/Al and the inherent better catalyst morphology compared to alkaline earth metal oxides. However, nonhomogeneity and sensitiveness to FFA and water are the major disadvantages for this type of catalysts. Navajas et al. (2018) studied the catalytic activity of Mg–Al hydrotalcite with Mg/Al ratios of 1.5–5 M, synthesized by coprecipitation, in transesterification of sunflower oil. They reported an optimum conversion of 96% under methanol/oil ratio of 48:1 M, 1 atm, 60°C, 2 wt.% of the catalyst after 24 h. They attributed the activity of catalyst to the presence of Brønsted-type basic sites at the edges of the crystal. Nowicki et al. (2016) studied the catalytic performance of Zr-doped Mg–Al hydrotalcite with varying Zr/Mg molar ratios in transesterification of refined rapeseed oil. They found that tetravalent cation of Zr (Zr4+) was effective in significantly increasing the catalytic activity of the catalyst. They reported the optimum oil conversion of 99.9% for hydrotalcite with the ratio of Zr/Mg/Al = 0.45:2.55:1 M and the reaction condition of 373–393 K temperature, 4.8−5.0 atm pressure, and 6 h reaction time. Trakarnpruk and Porntangjitlikit (2008) studied the catalytic activity of K-loaded calcined Mg–Al hydrotalcite (Mg/AL = 4) in transesterification of palm oil with methanol. They found that the catalyst acts as a basic catalyst. They also reported an 86.6% methyl ester yield at reaction parameters of 30:1 M methanol/oil ratio, 100°C, and 7 wt.% catalyst for 6 h. Zeng et al. (2009) reported that immobilization of Saccharomyces cerevisiae lipase (a biocatalyst) on Mg–Al hydrotalcite (Mg/Al molar ratio, 4.0) by physical adsorption markedly improved performance of the enzyme. The transesterification of refined rape oil was carried out with immobilized lipase (1.5 wt.%) and under atmospheric pressure at 45°C with 96% ester conversion in 4.5 h. The biocatalyst maintained high activity with 81.3% ester conversion after 10 reuse cycles.
Waste-based catalyst
Waste derived from industrial processes and surrounding environment can aid in the development of a low-cost solid base catalyst. These catalysts can promote a sustainable and environment-friendly approach toward biodiesel production (Majhi and Ray, 2016; Pandit and Fulekar, 2017). Calcium-enriched waste products, namely, shells of mussel, egg, cockle, snail, and oyster; fish scales; animal bones; and ash derived from plant species, etc. are easily available at low cost (Marwaha et al., 2018). Calcium obtained from these waste materials could be converted to CaO, which is the most versatile heterogeneous base catalyst, as discussed previously. Yaşar (2019) studied the catalytic activity of waste-eggshell-based CaO and compared it to the pure CaO. Transesterification of rapeseed oil was carried out at 9:1 M methanol/oil ratio, 4 wt.% catalyst, and 60°C reaction temperature for 1 h. The maximum yield for these conditions was 96.81 and 95.12% for CaO and waste-eggshell-based CaO, respectively. Sirisomboonchai et al. (2015) studied the catalytic activity of calcined scallop shell in the transesterification of waste cooking oil using methanol. A yield of 86% was observed in the presence of small amount of water with 5 wt.% catalyst loading, 6:1 M methanol/oil ratio, and 65°C reaction temperature for 2 h. The same catalyst was used for four cycles with 20% reduction of FAME yield owing to the formation of Ca-glyceroxide on its surface. Hu et al. (2011) studied the catalyst derived from waste freshwater mussel shell in transesterification of Chinese tallow oil. The catalyst was synthesized using the calcination–impregnation–activation method. The mussel shell was first calcined at 900°C followed by impregnation in deionized water and activated by calcination at 600°C for 3 h. Over 90% yields was obtainable with 12:1 M methanol/oil ratio, 5 wt.% catalyst, and reaction temperature of 70°C in 1.5 h. The catalyst also exhibited excellent reusability with only 10–15% decrease in yield after 12 runs.
Acid Catalysts
Heterogeneous acid catalysts have a less corrosive and toxic effect and give rise to fewer environmental problems compared to homogeneous acid catalysts (Aransiola et al., 2014). These catalysts contain a variety of acidic sites with different strengths of Brønsted or Lewis acidity. While these catalysts provide encouraging results under moderate reaction conditions, they react very slowly compared to solid base catalysts. In addition, high catalyst loading, high temperature, and long reaction time are required to employ this type of catalysts (Mansir et al., 2017).
Cation-exchange resins
Many researchers have used cation-exchange resin for biodiesel production at laboratory scale. Cation exchange resins are macroporous and contain numerous acidic sites to catalyze FFAs to biodiesel through heterogeneous esterification reactions and prevent saponification. Fu et al. (2015) studied the catalyst activity of sulfonated polystyrene-divinyl benzene (ST-DVB-SO3H) macroporous resin in the esterification of high FFA (acid value, 64.9 mg KOH/g) oil. Maximum FFA conversion of 97.8% was achieved when the reaction was carried out under the following conditions: 10 wt.% catalyst loading, methanol/oil ratio of 15:1 M, and 100°C for 3 h. Feng et al. (2011) studied continuous esterification of waste fried oil with an acid value of 36.0 mg KOH/g in a fixed bed reactor using commercial cation-exchange resin (NKC-9). They reported a 98% conversion rate during 500 h run under 2.8:1 methanol/oleic acid mass ratio, 44.0 cm catalyst bed height, 0.62 ml/min feed flowrate, and 65°C reaction temperature.
Heteropoly acid derivatives
Heteropoly acids (HPAs) and their salts as solid (heterogeneous) acid catalysts are also used frequently for the production of biodiesel (Hanif et al., 2017; Alcañiz-Monge et al., 2018). HPAs having a Keggin structure are preferably used because they have high thermal stability and can be synthesized easily compared to other types of HPAs. However, Keggin-type HPA has a low specific surface area, which can be overcome using appropriate supportive material. HPAs supported on the carriers are used in biodiesel production because of their structural mobility and superacidity. Kurhade and Dalai (2018) studied 12-tungstophosphoric acid (TPA) (H3PW12O40.nH2O) impregnated on the γ-Al2O3 catalyst for the biodiesel production. An optimized conversion of 94.9 ± 2.3% can be achieved with 10 wt.% of the catalyst loading, 17.5:1 M methanol/oil ratio, 200°C, and 4 MPa in 10 h. A conversion of 90.3 ± 4.3% was achieved after the second run. Siddiquee et al. (2011) studied the catalytic activity of mesoporous ordered silica, SBA-15 impregnated with the HPA in the production of biodiesel from the lipid of wastewater sludge. The experiment was carried out in a microreactor setup under varying operating conditions. A biodiesel yield of 30.14 wt.% was obtained with 15% HPA at a temperature of 135°C and a pressure of 135 psi in 3 h reaction time.
Sulfonic acid-based catalysts
Sulfonic acid group catalysts are characterized by sulfonated cross-linked polystyrene and are generally less corrosive and environmentally benign (Mansir et al., 2017). These type of catalysts have enhanced activity due to the attraction of fatty acids and tails of alcohol by the polymer support. In addition, the strong sulfonic acid group attached to the polymer chains increases the acidity of the sites (Vaccari, 1999). The typical examples of these catalysts are nonporous Nafion resins and porous Amberlysts. Liu et al. (2008a) studied the synthesis of mesoporous solid acid catalysts based on sulfonic acid functionalized ordered mesoporous carbons (OMC-SO3H) to use as a heterogeneous acid catalyst for esterification of oleic acid with ethanol. The esterification was performed under an N2 atmosphere in a closed flask at 80°C. The results showed that this catalyst is highly efficient due to its high acid density and hydrophobic surface property. Andrijanto et al. (2012) studied sulfonic acid catalysts supported on hypercrosslinked polystyrene (D5082) for the esterification of oleic acid with methanol. The results showed that D5082 had high catalytic activity despite showing low concentrations of acid sites and acid site strengths, which they attributed to the high accessibility of acid sites throughout the catalyst particles.
Sulfated oxide-based catalyst
Sulfated metal oxides generally work as an acid heterogeneous catalyst in esterification reaction (Chen et al., 2007; Shi et al., 2016). Kaur and Ali (2015) studied the efficacy and reusability of Ce/ZrO2-TiO2/, a catalyst in the esterification of oleic acid with methanol or ethanol. The catalyst activity of this catalyst is a function of its Brønsted acidic sites, which depends on the cerium concentration. A 2 wt.% cerium in the catalyst, when calcined at 600°C, showed the highest catalytic activity. With 5 wt.% catalyst, 6:1 M methanol/oil ratio at 65°C and 1 h reaction time achieved <98% conversion for the esterification of vegetable oil. They also reported no significant loss in the catalyst activity until the fifth cycle. Ropero-Vega et al. (2010) studied the catalytic activity of titania sulfated with ammonium sulfate [TiO2/-(NH4)2SO4] as well as sulfuric acid (TiO2/-H2SO4). They found that sulfated samples showed strong acidity as determined using Hammet indicators. The FTIR analysis of TiO2/-(NH4)2SO4 and TiO2/-H2SO4 showed the presence of both Lewis and Brønsted acid sites and only Lewis-type sites, respectively. Further analysis showed very high activity for the esterification of fatty acids with ethanol in a mixture of oleic acid (79%). Up to 82.2% conversion of oleic acid was achieved after 3 h of reaction at 80°C.
Acid/Base Catalyst
As discussed previously, oil with high FFA content requires an acid catalyst to esterify the FFA content before transesterification can take place. For biodiesel production from these type of oils, a heterogeneous catalytic system with both acidic and basic sites is capable of esterification and transesterification with minimal soap formation is highly sought after (Semwal et al., 2011). This type of catalysts possesses “Lewis acid” sites, which take part in the esterification reaction of the carboxylic acid with methanol as well as conjugated basic sites that influence the transesterification of triglyceride with methanol. Depending on the type of reactant and reaction parameters, this type of catalysts can work as an acid catalyst, base catalyst, or bifunctional one.
Zirconia and its derivatives
Zirconium dioxide (ZrO2), also known as zirconia, is used as both acid and the base heterogeneous catalyst. The primary nature of this catalyst is acidic, as it has strong surface acidity (Lam et al., 2010). Other derivatives of zirconia include sulfated ZrO2 (Shi et al., 2016), metal oxides with ZrO2 (Guldhe et al., 2017), metal-supported zirconia (Wan Omar and Amin, 2011), zirconia supported metal oxides (Kim et al., 2012), etc. Ibrahim et al. (2019) studied the catalytic activity of ZrO2 loaded into different supports, namely, Al2O3, Fe2O3, TiO2, and SiO2 using hybrid sol–gel autocombustion method. Highest conversion of biodiesel of 48.6% was achieved using alcohol/acid ratio of 120:1 M, 0.1 mass% catalyst, and 120°C reaction temperature for 3 h when ZrO2/SiO2 was used. In addition, the catalyst could be reused five times without significant loss of catalytic activity. Guldhe et al. (2017) studied tungstated zirconia (WO3/ZrO2) as a heterogeneous acid catalyst for the synthesis of biodiesel for S. obliquus lipids. FTIR characterization of catalyst showed the presence of both Brønsted and Lewis acid sites. Optimized biodiesel conversion of 94.58% was achieved at 100°C temperature, 12:1 M methanol/oil ratio, and 15 wt.% of catalyst amount in 3 h. Sun et al. (2010) studied ZrO2 supported La2O3 as a catalyst for the transesterification reaction of sunflower oil with methanol to produce biodiesel. The catalyst was prepared by an incipient wetness impregnation method, followed by drying at 110°C overnight and calcination at 600°C for 4 h in air. They reported that biodiesel conversion was possible due to the basic nature of the catalyst, which was determined using TPD of adsorbed CO2 and 21 wt.% La2O3 loaded on ZrO2 showed the highest basicity. The optimized test conditions were 30:1 M methanol/oil ratio, 200°C reaction temperature, and 5 h of reaction time that resulted in 84.9% biodiesel yield for each case.
Zeolite-based catalyst
Zeolites occur naturally in the form of microporous crystalline aluminosilicates interlinked by oxygen atoms. The chemical composition, pore size structure, and ion exchange properties of zeolites are responsible for their versatile catalytic behavior (De Lima et al., 2016). Zeolite framework structure contains molecular pores and channels of equal sizes, which can absorb molecules that fit into these and exclude the larger ones. This property of zeolite helps to exchange ions that in turn produce negative ion within the structure of the catalyst, thereby emerging as a base catalyst (Hattori, 1995; Mansir et al., 2017). The base strength of the alkali ion-exchanged zeolite increases with increasing electropositivity of the exchange cation. Both synthetic and natural zeolites are being pursued as promising catalysts. Zeolites have unique properties as catalysts, namely, shape selectivity, ability to maintain electro-neutrality through cation–polar molecules reversible interactions, etc. The weak basic strength and small catalytic pore diameter are the main problems reported for basic zeolite catalysts (Endalew et al., 2011). These can also be used as an acid heterogeneous catalyst.
Li et al. (2019) studied alkaline Li/NaY zeolite catalysts with different molar ratios of Li2CO3 to NaY zeolite in the transesterification of castor oil with ethanol. The ideal catalyst was synthesized from fly ash using coprecipitation method with ratio of Li2CO3 to NaY zeolite of 1:1 M calcined at 750°C for 4 h. The fatty acid ethyl ester yield of 98.6% was obtained for the reaction conditions of ethanol/oil ratio of 18:1 M, 3 wt.% catalyst, and reaction temperature of 75°C for 2 h. Du et al. (2018) studied the catalytic activity of NaY zeolite-supported La2O3 catalysts in the production of castor oil biodiesel. They produced the optimized catalyst, S-La2O3/NaY-800, by physically mixing the zeolite NaY, sodium carboxymethyl cellulose (CMC), lanthanum oxide and kaolin (mass ratio of NaY/kaolin/La2O3/CMC = 70:20:10:2.5). A surfactant (4 wt.%) was added to this mixture and then calcined at 800°C. Under the optimized reaction conditions: ratio of ethanol to oil of 15:1 M, 10 wt.% catalyst, and reaction temperature of 70°C for 50 min; 84.6% fatty acid ethyl ester was obtained. Doyle et al. (2016) studied the acidic catalytic activity of zeolite Y with Si/Al ratio of 3.1, in the esterification of oleic acid. The optimum oleic acid conversion using the zeolite catalyst was 85% with 6:1 M ethanol/oleic acid ratio, 5 wt.% catalyst loading, and 70°C for 1 h reaction time.
Biocatalysts
The pathway of biodiesel production through chemical catalysis is energy consuming and produces undesired by-products, namely, soaps and polymeric pigments, which hinder the separation of product from glycerol and di- and monoacylglycerols (Gog et al., 2012). Using biocatalysts, these impediments can be eliminated. Biocatalysts, also known as enzymes, are acquired from living organisms that promote chemical reactions without affecting themselves chemically (Amini et al., 2017a,b). Two types of enzymatic biocatalyst are usually used in biodiesel production, namely, extracellular lipases and intracellular lipases. Extracellular lipases are the enzymes that have been recovered from the microorganism broth and then purified. Intracellular lipase, on the other hand, remains either in the cell-producing walls or inside the cell. The major producer microorganisms for extracellular lipases are Rhizopus oryzae, Mucor miehei, Candida antarctica, and Pseudomonas cepacia. The commercial immobilized lipases that has been studied thoroughly are Novozym 435, Lipozyme TL IM, Lipozyme RM IM, and Lipase PS-C (Gog et al., 2012). The drawback of using extracellular enzymes as catalyst is the complexity of separation and purification procedures and its associated cost. This can be lowered using microbial cells as whole-cell biocatalysts with acceptable biodiesel yield. Filamentous fungi have been discerned as whole-cell biocatalysts. However, lipase used in whole-cell form cannot be reused at the end of the reaction.
Unlike the chemical catalysts, biocatalysts apply to a wide range of triglyceride sources, with FFA ranging from 0.5 to 80% (Aransiola et al., 2014). Other advantages of enzymatic biodiesel production include easy product removal, moderate process temperature (35–45°C), zero by-product, and reusability of catalysts (Christopher et al., 2014; Mardhiah et al., 2017). The key parameters affecting the biodiesel yield for enzymatic synthesis are presented in Figure 4. As seen in the figure, various factors such as choice of lipase, choice of substrate, substrate concentration, pH of the microenvironment, temperature, spacing between the enzyme molecules and the substrate, etc. affect the biodiesel yield for enzyme-catalyzed transesterification. Some investigations using enzyme-catalyzed transesterification of different biodiesel feedstocks are presented in Table 4. As seen from the table, the efficiency of biocatalyzed transesterification process is dependent on enzyme source and operational conditions.
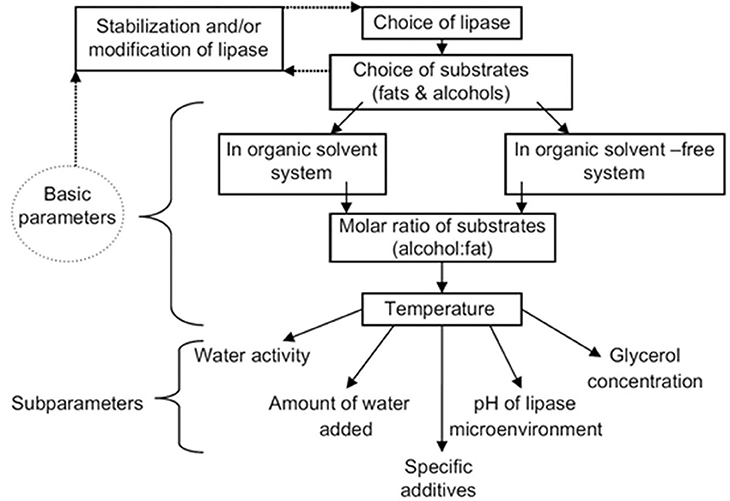
Figure 4. Crucial parameters affecting the biodiesel yield in enzymatic synthesis (Szczesna Antczak et al., 2009).
Nanocatalysts
Recently, nanocatalysts have gained significant attention for biodiesel production owing to their high catalytic efficiency (Qiu et al., 2011). These catalysts have a high surface area that results in increased activity compared to conventional catalysts. In addition, these catalysts possess high stability, superior resistance to saponification, efficient surface/volume ratio, and high reusability (Rahmani Vahid et al., 2017). Nanocatalysts can be synthesized using various methods. Some of the methods include self-propagating high-temperature synthesis, microwave combustion, conventional hydrothermal, microwave hydrothermal, microwave solvothermal, sol–gel technique, coprecipitation, impregnation, gas condensation, chemical vapor deposition, electrochemical deposition, vacuum deposition and evaporation, etc. (Quirino et al., 2016; Ambat et al., 2018). Characterization of these catalysts is critical before it can be used for biodiesel production. Different methods have been used to achieve this so far. The most commonly used method for characterization of composition and crystallinity of these catalysts is X-ray diffraction (XRD). The morphology of prepared nanocatalysts and their precursors are determined using scanning electron microscopy (SEM). To analyze the particle diameter and morphology of the catalysts, transmission electron microscopy (TEM) is used. Other characterization methods include Fourier transform infrared (FTIR) spectroscopy that is used for determining assimilation of phases, Barrett–Joyner–Hlenda (BJH) and Brunauer–Emmett–Teller (BET) methods for specific surface area calculation, and thermogravimetric analysis (TGA) for examining the decomposition nature of catalyst samples, etc. (Nayebzadeh et al., 2017; Baskar et al., 2018). Some of the latest works on nanocatalysts for the transesterification reaction are summarized in Table 5. As seen from the table, nanocatalyst can achieve high FAME yield with very mild reaction conditions and short reaction times. The reusability of these catalysts is also excellent, as these retain good activity even after 11 cycles depending on the catalyst.
Perspectives, Challenges, and Further Work
Table 6 summarizes the advantages and disadvantages of different types of catalyst based on the above discussion.
Homogeneous catalyst has been exhaustively studied, and challenges have been addressed in the literature. On the other hand, heterogeneous catalysts is a relatively new research area on which significant research is ongoing at present. Several challenges have been reported in the literature for these catalysts:
1. Short catalyst life, lower reaction rate, and instability have been reported as the main problems for heterogeneous catalysts.
2. Solid base catalysts were reported to be sensitive to CO2, water, and FFA. These consume and deactivate the catalyst via saponification.
3. Solid acid catalysts were reported to induce leaching and product contamination due to the ionic group being hydrolyzed by water.
4. Lipase inhibition has been reported in the presence of methanol during enzymatic transesterification.
5. In the case of nanocatalysts, at relatively mild operating conditions, it is necessary to increase the reaction time to achieve high performances. However, it is essential to apply severe operating conditions to achieve ordinary reaction times, thereby increasing the energy requirement.
The following aspects need to be addressed in future works:
1. Further investigation into waste-derived catalysts are necessary to develop new catalysts with improved catalytic performance.
2. Development of highly active and selective heterogeneous catalysts that are economically feasible for use in the industrial scale.
3. Exploring new catalyst supports with selective surface area and interconnected system of appropriate pore sizes.
4. Exploring biomass or waste as the source of catalyst to reduce the associated cost and improve sustainability for commercially available solid catalysts.
5. Improving preparation routes and treatment steps for hydrotalcite-based catalysts to transform their application from laboratory to industrial scale.
6. Improving the sensitiveness to FFA and water and the morphology by keeping high basic strength of zeolite-based catalyst.
7. Further investigation into industrial enzymatic biodiesel production for an ensuring viable future option.
8. Energy-efficient and low-cost methods for effective recovery and reuse of nanocatalysts.
Conclusion
Laboratory-scale biodiesel production using heterogeneous catalysts have been reported at length in the literature. Among the catalysts, base homogeneous catalysts possess rapid reaction rate and high yield and require mild operating conditions. However, those are sensitive to FFA content of the oil that causes undesired by-products, namely, soaps and polymeric pigments, making the purification process difficult and impossible to reuse the catalysts. Homogeneous acid catalysts are suitable for those high FFA content oil. However, they suffer some drawbacks, including relatively slow reaction rate, corrosive nature, difficulty in catalyst separation from product, etc. Heterogeneous base catalysts overcome some of the disadvantages of homogeneous base catalysts, namely, ease of separation, simple catalyst recovery techniques, and reusability of catalyst from the product. Heterogeneous acid catalysts show very less sensitivity to high FFA and water content in the feedstock and can easily be recovered, recycled, and reused after the biodiesel production process. Due to current interest in “green” alternatives to chemical catalysts, biocatalysts, i.e., enzymes, have drawn attention. The catalysts work under relatively lower reaction temperature conditions compared to other catalysts and can catalyze low-grade oils with extremely high FFA content. The results obtained have proved that high productivity, involving yield and numbers of reuse, as well as low reaction time, can be achieved when using enzymes. The major limitation is the reaction rate, which is the slowest among all the catalysts. Furthermore, the synthesis of catalysts is more expensive than those of both homogeneous acid and base catalysts. High catalytic efficiency at mild operating conditions has drawn attention to nanocatalysts recently. The development of highly active and selective heterogeneous catalysts, along with their economic feasibility for use in the industrial scale, is a subject that needs to be addressed.
Author Contributions
IR formulated the article, written heterogeneous base, and other sections, compiled the whole article. HO contributed heterogeneous acid catalyst section. TM oversaw the work and provided review. MM contributed the homogeneous catalyst section. AS contributed the biocatalyst section. SR contributed the nanocatalyst section. AA reviewed and improved the article. All authors contributed to the article and approved the submitted version.
Funding
This research was funded by research development fund of School of Information, Systems and Modeling, University of Technology Sydney, Australia.
Conflict of Interest
The authors declare that the research was conducted in the absence of any commercial or financial relationships that could be construed as a potential conflict of interest.
References
Abdulla, R., and Ravindra, P. (2013). Immobilized Burkholderia cepacia lipase for biodiesel production from crude Jatropha curcas L. oil. Biomass Bioenergy 56, 8–13. doi: 10.1016/j.biombioe.2013.04.010
Abedin, M. J., Masjuki, H. H., Kalam, M. A., Sanjid, A., Rahman, S. M. A., and Rizwanul Fattah, I. M. (2014). Performance, emissions, and heat losses of palm and jatropha biodiesel blends in a diesel engine. Ind. Crops Prod. 59, 96–104. doi: 10.1016/j.indcrop.2014.05.001
Aboelazayem, O., El-Gendy, N. S., Abdel-Rehim, A. A., Ashour, F., and Sadek, M. A. (2018). Biodiesel production from castor oil in Egypt: process optimisation, kinetic study, diesel engine performance and exhaust emissions analysis. Energy 157, 843–852. doi: 10.1016/j.energy.2018.05.202
Akubude, V. C., Nwaigwe, K. N., and Dintwa, E. (2019). Production of biodiesel from microalgae via nanocatalyzed transesterification process: a review. Mater. Sci. Energy Tech. 2, 216–225. doi: 10.1016/j.mset.2018.12.006
Alaei, S., Haghighi, M., Toghiani, J., and Rahmani Vahid, B. (2018). Magnetic and reusable MgO/MgFe2O4 nanocatalyst for biodiesel production from sunflower oil: Influence of fuel ratio in combustion synthesis on catalytic properties and performance. Ind. Crops Prod. 117, 322–332. doi: 10.1016/j.indcrop.2018.03.015
Alcañiz-Monge, J., Bakkali, B. E., Trautwein, G., and Reinoso, S. (2018). Zirconia-supported tungstophosphoric heteropolyacid as heterogeneous acid catalyst for biodiesel production. Appl. Cataly. B Environ. 224, 194–203. doi: 10.1016/j.apcatb.2017.10.066
Ambat, I., Srivastava, V., and Sillanpää, M. (2018). Recent advancement in biodiesel production methodologies using various feedstock: a review. Renew. Sust. Energy Rev. 90, 356–369. doi: 10.1016/j.rser.2018.03.069
Amini, Z., Ilham, Z., Ong, H. C., Mazaheri, H., and Chen, W.-H. (2017a). State of the art and prospective of lipase-catalyzed transesterification reaction for biodiesel production. Energy Convers. Manage. 141, 339–353. doi: 10.1016/j.enconman.2016.09.049
Amini, Z., Ong, H. C., Harrison, M. D., Kusumo, F., Mazaheri, H., and Ilham, Z. (2017b). Biodiesel production by lipase-catalyzed transesterification of Ocimum basilicum L. (sweet basil) seed oil. Energy Convers. Manage. 132, 82–90. doi: 10.1016/j.enconman.2016.11.017
Andrijanto, E., Dawson, E. A., and Brown, D. R. (2012). Hypercrosslinked polystyrene sulphonic acid catalysts for the esterification of free fatty acids in biodiesel synthesis. Appl. Cataly. B Environ. 115–116, 261–268. doi: 10.1016/j.apcatb.2011.12.040
Aransiola, E. F., Ojumu, T. V., Oyekola, O. O., Madzimbamuto, T. F., and Ikhu-Omoregbe, D. I. O. (2014). A review of current technology for biodiesel production: State of the art. Biomass Bioenerg. 61, 276–297. doi: 10.1016/j.biombioe.2013.11.014
Arbab, M. I., Varman, M., Masjuki, H. H., Kalam, M. A., Imtenan, S., Sajjad, H., et al. (2015). Evaluation of combustion, performance, and emissions of optimum palm–coconut blend in turbocharged and non-turbocharged conditions of a diesel engine. Energ. Convers. Manage. 90, 111–120. doi: 10.1016/j.enconman.2014.11.017
Arumugam, A., and Ponnusami, V. (2014). Biodiesel production from Calophyllum inophyllum oil using lipase producing Rhizopus oryzae cells immobilized within reticulated foams. Renew. Energ. 64, 276–282. doi: 10.1016/j.renene.2013.11.016
Arun, S. B., Suresh, R., Yatish, K. V., Omkaresh, B. R., and Channa Keshava Naik, N. (2017). Use of CaO and Na3PO4 catalysts in the synthesis of biodiesel and investigation of fuel properties. Mater. Today Proc. 4, 11111–11117. doi: 10.1016/j.matpr.2017.08.074
Ashraful, A. M., Masjuki, H. H., Kalam, M. A., Rizwanul Fattah, I. M., Imtenan, S., Shahir, S. A., et al. (2014). Production and comparison of fuel properties, engine performance, and emission characteristics of biodiesel from various non-edible vegetable oils: a review. Energy Convers. Manage. 80, 202–228. doi: 10.1016/j.enconman.2014.01.037
Baskar, G., Aberna Ebenezer Selvakumari, I., and Aiswarya, R. (2018). Biodiesel production from castor oil using heterogeneous Ni doped ZnO nanocatalyst. Bioresour. Technol. 250, 793–798. doi: 10.1016/j.biortech.2017.12.010
Benjapornkulaphong, S., Ngamcharussrivichai, C., and Bunyakiat, K. (2009). Al2O3-supported alkali and alkali earth metal oxides for transesterification of palm kernel oil and coconut oil. Chem. Eng. J. 145, 468–474. doi: 10.1016/j.cej.2008.04.036
Borges, M. E., and Díaz, L. (2012). Recent developments on heterogeneous catalysts for biodiesel production by oil esterification and transesterification reactions: a review. Renew. Sust. Energ. Rev. 16, 2839–2849. doi: 10.1016/j.rser.2012.01.071
Calero, J., Luna, D., Sancho, E. D., Luna, C., Bautista, F. M., Romero, A. A., et al. (2014). Development of a new biodiesel that integrates glycerol, by using CaO as heterogeneous catalyst, in the partial methanolysis of sunflower oil. Fuel 122, 94–102. doi: 10.1016/j.fuel.2014.01.033
Chen, X.-R., Ju, Y.-H., and Mou, C.-Y. (2007). Direct synthesis of mesoporous sulfated silica-zirconia catalysts with high catalytic activity for biodiesel via esterification. J. Phys. Chem. C 111, 18731–18737. doi: 10.1021/jp0749221
Choi, N., No, D. S., Kim, H., Kim, B. H., Kwak, J., Lee, J.-S., et al. (2018). In situ lipase-catalyzed transesterification in rice bran for synthesis of fatty acid methyl ester. Ind. Crops Prod. 120, 140–146. doi: 10.1016/j.indcrop.2018.04.049
Chouhan, A. P. S., and Sarma, A. K. (2011). Modern heterogeneous catalysts for biodiesel production: A comprehensive review. Renew. Sust. Energ. Rev. 15, 4378–4399. doi: 10.1016/j.rser.2011.07.112
Christopher, L. P., Hemanathan, K., and Zambare, V. P. (2014). Enzymatic biodiesel: challenges and opportunities. Appl. Energy 119, 497–520. doi: 10.1016/j.apenergy.2014.01.017
Chua, S. Y., Periasamy, L. A. P., Goh, C. M. H., Tan, Y. H., Mubarak, N. M., et al. (2020). Biodiesel synthesis using natural solid catalyst derived from biomass waste — a review. J. Industr. Eng. Chem. 81, 41–60. doi: 10.1016/j.jiec.2019.09.022
Clohessy, J., and Kwapinski, W. (2020). Carbon-based catalysts for biodiesel production—a review. Appl. Sci. 10:918. doi: 10.3390/app10030918
Dai, Y.-M., Wang, Y.-F., and Chen, C.-C. (2018). Synthesis and characterization of magnetic LiFe5O8-LiFeO2 as a solid basic catalyst for biodiesel production. Catal. Commun. 106, 20–24. doi: 10.1016/j.catcom.2017.12.002
De Lima, A. L., Ronconi, C. M., and Mota, C. J. A. (2016). Heterogeneous basic catalysts for biodiesel production. Catal. Sci. Technol. 6, 2877–2891. doi: 10.1039/C5CY01989C
Di Serio, M., Tesser, R., Pengmei, L., and Santacesaria, E. (2008). Heterogeneous catalysts for biodiesel production. Energy Fuels 22, 207–217. doi: 10.1021/ef700250g
Doyle, A. M., Albayati, T. M., Abbas, A. S., and Alismaeel, Z. T. (2016). Biodiesel production by esterification of oleic acid over zeolite Y prepared from kaolin. Renew. Energy 97, 19–23. doi: 10.1016/j.renene.2016.05.067
Du, L., Ding, S., Li, Z., Lv, E., Lu, J., and Ding, J. (2018). Transesterification of castor oil to biodiesel using NaY zeolite-supported La2O3 catalysts. Energy Convers. Manag. 173, 728–734. doi: 10.1016/j.enconman.2018.07.053
Du, L., Li, Z., Ding, S., Chen, C., Qu, S., Yi, W., et al. (2019). Synthesis and characterization of carbon-based MgO catalysts for biodiesel production from castor oil. Fuel 258:116122. doi: 10.1016/j.fuel.2019.116122
Dubey, P., and Gupta, R. (2017). Effects of dual bio-fuel (Jatropha biodiesel and turpentine oil) on a single cylinder naturally aspirated diesel engine without EGR. Appl. Therm. Eng. 115, 1137–1147. doi: 10.1016/j.applthermaleng.2016.12.125
Dueso, C., Muñoz, M., Moreno, F., Arroyo, J., Gil-Lalaguna, N., Bautista, A., et al. (2018). Performance and emissions of a diesel engine using sunflower biodiesel with a renewable antioxidant additive from bio-oil. Fuel 234, 276–285. doi: 10.1016/j.fuel.2018.07.013
Endalew, A. K., Kiros, Y., and Zanzi, R. (2011). Inorganic heterogeneous catalysts for biodiesel production from vegetable oils. Biomass Bioenergy 35, 3787–3809. doi: 10.1016/j.biombioe.2011.06.011
Fattah, I. M. R., Kalam, M. A., Masjuki, H. H., and Wakil, M. A. (2014a). Biodiesel production, characterization, engine performance, and emission characteristics of Malaysian Alexandrian laurel oil. RSC Advanc. 4, 17787–17796. doi: 10.1039/C3RA47954D
Fattah, I. M. R., Masjuki, H. H., Kalam, M. A., Mofijur, M., and Abedin, M. J. (2014b). Effect of antioxidant on the performance and emission characteristics of a diesel engine fueled with palm biodiesel blends. Energy Convers. Manag. 79, 265–272. doi: 10.1016/j.enconman.2013.12.024
Fattah, I. M. R., Masjuki, H. H., Kalam, M. A., Wakil, M. A., Ashraful, A. M., and Shahir, S. A. (2014c). Experimental investigation of performance and regulated emissions of a diesel engine with Calophyllum inophyllum biodiesel blends accompanied by oxidation inhibitors. Energy Convers. Manag. 83, 232–240. doi: 10.1016/j.enconman.2014.03.069
Fattah, I. M. R., Masjuki, H. H., Kalam, M. A., Wakil, M. A., Rashedul, H. K., and Abedin, M. J. (2014d). Performance and emission characteristics of a CI engine fueled with Cocos nucifera and Jatropha curcas B20 blends accompanying antioxidants. Ind. Crops Prod. 57, 132–140. doi: 10.1016/j.indcrop.2014.03.022
Fattah, I. M. R., Masjuki, H. H., Liaquat, A. M., Ramli, R., Kalam, M. A., and Riazuddin, V. N. (2013). Impact of various biodiesel fuels obtained from edible and non-edible oils on engine exhaust gas and noise emissions. Renew. Sust. Energ. Rev. 18, 552–567. doi: 10.1016/j.rser.2012.10.036
Fattah, I. M. R., Ming, C., Chan, Q. N., Wehrfritz, A., Pham, P. X., Yang, W., et al. (2018). Spray and combustion investigation of post injections under low-temperature combustion conditions with biodiesel. Energy Fuels 32, 8727–8742. doi: 10.1021/acs.energyfuels.8b00284
Feng, Y., Zhang, A., Li, J., and He, B. (2011). A continuous process for biodiesel production in a fixed bed reactor packed with cation-exchange resin as heterogeneous catalyst. Bioresour. Technol. 102, 3607–3609. doi: 10.1016/j.biortech.2010.10.115
Feyzi, M., Hassankhani, A., and Rafiee, H. R. (2013). Preparation and characterization of Cs/Al/Fe3O4 nanocatalysts for biodiesel production. Energ. Convers. Manage. 71, 62–68. doi: 10.1016/j.enconman.2013.03.022
Fu, J., Chen, L., Lv, P., Yang, L., and Yuan, Z. (2015). Free fatty acids esterification for biodiesel production using self-synthesized macroporous cation exchange resin as solid acid catalyst. Fuel 154, 1–8. doi: 10.1016/j.fuel.2015.03.048
Gerpen, J. V. (2005). Biodiesel processing and production. Fuel Process. Technol. 86, 1097–1107. doi: 10.1016/j.fuproc.2004.11.005
Gog, A., Roman, M., Toşa, M., Paizs, C., and Irimie, F. D. (2012). Biodiesel production using enzymatic transesterification – Current state and perspectives. Renew. Energ. 39, 10–16. doi: 10.1016/j.renene.2011.08.007
Goga, G., Chauhan, B. S., Mahla, S. K., and Cho, H. M. (2019). Performance and emission characteristics of diesel engine fueled with rice bran biodiesel and n-butanol. Energy Rep. 5, 78–83. doi: 10.1016/j.egyr.2018.12.002
Guldhe, A., Singh, P., Ansari, F. A., Singh, B., and Bux, F. (2017). Biodiesel synthesis from microalgal lipids using tungstated zirconia as a heterogeneous acid catalyst and its comparison with homogeneous acid and enzyme catalysts. Fuel 187, 180–188. doi: 10.1016/j.fuel.2016.09.053
Hanif, M. A., Nisar, S., and Rashid, U. (2017). Supported solid and heteropoly acid catalysts for production of biodiesel. Catal. Rev. 59, 165–188. doi: 10.1080/01614940.2017.1321452
Helwani, Z., Othman, M. R., Aziz, N., Kim, J., and Fernando, W. J. N. (2009). Solid heterogeneous catalysts for transesterification of triglycerides with methanol: a review. Appl. Catal. A Gen. 363, 1–10. doi: 10.1016/j.apcata.2009.05.021
Hoekman, S. K., and Robbins, C. (2012). Review of the effects of biodiesel on NOx emissions. Fuel Process. Technol. 96, 237–249. doi: 10.1016/j.fuproc.2011.12.036
Hu, S., Wang, Y., and Han, H. (2011). Utilization of waste freshwater mussel shell as an economic catalyst for biodiesel production. Biomass Bioenergy 35, 3627–3635. doi: 10.1016/j.biombioe.2011.05.009
Ibrahim, M. M., Mahmoud, H. R., and El-Molla, S. A. (2019). Influence of support on physicochemical properties of ZrO2 based solid acid heterogeneous catalysts for biodiesel production. Catal. Commun. 122, 10–15. doi: 10.1016/j.catcom.2019.01.008
Islam, A., Taufiq-Yap, Y. H., Ravindra, P., Teo, S. H., Sivasangar, S., and Chan, E.-S. (2015). Biodiesel synthesis over millimetric γ-Al2O3/KI catalyst. Energy 89, 965–973. doi: 10.1016/j.energy.2015.06.036
Jahirul, M. I., Brown, R. J., Senadeera, W., Ashwath, N., Rasul, M. G., Rahman, M. M., et al. (2015). Physio-chemical assessment of beauty leaf (Calophyllum inophyllum) as second-generation biodiesel feedstock. Energy Rep. 1, 204–215. doi: 10.1016/j.egyr.2015.10.003
Jayaraman, J., Alagu, K., Appavu, P., Joy, N., Jayaram, P., and Mariadoss, A. (2020). Enzymatic production of biodiesel using lipase catalyst and testing of an unmodified compression ignition engine using its blends with diesel. Renew. Energy 145, 399–407. doi: 10.1016/j.renene.2019.06.061
Jayed, M. H., Masjuki, H. H., Saidur, R., Kalam, M. A., and Jahirul, M. I. (2009). Environmental aspects and challenges of oilseed produced biodiesel in Southeast Asia. Renew. Sust. Energ. Rev. 13, 2452–2462. doi: 10.1016/j.rser.2009.06.023
Kamran, E., Mashhadi, H., Mohammadi, A., and Ghobadian, B. (2020). Biodiesel production from Elaeagnus angustifolia.L seed as a novel waste feedstock using potassium hydroxide catalyst. Biocatal. Agric. Biotechnol. 25:101578. doi: 10.1016/j.bcab.2020.101578
Kaur, M., Malhotra, R., and Ali, A. (2018). Tungsten supported Ti/SiO2 nanoflowers as reusable heterogeneous catalyst for biodiesel production. Renew. Energy 116, 109–119. doi: 10.1016/j.renene.2017.09.065
Kaur, N., and Ali, A. (2015). Preparation and application of Ce/ZrO2-TiO2/SO42- as solid catalyst for the esterification of fatty acids. Renew. Energy 81, 421–431. doi: 10.1016/j.renene.2015.03.051
Kazemifard, S., Nayebzadeh, H., Saghatoleslami, N., and Safakish, E. (2018). Assessment the activity of magnetic KOH/Fe3O4@Al2O3 core–shell nanocatalyst in transesterification reaction: effect of Fe/Al ratio on structural and performance. Environ. Sci. Pollut. Res. 25, 32811–32821. doi: 10.1007/s11356-018-3249-7
Kesserwan, F., Ahmad, M. N., Khalil, M., and El-Rassy, H. (2020). Hybrid CaO/Al2O3 aerogel as heterogeneous catalyst for biodiesel production. Chem. Eng. J. 385:123834. doi: 10.1016/j.cej.2019.123834
Kim, M., Dimaggio, C., Salley, S. O., and Simon Ng, K. Y. (2012). A new generation of zirconia supported metal oxide catalysts for converting low grade renewable feedstocks to biodiesel. Bioresour. Technol. 118, 37–42. doi: 10.1016/j.biortech.2012.04.035
Knothe, G., and Razon, L. F. (2017). Biodiesel fuels. Prog. Energ. Combust. 58, 36–59. doi: 10.1016/j.pecs.2016.08.001
Kurhade, A., and Dalai, A. K. (2018). Physiochemical characterization and support interaction of alumina-supported heteropolyacid catalyst for biodiesel production. Asia-Pac. J. Chem. Eng. 13:e2249. doi: 10.1002/apj.2249
Lam, M. K., Lee, K. T., and Mohamed, A. R. (2010). Homogeneous, heterogeneous and enzymatic catalysis for transesterification of high free fatty acid oil (waste cooking oil) to biodiesel: a review. Biotechnol. Advanc. 28, 500–518. doi: 10.1016/j.biotechadv.2010.03.002
Latchubugata, C. S., Kondapaneni, R. V., Patluri, K. K., Virendra, U., and Vedantam, S. (2018). Kinetics and optimization studies using response surface methodology in biodiesel production using heterogeneous catalyst. Chem. Eng. Res. Des. 135, 129–139. doi: 10.1016/j.cherd.2018.05.022
Lee, A. F., Bennett, J. A., Manayil, J. C., and Wilson, K. (2014). Heterogeneous catalysis for sustainable biodiesel production via esterification and transesterification. Chem. Soc. Rev. 43, 7887–7916. doi: 10.1039/C4CS00189C
Lee, H. V., Juan, J. C., and Taufiq-Yap, Y. H. (2015). Preparation and application of binary acid–base CaO–La2O3 catalyst for biodiesel production. Renew. Energy 74, 124–132. doi: 10.1016/j.renene.2014.07.017
Li, X., He, X.-Y., Li, Z.-L., Wang, Y.-D., Wang, C.-Y., Shi, H., et al. (2012). Enzymatic production of biodiesel from Pistacia chinensis bge seed oil using immobilized lipase. Fuel 92, 89–93. doi: 10.1016/j.fuel.2011.06.048
Li, Z., Ding, S., Chen, C., Qu, S., Du, L., Lu, J., et al. (2019). Recyclable Li/NaY zeolite as a heterogeneous alkaline catalyst for biodiesel production: Process optimization and kinetics study. Energy Convers. Manag. 192, 335–345. doi: 10.1016/j.enconman.2019.04.053
Limmanee, S., Naree, T., Bunyakiat, K., and Ngamcharussrivichai, C. (2013). Mixed oxides of Ca, Mg and Zn as heterogeneous base catalysts for the synthesis of palm kernel oil methyl esters. Chem. Eng. J. 225, 616–624. doi: 10.1016/j.cej.2013.03.093
Ling, J. S. J., Tan, Y. H., Mubarak, N. M., Kansedo, J., Saptoro, A., and Nolasco-Hipolito, C. (2019). A review of heterogeneous calcium oxide based catalyst from waste for biodiesel synthesis. SN Appl. Sci. 1:810. doi: 10.1007/s42452-019-0843-3
Liu, R., Wang, X., Zhao, X., and Feng, P. (2008a). Sulfonated ordered mesoporous carbon for catalytic preparation of biodiesel. Carbon 46, 1664–1669. doi: 10.1016/j.carbon.2008.07.016
Liu, X., He, H., Wang, Y., and Zhu, S. (2007). Transesterification of soybean oil to biodiesel using SrO as a solid base catalyst. Catal. Commun. 8, 1107–1111. doi: 10.1016/j.catcom.2006.10.026
Liu, X., He, H., Wang, Y., Zhu, S., and Piao, X. (2008b). Transesterification of soybean oil to biodiesel using CaO as a solid base catalyst. Fuel 87, 216–221. doi: 10.1016/j.fuel.2007.04.013
Lopresto, C. G., Naccarato, S., Albo, L., De Paola, M. G., Chakraborty, S., Curcio, S., et al. (2015). Enzymatic transesterification of waste vegetable oil to produce biodiesel. Ecotoxicol. Environ. Safe. 121, 229–235. doi: 10.1016/j.ecoenv.2015.03.028
Madhuvilakku, R., and Piraman, S. (2013). Biodiesel synthesis by TiO2-ZnO mixed oxide nanocatalyst catalyzed palm oil transesterification process. Bioresour. Technol. 150, 55–59. doi: 10.1016/j.biortech.2013.09.087
Mahlia, T. M. I., Syazmi, Z. A. H. S., Mofijur, M., Abas, A. E. P., Bilad, M. R., et al. (2020). Patent landscape review on biodiesel production: technology updates. Renew. Sust. Energ. Rev. 118:109526. doi: 10.1016/j.rser.2019.109526
Majhi, S., and Ray, S. (2016). A study on production of biodiesel using a novel solid oxide catalyst derived from waste. Environ. Sci. Pollut. Res. 23, 9251–9259. doi: 10.1007/s11356-015-4824-9
Maleki, E., Aroua, M. K., and Sulaiman, N. M. N. (2013). Castor oil — a more suitable feedstock for enzymatic production of methyl esters. Fuel Process. Technol. 112, 129–132. doi: 10.1016/j.fuproc.2013.03.003
Manríquez-Ramírez, M., Gómez, R., Hernández-Cortez, J. G., Zúñiga-Moreno, A., Reza-San Germán, C. M., et al. (2013). Advances in the transesterification of triglycerides to biodiesel using MgO–NaOH, MgO–KOH and MgO–CeO2 as solid basic catalysts. Catal. Today 212, 23–30. doi: 10.1016/j.cattod.2012.11.005
Mansir, N., Taufiq-Yap, Y. H., Rashid, U., and Lokman, I. M. (2017). Investigation of heterogeneous solid acid catalyst performance on low grade feedstocks for biodiesel production: a review. Energy Convers. Manag. 141, 171–182. doi: 10.1016/j.enconman.2016.07.037
Mardhiah, H. H., Ong, H. C., Masjuki, H. H., Lim, S., and Lee, H. V. (2017). A review on latest developments and future prospects of heterogeneous catalyst in biodiesel production from non-edible oils. Renew. Sust. Energ. Rev. 67, 1225–1236. doi: 10.1016/j.rser.2016.09.036
Marín-Suárez, M., Méndez-Mateos, D., Guadix, A., and Guadix, E. M. (2019). Reuse of immobilized lipases in the transesterification of waste fish oil for the production of biodiesel. Renew. Energ. 140, 1–8. doi: 10.1016/j.renene.2019.03.035
Marwaha, A., Rosha, P., Mohapatra, S. K., Mahla, S. K., and Dhir, A. (2018). Waste materials as potential catalysts for biodiesel production: current state and future scope. Fuel Process. Technol. 181, 175–186. doi: 10.1016/j.fuproc.2018.09.011
Mata, T. M., Sousa, I. R. B. G., Vieira, S. S., and Caetano, N. S. (2012). Biodiesel production from corn oil via enzymatic catalysis with ethanol. Energy Fuels 26, 3034−3041. doi: 10.1021/ef300319f
Mckenzie, A. L., Fishel, C. T., and Davis, R. J. (1992). Investigation of the surface structure and basic properties of calcined hydrotalcites. J. Catal. 138, 547–561. doi: 10.1016/0021-9517(92)90306-3
Melero, J. A., Iglesias, J., and Morales, G. (2009). Heterogeneous acid catalysts for biodiesel production: current status and future challenges. Green Chem. 11, 1285–1308. doi: 10.1039/b902086a
Mofijur, M., Masjuki, H. H., Kalam, M. A., Atabani, A. E., Rizwanul Fattah, I. M., and Mobarak, H. M. (2014). Comparative evaluation of performance and emission characteristics of Moringa oleifera and Palm oil based biodiesel in a diesel engine. Ind. Crops Prod. 53, 78–84. doi: 10.1016/j.indcrop.2013.12.011
Mohebbi, S., Rostamizadeh, M., and Kahforoushan, D. (2020). Effect of molybdenum promoter on performance of high silica MoO3/B-ZSM-5 nanocatalyst in biodiesel production. Fuel 266:117063. doi: 10.1016/j.fuel.2020.117063
Mootabadi, H., Salamatinia, B., Bhatia, S., and Abdullah, A. Z. (2010). Ultrasonic-assisted biodiesel production process from palm oil using alkaline earth metal oxides as the heterogeneous catalysts. Fuel 89, 1818–1825. doi: 10.1016/j.fuel.2009.12.023
Moradi, G., Mohadesi, M., Rezaei, R., and Moradi, R. (2015). Biodiesel production using CaO/γ-Al2O3 catalyst synthesized by sol-gel method. Canad. J. Chem. Eng. 93, 1531–1538. doi: 10.1002/cjce.22258
Navajas, A., Campo, I., Moral, A., Echave, J., Sanz, O., Montes, M., et al. (2018). Outstanding performance of rehydrated Mg-Al hydrotalcites as heterogeneous methanolysis catalysts for the synthesis of biodiesel. Fuel 211, 173–181. doi: 10.1016/j.fuel.2017.09.061
Nayebzadeh, H., Saghatoleslami, N., Haghighi, M., and Tabasizadeh, M. (2017). Influence of fuel type on microwave-enhanced fabrication of KOH/Ca12Al14O33 nanocatalyst for biodiesel production via microwave heating. J. Taiwan Inst. Chem. Eng. 75, 148–155. doi: 10.1016/j.jtice.2017.03.018
Nowicki, J., Lach, J., Organek, M., and Sabura, E. (2016). Transesterification of rapeseed oil to biodiesel over Zr-dopped MgAl hydrotalcites. Appl. Catal. A Genrl. 524, 17–24. doi: 10.1016/j.apcata.2016.05.015
Ong, H. C., Masjuki, H. H., Mahlia, T. M. I., Silitonga, A. S., Chong, W. T., and Yusaf, T. (2014). Engine performance and emissions using Jatropha curcas, Ceiba pentandra and Calophyllum inophyllum biodiesel in a CI diesel engine. Energy 69, 427–445. doi: 10.1016/j.energy.2014.03.035
Ong, H. C., Milano, J., Silitonga, A. S., Hassan, M. H., Shamsuddin, A. H., Wang, C.-T., et al. (2019). Biodiesel production from Calophyllum inophyllum-Ceiba pentandra oil mixture: Optimization and characterization. J. Clean. Prod. 219, 183–198. doi: 10.1016/j.jclepro.2019.02.048
Palash, S. M., Masjuki, H. H., Kalam, M. A., Atabani, A. E., Rizwanul Fattah, I. M., and Sanjid, A. (2015). Biodiesel production, characterization, diesel engine performance, and emission characteristics of methyl esters from Aphanamixis polystachya oil of Bangladesh. Energy Convers. Manag. 91, 149–157. doi: 10.1016/j.enconman.2014.12.009
Pandit, P. R., and Fulekar, M. H. (2017). Egg shell waste as heterogeneous nanocatalyst for biodiesel production: optimized by response surface methodology. J. Environ. Manage. 198, 319–329. doi: 10.1016/j.jenvman.2017.04.100
Qiu, F., Li, Y., Yang, D., Li, X., and Sun, P. (2011). Heterogeneous solid base nanocatalyst: Preparation, characterization and application in biodiesel production. Bioresour. Technol. 102, 4150–4156. doi: 10.1016/j.biortech.2010.12.071
Quirino, M. R., Oliveira, M. J. C., Keyson, D., Lucena, G. L., Oliveira, J. B. L., and Gama, L. (2016). Synthesis of zinc aluminate with high surface area by microwave hydrothermal method applied in the transesterification of soybean oil (biodiesel). Mater. Res. Bull. 74, 124–128. doi: 10.1016/j.materresbull.2015.10.027
Rahmani Vahid, B., Haghighi, M., Alaei, S., and Toghiani, J. (2017). Reusability enhancement of combustion synthesized MgO/MgAl2O4 nanocatalyst in biodiesel production by glow discharge plasma treatment. Energy Convers. Manag. 143, 23–32. doi: 10.1016/j.enconman.2017.03.075
Ropero-Vega, J. L., Aldana-Pérez, A., Gómez, R., and Niño-Gómez, M. E. (2010). Sulfated titania [TiO2/SO42-]: a very active solid acid catalyst for the esterification of free fatty acids with ethanol. Appl. Catal. A-Gen. 379, 24–29. doi: 10.1016/j.apcata.2010.02.020
Roschat, W., Phewphong, S., Khunchalee, J., and Moonsin, P. (2018). Biodiesel production by ethanolysis of palm oil using SrO as a basic heterogeneous catalyst. Mater. Today Proc. 5, 13916–13921. doi: 10.1016/j.matpr.2018.02.040
Roschat, W., Siritanon, T., Yoosuk, B., and Promarak, V. (2016). Biodiesel production from palm oil using hydrated lime-derived CaO as a low-cost basic heterogeneous catalyst. Energy Convers. Manag. 108, 459–467. doi: 10.1016/j.enconman.2015.11.036
Ruhul, A. M., Kalam, M. A., Masjuki, H. H., Fattah, I. M. R., Reham, S. S., and Rashed, M. M. (2015). State of the art of biodiesel production processes: a review of the heterogeneous catalyst. RSC Advanc. 5, 101023–101044. doi: 10.1039/C5RA09862A
Ruhul, A. M., Kalam, M. A., Masjuki, H. H., Shahir, S. A., Alabdulkarem, A., Teoh, Y. H., et al. (2017). Evaluating combustion, performance and emission characteristics of Millettia pinnata and Croton megalocarpus biodiesel blends in a diesel engine. Energy 141, 2362–2376. doi: 10.1016/j.energy.2017.11.096
Salinas, D., Sepúlveda, C., Escalona, N., Gfierro, J. L., and Pecchi, G. (2018). Sol–gel La2O3-ZrO2 mixed oxide catalysts for biodiesel production. J. Energ. Chem. 27, 565–572. doi: 10.1016/j.jechem.2017.11.003
Sani, Y. M., Daud, W. M. A. W., and Abdul Aziz, A. R. (2014). Activity of solid acid catalysts for biodiesel production: a critical review. Appl. Catal. A Gen. 470, 140–161. doi: 10.1016/j.apcata.2013.10.052
Santiago-Torres, N., Romero-Ibarra, I. C., and Pfeiffer, H. (2014). Sodium zirconate (Na2ZrO3) as a catalyst in a soybean oil transesterification reaction for biodiesel production. Fuel Process. Technol. 120, 34–39. doi: 10.1016/j.fuproc.2013.11.018
Saravanan, A., Murugan, M., Sreenivasa Reddy, M., and Parida, S. (2020). Performance and emission characteristics of variable compression ratio CI engine fueled with dual biodiesel blends of Rapeseed and Mahua. Fuel 263:116751. doi: 10.1016/j.fuel.2019.116751
Schuchardt, U., Sercheli, R., and Vargas, R. M. (1998). Transesterification of vegetable oils: a review. J. Braz. Chem. Soc. 9, 199–210. doi: 10.1590/S0103-50531998000300002
Seffati, K., Honarvar, B., Esmaeili, H., and Esfandiari, N. (2019). Enhanced biodiesel production from chicken fat using CaO/CuFe2O4 nanocatalyst and its combination with diesel to improve fuel properties. Fuel 235, 1238–1244. doi: 10.1016/j.fuel.2018.08.118
Sekoai, P. T., Ouma, C. N. M., Du Preez, S. P., Modisha, P., Engelbrecht, N., Bessarabov, D. G., et al. (2019). Application of nanoparticles in biofuels: an overview. Fuel 237, 380–397. doi: 10.1016/j.fuel.2018.10.030
Semwal, S., Arora, A. K., Badoni, R. P., and Tuli, D. K. (2011). Biodiesel production using heterogeneous catalysts. Bioresour. Technol. 102, 2151–2161. doi: 10.1016/j.biortech.2010.10.080
Shah, S., and Gupta, M. N. (2007). Lipase catalyzed preparation of biodiesel from Jatropha oil in a solvent free system. Process Biochemi. 42, 409–414. doi: 10.1016/j.procbio.2006.09.024
Shan, R., Lu, L., Shi, Y., Yuan, H., and Shi, J. (2018). Catalysts from renewable resources for biodiesel production. Energ. Convers. Manage. 178, 277–289. doi: 10.1016/j.enconman.2018.10.032
Shi, G., Yu, F., Wang, Y., Pan, D., Wang, H., and Li, R. (2016). A novel one-pot synthesis of tetragonal sulfated zirconia catalyst with high activity for biodiesel production from the transesterification of soybean oil. Renew. Energy 92, 22–29. doi: 10.1016/j.renene.2016.01.094
Siddiquee, M. N., Kazemian, H., and Rohani, S. (2011). Biodiesel production from the lipid of wastewater sludge using an acidic heterogeneous catalyst. Chem. Eng. Technol. 34, 1983–1988. doi: 10.1002/ceat.201100119
Silitonga, A. S., Masjuki, H. H., Mahlia, T. M. I., Ong, H. C., Chong, W. T., and Boosroh, M. H. (2013a). Overview properties of biodiesel diesel blends from edible and non-edible feedstock. Renew. Sust. Energ. Rev. 22, 346–360. doi: 10.1016/j.rser.2013.01.055
Silitonga, A. S., Ong, H. C., Mahlia, T. M. I., Masjuki, H. H., and Chong, W. T. (2013b). Characterization and production of Ceiba pentandra biodiesel and its blends. Fuel 108, 855–858. doi: 10.1016/j.fuel.2013.02.014
Silitonga, A. S., Shamsuddin, A. H., Mahlia, T. M. I., Milano, J., Kusumo, F., Siswantoro, J., et al. (2020). Biodiesel synthesis from Ceiba pentandra oil by microwave irradiation-assisted transesterification: ELM modeling and optimization. Renew. Energy 146, 1278–1291. doi: 10.1016/j.renene.2019.07.065
Sirisomboonchai, S., Abuduwayiti, M., Guan, G., Samart, C., Abliz, S., Hao, X., et al. (2015). Biodiesel production from waste cooking oil using calcined scallop shell as catalyst. Energy Convers. Manage. 95, 242–247. doi: 10.1016/j.enconman.2015.02.044
Sulaiman, N. F., Wan Abu Bakar, W. A., and Ali, R. (2017). Response surface methodology for the optimum production of biodiesel over Cr/Ca/γ-Al2O3 catalyst: Catalytic performance and physicochemical studies. Renew. Energy 113, 697–705. doi: 10.1016/j.renene.2017.06.007
Sun, H., Ding, Y., Duan, J., Zhang, Q., Wang, Z., Lou, H., et al. (2010). Transesterification of sunflower oil to biodiesel on ZrO2 supported La2O3 catalyst. Bioresour. Technol. 101, 953–958. doi: 10.1016/j.biortech.2009.08.089
Szczesna Antczak, M., Kubiak, A., Antczak, T., and Bielecki, S. (2009). Enzymatic biodiesel synthesis – Key factors affecting efficiency of the process. Renew. Energ. 34, 1185–1194. doi: 10.1016/j.renene.2008.11.013
Tan, Y. H., Abdullah, M. O., Kansedo, J., Mubarak, N. M., Chan, Y. S., and Nolasco-Hipolito, C. (2019). Biodiesel production from used cooking oil using green solid catalyst derived from calcined fusion waste chicken and fish bones. Renew. Energ. 139, 696–706. doi: 10.1016/j.renene.2019.02.110
Teo, S. H., Rashid, U., Thomas Choong, S. Y., and Taufiq-Yap, Y. H. (2017). Heterogeneous calcium-based bimetallic oxide catalyzed transesterification of Elaeis guineensis derived triglycerides for biodiesel production. Energy Convers. Manage. 141, 20–27. doi: 10.1016/j.enconman.2016.03.042
Tichit, D., Lhouty, M. H., Guida, A., Chiche, B. H., Figueras, F., Auroux, A., et al. (1995). Textural properties and catalytic activity of hydrotalcites. J. Catal. 151, 50–59. doi: 10.1006/jcat.1995.1007
Trakarnpruk, W., and Porntangjitlikit, S. (2008). Palm oil biodiesel synthesized with potassium loaded calcined hydrotalcite and effect of biodiesel blend on elastomer properties. Renew. Energ. 33, 1558–1563. doi: 10.1016/j.renene.2007.08.003
Vaccari, A. (1999). Clays and catalysis: a promising future. Appl. Clay Sci. 14, 161–198. doi: 10.1016/S0169-1317(98)00058-1
Wakil, M. A., Kalam, M. A., Masjuki, H. H., and Rizwanul Fattah, I. M. (2016). Rice bran: a prospective resource for biodiesel production in Bangladesh. Int. J. Green Energy 13, 497–504. doi: 10.1080/15435075.2014.966374
Wan Omar, W. N. N., and Amin, N. A. S. (2011). Biodiesel production from waste cooking oil over alkaline modified zirconia catalyst. Fuel Process. Technol. 92, 2397–2405. doi: 10.1016/j.fuproc.2011.08.009
Yaşar, F. (2019). Biodiesel production via waste eggshell as a low-cost heterogeneous catalyst: Its effects on some critical fuel properties and comparison with CaO. Fuel 255:115828. doi: 10.1016/j.fuel.2019.115828
Yoo, S. J., Lee, H.-S., Veriansyah, B., Kim, J., Kim, J.-D., and Lee, Y.-W. (2010). Synthesis of biodiesel from rapeseed oil using supercritical methanol with metal oxide catalysts. Bioresour. Technol. 101, 8686–8689. doi: 10.1016/j.biortech.2010.06.073
Zabeti, M., Wan Daud, W. M. A., and Aroua, M. K. (2009). Activity of solid catalysts for biodiesel production: a review. Fuel Process. Technol. 90, 770–777. doi: 10.1016/j.fuproc.2009.03.010
Zeng, H.-Y., Liao, K.-B., Deng, X., Jiang, H., and Zhang, F. (2009). Characterization of the lipase immobilized on Mg–Al hydrotalcite for biodiesel. Process Biochem. 44, 791–798. doi: 10.1016/j.procbio.2009.04.005
Zhang, G., Hattori, H., and Tanabe, K. (1988). Aldol addition of acetone, catalyzed by solid base catalysts: magnesium oxide, calcium oxide, strontium oxide, barium oxide, lanthanum (III) oxide and zirconium oxide. Appl. Catal. 36, 189–197. doi: 10.1016/S0166-9834(00)80114-1
Keywords: biodiesel, transesterification, homogeneous catalyst, heterogeneous catalyst, biocatalyst, nanocatalyst
Citation: Rizwanul Fattah IM, Ong HC, Mahlia TMI, Mofijur M, Silitonga AS, Rahman SMA and Ahmad A (2020) State of the Art of Catalysts for Biodiesel Production. Front. Energy Res. 8:101. doi: 10.3389/fenrg.2020.00101
Received: 27 March 2020; Accepted: 07 May 2020;
Published: 19 June 2020.
Edited by:
Abdul-Sattar Nizami, Government College University, PakistanReviewed by:
Muhammad Mostafa Kamal Bhuiya, Chittagong University of Engineering & Technology, BangladeshMd. Asraful Alam, Zhengzhou University, China
Md. Jahirul Islam, Central Queensland University, Australia
Copyright © 2020 Rizwanul Fattah, Ong, Mahlia, Mofijur, Silitonga, Rahman and Ahmad. This is an open-access article distributed under the terms of the Creative Commons Attribution License (CC BY). The use, distribution or reproduction in other forums is permitted, provided the original author(s) and the copyright owner(s) are credited and that the original publication in this journal is cited, in accordance with accepted academic practice. No use, distribution or reproduction is permitted which does not comply with these terms.
*Correspondence: I. M. Rizwanul Fattah, islammdrizwanul.fattah@uts.edu.au; rizwanul.buet@gmail.com